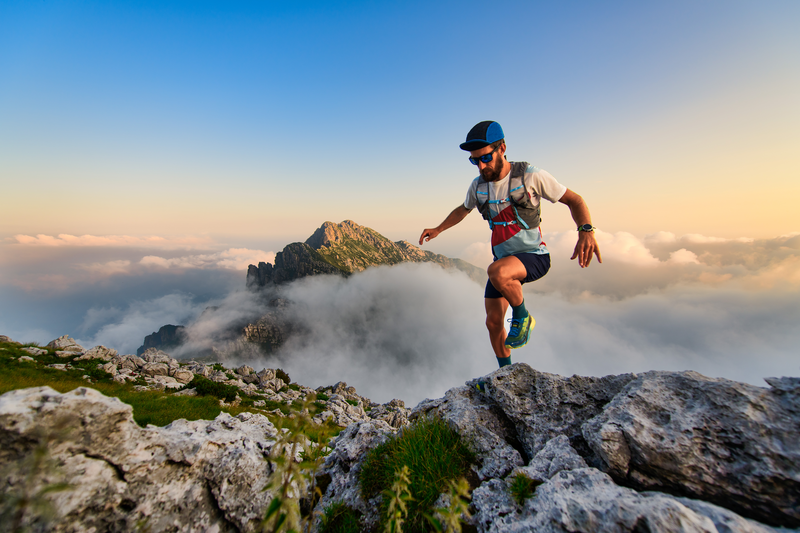
95% of researchers rate our articles as excellent or good
Learn more about the work of our research integrity team to safeguard the quality of each article we publish.
Find out more
ORIGINAL RESEARCH article
Front. Plant Sci. , 21 January 2025
Sec. Functional Plant Ecology
Volume 15 - 2024 | https://doi.org/10.3389/fpls.2024.1531654
This article is part of the Research Topic Ecophysiological Traits-Based Community Assembly and Maintenance of Ecosystem Functioning in Tropical Rainforests View all 10 articles
Understanding the mechanisms governing biodiversity-biomass relationships across temporal and spatial scales is essential for elucidating how abiotic and biotic factors influence ecosystem function in natural forests. However, the simultaneous contributions of multiple abiotic (e.g., topography) and biotic factors (e.g., structural diversity) to aboveground biomass dynamics (ΔAGB) over time and across habitat types remain inadequately understood. To address this gap, we evaluated changes in aboveground biomass across a decade and various habitats, disentangling the relative influences of topography and multidimensional diversity on ΔAGB through datasets from forest inventories conducted between 2007 and 2017, along with phylogenetic relatedness, functional traits, and environmental variables from a subtropical forest in China. Our findings indicate that aboveground biomass at community level experienced a significant decline followed by an increase over the decade, predominantly driven by changes in the low-valley habitat. In contrast, no statistically significant alterations were detected in the aboveground biomass of mid-hillside and high-ridge habitats. Furthermore, the determinants of ΔAGB exhibited temporal variation. During the 2007-2012 period, ΔAGB was primarily influenced by functional and structural diversity, accounting for 66.11% and 21.35% of relative importance, respectively. In the subsequent 2012-2017 period, phylogenetic and structural diversity emerged as key factors, explaining 48.46% and 36.43% of relative importance, respectively. Additionally, we observed that the drivers and effects impacting ΔAGB exhibited significant variability across different habitat types. In summary, our study underscores the significant spatiotemporal dependence of abiotic and biotic drivers on biomass dynamics within forest ecosystems, thereby enhancing our understanding of the complex biodiversity-ecosystem functioning relationships.
Anthropogenic global climate change and habitat destruction have exacerbated biodiversity loss worldwide (Synes et al., 2020; Richardson et al., 2023), resulting in irreversible negative impacts on species coexistence, services and functions of ecosystems (Isbell et al., 2017; He et al., 2024). Given that forests are indispensable to the worldwide carbon cycle and maintenance of carbon neutrality (Canadell and Raupach, 2008; Pan et al., 2011), the biodiversity-ecosystem functioning (BEF) relationships in forest communities have garnered considerable attention and pose a significant challenge in ecology (Gamfeldt et al., 2013; Liang et al., 2016; Ray et al., 2023; Zemp et al., 2023). Theoretically, a positive BEF relationship is expected when biodiversity promotes niche complementarity (i.e. the complementary effect) or the average competitive ability of species (i.e. the positive selection effect) (Lasky et al., 2014). Alternatively, a negative BEF relationship may occur if increased biodiversity results in a decrease of the average competitive ability of species (i.e. the negative selection effect) (Huston, 1997; Tilman, 1999). However, the relationship between biodiversity and aboveground biomass (AGB) within forest communities is complex and context-dependent, and potentially varying over time and across spatial scales (Cardinale et al., 2007; Reich et al., 2012; Forrester and Bauhus, 2016; Gottschall et al., 2022). It remains ambiguous how multiple abiotic and biotic factors simultaneously contribute to aboveground biomass dynamics (ΔAGB) over temporal scales and across various habitat types.
Considering multiple dimensions of biodiversity concurrently is able to facilitate a comprehensive and accurate insight into the ecological mechanism underlying BEF relationships (Cadotte et al., 2009; Srivastava et al., 2012; Wang et al., 2024). Previous researchers have already investigated how species and functional diversity influence AGB, revealing that species richness alone may inadequately capture the ecological differences or similarities among species (van der Sande et al., 2017; Yuan et al., 2019). In comparison to species diversity, functional diversity plays a more crucial role in ecosystem functions by reflecting a suite of core attributes essential for plant growth and reproduction within a community (Petchey and Gaston, 2002; Lian et al., 2022). A higher diversity of traits related to resource uptake enables a community to utilize resources more effectively, with resource use complementarity serving as an underlying mechanism linking functional diversity to ecosystem function (Fotis et al., 2018; Chen et al., 2023).
Structural diversity and phylogenetic diversity have been recognized as critical drivers of ecosystem functions (Dănescu et al., 2016; Yuan et al., 2018; Yang et al., 2024), as they offer insights into resource use efficiency and evolutionary history in forest communities, respectively (Cadotte et al., 2009; Zhu et al., 2021). Notably, due to its capacity to estimate both the actual volumetric occupancy and arrangement within niche spaces, stand structure is increasingly acknowledged in the context of the BEF relationship (Ali, 2019; Chen et al., 2023). Although it has been demonstrated that changes in BEF relationships may arise from the complementary trends in resource use strategies among species over time (Huang et al., 2018; Zheng et al., 2024), our understanding of how multiple dimensions of biodiversity contribute to ΔAGB over time remains limited. In the context of climate change, exploring the impact of multidimensional biodiversity on ΔAGB across temporal scales in natural forests is essential for elucidating the variation in biodiversity effects (Kardol et al., 2018).
