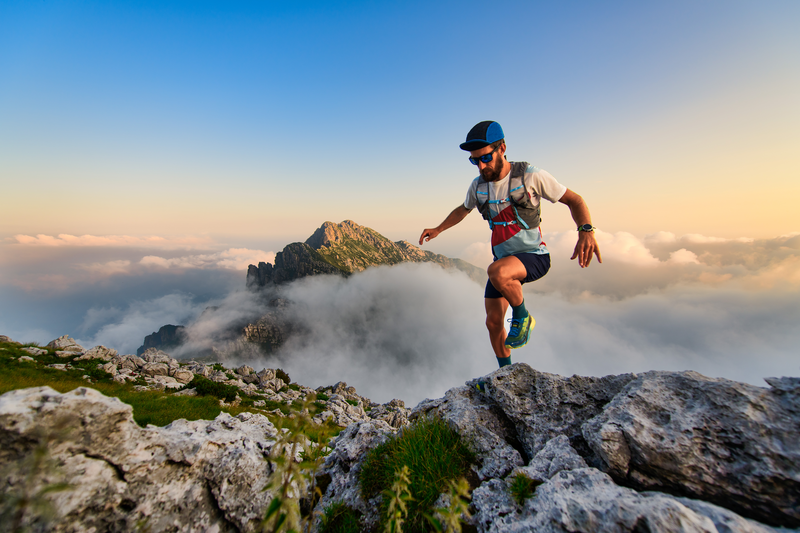
95% of researchers rate our articles as excellent or good
Learn more about the work of our research integrity team to safeguard the quality of each article we publish.
Find out more
REVIEW article
Front. Plant Sci. , 21 February 2025
Sec. Plant Metabolism and Chemodiversity
Volume 16 - 2025 | https://doi.org/10.3389/fpls.2025.1530814
This article is part of the Research Topic Bioactive Compounds, Functional Ingredients, Antioxidants, and Health Benefits of Edible Plants Volume II View all 9 articles
Amorphophallus konjac is a perennial plant native to Southeast Asia, renowned for its edible corms and rich nutritional value. The bioactive component, konjac glucomannan (KGM), has garnered significant attention due to its broad applications. This review aims to provide a comprehensive overview of the traditional uses, chemical and physical properties, and modern health applications of KGM. It highlights cutting-edge research, discusses challenges and limitations, and identifies future directions for advancing the utility of KGM in health and nutrition. KGM demonstrates remarkable health benefits, including improving metabolic health through weight management, blood glucose stabilization, and lipid profile enhancement. It also plays a vital role in gut health. Emerging evidence highlights its anti-inflammatory and immune-regulatory effects, with applications in managing inflammatory bowel disease, hyperthyroidism, and colorectal cancer (CRC). Recent advancements in multi-omics analyses and high-throughput screening (HTS) approaches have improved KGM extraction, characterization, and evaluation. However, potential side effects such as gastrointestinal discomfort and allergenicity, along with challenges in maintaining purity and molecular consistency, require careful consideration. KGM is a versatile dietary fiber with extensive applications in functional foods, nutraceuticals, and therapeutic interventions. Future research should focus on enhancing KGM's bioavailability, developing targeted delivery systems, and formulating novel applications.
Amorphophallus konjac, commonly known for its edible corm and the production of KGM, has a rich cultural and medical history, particularly in East Asia (Pedrosa L de and Fabi, 2024). Traditionally, KGM has been utilized in Chinese medicine for over 2000 years, serving various health purposes such as detoxification, tumor suppression, and treatment of respiratory and skin disorders (Islam et al., 2023). This historical context underscores the significance of konjac in both dietary and medicinal practices, highlighting its role as a staple food source in countries like China and Japan (Ye et al., 2021). The chemical and physical properties of KGM contribute to its health benefits. KGM is a soluble dietary fiber that exhibits unique gel-forming capabilities, which are essential for its applications in food and health products (Sun et al., 2023). Modern research has revealed that KGM can significantly lower plasma cholesterol levels, improve carbohydrate metabolism, and enhance bowel movement, thereby promoting gut health (Du et al., 2021). As a prebiotic, KGM supports the regulation of gut microbiota, which is crucial for maintaining digestive health and overall metabolic function (Xia et al., 2024).
In terms of metabolic health, KGM has shown promise in weight management and blood sugar regulation, making it a valuable component in diabetes management strategies (Fang et al., 2023a). Additionally, its anti-inflammatory properties have been studied for their potential to manage chronic diseases and improve immune function, further expanding its therapeutic applications (Liu et al., 2025). Emerging research is increasingly focused on the diverse biological activities of KGM, exploring its potential in treating lifestyle-related diseases such as obesity and diabetes (Dai et al., 2019). Advanced methodologies, including multi-omics analysis and HTS, are being employed to enhance the extraction, purification, and functional evaluation of KGM, paving the way for innovative health applications (Liu et al., 2023). Emerging research has begun to uncover the broader potential of konjac in addressing metabolic disorders, particularly in combating rising global rates of obesity and type 2 diabetes (Au-Yeung et al., 2018). Its ability to low-density lipoprotein cholesterol (LDL-C) levels and improve blood lipid profiles has positioned it as a promising natural intervention for cardiovascular health. Moreover, the prebiotic properties of glucomannan foster a healthy gut microbiome, offering therapeutic possibilities for managing conditions such as irritable bowel syndrome (IBS) and other digestive disorders (Lasrado and Rai, 2022). This growing body of evidence underscores the need for further research on the mechanisms of action of konjac and its full spectrum of health benefits (Wilianto et al., 2024). As the world increasingly turns to plant-based and natural solutions for health management, A. konjac stands out as a traditional remedy with vast modern relevance, offering both preventative and therapeutic potential in the context of contemporary health challenges (Pan et al., 2024). Despite the promising benefits of KGM, challenges remain in standardizing its commercial production and ensuring quality control across different markets. Future research should address these limitations while exploring the full spectrum of KGM's health benefits and applications. This review was aimed to provide a comprehensive overview of the traditional uses, modern health applications, and cutting-edge research surrounding KGM, ultimately contributing to a deeper understanding of its potential in health and nutrition. This review article stands out by offering a holistic view of A. konjac, from its traditional uses to its modern health applications, supported by scientific research and innovative approaches like multi-omics analysis and HTS.
A. konjac, commonly known as konjac, is a perennial plant native to Southeast Asia, particularly valued for its edible corms and traditional applications in food and medicine. This plant has been cultivated for thousands of years, not only as a food source but also for its medicinal properties in traditional Chinese medicine (TCM) (Derosa et al., 2024). The cultivation practices of A. konjac involve planting corms, where factors such as corm size and plant density play a crucial role in determining the yield (Xu et al., 2024). Globally, A. konjac is produced in significant quantities, with major cultivation occurring in China and Japan, and its production is expanding into other Southeast Asian countries (Luo et al., 2024). The plant prefers warm subtropical to tropical climates, requiring well-drained, nutrient-rich soils that retain moisture without becoming waterlogged (Srzednicki and Borompichaichartkul, 2020). Additionally, moderate shading (50%-70%) is beneficial as it enhances photosynthesis and increases corm weight, making it an essential factor in cultivation (Qin et al., 2019; Nurshanti et al., 2023). The economic importance of A. konjac cannot be overstated, as it is in high demand within the food and health industries, primarily due to its glucomannan content, which is valued for its health benefits (Chua et al., 2010). The extraction of KGM from the corms is a key aspect of its commercial processing. This process typically involves washing, slicing, and drying the corms to obtain the soluble fiber used in various applications, including food products and dietary supplements (Kapoor et al., 2024; Widjanarko et al., 2024). The nutritional composition of A. konjac corms is noteworthy, as they are rich in glucomannan, starch, and various inorganic elements, contributing to their value as a dietary component (Kulkarni et al., 2024). Despite its benefits, the cultivation of A. konjac faces several challenges, including environmental factors, disease management, and the need to optimize growth conditions to enhance yields (Niu et al., 2024; Rahman et al., 2024). These challenges can impact the overall production and availability of A. konjac in the global market.
Its historical significance is underscored by its first documentation in the 'Shen Nong Materia Medica' during the Western Han Dynasty, which highlights its longstanding role in ancient Chinese medicine (Chua et al., 2010). Over the centuries, konjac has been integrated into various cultural practices, especially in China and Japan, where it has been consumed for its health benefits (Qi et al., 2024). The primary component of konjac is KGM, a water-soluble dietary fiber extracted from its corm. KGM has been utilized in TCM for thousands of years, often for detoxification and tumor suppression (Lin and Rezaei, 2024). The health benefits associated with KGM are well-documented, including its roles in weight management, cholesterol reduction, and digestive health (Devaraj et al., 2019a). Research indicates that KGM can enhance satiety, reduce body weight, and improve metabolic parameters by increasing the transit time of food and prolonging gastric emptying (Shang et al., 2020; Guo et al., 2021). Culinary applications of konjac are also significant, as it is used to create various dishes such as noodles, tofu, and snacks, showcasing its versatility as a food ingredient (Chua et al., 2010; Zhang et al., 2020). The refined konjac flour, which contains a high percentage of KGM, has been introduced into Western markets as a food additive and dietary supplement, reflecting its growing global appeal (Zhu, 2018). Modern research has focused on the extraction, characterization, and health benefits of KGM, expanding its applications in nutrition and medicine (Devaraj et al., 2019a). Studies have demonstrated that KGM possesses pharmacological properties, including anti-obesity and cholesterol-lowering effects, making it a valuable component in health-related products (Table 1; Figure 1) (Behera and Ray, 2016).
A. konjac is rich in various bioactive compounds, making it both nutritionally and pharmacologically valuable. The predominant compound in these corms is glucomannan, which accounts for approximately 49–60% of the corm weight (Alamgir, 2018). Additionally, corms contain 10–30% starch and 2.6–7% inorganic elements, including key minerals such as calcium, aluminum, magnesium, manganese, iron, chromium, and cobalt (Gómez et al., 2017). These corms also possess crude protein content ranging from 5% to 14%, 3% to 5% soluble sugars, and 3.4–5.3% ash. Furthermore, small quantities of bioactive compounds, such as alkaloids (such as trigonelline) and saponins, are present, particularly near the stem base (Sengupta et al., 2022). In addition to the essential organic and inorganic compounds, A. konjac corms are abundant in vitamins and other bioactive components. Corms are notable for their content of some organic compounds, which enhance their nutritional value. Furthermore, fresh corm tissue contains serotonin and its derivatives. These serotonin derivatives are interesting because of their potential therapeutic properties (Shi et al., 2019). The precise chemical composition of mature Amorphophallus corms can vary depending on species, geographic region, and environmental factors during cultivation. Among the nine species of Amorphophallus grown in China, A. konjac and A. albus are especially important for storing glucomannan as their primary carbohydrate, making them important for both traditional and modern applications (Mekkerdchoo et al., 2016). This variation in composition highlights the importance of understanding the specific growth conditions and species characteristics when utilizing these corms for their health benefits and industrial applications. The study of these variations can lead to optimized cultivation practices, potentially enhancing the yield of desired compounds, such as glucomannan and other bioactive constituents (Gao et al., 2023).
