- 1Institute of Plant Protection, Beijing Academy of Agriculture and Forestry Sciences, Beijing, China
- 2Beijing Key Laboratory of Environment Friendly Management on Fruit Diseases and Pests in North China, Beijing Academy of Agriculture and Forestry Sciences, Beijing, China
- 3College of Life Sciences, Yangtze University, Jingzhou, Hubei, China
- 4Department of Biology, Xinzhou Normal University, Xinzhou, Shanxi, China
Hydrangea macrophylla (Thunb.) Ser. is one of the widely cultivated plants in home gardens and scenic areas of China. Anthracnose disease is commonly observed during the normal growth of H. macrophylla, significantly impacting its ornamental and economic values. From 2021 to 2023, an investigation on H. macrophylla anthracnose was carried out in nine parks of Beijing, China, and a total of 114 Colletotrichum isolates were obtained from the diseased leaves with typical anthracnose symptoms. Based on morphological characteristics and phylogenetic analysis of six genomic loci including rDNA-ITS, ACT, TUB2, CAL, CHS-1, and GAPDH, these isolates were identified as belonging to six Colletotrichum species. Among which, C. gloeosporioides was the most abundant (65 isolates, 57.0%), followed by C. fructicola (33 isolates, 28.9%), C. aenigma (8 isolates, 7.0%), C. truncatum (4 isolates, 3.5%), C. subacidae (2 isolates, 1.8%) and C. sojae (2 isolates, 1.8%). Pathogenicity test conducted on detached leaves of H. macrophylla revealed a distinct variation in virulence among isolates from different Colletotrichum species, and wounding was either essential or conducive to successful infection. Specifically, C. gloeosporioides exhibited greater aggressiveness, resulting in larger lesions, while C. subacidae induced lesions most quickly. Fungicide sensitivity assays demonstrated that prochloraz exerted a remarkable inhibitory effect on the mycelial growth of representative isolates belonging to the three predominant Colletotrichum species. In contrast to difenoconazole and tebuconazole, the mean EC50 values for prochloraz against C. gloeosporioides, C. fructicola, and C. aenigma were 0.062, 0.033, and 0.023 μg/ml, respectively. This is the first report of C. aenigma, C. truncatum, C. subacidae and C. sojae causing H. macrophylla anthracnose worldwide including China. These findings have elucidated the Colletotrichum species associated with H. macrophylla anthracnose as well as their fungicides sensitivities in Beijing, China. This provides a scientific foundation for the accurate diagnosis and local management of H. macrophylla anthracnose.
1 Introduction
Hydrangea macrophylla (Thunb.) Ser., commonly known as an ornamental garden plant of the Hydrangea genus, enjoys great popularity due to its sizable inflorescence and abundant variety of flower colors (Mmbaga et al., 2012). The economic values have stimulated an increasing demand for cultivation and management of H. macrophylla, with particular emphasis on disease diagnosis and management (Wu et al., 2021). As one of the typical woody plants worldwide, H. macrophylla is susceptible to many diseases caused by a variety of fungal pathogens throughout the growth period, such as Alternaria alternata (Garibaldi et al., 2007; Liu et al., 2017), Cladosporium tenuissimum (Li et al., 2021), Colletotrichum spp. (Mmbaga et al., 2012), Corynespora cassiicola (Mmbaga et al., 2012), and Phoma exigua (Garibaldi et al., 2006). Among which, anthracnose is one of the most important fungal diseases on H. macrophylla. It usually affects the leaves and flowers, leading to seriously influence on the ecological landscape construction.
Plant anthracnose is commonly caused by species within the genus Colletotrichum, which is a large group of plant pathogenic fungi and is ranked eighth among the top ten fungal plant pathogens worldwide (Dean et al., 2012). Morphological characteristics comparison and multi-loci sequence analyses have been highly valued by mycologists for identifying Colletotrichum species. To date, a total number of about 280 species have been clarified (Dowling et al., 2020; Liu et al., 2022). However, the composition of Colletotrichum populations and the dominant pathogenic species responsible for anthracnose varied among different plants (Guarnaccia et al., 2021; Zhou et al., 2023). Currently, four Colletotrichum species, namely C. gloeosporioides (Mmbaga et al., 2012), C. fructicola (Guarnaccia et al., 2021), C. dematium (Maheswari et al., 2015), C. siamense (Hu et al., 2023) have been documented on H. macrophylla anthracnose worldwide. Among which, C. gloeosporioides has been identified as a pathogen causing H. macrophylla anthracnose in Qinhuangdao, Hebei province, China (Qi et al., 2008) and in Kaili, Guizhou province, China (Zhang et al., 2016). Meanwhile, C. siamense was reported as the causal agent of H. macrophylla anthracnose in Nanchang, Jiangxi province, China (Hu et al., 2023). A more comprehensive understanding of the dominant Colletotrichum species associated with H. macrophylla anthracnose will provide a foundation for developing novel strategies to manage the disease. However, the specific Colletotrichum taxa responsible for H. macrophylla anthracnose remained unknown in Beijing, China.
QoIs and DMIs are currently the major groups of fungicides employed for controlling anthracnose in agricultural crops (Kongtragoul et al., 2020; Wang et al., 2020). Certain Colletotrichum species have exhibited varying degrees of reduced sensitivity or even resistance to such fungicides (Shi et al., 2020). However, in actual production of H. macrophylla, unified management measures were frequently implemented but without significant efficacy. Moreover, the sensitivity of Colletotrichum species associated with H. macrophylla anthracnose to the commonly used fungicides were still uncertain. Thus, the aim of this study was thus (1) to identify the diversity of Colletotrichum species associated with H. macrophylla anthracnose by analyzing morphological characteristics coupled with multi-loci phylogenetic analysis, (2) to validate the pathogenicity and virulence of the identified Colletotrichum species by fulfilling Koch’s postulates, (3) to evaluate the sensitivity of the dominant Colletotrichum species to the commonly used fungicides, thereby providing a theoretical foundation for disease diagnosis and scientific management of anthracnose on H. macrophylla in Beijing, China.
2 Materials and methods
2.1 Field investigation and sample collection
From the summer of 2021 to 2023, investigations into the incidence and severity of anthracnose were carried out in H. macrophylla growing nurseries of nine parks in Beijing, China (Table 1). Disease incidence was calculated as the proportion of H. macrophylla plants exhibiting anthracnose symptoms relative to the total number of plants under evaluation. A total of 46 leaf samples of H. macrophylla exhibiting typical symptoms of anthracnose were collected for further study. Small pieces (5*5 mm) of leaf tissues were cut from the margin of anthracnose lesions, surface sterilized with 70% ethanol for 30 s, 1% NaClO for 30 s, then rinsed in sterile distilled water for three times, finally transferred onto potato dextrose agar (PDA) with lactic acid (0.1%) and incubated at 28°C for 3 days. Subsequently, the growing edges of the fungal colonies were aseptically transferred onto new PDA plates. The obtained fungal isolates were purified by single spore method and then reserved in 25% (v/v) glycerol at -80°C. The prevalence of Colletotrichum species was estimated as isolation rate (RI) and calculated using the formula RI% = (NS/NI) × 100%, Where NS is the number of isolates belonging to a specific species and NI is the total number of isolates (Vieira et al., 2014). All the 114 Colletotrichum isolates were deposited in the Laboratory of Biocontrol Microorganisms, Institute of Plant Protection, Beijing Academy of Agricultural and Forestry Sciences.