The critical role of abiotic factors in shaping BEF relationships has been extensively examined (Ferry et al., 2010; McEwan et al., 2011; Quesada et al., 2012; Werner and Homeier, 2015). The “multivariate productivity-diversity hypothesis” posits that environmental conditions indirectly influence community productivity by affecting species diversity, thereby providing a theoretical framework for understanding spatial variation in BEF relationships (Cardinale et al., 2009). Habitat types comprehensively reflect topographic factors (e.g., elevation, slope, aspect, and convexity), which mediate microclimates and soil nutrients (Man et al., 2011; Lin et al., 2012), thus potentially impacting the ΔAGB of forests both directly or indirectly (McEwan et al., 2011; Lin et al., 2012; Zhang et al., 2024). For instance, certain habitat types such as ridges and steep slopes may experience periodic water stress, poor soil nutrients availability, and strong winds, where only species with stress-tolerant life history strategies can thrive (Paoli, 2006; Tanner et al., 2014). Distinct environmental factors across various habitats influence the species composition of plant communities as well as the growth performance of species, which in turn indirectly affect the AGB within forest communities (Chadwick and Asner, 2016; Jucker et al., 2018). Therefore, we argue that considering habitat types could further elucidate the spatial variation in diversity-ΔAGB relationships.
Subtropical forests, despite their relatively limited global distribution (Fang et al., 2001), rank second only to tropical forests in terms of species richness and serve as a significant carbon sink on the earth (Houghton, 2005; Piao et al., 2009; Li et al., 2019). They are essential in the worldwide carbon cycle and climate regulation (Yu et al., 2014). The forest within the Gutianshan National Nature Reserve exemplifies typical subtropical evergreen broad-leaved mature forest of China (Jiang et al., 2022), and large sustained forest dynamics monitoring plots with systematic vegetation inventories here facilitate the critical framework for linking abiotic and biotic drivers of carbon dynamics to spatiotemporal variation (Lin et al., 2012; Mi et al., 2021). To evaluate the potential contributions of multiple abiotic and biotic factors to ΔAGB over time and across habitat types in the subtropical forest, we examined the changes of AGB over time and across habitat types, disentangling the comparative impacts of these factors on ΔAGB across different temporal periods and habitat types. Specifically, we focused on the following three questions: (1) How did the AGB vary over time and across habitats during the past decade? (2) How did the abiotic and biotic determinants and their influences on ΔAGB differ across temporal periods? and (3) how did they vary across different habitat types?
Our study was conducted within the Gutianshan National Nature Reserve in Quzhou City, Zhejiang Province, southeastern China, with a total area of 8107 hectares. The reserve is distinguished by its subtropical humid monsoon climate, exhibiting an average annual temperature of 15.3 °C and an average annual precipitation of 1963.7 mm (Yu et al., 2001). The predominant soil types in the area comprise red soil, yellow-red soil, red-yellow soil, as well as swamp soil, with a pH ranging mostly between 5.5 and 6.5. The evergreen broad-leaved forest, dominated by Castanopsis eyrei and Schima superba, is the main vegetation type in Gutianshan, commonly found below 800 meters and characterized as typical subtropical zonal vegetation (Yu et al., 2001; Legendre et al., 2009).
The 5-ha forest plot was established in 2002 according to the standard of the CTFS-ForestGEO protocol. The plot spans 200 meters in an east-west direction and 250 meters in a north-south orientation, containing two hillsides on the northern and southern sides and a valley in the middle, with a cross-section resembling an irregular “V” shape (Jiang et al., 2022). In this plot, all woody stems with DBH (diameter at breast height, 1.3 m) ≥ 1 cm were tagged, spatially mapped, identified to species, and measured DBH, number of sprouts and branches. Tree census is conducted every 5 years for the 5-ha long-term forest dynamics monitoring plot. More than 18000 free-standing individuals belonging to 161 plant species were recorded during the 2007-2017 period (Table 1).
Table 1. The species richness and individual abundance of the whole plot and different habitat types from 2007 to 2017.
To estimate aboveground biomass (AGB), the tree height was first calculated by referring to Lin et al. (2012) (Equation 1). There were 47 species-specific tree height equations fitted in our study site, and an equation based on combined data from all species was used for the remaining 114 species. Then the AGB of each tree and branch was calculated by using the allometric growth equation improved by Chave et al. (2014) (Equation 2):
Where D is DBH (cm), a and b are estimated species-specific coefficients and CF is the correction factor. The wood density (WD) was obtained mainly from Liu (2012) in-situ measured data and through the search of the TRY database (Kattge et al., 2020). Among them, 14.9% of species without WD data were replaced by the mean WD of species of the same genus or family in the same climate region.
We defined abiotic factors within the context of topography and habitat types in our study. Four topographic variables were calculated (i.e. elevation, slope, aspect, and convexity) for every 20 m × 20 m plot following Harms et al. (2001). To test how the drivers of ΔAGB vary with habitat types, the 5-ha forest plot was divided into three habitat types at 20 m × 20 m scale (The extra 20 m × 10 m is not included): low-valley (H1, 50 plots), mid-hillside (H2, 45 plots), and high-ridge (H3, 25 plots). More detailed information about habitat classification could be found in Jiang et al. (2022).
To test the impacts of biotic factors on ΔAGB, we measured four dimensions of biodiversity indices: species (taxonomic) diversity, structural diversity, phylogenetic diversity, and functional diversity. Species diversity was measured by Shannon-Wiener index (H), Simpson index (D) and Pielou evenness index (J) (Ma and Liu, 1994). The change values of Shannon-Wiener index (cH), Simpson index (cD), and Pielou evenness index (cJ) were represented as the differences between each 5-year period (the same below). Stand density (SD) and the coefficient of variation of DBH (CVDBH) were calculated for structural diversity variables (Ren et al., 2021). SD was the number of individual plants with DBH ≥1 cm per unit area (quadrat). The equation for the CVDBH is as follows (Zhang and Chen, 2015):
Where σ is the standard deviation of DBH in the quadrat, μ is the mean DBH in the quadrat, and the change values of SD (cSD) and CVDBH (cCVDBH) were calculated at the same time.
According to the Angiosperm Phylogeny Group IV (APG IV), a phylogenetic tree was first constructed (Jin and Qian, 2022), and then the mean pairwise distance (MPD) and mean nearest taxon distance (MNTD) were calculated for all species in the community (Webb et al., 2002; Swenson et al., 2006):
Where MPDsample and MNTDsample are the actual observed values, while MPDnull and MNTDnull are the values of MPD and MNTD for randomly generated null communities under the null model, sdMPDnull and sdMNTDnull are the standard deviations of these values, meanMPDnull and meanMNTDnull are the average of these values. The change values of MPD (cMPD) and MNTD (cMNTD) were also calculated.
