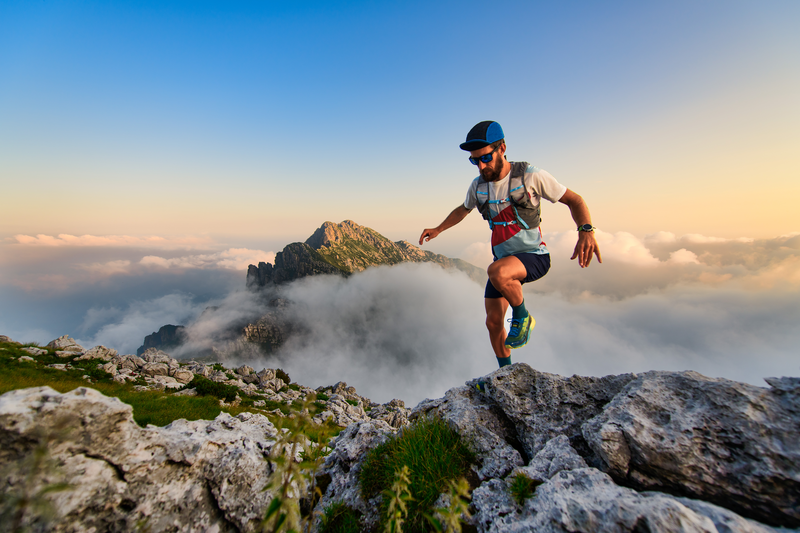
94% of researchers rate our articles as excellent or good
Learn more about the work of our research integrity team to safeguard the quality of each article we publish.
Find out more
ORIGINAL RESEARCH article
Front. Plant Sci.
Sec. Plant Abiotic Stress
Volume 16 - 2025 | doi: 10.3389/fpls.2025.1517434
The final, formatted version of the article will be published soon.
You have multiple emails registered with Frontiers:
Please enter your email address:
If you already have an account, please login
You don't have a Frontiers account ? You can register here
The secondary metabolites of Bupleurum chinense DC. , primarily consist of triterpenoid saikosaponin, which have anti-inflammatory pharmacological effects. Brassinolides (BRs), play a key role in the synthesis and regulation of plant secondary metabolites. However, research on the variation of saikosaponin content in B. chinense treated with different concentrations of BRs solution is still relatively limited. In the present study, experiments were conducted in which different concentrations (0 mg /L as CK, 0.1 mg /L, 0.2 mg /L, and 0.4 mg /L) of BRs solution were sprayed on B. chinense taproot. We measured the growth indicators of each group of B. chinense, used quantitative real-time PCR (qRT-PCR) to determine the expression level of genes related to the biosynthesis of saikosaponin, used terpenoid-targeted metabolomics to determine the accumulation of saikosaponin, and verified the metabolomics results by HPLC. Following a 12-day treatment with the 0.2 mg /L BRs solution, the fresh and dry root weights, the taproot length, and the taproot diameter of B. chinense escalated by 60.35 %, 60.11 %, 25.17 %, and 28.07 % respectively, in comparison with the CK group. The expression of genes related to the biosynthesis of saikosaponin (HMGR, DXR, IPPI, FPS, SE, P450 -2, and P450 -3) significantly increased. Moreover, a terpenoid-targeted metabolomic investigation identified 27 distinct saikosaponins, inclusive of saikosaponin A and D, with a notable accumulation observed in 17 saikosaponins. The HPLC findings indicated that the contents of saikosaponin A and D elevated by 72.64 % and 80.75 % respectively when treated with 0.2 mg /L BRs solution. Conversely, the treatment of 0.4 mg/L BRs solution did not exhibit any significant alteration in the concentrations of saikosaponin A and D when compared to the CK group. These results align with the outcomes from both qRT-PCR and terpene-targeted metabolomic analysis. In conclusion, the 0.2 mg /L BRs solution demonstrates a more pronounced regulatory impact on the synthesis of saikosaponin A and D. Our investigation revealed that the accumulation of these crucial medicinal bioactive compounds, saikosaponin A and D, can be enhanced through the application of a 0.2 mg/L BRs solution in the ecological cultivation.
Keywords: Bupleurum chinense DC., brassinolides, Saikosaponin, quantitative real-time PCR, Terpenoid-targeted metabolomics, Secondary metabolism synthesis
Received: 26 Oct 2024; Accepted: 04 Mar 2025.
Copyright: © 2025 Zhou, Yang, wan, Chen, Gu, Qiao, Chu, Dong, Dong and Feng. This is an open-access article distributed under the terms of the Creative Commons Attribution License (CC BY). The use, distribution or reproduction in other forums is permitted, provided the original author(s) or licensor are credited and that the original publication in this journal is cited, in accordance with accepted academic practice. No use, distribution or reproduction is permitted which does not comply with these terms.
* Correspondence:
Linlin Yang, College of Pharmacy, Henan University of Traditional Chinese Medicine, Zhengzhou, China
Disclaimer: All claims expressed in this article are solely those of the authors and do not necessarily represent those of their affiliated organizations, or those of the publisher, the editors and the reviewers. Any product that may be evaluated in this article or claim that may be made by its manufacturer is not guaranteed or endorsed by the publisher.
Research integrity at Frontiers
Learn more about the work of our research integrity team to safeguard the quality of each article we publish.