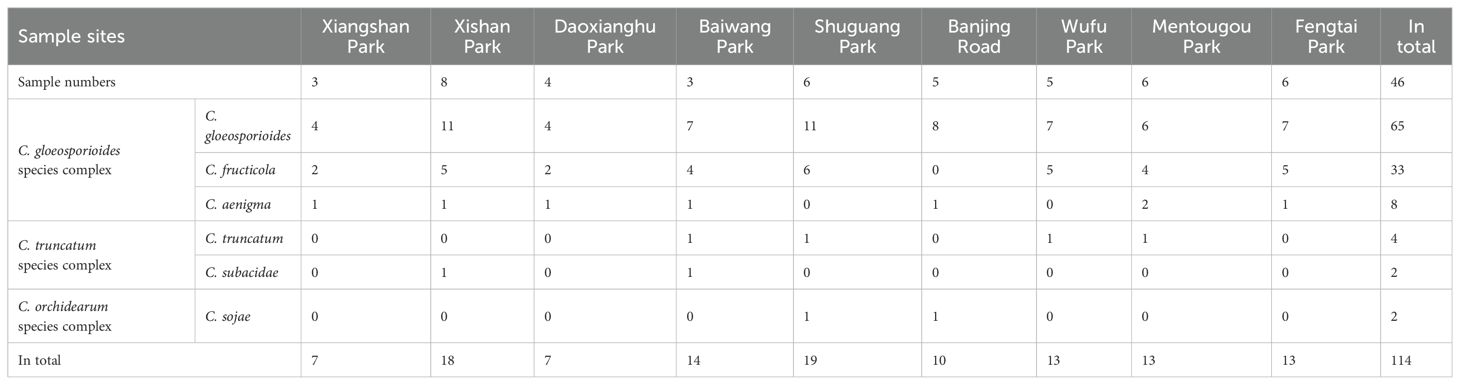
Table 1. Sample sites of Hydrangea macrophylla leaves with anthracnose symptoms and prevalence of the obtained Colletotrichum species.
2.2 DNA extraction, PCR amplification and phylogenetic analysis
Genomic DNA of the fungal isolates was extracted using the Solarbio® Fungi Genomic DNA Extraction Kit (Solarbio, China) according to the manufacturer’s instructions. The internal transcribed spacer region of ribosomal DNA (rDNA-ITS) was amplified using PCR primers ITS1 and ITS4 in order to screen the Colletotrichum sp. (White et al., 1990). For further precise identification, the representative Colletotrichum sp. were subjected to amplification of the partial sequences of actin (ACT) (Carbone and Kohn, 1999), beta tubulin (TUB2) (Glass and Donaldson, 1995; O’Donnell and Cigelnik, 1997), Calmodulin (CAL) (Weir et al., 2012), chitin synthase (CHS-1) (Carbone and Kohn, 1999), glyceraldehyde-3-phosphate dehydrogenase (GAPDH) (Templeton et al., 1992) genes with the corresponding primers listed in Supplementary Table S1. The amplifications were performed in a 25 μL mixture containing 10.5 μL ddH2O, 12.5 μL 2×PCR MasterMix, 1 μL DNA template, and 0.5 μL of each primer (10 μM) as described by Weir et al. (2012). DNA sequencing was performed by Beijing B&M Biotech Co., Ltd., China, using forward and reverse primers. Sequences were subjected to BLAST searches and submitted in the National Center for Biotechnology Information (NCBI) database with accession numbers of PP709475-PP709509 for rDNA-ITS, PP768443-768477 for ACT, PP768478-768512 for TUB2, PP768513-768547 for CAL, PP768548-768582 for CHS-1, PP768583-PP768617 for GAPDH, respectively (Table 2).
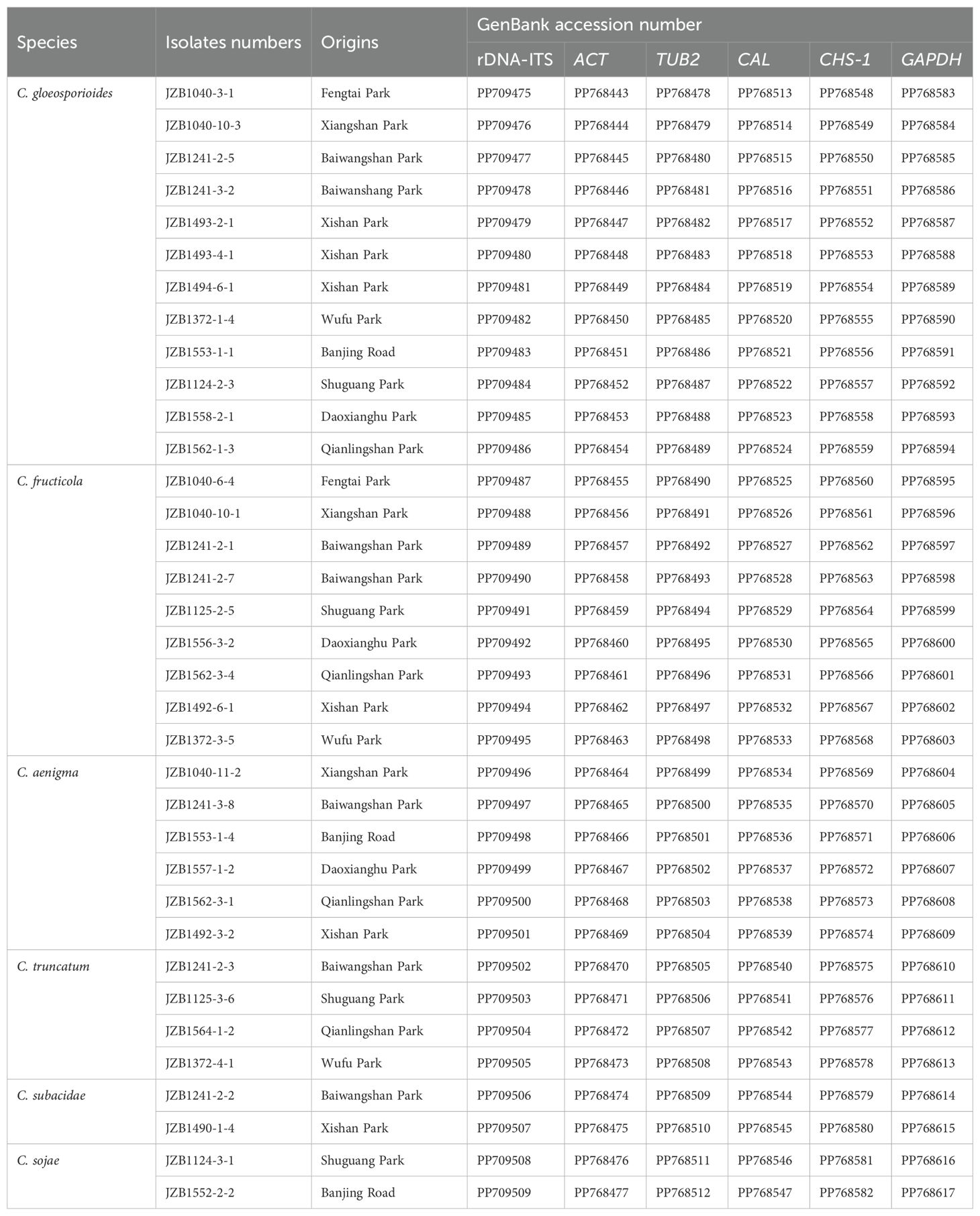
Table 2. Information of 35 representative Colletotrichum isolates from Hydrangea macrophylla anthracnose used for morphological characterization, phylogenetic analysis and pathogenicity test.