The WD, maximum tree height, and life form of plant species are closely related to forest AGB and are considered important functional traits (Ruiz-Benito et al., 2014; Ouyang et al., 2019). Therefore, we used these three types of functional traits to assess functional diversity. The WD used the data previously employed for calculating the AGB. The maximum tree height and species life form data were sourced from the Flora of Zhejiang (New Edition) (Li, 2021) and Flora of China (Wu et al., 1994-2009), with life forms categorized into three types: evergreen broad-leaved, deciduous broad-leaved, and coniferous. Then we calculated Rao’s quadratic entropy (RaoQ) and Functional dispersion (FDis) for functional diversity (Laliberté and Legendre, 2010). And the trait mean pairwise distance (traitMPD) and trait mean nearest taxon distance (traitMNTD) were calculated based on the functional trait dendrogram (Swenson et al., 2006; Shui et al., 2022):
Where traitMPDsample and traitMNTDsample are the actual observed values, while traitMPDnull and traitMNTDnull are the values of traitMPD and traitMNTD for randomly generated null communities under the null model, sdtraitMPDnull and sdtraitMNTDnull are the standard deviations of these values, meantraitMPDnull and meantraitMNTDnull are the average of these values. The change values of FDis (cFDis), RaoQ (cRaoQ), traitMPD (ctraitMPD) and traitMNTD (ctraitMNTD) were also calculated.
First, we used the Wilcoxon rank-sum test to determine if there were significant differences in AGB across the three tree censuses. Second, the generalized linear model was used to examine the impact of biotic (including the change values of them) and abiotic variables on ΔAGB. The ΔAGB was represented as the difference in AGB between each 5-year period. We divided the study decade (2007-2017) into two 5-year periods (2007-2012 and 2012-2017) to investigate the temporal changes in the drivers of ΔAGB. Furthermore, the plot was categorized into three habitats, and we examined the relationship between the explanatory variables and the response variables within them. The initial values and the change values were used for all biotic variables. To enhance the comparative analysis among drivers and models, all variables were scaled and variables with too high collinearity of variance inflation factor (VIF) >5 were removed (Fox and Weisberg, 2018). Finally, we selected our optimal models with lowest Akaike information criterion (AIC) (Bartoń, 2012), then performed hierarchical partition on them to assess the relative importance of all variables influencing ΔAGB (Lai et al., 2022; Lai et al., 2023). Some variables are not represented in our figures because they are not included in the optimal model. The initial data proofreading and organization were completed in Excel 16.0, while the subsequent calculations of indices, data analysis, and plotting were all conducted in R (version 4.2.2).
By estimating the AGB in Gutianshan 5-ha plot, we found that the AGB at community level showed a significant decrease followed by a nonsignificant increase from 2007 to 2017 (Table 2, Figure 1A), which was mainly driven by the biomass change in low-valley habitat (H1) (Table 2, Figure 1B). The AGB in the mid-hillside (H2) and high-ridge habitats (H3) from 2007 to 2017 also showed a decreasing and then increasing change, but variations at each stage were not significant (Table 2, Figures 1C, D).
Figure 1. The aboveground biomass changes of the whole plot (A), Low-valley habitat (B), Mid-hillside habitat (C) and High-ridge habitat (D) from 2007 to 2017. *p< 0.05, **p< 0.01, ***p< 0.001.
The main impact factors on ΔAGB were functional diversity (66.11% of relative importance) and structural diversity (21.35% of relative importance) during 2007-2012 period (Figure 2A). Specifically, ΔAGB was significantly negatively correlated with SD, FDis and ctraitMPD, but significantly positively correlated with ctraitMNTD. However, during 2012-2017 period, ΔAGB was mainly affected by phylogenetic diversity (48.46% of relative importance) and structural diversity (36.43% of relative importance) (Figure 2B). It showed a significant positive relationship with cMPD and cCVDBH, but a significant negative relationship with cSD and cMNTD.
Figure 2. The main impact factors of aboveground biomass dynamics from 2007 to 2012 (A) and 2012 to 2017 (B). Solid and open circles indicate significant or nonsignificant community diversity effects at p< 0.05, respectively. H, Shannon-Wiener index; CVDBH, coefficient of variation in DBH; SD, stand density; FDis, functional dispersion; MPD, mean pairwise distance; MNTD, mean nearest taxon distance; traitMPD and traitMNTD, trait mean pairwise distance and trait mean nearest taxon distance; J, Pielou evenness index. c indicates the change values of each variable. *p< 0.05, **p< 0.01, ***p< 0.001.
The main impact factors on ΔAGB in low-valley habitat were similar to the whole plot (Figures 3A, B). Moreover, we also found that it was significantly negatively correlated with aspect and elevation, but significantly positively correlated with convexity (Figure 3B). ΔAGB in mid-hillside habitat was significantly positively affected by phylogenetic diversity (Figures 3C, D). Besides, ΔAGB was significantly negatively associated with traitMPD but significantly positively associated with ctraitMNTD (Figure 3D). In high-ridge habitat, all dimensions of biodiversity as well as topographic factors showed significant effects on ΔAGB (Figures 3E, F). Specifically, ΔAGB was significantly negatively influenced by H, MNTD, cMNTD, cSD and slope, but was significantly positively influenced by cFDis, FDis, cCVDBH, MPD, SD and elevation. Whereas CVDBH had a variable relationship with ΔAGB.
Figure 3. The main impact factors of aboveground biomass dynamics in Low-valley habitat (A, B), Mid-hillside habitat (C, D), and High-ridge habitat (E, F) from 2007 to 2017. Solid and open circles indicate significant or nonsignificant community diversity effects at p< 0.05, respectively. The meanings of abbreviations are the same as in Figure 2. *p< 0.05, **p< 0.01, ***p< 0.001.
The AGB at community level in Gutianshan showed a significant decrease during the 2007-2012 period. The large-scale ice storm occurred in this region in 2008 killed many trees especially the larger-diameter trees with higher biomass (Jin et al., 2015), which might cause a significant decrease in AGB of the plot (Zhang et al., 2012). During the 2012-2017 period, the AGB increased but did not recover to the initial level, which was probably due to the stable forest type here. Most of the forest here is in the middle and late successional stages, with well-developed, typical, and stable vegetation, belonging to a mature subtropical evergreen broad-leaved forest (Legendre et al., 2009). However, the more stable a forest is before a disaster, the slower it recovers afterwards (Sun et al., 2012). Furthermore, the restoration of some forest ecosystem functions, such as the AGB and carbon sequestration, could span decades or potentially even longer periods (Amiro et al., 2010).
After examining the AGB across the three habitats within the plot, our results revealed that the trend of AGB variation at the community level was primarily driven by changes in low-valley habitat. In contrast, the AGB in mid-hillside and high-ridge habitats showed nonsignificant variation, indicating that the damage to trees in low-altitude valley was more severe than in mid- and high-altitude regions (Man et al., 2011). This was not aligned with the established impact of natural disasters on forest vegetation (Zhang et al., 2012; Tanner et al., 2014). The discrepancy may be due to the fact that the elevation differences within this plot are not substantial enough to reflect the influence of altitude. Additionally, the impact of elevation on the severity of damage to forest vegetation after disasters can be shaped by the distinctive characteristics of the local environment (Man et al., 2011).
