- 1College of Plant Protection of Shanxi Agricultural University, Jinzhong, China
- 2State Key Laboratory for Biology of Plant Diseases and Insect Pests, Institute of Plant Protection, Chinese Academy of Agricultural Sciences, Beijing, China
The rice water weevil, Lissorhoptrus oryzophilus Kuschel (Coleoptera: Curculionidae), threatens global rice production, with invasion events driven by its parthenogenetic populations. However, the global establishment risk in global rice-growing areas and potential economic losses and control benefits of the populations in invaded areas remain unclear. We applied an optimized MaxEnt model to predict the global suitable areas of the populations under current and future climate scenarios. Furthermore, we used @Risk software to estimate the potential economic losses and controlling benefit of this populations to rice production in China. Compared to its native range (North America), this populations has explored novel climates ecological niches in invaded areas (Europe and Asia) and occupies the broadest range of climatic ecological niches in Asia. The highly suitable area is primarily covered in rice-growing areas in China, the Korean Peninsula, and Japan, with all major rice-growing areas concentrated in these countries and regions. Under SSP1-2.6 and SSP5-8.5 emission scenarios, sum of suitable area for the populations in global rice-growing regions is projected to decrease by 0.35% and 0.26%, respectively, by the 2030s and 2050s. Moreover, @Risk analysis indicates that without control measures, the populations could cost China’s rice industry $18.95 billion, but management efforts could recover $17.54 billion. These results provide in-depth reference about the impact of climatic changes on the potential global suitable range of L. oryzophilus parthenogenetic populations and its economic impact on the rice industry in China.
1 Introduction
The advent of globalization and the concomitant enhancement of connectivity through international trade and the frequent movement of agricultural commodities has led to an increase in the incidence of invasive alien pests (IAPs) (Vilà et al., 2011). These present a substantial economic risk to worldwide agricultural crops, causing considerable losses and having a detrimental impact on human livelihoods (Cardinale et al., 2006; Daehler, 2003). A multitude of IAPs have undergone rapid evolutionary changes within their novel environments, driven by a combination of factors including genotype sorting, genetic recombination, interspecies hybridization, and natural selection. These changes have also occurred in response to the unique climatic conditions that they have encountered in their new habitats. (Guisan et al., 2014; Sexton et al., 2017). Notably, the IAPs with parthenogenetic reproduction have accelerated their spread and establishment in new environments due to their high adaptability and low energy consumption in reproduction (Graziosi and Rieske, 2014). Additionally, the impacts of climate change will lead to the higher rates, severity, damage, voltinism and geographic spread of IAPs to profound impact on agriculture production (Harrington et al., 2007; Hill et al., 2016; Ramasamy et al., 2022). To measure this threat and create effective biosecurity policies, it is essential to comprehend the origins of IAPs, their chances of establishing themselves in novel region, the region of establishing under climate change, and their possible economic impact in invaded areas. Therefore, assessing the distribution of globally suitable areas for IAPs under climate change, as well as estimating potential economic losses to staple crops in invaded areas and the benefits of control measures, is crucial for formulating pest management strategies to safeguard global food security (Ma and Ma, 2022).
Lissorhoptrus oryzophilus Kuschel (Coleoptera: Curculionidae) is native to the southern and eastern United States (Aghaee and Godfrey, 2014). In the southern region of Texas, bisexual and parthenogenetic populations of L. oryzophilus are present, but in California, only the parthenogenetic populations has been found to posed a huge threat to local rice production (Jiang et al., 2008; Aghaee and Godfrey, 2015). The adult weevils feed on rice leaves and lay their eggs after the fields are flooded, while the larvae feed on the roots, leading to poor root development, seeds dislodgement and reduced grain yields (Grigarick and Beards, 1965; Zou et al., 2004). L. oryzophilus is renowned for its highly invasive nature due to its ability to spread through a variety of mechanisms, including airborne dispersal, flight, swimming, and human-mediated transport. Additionally, it exhibits a great poliphagy (consuming a wide range of wild grasses) and adapts through various overwintering strategies (utilizing substrates like bunchgrass and leaf litter) (Chen et al., 2005; Saito et al., 2005; Adams et al., 2015). It is also important to recognize that a single individual is theoretically enough to initiate a new infestation due to its parthenogenetic reproduction in invaded areas (Saito et al., 2005). In the latter half of the 20th century, its parthenogenetic populations became world populations and invaded several high-yielding rice cultivation areas worldwide, including Europe (Italy), East Asia (Japan, China, South Korea and North Korea) and South Asia (India), causing significant economic losses in local agricultural production (Huang et al., 2017; Lupi et al., 2015; Saito et al., 2005; Ferrero, 2005; Giantsis et al., 2017; Lupi et al., 2010). These invasion events demonstrate that the L. oryzophilus females have a strong adaptive capability to new climatic environments (Saito et al., 2005; Caldara et al., 2004; Lupi et al., 2015). Previous studies have used CLIMEX to predict the suitable areas for this populations in China based on its biological characteristics and environmental adaptations (Mao et al., 1997). Qi et al. (2012) used MaxEnt to explored the suitable areas of the populations in rice-growing areas in China. Xie et al. (2022) predicted the suitable areas of the populations under current and future climatic conditions by MaxEnt. However, as a widely prevalent invasive populations globally, the establishment risk of this populations in global rice cultivation areas remain unknown, and there have been no quantitative assessments reported regarding the regional potential economic losses and control benefits in invaded areas, especially in China where there is high dependence on rice supply (Bin Rahman and Zhang, 2023). It is essential to predict the suitable areas of L. oryzophilus parthenogenetic populations in global rice growing areas under climate change conditions so that targeted management and control measures to mitigate increasing losses.
Species distribution models (SDMs) use environmental variables from known species locations to predict their distributions and ecological needs; these models are commonly applied in invasion biology, conservation biology, global change biology, and pathogen risk assessments (Raffini et al., 2020; Pili et al., 2020; Larson and Olden, 2012). The MaxEnt model combines machine learning with the principle of maximum entropy, and it is widely used in invasive species risk assessment due to its flexibility and ease of interpretation (Phillips and Dudík, 2008; Elith et al., 2011; Jin et al., 2022; Yang et al., 2022). The ecological niche shifts of IAPs in invaded habitats have been extensively documented (Sexton et al., 2017; Guisan et al., 2014). Understanding the ecological niche characteristics occupied by invasive species in invaded areas also provides new insights into their impacts. The n-dimensional hypervolume framework has been used to build and compare ecological niche characteristics, which are more comprehensive than two-dimensional methods that condense multiple niche variables into principal components (Blonder et al., 2018, 2014). Multidimensional methods provide a fuller depiction of niche differences by capturing all variability across niche dimensions (Pack et al., 2022; Pili et al., 2020). Additionally, we are interested in exploring changes in niche characteristics of specific variables related to the environmental suitability of L. oryzophilus parthenogenetic populations. This is feasible within the n-dimensional framework, as centroids for each niche dimension can be computed, further quantifying how species differ across various environmental factors during the invasion process. Typically, predictions of potential economic losses caused by harmful organisms rely on @RISK software, which utilizes Monte Carlo stochastic simulation methods. By constructing models of potential economic losses under different scenarios and simulating outcomes using various probability distributions, @RISK software can estimate the potential economic impact of harmful organisms (Li and Qin, 2018). Previous studies have used @RISK software to predict the potential economic losses and control costs caused by Bactrocera cucurbitae and Spodoptera frugiperda on host plants in China (Sun et al., 2018; Qin et al., 2020). Given that China is the largest rice producer globally (https://www.fao.org/), this study aims to utilize the @RISK stochastic model to assess the potential economic impact of L. oryzophilus parthenogenetic populations on China’s rice industry.
Despite extensive efforts both domestically and internationally in developing quantitative risk assessment models and software for IAP, existing models and software to date cannot independently achieve comprehensive assessments covering the invasion potential, suitable areas, and potential economic losses from IAPs (Li and Qin, 2018). Therefore, it is necessary to integrate these models and software organically in IAPs risk analysis framework (Li and Qin, 2018). We construct a new framework for global establishment risk assessment of L. oryzophilus parthenogenetic populations (Supplementary Figure S1). Specifically, (1) reconstruct the invasion history of the populations across recorded countries; (2) identify significant environmental variables affecting the suitable areas of the populations; (3) predict the suitable areas of the populations in global rice-growing areas under two climate change conditions; (4) construct ecological niche characterizations for North America, Europe, and Asia using four major bioclimatic factors that largely impact model results to characterize ecological differences in suitable habitats for the populations. (5) evaluate the potential economic losses of the populations to rice industry under unmanaged scenario and managed scenario.
2 Materials and methods
2.1 Species distribution record
We obtained global occurrence records of L. oryzophilus parthenogenetic populations from the Global Biodiversity Information Facility (https://www.gbif.org/; accessed on February 7, 2023 https://doi.org/10.15468/dl.tnzme5), Bold Systems v4 (http://www.boldsystems.org/; accessed on February 21, 2023) and published literature (China National Knowledge Infrastructure: https://www.cnki.net; Web of Science, https://www.webofscience.com/; accessed on May 9, 2023). A total of 1991 global occurrence records for L. oryzophilus parthenogenetic populations were collected. Most of the sampling points are concentrated in central and northeastern China, as well as mainland Japan. Only a few samples have been recorded in the eastern and southern United States, and southern Europe. To avoid spatial redundancy of the sample points, we also used ENMTools to eliminate spatial autocorrelation by screening occurrence records, ensuring each raster retained a single occurrence record (Warren et al., 2010). Finally, 1500 occurrence records of L. oryzophilus parthenogenetic populations were retained for constructing the MaxEnt model (Figure 1).
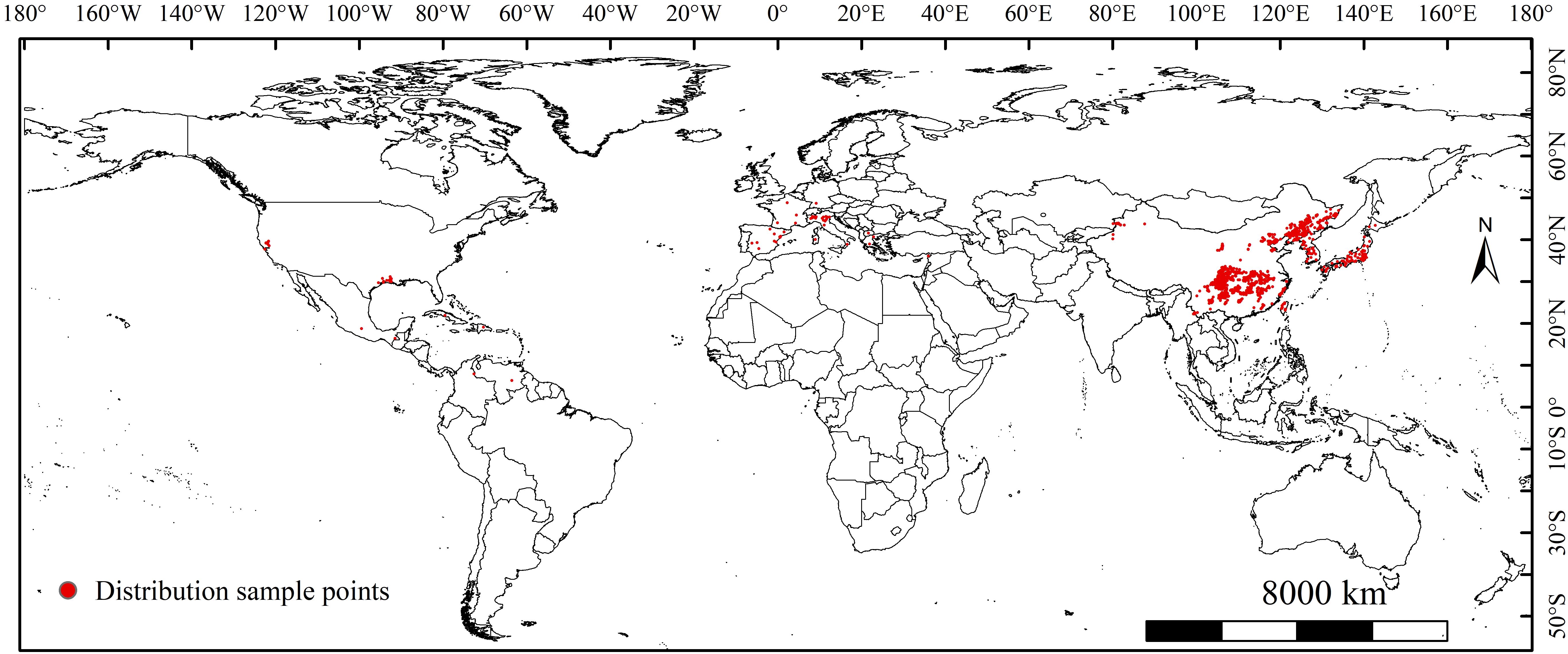
Figure 1. Global occurrence sampling points of Lissorhoptrus oryzophilus parthenogenetic populations.
