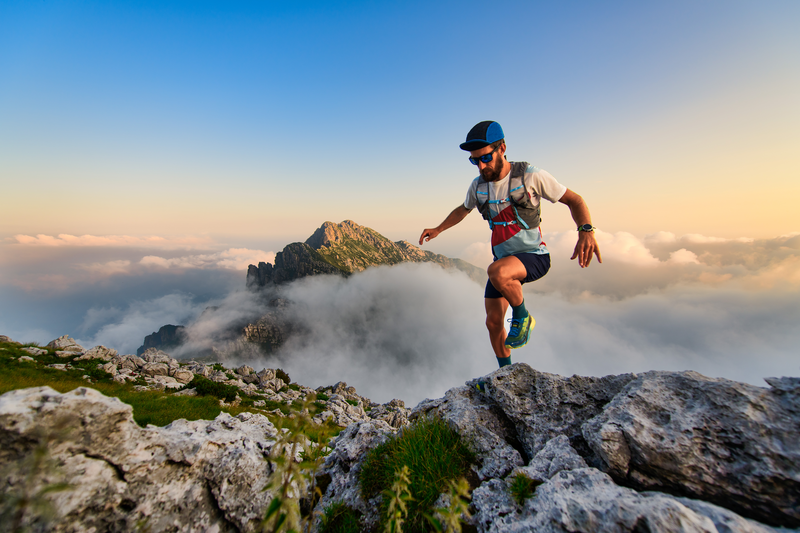
95% of researchers rate our articles as excellent or good
Learn more about the work of our research integrity team to safeguard the quality of each article we publish.
Find out more
ORIGINAL RESEARCH article
Front. Plant Sci. , 21 March 2025
Sec. Plant Pathogen Interactions
Volume 16 - 2025 | https://doi.org/10.3389/fpls.2025.1516644
Fusarium species are widespread pathogens of maize (Zea mays L.), leading to various diseases throughout the plant’s lifecycle, including Fusarium ear rot (FER), a significant disease that impacts both yield and quality. FER begins at the silking stage when Fusarium conidia infect maize silks, particularly in tropical regions where F. verticillioides and F. proliferatum dominate. These pathogens not only lead to economic losses but also produce mycotoxins such as fumonisins, posing significant health risks to humans and animals. This study aimed to identify toxigenic Fusarium species associated with maize ear rot in North India and evaluate their fumonisin production potential under laboratory conditions. Out of the 48 Fusarium isolates collected, 40 amplified VERTF-1/2 primers, 41 amplified the FUM1 gene, while 36 amplified the FUM13 gene, indicating their potential to produce fumonisins. Sequencing analysis revealed that F. verticillioides was the predominant species associated with FER under North Indian conditions, with Fus 48 being identified as F. proliferatum. To assess their fumonisin production potential, Fus 15- the most virulent F. verticillioides isolate along with F. proliferatum isolate- Fus 48 were selected for further analysis. These isolates were artificially inoculated onto maize grains of PMH 1 and PMH 2 hybrids and fumonisin (FB1 and FB2) levels were quantified using liquid chromatography-mass spectrometry (LC-MS/MS). The results revealed that F. verticillioides (Fus 15) exhibited a higher fumonisin production capacity than F. proliferatum (Fus 48), with significantly greater fumonisin accumulation in PMH 2 hybrid. This increased fumonisin production in PMH 2 was positively correlated with FER severity under field conditions. Overall, this study provides critical insights into the prevalence and toxigenic potential of Fusarium species in North India, which could inform future management strategies to mitigate the impact of FER and its associated mycotoxins on maize production.
Fusarium, a ubiquitous pathogen of maize (Zea mays L.), consistently associates with the plant throughout its life cycle, inducing various diseases such as seed rot and seedling blight, root rot, post-flowering stalk rots and ear rots (Singh and Kaur, 2023; Kaur et al., 2016; Munkvold, 2003; Logrieco et al., 2002). Among these, Fusarium ear rot (FER) is particularly damaging, significantly reducing both yield and quality of maize. Globally, FER is estimated to cause economic losses of around 26.7%, with potential losses increasing to 48% under favorable environmental conditions (Vigier et al., 2001; Anonymous, 1988). Beyond yield reductions, FER impairs crop quality by producing various mycotoxins which pose serious health risks to humans and animals. Among these, fumonisins are particularly significant contributing to economic losses of up to 46 million US dollars annually (Wu, 2007). First identified by Gelderblom et al. (1988) in South Africa, fumonisins are primarily produced by Fusarium verticillioides and F. proliferatum under tropical conditions (Huffman et al., 2010; Marasas, 2001). Their presence raises major health concerns due to potential links with human cancer, neural tube defects and other birth abnormalities, and its toxicity to domestic livestock (Missmer et al., 2006; Logrieco et al., 2003; Gelderblom et al., 1988). In India, an outbreak of fumonisin toxicosis affecting 10,000 hens was reported in Andhra Pradesh in the last quarter of 1995 (Kumar et al., 1997). To mitigate these losses, it is crucial to detect fumonisins using molecular tools. Fumonisin production is regulated by the “FUM cluster”, a set of 16 co-regulated genes essential for biosynthesis, including the key FUM1 gene, which encodes polyketide synthase. The utilization of molecular markers for identifying toxigenic strains and detecting the FUM genes across species, represents the most effective strategy for elucidating their mycotoxin potential (Patiño et al., 2004; Proctor et al., 1999; Stepien et al., 2011).