Changes in community performance may be attributed to plant ecological strategies, which impact the efficacy and interplay of species, thereby affecting the ecological processes and functions of ecosystem (Huang et al., 2018; Zheng et al., 2024). Our study showed that the factors influencing ΔAGB varied across time scales. During the 2007-2012 period, the main influencing factors on ΔAGB were functional diversity and structural diversity. It is generally believed that both functional diversity and structural diversity are beneficial for increasing ecosystem biomass accumulation or productivity (Li et al., 2019; Lian et al., 2022) as both can promote the resource use efficiency of the community (Zhu et al., 2021; Chen et al., 2023). However, in our study, functional diversity and structural diversity were mainly negatively correlated with ΔAGB. This is likely because of the negative relationship between plant diversity and resource availability in natural ecosystems due to resource constraints and interspecific competition (Fraser et al., 2015). The communities in the study plots are mostly in the middle and late successional stages, where resources are relatively limited (Lasky et al., 2014). In addition, the increase of plant diversity and individuals at this stage tended to intensify interspecific competition (Table 1), which further reduced the available resources for species and ultimately resulted in a decrease of community productivity (Wu et al., 2018). As a result, a negative BEF relationship occurred during this period.
In the subsequent period of 2012-2017, we found that the main impact factors on ΔAGB shifted to phylogenetic diversity and structural diversity. This is in line with previous studies suggesting that BEF relationships in forests could change over time (Lasky et al., 2014; Gottschall et al., 2022). The shift could be a consequence of the formation of canopy gaps in this forest (Man et al., 2011), which could increase the light availability of understory vegetation (Zhu et al., 2014; Song et al., 2018). This increase could promote the recruitment of early-successional species that struggle to reproduce under low light conditions, as well as the regeneration of late-successional species (Song et al., 2018). As a result, phylogenetic diversity might have encapsulated certain inherent functional characteristics that were not directly assessed during this period, including traits related to roots or herbivores (Cadotte et al., 2009; Liu et al., 2015). The ΔAGB was mainly positively correlated with phylogenetic diversity and structural diversity during the 2012-2017 period. This could be attributed to the balance achieved between the high productivity but high mortality rates of early-successional species (acquisition strategy), and the lower productivity but also low mortality rates of late-successional species (conservative strategy), resulting in an increase in community productivity (Lasky et al., 2014; Zhang et al., 2023). However, we also found that ΔAGB was significantly negatively correlated with cSD and cMNTD. The variability observed in the correlations between various biodiversity indices and ΔAGB could be due to the varying capacities of each metric to capture the intensity of interactions within the forest ecosystems being studied, rather than an inherent ecological process (Yuan et al., 2018). Our results underscore the important role of multidimensional biodiversity and community context in elucidating the dynamic BEF relationships across temporal scales.
The spatial heterogeneity in resource supply rates can directly influence the biomass of producers, or indirectly impact producer biomass by limiting the variety of species that can coexist within an ecosystem (Cardinale et al., 2009; Ferry et al., 2010). Our results revealed that both the drivers and effects on ΔAGB significantly varied across different habitat types. The factors influencing ΔAGB in low-valley habitat were extremely similar to those of the whole plot, possibly because this habitat has the highest number of plots (50), closely resembling the resource supply and utilization patterns of the whole plot. The result does not align with the discoveries from the low-altitude area in Dinghai, Zhejiang Province, where a nonsignificant relationship was found between biodiversity and biomass or productivity (Wu et al., 2018). This inconsistency may be a result of different dimensions of biodiversity, or the complex mediating role of environmental factors in the BEF relationship (Zhu et al., 2021). Additionally, we found that ΔAGB was significantly negatively correlated with aspect and elevation, but significantly positively correlated with convexity in this habitat. The low-valley habitat is defined by its significant topographical heterogeneity, featuring prominent rocks and small streams, making it particularly prone to regular disturbances like tree falls and seasonal stream flooding (Xu et al., 2015). Therefore, topographic factors had a significant effect on ΔAGB in this period.
In mid-hillside habitat, ΔAGB mainly showed a significant positive relationship with phylogenetic diversity. Liu et al. (2022) also found that phylogenetic diversity reaches its maximum in the mid-elevation region. It may be because the mid-hillside habitat serves as a transitional area between the low-valley and high-ridge habitats, where the favorable supply of light and water resources allows for the growth of most species in this environment (Legendre et al., 2009; Coomes et al., 2014). In addition, there were many broken and uprooted large trees found in slopes perhaps due to steep hillsides and shallow soil (Ferry et al., 2010; Xu et al., 2015), which could increase the openness of the canopy gaps, resulting in rapid regeneration of understory species (Song et al., 2018). Such an environment might offer opportunities for species with greater phylogenetic distance and different life history strategies to survive. In summary, it can be concluded that such ecological environment and resource supply enhance the positive effect of phylogenetic diversity on ΔAGB.
In high-ridge habitat, we found that ΔAGB was significantly influenced by all dimensions of biodiversity and topographical factors, which is consistent with the findings of most studies (Lasky et al., 2014; Yuan et al., 2018; Tiwari et al., 2023). The relationships among species in high-ridge habitat (high-altitude area) tend to be more intimate (Liu et al., 2022). The canopy gaps might increase the opportunities for species less associated with the vegetation in high-ridge habitat to recolonize from neighboring areas (Roxburgh et al., 2004). Given that the habitat is less disturbed (Man et al., 2011), the diversity of communities could reach the maximum and the coexistence of species will be promoted according to the Intermediate Disturbance Hypothesis (IDH) (Roxburgh et al., 2004). Moreover, the competition for resources especially light is minimal due to the lower stand density within high-ridge habitat (Ullah et al., 2021; Su et al., 2023), providing favorable conditions for the growth of recolonizing species. Overall, the increase in diversity had enhanced their influence on ΔAGB (Table 1). Synthesizing the results garnered from the various habitat types examined, our findings highlight that habitat heterogeneity constitutes a pivotal driver influencing the BEF relationship, providing a plausible perspective for investigating the spatial variations in BEF relationships.
In the present study, our comprehensive analysis elucidates the intricate interplay between multidimensional diversity and ΔAGB within natural forest ecosystems. We have demonstrated that both abiotic factors, such as topography, and biotic factors including functional diversity, phylogenetic diversity and structural diversity exert a significant influence on the ΔAGB over time and across various habitat types. Our decade-long analysis revealed a notable decline followed by an increase in community-level AGB, primarily within low-valley habitat, with no significant alterations observed in mid-hillside and high-ridge habitats. The determinants of ΔAGB exhibited substantial temporal shifts; functional and structural diversity were pivotal during the earlier period, while phylogenetic and structural diversity became increasingly influential in the subsequent period. Moreover, the drivers and effects on ΔAGB significantly varied across different habitat types. Our findings underscore the necessity of considering the spatiotemporal variability of both abiotic and biotic factors when assessing ecosystem function. This study not only addresses a critical gap in our understanding of BEF relationships but also provides valuable insights for conservation and management strategies aimed at preserving the health and resilience of forest ecosystems.