We reconstruct parthenogenetic and bisexual populations of L. oryzophilus invasion history in native (the United States) and invasive range based on the time of the first reported in EPPO Global Database (https://www.eppo.int/; accessed on February 21, 2023) and the literature for different invasive region (Web of Science, https://www.webofscience.com/; accessed on May 9, 2023) (Supplementary Table S1). For the first occurrence records of L. oryzophilus in different provinces of China, we conducted a detailed search based on CNKI (China National Knowledge Infrastructure: https://www.cnki.net) and the National Agricultural Plant Quarantine Pests Distribution Administrative Region Directory (www.moa.gov.cn) (Supplementary Table S1). All the countries, provinces and states with documented first reported are visualized using different colors in ArcGIS 10.8 software.
2.2 Environmental variables
For climatic parameters, we obtained 19 bioclimatic factors (Supplementary Table S2) and altitude variables from the World Climate Database (http://www.worldclim.org/; accessed on October 3, 2022) at a resolution of 5 arc-min (10.0 km resolution at the equator). These 19 bioclimatic factors were derived from minimum temperature, maximum temperature, and precipitation data from 1970 to 2000 to create suitability maps under current climate conditions. We also incorporated the HII (Human Influence Index (https://beta.sedac.ciesin.columbia.edu/data/set/wildareas-v2-human-influence-index-geographic)) into our model. To avoid model overfitting, we used the ENMTools to examine correlations among 21 predictor variables and remove highly correlated environmental factors (| r |< 0.8, Pearson) to reduce the collinearity between predictor variables (Supplementary Figure S2) (Graham, 2003; Favretti, 2018). Finally, the ten predictor variables were chosen based on their importance for the MaxEnt model: mean diurnal range (Bio 2), temperature seasonality (Bio 4), mean temperature of warmest Quarter (Bio10), precipitation of driest month (Bio 14), precipitation seasonality (Bio 15), precipitation of wettest quarter (Bio 16), precipitation of warmest quarter (Bio 18), precipitation of coldest quarter (Bio 19), altitude (Alt) and Human Influence Index (HII) (Supplementary Table S3).
We also obtained bioclimatic variables were from the Beijing Climate Center Climate System Model (BCC-CSM2-MR) (http://www.worldclim.org/; accessed on October 3, 2022), developed by the National Climate Center based on the CMIP6 of the sixth assessment report (AR6) of the Intergovernmental Panel on Climate Change (IPCC). Compared to BCC-CSM1.1m, the BCC-CSM2-MR shows notable advancements in tropospheric temperature and circulation on global and East Asian scales, as well as in climate variability across different time scales (Wu et al., 2019). In our study, SSP1-2.6 (low-emission scenario) and SSP5-8.5 (high-emission scenario) in the 2030s (average of 2041–2060) and 2050s (average of 2041–2060) were included to predict the suitable areas under future climatic conditions.
2.3 Model optimization and parameterization
The default parameter settings in MaxEnt can lead to models that are either overly complex or too simplistic (Warren and Seifert, 2011). Therefore, the alternative combinations of feature classes (FCs) and regularization multipliers (RMs) optimization of the MaxEnt model was conducted using the ENMeval package in the R software to obtain appropriate parameter settings (Morales et al., 2017; Muscarella et al., 2014). The MaxEnt model has five basic features: linear (L), quadratic (Q), hinge (H), product (P), and threshold (T) (Santana et al., 2019). We set five parameter combinations for these five arithmetic features as L, LQ, H, LQH, and LQHP (Muscarella et al., 2014). The regularization multipliers were set from 0.5 to 6, with a 0.5 interval, resulting in 60 combinations of model parameters.
We selected the minimum values of delta Akaike information criterion correction (ΔAICc) as criteria (Jarnevich et al., 2015). A model with a lower AICc score indicates lower complexity and better fit, making it the preferred choice. Thus, select the model with the minimum ΔAICc value (choose ΔAICc = 0) to build the final MaxEnt model. The FC parameters were set as LQHP with a regularization multiplier of 0.5. The global occurrence records of L. oryzophilus were divided, and 75% and 25% were selected as the training and testing datasets, respectively (Merow et al., 2013; Dudík et al., 2005). We also set the maximum iteration value to 500, selected the run type as “Bootstrap”, the maximum background points were 10,000, 10 times running repetition of the model and random seeds were selected to enhance model randomness (Warren and Seifert, 2011). The other parameters were maintained at their default settings.
2.4 Model result evaluation and GIS analysis
We used the AUC of the receiver operating characteristic (ROC) analysis to assess and calibrate the accuracy of the MaxEnt model. The AUC was examined for additional accuracy analyses, including the agreement between introduced observations and predictive logistic regression results (Elith et al., 2011; Ficetola et al., 2007). AUC values range from 0 to 1, with larger values indicating greater credibility in model performance: the values< 0.6, 0.6–0.9, and > 0.9 were considered poor, useful, and excellent, respectively (Liu et al., 2005). The probabilistic prediction results generated by the MaxEnt model are presented in a grid raster format, with values ranging from 0 to 1. Using the spatial projection outputs from the MaxEnt model, we estimated the future suitable areas of the species under climate change scenarios (SSP1-2.6 and SSP5-8.5). The suitable areas were divided into suitable habitats and unsuitable habitats based on the cloglog threshold value of the maximum training presence (MTP). The cloglog threshold for the MTP was set at 0.1039, classifying areas below this threshold as unsuitable habitats and those above as suitable (Liu et al., 2005). Accordingly, the suitable areas of the pest were reclassified into four categories: unsuitable habitats (0-MTP), low suitable habitats (MTP-0.4), moderate suitable habitats (0.4-0.6), and high suitable habitats (0.6-1).
The successful invasion and establishment of new areas by IAPs also depend on the suitability of the preferred host (de Godoy et al., 2023; Huber and Geist, 2019; Dukes and Mooney, 1999). To assess the impact of L. oryzophilus parthenogenetic populations on global rice- growing areas, we obtained distribution data in global rice-growing areas from the EarthStat database (Supplementary Figure S3; www.earthstat.com). The global rice-growing area was overlaid on the suitable areas of the populations under current and future climate scenarios. Additionally, we evaluated the proportion of the range of suitable areas for the species in the global rice-growing area to quantify the impact of this species parthenogenetic populations on global rice cultivation areas under current and future climate scenarios.
2.5 Hypervolume of climate ecological niche
In this study, we used four predictor variables (Bio18, Bio4, Bio10 & Bio16), which significantly contribute to the model results, to constructed ecological niche characterization of L. oryzophilus parthenogenetic populations for North America, Europe, and Asia. The analysis data comes from extracting values from four predictor variables using known occurrence points in ArcGIS 10.8. For each continent, we used a full set of occurrence records to construct a complete ecological niche hypervolume. We used the “hypervolume” package to analysis in R software (Blonder et al., 2018). Based on the selected four climate variables, we used the Z-score to standardize the four predictor variables for constructing three niche hypervolume structures in three continent and adopted a cross-validation approach to select the bandwidth of each variable axis for optimization (Blonder et al., 2018; Jin et al., 2024). Then we mapped hypervolumes onto orthogonal axes comprising pairs of climatic variables and characterized the ecological niche similarity of L. oryzophilus across the three continents by calculating hypervolume distances between centers of mass (Euclidean distances) and similarities between hypervolume structures (Sorensen indices) (Mammola, 2019).
2.6 Assessment of economic losses to rice production in China under current climate scenarios
We analyzed and assesses the potential economic losses to rice production in China caused by L. oryzophilus parthenogenetic populations under unmanaged scenario and managed scenario (Ullah et al., 2023; Xu et al., 2020):
Unmanaged scenario:
Potential economic loss caused by production quantity decline-F1
Managed scenario:
Management costs-F2
Potential economic loss after management-F3
Potential economic loss under management scenario-F4
Potential savings after management-F5
We obtained the statistical data on rice production and planting area for each province from 2018 to 2022 from the National Bureau of Statistics (http://www.stats.gov.cn/) and Strategic Reserves Administration (http://www.chinagrain.gov.cn/). Based on the market price of pesticides in China and the suitable area of the populations, we assessed the required investment in prevention cost (Xu et al., 2020; He et al., 2016). We combined the rice production and planting area data with the proportion of suitable areas for the populations in each province to calculate the annual rice production and planting area within its suitable areas (Qin et al., 2020). The specific indicator setting for harm and economic parameters under Unmanaged and managed scenarios are shown in Table 1. All parameters were entered into the @Risk software as distribution functions (using the Pert function for fitting), and a Latin hypercube sampling method was employed to draw samples from each distribution with a total of 100,000 iterations conducted (Ullah et al., 2023).
3 Results
3.1 Invasion history of L. oryzophilus
Since the first discovery the bisexual populations of this species in Texas in 1904, subsequent findings of the bisexual populations occurred within 20 years in Louisiana, Kansas, and Florida (Figures 2A, B). Following the initial report of the parthenogenetic populations in California in 1958, this populations rapidly invaded northern Latin America, eastern Asia, and southern Europe over the next 70 years, propelled by the acceleration of global trade (Figure 2A). As of 2020, the distribution of this species is primarily concentrated in the Northern Hemisphere, particularly in regions with extensive rice cultivation, such as China and Japan (Figure 2). Especially in China, since the first discovery of the parthenogenetic populations of L. oryzophilus in Hebei Province in 1988, this populations rapidly spread along the coastal regions of China during the 1990s. After entering the 21st century, the populations gradually showed a trend of spreading from the coastal areas to the inland regions. As of 2024, approximately 5/6 of the provinces have recorded the distribution of the parthenogenetic populations in China (Figure 2C).
