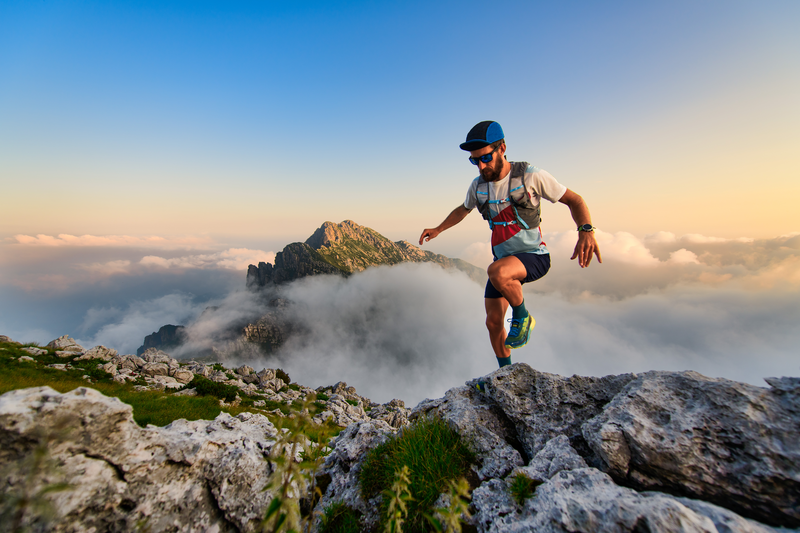
95% of researchers rate our articles as excellent or good
Learn more about the work of our research integrity team to safeguard the quality of each article we publish.
Find out more
ORIGINAL RESEARCH article
Front. Plant Sci. , 18 March 2025
Sec. Functional Plant Ecology
Volume 16 - 2025 | https://doi.org/10.3389/fpls.2025.1483774
Introduction: This research aimed to evaluate the impact of ruminant digestion on viability and germination pattern of the seeds of 9 weed species (A. theophrasti, A. myosuroides, A. retroflexus, A. sterilis, C. album, D. stramonium, E. crus-galli, L. multiflorum and S. halepense).
Methods: One hundred seeds of each species were included in nylon bags and exposed to in vitro procedures simulating the rumen fermentation according to an experimental design that considered: 9 weed species, 3 incubation times in the artificial rumen (12, 24 and 48 h), 2 diets (lactating cows, and heifers), 4 replications, plus 4 additional replicas per species with seeds not subjected to the in vitro digestion as a control. This design was repeated two times (2 batches), involving a total of 504 replicas. Results were expressed in relative terms, using the data from the untreated seeds as a scaling factor. Data were analyzed, by species, with a model that considered diet and incubation time, and their interaction as fixed factors, and the batch as a random effect.
Results and conclusions: Incubation time evidenced the greatest impact on seed germination and viability (6 species), where diet (4 species) and Incubation time x diet interaction (3 species) had lower impact. Compared to the control, A. theophrasti germination increased to 150% after 12 h but dropped to ~20% after 48 h under the lactating cows’ diet. Germination of A. myosuroides remained stable initially but fell to ~60% after 48 h, while A. sterilis showed consistently low germination, further declining with digestion. Germination of C. album rose to ~130% after 48 h, and the one of E. crus-galli to ~140%. For D. stramonium, germination decreased to ~20%, with heifers’ diet causing greater losses. No significant effects were noted for L. multiflorum, S. halepense, or A. retroflexus. Viability losses were significant for A. theophrasti and A. myosuroides under the lactating cows’ diet and for D. stramonium under the heifers’ diet. Possible variation causes were evidenced in the thickness and the fibrous content (NDF, ADF, ADL) of the seed coats, correlated with the rumen microbial activity.
Animal husbandry is heavily dependent on plant production to provide feed for the animals and can be strongly influenced by farmland management and different agricultural practices (Makkar, 2018). In cattle production, different fodder types, such as hay, silage, grains, and other plant parts are used to ensure a balanced diet and maintain animal health. All of these fodders could contain a certain percentage of viable weed seeds which could be ingested by cattle (Blackshaw and Rode, 1991). Some of the main crops used in cattle rations are maize (Zea mays L.) and soybean (Glycine max [L.] Merr.), which could also potentially be infested by different weed species during their growth. Other than just reducing the yield of these important crops, some of the seeds of different weed species may contaminate the harvested crop seeds, eventually finding their way into the cattle fodder (Wilson et al., 2016; Anwar et al., 2021; Rubenstein et al., 2021). The presence of the weed seeds in these products is dependent on the harvest time and the storage of the obtained products. Therefore, the raw materials could contain up to 20% of whole and viable weed seeds (Blackshaw and Rode, 1991).
Previous research concluded that a significant number of weed species seeds could survive the passage through the animal digestive system and germinate afterwards (Salazar-Rivera et al., 2020; Lee et al., 2021). In some cases, the seeds that went through the animal digestive system became even more germinable, compared to the intact seeds, or were able to germinate faster (Katovich et al., 1993; Salazar-Rivera et al., 2020; Oveisi et al., 2021). Finally, after the processes inside the animals, the digested fodder, and with it, some of the still viable weed seeds, exits the animals as excrement. In various research conducted, a high proportion of viable weed seeds have been found in these excrements both in wild and farm animals (Cudney et al., 1992; Lee et al., 2021; Liu et al., 2020; Oveisi et al., 2021; Pleasant and Schlather, 1994; Rahimi et al., 2016; Salazar-Rivera et al., 2020). With farm animals, these excrements become part of manure and slurry, which are distributed in the fields as organic fertilizers (Du et al., 2020; Prado et al., 2022; Schröder, 2005; Schröder and Dilz, 1987). If some of the weed seeds are still viable after the passage through cattle digestive system, they could find their way into the fields as part of the manure or slurry, further increasing the weed infestation of the fields, which has been confirmed by different studies (Cudney et al., 1992; Katovich et al., 1993; Lowry, 1996; Materechera and Modiakgotla, 2006; Platis et al., 2023; Pleasant and Schlather, 1994). This comes as no surprise, considering that, once viable seeds have germinated, manure provides the nutrients that seedlings require for their development (Hogan and Phillips, 2011). Beyond its localized impact, the survival of weed species within the digestive systems of cattle could potentially facilitate the spread of these species on a larger scale. There is evidence that historically landscapes were shaped by grazing cattle at long distances, and also through cattle trade, bringing new plant species even across continents (Bruun and Fritzbøger, 2002; Fuller and Boivin, 2009). In addition, the potential for introduced species to become invasive due to their absence in the new environment increases the risk of introducing resistant weed species to novel areas. This increased risk poses a significant threat to both agricultural production and agroecosystems (Andrews, 1995; Bruun and Fritzbøger, 2002; De-Clerck-Floate, 1997; Fuller and Boivin, 2009; Hogan and Phillips, 2011; Malo and Suárez, 1995; Rahimi et al., 2016).
Given the various issues and adverse effects associated with the presence of weed seeds in cattle diets, it is crucial to gain a deeper understanding of the fate of seeds from key weed species after they pass through the cattle digestive system. This serves as the starting point for identifying livestock and manure management strategies to effectively reduce weed seed viability, defined as both germinable and viable but dormant seeds, and their spread. Considering the major crops grown in the region, used also as animal fodder, more precisely maize (Zea mays) and soybean (Glycine max) it was decided to focus on some of the agronomically most important weed species of these crops in north-eastern Italy. Therefore, this study aimed to evaluate the degradation and the germination of nine weed species seeds (Abutilon theophrasti Medik.; Alopecurus myosuroides Huds.; Amaranthus retroflexus L.; Avena sterilis L.; Chenopodium album L.; Datura stramonium L.; Echinochloa crus-galli [L.] P. Beauv.; Lolium multiflorum Lam.; Sorghum halepense L.) after different times (12, 24, and 48 hours) of permanence in the rumen fluid with diets for lactating cows and heifers, and after the post-ruminal digestion with in vitro trials.
All the experimental procedures were carried out according to the Italian law on animal care and welfare. In order to obtain the rumen fluid for in vitro fermentations, all the practices were approved by the ethical committee at the University of Padova (OPBA, approval number 1312041/2022).
