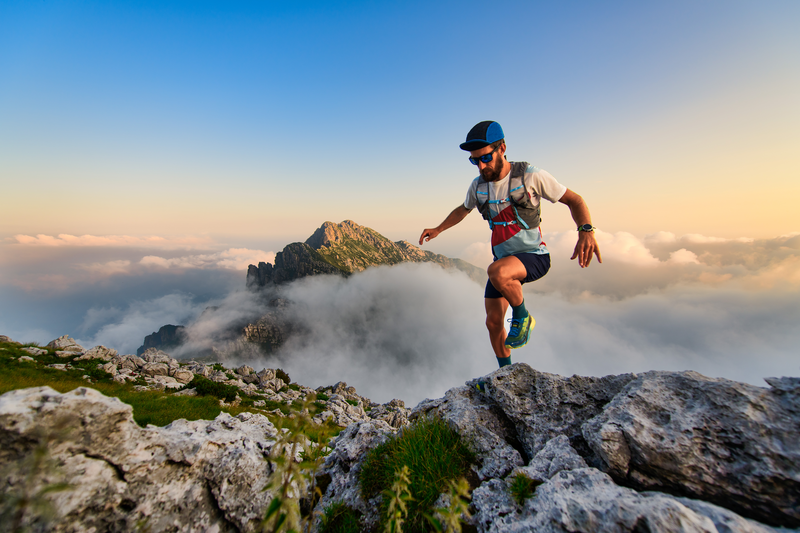
94% of researchers rate our articles as excellent or good
Learn more about the work of our research integrity team to safeguard the quality of each article we publish.
Find out more
ORIGINAL RESEARCH article
Front. Plant Sci.
Sec. Plant Bioinformatics
Volume 16 - 2025 | doi: 10.3389/fpls.2025.1500429
The final, formatted version of the article will be published soon.
You have multiple emails registered with Frontiers:
Please enter your email address:
If you already have an account, please login
You don't have a Frontiers account ? You can register here
Zinc finger protein (ZFP) represent a significant class of transcription factors in plants, involved in various functions, including tissue development, signal transduction, and responses to both biotic and abiotic stresses. ZFPs are categorized into 10 distinct subfamilies, among which the C3H gene family is recognized as a functionally significant group of transcription factors.To date, no studies have been reported regarding the C3H gene family in melon (Cucumis melo). In this study, 38 CmC3H genes were identified in the melon genome, and these genes are unevenly distributed across the 12 chromosomes. Phylogenetic analysis classified the C3H family members into four groups, with significant differences observed in sequence, protein motifs, and gene structure among CmC3H genes within the same group. The CmC3H family contains one pair of segmentally duplicated genes and shares 20, 7, 39, and 38 pairs of homologous C3H genes with Arabidopsis thaliana, rice (Oryza sativa), cucumber (Cucumis sativus), and watermelon (Citrullus lanatus), respectively.Promoter region analysis revealed a high abundance of cis-elements associated with growth and development, hormone regulation, and stress responses. Expression profiling revealed that CmC3H family members exhibit significant tissue-specific expression patterns. Quantitative PCR analysis indicated that six genes (CmC3H4, CmC3H7, CmC3H13, CmC3H24, CmC3H33, and CmC3H38) may play roles in melon's drought stress resistance. Heavy metal lead stress appears to suppress the expression of CmC3H genes. The genes CmC3H24 and CmC3H33 may be involved in regulating melon's resistance to Fusarium wilt infection. CmC3H11 and CmC3H21 can be considered as the key candidate genes for improving the melon's ability to resist both biotic and abiotic stresses.This study provides preliminary insights into the expression profiles of CmC3H genes under drought stress, heavy metal lead stress, and Fusarium wilt infection, offering a theoretical foundation for the molecular mechanisms underlying melon improvement and stress resistance.
Keywords: Cucumis melo, C3H gene family, Drought stress, heavy metal lead stress, Fusarium wilt infection
Received: 23 Sep 2024; Accepted: 18 Feb 2025.
Copyright: © 2025 Zheng, Dai, Mu, Li and Cheng. This is an open-access article distributed under the terms of the Creative Commons Attribution License (CC BY). The use, distribution or reproduction in other forums is permitted, provided the original author(s) or licensor are credited and that the original publication in this journal is cited, in accordance with accepted academic practice. No use, distribution or reproduction is permitted which does not comply with these terms.
* Correspondence:
Ling Zheng, Biology, Luoyang Normal University, Luoyang, China
Yanwei Cheng, Biology, Luoyang Normal University, Luoyang, China
Disclaimer: All claims expressed in this article are solely those of the authors and do not necessarily represent those of their affiliated organizations, or those of the publisher, the editors and the reviewers. Any product that may be evaluated in this article or claim that may be made by its manufacturer is not guaranteed or endorsed by the publisher.
Research integrity at Frontiers
Learn more about the work of our research integrity team to safeguard the quality of each article we publish.