The production of fumonisins in standing maize crop is closely linked with FER severity. This disease typically initiates at the silking stage, as airborne conidia of the fungus land on the silks and enter the cob through insect-caused injuries or direct contact with the silks. In addition, systemic infection can occur via contaminated seeds, which leads to early establishment of the pathogen before the silking stage (Foroud et al., 2014). Various Fusarium spp. viz., F. subglutinans, F. graminearum, F. cerealis, F. avenaceum and F. equiseti have been found associated with ear rot throughout the world. However, under tropical environmental conditions, two Fusarium spp. viz., F. verticillioides and F. proliferatum belonging to Fusarium section Lesiola are more predominant (Pfordt et al., 2020). Despite the global significance of these pathogens, knowledge about the prevalence of toxigenic Fusarium species associated with maize ear rot in North India remains limited. This study aimed to bridge this gap by identifying Fusarium species using species-specific and gene-specific primers and evaluating their fumonisin production potential under laboratory conditions. The findings aim to enhance our understanding of the current status of toxigenic Fusarium species and guide future management strategies.
The cob samples showing peculiar symptoms of ear rot were collected from major maize growing areas of Punjab state viz., Gurdaspur, Hoshiarpur, Shaheed Bhagat Singh Nagar, Rupnagar, Jalandhar, Kapurthala, Ludhiana during Spring and Kharif seasons of 2020 as well as from Sirmour district of Himachal Pradesh during Kharif 2021. The isolations were performed on potato dextrose agar (PDA) medium, resulting in the recovery of 48 Fusarium isolates (Fus 1 to Fus 48). The pure cultured isolates were maintained at 4°C for further studies.
For extraction of fungal genomic DNA, the pure cultures of all 48 Fusarium isolates were grown on 100 ml Potato Dextrose Broth (PDB) medium and were incubated at 25 ± 2°C for 10 days. Ten days old fungal mycelial mat obtained through filtering on Whatman filter paper No. 1 was ground to fine powder using liquid nitrogen and transferred into 2 ml eppendorf tube containing 800 µl preheated (65°C) Cetyl trimethyl ammonium bromide (CTAB) extraction buffer and mixed thoroughly with buffer. Fungal DNA from all the isolates was isolated using CTAB method as described by Saghai-Maroof et al. (1984). The extracted DNA was subjected to RNAse (2 µl) treatment and the samples were incubated at 37°C for 45 minutes. Quantity and quality of DNA was checked on Eppendorf Bio Spectrometer and 0.8% agarose gel. The DNA samples were then stored at -20°C for further studies.
Using VERTF-1/2primers: The fumonisin producing F. verticillioides isolates were distinguished from non-producers using a set of VERTF-1/2 primers (F- GCGGGAATTCAAAAGTGGCC, R- GAGGGCGCGAAACGGATCGG) as described by Patiño et al. (2004). Polymerase chain reaction (PCR) amplification was carried out in an Eppendorf Mastercycler Pro S (Eppendorf, Germany) using the same amplification profile as stated by Patiño et al. (2004). PCR analysis was carried out in the reaction volume of 25 µl/sample containing-0.4 µl of GoTaq™ DNA polymerase (Promega Inc.), 5 µl of 1X GoTaq™ Reaction Buffer (Green), 1.5 µl of 2.5 mM MgCl2 (Promega Inc.), 1.5 µl each of 10 pmol forward/reverse primers, 0.5 µl of 0.1 mM dNTPs (Promega Inc.), 2 µl of template DNA and 12.60 µl of nuclease free water (Promega Inc.).
Using FUM gene specific primers: The ability of Fusarium isolates to produce fumonisins was studied by amplification and sequencing of segments of two FUM genes namely- FUM1 and FUM13. Both these genes were amplified using sets of FUM1F1/R2 (F-CACATCTGTGGGCGATCC, R-ATATGGCCCCAGCTGCATA) (Stepien et al., 2011), and FUM13F/13R (F-AGTCGGGTCAAGAGCTTGT, R-TGCTGAGCCGACATCATAATC) (Ramana et al., 2011), respectively. In vitro amplification was performed in an Eppendrof master cycler Pro S (Eppendorf, Germany) using FUM1 and FUM13 gene specific primers. PCR reaction mixture consisted of 25 µl volume/sample as mentioned above. The amplification of FUM1 gene was performed with one cycle of initial denaturation at 94°C for 5 minutes followed by 35 cycles of denaturation at 94°C for 45 seconds, annealing at 55°C for 45 seconds, extension at 72°C for 2 minutes with one cycle of final extension at 72°C for 7 minutes. The FUM13 gene was amplified using same profile as stated by Ramana et al. (2011).