For phylogenetic analysis, additional reference sequences were selected based on related studies on Colletotrichum species (Weir et al., 2012; Guarnaccia et al., 2017) and retrieved from GenBank. Individual gene datasets of representative isolates were aligned using MAFFT v. 7 (https://mafft.cbrc.jp/alignment/server/) and adjusted manually with BioEdit v. 7.0.9.0 where necessary. The maximum parsimony analyses (MP) were performed based on the multi-loci alignment using PAUP v. 4.0b10. The analysis involved running 1000 replicates of a heuristic search, which utilized random sequence addition for initial tree construction followed by tree bisection reconnection branch swapping (Swofford, 2002). Bayesian inference analysis was conducted using MrBayes 3.1.2. The phylogenetic trees were visualized via TreeviewX v. 0.5.0.
2.3 Morphological characterization
For morphological characterization, mycelial discs from growing edge of the fungal cultures were transferred to fresh PDA plates and incubated at 28°C for 5 days (Cai et al., 2009). Appressoria was produced by dropping 50 μL conidial suspension (106 conidia/mL) on a concavity slide containing moistened filter papers with distilled sterile water, and then incubating at 28°C in the dark for 48 h (Weir et al., 2012). The shape, color and size of conidia (n=40) and appressoria (n=40) for each test Colletotrichum isolate were observed by light microscopy, and their dimensions were examined using an Axioscope 5 microscope (Carl Zeiss Microscopy, Germany). Mycelial growth rate of the representative Colletotrichum isolates was calculated by incubating the fresh mycelia blocks on new plates at 28°C with a photoperiod of 12 h/12 h for 5 days.
2.4 Pathogenicity test
Colletotrichum isolates representing different sampling sites or belonging to different Colletotrichum species were selected to conduct the pathogenicity test using mycelial plug method. Healthy leaves of H. macrophylla variety “Wujinxia” were collected, surface sterilized with 70% ethanol, washed three times with sterile distilled water, and then air dried on a sterilized tissue paper. Ten leaves per isolate with three replications were wounded by pin-pricking on both sides of the midrib with a sterilized needle, and 7-mm-diameter mycelia discs of 5-day-old cultures were inoculated, agar blocks without fungi were inoculated as control. Unwounded leaves were inoculated in the same way as described above. All the leaves were placed within a plastic box containing sterile water-soaked filter paper. The box was covered with plastic film and maintained in a growth chamber under condition of 85% relative humidity, a temperature of 28°C, and a 12/12 h light/dark photoperiod. Symptom development and lesion diameters on leaves were examined 7 days post inoculation. The fungus was re-isolated from lesions and recognized by integrated methods of morphological and molecular characteristics in order to fulfill Koch’s postulates.
2.5 Fungicide sensitivity of dominant Colletotrichum species
Representative isolates from the dominant Colletotrichum species, namely C. gloeosporioides, C. fructicola, and C. aenigma were selected and their sensitivities to three DMIs fungicides were tested using mycelial growth rate method (Zhang et al., 2020; Kim et al., 2020). The fungicides including prochloraz, difenoconazole, and tebuconazole were dissolved and adjusted to a concentration of 10 mg/ml as the stock solution. Each fungicide was prepared separately and the stock solutions were serially diluted as follows: prochloraz (0.005, 0.01, 0.02, 0.04, 0.08, 0.1 mg/mL), difenoconazole (0.005, 0.01, 0.05, 0.1, 0.5, 1, 5 mg/mL), tebuconazole (0.01, 0.05, 0.1, 0.5, 1, 5, 10 mg/mL), and then added to the sterilized PDA (approximately 50°C) at a ratio of 1:1000 (Supplementary Table S2). The margin of 5-day-old culture was used to produce 5-mm-diameter mycelial discs, which were then placed at the center of PDA plates with varying concentrations of fungicides. The diameter of each colony was measured in two perpendicular directions, after incubated at 28°C in the dark for 7 days. The percentage inhibition of mycelial growth for each Colletotrichum isolate at each test concentration (I) was also calculated as the difference between the radial growth of nonamended control (C) and the radial growth of each test concentration (T) as follows: I (%) = (C-T)/C×100. Each treatment was tested three times, with three plates for each replication. The EC50 values of the fungicides were calculated and displayed as the mean values derived from 12, 9, and 6 representative isolates of C. gloeosporioides, C. fructicola, and C. aenigma, respectively.
2.6 Statistical analysis
All the data were expressed as mean ± standard deviation of three replications unless otherwise mentioned. Significance of the differences (P < 0.05) was evaluated by one-way analysis of the variance (ANOVA) using the SPSS v21 software. The EC50 values were calculated by linear regression of the probit-transformed relative inhibition value on the log10- transformed fungicide concentration using the statistical algorithms.
3 Results
3.1 Disease survey and prevalence of Colletotrichum species
During the investigation of H. macrophylla foliar disease from 2021-2023, severe anthracnose symptoms were observed with disease incidence ranging from 23.3%-56.7% in nine parks across Beijing, China. The disease was first observed on newly emerged leaves of H. macrophylla, the infection quickly spread to the around plants in the late growing season. Typical symptoms were initially manifested as tiny purplish-red spots, approximately the size of pinheads with a yellow halo, which subsequently transitioned to light brown or grayish white with brown margins. As the symptoms advanced, these spots ultimately enlarged and merged, resulting in the formation of extensive necrotic regions (Figure 1). A total of 154 monosporic fungal isolates were recovered from symptomatic H. macrophylla leaves. In addition, certain species belonging to other genera like Pythium, Alternaria, and Fusarium were also detected during the isolation process but not shown in this study.