The original contributions presented in the study are included in the article/supplementary material. Further inquiries can be directed to the corresponding author.
YB: Conceptualization, Formal analysis, Methodology, Visualization, Writing – original draft, Writing – review & editing. QW: Validation, Visualization, Writing – original draft. RZ: Data curation, Formal analysis, Investigation, Writing – original draft. JF: Formal analysis, Methodology, Visualization, Writing – original draft. JC: Conceptualization, Data curation, Supervision, Writing – review & editing. XM: Data curation, Investigation, Writing – review & editing. MY: Data curation, Funding acquisition, Investigation, Writing – review & editing. YW: Conceptualization, Data curation, Funding acquisition, Investigation, Methodology, Validation, Writing – original draft, Writing – review & editing.
The author(s) declare financial support was received for the research, authorship, and/or publication of this article. This study was financially supported by the “Pioneer” and “Leading Goose” R&D Program of Zhejiang (2023C03137), Zhejiang Undergraduate Science and Technology Innovation Activity Program (Xinmiao Talents Program) (2024R404A014) and Zhejiang Provincial Natural Science Foundation of China (LQ22C030001).
The authors declare that the research was conducted in the absence of any commercial or financial relationships that could be construed as a potential conflict of interest.
The author(s) declare that no Generative AI was used in the creation of this manuscript.
All claims expressed in this article are solely those of the authors and do not necessarily represent those of their affiliated organizations, or those of the publisher, the editors and the reviewers. Any product that may be evaluated in this article, or claim that may be made by its manufacturer, is not guaranteed or endorsed by the publisher.
Ali, A. (2019). Forest stand structure and functioning: Current knowledge and future challenges. Ecol. Indic. 98, 665–677. doi: 10.1016/j.ecolind.2018.11.017
Amiro, B. D., Barr, A. G., Barr, J., Black, T. A., Bracho, R., Brown, M., et al. (2010). Ecosystem carbon dioxide fluxes after disturbance in forests of North America. J. Geophys. Res. 115, G00K02. doi: 10.1029/2010JG001390
Bartoń, K. (2012). MuMIn: Multi-model inference. R package version 1.7.2 Available online at: http://CRAN.R-project.org/package=MuMIn (Accessed September 1, 2022).
Cadotte, M. W., Cavender-Bares, J., Tilman, D., Oakley, T. H. (2009). Using phylogenetic, functional and trait diversity to understand patterns of plant community productivity. PloS One 4, e5695. doi: 10.1371/journal.pone.0005695
Canadell, J. G., Raupach, M. R. (2008). Managing forests for climate change mitigation. Science 320, 1456–1457. doi: 10.1126/science.1155458
Cardinale, B. J., Bennett, D. M., Nelson, C. E., Gross, K. (2009). Does productivity drive diversity or vice versa? A test of the multivariate productivity-diversity hypothesis in streams. Ecology 90, 1227–1241. doi: 10.1890/08-1038.1
Cardinale, B. J., Wright, J. P., Cadotte, M. W., Carroll, I. T., Hector, A., Srivastava, D. S., et al. (2007). Impacts of plant diversity on biomass production increase through time because of species complementarity. Proc. Natl. Acad. Sci. U.S.A. 104, 18123–18128. doi: 10.1073/pnas.0709069104
Chadwick, K. D., Asner, G. P. (2016). Tropical soil nutrient distributions determined by biotic and hillslope processes. Biogeochemistry 127, 273–289. doi: 10.1007/s10533-015-0179-z
Chave, J., Réjou-Méchain, M., Búrquez, A., Chidumayo, E., Colgan, M. S., Delitti, W. B., et al. (2014). Improved allometric models to estimate the aboveground biomass of tropical trees. Glob. Change Biol. 20, 3177–3190. doi: 10.1111/gcb.12629
Chen, G. P., Cai, Q., Ma, S. H., Feng, Y. H., Fang, W. J., Ji, C. J., et al. (2023). Climate and forest attributes influence above-ground biomass of deciduous broadleaf forests in China. J. Ecol. 111, 495–508. doi: 10.1111/1365-2745.14042
Coomes, D. A., Flores, O., Holdaway, R., Jucker, T., Lines, E. R., Vanderwel, M. C. (2014). Wood production response to climate change will depend critically on forest composition and structure. Glob. Change Biol. 20, 3632–3645. doi: 10.1111/gcb.12622
Dănescu, A., Albrecht, A. T., Bauhus, J. (2016). Structural diversity promotes productivity of mixed, uneven-aged forests in southwestern Germany. Oecologia 182, 319–333. doi: 10.1007/s00442-016-3623-4
Fang, J. Y., Chen, A. P., Peng, C. H., Zhao, S. Q., Ci, L. J. (2001). Changes in forest biomass carbon storage in China between 1949 and 1998. Science 292, 2320–2322. doi: 10.1126/science.1058629
Ferry, B., Morneau, F., Bontemps, J. D., Blanc, L., Freycon, V. (2010). Higher treefall rates on slopes and waterlogged soils result in lower stand biomass and productivity in a tropical rain forest. J. Ecol. 98, 106–116. doi: 10.1111/j.1365-2745.2009.01604.x
Forrester, D. I., Bauhus, J. (2016). A review of processes behind diversity-productivity relationships in forests. Curr. For. Rep. 2, 45–61. doi: 10.1007/s40725-016-0031-2
Fotis, A. T., Murphy, S. J., Ricart, R. D., Krishnadas, M., Whitacre, J., Wenzel, J. W., et al. (2018). Above-ground biomass is driven by mass-ratio effects and stand structural attributes in a temperate deciduous forest. J. Ecol. 106, 561–570. doi: 10.1111/1365-2745.12847