The amplified PCR products were visualized on 1% agarose gel under UV light and photographed using SYNGENE gel documentation system with “GeneSnap” software program. The band size of PCR products was determined by comparing with known marker (100 bp ladder, G Biosciences, USA).
Four representative isolates (Fus 15, Fus 28, Fus 44 and Fus 45) showing positive amplification for both FUM1 and FUM13 genes along with Fus 48 isolate amplifying FUM1 gene were selected for sequencing. The amplified PCR products were purified by using NucleoSpin Gel and PCR Clean-up kit (Macherey-Nagel, Germany), following the manufacturer’s instructions. Around 18 µl of positive purified products (100ng/µl) of these five isolates were sequenced (Barcode Biosciences Bangalore). The retrieved forward and reverse sequences were aligned using BioEdit software, and saved as a single contig file. Contigs were generated and screened against online Gene DNA sequence database using BLASTn (Nucleotide Basic Local Alignment Search Tool) program available at NCBI website (https://blast.ncbi.nlm.nih.gov). Matching sequences were retrieved from BLASTn analysis and further used for phylogenetic analysis or sequence comparison.
Statistical analysis: Multiple sequence alignment (MSA) was performed using Mega X (Kumar et al., 2018) with Neighbor- Joining Tree option. The phylogenetic trees were constructed and the sequences of the identified Fusarium species were submitted to NCBI nucleotide database.
Selection of maize hybrids: Two maize hybrids- PMH 1 and PMH 2 differing in maturity groups as well as response to Fusarium spp. were selected for the quantitative analysis of fumonisins. PMH 1, late-maturity hybrid known for its resistance to F. verticillioides (Kaur et al., 2016), and PMH 2, early-duration hybrid susceptible to FER (Singh and Kaur, 2024), were selected to understand the relationship between FER infection and fumonisin accumulation.
Selection of Fusarium isolates: Among F. verticillioides isolates, the most virulent isolate- Fus 15 and F. proliferatum isolate- Fus 48 showing higher FER severity under artificially inoculated field conditions were selected for quantitative analysis of fumonisins.
Estimation of fumonisins: The fumonisin content of FB1 and FB2 in artificially inoculated maize grains of PMH 1 and PMH 2 was analyzed by using triple quadrupole liquid chromatography mass spectrometer (LC MS MS) on LCMS-MS-8045 (Shimadzu). The control treatment consisted of seed mixed with sterile distilled water. These inoculated grains were incubated at 25 ± 2°C and sampling for quantification of fumonisin production was done at 7, 14, 21 and 28 days after inoculations. Two dilutions of standards FB1 and FB2 (1 ppm and 0.1 ppm) (LGC Standards GmbH, Germany) were prepared with HPLC grade acetonitrile from stock solution for constructing a calibration curve. The sample preparation for fumonisins estimation was done as stated by Li et al. (2012). Recovery experiments were carried out to know the efficacy of the analytical method used and samples (5 g) of healthy maize grains were fortified with fumonisin B1 and B2 at level of 10, 50 and 100 µg kg-1 with three replications of each. The sample was processed and per cent recovery was calculated. The chromatographic separation of analytes was done using C18 (2.1 ×150 mm) 2μm (HSS) column, in positive ion mode using multiple reaction monitoring (MRM) of the transitions m/z (Table 1). The mobile phase of 10 mM ammonium formate+0.1% formic acid in water: 0.1% formic acid in methanol (A: B 10: 90 v/v) was used with flow rate of 250 µL/min. MS conditions were: column temperature 40°C, interface temperature 250°C, desolvation temperature 444°C, DL temperature 250°C, heat block temperature 300°C, nebulizing gas flow rate at 2.5 L/min, heating gas flow rate at 5 L/min and drying gas flow rate at 5 L/min. The precursor ions m/z and collision energy for each of the compound is given in Table 1. Lab solutions software was used to acquire and analyze the data. The fumonisins content were estimated by comparing peak height/peak area of the standard with that of unknown or spiked samples ran under identical conditions. The data was analyzed for mean ± standard error for both fumonisins-FB1 and FB2.
Out of 48 Fusarium isolates collected from maize ear rot samples, 40 showed a successful amplification with VERTF-1/2 primers, producing a 400 bp band, confirming them as fumonisin-producing F. verticillioides strains (Figure 1). Additionally, FUM1 gene specific primers amplified a band of 1126 bp in 41 isolates (Figure 2). Moreover, FUM13 specific primer produced a band of 998 bp in 36 Fusarium isolates, and the rest of 12 isolates lacking this gene did not show any amplification (Figure 3). Data in Table 2 showed that five isolates (Fus 8, Fus 18, Fus 20, Fus 24 and Fus 40) did not show any amplification with VERTF-1/2 primers, FUM1 and FUM13 genes. Only six isolates viz., Fus 12, Fus 16, Fus 17, Fus 21, Fus 26 and Fus 30 showed amplification with VERTF-1/2 primers and FUM1 gene and only two isolates viz. Fus 13 and Fus 25 gave amplification with FUM13F/13R primers. One F. proliferatum isolate -Fus 48 showed amplification only with FUM1F1/R2 primer; there was no amplification with VERTF-1/VERTF-2 and FUM13F/13R primers (Table 2).