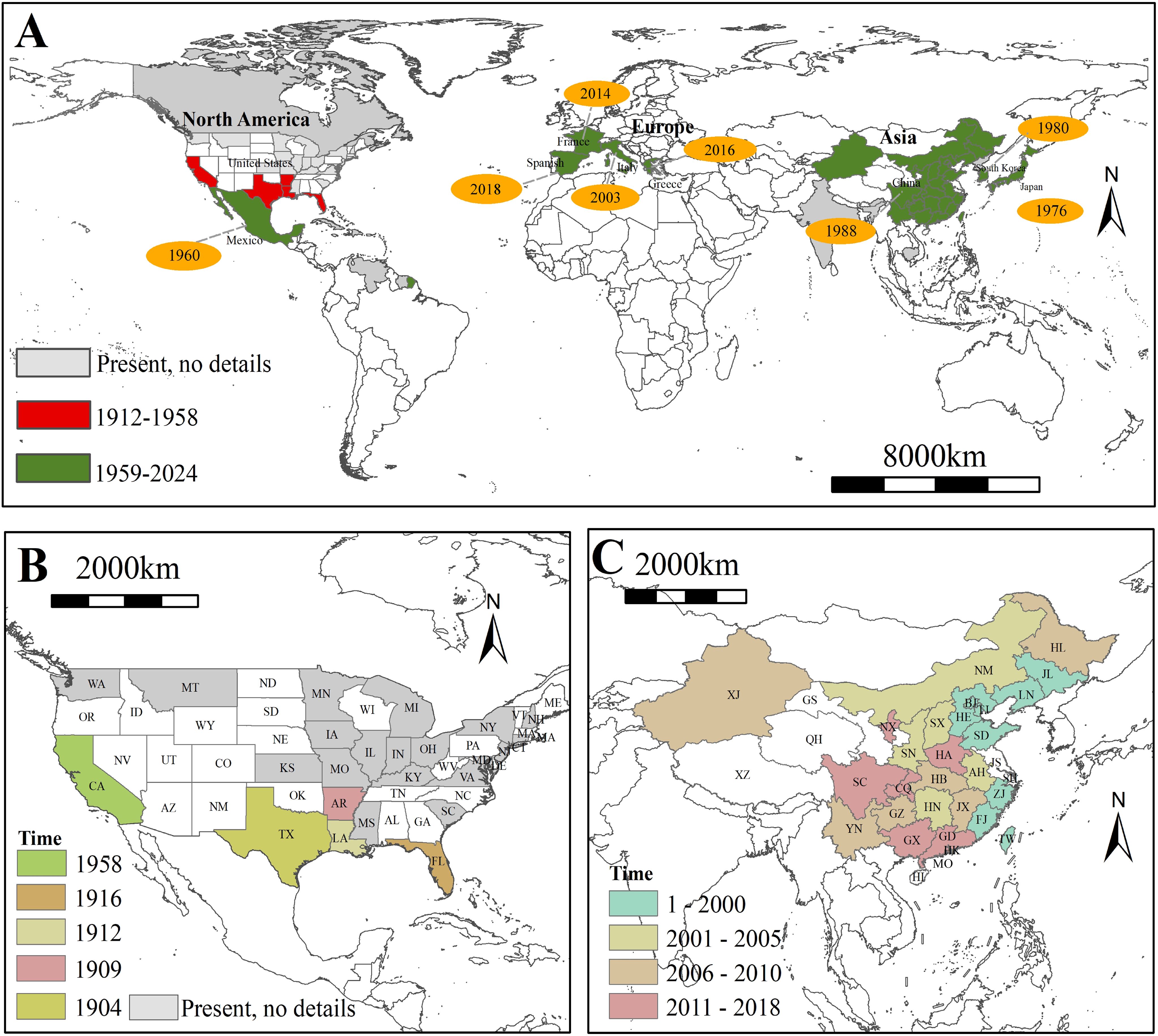
Figure 2. (A) Global invasion history reconstruction of Lissorhoptrus oryzophilus; (B) Historical spread dynamics of L. oryzophilus in the United States; (C) Historical spread dynamics of L. oryzophilus in China.
3.2 Model accuracy evaluation and importance of bioclimatic variables
Based on the model optimization results, with RM = 0.5 and FC = LQHP, the ΔAICc value was 0. Therefore, these were selected as the final parameter settings (Supplementary Figure S4A). The resulting mean training and testing AUC values were 0.939 (Supplementary Figure S4B), indicating the outcomes of the model were reliable. The precipitation of the warmest quarter (Bio18, 57.9%), temperature seasonality (Bio4, 22%), mean temperature of the warmest quarter (Bio10, 13.7%), and precipitation of the wettest quarter (Bio16, 1.7%) were the four most significant bioclimatic variables affecting the suitable areas of parthenogenetic populations (Supplementary Table S3). The effect of precipitation on the suitable areas of the populations was more significant than that of temperature, as indicated through the jackknife and percent contributions (Supplementary Table S1, Supplementary Figure S5). According to the precipitation during the warmest quarter (Bio18), the probability of survivability increased from 0.03 to 0.86 within the range of 26.50 to 562.20 mm and subsequently declined rapidly (Supplementary Figure S6A). The response curves of temperature seasonality (Bio4) showed that within the range of 1.86–751.26, the probability of occurrence increased from 0.006 to 0.92, rapidly decreased until approximately 976.63, the probability of occurrence increased from 0.92 to 0.38 (Supplementary Figure S6B). Regarding the Bio10 and Bio16 precipitation variables, the probability of occurrence peaked when the variables were 26.36°C and 1567.56 mm, respectively (Supplementary Figures S6C, D).
3.3 Suitable areas of L. oryzophilus parthenogenetic populations under the current climate
Current global suitable areas forecast of the populations are depicted in Figure 3. The global suitable areas for the parthenogenetic populations establishment was 1068.4 × 104 km2 under the current climate (Table 2). Highly suitable habitats for the populations are predicted in the northeastern and southern of China, the western Japan, and Korea peninsula (Figure 3A). The area of high suitability was 143.83 × 104 km2 (Table 2). Moderately suitable habitats for the populations are predicted in the northeastern and southern of China (Figure 3A). The area of moderate suitability was 209.67 × 104 km2 (Table 1). Lowly suitable habitats for the populations are predicted in eastern and southeastern United States, northern Argentina, southern Europe (Italy, Macedonia, Greece, France, Spain), western Asia (Turkey and Kazakhstan), northern Southeast Asia (Vietnam, Myanmar, Laos and northeastern India) and the northeastern and southern of China (Figure 3A). The area of low suitability was 714 × 104 km2 (Table 2).
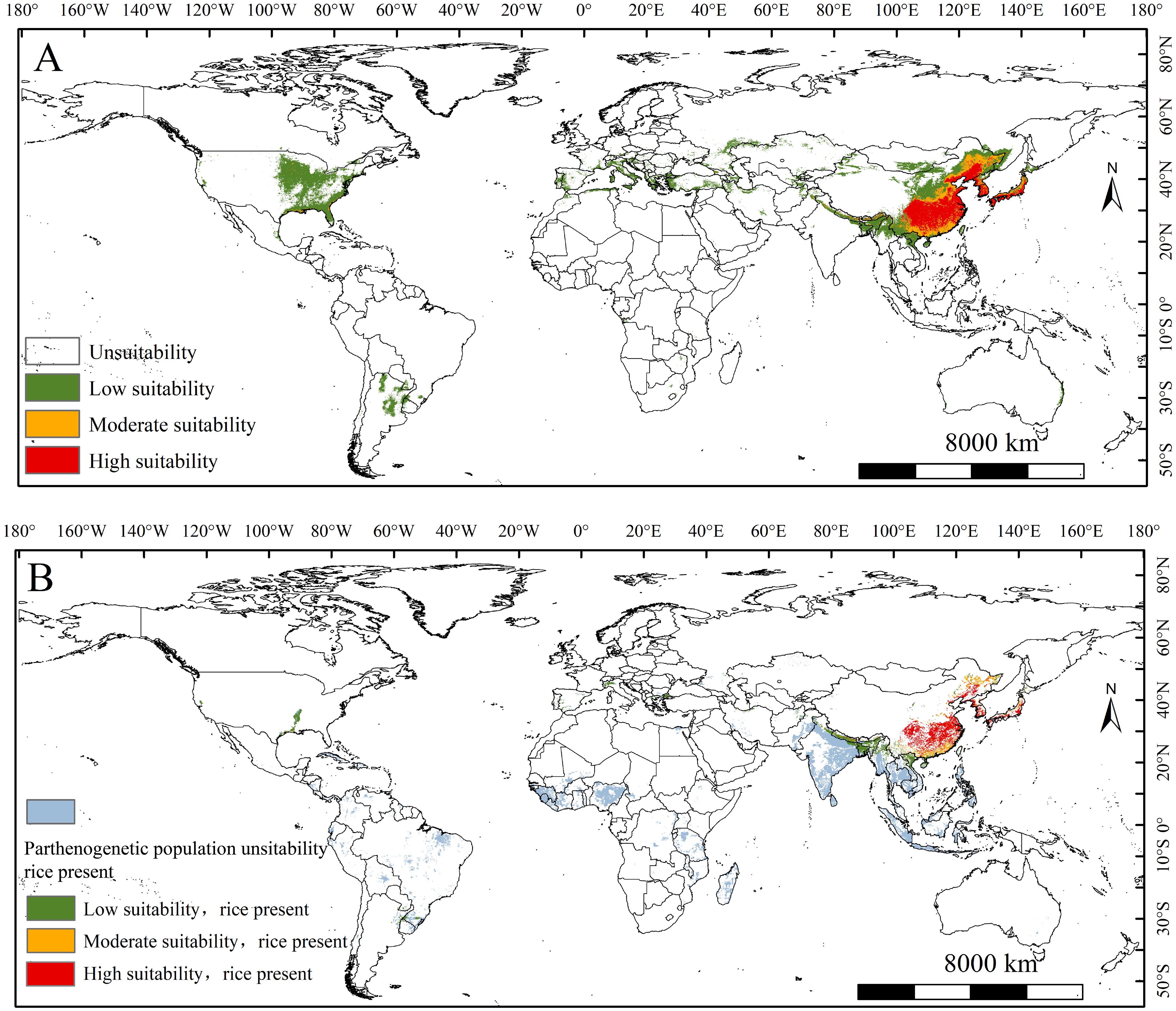
Figure 3. (A) The suitable areas of Lissorhoptrus oryzophilus parthenogenetic populations under current climatic scenarios. (B) The suitable areas of L. oryzophilus parthenogenetic populations in global rice-growing areas under current climatic scenarios.
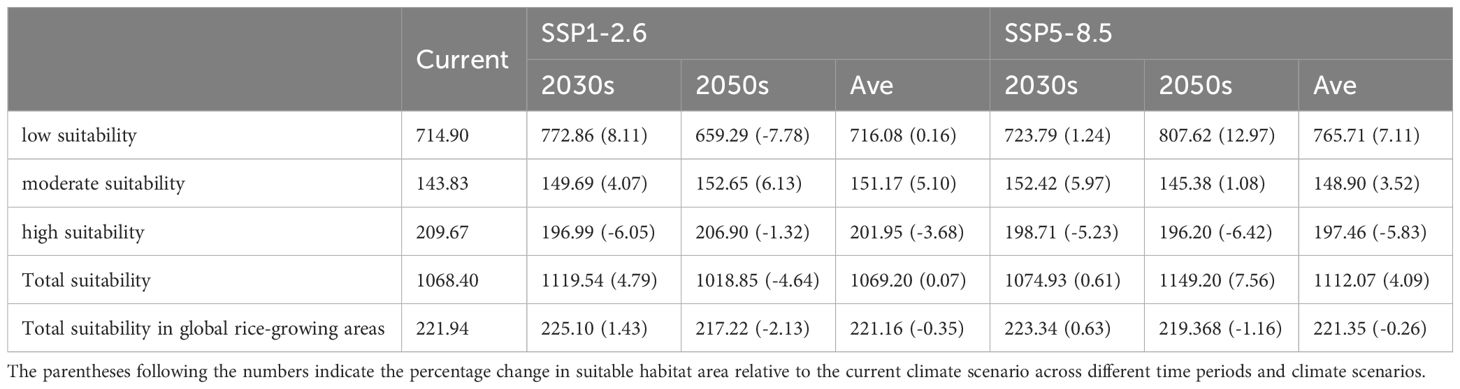
Table 2. Predicted suitable habitat areas for Lissorhoptrus oryzophilus parthenogenetic populations under current and future climatic scenarios (×104km2).