Fraser, L. H., Pither, J., Jentsch, A., Sternberg, M., Zobel, M., Askarizadeh, D., et al. (2015). Worldwide evidence of a unimodal relationship between productivity and plant species richness. Science 349, 302–305. doi: 10.1126/science.aab3916
Gamfeldt, L., Snäll, T., Bagchi, R., Jonsson, M., Gustafsson, L., Kjellander, P., et al. (2013). Higher levels of multiple ecosystem services are found in forests with more tree species. Nat. Commun. 4, 1340. doi: 10.1038/ncomms2328
Gottschall, F., Cesarz, S., Auge, H., Kovach, K. R., Mori, A. S., Nock, C. A., et al. (2022). Spatiotemporal dynamics of abiotic and biotic properties explain biodiversity-ecosystem-functioning relationships. Ecol. Monogr. 92, e01490. doi: 10.1002/ecm.1490
Harms, K. E., Condit, R., Hubbell, S. P., Foster, R. B. (2001). Habitat associations of trees and shrubs in a 50-ha neotropical forest plot. J. Ecol. 89, 947–959. doi: 10.1111/j.1365-2745.2001.00615.x
He, J. Y., Lu, L. Q., He, H. J., Zhang, Z. H., Hao, M. H., Zhang, C. Y., et al. (2024). Estimating the dynamics of ecosystem functions under climate change in a temperate forest region. Ecol. Indic. 166, 112353. doi: 10.1016/j.ecolind.2024.112353
Houghton, R. (2005). Aboveground forest biomass and the global carbon balance. Glob. Change Biol. 11, 945–958. doi: 10.1111/j.1365-2486.2005.00955.x
Huang, Y. Y., Chen, Y. X., Castro-Izaguirre, N., Baruffol, M., Brezzi, M., Lang, A., et al. (2018). Impacts of species richness on productivity in a large-scale subtropical forest experiment. Science 362, 80–83. doi: 10.1126/science.aat6405
Huston, M. A. (1997). Hidden treatments in ecological experiments: re-evaluating the ecosystem function of biodiversity. Oecologia 110, 449–460. doi: 10.1007/s004420050180
Isbell, F., Gonzalez, A., Loreau, M., Cowles, J., Díaz, S., Hector, A., et al. (2017). Linking the influence and dependence of people on biodiversity across scales. Nat. Commun. 546, 65–72. doi: 10.1038/nature22899
Jiang, C. C., Fu, J. Q., Wang, Y. Q., Chai, P. T., Yang, Y. D., Mi, X. C., et al. (2022). The habitat type and scale dependences of interspecific associations in a subtropical evergreen broad-leaved forest. Forests 13, 1334. doi: 10.3390/f13081334
Jin, Y., Chen, J. H., Mi, X. C., Ren, H. B., Ma, K. P., Yu, M. J. (2015). Impacts of the 2008 ice storm on structure and composition of an evergreen broad-leaved forest community in eastern China. Biodivers. Sci. 23, 610. doi: 10.17520/biods.2015051
Jin, Y., Qian, H. (2022). V. PhyloMaker2: An updated and enlarged R package that can generate very large phylogenies for vascular plants. Plant Divers. 44, 335–339. doi: 10.1016/j.pld.2022.05.005
Jucker, T., Bongalov, B., Burslem, D. F., Nilus, R., Dalponte, M., Lewis, S. L., et al. (2018). Topography shapes the structure, composition and function of tropical forest landscapes. Ecol. Lett. 21, 989–1000. doi: 10.1111/ele.12964
Kardol, P., Fanin, N., Wardle, D. A. (2018). Long-term effects of species loss on community properties across contrasting ecosystems. Nature 557, 710–713. doi: 10.1038/s41586-018-0138-7
Kattge, J., Bönisch, G., Díaz, S., Lavorel, S., Prentice, I. C., Leadley, P., et al. (2020). TRY plant trait database-enhanced coverage and open access. Glob. Change Biol. 26, 119–188. doi: 10.1111/gcb.14904
Lai, J. S., Zhu, W. J., Cui, D. F., Mao, L. F. (2023). Extension of the glmm.hp package to zero-inflated generalized linear mixed models and multiple regression. J. Plant Ecol. 16, rtad038. doi: 10.1093/jpe/rtad038
Lai, J. S., Zou, Y., Zhang, S., Zhang, X. G., Mao, L. F. (2022). glmm. hp: an R package for computing individual effect of predictors in generalized linear mixed models. J. Plant Ecol. 15, 1302–1307. doi: 10.1093/jpe/rtac096
Laliberté, E., Legendre, P. (2010). A distance-based framework for measuring functional diversity from multiple traits. Ecology 91, 299–305. doi: 10.1890/08-2244.1
Lasky, J. R., Uriarte, M., Boukili, V. K., Erickson, D. L., John Kress, W., Chazdon, R. L. (2014). The relationship between tree biodiversity and biomass dynamics changes with tropical forest succession. Ecol. Lett. 17, 1158–1167. doi: 10.1111/ele.12322
Legendre, P., Mi, X. C., Ren, H. B., Ma, K. P., Yu, M. J., Sun, I. F., et al. (2009). Partitioning beta diversity in a subtropical broad-leaved forest of China. Ecology 90, 663–674. doi: 10.1890/07-1880.1
Li, G. Y. (2021). Flora of zhejiang (New edition) (Hangzhou: Zhejiang Science and Technology Publishing House).
Li, Y., Bao, W., Bongers, F., Chen, B., Chen, G., Guo, K., et al. (2019). Drivers of tree carbon storage in subtropical forests. Sci. Total Environ. 654, 684–693. doi: 10.1016/j.scitotenv.2018.11.024
Lian, Z. H., Wang, J., Fan, C. Y., Von Gadow, K. (2022). Structure complexity is the primary driver of functional diversity in the temperate forests of northeastern China. For. Ecosyst. 9, 100048. doi: 10.1016/j.fecs.2022.100048
Liang, J. J., Crowther, T. W., Picard, N., Wiser, S., Zhou, M., Alberti, G., et al. (2016). Positive biodiversity-productivity relationship predominant in global forests. Science 354, aaf8957. doi: 10.1126/science.aaf8957
Lin, D. M., Lai, J. S., Muller-Landau, H. C., Mi, X. C., Ma, K. P. (2012). Topographic variation in aboveground biomass in a subtropical evergreen broad-leaved forest in China. PloS One 7, e48244. doi: 10.1371/journal.pone.0048244
Liu, X. J. (2012). Distribution pattern of functional traits of woody plants in subtropical forests and their relationship with the environment. University of the Chinese Academy of Sciences, Beijing.