Figure 1. Amplification of fumonisin producing F. verticillioides isolates using VERTF-1/2 primers showing amplification band of 400 bp, Lane M contains- 100 bp ladder; Lane 1-48 contains DNA from Fusarium isolates Fus 1 to Fus 48 from different locations.
Figure 2. Amplification of fumonisin producing Fusarium isolates using FUM1 gene specific primers showing amplification band of 1126 bp, Lane M contains- 100 bp ladder; Lane 1-48 contains DNA from Fusarium isolates Fus 1 to Fus 48 from different locations.
Figure 3. Amplification of fumonisin producing Fusarium isolates using FUM13 gene specific primers showing amplification band of 998 bp, Lane M contains- 100 bp ladder; Lane 1-48 contains DNA from Fusarium isolates Fus 1 to Fus 48 from different locations.
The sequences of FUM1 gene for Fus 15, Fus 28, Fus 44 and Fus 45 submitted to BLAST revealed 99-100% similarity with F. verticillioides (having reference id XM_018886754.1). However, Fus 48 had shown 98% similarity with F. proliferatum (reference id CP128308.1) when subjected to BLAST. The FUM13 gene sequences of Fus 15, Fus 28, Fus 44 and Fus 45 isolates showed 99-100% similarity with F. verticillioides (reference id XM_018886761.1). The phylogenetic analysis of Fusarium isolates for both FUM1 (5 isolates) and FUM13 (4 isolates) genes resulted in 99-100% similarity with respective species (Supplementary Figures S4, S5). The above studies confirmed that FUM1 and FUM13 genes were present in 85.41% and 75% of the isolates, respectively. Since these genes are essential for fumonisin biosynthesis, their presence suggests a potential for toxin production. Furthermore, to assess and confirm the mycotoxin production potential of these isolates fumonisin content was analyzed using LC MS MS method.
Recovery of fumonisins: The average recoveries of FB1 from maize grains fortified at 10, 50, and 100 µg/kg were 75.67%, 90.63%, and 85.14%, respectively. For FB2, the recoveries were 73.01%, 98.40%, and 82.04% at the same fortification levels (Table 3). The control samples from untreated grains and reagent blanks were also processed in the same way so as to find out the interferences, if any, due to the substrate and reagents, respectively and no interferences were observed at the retention time (RT) of FB1 and FB2 (Figure 4). The recoveries obtained were within the international acceptable levels. The limit of quantitation (LOQ) of the method was 10 µg kg-1 (Figure 5). The linearity curves for both the fumonisins standards (FB1 and FB2) were constructed by injecting 10, 20, 30, 40 and 50 ng/ml of each of the standard and the response was found to be linear with R2 values of 0.9935 and 0.9951 for FB1 and FB2, respectively (Figures 6, 7).
Fumonisin content in maize samples: The data presented in Table 4 and Table 5 revealed that FB1 and FB2 were produced by both the isolates of Fusarium species. When comparing the isolates, F. verticillioides isolate (Fus 15) produced greater quantity of fumonisins than F. proliferatum isolate (Fus 48) across all four sampling intervals. Seven days after inoculation, Fus 15 isolate produced lower concentration of FB1 in the PMH 1 (0.03 mg kg-1) and PMH 2 (1.06 mg kg-1) hybrids. By 21 days after inoculation, FB1 levels rose to 17.29 mg kg-1 in PMH 1 and 96.93 mg kg-1 in PMH 2 (Table 4) (Supplementary Figure S6). A similar trend was observed with Fus 48 isolate. The perusal of data presented in Table 5 revealed that at seven days after inoculation, Fus 15 isolate produced FB2 only in PMH 2 grains (0.22 mg kg-1), however its quantity in PMH 1 hybrid was below LOQ. Upto 14 days after inoculation, FB2 production by Fus 48 isolate was below LOQ in both hybrids.
Table 4. Fumonisin FB1 (mg kg-1) production in maize grains artificial inoculated with Fusarium isolates.
Table 5. Fumonisin FB2 (mg kg-1) production in maize grains artificial inoculated with Fusarium isolates.
PMH 2 consistently exhibited higher levels of fumonisins (FB1 and FB2) than PMH 1 across all sampling days. In both hybrids, fumonisin levels gradually increased with inoculation time, reaching their peak at 21 days, with PMH 2 showing the highest FB1 (96.93 mg/kg) and FB2 (22.61 mg/kg) accumulation.
The study explored the fumonisin production potential of Fusarium species, particularly focusing on F. verticillioides and F. proliferatum. The results demonstrated that the majority of F. verticillioides isolates (85.10%) were fumonisin producers, as confirmed by VERTF-1/2 primers. These findings align with previous studies, such as those by Magculia and Cumagun (2011), who found that 38 out of 49 F. verticillioides isolates were potential fumonisin producers based on the amplification of a 400 bp DNA fragment specific to these primers. Similarly, Maheshwar et al. (2009) and Szecsi et al. (2011) identified fumonisin production in F. verticillioides using species-specific primers, further corroborating the utility of VERTF-1/2 in detecting toxigenic strains.