The range of suitable areas for the populations within the global rice-growing area is 221.94 × 104 km2, accounting for 30.6% of the total global rice-growing area under current conditions (Figure 3B, Table 2). The regions primarily affected by this populations in the rice industry include North America (the southern unite states), South America (Southern Brazil), southern Europe (Northern Italy and coastal areas of Spain), and Asia (northern Vietnam, northern Myanmar, northeastern and northern India, northeastern and southern of China, Western Korean Peninsula, Southern and Western Japan). Among these regions, the populations have notably impacted rice-growing areas in China, the Korean Peninsula, and Japan, with all major rice-growing areas concentrated in these countries and regions (Figure 3B).
3.4 Suitable areas of L. oryzophilus parthenogenetic populations under future climate scenarios
The suitable areas of the parthenogenetic populations under two climate change scenarios (SSP1-2.6 and SSP5-8.5) and in two time periods (2030s and 2050s) is shown in Figure 4. Overall, compared to current climate scenarios, the populations present insignificant changes in global suitability under two future climate scenarios. Its primary suitability remains concentrated in eastern North America, southern South America, southern Europe, and eastern Asia. Northeastern China, western Korean Peninsula, and western and southern parts of Japan continue to be identified as major high suitability areas (Figure 4). The low-emission SSP1-2.6 scenario suggested the total suitable habitat areas are 1069.20 × 104 km2, with an increase of 0.07% in the current suitable area for the establishment of the populations (Table 2). The low and moderate suitability areas cover 716.07 × 104 km2 and 151.17 × 104 km2 (increase 0.16% and 5.10% compared to the current area), while high suitability areas are 201.95 × 104 km2 (decrease 3.63% compared to the current area) (Table 2). The MaxEnt model further predicted higher habitat suitability areas and spread of RSW under high-emission scenario SSP5-8.5 in 2030s and 2050s. The high-emission SSP5-8.5 scenario suggested the total suitable habitat areas are 1111.80 × 104 km2, with an increase of 4.0% in the current suitable area for the establishment of the populations (Table 2). The low and moderate suitability areas cover 765.71 × 104 km2 and 151.17 × 104 km2 (increase 7.1% and 3.5% compared to the current area), while high suitability areas are 201.95 × 104 km2 (decrease 5.82% compared to the current area) (Table 2).
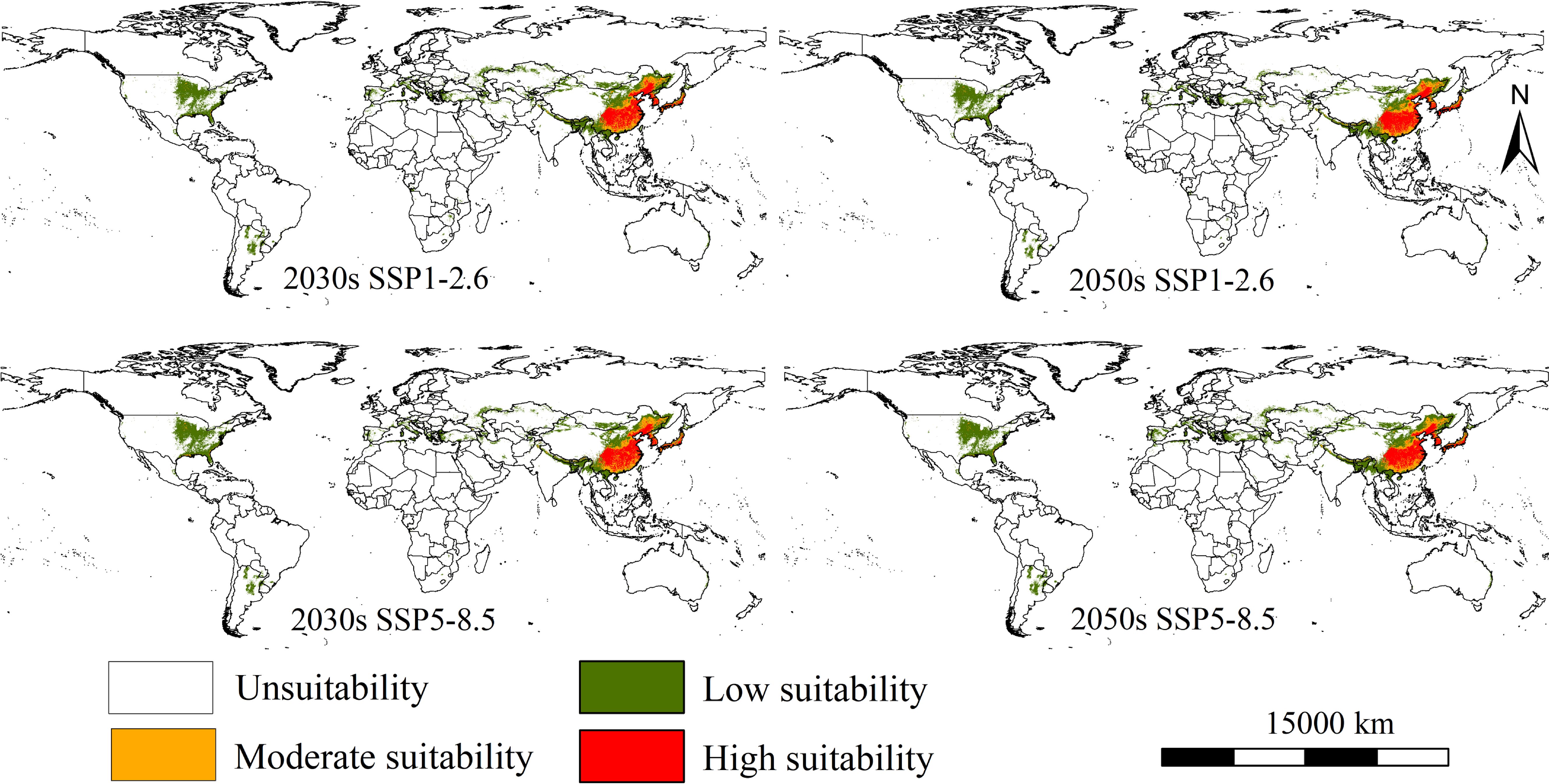
Figure 4. The suitable areas of Lissorhoptrus oryzophilus parthenogenetic populations under two future climatic scenarios in 2030s and 2050s (SSP1-2.6 (low-emission scenario) and SSP5-8.5 (high-emission scenario)).
Changes in the establishment risk of the populations in global rice-growing areas under two future climate scenarios is shown in Figure 5. Overall, sum of suitability areas will decrease to 221.16×104 km2 and 221.35×104 km2 (decrease 0.35% and 0.26% compared to the current area) (Table 2). The establishment risk of this populations in global rice-growing areas is primarily concentrated in North America (the southern unite states), South America (Southern Brazil), southern Europe (Northern Italy and coastal areas of Spain), and Asia (northern Vietnam, northern Myanmar, northeastern and northern India, northeastern and southern of China, Western Korean Peninsula, Southern and Western Japan) under SSP1-2.6 and SSP5-8.5 scenarios (Figure 5). Fortunately, the increased suitability areas for the establishment of the populations are generally stable, concentrated in northern and northeastern India, and northern and central Myanmar (Figure 5).
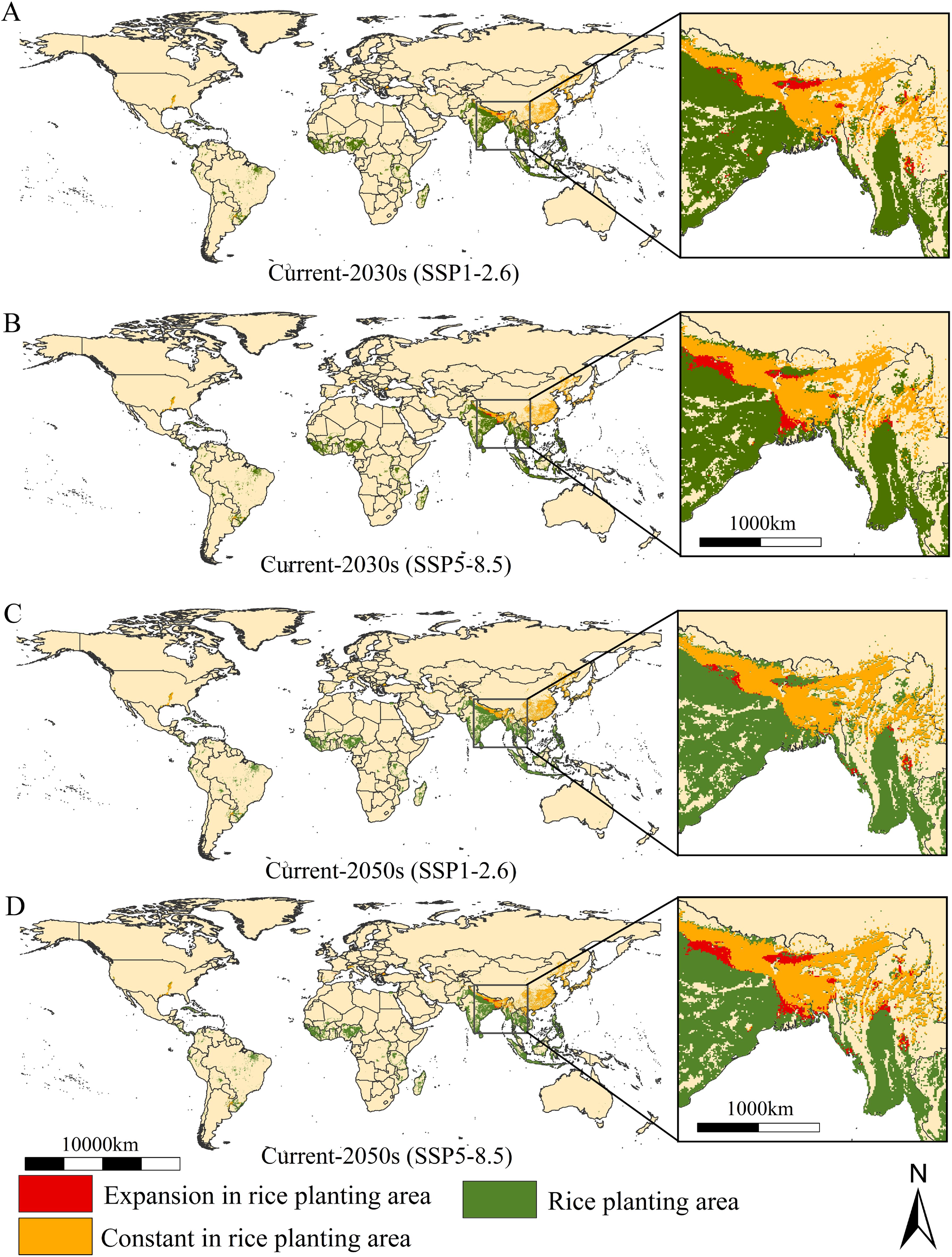
Figure 5. The change of the suitable areas of Lissorhoptrus oryzophilus parthenogenetic populations in global rice growing areas under future climatic scenarios from current to 2030s (A, B) and 2050s (C, D) under SSP1-2.6 (low-emission scenario) and SSP5-8.5 (high-emission scenario).
3.5 Ecological niche similarity of L. oryzophilus parthenogenetic populations in three continents
Here, we utilized the n-hypervolume framework to comprehensively assess the niche similarity between the native range (North America) and invasive ranges (Europe and Asia) using the Sorensen index and Euclidean distance (Table 3). Overall, this population exhibits significant differences in climatic niche occupancy between the invasive and native ranges. The Euclidean distance is 0.5495 between European and North American populations, with a Sorensen index of 0.1482. However, Asian population shows even greater Euclidean distance (0.6727) and a smaller Sorensen index (0.0133) relative to North American populations. Furthermore, compared to North America and Europe, this population occupies a larger and more distinct climatic niche space in Asia (Figure 6). Compared to its native range, this population occupies a climatic niche in Asia where, except for Bio10 (mean temperature of the warmest quarter) which has expanded towards lower levels, the other three variables (Bio4 (Temperature Seasonality), Bio16 (Precipitation of Wettest Quarter), Bio18 (Precipitation of Warmest Quarter)) have expanded towards higher levels (Figure 6).