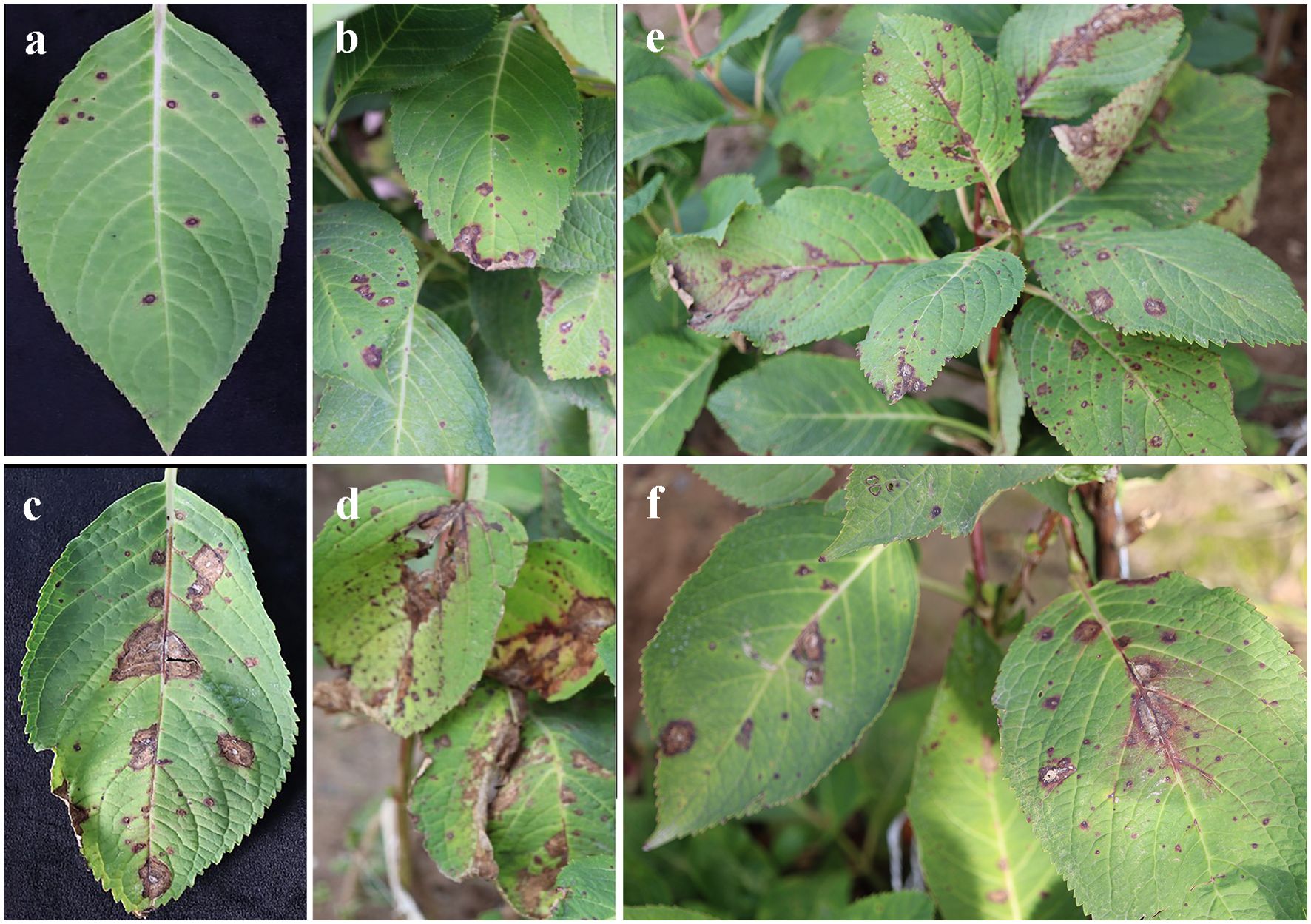
Figure 1. Typical symptoms of anthracnose on the leaves of Hydrangea macrophylla in Beijing, China. (A, B). Symptoms of small spots on the leaves; (C, D). Coalesce of brown necrotic lesions on the leaves; (E, F). Anthracnose symptoms on the whole plants.
Based on morphology and rDNA-ITS sequence data, the remained 114 isolates resembling Colletotrichum were primarily assigned to three groups, C. gloeosporioides species complex (106 isolates), C. truncatum species complex (6 isolates), C. orchidearum species complex (2 isolates). Further identification based on sequence analysis of six gene loci indicated that, C. gloeosporioides was the most prevalent species (65 isolates, 57.0%) associated with H. macrophylla anthracnose, followed by C. fructicola (33 isolates, 28.9%), C. aenigma (8 isolates, 7.0%), C. truncatum (4 isolates, 3.5%), C. subacidae and C. sojae (2 isolates, 1.8% each). Among which, C. gloeosporioides was the prevalent species in all the parks, C. subacidae was only found in Xishan and Baiwang parks, while C. sojae was only detected in Shuguang Park and Banjing Road (Table 1; Supplementary Figure S1).
3.2 Multi-loci phylogenetic analysis
A total of 35 representative Colletotrichum isolates from different sampling sites or belonging to different species were further subjected to multi-loci phylogenetic analysis with concatenated datasets of rDNA-ITS, ACT, TUB2, CAL, CHS-1 and GAPDH sequences (Figure 2; Table 2). Phylogenetic analysis showed that the present Colletotrichum isolates from H. macrophylla anthracnose clearly clustered into three clades with Monilochaetes infuscans CBS 869.96 included as the outgroup (Supplementary Table S3). Among which, twelve isolates within the C. gloeosporioides species complex grouped together to formed a clade with the ex-type isolate of C. gloeosporioides LF604, nine isolates clustered with ex-type isolate of C. fructicola LF130, while the remaining six isolates constituted a distinct clade along with the ex-type isolate of C. aenigma ICMP18608 and JFRL03-1005. For phylogenetic analysis of the C. truncatum species complex, four isolates were grouped together with the ex-type isolates of C. truncatum CBP002, while two isolates formed a clade in conjunction with C. subacidae NN054609. In addition, two Colletotrichum isolates from C. orchidearum complex were clustered with C. sojae ATCC62257.
