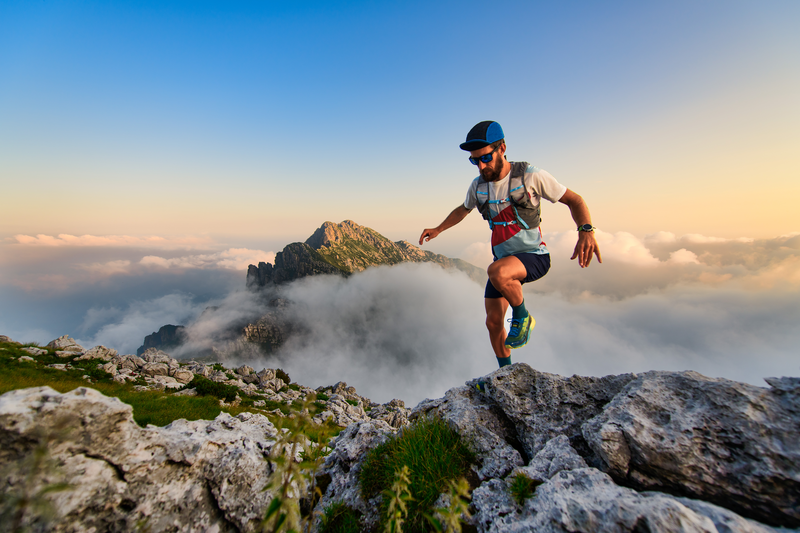
94% of researchers rate our articles as excellent or good
Learn more about the work of our research integrity team to safeguard the quality of each article we publish.
Find out more
EDITORIAL article
Front. Plant Sci. , 10 January 2025
Sec. Plant Abiotic Stress
Volume 15 - 2024 | https://doi.org/10.3389/fpls.2024.1550257
This article is part of the Research Topic Surviving and Thriving: How Crops Perceive and Respond to Temperature Stress View all 13 articles
Editorial on the Research Topic
Surviving and thriving: how crops perceive and respond to temperature stress
Climate change-driven temperature fluctuations pose a significant threat to crop productivity, emphasizing the crucial need to elucidate how plants mitigate temperature stress-induced cellular damage to ensure agricultural sustainability and food security (Jin et al., 2024; Ding and Yang, 2022; Shi et al., 2018, 2015; Zhu, 2016). In this Research Topic, “Surviving and Thriving: How Crops Perceive and Respond to Temperature Stress,” 12 original research articles have presented new and valuable insights into the genetic basis of temperature extremes (e.g. cold/freezing and/or high heat) in a wide range of cereal (e.g. wheat, rice, pearl millet), vegetable (i.e. pepper), legume (e.g. soybean), and fiber (e.g. cotton) crops, paving the way for enhancing crop resilience and contributing to global food security, with potential biotechnological applications ranging from textiles to biofuels. We briefly summarize these contributions below.
Spring frost poses a serious threat to wheat production and grain quality, however, the molecular mechanisms conferring frost tolerance remain poorly understood. By taking advantage of a frost-tolerant wheat cultivar and subjecting it to freezing stress at the meiotic stage, Yao et al. revealed that key phenotypic changes (e.g. reduction in plant height, seed setting rate, and cell wall thickening in the stem vascular tissue) coincide with transcriptomic changes impacting hormone signaling, cell wall biosynthesis, and transcription factor regulation pathways.
By leveraging comparative transcriptomic analysis of chilling stress and recovery in resistant and susceptible rice cultivars, Zhang et al. successfully inferred various time-series weighted gene co-expression networks associated with chilling resistance and susceptibility. Several biological processes, including abscisic acid responses, water deprivation responses, protein metabolism, and transcription regulation, were significantly enriched. Using machine learning methods, five genes (e.g. C-repeat binding factor, OsCBF3) were further prioritized to be pivotal for chilling resistance in rice.
Genome-wide analysis of the plant-specific BREVIS RADIX (BRX) family in upland cotton revealed 12, 6, and 6 BRX candidate genes in Gossypium hirsutum, G. raimondii and G. arboreum, respectively (Wei et al.). Chromosomal localization and collinearity analysis suggest that segmental duplications were the primary driver of BRX gene amplification. Silencing of abiotic-stress responsive GhBRX.1, GhBRX.2, and GhBRXL4.3 further confirmed their pivotal roles in plant tolerance to salt and low-temperature stress, extending their function beyond root and shoot growth (Beuchat et al., 2010).