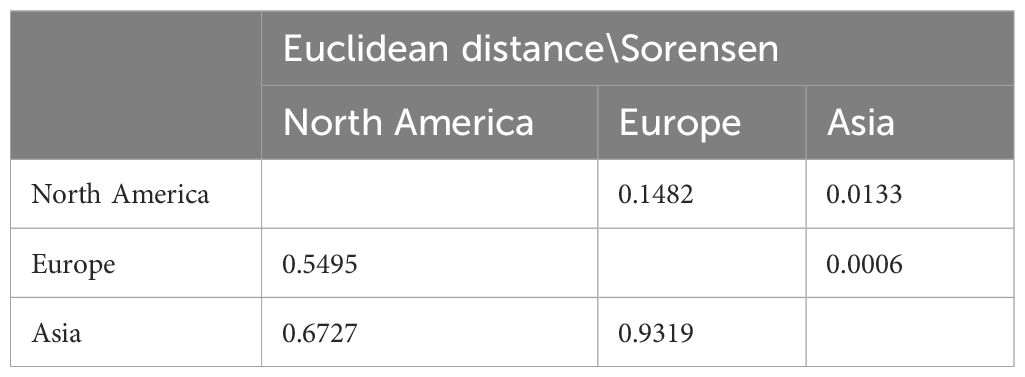
Table 3. Climatic ecological niche similarity of Lissorhoptrus oryzophilus parthenogenetic populations in North America, Europe, and Asia (above the diagonal is the Sorensen index, below the diagonal is centroid distance).
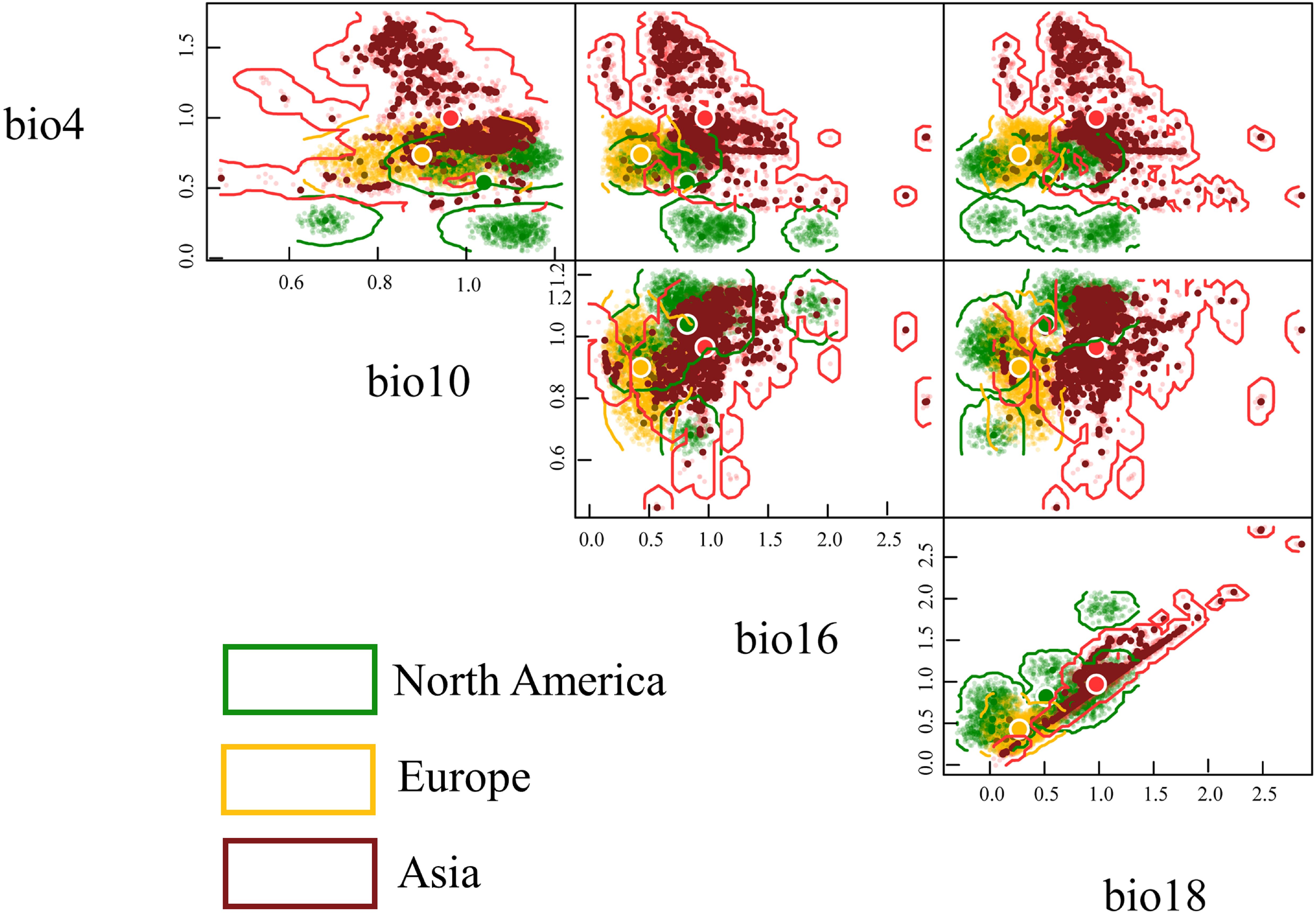
Figure 6. Based on the n-hypervolume and four key climatic variables to construct the climatic niche characteristics of Lissorhoptrus oryzophilus parthenogenetic populations across North America, Europe, and Asia (bio4 (Temperature Seasonality), bio10 (Mean Temperature of Warmest Quarter), bio16 (Precipitation of Wettest Quarter), bio18(Precipitation of Warmest Quarter)).
3.6 The potential economic losses caused by L. oryzophilus parthenogenetic populations in China and their controlling benefit
Under the unmanaged scenario (F1), the potential economic losses of L. oryzophilus parthenogenetic populations on the rice industry were estimated to range from $5.44 billion to $32.46 billion USD at a 95% confidence level. Sensitivity analysis results showed that the ranking of the factors influencing these potential losses under unmanaged scenario include the rice yield loss rate (R), the damage rate of rice (I), the rice market price (Pa), and the yield of rice in suitable habitats (Q) (Supplementary Figure S7A).
Under the management scenario (F2), the potential economic loss of rice in China due to the populations range from $0.10 billion to $0.83 billion USD at a 95% confidence level, with a mean value of $0.47 billion USD. The potential economic loss from management costs (F3) ranges from $0.26 billion to $2.19 billion USD at a 95% confidence level, while the loss after management (F4) ranges from $0.36 billion to $3.02 billion USD, with mean values of $1.22 billion and $1.69 billion USD, respectively. Potential savings after management (F5) range from $4.41 billion to $30.68 billion USD at a 95% confidence level, with a mean value of $17.54 billion USD. Sensitivity analysis results showed that the ranking of the factors influencing these potential losses under the management scenario includes the loss rate after the unit prevention cost (C), the damage rate of rice by the populations (I), the area of rice plants in suitable habitats (S), the yield of rice in suitable habitats (Q), the rice market price (Pa), and the control effect of the populations (M) (Supplementary Figure S7B).
4 Discussion
Rice is the most important crop in the world because half of the global populations consume it daily. In some Asian countries, rice provides over 70% of the calorie supply (GRiSP, 2013). Therefore, rice is considered one of the most strategically significant commodities worldwide, closely intertwined with global food security, economic growth, employment, social stability, and regional peace (Mehrabi et al., 2018). However, the transport of pests beyond their native ranges by human actions is breaking down biogeographical barriers and threatening local rice production, and invasive alien pests also continually explore new climate characteristics during this process (Capinha et al., 2015; Vilà et al., 2011). Indirectly, negative impact of climate change on rice yield by altering the suitable areas range of pests, increasing the occurrence of pests and diseases and exacerbating natural disasters, thus posing a threat to food security (Tilman et al., 2011; Lori, 2021; Rezaei et al., 2023).
4.1 Humid and hot environments are favorable for the occurrence of the populations
Our results revealed that the effect of precipitation factors on the prevalence of L. oryzophilus parthenogenetic populations was significantly greater than that of temperature factors. Specifically, the precipitation of the warmest quarter (Bio18) and accounted for 57.9% and 13.7% of the contribution rate to the MaxEnt result and ranging from 685.19 to 753.33 mm for the precipitation of the warmest quarter were considered high suitability for the populations. Previous studies showed that the populations oviposits in the submerged portion of the rice seedlings in summer and the peak oviposition typically coincides with the establishment of a flood, fostering accelerated larval growth rates under these conditions (Bowling, 1972; Tindall et al., 2013; Stout et al., 2002). Previous studies indicate that most 1st-generation adults end diapause after exposure to 10°C, 15°C, and 20°C, while diapause intensity is higher at 25°C, possibly to avoid extreme high temperatures after emergence (Jiang et al., 2004). However, the 2nd-generation adults are less likely to face high temperatures, resulting in lower diapause intensity (Jiang et al., 2004). In our result, the mean temperature the warmest quarter (Bio10) maintained between 10°C and 33°C, the probability of presence for this populations will exceed 0. The previous results corroborate our findings indirectly.
4.2 Frequent invasion events of the populations concentrated in the Northern Hemisphere for over half a century
In the late 1950s, a parthenogenic populations of L. oryzophilus was first recorded in northern California (Lange and Grigarick, 1959). In the mid-1970s, the parthenogenetic populations of rice water weevils presumably “hitchhiked” across the Pacific into Aichi Prefecture, Japan (Iwata, 1979). After a decade of spreading, this populations quickly proliferated across the entire Japanese archipelago (Matsui, 1987). In Aichi Prefecture, Japan, the population is univoltine, with overwintered adults migrating to rice fields during daytime when temperatures exceed 20°C in May and June. By late July, eggs and larvae are found in the rice fields (Matsui, 1987). In southern Japan, the population is bivoltine, with very low larval densities on late-planted rice seedlings (Matsui, 1987). Despite agricultural and chemical control measures targeting the parthenogenetic populations in Japan, some individuals among the 150 rice varieties planted still exhibit high tolerance (Matsui, 1987). In 1988, the populations were recorded in mainland China and the Korean Peninsula, while Taiwan first reported their presence in Taoyuan County in March 1990 (Sun et al., 1996). In Korea, the populations were primarily univoltine, with occasional second-generation appearances in some years (Li, 1993; Sun et al., 1996). The spread and velocity of the populations in Korea are influenced by wind, mountainous terrain, and ground transportation (Uhm et al., 1989). Since the 21st century began, the populations were detected for the first time in Europe in 2004 (Caldara et al., 2004). Since then, the pest has rapidly spread across northern Italy, where rice cultivation is prevalent and the interconnected rice fields create corridors that aid its spread (Lupi et al., 2010; Wang et al., 2011). By 2020, this populations had established widespread populations in rice-growing regions across Europe, including Macedonia, Greece, France, Spain (Giantsis et al., 2017; Montauban et al., 2021).