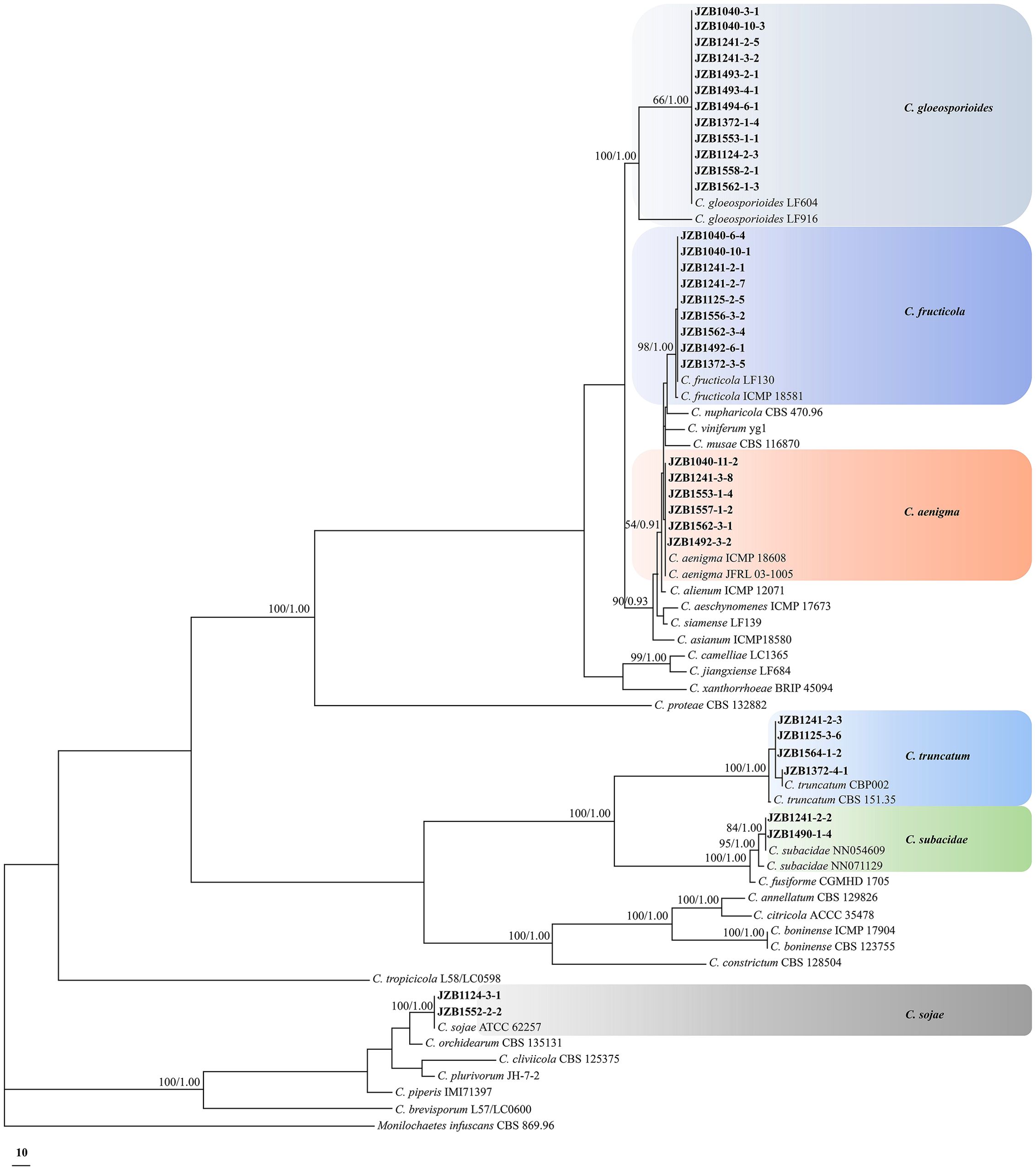
Figure 2. Phylogenetic tree based on the concatenated sequences of rDNA-ITS, ACT, TUB2, CAL, CHS-1, and GAPDH genomic regions of 35 representative Colletotrichum isolates obtained from Hydrangea macrophylla in Beijing, China. The species Monilochaetes infuscans CBS 869.96 was selected as an outgroup. Maximum likelihood boot strap support values (ML≥50) and Bayesian posterior probability values (PP≥0.90) were shown at the nodes, respectively. Colored blocks indicated clades containing isolates from different Colletotrichum species in this study.
3.3 Morphological characterization
Distinct morphological features including colony, conidia and appressoria of 35 representative Colletotrichum isolates (Table 2) were observed for each identified Colletotrichum species after 7 days incubation on PDA (Figure 3). Most isolates in C. gloeosporioides species complex developed greyish white to pale grey colonies, while the reverse sides of C. fructicola and C. aenigma were grayish green to olivaceous grey with white margin. The conidia were all cylindrical with obtuse to slightly rounded ends. Appressoria were pale brown to dark brown, subglobose or ellipsoid, and rarely irregular. The C. truncatum and C. orchidearum species complex were easily distinguishable from C. gloeosporioides species complex in terms of conidia or appressoria shape (Table 3). Conidia of C. truncatum was crescent-shaped, smooth-walled, and slightly curved with parallel walls. Conidia of C. subacidae was slightly curved, acute apex, the central part was almost straight with parallel walls. The conidia of C. sojae were cylindrical with obtuse to slightly rounded ends, and their appressoria were dark brown, oval or bullet-shaped. There was a considerable variation in mycelial growth rate among the representative isolates belonging to different Colletotrichum species (Table 3). The average mycelial growth rate of C. aenigma reached 12.6 ± 0.4 mm/d followed by C. gloeosporioides and C. fructicola, while the growth rate of C. sojae was only 7.1 ± 0.3 mm/d.
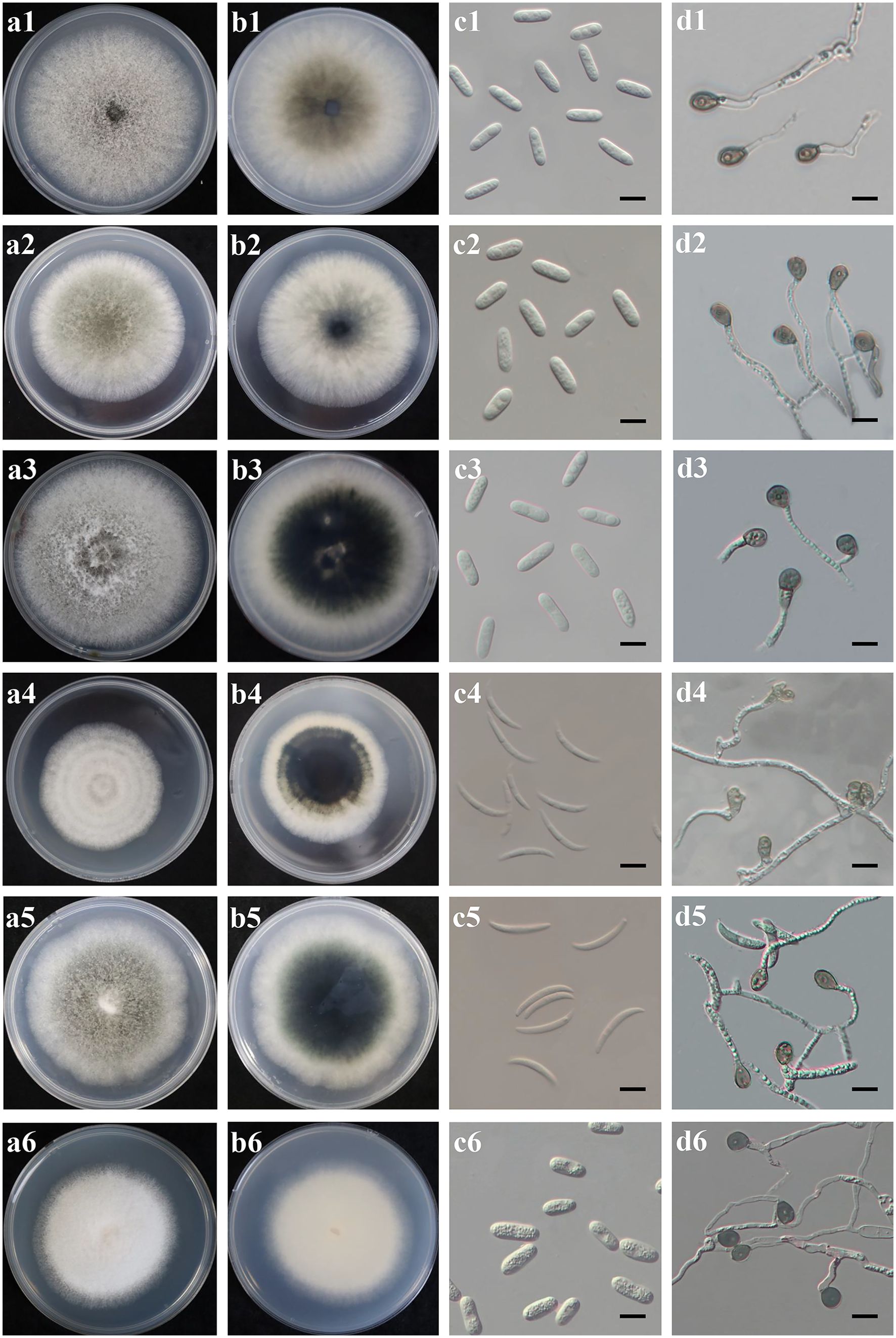
Figure 3. Culture characteristics and microscopic features of six Colletotrichum species obtained from Hydrangea macrophylla in Beijing, China. Each species was represented in four pictures (A-D). (A, B). Upper and reverse view of colony on potato dextrose agar at 28°C; (C). Conidia, scale bars = 20 µm; (D). Appressoria, scale bars = 20 µm. Plates 1-6 referred to C. gloeosporides JZB1040-3-1, C. fructicola JZB1241-2-7, C. aenigma JZB1040-11-2, C. truncatum JZB1564-1-2, C.subacidae JZB1490-1-4, and C. sojae JZB1124-3-1, respectively.