The chemical structure of polysaccharides plays a significant role in determining their functional and nutritional properties as well as their biological activities (Yu et al., 2018). The primary structure of KGM consists of repeating units of D-glucose and D-mannose in a molar ratio of 1:1.6–1.7, connected by β-1,4-glycosidic bonds. Some side chains may branch from the main mannose backbone at the C-3 position or from the sugar unit at the C-6 position through acetyl-group linkages. KGM exists in two native conformations, alpha (amorphous) and beta (crystalline), which affect its physical and functional properties, respectively. The molecular weight of KGM is relatively homogenous and normally distributed, although it can vary depending on factors such as origin, processing methods, and storage conditions. KGM is reported to have an average molecular weight of 5.83 × 105 g/mol, contributing to its unique functional properties in both food and health applications. The unique internal structure of the two-year-old KGM provides insight into the tissue composition of the plant. As the size and quantity of these idioblasts increase toward the center of the corm, the central region contains idioblasts that can reach up to 650 µm in diameter. This branching was relatively minimal, with approximately three branches for each of the 32 sugar units (Felix da Silva et al., 2020). In addition, these calcium oxalate formations are located within KGM-containing idioblasts and in the surrounding parenchyma. The dual presence of KGM and calcium oxalate within the corm tissue highlights the complex structure of A. konjac corms. KGM and calcium oxalate are deposited within corms, forming needle-shaped raphide crystals and multi-crystal druses, also known as cluster crystals (Chua et al., 2013). The molecular configuration of KGM includes a mannose-to-glucose ratio of approximately 1.6:1. These acetyl groups are crucial for enhancing the solubility of KGM (Kulkarni et al., 2024). The molecular weight of KGM varies widely, ranging from 200 to 2000 kDa, and is influenced by factors such as the specific cultivar, growing region, and processing and storage techniques used (Liu et al., 2021a). One of KGM’s distinctive properties of KGM is its ability to dissolve in hot and cold water. The solubility of KGM can be further enhanced by applying heat and mechanical agitation, which makes it versatile for various applications across the food and industrial sectors (Shi et al., 2019). KGM solutions may decrease over time, possibly because of bacterial contamination or enzymatic hydrolysis, particularly by β-mannanase. When mild alkali is added to KGM solutions, a thermostable gel is formed, which has applications in food and industrial products (Liu et al., 2021a). This unique combination of structural and physicochemical properties renders KGM a valuable compound for various applications (Table 2).
KGM is a soluble fiber derived from the konjac plant, known for its potential health benefits, particularly in managing metabolic disorders. One of the primary advantages of KGM is its ability to ameliorate conditions such as diabetes and hypercholesteremia, suggesting its role as a functional food in metabolic health (Jian et al., 2024). KGM is recognized as an effective weight loss aid, promoting satiety and reducing overall caloric intake. This is largely due to its capacity to form a gel-like substance in the stomach, which enhances feelings of fullness and helps control hunger (Jin et al., 2024). Consequently, individuals may experience reduced food intake, contributing to weight management efforts. Moreover, KGM supports digestive health by slowing down the digestive process, which can improve bowel movements and overall gut function (Deng et al., 2024). This property not only aids digestion but also plays a role in regulating blood sugar levels by slowing the absorption of glucose in the bloodstream after meals, thereby helping to maintain stable blood sugar levels (Fang et al., 2023a). The health benefits of KGM encompass weight loss support, appetite suppression, improved digestive health, and blood sugar regulation, making it a valuable addition to a balanced diet aimed at enhancing overall well-being (Table 3).
KGM, derived from the tubers of A. konjac, has gained attention for its multifaceted health benefits, particularly in the realm of metabolic health (Behera and Ray, 2016; Devaraj et al., 2019a). Its role in weight management, blood glucose regulation, and lipid profile improvement positions it as a valuable dietary intervention for conditions such as obesity and diabetes (Fang et al., 2023a). One of the primary applications of KGM is its effectiveness in promoting weight loss. Research indicates that incorporating glucomannan into a low-calorie diet significantly enhances weight loss outcomes compared to a diet alone (Jian et al., 2024). In a study involving 30 patients, those who consumed glucomannan alongside a 1,200 kcal diet experienced greater reductions in body weight and fat mass, alongside improved satiety and adherence to dietary regimens (Koncz et al., 2021; Mah et al., 2022). This is particularly relevant given the rising prevalence of obesity in many populations. In an 8-week study, participants who added KGM to a hypocaloric diet lost an average of 5.5 lbs, with a notable reduction in body fat, compared to the diet-only group (Hasbay, 2019; Xu et al., 2023). Regular consumption of KGM has been shown to significantly decrease body fat, particularly in individuals with a higher body mass index (BMI). In a clinical trial involving children, the glucomannan treatment group experienced a notable reduction in mean overweight from 49.5% to 41%, indicating a substantial decrease in body fat over the course of the study (Ngondi et al., 2005). Additionally, another study reported that treated obese patients exhibited a significant decrease in excess weight of 51 ± 16% compared to controls after four months, further supporting the effectiveness of KGM in weight reduction (Behera and Ray, 2016).
In addition to weight loss, KGM has demonstrated positive effects on metabolic health by regulating blood glucose levels. The consumption of glucomannan has been associated with improved insulin sensitivity and lower blood sugar levels, which are crucial for managing diabetes (Shah et al., 2015a). This regulation of blood glucose is complemented by KGM's ability to enhance carbohydrate tolerance, further supporting its role in diabetes management (Devaraj et al., 2019a). Research has shown that KGM has demonstrated significant efficacy in reducing glucose levels by 55.37% when administered at a dose of 1.5 g/kg in both animal models and clinical settings (Ling et al., 2013). Additionally, konjac flour, which contains glucomannan, resulted in a 40.9% reduction in blood sugar levels under similar experimental conditions. In clinical trials involving type 2 diabetic patients, KGM supplementation has been associated with a reduction in fasting glucose levels by 23.2% compared to placebo (Shah et al., 2015a; Behera and Ray, 2016). Dosage differences also play a crucial role in the effectiveness of KGM. Studies have reported a wide range of KGM dosages, from 0.7 g to 15 g per day, which can significantly influence the outcomes related to blood sugar regulation and cholesterol reduction (Devaraj et al., 2019a; Fang et al., 2023a). Higher dosages may yield more pronounced effects, while lower dosages might not be sufficient to achieve significant results. Furthermore, the demographic and health characteristics of study participants can affect KGM's efficacy. Factors such as age, BMI, and pre-existing health conditions can lead to variations in how individuals respond to KGM supplementation (Devaraj et al., 2019a; Colantonio et al., 2020). By forming a gel-like substance in the stomach, KGM not only slows the absorption of sugars but also helps regulate the release of insulin (Xia et al., 2024). Improved insulin sensitivity indicates that cells become more responsive to insulin, facilitating better regulation of blood glucose levels (Kapoor et al., 2024). Additionally, some studies suggest that KGM may help lower HbA1c levels, which are indicators of long-term blood sugar control and are vital for preventing diabetes-related complications. In addition to these direct effects, KGM also supports metabolic health through its impact on weight. Additional weight is a main risk factor for Type 2 diabetes, and the ability of KGM to induce satiety can help with weight management and reduce overall caloric intake. This weight-lowering effect can further enhance insulin sensitivity and glycemic control (Watanabe et al., 2020). Additionally, KGM, a soluble dietary fiber derived from the konjac plant, has demonstrated significant potential in improving cholesterol levels, particularly by lowering LDL-C and triglycerides, which are crucial for cardiovascular health. In a controlled study, subjects taking glucomannan experienced a notable reduction in serum cholesterol by −32.0 mg/dl and LDL-C by −28.7 mg/dl, indicating its effectiveness in managing lipid profiles (Behera and Ray, 2016). The mechanism behind KGM's cholesterol-lowering effects is attributed to its ability to bind bile acids in the intestine, promoting their excretion and subsequently leading to a reduction in cholesterol levels as the body utilizes cholesterol to replenish bile acids (Kapoor et al., 2024). Meta-analyses of KGM studies suggest overall benefits in lowering cholesterol and blood glucose, but they also highlight the need for standardized methodologies to improve reliability and comparability of results (Ho et al., 2017). Clinical trials have reported significant reductions in fasting glucose and cholesterol levels with KGM supplementation, yet these results can vary based on trial conditions (Chen et al., 2003; Xia et al., 2024).
Moreover, KGM contributes to the improvement of lipid profiles, particularly in lowering total serum cholesterol and enhancing overall lipid status. The same study that highlighted weight loss also reported significant improvements in lipid parameters for participants consuming glucomannan, indicating its potential for cholesterol management (Zhu et al., 2024). This is particularly important as elevated cholesterol levels are a major risk factor for cardiovascular diseases. The health benefits of KGM extend beyond individual metrics; its soluble fiber content aids in the maintenance of normal blood cholesterol levels, making it a promising candidate for dietary interventions aimed at improving metabolic health (Kapoor et al., 2024). In a study involving 110 elderly individuals with hyperlipidemia, those who consumed refined konjac meal experienced notable decreases in triglyceride (TG), total cholesterol (TC), and LDL-C levels, along with increases in HDL-C and apoprotein (AI) levels. In contrast, the control group, which did not consume KGM, showed no changes in blood lipid and cholesterol (Mah et al., 2022). In a study assessing the efficacy of Minolest, which contains KGM, participants experienced a significant decrease in TC by 3.24%. Additionally, the study highlighted a more pronounced reduction in LDL-C, which decreased by 5.45% (Reimer et al., 2013; Li et al., 2021). These findings suggest that KGM supplementation can be beneficial for individuals with mild hypercholesterolemia, particularly those at a lower risk for coronary artery disease (Koyama, 2017). KGM has been shown to be effective in managing childhood obesity, particularly in reducing excess weight among obese children. In a clinical study involving 23 obese children aged between 5.2 and 15.8 years, those treated with highly purified glucomannan fibers experienced a significant reduction in excess weight, averaging 51 ± 16% compared to controls. This treatment involved administering 2-3 capsules of glucomannan twice daily, alongside a balanced diet, which ensured adequate caloric intake for all participants (Behera and Ray, 2016). Moreover, another study indicated that children under glucomannan treatment demonstrated a decrease in mean overweight from 49.5% to 41% over a two-month period, highlighting its effectiveness in weight management (Behera and Ray, 2016). In hypercholesterolemic children, the incorporation of KGM into a low-fat, fiber-rich diet has demonstrated significant efficacy in reducing cholesterol levels. Specifically, the treatment resulted in a 5.1% reduction in TC levels and a 7.3% decrease in LDL-C levels (Santos et al., 2011). These findings highlight the potential of KGM as a beneficial dietary intervention for managing cholesterol levels in this population. Moreover, gender differences were observed in the effectiveness of KGM treatment. In females, the reduction in TC was reported at 6.1%, while the decrease in LDL-C was even more pronounced at 9% (Vuorio et al., 2017; Ding et al., 2020). The ability of KGM to act as a natural polysaccharide with excellent biocompatibility and biodegradability further enhances its appeal for use in functional foods and supplements (Chandarana et al., 2024). KGM serves as a powerful tool in modern health applications, particularly for metabolic health. Its efficacy in weight loss, blood glucose regulation, and lipid profile improvement underscores its potential as a dietary intervention for managing obesity and diabetes, making it a significant focus for future research and public health initiatives (Figure 2) (Liu et al., 2025).