4.3 The establishment hotspot areas concentrated in East Asia for the populations
The suitable areas of L. oryzophilus parthenogenetic populations were predominantly concentrated in rice-planting areas of the United States, Brazil, Argentina, Italy, Spain, Bulgaria, China, Nepal, Myanmar, Laos, Vietnam, India, the Korean Peninsula and Japan under current and future climate scenarios. It’s noteworthy that nearly all rice-growing areas in East Asia are covered by high-suitability areas for this populations, including China (northeastern, central and southern China), the Korean Peninsula, and Japan. Based on the results predicted by CLIMEX, Mao et al. (1997) found that the suitable areas are concentrated in Northeast, North, East, Central, South, and Southwest China. Qi et al. (2012) and Xie et al. (2022) used the MaxEnt model to analyze the suitable areas pattern of the populations in China reported high-suitability areas located primarily in northeastern, central and southern China. This also aligns with our results in China. Based on the analysis of climatic niche characteristics using the ecological niche hypervolume framework, the results indicate that compared to the native North American populations, the Asian populations occupied a larger climatic niche space, with significant climatic niche differentiation between the two populations. This isn’t surprising, as populations of L. oryzophilus that reproduce through parthenogenesis possess allowing them to rapidly adapt to changing environments and establish a sustainable populations within a short timeframe (Huang et al., 2017). Populations genetic studies have shown that there is a certain degree of genetic differentiation within the parthenogenetic populations between populations from the United States, Europe, and Asia, and among different geographic subgroups within Asia (Yang, 2008). Additionally, species invasion depends on the availability of host plants and the extent to which intruders require specific host plant species or are able to adapt to new species (Aljaryian et al., 2016). The extensive rice-growing areas in Asia have indeed provided a conducive environment for the spread of this populations to a certain extent (Huang et al., 2017). Furthermore, human activities have contributed to the spread of this beetle in Asia, especially in China. Despite geographical barriers, transporting rice seedlings, straw, and soil contaminated by the beetle could inadvertently introduce it to neighboring villages and counties (Huang et al., 2017). Notably, it appears that countries in West Africa, despite being major rice-growing regions, do not seem to face potential invasion by this populations. The two climatically distinct rice growing periods – dry and wet seasons – are crucial for rice double cropping in West Africa (Dingkuhn and Miezan, 1995). During the dry season, the region experiences low air temperatures and minimal solar radiation due to dry and dusty conditions. In the late dry season, temperature gradually temperatures gradually rise. During the wet season, the region shows a climate gradient from hot, humid and rainy (Average annual rainfall of 1150 mm) in the south to very hot and dry with low rainfall (Annual precipitation is about 200mm) in the north (Ibrahim et al., 2024). In Southeast Asia, the probability of extreme precipitation has increased from 1979 to 2019, with areas receiving over 2000 mm of annual rainfall accounting for 90.59% (Xu et al., 2022; Jin et al., 2023). This has contributed to a rise in flooding events, increasing the risk of waterlogging for the populations in this region (Aghaee and Godfrey, 2017). Therefore, extreme environmental changes have limited the occurrence of the rice weevil in West Africa to some extent. In the future, the establishment range of expansion will primarily be located in higher-altitude regions, such as the northeastern parts of Nepal and India. This is not surprising, as past studies have shown that global warming will further drive the beetles to expand into higher-altitude areas (Michael et al., 2022). This reflects the geographical response of insect to environmental changes, and it also indicates that the rice industry of these new regions will likely receive more attention in the future.
4.4 The potential economic loss of the populations is huge but recoverable in China
In recent decades, research on the potential economic impacts of IAPs on staple crop industry has significantly increased, focusing primarily on post-event impact assessments and evaluations of management effectiveness. For instance, Qin et al. (2020) utilized @RISK methodology to forecast the potential economic impact of Spodoptera frugiperda on maize industry in China. Without management measures, S. frugiperda is projected to cause potential economic losses ranging from 5.27 billion to 46.04 billion dollars (90% confidence level), while potential savings with management could amount to 3.57 billion to 40.93 billion dollars (90% confidence level).
In 1986, this species was classified as a national quarantine pest (Zhou, 1987). Two years later, a parthenogenetic population was first discovered in Tanghai, Hebei Province, and it rapidly spread along the coastal areas. Since the early 21st century, it has begun to move into central, southwestern, northwestern, and northeastern China (Huang et al., 2017). By July 2024, it had spread to 5/6 provinces in China (http://www.moa.gov.cn/govpublic/ZZYGLS/202409/t20240902_6461580.htm), becoming one of the most severe and rapidly spreading invasive pests in the country. Considering China is the largest producer of rice around the world, and the suitable areas of L. oryzophilus parthenogenetic populations cover nearly all rice-growing areas in China. Therefore, our study on potential economic losses focuses on China as a representative case. Based on @RISK software, the forecasted potential economic losses caused by L. oryzophilus parthenogenetic populations on rice industry show that the potential economic losses range from $5.44 billion to $32.46 billion USD (95% confidence level) under nil management scenario. However, the potential economic losses range from $0.10 billion to $0.83 billion USD (95% confidence level) after factoring in management costs, recovering over 90% of the potential economic losses. Sensitivity analysis reveals that the infestation rate of the populations and unit prevention cost is key input variable influencing potential economic losses under unmanaged scenario and managed scenario, respectively. Therefore, from an economic benefit perspective, implementing appropriate control measures in rice-growing regions affected by this global invasive pest is necessary and effective.
4.5 Better management of the establishment and spread of the populations
Since the initial discovery of L. oryzophilus parthenogenetic populations in the United States in 1958, the populations have rapidly spread across global rice-growing areas and establish stable populations. Our research highlights that East Asia contains extensive highly suitable areas for this populations, posing a significant threat to rice production in the region. Fortunately, a part of affected countries have implemented various control measures to mitigate the impact of the populations on local rice crops. In the U.S, as a semi-aquatic insect that relies on flooded conditions for larval development, the populations can be lower in a furrow irrigated rice system than the flooded field. Therefore, the furrow irrigated rice system may reduce the damage caused by this population (Kelly et al., 2021). During the fallow period, removing weeds from the rice field embankments and surrounding areas to reduce the abundance of host plants for the rice weevil may effectively lower its population density, thereby reducing the overall density in the rice fields (Palrang et al., 1994). This approach could be highly effective as it is easy for farmers to implement, making it suitable for promotion in China and other countries where the pest has become invasive (Huang et al., 2017). As a more efficient chemical control method, Chlorantraniliprole and its mixtures with other neonicotinoids chemicals (e.g., triazophos and thiamethoxam) are the primary chemical controls used in China (Huang et al., 2017). However, diamide seed treatments offer superior yield protection compared to neonicotinoids under high densities of the pest (Nicholas et al., 2024). Biological control and tolerance host plants breeding has shown applied promise. For instance, the highly resistant variety T03 was identified by comparing the adult feeding and oviposition preferences, egg hatching rate, larval survival rate among different rice varieties (Li et al., 2011). For potential but not yet realized suitable areas, it is crucial to establish official quarantine zones to prevent further spread. Strict quarantine on importing seedlings, straw, and soil from infected to non-infected areas, and avoiding the use of quarantine straw as packaging material, are essential (Huang et al., 2017).
5 Conclusion
Based on the analysis using the MaxEnt model, the precipitation of the warmest quarter (Bio18) has been identified as the primary environmental factor influencing the habitat suitability of L. oryzophilus parthenogenetic populations. During its invasion process, the populations has explored new climatic conditions, particularly in East Asia. The results indicate that this populations pose the greatest threat to major rice-producing regions in Asia under current and future climate scenarios, particularly rice-growing areas in China, the Korean Peninsula, and Japan. Specially, the populations would cost the rice industry in China 18.95 billion US dollars under unmanaged measures. Fortunately, climate change has not significantly altered the current global distribution pattern of this populations within the rice-growing regions and it is possible to recover 93% of the economic losses in China through reasonable chemical control, such as the application of triazophos and thiamethoxam. Our research findings will support in monitoring, surveillance, and the development of early warning systems to control L. oryzophilus parthenogenetic populations worldwide. Policymakers and governments can utilize these findings to develop effective integrated pest management strategies to handle potential future outbreaks of this populations.
Data availability statement
The raw data supporting the conclusions of this article will be made available by the authors, without undue reservation.
Author contributions
LL: Data curation, Writing – original draft. ZJ: Conceptualization, Data curation, Methodology, Writing – review & editing. ML: Methodology, Writing – review & editing. YX: Conceptualization, Writing – review & editing. JG: Conceptualization, Writing – review & editing. DJ: Conceptualization, Writing – review & editing. RM: Conceptualization, Writing – review & editing. ZL: Conceptualization, Writing – review & editing. XX: Conceptualization, Writing – review & editing. WL: Conceptualization, Funding acquisition, Writing – review & editing.
Funding
The author(s) declare financial support was received for the research, authorship, and/or publication of this article. This work was supported by the National Key R&D Program of China (Grant No. 2022YFC2601400 and 2021YFC2600400) and the Innovation Program of the Chinese Academy of Agricultural Sciences (Grant No. caascx-2022-2025-IAS).
Conflict of interest
The authors declare that the research was conducted in the absence of any commercial or financial relationships that could be construed as a potential conflict of interest.
Publisher’s note
All claims expressed in this article are solely those of the authors and do not necessarily represent those of their affiliated organizations, or those of the publisher, the editors and the reviewers. Any product that may be evaluated in this article, or claim that may be made by its manufacturer, is not guaranteed or endorsed by the publisher.
Supplementary material
The Supplementary Material for this article can be found online at: https://www.frontiersin.org/articles/10.3389/fpls.2024.1506418/full#supplementary-material
References
Adams, A., Gore, J., Musser, F., Cook, D., Catchot, A., Walker, T., et al. (2015). Impact of water management on efficacy of insecticide seed treatments against Rice water weevil (Coleoptera: Curculionidae) in Mississippi Rice. J. Econ. Entomol. 108, 1079–1085. doi: 10.1093/jee/tov095
Aghaee, M. A., Godfrey, L. D. (2014). A century of rice water weevil (Coleoptera: curculionidae): A history of research and management with an emphasis on the United States. J. Integr. 5, D1–D14. doi: 10.1603/IPM14011
Aghaee, M. A., Godfrey, L. D. (2015). The Efficacy of Bacillus thuringiensis spp. galleriae Against Rice Water Weevil (Coleoptera: Curculionidae) for Integrated Pest Management in California Rice. J. Econ. Entomol. 108, 45–52. doi: 10.1093/jee/tou024
Aghaee, M. A., Godfrey, L. D. (2017). Winter flooding of California rice fields reduces immature population of Lissorhoptrus oryzophilus (Coleoptera: Curculionidae) in the spring. Pest. Manage. Sci. 73, 1538–1546. doi: 10.1002/ps.4507
Aljaryian, R., Kumar, L., Taylor, S. (2016). Modelling the current and potential future distributions of the sunn pest Eurygaster integriceps (Hemiptera: Scutelleridae) using CLIMEX. Pest. Manage. Sci. 72, 1989–2000. doi: 10.1002/ps.4247
Bin Rahman, A. N. M., Zhang, J. (2023). Trends in rice research: 2030 and beyond. Food. Energy. Secur. 12, e390. doi: 10.1002/fes3.390
Blonder, B., Lamanna, C., Violle, C., Enquist, B. J. (2014). The n-dimensional hypervolume. Glob. Ecol. Biogeogr. 23, 595–609. doi: 10.1111/geb.12146
Blonder, B., Morrow, C. B., Maitner, B., Harris, D. J., Lamanna, C., Violle, C., et al. (2018). New approaches for delineating n-dimensional hypervolumes. Methods Ecol. Evol. 9, 305–319. doi: 10.1111/2041-210X.12865
Bowling, C. C. (1972). Note on the biology of rice water weevil, Lissorhoptrus oryzophilus1. Ann. Entomol. Soc Am. 65, 990–991. doi: 10.1093/aesa/65.4.990
Caldara, R., Diotti, L., Regalin, R. (2004). First record for Europe of the rice water weevil, Lissorhoptrus oryzophilus Kuschel (Coleoptera, Curculionoidea, Erirhinidae). Boll. Zool. Agr. Bachic. Ser. II. 36, 165–171. Available at: https://api.semanticscholar.org/CorpusID:82482079 (Accessed February 25, 2024).