The fumonisin biosynthesis pathway, driven by the FUM gene cluster, plays a crucial role in determining the toxigenic potential of Fusarium species (Proctor et al., 2003; Sánchez-Rangel et al., 2005; Niehaus et al., 2017; Choi et al., 2018; Zyl et al., 2019; Mohiddin et al., 2021). The study’s focus on FUM1 and FUM13 genes, which are vital for fumonisin production, is consistent with findings from previous research (Qiu et al., 2020; Kaur, 2015; Proctor et al., 2013; Medina et al., 2013; Ramana et al., 2011). Notably, the presence of these genes was also detected in other Fusarium species within the FFSC, such as F. proliferatum and F. fujikuroi, as reported by Choi et al. (2018). However, it is important to note that the detection of the FUM gene cluster does not always correlate with fumonisin production, as some non-detectable fumonisin-producing strains also harbor these genes (Sultana et al., 2019). Variations in fumonisin production among Fusarium species and even among different isolates of the same species have been attributed to factors such as pathogen strain differences, environmental conditions, varietal susceptibility and the origin of the species (Singh and Kaur, 2024; Kamle et al., 2019). In this study, F. verticillioides exhibited a higher fumonisin production potential than F. proliferatum, which is consistent with previous studies (Ismail et al., 2017; Ghiasian et al., 2005; Tancic et al., 2012; Acuna et al., 2005; Logrieco et al., 2002; Covarelli et al., 2012). The study also observed that fumonisin B1 production increased with inoculation time, a trend that has been documented in several studies (Altinok, 2009; Dilkin et al., 2002; Bailly et al., 2002).
Resistance to FER is polygenic in nature and no maize cultivar has been reported to exhibit complete resistance. Furthermore, no information is available regarding FER resistance in maize under North Indian conditions. Based on our previous studies in north zone (Kaur et al., 2016; Singh and Kaur, 2024) hybrids showed varied response against F. verticillioides infection. Two hybrids- PMH 1 exhibiting resistance while PMH 2 showing susceptibility to F. verticillioides were further selected for fumonisin analysis. The PMH 1 hybrid exhibited lower fumonisin accumulation compared to the PMH 2 hybrid, suggesting a potential resistance mechanism against fumonisin production in PMH 1. This finding aligns with previous reports that have shown a positive correlation between FER severity and fumonisin concentration (Junior et al., 2019; Netshifhefhe et al., 2018; Maschietto et al., 2017; Balconi et al., 2014; Afolabi et al., 2007).
The study reinforces the role of molecular techniques, particularly the use of species-specific primers and the analysis of the FUM gene cluster, in differentiating between toxigenic and non-toxigenic Fusarium strains. The results also underscore the variability in fumonisin production among Fusarium species and isolates, influenced by genetic and environmental factors. The observed correlation between FER severity and fumonisin concentration highlights the importance of developing resistant maize hybrids to mitigate the risks associated with fumonisin contamination.
The datasets presented in this study can be found in online repositories. The names of the repository/repositories and accession number(s) can be found in the article/Supplementary Material.
HS: Conceptualization, Formal analysis, Investigation, Methodology, Software, Writing – original draft, Writing – review & editing. HK: Conceptualization, Funding acquisition, Investigation, Methodology, Project administration, Resources, Writing – review & editing. MH: Conceptualization, Formal analysis, Investigation, Methodology, Supervision, Validation, Visualization, Writing – review & editing. SS: Conceptualization, Formal analysis, Investigation, Methodology, Software, Supervision, Writing – review & editing.
The author(s) declare that no financial support was received for the research and/or publication of this article.
The authors declare that they have no known competing financial interests or personal relationships that could have appeared to influence the work reported in this paper.
The author(s) declare that no Generative AI was used in the creation of this manuscript.
All claims expressed in this article are solely those of the authors and do not necessarily represent those of their affiliated organizations, or those of the publisher, the editors and the reviewers. Any product that may be evaluated in this article, or claim that may be made by its manufacturer, is not guaranteed or endorsed by the publisher.
The Supplementary Material for this article can be found online at: https://www.frontiersin.org/articles/10.3389/fpls.2025.1516644/full#supplementary-material
Acuna, A. M. C., Lozano, M. C., García, D., Diaz, G. J. (2005). Prevalence of Fusarium species of the Liseola section on selected Colombian animal feedstuffs and their ability to produce fumonisins. Mycopathologia 160, 63–66. doi: 10.1007/s11046-005-1590-0
Afolabi, C. G., Ojiambo, P. S., Ekpo, E. J. A., Menkir, A., Bandyopadhyay, R. (2007). Evaluation of maize inbred lines for resistance to Fusarium ear rot and fumonisin accumulation in grain in tropical Africa. Plant Dis. 91, 279–286. doi: 10.1094/PDIS-91-3-0279
Altinok, H. H. (2009). In vitro production of fumonisin B1 and B2 by Fusarium moniliforme and the biocontrol activity of Trichoderma harzianum. Ann. Microbiol. 59, 509–516. doi: 10.1007/BF03175139
Bailly, J. D., Querin, A., Tardieu, D., Guerre, P. (2002). Production and purification of fumonisins from a highly toxigenic Fusarium verticillioides strain. Rev. Méd. Vét. 156, 547–554.