Mature seeds of nine weed species, Abutilon theophrasti, Alopecurus myosuroides, Amaranthus retroflexus, Avena sterilis, Chenopodium album, Datura stramonium, Echinochloa crus-galli, Lolium multiflorum and Sorghum halepense, were harvested by hand, during the season of 2021, at the experimental farm “Lucio Toniolo” of the University of Padova situated in Legnaro, Italy. Following harvest, the seeds were cleaned using a two-step process. Initially, they were manually processed by rubbing against a wooden board fitted with a rubber lining to prevent damage. This step helped grind and reduce the weight of various impurities. Subsequently, a seed blower was employed to separate the seeds from the remaining impurities by blowing away the lighter particles. After completing the cleaning process, the seeds were stored in a dry environment at 4°C before being subjected to the in vitro rumen fermentation. The experimental setup involved 4 replicates for each of 9 seed species × 3 incubation times × 2 diets. In addition, there were 4 additional replicates per species as untreated controls, i.e. not subjected to in vitro rumen fermentation (36 replicates). The data obtained from the control replicates were used to estimate the dormancy and viability of the weed species tested (Supplementary Table S1). This experimental plan was repeated two times (2 batches), for a total of 504 replicas, 56 replicas per each species. Each bag (replicate) comprised 100 seeds of the same species.
The seeds of each replicate, used for the in vitro simulation of rumen fermentation, were enclosed in woven nylon bags with a porosity of 50 ± 10 μm, manufactured by Ankom (Ankom Technology®, Macedon, NY, USA). The nylon bags, proven effective in previous analyses, permit the entrance of the rumen fluid microorganisms into the bag, while securely containing the studied material (Weakley et al., 1983; Vogel et al., 1999). To prevent potential degradation and bag opening that could lead to seed loss, the seeds were tightly secured within the bags using nylon thread after placement.
Rumen fluid (RF), was sampled, according to consolidated procedures (Tagliapietra et al., 2012) using an esophageal probe by two fasting Simmental cows and by two Simmental heifers in the experimental farm “Lucio Toniolo” of the University of Padova (Legnaro, Italy). The collected RF (approximately 1-L per animal) was transported from the field to the laboratory, maintaining the temperature of 39°C in thermos. When the RF arrived in the laboratory, it was filtered with 4 layers of cheesecloth to remove the particles and then maintained under a flux of carbon dioxide (CO2) and at 39°C in a warm bath.
The degradative impact of rumen incubation was assessed for both lactating cow and heifer diets. Samples representative of the two diets were prepared by mixing the single ingredients (dried and milled at 1 mm screen) in the proportions reported in Table 1. The medium solution was prepared according to Menke and Steingass (1988). The medium solution was kept at 39°C under a flux of CO2 until the arrival of the RF in the laboratory.
The in vitro fermentations were performed using the DaisyII incubator (Ankom Technology ®, Macedon, NY, USA), equipped with four vessels. The system is designed to maintain stable anaerobic and temperature conditions and it provides measurements of fiber degradability closely correlated to the fiber degradability measured in situ (Spanghero et al., 2003).
For our experiment, we used only two vessels, one designated to simulate the rumen fermentation of a lactating cow diet and the other one that of the heifers. Before the incubation process, the samples of RF collected from the two lactating cows were pooled, as well as the RF samples from the two heifers, resulting in two distinct RFs that were poured together with 12.5 g of dry matter (DM) of each diet in the vessel.
The anaerobic and temperature conditions in the vessels were assured by purging CO2 and preheating all the materials and fluids at 39°C before incubation. The vessels were filled with 800 mL of the respective RF and 1600 mL of the medium solution, according to the proportions indicated by Menke and Steingass (1988). In each batch, 108 bags (4 replicates × 9 species × 3 incubation times) containing the weed seeds were incubated in each vessel for 12, 24, or 48 h. At each incubation time, a third of the bags were removed from the fermentation vessels. The three incubation times were used to evidence differences in the loss of germinability and viability of the fodders weed seeds up to 48 h, which is the conventional duration to assess the ruminal degradability of fodders (Van Soest and Wine, 1967).
Huhtanen and Vanhatalo (1997) indicated that post ruminal digestion contributes less than 5% to the degradation of the fibrous constituents, and consequently we can assume that it has a comparable impact on seed scarification. To simulate post-ruminal digestion in cattle, the methodology proposed by Boisen and Fernández (1997) was used. The 54 bags removed from the Daisy incubator at each incubation time were placed in a 5-litre plastic beaker and exposed to an in vitro simulated gastric environment. The beaker was filled with a solution consisting of two liters of a 0.1M phosphate buffer plus approximately 10 ml of 0.2 M HCl to adjust the solution pH to 2.0. One ml of a fresh solution containing 25 mg of pepsin (porcine pepsin, 2000 FIP-U/g, Merck, Darmstadt, Germany) was added to this solution. The nylon bags extracted from the Daisy incubator were immersed in this solution for 4 hours.
To simulate digestion in the small intestine, after the fourth hour, the pH of the solution containing the nylon bags was raised to 6.8 by adding 10 ml of a 0.2 M phosphate buffer at pH 6.8 and approximately 5 ml of 0.6 M NaOH. One ml of a fresh solution containing 100 mg of pancreatin (porcine pancreatin, Merck, Darmstadt, Germany) was then added to the solution. The bags with the seeds were exposed to this solution for 7.5 hours.
To simulate large intestine digestion, 0.5 kg of fresh feces from the experimental lactating cows and heifers were collected and separately mixed with 1 liter of a buffer solution prepared as described by Menke and Steingass (1988). At the end of the simulated small intestine digestion, the nylon bags were immersed in this mixture for 3.5 hours. After that, the bags were removed from the mixture, washed with warm water, and prepared for the germination test.
In each batch, after the rumen and post-rumen digestion, the seeds were sown onto filter paper saturated with 2 mL of distilled water in individual Petri dishes. Each bag resulting from the previous treatments was assigned to a Petri dish. In addition, 36 control Petri dishes containing the weed seeds of the 9 species (four for each species) not subjected to the in vitro digestion procedures were included as control. After sowing, the Petri dishes were sealed with parafilm and placed inside germination chambers. Two alternating temperature regimes were employed based on the optimal germination temperatures for each species, maintaining a 12-hour light/12-hour dark photoperiod. Specifically, temperatures of 15°C/25°C were maintained for A. myosuroides, A. sterilis, C. album, and L. multiflorum, while 18°C/30°C were applied for A. theophrasti, A. retroflexus, D. stramonium, E. crus-galli, and S. halepense. The selection of these specific temperature ranges is based on the germination periods of the species studied. The first four species are generally classified as spring species, while the latter four are categorized as summer species. Consequently, the chosen temperatures were intended to replicate the optimal conditions for germination and growth of these species, as demonstrated in studies by Nikolić et al. (2022) and Song et al. (2024). Germination progress was monitored every 2-3 days, counting and removing germinated seeds and additional water was added if necessary. Germination trials were considered complete when all seeds germinated or after 10 d without germination (Baskin and Baskin, 2014).
Upon conclusion of germination, non-germinated seeds underwent a tetrazolium test (Borza et al., 2007) to determine their viability, distinguishing between dormant viable seeds and those that had lost viability. Statistical analyses were performed on both the germinated seeds and the viable seeds (germinated + viable after the tetrazolium test) to comprehensively assess the impact of passage through the cattle digestive system on the survival and germination of weed species seeds. To optimize the dataset and highlight the possible effects of different treatments on the germination and viability of different species, the number of germinated and viable seeds for each species under treatment was expressed as percentage on the respective untreated control.
Given the extensive literature linking seed viability and germination to the chemical composition of seeds, these analyses were conducted to complement the findings of this study. By assessing the chemical composition of the seeds, we aimed to contextualize the observed results and compare them with existing research, for a more comprehensive understanding of the factors influencing seed survival and germination.
For this, samples (5 g) of all the weed seeds used in the two years were collected and grounded at 1 mm (Retsch ZM 200, Retsch GmbH, Germany) to be analyzed.
The sample DM was determined using an oven at 101-103°C overnight (AOAC, 2016; method 978.01). Ash content was obtained after placing the samples in a muffle at 550°C for 4 hours and weighted after cooling in a desiccator (AOAC, 2016; method 942.05). Crude protein (CP) content was measured according to the Kjeldahal method (AOAC, 2016; method 978.04) using a Kjeltec™ 8400 apparatus (Foss Electric A/S, Hillerød, Denmark). Ether extract (EE) was obtained according to the AOAC guidelines (AOAC, 2016; method 2003.05).
Fiber fractions were determined using the Van Soest methodology (Van Soest et al., 1991). Neutral detergent fiber (aNDF) and acid detergent fiber (ADF) were performed sequentially using the Ankom fiber analyzer (Ankom, Rochester, NY, USA), and following the Ankom procedures (Ankom Technology, 2023). Neutral detergent fiber was determined with heat-stable α-amylase and without sulfite (aNDF) (AOAC, 2000; method 2002.04). Acid detergent lignin (ADL) was obtained after ADF analysis using the analytical method proposed by Ankom (Ankom Technology, 2022). After all the determinations, the hemicellulose and the cellulose content of each sample were calculated:
where AIA indicates the acid insoluble ash.
