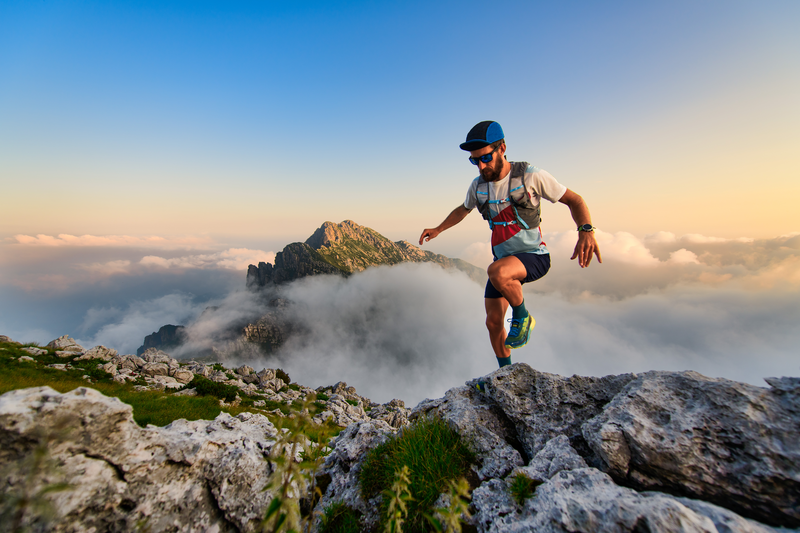
94% of researchers rate our articles as excellent or good
Learn more about the work of our research integrity team to safeguard the quality of each article we publish.
Find out more
ORIGINAL RESEARCH article
Front. Plant Sci.
Sec. Aquatic Photosynthetic Organisms
Volume 16 - 2025 | doi: 10.3389/fpls.2025.1513624
The final, formatted version of the article will be published soon.
You have multiple emails registered with Frontiers:
Please enter your email address:
If you already have an account, please login
You don't have a Frontiers account ? You can register here
Salt marshes are known for their higher carbon absorption capacity and productivity compared to terrestrial ecosystems. However, in Korea, human activities have led to a reduction in these vital coastal habitats. This study observed seasonal changes in vegetation, photosynthetic efficiency, carbon and nitrogen content of Suaeda japonica at two salt marsh ecosystems in Incheon, Korea, Yeongjong and Sorae, namely YJ and SR. In spring, plant density at S. was significantly higher than at YJ. This higher density at SR. inhibited growth and survival during summer and fall. In addition, photosynthetic efficiency at SR. was greatly decreased in summer compared to spring. The different habitat densities between the sites resulted in morphological differences. The plants at YJ, with lower density, grew wider with more branches and showed higher dry weight in comparison to those at SR. Carbon sequestration by S. japonica per unit area was about 113.70 g/m 2 at YJ, which is twice as high as at SR. The tissue carbon content was highest in the roots, suggesting that carbon absorbed by the leaves is effectively stored as sediment. This study confirmed annual changes in salt marsh vegetation and quantified carbon sequestration. The characteristics of salt marsh plant as regional and seasonal change highlight the importance of salt marshes as a blue carbon resource and underscore the need for salt marsh restoration projects.
Keywords: Salt marsh plant, Suaeda japonica, Carbon Sequestration, photosynthesis, Incheon, Incheon
Received: 18 Oct 2024; Accepted: 18 Feb 2025.
Copyright: © 2025 Hwang, Park, Han, Yarish and Kim. This is an open-access article distributed under the terms of the Creative Commons Attribution License (CC BY). The use, distribution or reproduction in other forums is permitted, provided the original author(s) or licensor are credited and that the original publication in this journal is cited, in accordance with accepted academic practice. No use, distribution or reproduction is permitted which does not comply with these terms.
* Correspondence:
Jang Kyun Kim, Incheon National University, Incheon, Republic of Korea
Disclaimer: All claims expressed in this article are solely those of the authors and do not necessarily represent those of their affiliated organizations, or those of the publisher, the editors and the reviewers. Any product that may be evaluated in this article or claim that may be made by its manufacturer is not guaranteed or endorsed by the publisher.
Research integrity at Frontiers
Learn more about the work of our research integrity team to safeguard the quality of each article we publish.