Balconi, C., Berardo, N., Locatelli, S., Lanzanova, C., Torri, A., Redaelli, R. (2014). Evaluation of ear rot (Fusarium verticillioides) resistance and fumonisin accumulation in Italian maize inbred lines. Phytopathol. Mediterr 53, 14–26. doi: jstor.org/stable/43871755
Choi, J., Lee, S., Nah, J., Kim, H., Paek, J., Lee, S., et al. (2018). Species composition of and fumonisin production by the Fusarium fujikuroi species complex isolated from Korean cereals. Int. J. Food Microbiol. 267, 62–69. doi: 10.1016/j.ijfoodmicro.2017.12.006
Covarelli, L., Stifano, S., Beccari, G., Raggi, L., Lattanzio, V. M. T., Albertini, E. (2012). Characterization of Fusarium verticillioides strains isolated from maize in Italy: Fumonisin production, pathogenicity, and genetic variability. Food Microbiol. 31, 17–24. doi: 10.1016/j.fm.2012.02.002
Dilkin, P., Mallmann, C. A., Almeida, C. A. A., Stefanon, E. B., Fontana, F. Z., Milbradt, E. L. (2002). Production of fumonisins by strains of Fusarium moniliforme according to temperature, moisture, and growth period. Braz. J. Microbiol. 33, 111–118. doi: 10.1590/S1517-83822002000200003
Foroud, N. A., Chatterton, S., Reid, L. M., Turkington, T. K., Tittlemier, S. A., Gräfenhan, T. (2014). “Fusarium diseases of Canadian grain crops: impact and disease management strategies,” in Future Challenges in Crop Protection Against Fungal Pathogens. Eds. Goyal, A., Manoharachary, C. (Springer, New York, NY), 267–316. doi: 10.3389/fmicb.2018.01909
Gelderblom, W. C. A., Jaskiewicz, K., Marasas, W. F. O., Thiel, P. G., Horak, R. M., Vlleggaar, R., et al. (1988). Fumonisins: Novel mycotoxins with cancer-promoting activity produced by Fusarium moniliforme. Appl. Environ. Microbiol. 54, 1806–1811. doi: 10.1128/aem.54.7.1806-1811.1988
Ghiasian, S. A., Rezayat, S. M., Kord-Bacheh, P. (2005). Fumonisin production by Fusarium species isolated from freshly harvested corn in Iran. Mycopathologia 159, 31–40. doi: 10.1007/s11046-004-3899-5
Huffman, J., Gerber, R., Du, L. (2010). Recent advancements in the biosynthetic mechanisms for polyketide-derived mycotoxins. Biopolymers 93, 764–776. doi: 10.1002/bip.21483
Ismail, N. A., Mohd, M. H., Nor, N. M. I. M., Zakaria, L. (2017). Fumonisin B1 producing Fusarium species from agricultural crops in Malaysia. Crop Prot. 98, 70–75. doi: 10.1016/j.cropro.2017.03.014
Junior, O. F. R., Dalcin, M. S., Nascimento, V. L., Haesbaert, F. M., Ferreira, T. P. S., Fidelis, R. R., et al. (2019). Fumonisin production by Fusarium verticillioides in maize genotypes cultivated in different environments. Toxins 11, 215–221. doi: 10.3390/toxins11040215
Kamle, M., Mahato, D. K., Devi, S., Lee, K. E., Kang, S. G., Kumar, P. (2019). Fumonisins: Impact on agriculture, food, and human health and their management strategies. Toxins 11, 328. doi: 10.3390/toxins11060328
Kaur, H., Mohan, C., Hunjan, M. (2016). Morphological and pathological characterization of Fusarium verticillioides from different maize growing areas of Punjab. Indian Phytopathol. 69, 190–194.
Kaur, K. (2015). Detection of fumonisins producing Fusarium spp. on maize in Punjab. Punjab Agricultural University, Ludhiana (Punjab.