The starch content was also determined using an enzymatic method via HPLC (High Pressure Liquid Chromatography), developing from the method proposed by AOAC (2016; method 996.11) and (Hall, 2000). All the samples were analyzed in duplicate. One gram of samples was weighed and dissolved with 50 mL of potassium hydroxide 0.5M for 1 hour at 60°C, and, after that, the pH was raised to 4.5. One of the two duplicates of each sample was incubated without enzyme, and the second was inoculated with amino-glucosidase and incubated (8 hours at 40°C). At the end of the 8 hours, the samples were filtered and injected in HPLC. The sample without inoculation provided the amount of free glucose. The sample with the inoculum provided the amount of total glucose. Subtracting the free glucose from the total glucose we obtained the starch.
The rumen fluids and feces collected from dairy cows and heifers, used as microbial inoculums in in vitro fermentations, were analyzed for pH in triplicate. For each sample, 4 mL of rumen fluid and feces (diluted and homogenized 1:5 with distilled water) were collected in triplicates and stored at -30°C after the addition of 1 mL of metaphosphoric acid solution (25% w/v) to stabilize them prior to analysis. Ammonia nitrogen (N) concentrations were quantified using the ammonia rapid assay kit (K-AMIAR 02/20; Megazyme, Bray, Ireland). Volatile fatty acid (VFA) concentrations were determined via high-performance liquid chromatography (HPLC) using a Jasco system equipped with a PU-2080 pump (Jasco, Tokyo, Japan), a model RI-2031 refractive index detector, a model AS-2055 autosampler, and a model CO-2060 column oven. The chromatographic separation was carried out using an Aminex HPX 87H column (300 mm × 7.8 mm; Bio-Rad Laboratories, Hercules, CA, USA). Data acquisition and analysis were performed using ChromNAV software (Version 2.0, Jasco).
The cellulolytic microbial activity, which was estimated as a proxy for seed degradation, was quantified as described by Nikolić et al. (2024) by measuring the resistance to breakage of cotton threads incubated in the Daisy System together with the weed seeds. The cotton threads (n.16, Cucirini Tre Stelle, Caleppio di Settala, Milan, Italy) were cut to a length of 10 cm and placed into the Ankom nylon bags (5 × 5 cm; porosity of 50 ± 10 μm, Ankom Technology®, Macedon, NY, USA). The bags, each containing 6 cotton threads, were securely stitched to prevent thread loss and incubated for 12, 24 or 48 h together with the weed seeds. In each batch, 24 bags were incubated (2 diets × 3 incubation times × 4 replications). After exposure to the rumen microbial activity, the bags were opened, and the threads were retrieved and air-dried. Once dry, their resistance to breakage was measured using a digital dynamometer (IMADA ZP, ELIS Electronic Instruments and Systems, Rome, Italy). For the measurement, each thread was fixed to a keyring by pinching one end and coiling the thread several times around the ring. The dynamometer was set to the “peak” function mode, signifying the maximum load endured by the thread before breaking. This peak force was then determined by pulling the ends of the thread until they broke. In addition to the treated threads, non-treated cotton and silk threads were measured as standards to ascertain the increased fragility of the treated threads. The data obtained from the measurements of the treated threads were then compared to the average values of the non-treated thread standards to estimate the degree of microbial cellulolytic activity.
Statistical analyses were performed within the R environment (RStudio Team, 2023). Data of viability and germination of the seeds, separately for each species, were analyzed with an ANOVA model that included as fixed factors the incubation time (I; 2 d.f.), diet (D; 1 d.f.), batch (1 d.f.) and the incubation time × batch interaction (2 d.f.), after a check for homoscedasticity completed with the Leven test. The experimental unit was the single bag, containing 100 seeds. Data from the experiments (batches) were pooled as ANOVA showed no significant difference in treatment x experiment interactions. Post hoc analysis for significant mean differences was conducted using the Tukey post-hoc test for multiple comparisons, while the correlation analysis was conducted using the cor.test function of the R stats package.
The Fertimetro® data, analyzed with a similar model that considered as fixed factors the diet, the incubation time and their interaction, were already published in Nikolić et al. (2024). The least square means resulting from this analysis were used to evaluate the correlation between the seed viability and the threads degradation traits, representing the rumen microbial activity.
The chemical composition of the inoculums used to simulate in vitro the fermentation occurring in the rumen and the large intestine of dairy cows and heifers is reported in Table 2. Feces contain higher amounts of ammonia nitrogen compared to ruminal fluid but lower concentrations of volatile fatty acids (VFA). Lactating cows, in comparison to heifers, exhibit significantly higher VFA concentrations, lower pH values and comparable N-NH3 concentrations in both ruminal fluid and feces.
Table 2. Rumen fluid and faeces content of ammonia nitrogen (N-NH3, mmol/L) and volatile fatty acid (VFA, g/L) and pH collected from lactating cows and heifers and used as inoculum for in vitro incubations. Each value is the mean of 3 replicate samples.
The chemical composition of the seeds is detailed in Table 3. Acid Detergent Fiber (ADF) varied from a minimum of 10.31% in L. multiflorum to a maximum of 39.12% in D. stramonium, and was high in A. theophrasti, C. album and S. halepense. Lignin content peaked 24.14% in the seeds of C. album, while the content of hemicellulose and cellulose in these species was low. Moreover, the protein content is elevated in A. theophrasti (CP 21.19%). The seed composition of each species will be discussed afterwards in the paper in relation to the viability and germination response after the transit through the digestive tract of cattle.
Seed germination and viability after the digestive processes are illustrated in the Figures 1, 2. Different letters indicate a significant difference and are placed only where the interaction I x D was significant (Tables 4, 5). Compared to control, the germination percentage of A. theophrasti seeds was increased (150%) for short exposure to the simulated rumen microbial activity, but it decreased for prolonged incubations (Figure 1). Notably, the diet for lactating cows had a more pronounced negative effect on the germinability of A. theophrasti seeds compared to the diet for heifers during extended seed residence in the rumen. Consistently with the findings on germination, the viability of A. theophrasti seeds (Figure 2), was significantly influenced by the incubation time (P< 0.001) and diet (P< 0.001), along with their interaction (P< 0.001). The viability of A. theophrasti seeds remained high after 24 hours in the rumen, while it decreased after 48 hours of rumen residence with both diets.
Figure 1. Germination of the species studied after the passage through cattle digestive system. The results are expressed as percentage of control. Different letters are reported when the Diet x incubation time interaction was significant (P-values< 0.05). Statistical difference due to the Diet and the incubation time are provided in Table 3. Error bars represent the standard error.
Figure 2. Viability of the species studied after the passage through cattle digestive system. The results are expressed as percentage of control. Different letters are reported when the diet x incubation time interaction was significant (P-values< 0.05). Statistical difference due to the diet and the incubation time are provided in Table 3. Error bars represent the standard error.
Similar to the findings for A. theophrasti, both incubation time and diet, and their interaction, had a significant impact on the germination and the viability of A. myosuroides seeds. The germinability of A. myosuroides remained relatively stable for short and medium periods of residence in buffered rumen fluid, with a significant decrease after extended permanence. Notably, a more pronounced reduction in germination was evident for lactating cows compared to heifers. Additionally, it is noteworthy that there was little or no induction to germination after the passage through the simulated cattle digestive system for this species. The outcomes regarding the viability of A. myosuroides seeds closely mirrored those of the germination results for this weed species. Once again, it was evident that a significant loss of seed viability occurred only after an extended duration in the rumen, particularly in the presence of the diet designed for lactating cows.
Both the germination and the viability of A. retroflexus were influenced only by the incubation time (P > 0.01) and less evidently by diet (P = 0.023). The germination of A. retroflexus seeds remained relatively high with a slight induction compared to the control during short and medium incubation times. However, a slight reduction of germination was observed at longer incubation times. As for the diets, there were variations in the germination percentage when the seeds of A. retroflexus were exposed to the rumen fermentation with different diets. More seeds germinated when exposed to heifers’ diet compared to those for lactating cows, but the difference was modest. Similar to the germination results, for seed viability, the only significant influencing factors were the incubation time (P< 0.001) and only marginally by the diet (P = 0.021), but there was no influence of the diet x incubation time interaction. The seed viability percentage remained quite high, with only a slight reduction observed after an extended duration in the rumen. These results align with the observations made for the germination of A. retroflexus seeds, suggesting a slightly higher survival rate with heifers’ diet compared to that for lactating cows.
