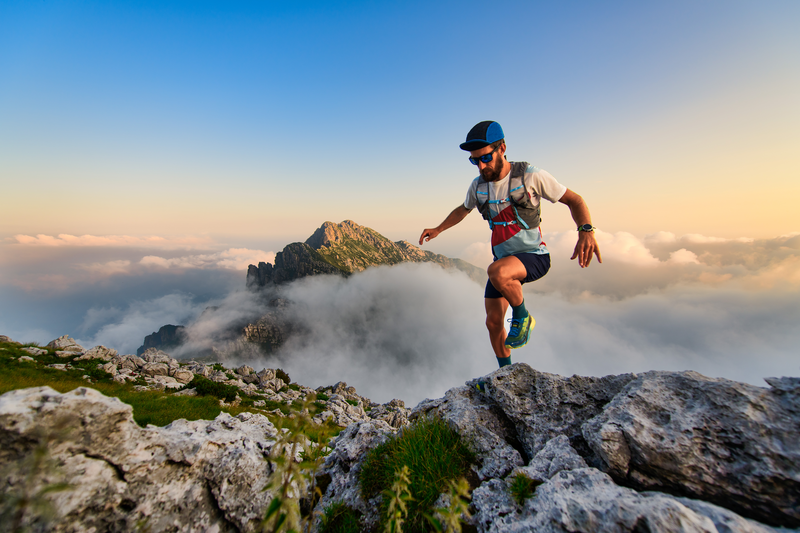
94% of researchers rate our articles as excellent or good
Learn more about the work of our research integrity team to safeguard the quality of each article we publish.
Find out more
ORIGINAL RESEARCH article
Front. Plant Sci.
Sec. Functional Plant Ecology
Volume 16 - 2025 | doi: 10.3389/fpls.2025.1510676
This article is part of the Research Topic Integrating Nature-based Solutions for Land Degradation Neutrality and Deriving Co-Benefits View all 5 articles
The final, formatted version of the article will be published soon.
You have multiple emails registered with Frontiers:
Please enter your email address:
If you already have an account, please login
You don't have a Frontiers account ? You can register here
Nature-based Solutions (NbS) provide a comprehensive strategy for environmental management, focusing on the protection, sustainable use, and restoration of natural and modified ecosystems. Cultivated grasslands are a form of NbS, offering benefits such as increased biodiversity, improved soil fertility, and greater ecosystem resilience. They are widely acknowledged for their positive impact on restoring degraded grasslands. Kentucky bluegrass (Poa pratensis L.) is widely used for restoring degraded grasslands on the Qinghai-Tibet Plateau. However, long-term cultivation of Kentucky bluegrass can lead to above-ground degradation, which challenges its effectiveness in restoring ecosystem health. This study investigates the impacts of Kentucky bluegrass cultivation on soil quality, focusing on soil nutrients, enzyme activities, and microbial communities across different recovery stages. Field experiments were conducted to analyze soil quality dynamics during early (2nd year), mid (6th year), and late (10th year) succession stages of cultivated grasslands on the Qinghai-Tibet Plateau. Our results show that in the early and mid-stages, soil total nitrogen, total phosphorus, and organic carbon storage were significantly lower compared to undegraded grasslands, with the lowest soil quality observed in the early stage (P < 0.05). However, by the late stage, soil quality significantly improved, with total nitrogen, total phosphorus, and organic carbon contents exceeding those of undegraded grasslands by 14.59%. These improvements were driven by enhanced microbial community dynamics and increased nitrogen and carbon cycling enzyme activities, which promoted nutrient utilization and organic matter decomposition. This process was accompanied by a rise in microbial diversity, supporting soil resilience and ecosystem function. Soil total nitrogen emerged as a key determinant of soil quality in both natural and cultivated grasslands, and appropriate nitrogen fertilization strategies were found to effectively enhance grassland productivity and ecosystem health. Overall, this study highlights the potential of Kentucky bluegrass in restoring degraded grasslands by improving soil fertility and microbial community structure over time, providing insights into sustainable management practices to maintain soil fertility and ecosystem services on the Qinghai-Tibet Plateau.
Keywords: Qinghai-Tibet Plateau, Cultivated grassland, soil microbial communities, soil quality, Kentucky bluegrass
Received: 13 Oct 2024; Accepted: 04 Mar 2025.
Copyright: © 2025 Li, Shi, Liu, Liang, Li and Liu. This is an open-access article distributed under the terms of the Creative Commons Attribution License (CC BY). The use, distribution or reproduction in other forums is permitted, provided the original author(s) or licensor are credited and that the original publication in this journal is cited, in accordance with accepted academic practice. No use, distribution or reproduction is permitted which does not comply with these terms.
* Correspondence:
Wen-Hui Liu, Qinghai University, Xining, China
Disclaimer: All claims expressed in this article are solely those of the authors and do not necessarily represent those of their affiliated organizations, or those of the publisher, the editors and the reviewers. Any product that may be evaluated in this article or claim that may be made by its manufacturer is not guaranteed or endorsed by the publisher.
Research integrity at Frontiers
Learn more about the work of our research integrity team to safeguard the quality of each article we publish.