Kumar, P., Rao, V. S., Paramkishan, R. J., Bhat, R. V. (1997). Disease outbreak in laying hens arising from the consumption of fumonisin-contaminated food. Brit. Poult. Sci. 38, 475–479. doi: 10.1080/00071669708418024
Kumar, S., Stecher, G., Li, M., Knyaz, C., Tamura, K. (2018). MEGA X: Molecular evolutionary genetics analysis across computing platforms. Mol. Biol. Evol. 35, 1547–1549. doi: 10.1093/molbev/msy096
Li, C., Wu, Y., Yang, T., Huang-Fu, W. (2012). Rapid determination of fumonisins B1 and B2 in corn by liquid chromatography–tandem mass spectrometry with ultrasonic extraction. J. Chromatog. Sci. 50, 57–63. doi: 10.1093/chromsci/bmr009
Logrieco, A., Bottalico, A., Mulè, G., Moretti, A., Perrone, G. (2003). Epidemiology of toxigenic fungi and their associated mycotoxins for some Mediterranean crops. Eur. J. Plant Pathol. 109, 645–667. doi: 10.1023/A:1026033021542
Logrieco, A., Mulè, G., Moretti, A., Bottalico, A. (2002). “Toxigenic Fusarium species and mycotoxins associated with maize ear rot in Europe,” in Mycotoxins in Plant Disease. Eds. Logrieco, A., Bailey, J. A., Corazza, L., Cooke, B. M. (Springer, Dordrecht). doi: 10.1007/978-94-010-0001-7_1
Magculia, N. J. F., Cumagun, C. J. R. (2011). Genetic diversity and PCR-based identification of potential fumonisin-producing Fusarium verticillioides isolates infecting corn in the Philippines. Trop. Plant Pathol. 36, 225–232.
Maheshwar, P. K., Moharram, S. A., Janardhana, G. R. (2009). Detection of fumonisin-producing Fusarium verticillioides in paddy (Oryza sativa L.) using polymerase chain reaction (PCR). Braz. J. Microbiol. 40, 134–138. doi: 10.1590/S1517-838220090001000023
Marasas, W. (2001). Discovery and occurrence of the fumonisins: A historical perspective. Environ. Health Perspect. 109, 239–243. doi: 10.1289/ehp.01109s2239
Maschietto, V., Colombi, C., Pirona, R., Pea, G., Strozzi, F., Marocco, A., et al. (2017). QTL mapping and candidate genes for resistance to Fusarium ear rot and fumonisin contamination in maize. BMC Plant Biol. 17, 20. doi: 10.1186/s12870-017-0970-1
Medina, A., Schmidt-Heydt, M., Cárdenas-Chávez, L., Parra, R., Geisen, R., Magan, N. (2013). Integrating toxin gene expression, growth and fumonisin B1 and B2 production by a strain of Fusarium verticillioides under different environmental factors. J. R. Soc Interface 10, 20130320. doi: 10.1098/rsif.2013.0320
Missmer, S. A., Suarez, L., Felkner, M., Wang, E., Merrill, A. H., Rothman, K. J., et al. (2006). Exposure to fumonisins and the occurrence of neural tube defects along the Texas–Mexico border. Environ. Health Perspect. 114, 237–241. doi: 10.1289/ehp.8221
Mohiddin, F. A., Majid, R., Bhat, A., Dar, M. S. (2021). Molecular phylogeny, pathogenic variability and phytohormone production of Fusarium species associated with bakanae disease of rice in temperate agro-ecosystems. Mol. Biol. Rep. 48, 1–12. doi: 10.1007/s11033-021-06337-6
Munkvold, G. P. (2003). Epidemiology of Fusarium diseases and their mycotoxins in maize ears. Eur. J. Plant Pathol. 109, 705–713. doi: 10.1023/A:1026078324268
Netshifhefhe, N. E. I., Flett, B. C., Viljoen, A. (2018). Inheritance and genotype by environment analyses of resistance to Fusarium verticillioides and fumonisin contamination in maize F1 hybrids. Euphytica 214, 235. doi: 10.1007/s10681-018-2310-4
Niehaus, E. M., Kim, H. K., Münsterkötter, M., Janevska, S., Arndt, B., Kalinina, S. A., et al. (2017). Comparative genomics of geographically distant Fusarium fujikuroi isolates revealed two distinct pathotypes correlating with secondary metabolite profiles. PloS Pathog. 13, e1006670. doi: 10.1371/journal.ppat.1006670
Patiño, B., Mirete, S., Gonzalez-Jaen, M. T., Mulè, G., Rodriguez, M. T., Vazquez, C. (2004). PCR detection assay of fumonisin-producing Fusarium verticillioides strains. J. Food Prot. 67, 1278–1283. doi: 10.4315/0362-028x-67.6.1278
Pfordt, A., Romero, R. L., Schiwek, S., Karlovsky, P., von Tiedemann, A. (2020). Impact of environmental conditions and agronomic practices on the prevalence of Fusarium species associated with ear- and stalk rot in maize. Pathogens 9, 236. doi: 10.3390/pathogens9030236
Proctor, R. H., Brown, D. W., Plattner, R. D., Desjardins, A. E. (2003). Co-expression of 15 contiguous genes delineates a fumonisin biosynthetic gene cluster in Gibberella moniliformis. Fungal Genet. Biol. 38, 237–249. doi: 10.1016/s1087-1845(02)00525-x
Proctor, R. H., Desjardins, A. E., Plattner, R. D., Hohn, T. M. (1999). A polyketide synthase gene required for biosynthesis of fumonisin mycotoxins in Gibberella fujikuroi mating population A. Fungal Genet. Biol. 27, 100–112. doi: 10.1006/fgbi.1999.1141