The only factor influencing the germination and viability of A. sterilis was the incubation time (P< 0.001). Both seed germination and viability of A. sterilis were remarkably low after passage through the simulated cattle digestive system (Figures 1, 2). Even at the shortest exposure time, less than 20% of the seeds germinated, and this percentage further decreases with increasing incubation time.
The results concerning the viability and germination of C. album after the passage through the cattle digestive system revealed significant patterns. Data from Table 4 illustrates that incubation time, diet and their interaction had significant impact on germination (Figure 1). However, none of the factors included in the statistical model had significant effect on the viability of C. album seeds, which was maintained very high and close to 100%. The results reveal an increase in the germination rate of seeds for each time x diet combination compared to the control (Figure 1). Notably, the highest germination percentage was observed for seeds exposed to rumen activity for 48 hours. Additionally, it is noteworthy that in this species the diet of lactating cows induced more germination, even at shorter permanence periods in the rumen, compared to that of the heifers.
The results regarding the germination of D. stramonium seeds were distinctive. It was evident that compared to control both diets reduced the germination percentage of the seeds, especially after 48 hours of permanence in the rumen. However, this reduction in germination was more pronounced with heifers’ diet, considering that the germination percentage remained quite high after 12 and 24 hours. Meanwhile, for lactating cows’ diet, the reduction in germination over time was less abrupt, even though it exhibits lower values even at shorter permanence in the rumen. The results obtained concerning the seed viability of this weed species (Figure 2), suggest that the reduction in seed viability becomes significant only after an extended permanence in the rumen, specifically after 48 hours. Notably, after 48 hours, more seeds of this species lost their viability under the influence of the heifers’ diet compared to those of lactating cows.
The data of E. crus-galli present in Table 5 show that, also for this species, there was no significant impact on the seed viability after the passage through the cattle digestive system. On the other hand, the incubation time significantly impacted the germination of this weed species. Each incubation period had a promoting effect on E. crus-galli seed germination compared to the control (Figure 1). This effect is particularly noticeable after 48 hours of incubation (P<0.001).
The seed viability and germination of the seeds of L. multiflorum were affected by the diet and the incubation time, but not by their interaction (Tables 4, 5). In addition, as in the case of A. sterilis, the only viable seeds were the ones that germinated. The results demonstrate that each incubation time led to a reduction in the seed germination of L. multiflorum compared to the control (Figure 1). However, even though there were significant differences indicating a major reduction in seed germination after 48 hours of incubation, it is noteworthy that the germination percentage remained higher than 60%. In addition, seeds exposed to the lactating cows’ diet exhibited a lower germination percentage compared to those of heifers.
Ultimately, the results concerning both seed germination (Table 4) and seed viability (Table 5) showed no significant influence of any of the factors or their interactions on S. halepense. Meaning that the seed of this weed species remained intact during this process.
Data from the Fertimetro suggested a progressive increase in cellulolytic microbial activity of the rumen fluid with increasing incubation times (Figure 3). At 24 and 48 h of incubation, microbial activity was greater in the diet for lactating cows compared to that of heifers. As evidenced in Figure 4, there were negative correlations (from -0.32 to -0.90) between the cellulolytic properties of the rumen fluid, measured from the cotton threads resistance at pulling, and the seed viability after the passage through the simulated digestive system. As expected, there was no significant correlation for the same species that also exhibited little to no changes in their viability after being exposed to rumen microbial activity (E. crus-galli, C. album, S. halepense). Furthermore, depending on the species and the composition of the seed coat, this measurement may also suggest a higher germination percentage for seeds in environments with elevated cellulolytic microbial activity. In such environments, the microbial activity may effectively scarify the seed coat, facilitating the initiation of the germination process. Conversely, in environments with lower microbial activity, the seed coat may remain intact, hindering germination. The Fertimetro method has proven to be indicative of seed degradation in rumen, as observed in other environments, such as soil (Nikolić et al., 2020, 2024).
Figure 3. Degradation percentage of cotton threads, as estimation of rumen microbial activity, exposed to different diets and to different incubation times. The letters indicate a significant difference p-value< 0.05 (Nikolić et al., 2024).
Figure 4. Correlation between the microbial activity measured inside the rumen at different times and estimated weed seed degradation.
The seeds of various weed species exhibit different shapes, sizes, and compositions, including variations in cellulose, hemicellulose, and lignin content (Biswas et al., 1970). These distinctions in seed-building materials can significantly impact seed degradation, particularly when influenced by microorganisms (Davis et al., 2008; Krzyzanowski et al., 2023; Talbot and Treseder, 2012). The results of our study unveiled distinct behavioral patterns among various weed species concerning germination and seed viability following their passage through the cattle digestive system. This diversity aligns with existing research, affirming the wide range of degradation percentages exhibited by seeds of different shapes and sizes from varied plant families when subjected to the cattle digestive system (Blackshaw and Rode, 1991; Lowry, 1996; Ocumpaugh and Swakon, 1993). Both seed germination and viability are intricately dependent on a myriad of biotic and abiotic factors and their interactions (Humphries et al., 2018; Javaid et al., 2022; Nikolić et al., 2020; Ranganathan and Groot, 2023). Monitoring the effects of these factors and their interactions becomes paramount, especially within environments like the cattle rumen.
The thickness and composition of the seed coat are usually considered crucial determinants in the degradation process, where species with larger seeds and thicker seed coats demonstrated prolonged survival under adverse conditions compared to species with smaller seeds and thinner seed coats (Dubbern de Souza and Marcos-Filho, 2001; Manonmani et al., 1996). The constituents of the seed coat, particularly lignin, cellulose, and hemicellulose, play pivotal roles in shielding the embryo from adversities, either individually or through their intricate interactions (Polo et al., 2020; Abati et al., 2022; Brzezinski et al., 2022).
The results from the current study align with these statements, with all species, except A. sterilis, maintaining viability throughout the trials or experiencing significant viability reduction only after 48 hours of incubation. Analysis of seed composition (Table 3) reveals that the seed species studied exhibit high content of cellulose, hemicellulose, lignin, or a combination of these. Notably, A. sterilis stands out with low content in all three substances. Given that these compounds are primarily located in the cell walls forming the seed coat, tasked with protecting the embryo (Grgas et al., 2023; Krzyzanowski et al., 2023; Polo et al., 2020; Ranganathan and Groot, 2023), their concentration likely exerts a significant influence on seed degradation.
Contrary to expectations, seed size alone exhibited minimal influence on degradation outcomes. Differently from A. sterilis with large seeds, S. halepense, A. theophrasti, and D. stramonium with equally large seeds maintained high seed viability. The latter three species exhibited this resilience due to their elevated content of cellulose, hemicellulose, and lignin compared to A. sterilis. In contrast, certain species such as C. album, S. halepense, and E. crus-galli displayed no effects on seed viability after passage through the cattle digestive system. The resistance of C. album can be attributed to its high lignin content (24.14%), while S. halepense relies on its abundant hemicellulose and cellulose. For E. crus-galli, whose content of these substances is medium to low, the puzzle may be unraveled by exploring different substances produced by the seeds, potentially possessing antimicrobial properties. Research by Odintsova et al. (2008) and Rogozhin et al. (2012) identified specific polypeptides named defensins in E. crus-galli seeds, expressing antimicrobial properties. Although our study did not delve into this investigation, the presence of these defensins may underpin the survival of E. crus-galli seeds and warrants further exploration.
A significant trend emerged among species influenced by the cattle digestive system, with the inoculum from lactating cows exerting a greater impact on germination and viability compared to that from heifers. These differences are mainly due to the different chemical composition of the diets, which influences the composition and activity of the ruminal fluid. As expected, the higher starch content in the lactating cows’ diets, compared to those of heifers, stimulates microbial activity, resulting in increased volatile fatty acid concentrations and a lower ruminal fluid pH.
The digestive effects observed with the in vitro approach applied in the current paper is not fully representative of the in vivo conditions, where the fodder is continuously chewed because of rumination, and moved along the digestive lumen. However, the in vitro simulation of the digestive processes was well reflected by the degradation of the cotton threads, which distinguished pretty well the effects of the two inoculum and diets on the cellulolytic activity of the rumen microbes. The positive correlations found between the seed viabilities and the cellulolytic properties of the rumen fluid aligns with previous findings of Nikolić et al. (2020), who evidenced a direct relationship between increased cellulolytic microbial activity and heightened seed degradation.