KGM is a soluble fiber that has garnered attention for its potential to modulate the gut microbiome, which may play a significant role in CRC prevention. One of the primary mechanisms by which KGM exerts its effects is through the enhancement of beneficial gut bacteria, particularly the Bifidobacterium genus and the Lactobacillus–Enterococcus group (Li et al., 2024). These populations significantly increase following the fermentation of KGM, suggesting a favorable modulation of gut microbiota that could contribute to protective effects against CRC (Gómez et al., 2017; Wu et al., 2011). Data from studies indicate that a daily intake of 3 grams of KGM results in an increase of 0.5 times per day and 3 additional evacuations per week compared to baseline values. When the dosage is increased to 4 grams per day, the effect is even more pronounced, with an increase of 0.9 times per day and 6 more evacuations per week. This demonstrates a clear dose-response relationship, where higher doses of KGM correlate with greater improvements in stool bulk and frequency of bowel movements (Hayeeawaema et al., 2020). KGM, a soluble dietary fiber, forms a gel-like substance when it enters the digestive tract. This gel can improve stool consistency, which is particularly beneficial for individuals with digestive disorders such as irritable bowel disease (IBD). KGM's ability to regulate stool consistency may help alleviate symptoms associated with IBD, such as diarrhea and constipation, thus contributing to better overall bowel health and comfort (Guarino et al., 2020).
KGM also acts as a prebiotic that fosters the growth of beneficial gut bacteria. The healthy gut microbiome plays a critical role in maintaining intestinal health and reducing inflammation. By supporting the proliferation of beneficial bacteria, KGM may contribute to a balanced gut environment, which is essential for managing IBD and promoting overall gut health (Du et al., 2021). Maintaining a healthy weight can be a challenge for patients due of symptoms such as malabsorption, reduced appetite, and dietary restrictions. KGM has been shown to induce a feeling of fullness, help manage appetite, and support weight control. This could be particularly beneficial for IBD patients striving to maintain a healthy weight, as proper weight management is linked to better overall well-being and could positively influence the management of IBD symptoms (Shah et al., 2020). Despite its potential benefits, there are some considerations when incorporating KGM into the diet of IBD patients. Tolerance to KGM can vary, and some individuals may experience gastrointestinal discomfort when introducing new fibers. It is recommended to start with small amounts of KGM and gradually increase intake while monitoring for any adverse effects (Zhang et al., 2021b). Additionally, KGM may affect the absorption of certain medications; therefore, it is crucial for patients to consult their healthcare provider before incorporating KGM into their diet to ensure that it does not interfere with their treatment plan (Pan et al., 2024).
KGM also promotes the production of short-chain fatty acids (SCFAs), which are crucial for gut health. The increase in cecal SCFA contents observed with KGM supplementation indicates that it not only supports beneficial bacteria but also enhances the metabolic byproducts that these bacteria produce. SCFAs are known to have anti-inflammatory properties and may help in reducing the risk of CRC by maintaining gut barrier integrity and modulating immune responses (Zhang et al., 2023; Jin et al., 2025). Research indicates that a diet rich in dietary fiber, including KGM, is associated with a lower risk of developing CRC. This protective effect is thought to arise from the fermentation of dietary fibers in the gut, leading to the production of SCFAs, which may exert chemopreventive properties on colonocytes (Devaraj et al., 2019a). Clinical trials have demonstrated that adjuvant therapies incorporating KGM, such as immunochemotherapy with polysaccharide K (PSK), can lead to improved survival rates and disease-free survival in patients with curatively resected CRC (Sawai et al., 2019). These findings highlight KGM's potential not only as a dietary supplement but also as a therapeutic agent that may mitigate the adverse effects of cancer treatments and enhance overall efficacy. High-fiber diets, particularly those rich in whole grains and vegetables, are consistently associated with a lower risk of CRC (Shah et al., 2015a).
The application of KGM to hyperthyroidism treatment is a novel area of research. Hyperthyroidism, characterized by an overactive thyroid gland, leads to the excessive production of thyroid hormones, causing symptoms such as weight loss, rapid heartbeat, and increased metabolism (Behera and Ray, 2016). Hyperthyroidism can affect digestive health, leading to diarrhea or malabsorption. KGM, with its fiber and prebiotic properties, can help manage gastrointestinal symptoms by improving gut health and regularity (Kalra et al., 2021). KGM’s appetite-suppressing effects of KGM and its role in promoting satiety could potentially aid in managing weight changes associated with hyperthyroidism. By reducing appetite and caloric intake, KGM may help balance metabolic changes caused by these conditions (Gan et al., 2024). In hyperthyroidism, absorption of certain nutrients may be disrupted. KGM’s role in improving overall digestive health could support better nutrient absorption and help manage deficiencies commonly seen in thyroid disorders (Devaraj et al., 2019b). Although the use of KGM in wound dressings has already been established and has clinical support, its application in hyperthyroidism treatment is less well documented and requires further investigation. Research on KGM’s potential benefits of KGM for hyperthyroidism is ongoing, and more studies are needed to validate its effectiveness and safety in this context.
Moreover, KGM has demonstrated anti-genotoxic effects, which are vital in preventing DNA damage that can lead to cancer. Studies have shown that KGM supplementation reduces fecal β-glucuronidase activity and secondary bile acid levels, both of which are associated with increased cancer risk. This reduction in harmful metabolites may lower the precancerous risk factors in the colon (Yan et al., 2024). The prebiotic potential of KGM is further supported by its ability to stimulate the growth of beneficial gut bacteria, thereby enhancing gut microbiome diversity and activity (Deng et al., 2024). This modulation of the gut microbiome not only contributes to improved gut health but also plays a role in the prevention of CRC by potentially influencing metabolic pathways related to cancer development. KGM's mechanisms for modulating the gut microbiome include enhancing beneficial bacterial populations, increasing SCFA production, and exerting anti-genotoxic effects (Zhang et al., 2024). These actions collectively contribute to a healthier gut environment, which is crucial for reducing the risk of CRC. Future research, particularly in vivo studies involving human subjects, is necessary to further elucidate these mechanisms and confirm the beneficial effects of KGM on gut health and cancer prevention (Ye et al., 2021).
Regular consumption of KGM has been shown to significantly alleviate skin inflammation, particularly in models of atopic dermatitis. KGM, a dietary fiber derived from the konjac plant, possesses notable anti-inflammatory properties that contribute to its effectiveness in managing skin conditions (Pan et al., 2024). Specifically, pulverized konjac glucomannan (PKGM) has been demonstrated to reduce the frequency of skin inflammation and associated symptoms in murine models. In studies involving PKGM, mice exhibited a marked decrease in the IL-4/IFN-γ ratio in the colonic lamina propria, indicating an improvement in immune response and a reduction in inflammation (Tian et al., 2023). Furthermore, the administration of PKGM not only mitigated skin inflammation but also suppressed hyper-IgE production, which is often linked to allergic reactions and dermatitis (Devaraj et al., 2019b). The anti-inflammatory activity of KGM is prominently demonstrated through its effects in a mouse model of oxazolone-induced colitis (OXA), where it significantly ameliorated the symptoms associated with this condition. In the context of OXA-induced colitis, KGM administration resulted in a marked reduction in the levels of critical inflammatory cytokines, specifically interleukin-4 (IL-4) and interleukin-13 (IL-13) (Onitake et al., 2015). These cytokines are pivotal in mediating inflammatory responses, and their suppression indicates a robust anti-inflammatory effect of KGM. The study revealed that PKGM not only improved histological markers of colonic inflammation but also led to a decrease in the population of NK1.1+ T cells in the liver, which is associated with the induction of Th1-polarized immune responses. The mechanism underlying KGM's anti-inflammatory activity appears to involve cytokine regulation, as it influences the levels of pro-inflammatory cytokines such as TNF-α and IL-1β, which are crucial in inflammatory processes (Handa et al., 2015). By modulating these cytokines, KGM can effectively reduce inflammation and enhance immune responses, showcasing its potential therapeutic applications in inflammatory diseases. Moreover, the study highlights that the preventive role of KGM in OXA-induced colitis was not observed in invariant natural killer T cell-deficient mice, further emphasizing the importance of immune modulation in its mechanism of action (Ye et al., 2021; Fang et al., 2023b).
Emerging research suggests that dietary fibers such as KGM may have anti-inflammatory properties. This fiber has the potential to modulate the inflammatory response in the gut, which is a key aspect in managing irritable bowel disease (IBD). Although preliminary findings are promising, more studies are required to fully understand the extent of KGM's anti-inflammatory effects of KGM and its practical applications for reducing inflammation in individuals with IBD (Changchien et al., 2021). KGM has shown significant potential in wound care due to its unique physical and chemical properties, making it an effective component in modern wound dressings. The primary benefit of KGM-based wound dressings is their ability to form gel-like substances when hydrated, which offers several advantages in wound management. One of the key features of KGM in wound dressings is its ability to maintain a moist environment around the wound. A moist environment is critical to promote faster and more efficient wound healing. This moisture prevents the wound from drying out and forms a crust, which can impede the healing process. KGM dressings help keep the wound bed hydrated, facilitating cell migration and tissue repair, ultimately accelerating the healing process (Zhou et al., 2022). Another important benefit of KGM is its ability to absorb wound exudates. As the gel formed by KGM absorbs excess wound fluid, it helps to manage the moisture levels at the wound site, reducing the risk of maceration. Maceration, which occurs when the skin becomes soft and breaks down owing to prolonged exposure to moisture, can lead to further complications in wound healing. By effectively managing the exudate, KGM dressings contribute to a more stable and controlled healing environment (Borbolla-Jiménez et al., 2023). KGM is highly biocompatible and non-toxic, making it particularly suitable for use in medical applications, such as wound care. It does not cause irritation or allergic reactions, thereby ensuring patient comfort and safety during the healing process. The non-reactive nature of KGM allows its use in a wide range of patients, including those with sensitive skin or allergies, making it a versatile and safe option for wound management (Veerasubramanian et al., 2018). In some formulations, KGM wound dressings are combined with antimicrobial agents to prevent infections, which can be a major complication of wound care. These antimicrobial KGM dressings help reduce the risk of bacterial infections at the wound site, support optimal healing, and minimize the chances of further complications. This combination of antimicrobial properties and KGM's natural benefits enhances the efficacy of these dressings in clinical settings (Alven et al., 2022).
KGM may influence the immune system primarily through its positive effects on gut health and its ability to modulate inflammation. One of the key mechanisms by which KGM supports immune function is through the modulation of the gut microbiota. Acting as a prebiotic, KGM promotes the growth of beneficial gut bacteria, such as Bifidobacteria and Lactobacilli, which are crucial for maintaining a balanced gut microbiome (Ye et al., 2021). Because a significant portion of the immune system is located in the gut-associated lymphoid tissue (GALT), the health of the gut microbiome directly affects immune responses. By promoting a healthy microbiome, KGM plays a role in strengthening immune defenses (Devaraj et al., 2019b). Another important way to support the immune system is through the production of SCFAs during fermentation by gut bacteria. SCFAs, including acetate, propionate, and butyrate, are essential for maintaining gut integrity and reducing inflammation, which can influence systemic immune responses (Zhang et al., 2022b). By promoting immune tolerance, KGM can help prevent excessive or inappropriate immune reactions, potentially reducing the risk of autoimmune disorders (Elshaghabee and Rokana, 2021). Additionally, a healthy gut microbiome is key to the development and activity of immune cells, such as T-cells and macrophages, which are central to immune defense mechanisms (Pan et al., 2024). Specifically, PKGM supplementation has been reported to suppress the OVA-specific IgE response, which is a critical marker of allergic reactions, without adversely affecting other immune responses such as IgG1 and IgG2a production (Suzuki et al., 2010). In addition to its effects on allergic rhinitis, KGM has demonstrated efficacy in preventing skin inflammation associated with atopic dermatitis (Guerreiro et al., 2023). Studies have shown that PKGM can ameliorate eczema and hyper-IgE production in mouse models, suggesting its potential as a dietary intervention for managing atopic diseases (Mao et al., 2022). However, it is important to note that the effects of KGM on the immune system vary among individuals. Those with immune-related disorders should consult healthcare professionals before incorporating KGM into their diet to ensure their safety and efficacy in specific health conditions (Srividya et al., 2024). Overall, KGM’s ability to support gut health and produce beneficial metabolites, such as SCFAs, positions it as a potential modulator of immune function (Figure 3).