Capinha, C., Essl, F., Seebens, H., Moser, D., Pereira, H. M. (2015). The dispersal of alien species redefines biogeography in the Anthropocene. Science 348, 1248–1251. doi: 10.1126/science.aaa8913
Cardinale, B. J., Srivastava, D. S., Emmett Duffy, J., Wright, J. P., Downing, A. L., Sankaran, M., et al. (2006). Effects of biodiversity on the functioning of trophic groups and ecosystems. Nature 443, 989–992. doi: 10.1038/nature05202
Chen, H., Chen, Z., Zhou, Y. (2005). Rice water weevil (Coleoptera: Curculionidae) in mainland China: Invasion, spread and control. Crop Prot. 24, 695–702. doi: 10.1016/j.cropro.2004.12.005
Daehler, C. C. (2003). Performance comparisons of co-occurring native and alien invasive plants: implications for conservation and restoration. Annu. Rev. Ecol. Evol. S. 34, 183–211. doi: 10.1146/annurev.ecolsys.34.011802.132403
de Godoy, I. B. S., McGrane-Corrigan, B., Mason, O., Moral, R. A., Godoy, W. A. C. (2023). Plant-host shift, spatial persistence, and the viability of an invasive insect population. Ecol. Model. 475, 110172. doi: 10.1016/j.ecolmodel.2022.110172
Dingkuhn, M., Miezan, K. M. (1995). Climatic determinants of irrigated rice performance in the Sahel — II. Validation of photothermal constants and characterization of genotypes. Agric. Syst. 48, 411–433. doi: 10.1016/0308-521X(94)00028-J
Dudík, M., Schapire, R. E., Phillips, S. J. (2005). Correcting sample selection bias in maximum entropy density estimation. In Proceedings of the 18th International Conference on Neural Information Processing Systems (NIPS'05). MIT Press, Cambridge, MA, USA, 323–330.
Dukes, J. S., Mooney, H. A. (1999). Does global change increase the success of biological invaders? Trends. Ecol. Evol. 14, 135–139. doi: 10.1016/S0169-5347(98)01554-7
Elith, J., Phillips, S. J., Hastie, T., Dudík, M., Chee, Y. E., Yates, C. J. (2011). A statistical explanation of MaxEnt for ecologists. Divers. Distrib. 17, 43–57. doi: 10.1111/j.1472-4642.2010.00725.x
Favretti, M. (2018). Remarks on the maximum entropy principle with application to the maximum entropy theory of ecology. Entropy 20, 11. doi: 10.3390/e20010011
Ferrero, A. (2005). Ecological and economic sustainability of rice cultivation in Europe and the Mediterranean region. First Asia-Europe Workshop on Sustainable Resource Management and Policy Options for Rice Ecosystems (SUMAPOL 2005), Hangzhou, Zhejiang Province, P.R. China.
Ficetola, G. F., Thuiller, W., Miaud, C. (2007). Prediction and validation of the potential global distribution of a problematic alien invasive species — the American bullfrog. Divers. Distrib. 13, 476–485. doi: 10.1111/j.1472-4642.2007.00377.x
Giantsis, I. A., Castells Sierra, J., Chaskopoulou, A. (2017). The distribution of the invasive pest, rice water weevil Lissorhoptrus oryzophilus Kuschel (Coleoptera: Curculionidae), is expanding in Europe: First record in the Balkans, confirmed by CO1 DNA barcoding. Phytoparasitica 45, 147–149. doi: 10.1007/s12600-017-0576-z
Graham, M. H. (2003). Confronting multicollinearity in ecological multiple regression. Ecology 84, 2809–2815. doi: 10.1890/02-3114
Graziosi, I., Rieske, L. K. (2014). Potential fecundity of a highly invasive gall maker, Dryocosmus kuriphilus (Hymenoptera: Cynipidae). Environ. Entomol. 43, 1053–1058. doi: 10.1603/EN14047
Grigarick, A. A., Beards, G. W. (1965). Ovipositional habits of the rice water weevil in California as related to a greenhouse evaluation of seed treatments. J. Econ. Entomol. 58, 1053–1056. doi: 10.1093/jee/58.6.1053
GRiSP (2013). Rice almanac. 4th ed (Los Baños, Laguna, Philippines: International Rice Research Institute).
Guisan, A., Petitpierre, B., Broenniman, O., Daehler, C., Kueffer, C. (2014). Unifying niche shift studies: Insights from biological invasions. Trends. Ecol. Evol. 29, 260–269. doi: 10.1016/j.tree.2014.02.009
Harrington, R., Clark, S. J., Welham, S. J., Verrier, P. J., Denholm, C. H., Hulle, M., et al. (2007). Environmental change and the phenology of European aphids. Glob. Change. Biol. 13, 1550–1564. doi: 10.1111/j.1365-2486.2007.01394.x
He, Y. F., Zhang, Z. C., Ye, Z. C., Zhang, C. R., Chen, X. J., He, Y. H., et al. (2016). The Control Efficiency of Different Insect Pesticides against Lissorhoptrus oryzophilus Larvae. AGROCHEMICALS 55, 607–609. doi: 10.16820/j.cnki.1006-0413.2016.08.018
Hill, M. P., Bertelsmeier, C., Clusella-Trullas, S., Garnas, J., Robertson, M. P., Terblanche, J. S. (2016). Predicted decrease in global climate suitability masks regional complexity of invasive fruit fly species response to climate change. Biol. Invasions. 18, 1105–1119. doi: 10.1007/s10530-016-1078-5
Huang, Y. S., Way, M. O., Jiang, M. (2017). “Rice water weevil Lissorhoptrus oryzophilus kuschel,” in Biological Invasions and Its Management in China, vol. 11 . Eds. Wan, F., Jiang, M., Zhan, A. (Springer, Dordrecht). doi: 10.1007/978-94-024-0948-2_9
Huber, V., Geist, J. (2019). Host fish status of native and invasive species for the freshwater mussel Anodonta anatina (Linnaeus 1758). Biol. Conserv. 230, 48–57. doi: 10.1016/j.biocon.2018.12.007
Ibrahim, A., Stuerz, S., Manneh, B., Rebolledo, M. C., Saito, K. (2024). Consistent yield performance of rice genotypes grown under irrigated conditions in wet and dry seasons in West Africa. Field. Crops. Res. 306, 109231. doi: 10.1016/j.fcr.2023.109231
Iwata, T. (1979). Invasion of the rice water weevil, Lissorhoptrus oryzae Kuschel, into Japan, spread of its distribution and abstract of the research experiments conducted in Japan. Jpn. Pestic. Inf. 36, 14–21. Available at: https://eurekamag.com/research/000/686/000686089.php (Accessed February 27, 2024).
Jarnevich, C. S., Stohlgren, T. J., Kumar, S., Morisette, J. T., Holcombe, T. R. (2015). Caveats for correlative species distribution modeling. Ecol. Inform. 29, 6–15. doi: 10.1016/j.ecoinf.2015.06.007
Jiang, M. X., Way, M. O., Du, X. K., Ji, X. H., He, Y. (2008). Reproductive biology of summer/fall populations of rice water weevil, Lissorhoptrus oryzophilus Kuschel, in Southeastern Texas. Southwest. Entomol. 33, 129–137. doi: 10.3958/0147-1724-33.2.129
Jiang, M. X., Zhang, W. J., Cheng, J. A. (2004). Termination of reproductive diapause in the rice water weevil with particular reference to the effects of temperature. Appl. Entomol Zool. 39, 683–689. doi: 10.1303/aez.2004.683
Jin, X. H., Li, P., Li, X., Zhang, G. Z. (2023). A CHELSA-based analysis of climate change characteristics and trends in South and Southeast Asia (SSEA) during 1980-2018. Prog. Geography. 42, 1365–1379. doi: 10.18306/dlkxjz.2023.07.011
Jin, Z. A., Yu, W. T., Zhao, H. X., Xian, X. Q., Jing, K. T., Yang, N. W., et al. (2022). Potential Global Distribution of Invasive Alien Species, Anthonomus grandis Boheman, under Current and Future Climate Using Optimal MaxEnt Model. Agriculture 12, 1759. doi: 10.3390/agriculture12111759
Jin, Z. A., Zhao, H. X., Xian, X. Q., Li, M., Qi, Y. H., Guo, J. Y., et al. (2024). Early warning and management of invasive crop pests under global warming: estimating the global geographical distribution patterns and ecological niche overlap of three Diabrotica beetles. Environ. Sci. pollut. Res. 31, 13575–13590. doi: 10.1007/s11356-024-32076-9
Kelly, F. R., Gore, J., Cook, D., Catchot, A. L., Golden, B. R., Krutz, L. J., et al. (2021). Influence of soil moisture zones on rice water weevil (Coleoptera: Curculionidae) population in furrow irrigated rice. Environ. Entomology. 50, 658–662. doi: 10.1093/ee/nvaa182
Lange, W. H., Grigarick, A. A. (1959). Rice water weevil, beetle pest in rice growing areas of southern states discovered in California. Calif. Agric. 13, 10–11.
Larson, E. R., Olden, J. D. (2012). Using avatar species to model the potential distribution of emerging invaders. Global. Ecol. Biogeogr. 21, 1114–1125. doi: 10.1111/j.1466-8238.2012.00758.x
Li, Z. H., Qin, Y. J. (2018). Review on the quantitative assessment models for pest risk analysis and their comparison. Zhiwu. Baohu. 44, 134–145. doi: 10.16688/j.zwbh.2018315
Li, Z. Q., Yu, F. Q., Tian, C. H., Sun, F. Y. (2011). Inheritance analysis of the resistance to rice water weevil in rice cultiva T03. Liaoning. Agric. Sci. 5, 25–28.
Liu, C., Berry, P. M., Dawson, T. P., Pearson, R. G. (2005). Selecting thresholds of occurrence in the prediction of species distributions. Ecography 28, 385–393. doi: 10.1111/j.0906-7590.2005.03957.x
Lori, L. (2021). Invasive ant establishment, spread, and management with changing climate. Curr. Opin. Insect. Sci. 47, 119–124. doi: 10.1016/j.cois.2021.06.008
Lupi, D., Colombo, M., Giudici, M. L., Villa, B., Cenghialta, C., Passoni, D. (2010). On the spatial spread of the Rice Water Weevil, Lissorhoptrus oryzophilus Kuschel (Coleoptera: Erirhinidae), in Italy. JEAR 42, 81–90. doi: 10.4081/jear.2010.81. (Accessed February 27, 2024)
Lupi, D., Jucker, C., Rocco, A., Giudici, M. L., Boattin, S., Colombo, M. (2015). Current status of the rice water weevil Lissorhoptrus oryzophilus in Italy: eleven-year invasion. EPPO. Bulletin. 45, 123–127. doi: 10.1111/epp.12183
Ma, G., Ma, C. S. (2022). Potential distribution of invasive crop pests under climate change: incorporating mitigation responses of insects into prediction models. Curr. Opin. Insect. Sci. 49, 15–21. doi: 10.1016/j.cois.2021.10.006
Mammola, S. (2019). Assessing similarity of n-dimensional hypervolumes: Which metric to use? J. Biogeogr. 46, 20122023. doi: 10.1111/jbi.13618,
Mao, Z. N., Sun, R. C., Dou, X. Z., Chen, H., Zhou, M., Qu, G. M., et al. (1997). Effects of low humidity and temperature on the mortality of rice water weevil and its potential distribution in China. Plant Quarantine (Chinese) 11, 26–29. doi: 10.19662/j.cnki.issn1005-2755.1997.s1.015
Matsui, M. (1987). Expansion of distribution area of the rice water weevil and methods of controlling the insect pest in Japan. Jpn. Agric. Res. Q. 20, 166–173.