Beyond their protective role, seed coats can present challenges for germination (Egley, 1989). While the seed coat acts as the primary defense for the seed embryo, germination and the subsequent emergence of a new plant necessitate partial or complete destruction of the seed coat (Mohamed-Yasseen et al., 1994; Muralidhara et al., 2016). This is particularly pertinent for species with very thick or resistant seed coats. Although complete destruction may risk embryo damage and seed death, minor damages, known as scarification, can stimulate seed germination (Paudyal et al., 2021; Jarrar et al., 2023). Germination-stimulating effects can also be obtained through microbial scarification (Cardarelli et al., 2022), this could explain the results of germination after the passage of the seeds through the digestive system.
Seeds with thicker seed coats, such as A. theophrasti, C. album, and E. crus-galli, exhibit germination stimulation when subjected to the ruminant digestive environment. This aligns with studies demonstrating improved germination in these species after scarification (Horowitz and Taylorson, 1985; Kinugasa et al., 2016; Paudyal et al., 2021). A. theophrasti’s germination percentage tends to decrease over time, with lactating cows’ diet significantly reducing it after 48 hours of microbial activity. This emphasizes the stronger cellulolytic microbial activity in lactating cows’ diet, potentially damaging seed embryos after prolonged rumen exposure. Despite this, studies indicate the presence of A. theophrasti seeds in cattle manure, even from dairy farms, suggesting resilience even under lactating cows’ microbial activity (Pleasant and Schlather, 1994). For C. album, the germination rate increases with incubation time. At low to medium incubation times, germination is higher with lactating cows’ diet, with no difference after 48 hours. The heightened microbial activity in lactating cows’ simulation likely accelerates seed coat scarification compared to heifers’ diet. As for E. crus-galli, germination is stimulated by rumen permanence, increasing with incubation time. This aligns with studies indicating that seed scarification induces germination in this weed species (Sung et al., 1987; Paudyal et al., 2021).
In an exception that confirms the rule, D. stramonium seed germination is significantly reduced compared to control, especially after prolonged rumen incubation. Brown and Bridglall (1987) found that seed scarification induces germination in D. stramonium, while Nikolić et al. (2022) demonstrated germination at extremely low pH values. The nature of the cattle rumen, with seeds immersed in rumen liquid, may explain this behavior, as certain species in this genus do not respond well to soaking (Reisman-Berman et al., 1989). Therefore, D. stramonium germination in the cattle digestive system may be more linked to the physical properties of the rumen than to chemical or microbiological factors.
Conversely, seeds with thinner seed coats, as exemplified by A. sterilis, and even L. multiflorum, experience rapid loss of germinability and viability when subjected to the digestive environment. The latter, however, retains a significantly greater proportion of germinable and viable seeds compared to A. sterilis. A closer look reveals that L. multiflorum seeds have slightly higher concentrations of fibrous compounds, coupled with smaller seed sizes, potentially contributing to their enhanced survival. Moreover, L. multiflorum, often used as forage, exhibits viable seeds found in cattle excrements, indicating potential adaptation to endozoochorous dispersal (Stanton et al., 2002). For seeds with thin seed coats like A. retroflexus and A. myosuroides, germinability remains high after short to medium exposure to microbial activity. Interestingly, both species exhibit higher germinability loss when subjected to the lactating cows’ diet than heifers. The higher lignin content in A. retroflexus seeds likely contributes to its survival. Additionally, peptides with antimicrobial properties, identified by Lipkin et al. (2005) in A. retroflexus seeds, could play a role in maintaining germinability. Despite lacking germination stimulation effects, A. retroflexus’s prolific seed production, coupled with herbicide-resistant populations, raises concerns about potential introductions and spread through cattle trade globally (McLachlan et al., 1995; Costea et al., 2004; Yanniccari et al., 2022). A. myosuroides seeds, possessing low lignin but higher concentrations of cellulose and hemicellulose, likely shield the embryo during low to medium exposure to microbial activity. Our findings align with Milotić and Hoffmann (2016), affirming similar effects on seed germinability post-cattle digestive system passage.
Finally, it is important to highlight some potential limitations of the current research. An important limitation might be related to the in vitro simulation of the animal digestion process, which cannot fully replicate the complex dynamics of digestion within the rumen, gastric, and intestinal compartments. In vivo, seeds move gradually through the gastrointestinal tract, following a Gaussian distribution, with some seeds excreted within a few hours and others much later. This is influenced by factors such as seed size and feed composition, where smaller seeds may travel faster and larger seeds may remain in the digestive system longer (Gardener et al., 1993; Lepková and Mašková, 2024).
On the other hand, the in vitro method exposes all seeds to the digestive factors for the same duration, potentially oversimplifying the digestive process. To address this limitation, we adopted different fermentation times (12, 24, and 48 hours) to simulate varying scenarios of seed fate within the rumen, aiming to closely simulate the real-life conditions.
Additionally, the conditions present in the rumen, such as microbial activity, temperature, and pH, which were replicated in vitro to measure germination and seed viability, may not perfectly match the variability and complexity of natural in vivo conditions. While these standardized measures help control experimental variables, they might not fully account for the dynamic and heterogeneous environment of the rumen during digestion and seed excretion.
Furthermore, although the results obtained are supported by the abundant literature available, it is important to clarify that the primary aim of this study was not to establish a strict correlation between the chemical composition of seeds and their degradation within the cattle rumen. While previous research highlights the importance of fibrous components—such as cellulose, hemicellulose, and lignin—in determining seed survival (Westerman and Gerowitt, 2013; Madureira et al., 2023), these factors alone are insufficient to explain the degradation process fully. This is because seed digestion is influenced by a complex interplay of multiple and specific traits, including seed size, coat thickness, degree of lignification, microfibrillar structure of fibrous compounds, and the relative proportions and anatomical distribution of hemicellulose, cellulose, and lignin (Bewley and Black, 1994; Moles and Westoby, 2004).
In addition, the presence of soluble fibers, such as pectin or beta-glucans, along with the imbibition capacity of seeds, plays a crucial role in determining their response to the digestive environment (Langenaeken et al., 2020; Choudhury et al., 2023). These traits often vary significantly between species and within species due to genetic and environmental factors. Therefore, while a correlation between specific fibrous compounds and seed germination or viability may be identified in studies focusing on a single species or closely related species (Lepková and Mašková, 2024), such correlations are unlikely to hold across a diverse set of weed species with differing seed morphologies and biochemical characteristics.
It is also worth noting that the ruminal degradation process is not purely chemical; it involves microbial activity, physical breakdown, and interactions with other feed components in the digestive matrix (Brice and Morrison, 1982; Blackshaw and Rode, 1991; Westerman and Gerowitt, 2013).
While our study does not seek to resolve this complexity, but to explore the survival of some agronomically important species, is still provides valuable insights into the general patterns and trends associated with weed seed survival in the rumen. Future research, focusing on single or similar species analyses, coupled with detailed biochemical profiling and controlled digestion trials, could elucidate the relationships between seed chemical composition and degradation outcomes.
This study reveals the intricate dynamics of weed seed germination and viability following passage through the cattle digestive system. Diverse behaviors were observed across weed species, emphasizing the influence of seed coat characteristics, microbial activity, and the compositional intricacies of cattle rumen fluid. The study underscores the significance of the chemical composition of seeds, particularly the content of lignin, cellulose, and hemicellulose in weed seed survival. However, it also highlights the complexity of natural systems, where exceptions exist and warrant further exploration.
Weed seed survival, and in some cases higher germination after passage through the cattle digestive system, raises concerns about the potential introduction of new weed species or resistant populations through cattle movement or trade. As noted in the introduction, grazing has historically been identified as a significant factor in the spread of weeds. Our findings reinforce this concern by demonstrating that seeds of certain weed species can survive passage through the digestive system of cattle and remain viable for extended periods. This highlights the potential for grazing cattle to ingest mature weed seeds in one location and deposit viable seeds in another via their feces, even after a delay of one or more days. The seasonal overlap between grazing periods and weed seed maturation exacerbates this risk, particularly given the species-specific variability in seed survival observed in our study.
Moreover, this dispersal mechanism is not limited to grazing systems. During the animal trade, cattle transported over both short and long distances may spread weed seeds, especially when they are fed with fodder sourced from their region of origin. This process could facilitate the introduction of weed populations into new locations. Importantly, the introduction of genetically distinct populations of the same species could promote interpopulation hybridization, potentially resulting in more aggressive and invasive weed variants. Our findings contribute valuable insights into the intricate interplay of factors affecting weed seed fate within the cattle digestive system. As weed management strategies evolve, understanding these nuanced dynamics becomes essential for mitigating potential risks associated with the spread of herbicide-resistant populations, ensuring sustainable agricultural practices.