Liu, J. J., Zhang, X. X., Song, F. F., Zhou, S. R., Cadotte, M. W., Bradshaw, C. J. (2015). Explaining maximum variation in productivity requires phylogenetic diversity and single functional traits. Ecology 96, 176–183. doi: 10.1890/14-1034.1
Liu, M. X., Zhang, G. J., Yin, F. L., Wang, S. Y., Li, L. (2022). Relationship between biodiversity and ecosystem multifunctionality along the elevation gradient in alpine meadows on the eastern Qinghai-Tibetan plateau. Ecol. Indic. 141, 109097. doi: 10.1016/j.ecolind.2022.109097
Ma, K. P., Liu, Y. M. (1994). Measurement of biotic community diversity I α diversity (Part 2). Biodivers. Sci. 2, 231–239. doi: 10.17520/biods.1994038
Man, X. X., Mi, X. C., Ma, K. P. (2011). Effects of an ice storm on community structure of an evergreen broad-leaved forest in Gutianshan National Nature Reserve, Zhejiang Province. Biodivers. Sci. 19, 197. doi: 10.3724/SP.J.1003.2011.09220
McEwan, R. W., Lin, Y. C., Sun, I. F., Hsieh, C. F., Su, S. H., Chang, L. W., et al. (2011). Topographic and biotic regulation of aboveground carbon storage in subtropical broad-leaved forests of Taiwan. For. Ecol. Manage. 262, 1817–1825. doi: 10.1016/j.foreco.2011.07.028
Mi, X. C., Feng, G., Hu, Y. B., Zhang, J., Chen, L., Corlett, R. T., et al. (2021). The global significance of biodiversity science in China: an overview. Natl. Sci. Rev. 8, nwab032. doi: 10.1093/nsr/nwab032
Ouyang, S., Xiang, W. H., Wang, X. P., Xiao, W. F., Chen, L., Li, S. G., et al. (2019). Effects of stand age, richness and density on productivity in subtropical forests in China. J. Ecol. 107, 2266–2277. doi: 10.1111/1365-2745.13194
Pan, Y. D., Birdsey, R. A., Fang, J. Y., Houghton, R., Kauppi, P. E., Kurz, W. A., et al. (2011). A large and persistent carbon sink in the world’s forests. Science 333, 988–993. doi: 10.1126/science.1201609
Paoli, G. D. (2006). Divergent leaf traits among congeneric tropical trees with contrasting habitat associations on Borneo. J. Trop. Ecol. 22, 397–408. doi: 10.1017/S0266467406003208
Petchey, O. L., Gaston, K. J. (2002). Functional diversity (FD), species richness and community composition. Ecol. Lett. 5, 402–411. doi: 10.1046/j.1461-0248.2002.00339.x
Piao, S. L., Fang, J. Y., Ciais, P., Peylin, P., Huang, Y., Sitch, S., et al. (2009). The carbon balance of terrestrial ecosystems in China. Nature 458, 1009–1013. doi: 10.1038/nature07944
Quesada, C. A., Phillips, O. L., Schwarz, M., Czimczik, C. I., Baker, T. R., Patiño, S., et al. (2012). Basin-wide variations in Amazon forest structure and function are mediated by both soils and climate. Biogeosciences 9, 2203–2246. doi: 10.5194/bg-9-2203-2012
Ray, T., Delory, B. M., Beugnon, R., Bruelheide, H., Cesarz, S., Eisenhauer, N., et al. (2023). Tree diversity increases productivity through enhancing structural complexity across mycorrhizal types. Sci. Adv. 9, eadi2362. doi: 10.1126/sciadv.adi2362
Reich, P. B., Tilman, D., Isbell, F., Mueller, K., Hobbie, S. E., Flynn, D. F., et al. (2012). Impacts of biodiversity loss escalate through time as redundancy fades. Science 336, 589–592. doi: 10.1126/science.1217909
Ren, S., Ali, A., Liu, H., Yuan, Z., Yang, Q., Shen, G., et al. (2021). Response of community diversity and productivity to canopy gap disturbance in subtropical forests. For. Ecol. Manage. 502, 119740. doi: 10.1016/j.foreco.2021.119740
Richardson, K., Steffen, W., Lucht, W., Bendtsen, J., Cornell, S. E., Donges, J. F., et al. (2023). Earth beyond six of nine planetary boundaries. Sci. Adv. 9, eadh2458. doi: 10.1126/sciadv.adh2458
Roxburgh, S. H., Shea, K., Wilson, J. B. (2004). The intermediate disturbance hypothesis: patch dynamics and mechanisms of species coexistence. Ecology 85, 359–371. doi: 10.1890/03-0266
Ruiz-Benito, P., Gómez-Aparicio, L., Paquette, A., Messier, C., Kattge, J., Zavala, M. A. (2014). Diversity increases carbon storage and tree productivity in S panish forests. Glob. Ecol. Biogeogr. 23, 311–322. doi: 10.1111/geb.12126
Shui, W., Feng, J., Li, H., Jiang, C., Sun, X., Liu, Y. M., et al. (2022). Phylogeny and functional traits structure of plant communities with different slope aspects in the degraded karst tiankeng. Acta Ecol. Sin. 42, 8050–8060. doi: 10.5846/stxb202107201965
Song, X. Y., Hogan, J., Lin, L. X., Wen, H. D., Cao, M., Yang, J. (2018). Canopy openness and topographic habitat drive tree seedling recruitment after snow damage in an old-growth subtropical forest. For. Ecol. Manage. 429, 493–502. doi: 10.1016/j.foreco.2018.07.038
Srivastava, D. S., Cadotte, M. W., Macdonald, A. A. M., Marushia, R. G., Mirotchnick, N. (2012). Phylogenetic diversity and the functioning of ecosystems. Ecol. Lett. 15, 637–648. doi: 10.1111/j.1461-0248.2012.01795.x
Su, L., Heydari, M., Omidipour, R., Soheili, F., Cheraghi, J., Villa, P. M., et al. (2023). Stand structural diversity and elevation rather than functional diversity drive aboveground biomass in historically disturbed semiarid oak forests. For. Ecol. Manage. 543, 121139. doi: 10.1016/j.foreco.2023.121139
Sun, Y., Gu, L. H., Dickinson, R. E., Zhou, B. Z. (2012). Forest greenness after the massive 2008 Chinese ice storm: integrated effects of natural processes and human intervention. Environ. Res. Lett. 7, 35702. doi: 10.1088/1748-9326/7/3/035702
Swenson, N. G., Enquist, B. J., Pither, J., Thompson, J., Zimmerman, J. K. (2006). The problem and promise of scale dependency in community phylogenetics. Ecology 87, 2418–2424. doi: 10.1890/0012-9658(2006)87[2418:TPAPOS]2.0.CO;2
Synes, N. W., Ponchon, A., Palmer, S. C., Osborne, P. E., Bocedi, G., Travis, J. M., et al. (2020). Prioritising conservation actions for biodiversity: Lessening the impact from habitat fragmentation and climate change. Biol. Conserv. 252, 108819. doi: 10.1016/j.biocon.2020.108819
Tanner, E. V., Rodriguez-Sanchez, F., Healey, J. R., Holdaway, R. J., Bellingham, P. J. (2014). Long-term hurricane damage effects on tropical forest tree growth and mortality. Ecology 95, 2974–2983. doi: 10.1890/13-1801.1
Tilman, D. (1999). The ecological consequences of changes in biodiversity: a search for general principles. Ecology 80, 1455–1474. doi: 10.1890/0012-9658(1999)080[1455:TECOCI]2.0.CO;2
Tiwari, R. M., Liu, J. L., Xie, Y. C., Yao, S. H., Liu, S. L., Wu, S. M., et al. (2023). Decoupling the impact of biodiversity and environmental factors on the biomass and biomass growth of trees in subtropical forests. J. Plant Ecol. 16, rtac040. doi: 10.1093/jpe/rtac040
Ullah, F., Gilani, H., Sanaei, A., Hussain, K., Ali, A. (2021). Stand structure determines aboveground biomass across temperate forest types and species mixture along a local-scale elevational gradient. For. Ecol. Manage. 486, 118984. doi: 10.1016/j.foreco.2021.118984
van der Sande, M. T., Peña-Claros, M., Ascarrunz, N., Arets, E. J., Licona, J. C., Toledo, M., et al. (2017). Abiotic and biotic drivers of biomass change in a Neotropical forest. J. Ecol. 105, 1223–1234. doi: 10.1111/1365-2745.12756
Wang, S. P., Hong, P. B., Adler, P. B., Allan, E., Hautier, Y., Schmid, B., et al. (2024). Towards mechanistic integration of the causes and consequences of biodiversity. Trends Ecol. Evol. 39, 689–700. doi: 10.1016/j.tree.2024.02.008
Webb, C. O., Ackerly, D. D., Mcpeek, M. A., Donoghue, M. J. (2002). Phylogenies and community ecology. Annu. Rev. Ecol. Syst. 33, 475–505. doi: 10.1146/annurev.ecolsys.33.010802.150448
Werner, F. A., Homeier, J. (2015). Is tropical montane forest heterogeneity promoted by a resource-driven feedback cycle? Evidence from nutrient relations, herbivory and litter decomposition along a topographical gradient. Funct. Ecol. 29, 430–440. doi: 10.1111/1365-2435.12351
Wu, C. P., Han, W. J., Jiang, B., Liu, B. W., Yuan, W. G., Shen, A. H., et al. (2018). Relationships between species richness and biomass/productivity depend on environmental factors in secondary forests of Dinghai, Zhejiang Province. Biodivers. Sci. 26, 545. doi: 10.17520/biods.2017320
Wu, Z. Y., Raven, P. H., Hong, D. Y. (1994-2009). Flora of China (Beijing and St Louis: Science Press and Missouri Botanical Garden Press).