KGM is emerging as a significant player in cutting-edge research within the fields of drug delivery and biological materials. KGM, derived from the corms of A. konjac, exhibits unique properties that make it an attractive candidate for various applications, particularly in the pharmaceutical sector due to its biocompatibility and biodegradability (Kulkarni et al., 2024; Zhuang et al., 2024). Recent studies have focused on the development of KGM-based microcapsules, which serve as innovative carriers for drug delivery. These microcapsules are prepared using a piercing method, where KGM forms the main membrane, encapsulating active ingredients such as N-Methyl-2-pyrrolidone (NMP) (Spirk, 2018; Khodadadi Yazdi et al., 2020). The preparation process involves blending KGM with Xanthan gum to create a gel that enhances the viscosity and tenacity of the microcapsules, thereby improving their drug delivery characteristics (Lin et al., 2024). The resulting konjac micro-balls (KMBs) demonstrate sustained-release properties, with delivery times exceeding 24 hours, indicating their potential for long-term therapeutic applications (Zhang et al., 2014). Key performance metrics such as embedding rate and encapsulation yield are critical in assessing the effectiveness of these KGM-based microcapsules. The embedding rate reflects the efficiency of drug loading, while encapsulation yield quantifies the success of the microcapsule formation process (Zhang et al., 2024). These metrics are essential for optimizing drug delivery systems, ensuring that therapeutic agents are effectively delivered to target sites within the body. Moreover, the preparation of KGM gel through alkali catalysis has opened new avenues for research in material science. The gel's properties, influenced by factors such as concentration, pH, and temperature, contribute to its potential applications in drug delivery systems (Sun et al., 2023). The molecular structure of KGM gel, characterized by advanced techniques like Fourier transform infrared spectroscopy and wide-angle X-ray diffraction, reveals a more regular arrangement compared to pure KGM, enhancing its functional capabilities in biological applications (Yang et al., 2017). The integration of KGM in drug delivery and biological materials research highlights its versatility and potential. The ongoing exploration of KGM-based systems promises to yield innovative solutions for effective drug delivery, addressing critical challenges in the pharmaceutical industry and paving the way for future advancements in health care (Zhou et al., 2022; Zhuang et al., 2024).
Furthermore, KGM serves as the primary membrane in the formation of microcapsules, which are designed to enhance drug delivery through their ability to control release and target specific sites within the body (Zhang et al., 2024). The encapsulation yield, which measures the effectiveness of KGM in encapsulating therapeutic agents, is a critical factor that underscores its potential in targeted delivery systems (Kapoor et al., 2024; Madawi et al., 2024). The structural integrity of KGM is significantly influenced by its hydrogen bonding network, particularly at the O (Xia et al., 2024) and O (Islam et al., 2023) positions on the KGM ring. These key linking points contribute to the stability of the hydrogen bonding network, which is essential for maintaining the functionality of KGM in drug delivery applications (Sun et al., 2023; Zheng et al., 2024a). The stability of this network is further enhanced through the process of deacetylation, which not only improves the hydrogen bonding structures but also increases the overall performance of KGM in drug delivery systems (Sun et al., 2023; Ye et al., 2021). Moreover, the gelation performance of KGM is crucial for its application in drug delivery. The ability of KGM to form gels allows it to encapsulate and release drugs in a controlled manner, thereby improving the therapeutic efficacy of the delivered agents (Lafarge and Cayot, 2018; Kapoor et al., 2024). The drug loading capacity of KGM-based microcapsules demonstrates how its unique structure can effectively accommodate and deliver therapeutic agents, ensuring that the drugs are released at the desired rate and location (Shen et al., 2024). The embedding rate of the microcapsules, which reflects the efficiency of KGM's structure in encapsulating the core substance, is another important aspect that enhances targeted drug delivery (Cui et al., 2021). The combination of these structural features—hydrogen bonding stability, gelation properties, and effective encapsulation—positions KGM as a promising candidate for developing advanced drug delivery systems (Table 4).
Multi-omics analysis and HTS are pivotal methodologies that enhance the functional evaluation of KGM, a polysaccharide known for its health benefits and applications in food and pharmaceuticals. Multi-omics analysis integrates data from various biological layers, including genomics, proteomics, and metabolomics, to provide a comprehensive understanding of KGM's biological functions and interactions within biological systems (Wang and Li, 2021). This integrative approach allows researchers to identify the molecular mechanisms through which KGM exerts its health benefits, such as its antioxidant properties and cellular protection capabilities (Zhang et al., 2021a). On the other hand, HTS facilitates the rapid assessment of KGM's biological activity by enabling the testing of numerous compounds and their effects on specific biological pathways. This method is crucial in drug discovery and functional evaluation, as it allows for the identification of active compounds that can enhance the extraction and purification processes of KGM (Zhang et al., 2021b; Shen et al., 2024). By employing HTS, researchers can efficiently screen various extraction methods and purification techniques, optimizing conditions to isolate pure KGM or its derivatives for further study (Franková and Fry, 2015; Qi and Chen, 2024). The functional evaluation of KGM is further supported by the assessment of its antioxidant activity, which can be measured through various assays that evaluate its ability to scavenge free radicals (Zhu, 2018). This evaluation is essential for understanding how KGM can protect cells from oxidative stress, a significant factor in many diseases. Additionally, the purification techniques employed to isolate KGM are critical, as they ensure that the final product retains its functional properties, including its viscosity and health benefits (Widjanarko et al., 2024). Moreover, enzymatic hydrolysis and γ-irradiation are techniques that can modify KGM to enhance its functional properties. Enzymatic hydrolysis breaks down KGM into oligo-glucomannan, which may exhibit improved bioactivity (Zhu, 2018; Zheng et al., 2024a). Similarly, γ-irradiation can induce structural changes that enhance the extraction efficiency and functional characteristics of KGM (Zheng et al., 2024b).
However, both techniques come with their own set of advantages and disadvantages. The advantages of multi-omics include comprehensive data integration and improved understanding of biological processes, which can lead to enhanced identification of therapeutic targets (Chen et al., 2021; Li et al., 2024). Conversely, the disadvantages of HTS may include high costs, the potential for false positives, and the necessity for extensive validation of results (Misra et al., 2019). These challenges can complicate the interpretation of data and may require additional resources to ensure reliability. The integration of multi-omics and HTS in KGM research holds significant potential for future studies. By combining the strengths of both approaches, researchers can gain deeper insights into KGM's properties and interactions, ultimately leading to improved applications in food preservation and drug delivery systems. For instance, understanding the gel formation mechanism of KGM, which involves molecular interactions such as hydrogen bonding and hydrophilic group interactions, can be enhanced through multi-omics data (Song et al., 2024). Additionally, chemical modifications of KGM can be systematically evaluated using HTS to optimize its properties for specific applications (Tsurumaki et al., 2015). The synergistic use of multi-omics analysis and HTS can significantly advance the functional evaluation of KGM, paving the way for innovative applications and improved product formulations in both the food and pharmaceutical industries (Table 5).
The quality of KGM products is influenced by several critical factors that play a significant role in their formulation and performance. Understanding these factors is essential for optimizing the efficacy of KGM-based applications, particularly in drug delivery systems. One of the primary factors is the concentration of KGM itself. The concentration directly affects the release degree of encapsulated drugs, such as cap, which is crucial for ensuring that the active ingredients are delivered effectively. Optimal conditions have been identified, with a concentration of 2.5% (w/v) KGM yielding a predicted release degree of 92.258% for cap (Chao et al., 2012). This highlights the importance of selecting the right concentration to achieve desired release profiles. Another significant factor is the embedding rate, which determines how efficiently active ingredients are incorporated into the KGM matrix. A higher embedding rate typically correlates with better delivery of the active compounds, thereby enhancing the overall quality of the KGM product (Sun et al., 2023). Additionally, the drug loading capacity is a direct measure of how well the KGM microcapsules can incorporate and deliver these active compounds, further influencing product quality (Shen et al., 2024). The encapsulation yield is also a critical parameter, reflecting the effectiveness of the microencapsulation process. A higher encapsulation yield indicates a more successful process, which is vital for the functionality of KGM products (Zhang et al., 2024). Furthermore, the physical properties of the microcapsules, such as particle size and distribution, are essential for understanding their performance. These characteristics can significantly impact the release behavior and stability of the KGM products (Halahlah et al., 2023). The preparation parameters, including mixing time, laying-time, and drying temperature, are crucial for ensuring the quality of KGM membranes. For instance, the duration of mixing affects the uniformity of the membrane, while the laying-time is important for proper formation (Yuan et al., 2017). The drying temperature, specifically at 65°C, has been shown to influence the final quality and release characteristics of the product (Ni et al., 2021). Lastly, the morphology of the KGM products provides insights into their structural characteristics, which are critical for assessing quality. Analyzing the morphology can help identify any potential issues in the formulation process that may affect performance (Sun et al., 2023; Kapoor et al., 2024). The safety and efficacy of KGM products are significantly influenced by the dosage forms and dosages used in clinical studies. A notable example is the specific dosage of 3.9 g of KGM administered daily, which was shown to effectively lower TC levels by 10% in a controlled study involving 63 healthy men (Behera and Ray, 2016; Jian et al., 2024). This dosage not only demonstrated a significant reduction in TC but also led to a 7.2% decrease in LDL-C and a 23% reduction in triglycerides, indicating a robust lipid-lowering effect (Nie and Luo, 2021; Cheung et al., 2023). This variability in dosage highlights the importance of tailoring KGM products to individual needs and health conditions to optimize safety and efficacy. In addition to dosage, the physiochemical properties of KGM can also be altered through processes such as γ-irradiation. Studies have shown that different doses of γ-irradiation (5, 20, 50, and 100 kGy) can affect the weight-average molecular weight (M_w) and apparent viscosity of KGM, which are critical factors influencing its functional properties and, consequently, its safety and efficacy (Xu et al., 2007; Jian et al., 2016).
The extraction and purification of KGM on a large scale presents significant sustainability challenges that must be addressed to minimize environmental impact. One of the primary difficulties lies in maintaining ecological balance during these processes, which often leads to adverse effects on local ecosystems (Behera and Ray, 2017; Pan et al., 2024). Resource efficiency is a crucial aspect of sustainable KGM production. The extraction and purification processes must be optimized to minimize waste and maximize the use of available resources. This involves not only improving the efficiency of the extraction methods but also considering the trade-offs between different resource efficiency objectives, such as reducing environmental impacts while ensuring cost-effectiveness (He et al., 2024). The complexity of these trade-offs highlights the need for a comprehensive approach to resource management in KGM production. Technological innovations present a promising avenue for addressing these sustainability challenges. Advancements in extraction techniques could lead to more sustainable practices that reduce environmental impacts and enhance resource efficiency (Mathura et al., 2024). However, the adoption of such technologies must be coupled with strict regulatory compliance to ensure that environmental standards are met throughout the production process. Additionally, the impact of climate change on the availability and quality of raw materials for KGM extraction cannot be overlooked. Fluctuations in climate conditions can affect the growth of konjac plants, thereby influencing the sustainability of KGM supply chains (Srzednicki and Borompichaichartkul, 2020). This underscores the importance of supply chain coordination among producers, suppliers, and manufacturers to enhance the overall sustainability of KGM production. Addressing the sustainability challenges associated with large-scale KGM extraction and purification requires a multifaceted approach that includes improved waste management, resource efficiency, technological innovations, regulatory compliance, and effective supply chain coordination. By tackling these limitations, the industry can move towards a more sustainable future for KGM production.
