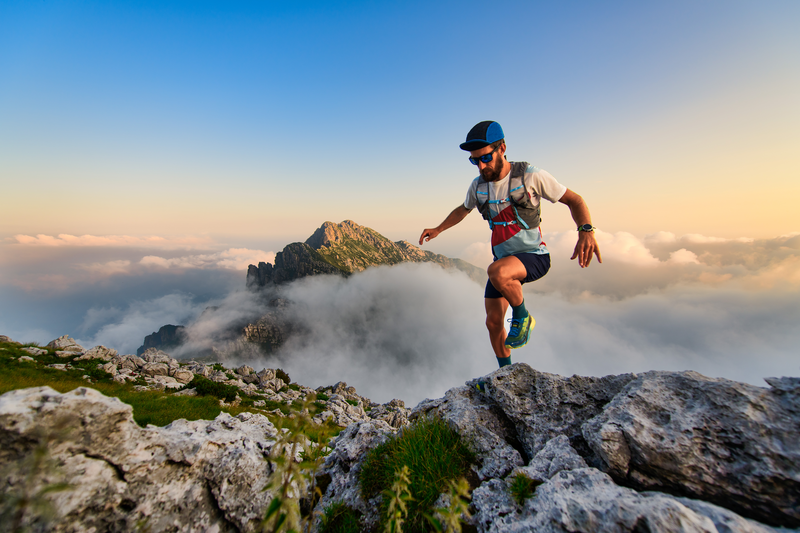
94% of researchers rate our articles as excellent or good
Learn more about the work of our research integrity team to safeguard the quality of each article we publish.
Find out more
ORIGINAL RESEARCH article
Front. Plant Sci.
Sec. Plant Symbiotic Interactions
Volume 16 - 2025 | doi: 10.3389/fpls.2025.1539336
This article is part of the Research Topic Cross-Kingdom Communications Among Plants, Fungi and Bacteria: From Molecules to Ecological Factors View all 10 articles
The final, formatted version of the article will be published soon.
You have multiple emails registered with Frontiers:
Please enter your email address:
If you already have an account, please login
You don't have a Frontiers account ? You can register here
Tetraena mongolica was established in the West Ordos Region of northwest China approximately 140 million years ago. It plays an irreplaceable role in maintaining local ecosystemstability. This study aimed to evaluate the effects of planting T. mongolica on soil nutrition and microbial communities by comparing the root zone soil (Rz_soil) and bare soil (B_soil) across three different plant communitie. The results showed that T. mongolica decreased soil pH and Na + while increasing available potassium, soil organic matter, organic carbon, total nitrogen, and potassium.T. mongolica significantly improved the diversity indices (Sobs and Ace), as well as the richness index (Chao), of bacterial and fungal communities across three plant communities. Meanwhile, the relative abundances of Rubrobacter and norank_c_Actinobacteria in the bacterial communities declined significantly in the Rz_soil compared with the B_soil across all three plant communities.In contrast, the relative abundances of Fusarium and Penicillium were higher, whereas those of Monosporascus and Darksidea were lower in Rz_soil than in B_soil in the two plant communities.T. mongolica decreased the soil bacterial co-occurrence networks while increasing the soil fungal co-occurrence networks. In addition, T. mongolica enhanced soil functions related to energy gain through denitrification, methylotrophy, hydrogen oxidation, photoconversion, and matter 2 acquisition through organic matter decomposition, aromatic compound degradation, and photoconversion. However, T. mongolica had no significant effect on the functional aspects of the fungal communities. These results provide a new perspective to understand the role of T. Mongolica in the desert ecosystems.
Keywords: Tetraena mongolica, Soil nutrition, Bacterial communities, Fungi communities, Root zone soil, bare soil
Received: 04 Dec 2024; Accepted: 25 Feb 2025.
Copyright: © 2025 Quan, Gan, Lu, Shi, Bai, Lin, Gou, Zhang, Zhang, Wei, Chang, Li and Liu. This is an open-access article distributed under the terms of the Creative Commons Attribution License (CC BY). The use, distribution or reproduction in other forums is permitted, provided the original author(s) or licensor are credited and that the original publication in this journal is cited, in accordance with accepted academic practice. No use, distribution or reproduction is permitted which does not comply with these terms.
* Correspondence:
Jianli Liu, College of Biological Science and Engineering, North Minzu University, Yinchuan, China
Disclaimer: All claims expressed in this article are solely those of the authors and do not necessarily represent those of their affiliated organizations, or those of the publisher, the editors and the reviewers. Any product that may be evaluated in this article or claim that may be made by its manufacturer is not guaranteed or endorsed by the publisher.
Research integrity at Frontiers
Learn more about the work of our research integrity team to safeguard the quality of each article we publish.