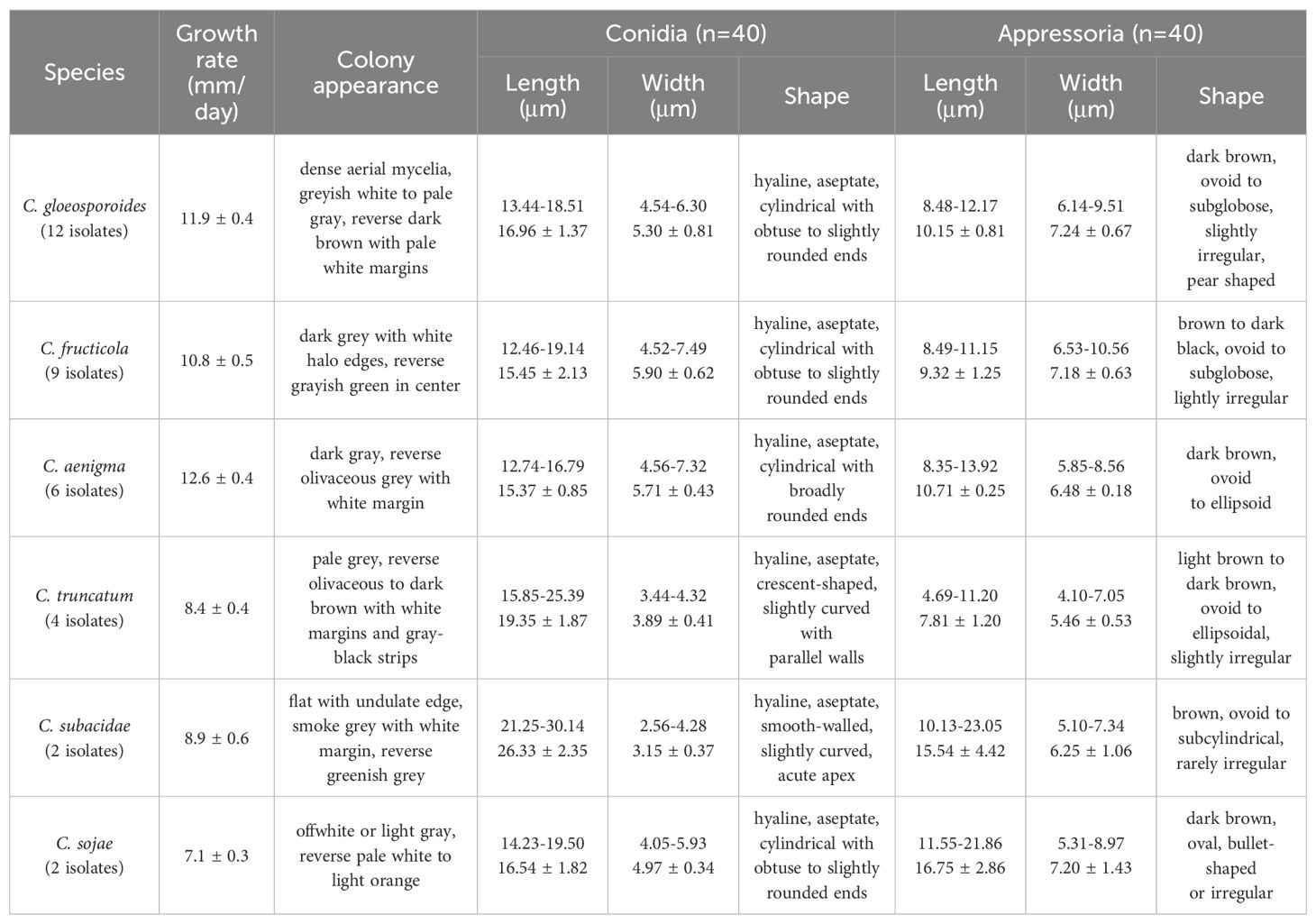
Table 3. Morphological data of six Colletotrichum species associated with Hydrangea macrophylla anthracnose in Beijing, China.
3.4 Pathogenicity test
Pathogenicity test demonstrated that the 35 representative Colletotrichum isolates from different sampling sites or belonging to different species (Table 2) exhibited varying degrees of aggression on H. macrophylla leaves. Seven days after inoculation, all the tested isolates caused symptoms on the wounded leaves. The symptoms mainly manifested as dark brown or brownish, irregular lesions with yellow halos around their peripher on the surface of leaves, consistent with the symptoms observed in field (Figure 4). No lesions were induced in the control leaves inoculated with sterile PDA discs. Notably, certain species such as C. gloeosporioides JZB1040-3-1, C. subacidae JZB1490-1-4 exhibited the highest level of aggressiveness among the tested isolates. In contrast, C. truncatum JZB1564-1-2 produced small necrotic lesions. The remaining isolates had an intermediate level of aggressiveness (Supplementary Figure S2). To fulfill Koch’s postulates, the Colletotrichum species were re-isolated from the lesions of inoculated leaves and identified based on integrated analysis of morphological characteristics and multi-loci sequencing data. The re-obtained isolates matched well with the original ones that were used for inoculation. Further analysis showed that lesions on the wounded leaves were much larger than those on the unwounded leaves, indicating that wound is a crucial prerequisite for the occurrence of H. macrophylla anthracnose.