Furthermore, this study provides a snapshot of weed seed behavior post-digestion in cattle. Additional research examining weed seeds in feed and manure is crucial to closing the cycle from field to field. Given the high adaptability potential and constant evolution of weed species, their seed behavior remains puzzling, even as we continue to assemble the pieces.
The raw data supporting the conclusions of this article will be made available by the authors, without undue reservation.
The animal study was approved by Ethical committee at the University of Padova (OPBA, approval number 1312041/2022). The study was conducted in accordance with the local legislation and institutional requirements.
NN: Conceptualization, Data curation, Formal analysis, Investigation, Methodology, Resources, Software, Validation, Writing – original draft. SM: Conceptualization, Data curation, Formal analysis, Investigation, Methodology, Resources, Validation, Writing – original draft. FT: Conceptualization, Funding acquisition, Resources, Supervision, Visualization, Writing – review & editing. SS: Conceptualization, Funding acquisition, Resources, Supervision, Visualization, Writing – review & editing. RM: Conceptualization, Funding acquisition, Project administration, Resources, Supervision, Visualization, Writing – review & editing.
The author(s) declare that financial support was received for the research and/or publication of this article. This work was supported by the PSR-Circular Farming, n. 5150328, Focus Area 4B.
The authors express their gratitude to Dr. Alberto Simonetto, for his assistance in collecting rumen fluid samples and throughout the trials.
The authors declare that the research was conducted in the absence of any commercial or financial relationships that could be construed as a potential conflict of interest.
All claims expressed in this article are solely those of the authors and do not necessarily represent those of their affiliated organizations, or those of the publisher, the editors and the reviewers. Any product that may be evaluated in this article, or claim that may be made by its manufacturer, is not guaranteed or endorsed by the publisher.
The Supplementary Material for this article can be found online at: https://www.frontiersin.org/articles/10.3389/fpls.2025.1483774/full#supplementary-material
Abati, J., Zucareli, C., Brzezinski, C. R., Lopes, I., de, O. N., Krzyzanowski, F. C., et al. (2022). Water absorption and storage tolerance of soybean seeds with contrasting seed coat characteristics. Acta Sci. - Agron. 44, 1–10. doi: 10.4025/actasciagron.v44i1.53096
Andrews, T. S. (1995). Dispersal of seeds of giant Sporobolus spp. after ingestion by grazing cattle. Aust. J. Exp. Agric. 35, 353–356. doi: 10.1071/EA9950353
Ankom Technology (2022). Method 8 – determining Acid Detergent Lignin in beakers. Ankom Technol. 1–3.
Anwar, M. P., Islam, A. K. M. M., Yeasmin, S., Rashid, M. H., Juraimi, A. S., Ahmed, S., et al. (2021). Weeds and their responses to management efforts in a changing climate. Agronomy 11, 1–20. doi: 10.3390/agronomy11101921
AOAC (2000). Official Methods of Analysis (Gaithersburg, MD, USA). Available online at: https://www.scirp.org/reference/ReferencesPapers?ReferenceID=1687699 (Accessed January 29, 2024).
AOAC (2016). Official Methods of Analysis (Washington). Available online at: https://www.techstreet.com/standards/official-methods-of-analysis-of-aoac-international-20th-edition-2016?product_id=1937367 (Accessed January 29, 2024).
Baskin, C. C., Baskin, J. M. (2014). “Ecologically meaningful germination studies,” in Seeds (Elsevier, Amsterdam, The Netherlands), 5–35. doi: 10.1016/B978-0-12-416677-6.00002-0
Biswas, P. K., Chakrabarti, A. G., Collins, H. A., Bettis, R. B. (1970). Histochemical studies in weed seed dormancy. Weed Sci. 18, 106–109. doi: 10.1017/S0043174500077444
Blackshaw, R. E., Rode, L. M. (1991). Effect of ensiling and rumen digestion by cattle on weed seed viability. Weed Sci. 39, 104–108. doi: 10.1017/s0043174500057957
Boisen, S., Fernández, J. A. (1997). Prediction of the total tract digestibility of energy in feedstuffs and pig diets by in vitro analyses. Animal Feed Science and Technology 68 (3–4), 277–286. doi: 10.1016/S0377-8401(97)00058-8
Borza, J. K., Westerman, P. R., Liebman, M. (2007). Comparing estimates of seed viability in three Foxtail (Setaria) species using the imbibed seed crush test with and without additional tetrazolium testing. Weed Technol. 21, 518–522. doi: 10.1614/WT-06-110
Brice, R. E., Morrison, I. M. (1982). The degradation of isolated hemicelluloses and lignin-hemicellulose complexes by cell-free, rumen hemicellulases. Carbohydr. Res. 101, 93–100. doi: 10.1016/S0008-6215(00)80797-1
Brown, N. A. C., Bridglall, S. S. (1987). Preliminary studies of seed dormancy in Datura stramonium. South Afr. J. Bot. 53, 107–109. doi: 10.1016/s0254-6299(16)32626-6
Bruun, H. H., Fritzbøger, B. (2002). The past impact of livestock husbandry on dispersal of plant seeds in the landscape of Denmark. Ambio 31, 425–431. doi: 10.1579/0044-7447-31.5.425
Brzezinski, C. R., Abati, J., Zucareli, C., Medri, C., Mertz-Henning, L. M., Krzyzanowski, F. C., et al. (2022). Structural analysis of soybean pods and seeds subjected to weathering deterioration in pre-harvest. Pesqui. Agropecu. Bras. 57, 1–10. doi: 10.1590/S1678-3921.PAB2022.V57.02697
Cardarelli, M., Woo, S. L., Rouphael, Y., Colla, G. (2022). Seed treatments with microorganisms can have a biostimulant effect by influencing germination and seedling growth of crops. Plants 11, 1–14. doi: 10.3390/plants11030259
Choudhury, A., Chandra, B., Viswavidyalaya, K., Bordolui, S. K., Chandra, B., Viswavidyalaya, K. (2023). Concept of seed deterioration: reason, factors, changes during deterioration and preventive measures to overcome seed degradation. Am. Int. J. Agric. Stud. 41–56. doi: 10.46545/aijas.v7i1.291
Costea, M., Weaver, S. E., Tardif, F. J. (2004). The biology of Canadian weeds. 130. Amaranthus retroflexus L., A. powellii S. Watson and A. hybridus L. Can. J. Plant Sci. 84, 631–668. doi: 10.4141/P02-183
Cudney, D., Wright, S., Shultz, T., Reints, J. (1992). Weed seed in dairy manure depends on collection site. Calif. Agric. 46, 31–32. doi: 10.3733/ca.v046n03p31
Davis, A. S., Schutte, B. J., Iannuzzi, J., Renner, K. A. (2008). Chemical and physical defense of weed seeds in relation to soil seedbank persistence. Weed Sci. 56, 676–684. doi: 10.1614/ws-07-196.1
De-Clerck-Floate, R. (1997). Cattle as dispersers of hound’s-tongue on rangeland in southeastern British Columbia. J. Range Manage. 50, 239–243. doi: 10.2307/4003722
Du, Y., Cui, B., Zhang, Q., Wang, Z., Sun, J., Niu, W. (2020). Effects of manure fertilizer on crop yield and soil properties in China: A meta-analysis. Catena 193, 1–10. doi: 10.1016/j.catena.2020.104617
Dubbern de Souza, F. H., Marcos-Filho, J. (2001). The seed coat as a modulator of seed-environment relationships in Fabaceae. Rev. Bras. Botânica 24, 365–375. doi: 10.1590/s0100-84042001000400002
Egley, G. H. (1989). Recent advances in the development and germination of seeds. Ed. Taylorson, R. B. (Boston, MA: Springer US). doi: 10.1007/978-1-4613-0617-7
Fuller, D. Q., Boivin, N. (2009). Crops, cattle and commensals across the Indian Ocean. Études Océan Indien 42–43, 13–46. doi: 10.4000/oceanindien.698
Gardener, C. J., McIvor, J. G., Jansen, A. (1993). Passage of legume and grass seeds through the digestive tract of cattle and their survival in faeces. J. Appl. Ecol. 30, 63. doi: 10.2307/2404271
Grgas, D., Rukavina, M., Bešlo, D., Štefanac, T., Crnek, V., Šikić, T., et al. (2023). The bacterial degradation of lignin—A Review. Water 15, 1272. doi: 10.3390/w15071272
Hall, M. B. (2000). “Starch Gelatinization & Hydrolysis Method,” in Neutral Detergent-Soluble Carbohydrates Nutritional Relevance and Analysis, vol. 29–32. (Gainesville, FL: University of Florida Extension - Institute of Food and Agricultural Sciences).