To enhance the future outlook for research on KGM, strategic recommendations can be made focusing on improving extraction efficiency and developing more effective functional food and drug delivery systems. Firstly, leveraging biotechnological methods to enhance the extraction efficiency of KGM from konjac tubers is crucial. Current extraction processes can be optimized through innovative biotechnological approaches, which may lead to higher yields and better quality of KGM. This aligns with the need for improved extraction techniques that can support the growing demand for KGM in various applications. Secondly, the development of KGM-based functional food and drug delivery systems should be prioritized. Research indicates that KGM can be effectively utilized in creating microcapsules that enhance the delivery of active ingredients, such as vitamins and drugs. The preparation of KGM-based microcapsules has shown promising results in terms of morphology, particle size distribution, and encapsulation efficiency, which are critical for ensuring the stability and bioavailability of the encapsulated substances. Moreover, optimizing the release properties of these systems is essential. Studies have identified key factors affecting the release degree of encapsulated compounds, such as concentration of KGM, mixing time, and drying conditions. For instance, optimal conditions for the release of a model drug were determined to be 2.5% (w/v) KGM mixed with specific parameters, achieving a predicted release degree of 92.258%. Future research should continue to explore these parameters using response surface analysis to refine the formulation processes further. Additionally, the synergistic effects of KGM with other polysaccharides, such as xanthan gum, should be investigated. This combination has demonstrated potential in creating sustained release systems, which can significantly improve the efficacy of drug delivery. Lastly, the development of innovative functional food products incorporating KGM can address health concerns such as weight management and cholesterol control. By focusing on these health benefits, researchers can create products that not only meet consumer demand but also contribute positively to public health. In conclusion, by enhancing extraction methods, optimizing drug delivery systems, and developing functional foods, future research can significantly advance the applications of KGM, making it a valuable component in health and nutrition sectors (Table 6).
A. konjac, particularly its bioactive component KGM, stands as a promising dietary fiber with extensive health benefits and industrial applications. Its unique physicochemical properties, such as high viscosity and gel-forming ability, enable its use in various health and nutritional applications, including blood glucose regulation, weight management, lipid metabolism improvement, and gastrointestinal health. KGM’s prebiotic and anti-inflammatory properties further enhance its therapeutic potential, with emerging applications in wound care, hyperthyroidism management, and CRC prevention. Despite its established benefits, the continued growth of KGM-based products requires rigorous quality control measures to ensure purity, molecular consistency, and safety. Addressing potential side effects, such as gastrointestinal discomfort and allergenicity, is essential for consumer safety. Future research focusing on bioavailability, targeted delivery systems, and novel formulations can significantly enhance KGM’s therapeutic efficacy. Innovations in sustainable cultivation and ethical sourcing practices will ensure the environmental and economic viability of KGM production. Expanding applications beyond current uses, particularly in pharmaceuticals, cosmetics, and medical devices, presents an exciting avenue for KGM research. Detailed mechanistic studies on KGM’s interaction with gut microbiota and metabolic pathways will deepen understanding of its health impacts and foster the development of targeted interventions. Population-based clinical trials and epidemiological studies are critical to establishing evidence-based guidelines for KGM consumption, ensuring its long-term safety and efficacy. In conclusion, KGM represents a versatile and beneficial component in functional foods, nutraceuticals, and therapeutic applications. With advancements in research, innovation, and sustainability, KGM holds immense potential to contribute significantly to global health and wellness, supporting diverse populations while promoting sustainable and ethical practices in its production and use.
AJ: Conceptualization, Data curation, Formal Analysis, Funding acquisition, Investigation, Methodology, Project administration, Resources, Software, Supervision, Validation, Visualization, Writing – original draft, Writing – review & editing. SS: Conceptualization, Data curation, Formal Analysis, Funding acquisition, Investigation, Methodology, Project administration, Resources, Software, Supervision, Validation, Visualization, Writing – original draft, Writing – review & editing. QG: Project administration, Resources, Supervision, Writing – review & editing. QW: Funding acquisition, Project administration, Resources, Software, Supervision, Writing – review & editing. J-SS: Conceptualization, Funding acquisition, Project administration, Resources, Software, Supervision, Visualization, Writing – review & editing.
The author(s) declare that financial support was received for the research, authorship, and/or publication of this article. Guizhou Science and Technology Corporation Platform Talents Fund (Grant No (2017).:5733-001 and CK-1130-002), the National Natural Science Foundation of China (U1812403, 82373981 and 82060750), 2011 Collaborative Innovation Centre of Traditional Chinese Medicine in Guizhou Province (No. (2022)026).
The authors express their gratitude for the financial support received through the Distinguished High-Level Talents Research Grant from the Guizhou Science and Technology Corporation Platform Talents Fund (Grant No.: [2017]5733-001 and CK-1130-002), the National Natural Science Foundation of China (U1812403, 82373981 and 82060750), 2011 Collaborative Innovation Centre of Traditional Chinese Medicine in Guizhou Province (No. [2022]026) and the support provided by the Zunyi Medical University, China. Special appreciation is extended to all laboratory colleagues and research staff members for their valuable insights, constructive guidance, and assistance throughout this study.
The authors declare that the research was conducted in the absence of any commercial or financial relationships that could be construed as a potential conflict of interest.
The author(s) declared that they were an editorial board member of Frontiers, at the time of submission. This had no impact on the peer review process and the final decision.
The author(s) declare that no Generative AI was used in the creation of this manuscript.
All claims expressed in this article are solely those of the authors and do not necessarily represent those of their affiliated organizations, or those of the publisher, the editors and the reviewers. Any product that may be evaluated in this article, or claim that may be made by its manufacturer, is not guaranteed or endorsed by the publisher.
Alamgir, A. N. M. (2018). Phytoconstituents–Active and inert constituents, metabolic pathways, chemistry and application of phytoconstituents, primary metabolic products, and bioactive compounds of primary metabolic origin. In: Therapeutic Use of Medicinal Plants and their Extracts: Volume 2. Progress in Drug Research, vol 74. (Cham: Springer), 25–164. doi: 10.1007/978-3-319-92387-1
Alven, S., Peter, S., Mbese, Z., Aderibigbe, B. A. (2022). Polymer-based wound dressing materials loaded with bioactive agents: potential materials for the treatment of diabetic wounds. Polymers (Basel). 14, 724. doi: 10.3390/polym14040724
Au-Yeung, F., Jovanovski, E., Jenkins, A. L., Zurbau, A., Ho, H. V. T., Vuksan, V. (2018). The effects of gelled konjac glucomannan fibre on appetite and energy intake in healthy individuals: a randomised cross-over trial. Br. J. Nutr. 119, 109–116. doi: 10.1017/S0007114517003233
Behera, S. S., Ray, R. C. (2016). Konjac glucomannan, a promising polysaccharide of Amorphophallus konjac K. Koch Health Care Int. J. Biol. Macromol. 92, 942–956. doi: 10.1016/j.ijbiomac.2016.07.098
Behera, S. S., Ray, R. C. (2017). Nutritional and potential health benefits of konjac glucomannan, a promising polysaccharide of elephant foot yam, Amorphophallus konjac K. Koch: A review. Food Rev. Int. 33, 22–43. doi: 10.1080/87559129.2015.1137310
Borbolla-Jiménez, F. V., Peña-Corona, S. I., Farah, S. J., Jiménez-Valdés, M. T., Pineda-Pérez, E., Romero-Montero, A., et al. (2023). Films for wound healing fabricated using a solvent casting technique. Pharmaceutics. 15, 1914. doi: 10.3390/pharmaceutics15071914
Chandarana, C., Bonde, S., Vashi, V., Akhter, M. S., Prajapati, B. (2024). Konjac glucomannan-based edible films: method, properties, and applications. J. Food Process Eng. 47 (12), e70009. doi: 10.1111/jfpe.v47.12
Changchien, C. H., Wang, C. H., ling, C. H. (2021). Konjac glucomannan polysaccharide and inulin oligosaccharide ameliorate dextran sodium sulfate-induced colitis and alterations in fecal microbiota and short-chain fatty acids in C57BL/6J mice. Biomedicine (Taipei). 11, 23–30. doi: 10.37796/2211-8039.1191
Chao, W., Mei, X., Yu-peng, Z., Yang, X., Wei-hua, Z., Dong-sheng, L. (2012). Study on preparation and optimization of konjac glucomannan coated soluble drug. In: Lee, G. (ed) Advances in Computational Environment Science. Advances in Intelligent and Soft Computing, vol 142. (Berlin, Heidelberg: Springer), 335–341. doi: 10.1007/978-3-642-27957-7_41
Chen, H., Nie, Q., Hu, J., Huang, X., Yin, J., Nie, S. (2021). Multiomics approach to explore the amelioration mechanisms of glucomannans on the metabolic disorder of type 2 diabetic rats. J. Agric. Food Chem. 69, 2632–2645. doi: 10.1021/acs.jafc.0c07871
Chen, H. L., Sheu, W. H. H., Tai, T. S., Liaw, Y. P., Chen, Y. C. (2003). Konjac supplement alleviated hypercholesterolemia and hyperglycemia in type 2 diabetic subjects—A randomized double-blind trial. J. Am. Coll. Nutr. 22, 36–42. doi: 10.1080/07315724.2003.10719273