Xu, Y. Z., Franklin, S., Wang, Q. G., Shi, Z., Luo, Y., Lu, Z. J., et al. (2015). Topographic and biotic factors determine forest biomass spatial distribution in a subtropical mountain moist forest. For. Ecol. Manage. 357, 95–103. doi: 10.1016/j.foreco.2015.08.010
Yang, B., Ma, R. H., Zhai, J., Du, J. R., Bai, J. H., Zhang, W. H. (2024). Stand spatial structure is more important than species diversity in enhancing the carbon sink of fragile natural secondary forest. Ecol. Indic. 158, 111449. doi: 10.1016/j.ecolind.2023.111449
Yu, G. R., Chen, Z., Piao, S. L., Peng, C. H., Ciais, P., Wang, Q. F., et al. (2014). High carbon dioxide uptake by subtropical forest ecosystems in the East Asian monsoon region. Proc. Natl. Acad. Sci. U.S.A. 111, 4910–4915. doi: 10.1073/pnas.1317065111
Yu, M. J., Hu, Z. H., Yu, J. P., Ding, B. Y., Fang, T. (2001). Forest vegetation types in Gutianshan natural reserve in Zhejiang. J. Zhejiang. Univ. Agric. Life Sci. 27, 375–380. Available online at: https://www.zjujournals.com/agr/CN/Y2001/V27/I4/375.
Yuan, Z. Q., Ali, A., Jucker, T., Ruiz-Benito, P., Wang, S. P., Jiang, L., et al. (2019). Multiple abiotic and biotic pathways shape biomass demographic processes in temperate forests. Ecology 100, e02650. doi: 10.1002/ecy.2650
Yuan, Z. Q., Wang, S. P., Ali, A., Gazol, A., Ruiz-Benito, P., Wang, X. G., et al. (2018). Aboveground carbon storage is driven by functional trait composition and stand structural attributes rather than biodiversity in temperate mixed forests recovering from disturbances. Ann. For. Sci. 75, 1–13. doi: 10.1007/s13595-018-0745-3
Zemp, D. C., Guerrero-Ramirez, N., Brambach, F., Darras, K., Grass, I., Potapov, A., et al. (2023). Tree islands enhance biodiversity and functioning in oil palm landscapes. Nature 618, 316–321. doi: 10.1038/s41586-023-06086-5
Zhang, Y., Chen, H. Y. (2015). Individual size inequality links forest diversity and above-ground biomass. J. Ecol. 103, 1245–1252. doi: 10.1111/1365-2745.12425
Zhang, Y. H., Jin, B. C., Zhang, X. L., Wei, H. H., Chang, Q. Q., Huang, F. Q., et al. (2023). Grazing alters the relationships between species diversity and biomass during community succession in a semiarid grassland. Sci. Total Environ. 887, 164155. doi: 10.1016/j.scitotenv.2023.164155
Zhang, R., Li, S. F., Huang, X. B., Li, C., Xu, C. H., Su, J. R. (2024). Diversity-biomass relationships are shaped by tree mycorrhizal associations and stand structural diversity at different spatial scales. For. Ecosyst. 11, 100234. doi: 10.1016/j.fecs.2024.100234
Zhang, F., Zhou, G. Y., Hiratsuka, M., Tanaka, K., Morikawa, Y. (2012). Influence of an ice storm on aboveground biomass of subtropical evergreen broadleaf forest in Lechang, Nanling mountains of Southern China. Int. J. For. Res. 2012, 467848. doi: 10.1155/2012/467848
Zheng, L. T., Barry, K. E., Guerrero-Ramírez, N. R., Craven, D., Reich, P. B., Verheyen, K., et al. (2024). Effects of plant diversity on productivity strengthen over time due to trait-dependent shifts in species overyielding. Nat. Commun. 15, 2078. doi: 10.1038/s41467-024-46355-z
Zhu, J. J., Lu, D. L., Zhang, W. D. (2014). Effects of gaps on regeneration of woody plants: a meta-analysis. J. For. Res. 25, 501–510. doi: 10.1007/s11676-014-0489-3
Keywords: ecosystem functioning, functional diversity, evolutionary diversity, structural diversity, niche complementarity, disturbance
Citation: Bian Y, Wu Q, Zheng R, Fu J, Chen J, Mi X, Yu M and Wang Y (2025) Temporal and habitat-specific variations in drivers of aboveground biomass dynamics in a Chinese subtropical forest. Front. Plant Sci. 15:1531654. doi: 10.3389/fpls.2024.1531654
Received: 20 November 2024; Accepted: 30 December 2024;
Published: 21 January 2025.
Edited by:
Hui Zhang, Hainan University, ChinaReviewed by:
Zuoqiang Yuan, Northwestern Polytechnical University, ChinaCopyright © 2025 Bian, Wu, Zheng, Fu, Chen, Mi, Yu and Wang. This is an open-access article distributed under the terms of the Creative Commons Attribution License (CC BY). The use, distribution or reproduction in other forums is permitted, provided the original author(s) and the copyright owner(s) are credited and that the original publication in this journal is cited, in accordance with accepted academic practice. No use, distribution or reproduction is permitted which does not comply with these terms.
*Correspondence: Yunquan Wang, eXF3YW5nQHZpcC4xMjYuY29t
Disclaimer: All claims expressed in this article are solely those of the authors and do not necessarily represent those of their affiliated organizations, or those of the publisher, the editors and the reviewers. Any product that may be evaluated in this article or claim that may be made by its manufacturer is not guaranteed or endorsed by the publisher.
Research integrity at Frontiers
Learn more about the work of our research integrity team to safeguard the quality of each article we publish.