Hogan, J. P., Phillips, C. J. C. (2011). Transmission of weed seed by livestock: a review. Anim. Prod. Sci. 51, 391–398. doi: 10.1071/AN10141
Horowitz, M., Taylorson, R. B. (1985). Behaviour of hard and permeable seeds of Abutilon theophrasti Medic. (velvetleaf). Weed Res. 25, 363–372. doi: 10.1111/j.1365-3180.1985.tb00657.x
Huhtanen, P., Vanhatalo, A. (1997). Ruminal and total plant cell-wall digestibility estimated by a combined in situ method utilizing mathematical models. Br. J. Nutr. 78, 583–598. doi: 10.1079/BJN19970176
Humphries, T., Chauhan, B. S., Florentine, S. K. (2018). Environmental factors effecting the germination and seedling emergence of two populations of an aggressive agricultural weed; Nassella trichotoma. PloS One 13, 1–25. doi: 10.1371/journal.pone.0199491
Jarrar, H., El-Keblawy, A., Ghenai, C., Abhilash, P. C., Bundela, A. K., Abideen, Z., et al. (2023). Seed enhancement technologies for sustainable dryland restoration: Coating and scarification. Sci. Total Environ. 904, 166150. doi: 10.1016/j.scitotenv.2023.166150
Javaid, M. M., Mahmood, A., Alshaya, D. S., AlKahtani, M. D. F., Waheed, H., Wasaya, A., et al. (2022). Influence of environmental factors on seed germination and seedling characteristics of perennial ryegrass (Lolium perenne L.). Sci. Rep. 12, 1–11. doi: 10.1038/s41598-022-13416-6
Katovich, J., Becker, R., Doll, J. (1993). Weed seed survival in livestock systems Vol. 6 (University of Minnesota and University of Wisconsin: Univ. Minnesota). Available at: https://conservationdistrict.org/wp-content/uploads/umn-uw-ext-weed-seed-survival-in-livestock-systems.pdf (Acessed January 31, 2024).
Kinugasa, T., Hozumi, Y., Nishizima, H., Ishitobi, A., Miyawaki, M. (2016). Germination characteristics of early successional annual species after severe drought in the Mongolian steppe. J. Arid Environ. 130, 49–53. doi: 10.1016/j.jaridenv.2016.03.010
Krzyzanowski, F. C., França-Neto, J., de, B., Henning, F. A. (2023). Importance of the lignin content in the pod wall and seed coat on soybean seed physiological and health performances. J. Seed Sci. 45, 1–19. doi: 10.1590/2317-1545v45268562
Langenaeken, N. A., Ieven, P., Hedlund, E. G., Kyomugasho, C., van de Walle, D., Dewettinck, K., et al. (2020). Arabinoxylan, β-glucan and pectin in barley and malt endosperm cell walls: a microstructure study using CLSM and cryo-SEM. Plant J. 103, 1477–1489. doi: 10.1111/tpj.14816
Lee, S. K., Shin, W. J., Ahn, S., Kim, Y., Kim, J. T., Lee, E. J. (2021). Seed recovery and germination rate after gut passage by Korean water deer (Hydropotes inermis argyropus). Seed Sci. Res. 31, 311–318. doi: 10.1017/S0960258521000246
Lepková, B., Mašková, T. (2024). Seeds in the guts: can seed traits explain seed survival after being digested by wild ungulates? Oecologia 205, 49–58. doi: 10.1007/s00442-024-05538-7
Lipkin, A., Anisimova, V., Nikonorova, A., Babakov, A., Krause, E., Bienert, M., et al. (2005). An antimicrobial peptide Ar-AMP from amaranth (Amaranthus retroflexus L.) seeds. Phytochemistry 66, 2426–2431. doi: 10.1016/j.phytochem.2005.07.015
Liu, T., Wang, M., Awasthi, M. K., Chen, H., Awasthi, S. K., Duan, Y., et al. (2020). Measurement of cow manure compost toxicity and maturity based on weed seed germination. J. Clean. Prod. 245, 118894. doi: 10.1016/j.jclepro.2019.118894
Lowry, A. A. (1996). Influence of ruminant digestive processes on germination of ingested seeds (Dept Rangel. Resour. Oregon State Univ). Available at: https://ir.library.oregonstate.edu/concern/graduate_thesis_or_dissertations/v405sg230?locale=en (Acessed 01,31,2024).
Madureira, A., Molinari, M. D. C., Marin, S. R. R., Pagliarini, R. F., Henning, F. A., Nepomuceno, A. L., et al. (2023). Correlations between lignin content and related genes, weathering deterioration, and soybean seed quality at pre-harvest. J. Crop Sci. Biotechnol. 26, 489–497. doi: 10.1007/s12892-023-00193-5
Makkar, H. P. S. (2018). Review: Feed demand landscape and implications of food-not feed strategy for food security and climate change. Animal 12, 1744–1754. doi: 10.1017/S175173111700324X
Malo, J. E., Suárez, F. (1995). Establishment of pasture species on cattle dung: the role of endozoochorous seeds. J. Veg. Sci. 6, 169–174. doi: 10.2307/3236211
Manonmani, V., Vanangamudi, K., Vinaya Rai, R. S. (1996). Effect of seed size on seed germination and vigour in Pongamia pinnata. J. Trop. For. Sci. 9, 1–5.
Materechera, S. A., Modiakgotla, L. N. (2006). Cattle manure increases soil weed population and species diversity in a semi-arid environment. South Afr. J. Plant Soil 23, 21–28. doi: 10.1080/02571862.2006.10634725
McLachlan, S. M., Murphy, S. D., Tollenaar, M., Weise, S. F., Swanton, C. J. (1995). Light limitation of reproduction and variation in the allometric relationship between reproductive and vegetative biomass in Amaranthus retroflexus (Redroot Pigweed). J. Appl. Ecol. 32, 157. doi: 10.2307/2404425
Menke, K. H., Steingass, H. (1988). Estimation of the energetic feed value obtained from chemical analysis and in vitro gas production using rumen fluid. Anim. Res. Dev. 28, 7–55.
Milotić, T., Hoffmann, M. (2016). Reduced germination success of temperate grassland seeds sown in dung: consequences for post-dispersal seed fate. Plant Biol. 18, 1038–1047. doi: 10.1111/plb.12506
Mohamed-Yasseen, Y., Barringer, S. A., Splittstoesser, W. E., Costanza, S. (1994). The role of seed coats in seed viability. Bot. Rev. 60, 426–439. doi: 10.1007/BF02857926
Moles, A. T., Westoby, M. (2004). Seedling survival and seed size: A synthesis of the literature. J. Ecol. 92, 372–383. doi: 10.1111/j.0022-0477.2004.00884.x
Muralidhara, B. M., Reddy, Y. T. N., Srilatha, V., Akshitha, H. J. (2016). Effect of seed coat removal treatments on seed germination and seedling attributes in Mango varieties. Int. J. Fruit Sci. 16, 1–9. doi: 10.1080/15538362.2015.1021885
Nikolić, N., Massaro, S., Tagliapietra, F., Squartini, A., Schiavon, S., Masin, R. (2024). Method to evaluate the microbial degradation activity in silage, cow rumen with in vitro test, and in manure and slurry. MethodsX 12, 102550. doi: 10.1016/j.mex.2024.102550
Nikolić, N., Šoštarčić, V., Pismarović, L., Šćepanović, M., Masin, R. (2022). Germination response of Datura stramonium L. @ to different pH and salinity levels under different temperature conditions. Plants 11, 1–12. doi: 10.3390/plants11233259
Nikolić, N., Squartini, A., Concheri, G., Stevanato, P., Zanin, G., Masin, R. (2020). Weed seed decay in no-till field and planted riparian buffer zone. Plants 9, 293. doi: 10.3390/plants9030293
Ocumpaugh, W. R., Swakon, D. H. D. (1993). Simulating grass seed passage through the digestive system of cattle: A laboratory technique. Crop Sci. 33, 1084–1090. doi: 10.2135/CROPSCI1993.0011183X003300050041X
Odintsova, T. I., Rogozhin, E. A., Baranov, Y., Musolyamov, A. K., Yalpani, N., Egorov, T. A., et al. (2008). Seed defensins of barnyard grass Echinochloa crus-galli (L.) Beauv. Biochimie 90, 1667–1673. doi: 10.1016/j.biochi.2008.06.007
Oveisi, M., Ojaghi, A., Rahimian Mashhadi, H., Müller-Schärer, H., Reza Yazdi, K., Pourmorad Kaleibar, B., et al. (2021). Potential for endozoochorous seed dispersal by sheep and goats: Risk of weed seed transport via animal faeces. Weed Res. 61, 1–12. doi: 10.1111/wre.12461