Cheung, B., Sikand, G., Dineen, E. H., Malik, S., Barseghian-El-Farra, A. (2023). Lipid-lowering nutraceuticals for an integrative approach to dyslipidemia. J. Clin. Med. 12, 3414. doi: 10.3390/jcm12103414
Chua, M., Baldwin, T. C., Hocking, T. J., Chan, K. (2010). Traditional uses and potential health benefits of Amorphophallus konjac K. Koch ex N.E.Br. J. Ethnopharmacol. 128, 268–278. doi: 10.1016/j.jep.2010.01.021
Chua, M., Hocking, T. J., Chan, K., Baldwin, T. C. (2013). Temporal and spatial regulation of glucomannan deposition and mobilization in corms of Amorphophallus konjac (Araceae). Am. J. Bot. 100, 337–345. doi: 10.3732/ajb.1200547
Colantonio, A. G., Werner, S. L., Brown, M. (2020). The effects of prebiotics and substances with prebiotic properties on metabolic and inflammatory biomarkers in individuals with type 2 diabetes mellitus: A systematic review. J. Acad. Nutr. Diet. 120, 587–607.e2. doi: 10.1016/j.jand.2018.12.013
Cui, T., Chen, C., Jia, A., Li, D., Shi, Y., Zhang, M., et al. (2021). Characterization and human microfold cell assay of fish oil microcapsules: Effect of spray drying and freeze-drying using konjac glucomannan (KGM)-soybean protein isolate (SPI) as wall materials. J. Funct. Foods. 83, 104542. doi: 10.1016/j.jff.2021.104542
Dai, S., Jiang, F., Shah, N. P., Corke, H. (2019). Functional and pizza bake properties of Mozzarella cheese made with konjac glucomannan as a fat replacer. Food Hydrocoll. 92, 125–134. doi: 10.1016/j.foodhyd.2019.01.045
Deng, L., Zhong, G., Zhang, D., Zhu, Z., Peng, Y. (2024). Effects of konjac glucomannan and its oligosaccharides on improvement of lactose intolerance as gut prebiotics. ACS Omega. 9, 29609–29619. doi: 10.1021/acsomega.4c02768
Derosa, G., D’Angelo, A., Maffioli, P. (2024). The role of selected nutraceuticals in management of prediabetes and diabetes: An updated review of the literature. Part II. Phytotherapy Res. 38, 5490–5532. doi: 10.1002/ptr.v38.11
Devaraj, R. D., Reddy, C. K., Xu, B. (2019a). Health-promoting effects of konjac glucomannan and its practical applications: A critical review. Int. J. Biol. Macromol. 126, 273–281. doi: 10.1016/j.ijbiomac.2018.12.203
Devaraj, R. D., Reddy, C. K., Xu, B. (2019b). Health-promoting effects of konjac glucomannan and its practical applications: A critical review. Int. J. Biol. Macromol. 126, 273–281. doi: 10.1016/j.ijbiomac.2018.12.203
Ding, Y. F., Sun, T., Li, S., Huang, Q., Yue, L., Zhu, L., et al. (2020). Oral colon-targeted konjac glucomannan hydrogel constructed through noncovalent cross-linking by cucurbit[8]uril for ulcerative colitis therapy. ACS Appl. Bio Mater. 3, 10–19. doi: 10.1021/acsabm.9b00676
Du, Q., Liu, J., Ding, Y. (2021). Recent progress in biological activities and health benefits of konjac glucomannan and its derivatives. Bioactive Carbohydrates Dietary Fibre. 26, 100270. doi: 10.1016/j.bcdf.2021.100270
Elshaghabee, F. M. F., Rokana, N. (2021). Dietary management by probiotics, prebiotics and synbiotics for the prevention of antimicrobial resistance. In: Panwar, H., Sharma, C., Lichtfouse, E. (eds) Sustainable Agriculture Reviews 49. Sustainable Agriculture Reviews, vol 49. (Cham: Springer). doi: 10.1007/978-3-030-58259-3_233–56
Fang, Y., Ma, J., Lei, P., Wang, L., Qu, J., Zhao, J., et al. (2023a). Konjac glucomannan: an emerging specialty medical food to aid in the treatment of type 2 diabetes mellitus. Foods. 12, 363. doi: 10.3390/foods12020363
Fang, Y., Ma, J., Lei, P., Wang, L., Qu, J., Zhao, J., et al. (2023b). Konjac glucomannan: an emerging specialty medical food to aid in the treatment of type 2 diabetes mellitus. Foods. 12, 363. doi: 10.3390/foods12020363
Felix da Silva, D., Ogawa, C. Y. L., Sato, F., Neto, A. M., Larsen, F. H., Matumoto-Pintro, P. T. (2020). Chemical and physical characterization of Konjac glucomannan-based powders by FTIR and 13C MAS NMR. Powder Technol. 361, 610–616. doi: 10.1016/j.powtec.2019.11.071
Franková, L., Fry, S. C. (2015). A general method for assaying homo- and hetero-transglycanase activities that act on plant cell-wall polysaccharides. J. Integr. Plant Biol. 57, 411–428. doi: 10.1111/jipb.12337
Gan, H. W., Cerbone, M., Dattani, M. T. (2024). Appetite- and weight-regulating neuroendocrine circuitry in hypothalamic obesity. Endocr. Rev. 45, 309–342. doi: 10.1210/endrev/bnad033
Gao, L., Xia, X., Shuai, Y., Zhang, H., Jin, W., Zhang, X., et al. (2023). Gut microbiota, a hidden protagonist of traditional Chinese medicine for acute ischemic stroke. Front. Pharmacol. 14. doi: 10.3389/fphar.2023.1164150
Gómez, B., Míguez, B., Yáñez, R., Alonso, J. L. (2017). Manufacture and properties of glucomannans and glucomannooligosaccharides derived from konjac and other sources. J. Agric. Food Chem. 65 (10), 2019–2031. doi: 10.1021/acs.jafc.6b05409
Gómez, B., Míguez, B., Yáñez, R., Alonso, J. L. (2017). Manufacture and properties of glucomannans and glucomannooligosaccharides derived from konjac and other sources. J. Agric. Food Chem. 65, 2019–2031. doi: 10.1021/acs.jafc.6b05409
Guarino, M., Altomare, A., Emerenziani, S., Di Rosa, C., Ribolsi, M., Balestrieri, P., et al. (2020). Mechanisms of action of prebiotics and their effects on gastro-intestinal disorders in adults. Nutrients. 12, 1037. doi: 10.3390/nu12041037
Guerreiro, F., Pontes, J. F., Gaspar, M. M., Rosa da Costa, A. M., Faleiro, M. L., Grenha, A. (2023). Respirable konjac glucomannan microparticles as antitubercular drug carriers: Effects of in vitro and in vivo interactions. Int. J. Biol. Macromol. 248, 125838. doi: 10.1016/j.ijbiomac.2023.125838
Guo, L., Yokoyama, W., Chen, M., Zhong, F. (2021). Konjac glucomannan molecular and rheological properties that delay gastric emptying and improve the regulation of appetite. Food Hydrocoll. 120, 106894. doi: 10.1016/j.foodhyd.2021.106894
Halahlah, A., Piironen, V., Mikkonen, K. S., Ho, T. M. (2023). Polysaccharides as wall materials in spray-dried microencapsulation of bioactive compounds: Physicochemical properties and characterization. Crit. Rev. Food Sci. Nutr. 63, 6983–7015. doi: 10.1080/10408398.2022.2038080
Handa, H., Saitoh, T., Murakami, H. (2015). Immunomodulatory effects of lenalidomide. Nihon Rinsho. 73, 156–161.
Hasbay, İ. (2019). “Dietary fiber and nutrition,” in Dietary fiber: properties, recovery, and applications (Netherlands: Elsevier), 79–123.
Hayeeawaema, F., Wichienchot, S., Khuituan, P. (2020). Amelioration of gut dysbiosis and gastrointestinal motility by konjac oligo-glucomannan on loperamide-induced constipation in mice. Nutrition. 73, 110715. doi: 10.1016/j.nut.2019.110715
He, Y., Liu, Y., Zhang, M. (2024). Hemicellulose and unlocking potential for sustainable applications in biomedical, packaging, and material sciences: A narrative review. Int. J. Biol. Macromol. 280, 135657. doi: 10.1016/j.ijbiomac.2024.135657
Ho, H. V. T., Jovanovski, E., Zurbau, A., Blanco Mejia, S., Sievenpiper, J. L., Au-Yeung, F., et al. (2017). A systematic review and meta-analysis of randomized controlled trials of the effect of konjac glucomannan, a viscous soluble fiber, on LDL cholesterol and the new lipid targets non-HDL cholesterol and apolipoprotein B. Am. J. Clin. Nutr. 105, 1239–1247. doi: 10.3945/ajcn.116.142158
Islam, F., Labib, R. K., Zehravi, M., Lami, M. S., Das, R., Singh, L. P., et al. (2023). Genus amorphophallus: A comprehensive overview on phytochemistry, ethnomedicinal uses, and pharmacological activities. Plants. 12, 3945. doi: 10.3390/plants12233945
Jian, X., Jian, S., Deng, B. (2024). Konjac Glucomannan: A functional food additive for preventing metabolic syndrome. J. Funct. Foods. 115, 106108. doi: 10.1016/j.jff.2024.106108
Jian, W., Wu, H., Wu, L., Wu, Y., Jia, L., Pang, J., et al. (2016). Effect of molecular characteristics of Konjac glucomannan on gelling and rheological properties of Tilapia myofibrillar protein. Carbohydr Polym. 150, 21–31. doi: 10.1016/j.carbpol.2016.05.001
Jin, H., Wang, S., Sheng, J., Yang, X., Li, J., Li, B. (2025). Konjac glucomannan and its degradation products inhibit intestinal lipid absorption by regulating gut microbiota and the production of short-chain fatty acids. J. Agric. Food Chem. 73 (2), 1203–1218. doi: 10.1021/acs.jafc.4c06280
Jin, H., Yao, L., Wang, S., Xia, P., Hou, T., Li, B., et al. (2024). Effects of KGM and degradation products on appetite regulation and energy expenditure in high-fat-diet mice via the adipocyte–hypothalamus axis. J. Agric. Food Chem. 72, 15765–15777. doi: 10.1021/acs.jafc.4c03819
Kalra, S., Aggarwal, S., Khandelwal, D. (2021). Thyroid dysfunction and dysmetabolic syndrome: the need for enhanced thyrovigilance strategies. Int. J. Endocrinol. 2021, 1–11. doi: 10.1155/2021/9641846
Kapoor, D. U., Sharma, H., Maheshwari, R., Pareek, A., Gaur, M., Prajapati, B. G., et al. (2024). Konjac glucomannan: A comprehensive review of its extraction, health benefits, and pharmaceutical applications. Carbohydr Polym. 339, 122266. doi: 10.1016/j.carbpol.2024.122266
Khodadadi Yazdi, M., Taghizadeh, A., Taghizadeh, M., Stadler, F. J., Farokhi, M., Mottaghitalab, F., et al. (2020). Agarose-based biomaterials for advanced drug delivery. J. Controlled Release. 326, 523–543. doi: 10.1016/j.jconrel.2020.07.028
Koncz, D., Tóth, B., Roza, O., Csupor, D. (2021). A systematic review of the european rapid alert system for food and feed: tendencies in illegal food supplements for weight loss. Front. Pharmacol. 11, 611361. doi: 10.3389/fphar.2020.611361
Koyama, T. (2017). 18. Bioactive foods and herbs in prevention and treatment of cardiovascular disease. In: Handbook of nutrition in heart health, vol 14. (Brill), 373–398. doi: 10.3920/978-90-8686-853-7_18
Kulkarni, D., Agnihotri, V., Bhinge, S., Ban, M., Bari, D., Pardeshi, C. V. (2024). Konjac glucomannan: A functional biopolymer for multifaceted drug delivery applications. Polym Adv. Technol. 35, 119–141. doi: 10.1002/pat.v35.7
Lafarge, C., Cayot, N. (2018). Potential use of mixed gels from konjac glucomannan and native starch for encapsulation and delivery of aroma compounds: A review. Starch - Stärke 70 (9–10), 1700159. doi: 10.1002/star.201700159