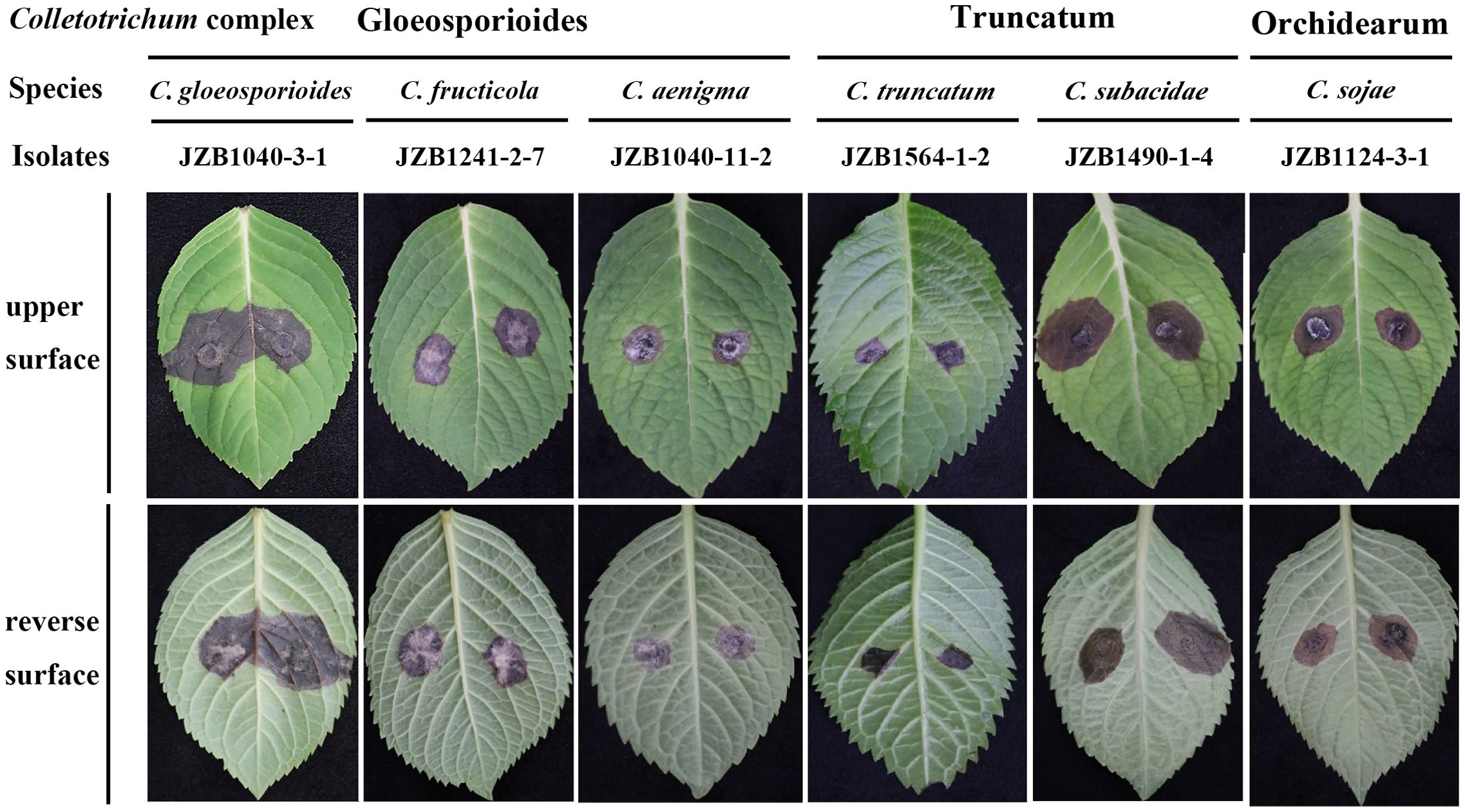
Figure 4. Symptoms of Hydrangea macrophylla leaves induced by inoculation of representative isolates from six Colletotrichum species under wounded conditions. The inoculation was conducted by dropping 5 mm mycelia disks of representative isolates on the detached leaves of H. macrophylla. Each leaf was wounded by pin-pricking with a sterilized needle. Control leaves were treated with sterilized agar blocks with the same size. The lesions on leaves were photographed 7 days post inoculation.
3.5 Fungicide sensitivity of dominant Colletotrichum species
A total of 27 representative isolates from the three dominant Colletotrichum species, namely C. gloeosporioides, C. fructicola, and C. aenigma (Supplementary Table S4), were chosen to determine their sensitivities to fungicides using the mycelial growth method (Supplementary Figure S3). Regarding the relative fungicide sensitivity of individual Colletotrichum species, we found that C. gloeosporioides, C. fructicola and C. aenigma exhibited greater sensitivity to prochloraz, since their mean EC50 values were only 0.062, 0.033, and 0.023 µg/ml. Colletotrichum fructicola exhibited significantly lower EC50 values against prochloraz than difenoconazole and tebuconazole. Among the three species, there were no significant differences in the EC50 values respect to difenoconazole and tebuconazole (Table 4). The fungicide sensitivities also varied among isolates within the same species. For prochloraz, the EC50 values of C. gloeosporioides spanned from 0.004 to 0.219 µg/ml, while those of C. fructicola ranged from 0.003 to 0.074 µg/ml and those of C. aenigma ranged from 0.007 to 0.064 µg/ml. Some isolates within C. gloeosporioides demonstrated an obviously reduced sensitivity to difenoconazole and tebuconazole, with EC50 values reaching 3.100 and 3.677 µg/ml, respectively (Supplementary Table S4).
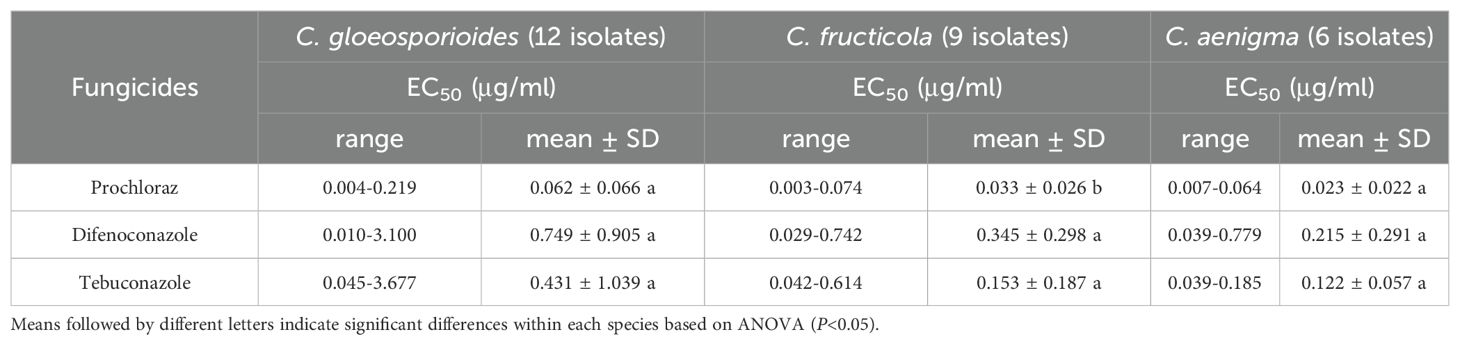
Table 4. Fungicides sensitivity of three dominant Colletotrichum species associated with Hydrangea macrophylla anthracnose in Beijing, China.