Plant-specific TIFY transcription factors (TFs), characterized by a conserved TIFY domain, are known to regulate growth, development, and stress tolerance (Vanholme et al., 2007). In this Research Topic, Wang et al. identified 16 TIFY plant transcription family candidate genes dispersed across seven subgroups in pepper (Capsicum annuum L.). In particular, CaTIFY7 and CaTIFY10b were highly induced in leaves during cold stress. Knockdown of CaTIFY7 and CaTIFY10b compromised cold tolerance, while their overexpression enhanced it, by influencing many cold-responsive and reactive oxygen species (ROS)-related genes.
Ding et al. investigated the impact of five physiologically relevant day/night temperature regimes in two thermotolerant and thermosensitive genotypes. A comprehensive evaluation of physiological, biochemical, and genetic analyses revealed that 35/27°C (T4) was the optimal regime for growth and yield in their study system. At this temperature, plants showed enhanced photosynthesis, antioxidant activity, and upregulation of key heat stress-responsive genes.
Singh et al. performed a multi-tissue comparative transcriptomic analysis and revealed distinct genotype (tolerant vs sensitive)- and tissue (root vs leaf)-specific heat-responsive expression. Enrichment of heat shock proteins (HSPs), ROS scavenging, diverse TF families, and secondary metabolism pathways were observed. Interestingly, foxtail millet shared a high degree of collinearity with pearl millet in specific heat-responsive HSP and TF genes, compared to other cereal grains.
Fábián et al. used cutting-edge histochemical and transcriptomic methods to compare the responses of heat-tolerant and heat-sensitive wheat cultivars to heat stress during male meiosis. Their study revealed pivotal structural and temporal alterations of wheat male meiocytes and tapetal cells and their transcriptional responses triggered by meiosis-timed heat stress. Notably, the heat-tolerant cultivar maintained cytoskeletal integrity and upregulated genes involved in stress response and meiosis, whereas the heat-sensitive cultivar displayed cytoskeletal disruption, impaired meiotic division, and aberrant tapetum development.
Growth-regulating factors (GRFs) are a plant-specific TF family with diverse roles including regulation of plant growth under stress conditions (Omidbakhshfard et al., 2015). In this Research Topic, 12 candidate GRF genes were identified in potatoes (Cui et al.) A systematic analysis of StGRF promoter regions identified numerous cis-acting elements associated with plant growth, development, abiotic stress, and hormone responses. Transcriptomic data supported the tissue-specific expression (e.g. stamens, roots, and young tubers) and differential expression of StGRFs under abiotic/biotic stress (e.g. heat and Phytophthora infestans infection) and hormone treatment.
In plants, mitogen-activated protein kinase (MAPK) modules (MAPKKK-MAPKK-MAPK) are activated by various environmental cues, including heat and cold stress, triggering a cascade of cellular signaling events (Bigeard and Hirt, 2018; Manna et al., 2023) In this Research Topic, Zhu et al.; Zhu et al. screened MAPK and MAPKK gene expression in potato (Solanum tuberosum) plants cultivated under mild (30°C) and/or acute (35°C) heat stress conditions, revealing that StMAPK1 and StMAPKK5 exhibited highly induced and sustained expression profiles. Overexpression of StMAPK1 modified photosynthesis and preserves membrane stability in response to heat stress by inducing the expression of heat stress genes (StHSP90, StHSP70, StHSP20, and StHSFA3) and regulating multiple redox balance and turgor maintenance pathways. Similarly, StMAPKK5 overexpression/silencing significantly affected both morphological (plant weight/height) and physiological (photosynthesis, transpiration) traits under heat stress by modulating various stress-responsive and antioxidant enzyme genes and associated biochemical defenses.
Plant GATA (TFs) regulate diverse developmental processes and organ development (e.g. photomorphogenesis, chlorophyll biosynthesis, stomata formation), however, their role in abiotic stress remains relatively unexplored in plants (Schwechheimer et al., 2022). Zhu et al. screened over 50 potato GATA transcription factor (TF) genes for heat stress response and found that StGATA2 was among the few exhibiting strong and prolonged induction. A deeper understanding of autophagy-related genes (ATG) in potatoes were also gained in this Research Topic (Zhu et al.). A systematic analysis of phylogenetic relationships, chromosome distribution, gene structure, interspecific collinearity, and organ- (e.g. leaf, flower, stem, root, stolon, petiole, and tuber) and stress- (i.e. heat) responsive expression was performed on six putative ATG18 subfamily members identified in the potato genome. StATG18a emerged to be highly induced by heat stress in several organs. StGATA2- and StATG18a-overexpression also elicited comparable phenotypic, biochemical, and genetic responses to heat stress atypical to transgenic potato lines overexpressing StMAPK1 and StMAPKK5.
ZW: Writing – original draft, Writing – review & editing. YW: Writing – review & editing. DW: Writing – review & editing.