Lasrado, L. D., Rai, A. K. (2022). Use of prebiotics for addressing gut dysbiosis and achieving healthy gut–brain axis. In: Chopra, K., Bishnoi, M., Kondepudi, K. K. (eds) Probiotic Research in Therapeutics. (Singapore: Springer), 5, 207–239. doi: 10.1007/978-981-16-8444-9_11
Li, Y., Liang, S., Shao, Y., Li, Y., Chen, C., You, C., et al. (2021). Impacts of dietary konjac glucomannan supplementation on growth, antioxidant capacity, hepatic lipid metabolism and inflammatory response in golden pompano (Trachinotus ovatus) fed a high fat diet. Aquaculture. 545, 737113. doi: 10.1016/j.aquaculture.2021.737113
Li, Y., Lu, H., Liao, C., Liu, X. (2024). Oxidized konjac glucomannan: A safe dietary fiber influencing mouse gut microbiota. Food Chem. X. 21, 101089. doi: 10.1016/j.fochx.2023.101089
Li, W., Xu, P., Qian, C., Zhao, X., Xu, H., Li, K. (2024). The combined analysis of the transcriptome and metabolome revealed the possible mechanism of flower bud formation in amorphophallus bulbifer. Agronomy. 14, 519. doi: 10.3390/agronomy14030519
Lin, D., Rezaei, M. J. (2024). Plant polysaccharides and antioxidant benefits for exercise performance and gut health: from molecular pathways to clinic. Mol. Cell Biochem. doi: 10.1007/s11010-024-05178-8
Lin, Y., Zhang, L., Li, X., Zhai, C., Liu, J., Zhang, R. (2024). Effect and characterization of konjac glucomannan on xanthan gum/κ-carrageenan/agar system. Int. J. Biol. Macromol. 257, 128639. doi: 10.1016/j.ijbiomac.2023.128639
Ling, L., Hua, D. R., Chen, N., Pan, J., Pang, J. (2013). Review of konjac glucomannan: isolation, structure, chain conformation and bioactivities. J. Single Molecule Res. 1, 7. doi: 10.12966/jsmr.07.03.2013
Liu, Q., Fang, J., Huang, W., Liu, S., Zhang, X., Gong, G., et al. (2023). The intervention effects of konjac glucomannan with different molecular weights on high-fat and high-fructose diet-fed obese mice based on the regulation of gut microbiota. Food Res. Int. 165, 112498. doi: 10.1016/j.foodres.2023.112498
Liu, Z., Ren, X., Cheng, Y., Zhao, G., Zhou, Y. (2021a). Gelation mechanism of alkali induced heat-set konjac glucomannan gel. Trends Food Sci. Technol. 116, 244–254. doi: 10.1016/j.tifs.2021.07.025
Liu, Z., Ren, X., Cheng, Y., Zhao, G., Zhou, Y. (2021b). Gelation mechanism of alkali induced heat-set konjac glucomannan gel. Trends Food Sci. Technol. 116, 244–254. doi: 10.1016/j.tifs.2021.07.025
Liu, F., Wan, H., Fan, H., Zhang, Z., Dai, H., He, H. (2025). Complexation of starch and konjac glucomannan during screw extrusion exhibits obesity-reducing effects by modulating the intestinal microbiome and its metabolites. Food Funct. 16, 232–248. doi: 10.1039/D4FO04275A
Luo, C., Luo, S., Chen, Z., Yang, R., He, X., Chu, H., et al. (2024). Genome-wide analysis of the Amorphophallus konjac AkCSLA gene family and its functional characterization in drought tolerance of transgenic arabidopsis. BMC Plant Biol. 24, 1033. doi: 10.1186/s12870-024-05747-5
Madawi, E. A., Manaa, H. M., Alattrach, D. G., Al Mogharbel, Z. A., Hussain, Z., Ahmed, I. S. (2024). Dry emulsions as a promising adaptation in pharmaceutical dosage formulations: A review of recent developments and biopharmaceutical significance. J. Drug Delivery Sci. Technol. 96, 105712. doi: 10.1016/j.jddst.2024.105712
Mah, E., Chen, O., Liska, D. J., Blumberg, J. B. (2022). Dietary supplements for weight management: A narrative review of safety and metabolic health benefits. Nutrients. 14, 1787. doi: 10.3390/nu14091787
Mao, Y. H., Xu, Y., Song, F., Wang, Z. M., Li, Y. H., Zhao, M., et al. (2022). Protective effects of konjac glucomannan on gut microbiome with antibiotic perturbation in mice. Carbohydr Polym. 290, 119476. doi: 10.1016/j.carbpol.2022.119476
Mathura, S. R., Landázuri, A. C., Mathura, F., Andrade Sosa, A. G., Orejuela-Escobar, L. M. (2024). Hemicelluloses from bioresidues and their applications in the food industry – towards an advanced bioeconomy and a sustainable global value chain of chemicals and materials. Sustain. Food Technology. 2, 1183–1205. doi: 10.1039/D4FB00035H
Mekkerdchoo, O., Borompichaichartkul, C., Perrigo, A., Srzednicki, G., Prakitchaiwattana, C., Antonelli, A. (2016). Tracing the Evolution and Economic Potential of Konjac Glucomannan in Amorphophallus species (Araceae) using Molecular Phylogeny and RAPD Markers. Phytotaxa. 282 (2), 81–106. doi: 10.11646/phytotaxa.282.2.1
Misra, B. B., Langefeld, C., Olivier, M., Cox, L. A. (2019). Integrated omics: tools, advances and future approaches. J. Mol. Endocrinol. 62, R21–R45. doi: 10.1530/JME-18-0055
Ngondi, J. L., Oben, J. E., Minka, S. R. (2005). The effect of Irvingia Gabonensis seeds on body weight and blood lipids of obese subjects in Cameroon. Lipids Health Dis. 4, 12. doi: 10.1186/1476-511X-4-12
Ni, Y., Liu, Y., Zhang, W., Shi, S., Zhu, W., Wang, R., et al. (2021). Advanced konjac glucomannan-based films in food packaging: Classification, preparation, formation mechanism and function. LWT. 152, 112338. doi: 10.1016/j.lwt.2021.112338
Nie, Y., Luo, F. (2021). Dietary fiber: an opportunity for a global control of hyperlipidemia. Oxid. Med. Cell Longev. 8, 5542342. doi: 10.1155/2021/5542342
Niu, L., Xia, T., Ren, Z., Tang, Z., Cao, Z., Jia, B., et al. (2024). Growth Promoting Characteristics of Bacillus amyloliquefaciens GZA69 and Its Biocontrol Potential Against Soft Rot of Amorphophallus konjac. J. Biobased Mater Bioenergy. 18, 819–826. doi: 10.1166/jbmb.2024.2442
Nurshanti, D. F., Lakitan, B., Hasmeda, M., Ferlinahayati, F. (2023). Shoot emergence, leaf expansion, and corm growth in amorphophallus muelleri blume treated with hydropriming and shading. AGRIVITA J. Agric. Sci. 45 (1), 98–109. doi: 10.17503/agrivita.v45i1.3837
Onitake, T., Ueno, Y., Tanaka, S., Sagami, S., Hayashi, R., Nagai, K., et al. (2015). Pulverized konjac glucomannan ameliorates oxazolone-induced colitis in mice. Eur. J. Nutr. 54, 959–969. doi: 10.1007/s00394-014-0772-2
Pan, X., Zong, Q., Liu, C., Wu, H., Fu, B., Wang, Y., et al. (2024). Konjac glucomannan exerts regulatory effects on macrophages and its applications in biomedical engineering. Carbohydr Polym. 345, 122571. doi: 10.1016/j.carbpol.2024.122571
Pedrosa L de, F., Fabi, J. P. (2024). Polysaccharides from medicinal plants: bridging ancestral knowledge with contemporary science. Plants. 13, 1721. doi: 10.3390/plants13131721
Qi, C., Chen, L. (2024). Progress of ligand-modified agarose microspheres for protein isolation and purification. Microchimica Acta 191, 149. doi: 10.1007/s00604-024-06224-4
Qi, Y., Gao, P., Yang, S., Li, L., Ke, Y., Zhao, Y., et al. (2024). Unveiling the impact of nitrogen deficiency on alkaloid synthesis in konjac corms (Amorphophallus muelleri Blume). BMC Plant Biol. 24, 923. doi: 10.1186/s12870-024-05642-z
Qin, Y., Yan, Z., Gu, H., Wang, Z., Jiang, X., Chen, Z., et al. (2019). Effects of different shading rates on the photosynthesis and corm weight of konjac plant. Not Bot. Horti Agrobot Cluj Napoca 47 (3), 716–721. doi: 10.15835/nbha47311437
Rahman, W., Cahyaningsih, R., Herawati, H., Aminah, A., Risliawati, A., Diantina, S., et al. (2024). Harnessing plant genetic diversity in research on industrial crop plants for environmental conservation concerns. In: Kumar, N. (ed) Industrial Crop Plants. Interdisciplinary Biotechnological Advances. (Singapore: Springer), 293–313. doi: 10.1007/978-981-97-1003-4_11
Reimer, R. A., Yamaguchi, H., Eller, L. K., Lyon, M. R., Gahler, R. J., Kacinik, V., et al. (2013). Changes in visceral adiposity and serum cholesterol with a novel viscous polysaccharide in Japanese adults with abdominal obesity. Obesity. 21 (9), E379–87. doi: 10.1002/oby.20435
Santos, R. D., Nasir, K., Blumenthal, R. S. (2011). “LDL targeted therapies,” in Asymptomatic atherosclerosis. Totowa (Humana Press;, NJ), 605–619.
Sawai, S., Mohktar, M. S., Safwani, W. K. Z. W., Ramasamy, T. S. (2019). Suppression of the viability and proliferation of hepG2 hepatocellular carcinoma cell line by konjac glucomannan. Anticancer Agents Med. Chem. 18, 1258–1266. doi: 10.2174/1871520618666180307143229
Sengupta, P., Tiwari, N., Bhatt, T., Paul, A. T. (2022). Mechanistically acting anti-obesity compositions/formulations of natural origin: a patent review (2010–2021). Expert Opin. Ther. Pat. 32, 29–46. doi: 10.1080/13543776.2021.1954161
Shah, B. R., Li, B., Al Sabbah, H., Xu, W., Mráz, J. (2020). Effects of prebiotic dietary fibers and probiotics on human health: With special focus on recent advancement in their encapsulated formulations. Trends Food Sci. Technol. 102, 178–192. doi: 10.1016/j.tifs.2020.06.010
Shah, B. R., Li, B., Wang, L., Liu, S., Li, Y., Wei, X., et al. (2015a). Health benefits of konjac glucomannan with special focus on diabetes. Bioactive Carbohydrates Dietary Fibre. 5, 179–187. doi: 10.1016/j.bcdf.2015.03.007
Shah, B. R., Li, B., Wang, L., Liu, S., Li, Y., Wei, X., et al. (2015b). Health benefits of konjac glucomannan with special focus on diabetes. Bioactive Carbohydrates Dietary Fibre. 5, 179–187. doi: 10.1016/j.bcdf.2015.03.007
Shang, L., Wang, Y., Ren, Y., Ai, T., Zhou, P., Hu, L., et al. (2020). In vitro gastric emptying characteristics of konjac glucomannan with different viscosity and its effects on appetite regulation. Food Funct. 11, 7596–7610. doi: 10.1039/D0FO01104E
Shen, C., Li, X., Qin, J., Duan, L. (2024). Characterization of miRNA profiling in konjac-derived exosome-like nanoparticles and elucidation of their multifaceted roles in human health. Front. Plant Sci. 15, 1444683. doi: 10.3389/fpls.2024.1444683
Shen, J., Li, Y., Yong, D., Tang, Y., Wang, Y. (2024). Research and functionalization of konjac glucomannan and its hydrogel in wound dressing. J. Polymer Eng. 2024 (10), 58–72. doi: 10.1515/polyeng-2024-0132
Shi, X. D., Yin, J. Y., Zhang, L. J., Huang, X. J., Nie, S. P. (2019). Studies on O-acetyl-glucomannans from Amorphophallus species: Comparison of physicochemical properties and primary structures. Food Hydrocoll. 89, 503–511. doi: 10.1016/j.foodhyd.2018.11.013
Song, H., Guo, R., Sun, X., Kou, Y., Ma, X., Chen, Y., et al. (2024). Integrated metabolomics and transcriptomics revealed the anti-constipation mechanisms of xylooligosaccharides from corn cobs. Food Funct. 15, 894–905. doi: 10.1039/D3FO04366E