Mehrabi, Z., Ellis, E. C., Ramankutty, N. (2018). The challenge of feeding the world while conserving half the planet. Nat. Sustain. 1, 409–412. doi: 10.1038/s41893-018-0119-8
Merow, C., Smith, M. J., Silander, J. A. (2013). A practical guide to MaxEnt for modeling species’ distributions: what it does, and why inputs and settings matter. Ecography 36, 1058–1069. doi: 10.1111/j.1600-0587.2013.07872.x
Michael, H., Lucas, P., Allan, C. (2022). Landscape predictions of western balsam bark beetle activity implicate warm temperatures, a longer growing season, and drought in widespread irruptions across British Columbia. For. Ecol. Management. 508, 120047. doi: 10.1016/j.foreco.2022.120047
Montauban, C., Mas, M., Wangensteen, O. S., Monteys, V. S., Fornós, D. G., Mola, X. F., et al. (2021). Bats as natural samplers: First record of the invasive pest rice water weevil Lissorhoptrus oryzophilus in the Iberian Peninsula. Crop Prot. 141, 105427. doi: 10.1016/j.cropro.2020.105427
Morales, N. S., Fernández, I. C., Baca-González, V. (2017). MaxEnt’s parameter configuration and small samples: are we paying attention to recommendations? A systematic review. Peer. J. 5, e3093. doi: 10.7717/peerj.3093
Muscarella, R., Galante, P. J., Soley-Guardia, M., Boria, R. A., Kass, J. M., Uriarte, M., et al. (2014). ENMeval: An R package for conducting spatially independent evaluations and estimating optimal model complexity for MaxEnt ecological niche models. Methods Ecol. Evol. 5, 1198–1205. doi: 10.1111/2041-210X.12261
Nicholas, R. B., Benjamin, C. T., Crow, W. D., Tyler, B. T., Don, R. C., Gus, M. L., et al. (2024). Potential exposure of honey bees to neonicotinoid seed treatments in US rice. Environ. Entomology 53, 716–722. doi: 10.1093/ee/nvae057
Pack, K. E., Mieszkowska, N., Rius, M. (2022). Rapid niche shifts as drivers for the spread of a non-indigenous species under novel environmental conditions. Divers. Distrib. 28, 596–610. doi: 10.1111/ddi.13471
Palrang, A. T., Grigarick, A. A., Oraze, M. J., Hesler, L. S. (1994). Association of levee vegetation to rice water weevil (Coleoptera: Curculionidae) infestation in California rice. J. Econ. Entomol. 87, 1701–1706. doi: 10.1093/jee/87.6.1701
Phillips, S. J., Dudík, M. (2008). Modeling of species distributions with MaxEnt: new extensions and a comprehensive evaluation. Ecography 31, 161–175. doi: 10.1111/j.0906-7590.2008.5203.x
Pili, A. N., Tingley, R., Sy, E. Y., Diesmos, M. L. L., Diesmos, A. C. (2020). Niche shifts and environmental non-equilibrium undermine the usefulness of ecological niche models for invasion risk assessments. Sci. Rep. 10, 7972. doi: 10.1038/s41598-020-64568-2
Qi, G. J., Gao, Y., Huang, D. C., Lü, L. H. (2012). Historical invasion, expansion process and the potential geographic distributions for the rice water weevil, Lissorhoptrus oryzophilus in China based on MAXENT. Acta Phytophylacica Sin. 39, 129–136. doi: 10.15244/pjoes/152169
Qin, Y. J., Lan, S., Zhao, Z. H., Sun, H. Y., Zhu, X. M., Yang, P. Y., et al. (2020). Potential geographical distribution of the fall armyworm (Spodoptera frugiperda) in China. Plant Prot. 45, 43–47. doi: 10.16688/j.zwbh.2019269
Raffini, F., Bertorelle, G., Biello, R., D’Urso, G., Russo, D., Bosso, L. (2020). From nucleotides to satellite imagery: approaches to identify and manage the invasive pathogen Xylella fastidiosa and its insect vectors in Europe. Sustainability 12, 4508. doi: 10.3390/su12114508
Ramasamy, M., Das, B., Ramesh, R. (2022). Predicting climate change impacts on potential worldwide distribution of fall armyworm based on CMIP6 projections. J. Pest. Sci. 95, 841–854. doi: 10.1007/s10340-021-01411-1
Rezaei, E. E., Webber, H., Asseng, S., Boote, K., Durand, J. L., Ewert, F., et al. (2023). Climate change impacts on crop yields. Nat. Rev. Earth. Environ. 4, 831–846. doi: 10.1038/s43017-023-00491-0
Saito, T., Hirai, K., Way, M. O. (2005). The rice water weevil, Lissorhoptrus oryzophilus Kuschel (Coleoptera: Curculionidae). Appl. Entomol. Zool. 40, 31–39. doi: 10.1303/aez.2005.31
Santana, P. A., Kumar, L., Silva, R. S. D., Pereira, J. L., Picanço, M. C. (2019). Assessing the impact of climate change on the worldwide distribution of Dalbulus maidis (DeLong) using MaxEnt. Pest. Manage. Sci. 75, 2706–2715. doi: 10.1002/ps.5379
Sexton, J. P., Montiel, J., Shay, J. E., Stephens, M. R., Slatyer, R. A. (2017). Evolution of ecological niche breadth. Annu. Rev. Ecol. Evol. Syst. 48, 183–206. doi: 10.1146/annurev-ecolsys-110316-023003
Stout, M. J., Rita Riggio, M., Zou, L., Roberts, R. (2002). Flooding influences ovipositional and feeding behavior of the rice water weevil (Coleoptera: Curculionidae). J. Econ. Entomol. 95, 715–721. doi: 10.1603/0022-0493-95.4.715
Sun, R., Mao, Z., Dai, D., Xiao, L., Chen, H., Wang, C. (1996). The Rice Water Weevil (Beijing: China Agric. Press).
Sun, H. Y., Qin, Y. J., Fang, Y., Zhao, Z. H., Pan, X. B., Zhao, S. Q., et al. (2018). The potential economic loss of bitter gourd industry in China caused by Zeugodacus cucurbitae (Coquillett) based on @RISK. Plant Quarantine 32, 64–69. doi: 10.19662/j.cnki.issn1005-2755.2018.06.016
Tilman, D., Balzer, C., Hill, J., Befort, B. L. (2011). Global food demand and the sustainable intensification of agriculture. PNAS 108, 20260–20264. doi: 10.1073/pnas.1116437108
Tindall, K. V., Bernhardt, J. L., Stout, M. J., Beighley, D. H. (2013). Effect of depth of flooding on the rice water weevil, Lissorhoptrus oryzophilus, and yield of rice. J. Insect. Sci. 13, 62. doi: 10.1673/031.013.6201
Uhm, K. B., Lee, Y. I., Kim, Y. H., Choi, K. M., Yon, K. S., Uhm, K. B., et al. (1989). Studies on the future dispersion of the rice water weevil Lissorhoptrus oryzophilus in korea. Res. Rep. Rural Dev. Administration 31, 23–28.
Ullah, F., Zhang, Y., Gul, H., Hafeez, M., Desneux, N., Qin, Y. (2023). Potential economic impact of Bactrocera dorsalis on Chinese citrus based on simulated geographical distribution with MaxEnt and CLIMEX models. Entomol. Gen. 43, 821–830. doi: 10.1127/entomologia/2023/1826
Vilà, M., Espinar, J. L., Hejda, M., Hulme, P. E., Jarošík, V., Maron, J. L., et al. (2011). Ecological impacts of invasive alien plants: a meta-analysis of their effects on species, communities and ecosystems. Ecol. Lett. 14, 702–708. doi: 10.1111/j.1461-0248.2011.01628.x
Wang, Z., Wu, J., Shang, H., Cheng, J. (2011). Landscape connectivity shapes the spread pattern of the rice water weevil: a case study from Zhejiang, China. Environ. Manage. 41, 254–262. doi: 10.1007/s00267-010-9595-y
Warren, D. L., Glor, R. E., Turelli, M. (2010). ENMTools: a toolbox for comparative studies of environmental niche models. Ecography 33, 607–611. doi: 10.1890/10-1171.1
Warren, D. L., Seifert, S. N. (2011). Ecological niche modeling in MaxEnt: the importance of model complexity and the performance of model selection criteria. Ecol. Appl. 21, 335–342. doi: 10.1890/10-1171.1
Wu, T., Lu, Y., Fang, Y., Xin, X., Li, L., Li, W., et al. (2019). The Beijing Climate Center Climate System Model (BCC-CSM): the main progress from CMIP5 to CMIP6. Geosci. Model. Dev. 12, 1573–1600. doi: 10.5194/gmd-12-1573-2019
Xie, W., Zhang, X., Wang, R., Zhang, Y., Mumtaz, M., Li, Q., et al. (2022). The Potential Distribution of Rice Water Weevil (Lissorhoptrus oryzophilus) in China under Current and Future Climatic Conditions. Pol. J. Environ. Stud. 31, 5915–5930. doi: 10.15244/pjoes/152169
Xu, Y. L., Li, Z. Y., Chen, J., Li., Z. H., Qin, Y. J. (2020). Assessment of potential economic loss of wheat industry caused by the fall armyworm Spodoptera frugiperda in China. J. Plant Prot. 47, 740–746. doi: 10.13802/j.cnki.zwbhxb.2020.2020810
Xu, L., Zhang, T., Wang, A., Yu, W., Yang, S. (2022). Variations of summer extreme and total precipitation over Southeast Asia and associated atmospheric and oceanic features. J. Climate. 35, 6395–6409. doi: 10.1175/JCLI-D-21-1020.1
Yang, Pu. (2008). Study on the Parthenogenetic Reproduction of Geographic Forms of Rice Water Weevil (Zhejiang University).
Yang, M., Zhao, H., Xian, X., Liu, H., Li, J., Chen, L., et al. (2022). Potential global geographical distribution of Lolium temulentum L. under climate change. Front. Plant Sci. 13. doi: 10.3389/fpls.2022.1024635
Zhou, S. W., Tan, X. P., Zhang, J. F., Zeng, Y. Q., Li, G. C., Du, Y. C. (2007). Influence of larva of rice water weevil on the development and output of rice. Plant Quarantine 6, 345–346. doi: 10.19662/j.cnki.issn1005-2755.2007.06.006
Keywords: invasive alien pest, Lissorhoptrus oryzophilus parthenogenetic populations, suitable area, ecological niche, potential economic loss
Citation: Li L, Jin Z, Li M, Xue Y, Guo J, Jia D, Ma R, Lü Z, Xian X and Liu W (2025) Assessing the establishment risk for parthenogenetic populations of Lissorhoptrus oryzophilus in global rice-growing areas and potential economic impact in China. Front. Plant Sci. 15:1506418. doi: 10.3389/fpls.2024.1506418
Received: 05 October 2024; Accepted: 16 December 2024;
Published: 22 January 2025.
Edited by:
Xiao Ming Zhang, Yunnan Agricultural University, ChinaReviewed by:
Prashant Sharma, Dr. Yashwant Singh Parmar University of Horticulture and Forestry, IndiaMichael J. Stout, Louisiana State University, United States
Daniela Lupi, University of Milan, Italy
Copyright © 2025 Li, Jin, Li, Xue, Guo, Jia, Ma, Lü, Xian and Liu. This is an open-access article distributed under the terms of the Creative Commons Attribution License (CC BY). The use, distribution or reproduction in other forums is permitted, provided the original author(s) and the copyright owner(s) are credited and that the original publication in this journal is cited, in accordance with accepted academic practice. No use, distribution or reproduction is permitted which does not comply with these terms.
*Correspondence: Wanxue Liu, bGl1d2FueHVlQGNhYXMuY24=; Xiaoqing Xian, eGlhbnhpYW9xaW5nQGNhYXMuY24=
†These authors share first authorship