The author(s) declare financial support was received for the research, authorship, and/or publication of this article. This Research Program was financially supported by The Research Program Sponsored by State Key Laboratory of Aridland Crop Science, Gansu Agricultural University (No. GSCS-2022-03 and GSCS-2023-04); The Undergraduate Scientific Research Training (SIETP) Program (202411034 and 202411035); the National Natural Science Foundation of China (32260518); and The Scientific Research Start-up Fund by Gansu Agricultural University (GAU-KYQD-2019-06 and GAUKYQD2020-26).
The authors declare that the research was conducted in the absence of any commercial or financial relationships that could be construed as a potential conflict of interest.
The author(s) declared that they were an editorial board member of Frontiers, at the time of submission. This had no impact on the peer review process and the final decision.
All claims expressed in this article are solely those of the authors and do not necessarily represent those of their affiliated organizations, or those of the publisher, the editors and the reviewers. Any product that may be evaluated in this article, or claim that may be made by its manufacturer, is not guaranteed or endorsed by the publisher.
Beuchat, J., Scacchi, E., Tarkowska, D., Ragni, L., Strnad, M., Hardtke, C. S. (2010). BRX promotes Arabidopsis shoot growth. New Phytol. 188, 23–29. doi: 10.1111/j.1469-8137.2010.03387.x
Bigeard, J., Hirt, H. (2018). Nuclear signaling of plant MAPKs. Front. Plant Sci. 9. doi: 10.3389/fpls.2018.00469
Ding, Y., Yang, S. (2022). Surviving and thriving: How plants perceive and respond to temperature stress. Dev. Cell 57, 947–958. doi: 10.1016/j.devcel.2022.03.010
Jin, X., Wang, Z., Li, X., Ai, Q., Wong, D. C. J., Zhang, F., et al. (2024). Current perspectives of lncRNAs in abiotic and biotic stress tolerance in plants. Front. Plant Sci. 14. doi: 10.3389/fpls.2023.1334620
Manna, M., Rengasamy, B., Sinha, A. K. (2023). Revisiting the role of MAPK signalling pathway in plants and its manipulation for crop improvement. Plant Cell Environ. 46, 2277–2295. doi: 10.1111/pce.14606
Omidbakhshfard, M. A., Proost, S., Fujikura, U., Mueller-Roeber, B. (2015). Growth-regulating factors (GRFs): A small transcription factor family with important functions in plant biology. Mol. Plant 8, 998–1010. doi: 10.1016/j.molp.2015.01.013
Schwechheimer, C., Schröder, P. M., Blaby-Haas, C. E. (2022). Plant GATA factors: their biology, phylogeny, and phylogenomics. Annu. Rev. Plant Biol. 73, 123–148. doi: 10.1146/annurev-arplant-072221-092913
Shi, Y., Ding, Y., Yang, S. (2015). Cold signal transduction and its interplay with phytohormones during cold acclimation. Plant Cell Physiol. 56, 7–15. doi: 10.1093/pcp/pcu115
Shi, Y., Ding, Y., Yang, S. (2018). Molecular regulation of CBF signaling in cold acclimation. Trends Plant Sci. 23, 623–637. doi: 10.1016/j.tplants.2018.04.002
Vanholme, B., Grunewald, W., Bateman, A., Kohchi, T., Gheysen, G. (2007). The tify family previously known as ZIM. Trends Plant Sci. 12, 239–244. doi: 10.1016/j.tplants.2007.04.004
Keywords: abiotic & biotic stress, transcript factor, tolerance, extreme temperature stress, crop
Citation: Wang Z, Wang Y and Wong DCJ (2025) Editorial: Surviving and thriving: how crops perceive and respond to temperature stress. Front. Plant Sci. 15:1550257. doi: 10.3389/fpls.2024.1550257
Received: 23 December 2024; Accepted: 30 December 2024;
Published: 10 January 2025.
Edited and Reviewed by:
Luisa M. Sandalio, Spanish National Research Council (CSIC), SpainCopyright © 2025 Wang, Wang and Wong. This is an open-access article distributed under the terms of the Creative Commons Attribution License (CC BY). The use, distribution or reproduction in other forums is permitted, provided the original author(s) and the copyright owner(s) are credited and that the original publication in this journal is cited, in accordance with accepted academic practice. No use, distribution or reproduction is permitted which does not comply with these terms.
*Correspondence: Zemin Wang, d3ptNDA2NDMzMjU0QDEyNi5jb20=
Disclaimer: All claims expressed in this article are solely those of the authors and do not necessarily represent those of their affiliated organizations, or those of the publisher, the editors and the reviewers. Any product that may be evaluated in this article or claim that may be made by its manufacturer is not guaranteed or endorsed by the publisher.
Research integrity at Frontiers
Learn more about the work of our research integrity team to safeguard the quality of each article we publish.