Paudyal, P. S., Limbu, S., Das, D., Paudel, N., Rahman, M. M. (2021). Effect of mechanical scarification on seed germination of selected weeds occurring in rice field. J. Agric. Food Environ. 02, 37–43. doi: 10.47440/JAFE.2021.2207
Platis, P., Damalas, C. A., Koutroubas, S. D. (2023). Weed flora composition in a young olive orchard as affected by fertilizer types. Phytoparasitica 51, 1133–1146. doi: 10.1007/s12600-023-01090-x
Pleasant, J., Schlather, K. (1994). Incidence of Weed Seed in Cow (Bos sp.) Manure and its importance as a weed source for cropland Author (s): Jane Mt. Pleasant and Kenneth J. Schlather Published by : Cambridge University Press on behalf of the Weed Science Society of America Stable. Weed Technol. 8, 304–310. doi: 10.1017/S0890037X00038823
Polo, C. C., Pereira, L., Mazzafera, P., Flores-Borges, D. N. A., Mayer, J. L. S., Guizar-Sicairos, M., et al. (2020). Correlations between lignin content and structural robustness in plants revealed by X-ray ptychography. Sci. Rep. 10, 1–11. doi: 10.1038/s41598-020-63093-6
Prado, J., Ribeiro, H., Alvarenga, P., Fangueiro, D. (2022). A step towards the production of manure-based fertilizers: Disclosing the effects of animal species and slurry treatment on their nutrients content and availability. J. Clean. Prod. 337, 130369. doi: 10.1016/j.jclepro.2022.130369
Rahimi, S., Mashhadi, H. R., Banadaky, M. D., Mesgaran, M. B. (2016). Variation in weed seed fate fed to different holstein cattle groups. PloS One 11, 1–15. doi: 10.1371/journal.pone.0154057
Ranganathan, U., Groot, S. P. C. (2023). Seed longevity and deterioration. Seed Sci. Technol. Biol. Prod. Qual. 91–108. doi: 10.1007/978-981-19-5888-5_5
Reisman-Berman, O., Kigel, J., Rubin, B. (1989). Short soaking in water inhibits germination of Datura ferox L. and D. stramonium L. seeds. Weed Res. 29, 357–363. doi: 10.1111/j.1365-3180.1989.tb01306.x
Rogozhin, E. A., Ryazantsev, D. Y., Grishin, E. V., Egorov, T. A., Zavriev, S. K. (2012). Defense peptides from barnyard grass (Echinochloa crus-galli L.) seeds. Peptides 38, 33–40. doi: 10.1016/j.peptides.2012.08.009
RStudio Team (2023). RStudio | Open source & professional software for data science teams - RStudio (RStudio Inc). Available online at: https://rstudio.com/ (Accessed February 10, 2021).
Rubenstein, J. M., Hulme, P. E., Buddenhagen, C. E., Rolston, M. P., Hampton, J. G. (2021). Weed seed contamination in imported seed lots entering New Zealand. PloS One 16, 1–13. doi: 10.1371/journal.pone.0256623
Salazar-Rivera, G. I., Sánchez-Velásquez, L. R., Inzunza, E. R. (2020). Passage through a bird’s gut confers a germination head start to the seeds of the Nightshade Witheringia stramoniifolia. Trop. Conserv. Sci. 13, 1–8. doi: 10.1177/1940082920931093
Schröder, J. (2005). Revisiting the agronomic benefits of manure: A correct assessment and exploitation of its fertilizer value spares the environment. Bioresour. Technol. 96, 253–261. doi: 10.1016/j.biortech.2004.05.015
Schröder, J., Dilz, K. (1987). “Cattle slurry and farmyard manure as fertilizers for forage maize,” in Animal Manure on Grassland and Fodder Crops. Fertilizer or Waste? (Springer Netherlands, Dordrecht), 137–156. doi: 10.1007/978-94-009-3659-1_9
Song, G., Liu, S., Jiang, X., Gong, S., Hao, W., Cui, Y., et al. (2024). Seasonal dynamics of seed dormancy and germination in the weed Diplachne fusca. PeerJ 12, 1–16. doi: 10.7717/peerj.17987
Spanghero, M., Boccalon, S., Gracco, L., Gruber, L. (2003). NDF degradability of hays measured in situ and in vitro. Anim. Feed Sci. Technol. 104, 201–208. doi: 10.1016/S0377-8401(02)00327-9
Stanton, R., Piltz, J., Pratley, J., Kaiser, A., Hudson, D., Dill, G. (2002). Annual ryegrass (Lolium rigidum) seed survival and digestibility in cattle and sheep. Aust. J. Exp. Agric. 42, 111–115. doi: 10.1071/EA01069
Sung, S.-J., Leather, G., Maynard, H. (1987). Development and germination of Barnyardgrass (Echinochloa crus-galli) Seeds. Weed Sci. 35, 211–215. doi: 10.1017/S0043174500079078
Tagliapietra, F., Cattani, M., Hindrichsen, I. K., Hansen, H. H., Colombini, S., Bailoni, L., et al. (2012). True dry matter digestibility of feeds evaluated in situ with different bags and in vitro using rumen fluid collected from intact donor cows. Anim. Prod. Sci. 52, 338–346. doi: 10.1071/AN11206
Talbot, J. M., Treseder, K. K. (2012). Interactions among lignin, cellulose, and nitrogen drive litter chemistry — decay relationships Author (s): Jennifer M. Talbot and Kathleen K. Treseder Published by : Wiley on behalf of the Ecological Society of America Stable URL : https://www.jsto. Ecology 93, 345–354. doi: 10.1890/11-0843.1
Van Soest, P. J., Robertson, J. B., Lewis, B. A. (1991). Methods for dietary fiber, neutral detergent fiber, and nonstarch polysaccharides in relation to animal nutrition. J. Dairy Sci. 74, 3583–3597. doi: 10.3168/jds.S0022-0302(91)78551-2
Van Soest, P. J., Wine, R. H. (1967). Use of detergents in the analysis of fibrous feeds. IV. determination of plant cell-wall constituents. J. AOAC Int. 50, 50–55. doi: 10.1093/jaoac/50.1.50
Vogel, K. P., Pedersen, J. F., Masterson, S. D., Toy, J. J. (1999). Evaluation of a filter bag system for NDF, ADF, and IVDMD forage analysis. Crop Sci. 39, 276–279. doi: 10.2135/cropsci1999.0011183X003900010042x
Weakley, D. C., Stern, M. D., Satter, L. D. (1983). Factors affecting disappearance of feedstuffs from bags suspended in the rumen. J. Anim. Sci. 56, 493–507. doi: 10.2527/JAS1983.562493X
Westerman, P. R., Gerowitt, B. (2013). Weed seed survival during anaerobic digestion in biogas plants. Bot. Rev. 79, 281–316. doi: 10.1007/s12229-013-9118-7
Wilson, C. E., Castro, K. L., Thurston, G. B., Sissons, A. (2016). Pathway risk analysis of weed seeds in imported grain: A Canadian perspective. NeoBiota 30, 49–74. doi: 10.3897/neobiota.30.7502
Keywords: germinability, ruminant digestion, viability, weed seeds, in vitro
Citation: Nikolić N, Massaro S, Tagliapietra F, Schiavon S and Masin R (2025) Post digestion weed seed survival in cattle. Front. Plant Sci. 16:1483774. doi: 10.3389/fpls.2025.1483774
Received: 20 August 2024; Accepted: 28 February 2025;
Published: 18 March 2025.
Edited by:
Jordi Izquierdo Figarola, Polytechnic University of Catalonia, SpainReviewed by:
Simerjeet Kaur, Punjab Agricultural University, IndiaCopyright © 2025 Nikolić, Massaro, Tagliapietra, Schiavon and Masin. This is an open-access article distributed under the terms of the Creative Commons Attribution License (CC BY). The use, distribution or reproduction in other forums is permitted, provided the original author(s) and the copyright owner(s) are credited and that the original publication in this journal is cited, in accordance with accepted academic practice. No use, distribution or reproduction is permitted which does not comply with these terms.
*Correspondence: Selene Massaro, c2VsZW5lLm1hc3Nhcm9AcGhkLnVuaXBkLml0
Disclaimer: All claims expressed in this article are solely those of the authors and do not necessarily represent those of their affiliated organizations, or those of the publisher, the editors and the reviewers. Any product that may be evaluated in this article or claim that may be made by its manufacturer is not guaranteed or endorsed by the publisher.
Research integrity at Frontiers
Learn more about the work of our research integrity team to safeguard the quality of each article we publish.