Proctor, R. H., Van Hove, F., Susca, A., Stea, G., Busman, M., van der Lee, T. (2013). Birth, death, and horizontal gene transfer of the fumonisin biosynthetic gene cluster during the evolutionary diversification of Fusarium. Mol. Microbiol. 90, 290–306. doi: 10.1111/mmi.12362
Qiu, J., Lu, Y., He, D., Lee, Y., Ji, F., Xu, J., et al. (2020). Fusarium fujikuroi species complex associated with rice, maize, and soybean from Jiangsu province, China: Phylogenetic, pathogenic, and toxigenic analysis. Plant Dis. 104, 2193–2201. doi: 10.1094/PDIS-09-19-1909-RE
Ramana, M. V., Balakrishna, K., Murali, H. C. S., Batra, H. V. (2011). Multiplex PCR-based strategy to detect contamination with mycotoxigenic Fusarium species in rice and fingermillet collected from southern India. J. Sci. Food Agric. 91, 1666–1673. doi: 10.1002/jsfa.4365
Saghai-Maroof, M. A., Soliman, K. M., Jorgensen, R. A., Allard, R. W. (1984). Ribosomal DNA spacer-length polymorphisms in barley: Mendelian inheritance, chromosomal location, and population dynamics. Proc. Natl. Acad. Sci. U.S.A. 81, 8014–8018. doi: 10.1073/pnas.81.24.8014
Sánchez-Rangel, D., SanJuan-Badillo, A., Plasencia, J. (2005). Fumonisin production by Fusarium verticillioides strains isolated from maize in Mexico and development of a polymerase chain reaction to detect potential toxigenic strains in grains. J. Agric. Food Chem. 53, 8565–8571. doi: 10.1021/jf0514827
Singh, H., Kaur, H. (2023). Needle inoculation: an efficient cob inoculation technique for Fusarium ear rot of maize. Indian Phytopathol. 76, 1–6. doi: 10.1007/s42360-023-00690-1
Singh, H., Kaur, H. (2024). Unveiling the impact of varied growing seasons on Fusarium ear rot severity in maize under north Indian conditions. Plant Dis. Res.
Stepien, L., Koczyk, G., Waskiewicz, A. (2011). FUM cluster divergence in fumonisins-producing Fusarium species. Fungal Biol. 115, 112–123. doi: 10.1016/j.funbio.2010.10.011
Sultana, S., Kitajima, M., Kobayashi, H., Nakagawa, H., Shimizu, M., Kageyama, K., et al. (2019). A natural variation of fumonisin gene cluster associated with fumonisin production difference in Fusarium fujikuroi. Toxins 11, 200. doi: 10.3390/toxins11040200
Szecsi, A., Koncz, Z., Magyar, D. (2011). Morphological and molecular identification of airborne Fusarium propagules trapped in a maize field in Hungary. Acta Phytopathol. Entomol. Hung. 46, 175–184. doi: 10.1556/APhyt.46.2011.2.1
Tancic, S., Stankovic, S., Levic, J., Krnjaja, V., Vukojevic, J. (2012). Diversity of Fusarium verticillioides and F. proliferatum isolates according to their fumonisin B1 production potential and origin. Genetika 44, 163–173. doi: 10.2298/GENSR1201163T
Vigier, B., Reid, L. M., Dwyer, L. M., Stewart, D. W., Sinha, R. C., Arnason, J. T., et al. (2001). Maize resistance to gibberella ear rot: Symptoms, deoxynivalenol, and yield. Can. J. Plant Pathol. 23, 99–105. doi: 10.1080/07060660109506915
Wu, F. (2007). Measuring the economic impacts of Fusarium toxins in animal feeds. Anim. Feed Sci. Technol. 137, 363–374. doi: 10.1016/j.anifeedsci.2007.06.010
Keywords: fumonisins, fum genes, Fusarium ear rot, Fusarium verticillioides, maize, quantitative analysis
Citation: Singh H, Kaur H, Hunjan MS and Sharma S (2025) Unveiling toxigenic Fusarium species causing maize ear rot: insights into fumonisin production potential. Front. Plant Sci. 16:1516644. doi: 10.3389/fpls.2025.1516644
Received: 31 October 2024; Accepted: 03 March 2025;
Published: 21 March 2025.
Edited by:
Sabrina Sarrocco, University of Pisa, ItalyReviewed by:
Chaofeng Wang, University of Nebraska-Lincoln, United StatesCopyright © 2025 Singh, Kaur, Hunjan and Sharma. This is an open-access article distributed under the terms of the Creative Commons Attribution License (CC BY). The use, distribution or reproduction in other forums is permitted, provided the original author(s) and the copyright owner(s) are credited and that the original publication in this journal is cited, in accordance with accepted academic practice. No use, distribution or reproduction is permitted which does not comply with these terms.
*Correspondence: Harinder Singh, aW5kZXJicmFyMjIxM0BnbWFpbC5jb20=
Disclaimer: All claims expressed in this article are solely those of the authors and do not necessarily represent those of their affiliated organizations, or those of the publisher, the editors and the reviewers. Any product that may be evaluated in this article or claim that may be made by its manufacturer is not guaranteed or endorsed by the publisher.
Research integrity at Frontiers
Learn more about the work of our research integrity team to safeguard the quality of each article we publish.