Spirk, S. (2018). Polysaccharides in batteries. (Cham: Springer), 1, 9–57. doi: 10.1007/978-3-319-65969-5
Srividya, N., Haldipur, A. C., Yerra, H. (2024). Lifestyle modifications and nutritional modulation of immune system for prevention and management of diabetes mellitus: Current perspectives. In: Biochem. Immunol. Diabetes Associated Complications. Elsevier, 313–330. doi: 10.1016/B978-0-443-13195-0.00016-8
Sun, Y., Xu, X., Wu, Z., Zhou, H., Xie, X., Zhang, Q., et al. (2023). Structure, merits, gel formation, gel preparation and functions of konjac glucomannan and its application in aquatic food preservation. Foods. 12, 1215. doi: 10.3390/foods12061215
Sun, Y., Xu, X., Zhang, Q., Zhang, D., Xie, X., Zhou, H., et al. (2023). Review of konjac glucomannan structure, properties, gelation mechanism, and application in medical biology. Polymers (Basel). 15 (8), 1852. doi: 10.3390/polym15081852
Suzuki, H., Oomizu, S., Yanase, Y., Onishi, N., Uchida, K., Mihara, S., et al. (2010). Hydrolyzed konjac glucomannan suppresses igE production in mice B cells. Int. Arch. Allergy Immunol. 152, 122–130. doi: 10.1159/000265533
Tian, Y., Li, C., Xue, W., Huang, L., Wang, Z. (2023). Natural immunomodulating substances used for alleviating food allergy. Crit. Rev. Food Sci. Nutr. 63, 2407–2425. doi: 10.1080/10408398.2021.1975257
Tsurumaki, M., Kotake, M., Iwasaki, M., Saito, M., Tanaka, K., Aw, W., et al. (2015). The application of omics technologies in the functional evaluation of inulin and inulin-containing prebiotics dietary supplementation. Nutr. Diabetes. 5, e185–e185. doi: 10.1038/nutd.2015.35
Veerasubramanian, P. K., Thangavel, P., Kannan, R., Chakraborty, S., Ramachandran, B., Suguna, L., et al. (2018). An investigation of konjac glucomannan-keratin hydrogel scaffold loaded with Avena sativa extracts for diabetic wound healing. Colloids Surf B Biointerfaces. 165, 92–102. doi: 10.1016/j.colsurfb.2018.02.022
Vuorio, A., Kuoppala, J., Kovanen, P. T., Humphries, S. E., Tonstad, S., Wiegman, A., et al. (2017). Statins for children with familial hypercholesterolemia. Cochrane Database Syst. Rev. 2017 (7), CD006401. doi: 10.1002/14651858.CD006401.pub4
Wang, H., Li, K. (2021). Research status and prospect in molecular biology of amorphophallus. Medicinal Plant Res. 11 (2), 1–7. doi: 10.5376/mpr.2021.11.0002
Watanabe, M., Risi, R., Masi, D., Caputi, A., Balena, A., Rossini, G., et al. (2020). Current evidence to propose different food supplements for weight loss: A comprehensive review. Nutrients. 12, 2873. doi: 10.3390/nu12092873
Widjanarko, S. B., Mubarok, A. Z., Hermanto, M. B., Nuriyanto, A. F. P., Sianturi, M. T. (2024). Comparative analysis of glucomannan properties from wet and dry-extractions of porang (Amorphophallus muelleri blume) and commercial konjac glucomannan. Trends Sci. 22 (2), 8278. doi: 10.48048/tis.2025.8278
Wilianto, Y. R., Tjahjono, Y., Foe, K., Wijaya, S., Ervina, M., Setiadi, D. A., et al. (2024). A novel konjac rice formula with glucomannan and tapioca starch improve postprandial glycemic response – a randomized single-blind clinical trial. Nutr. Food Sci. 54, 1437–1450. doi: 10.1108/NFS-12-2023-0290
Wu, W. T., Cheng, H. C., Chen, H. L. (2011). Ameliorative effects of konjac glucomannan on human faecal β-glucuronidase activity, secondary bile acid levels and faecal water toxicity towards Caco-2 cells. Br. J. Nutr. 105, 593–600. doi: 10.1017/S0007114510004009
Xia, P., Zheng, Y., Sun, L., Chen, W., Shang, L., Li, J., et al. (2024). Regulation of glycose and lipid metabolism and application based on the colloidal nutrition science properties of konjac glucomannan: A comprehensive review. Carbohydr Polym. 331, 121849. doi: 10.1016/j.carbpol.2024.121849
Xu, Z., Sun, Y., Yang, Y., Ding, J., Pang, J. (2007). Effect of γ-irradiation on some physiochemical properties of konjac glucomannan. Carbohydr Polym. 70, 444–450. doi: 10.1016/j.carbpol.2007.05.011
Xu, C., Yu, C., Yang, S., Deng, L., Zhang, C., Xiang, J., et al. (2023). Effects of physical properties of konjac glucomannan on appetite response of rats. Foods. 12, 743. doi: 10.3390/foods12040743
Xu, R., Zheng, X., Chen, C., Li, M., Li, J., Zhou, H., et al. (2024). Effects of different substrates on the growth and yield of Amorphophallus muelleri. Heliyon. 10, e31501. doi: 10.1016/j.heliyon.2024.e31501
Yan, Q., Wang, W., Fan, Z., Li, B., Wei, Y., Yu, R., et al. (2024). Gut microbes mediate prebiotic-like effects of resistant starch. Food Biosci. 61, 104627. doi: 10.1016/j.fbio.2024.104627
Yang, D., Yuan, Y., Wang, L., Wang, X., Mu, R., Pang, J., et al. (2017). A review on konjac glucomannan gels: microstructure and application. Int. J. Mol. Sci. 18, 2250. doi: 10.3390/ijms18112250
Ye, S., Zongo, A. W. S., Shah, B. R., Li, J., Li, B. (2021). Konjac glucomannan (KGM), deacetylated KGM (Da-KGM), and degraded KGM derivatives: A special focus on colloidal nutrition. J. Agric. Food Chem. 69, 12921–12932. doi: 10.1021/acs.jafc.1c03647
Yu, Y., Shen, M., Song, Q., Xie, J. (2018). Biological activities and pharmaceutical applications of polysaccharide from natural resources: A review. Carbohydr Polym. 183, 91–101. doi: 10.1016/j.carbpol.2017.12.009
Yuan, Y., Hong, X., Mu, R., Gong, J., Wang, L., Huang, R., et al. (2017). Structure and properties of konjac glucomannan/galactoglucomannan nanofiber membrane. Macromol Res. 25, 963–970. doi: 10.1007/s13233-017-5125-6
Zhang, Y., Aldamarany, W. A. S., Deng, L., Zhong, G. (2023). Carbohydrate supplementation retains intestinal barrier and ameliorates bacterial translocation in an antibiotic-induced mouse model. Food Funct. 14, 8186–8200. doi: 10.1039/D3FO01343J
Zhang, C., da, C. J., qing, Y. F. (2014). Konjac glucomannan, a promising polysaccharide for OCDDS. Carbohydr Polym. 104, 175–181. doi: 10.1016/j.carbpol.2013.12.081
Zhang, Y., Huo, W., Hou, J., Liu, L., Yu, X., Xu, L. (2022a). Effects and benefits of orchid mycorrhizal symbionts on dendrobium officinale. Horticulturae. 8 (10), 861. doi: 10.3390/horticulturae8100861
Zhang, Y., Tong, C., Chen, Y., Xia, X., Jiang, S., Qiu, C., et al. (2024). Advances in the construction and application of konjac glucomannan-based delivery systems. Int. J. Biol. Macromol. 262, 129940. doi: 10.1016/j.ijbiomac.2024.129940
Zhang, B., Zhang, M., Tian, J., Zhang, X., Zhang, D., Li, J., et al. (2024). Advances in the regulation of radiation-induced apoptosis by polysaccharides: A review. Int. J. Biol. Macromol. 263, 130173. doi: 10.1016/j.ijbiomac.2024.130173
Zhang, H., Zhang, F., Yuan, R. (2020). Applications of natural polymer-based hydrogels in the food industry. In: Hydrogels Based Natural Polymers. Elsevier;, 357–410. doi: 10.1016/B978-0-12-816421-1.00015-X
Zhang, Y., Zhao, Y., Yang, W., Song, G., Zhong, P., Ren, Y., et al. (2022b). Structural complexity of Konjac glucomannan and its derivatives governs the diversity and outputs of gut microbiota. Carbohydr Polym. 292, 119639. doi: 10.1016/j.carbpol.2022.119639
Zhang, Q., Zhong, D., Ren, Y. Y., kuan, M. Z., Pegg, R. B., Zhong, G. (2021a). Effect of konjac glucomannan on metabolites in the stomach, small intestine and large intestine of constipated mice and prediction of the KEGG pathway. Food Funct. 12, 3044–3056. doi: 10.1039/D0FO02682D
Zhang, Q., Zhong, D., Sun, R., Zhang, Y., Pegg, R. B., Zhong, G. (2021b). Prevention of loperamide induced constipation in mice by KGM and the mechanisms of different gastrointestinal tract microbiota regulation. Carbohydr Polym. 256, 117418. doi: 10.1016/j.carbpol.2020.117418
Zheng, Y., Liu, Q., Luo, H., Zheng, J., Li, W. (2024a). Effect of pretreatment with electron beam irradiation on the deacetylation efficiency of konjac glucomannan and its structural, physicochemical and gel properties. Int. J. Biol. Macromol. 276 (1), 133887. doi: 10.1016/j.ijbiomac.2024.133887
Zheng, Y., Luo, H., Liu, Q., Zheng, J., Li, W. (2024b). Electron beam irradiation-assisted preparation of oxidized konjac glucomannan and its structural and physicochemical properties. Food Hydrocoll. 154, 110088. doi: 10.1016/j.foodhyd.2024.110088
Zhou, N., Zheng, S., Xie, W., Cao, G., Wang, L., Pang, J. (2022). Konjac glucomannan: A review of structure, physicochemical properties, and wound dressing applications. J. Appl. Polym Sci. 139 (11), 51780. doi: 10.1002/app.v139.11
Zhu, F. (2018). Modifications of konjac glucomannan for diverse applications. Food Chem. 256, 419–426. doi: 10.1016/j.foodchem.2018.02.151
Zhu, S., Yang, J., Xia, P., Li, S., Wang, Q., Li, K., et al. (2024). Effects of konjac glucomannan intake patterns on glucose and lipid metabolism of obese mice induced by a high fat diet. Food Funct. 15, 9116–9135. doi: 10.1039/D4FO02442G
Keywords: Amorphophallus konjac, konjac glucomannan, dietary fiber, health benefits, antiinflammatory, multi-omics
Citation: Jain A, Sarsaiya S, Gong Q, Wu Q and Shi J (2025) Amorphophallus konjac: traditional uses, bioactive potential, and emerging health applications. Front. Plant Sci. 16:1530814. doi: 10.3389/fpls.2025.1530814
Received: 19 November 2024; Accepted: 27 January 2025;
Published: 21 February 2025.
Edited by:
Eman A. Mahmoud, Damietta University, EgyptReviewed by:
Devesh U. Kapoor, Gujarat Technological University, IndiaCopyright © 2025 Jain, Sarsaiya, Gong, Wu and Shi. This is an open-access article distributed under the terms of the Creative Commons Attribution License (CC BY). The use, distribution or reproduction in other forums is permitted, provided the original author(s) and the copyright owner(s) are credited and that the original publication in this journal is cited, in accordance with accepted academic practice. No use, distribution or reproduction is permitted which does not comply with these terms.
*Correspondence: Jingshan Shi, c2hpanNAem11LmVkdS5jbg==
†These authors share first authorship
Disclaimer: All claims expressed in this article are solely those of the authors and do not necessarily represent those of their affiliated organizations, or those of the publisher, the editors and the reviewers. Any product that may be evaluated in this article or claim that may be made by its manufacturer is not guaranteed or endorsed by the publisher.
Research integrity at Frontiers
Learn more about the work of our research integrity team to safeguard the quality of each article we publish.