4 Discussion
Plant anthracnose can be induced by numerous Colletotrichum species. Extensive host range and wide geographic distribution of Colletotrichum species might be ascribed to their enhanced genetic diversity to adapt to various environmental conditions. Series studies have been conducted on the pathogen composition of Colletotrichum associated with plant anthracnose (Guarnaccia et al., 2017; Zhou et al., 2023). In this study, based on morphological observation and phylogenetic analysis, the Colletotrichum isolates associated with H. macrophylla anthracnose were identified as belonging to six species including C. gloeosporioides, C. fructicola, C. aenigae, C. truncatum, C. subacidae and C. sojae. This is the first report of the later four Colletotrichum species causing H. macrophylla anthracnose worldwide including China.
Traditionally, the species delimitation in Colletotrichum was mainly based on host range and morphological characteristic, such as the shape, color, dimension of colonies, conidia, and appressoria (Cai et al., 2009). In recent years, the number of new Colletotrichum species has increased dramatically with the development of molecular technologies, as the formerly recognized species have been dissected into species complexes, each of which encompasses numerous phylogenetically distinct species. For example, based on the combined sequence data of six gene loci, all the Colletotrichum isolates associated with strawberry anthracnose were grouped into three clades, namely C. siamense, C. fructicola, and C. aenigma, which were formerly part of C. gloeosporioides species complex (Zhang et al., 2020). Among the multiple genomic regions, TUB2 could discriminate all species within the C. orchidearum complex. CHS-1 can assist in distinguishing and corroborating recently diverged species, and thus serves as an informative marker for Colletotrichum species complexes (Vieira et al., 2020). In this study, phylogenetic analysis derived from the molecular data of ITS, ACT, TUB2, CAL, CHS-1, and GAPDH gene sequences attributed all the Colletotrichum isolates into six species, which was in full accordance with the results of the morphological groupings.
Colletotrichum gloeosporioide, C. fructicola, and C. aenigma within the C. gloeosporioides species complex are globally distributed and possess a wide variety of host species (Zhou et al., 2023). Besides, C. truncatum has also been cited as a pathogen of many economically important plants worldwide, such as papaya (Aktaruzzaman et al., 2018), watermelon (Guo et al., 2022). Colletotrichum subacidae were obtained from the diseased stem of Asparagus officinalison and the leaf petiole of Ailanthus altissima in China (Liu et al., 2022). Colletotrichum sojae was described as causal agent of anthracnose on pepper (Zhang et al., 2022) and American Ginseng (Guan et al., 2021) in China. This study demonstrated that all the six Colletotrichum species were capable of infecting leaves of H. macrophylla with C. gloeosporioides being the most prevalent species. Previous results indicated that wounding can break the quiescent infection and enhance the infectivity of Colletotrichum species, thereby resulting in a more rapid progression in wounded leaves (Jiang et al., 2014). Many Colletotrichum isolates cause obvious lesions on leaves under wounded conditions, but not under unwounded conditions (Fu et al., 2019). In this study, pathogenicity test of Colletotrichum species were conducted under both wounded and unwounded conditions, it was found that wound is the essential condition for the occurrence of H. macrophylla anthracnose. Therefore, wounds when transplanting or pruning should be avoided in actual production so as to prevent pathogen infection and disease transmission.
Prochloraz, difenoconazole, and tebuconazole are the DMI fungicides that have been employed in the management of anthracnose in China (Zhang et al., 2017). In this study, three DMI type fungicides were used to assess their inhibitory activity against the dominant Colletotrichum species. Three species C. glosporioides, C. fructicola, and C. aenigma differed in sensitivity to certain fungicides with no variation. Among which, the effect of prochloraz was superior to that of difenoconazole and tebuconazole. Colletotrichum glosporioides exhibited reduced sensitivity to difenoconazole and tebuconazole compared with the isolates from the other two species, with three being species all from the C. gloeosporioides complex. DMI fungicides are classified as moderately risk in terms of the development of fungicide resistance, moreover, DMI-resistant strains have been detected on numerous crops (Shi et al., 2020). Reduced sensitivity or even resistance to tebuconazole have been reported on C. gloeosporioides, the causal agent of anthracnose on walnut (Wang et al., 2020) and chili (Wei et al., 2020). Therefore, attention should be paid to the sensitivity of the dominant Colletotrichum species of H. macrophylla to such fungicides, to prevent the emergence of resistance. In addition, the combined or alternative application of fungicides with different action modes should be adopted to reduce the risk of resistance (Wang et al., 2020).
Better understanding of species distribution and individual characteristics of the Colletotrichum species associated with plant anthracnose is critical for development of disease management plans. This study represents the first comprehensive investigation of Colletotrichum species occurring on H. macrophylla anthracnose in Beijing, China. The knowledge acquired and the diversity of Colletotrichum species in H. macrophylla gathered provides a useful clue for resistant-germplasm selection and disease management. Future work may focus on the pathogenic mechanism of the dominant Colletotrichum species responsible for H. macrophylla anthracnose, as well as fungicide resistance risk assessment or resistance gene identification.
5 Conclusions
Here we provided the first detailed investigation of Colletotrichum species associated with anthracnose of Hydrangea macrophylla in Beijing, China. A total number of 114 Colletotrichum isolates belonging to six Colletotrichum species were characterized, and three species in C. gloeosporioides species complex were confirmed as the dominant species. We also demonstrated, for the first time, that C. aenigae, C. truncatum, C. subacidae and C. sojae were responsible for H. macrophylla in Beijing, China. Our results disclosed that these Colletotrichum taxa were pathogenic to H. macrophylla with varied aggressiveness, wound was the crucial condition for pathogen infection. Additionally, fungicide sensitivity showed that the inhibition effect of prochloraz were superior to that of difenoconazole and tebuconazole. Overall, this study provides crucial information for the management of H. macrophylla anthracnose in Beijing, China.
Data availability statement
The datasets presented in this study can be found in online repositories. The names of the repository/repositories and accession number(s) can be found in the article/Supplementary Material.
Author contributions
JZ: Data curation, Funding acquisition, Writing – original draft, Writing – review & editing. YC: Data curation, Investigation, Methodology, Writing – review & editing. YL: Methodology, Writing – review & editing. XS: Data curation, Writing – review & editing. TZ: Investigation, Methodology, Writing – review & editing. WQ: Conceptualization, Funding acquisition, Project administration, Writing – review & editing.
Funding
The author(s) declare financial support was received for the research, authorship, and/or publication of this article. The study was funded by the youth foundation of Beijing Academy of Agriculture and Forestry Sciences (QNJJ202313), the Innovation Capacity Foundation of Beijing Academy of Agriculture and Forestry Sciences, China (KJCX20230115) and Beijing Science and Technology Plan Project (Z231100003723001).
Conflict of interest
The authors declare that the research was conducted in the absence of any commercial or financial relationships that could be construed as a potential conflict of interest.
Generative AI statement
The author(s) declare that no Generative AI was used in the creation of this manuscript.
Publisher’s note
All claims expressed in this article are solely those of the authors and do not necessarily represent those of their affiliated organizations, or those of the publisher, the editors and the reviewers. Any product that may be evaluated in this article, or claim that may be made by its manufacturer, is not guaranteed or endorsed by the publisher.
Supplementary material
The Supplementary Material for this article can be found online at: https://www.frontiersin.org/articles/10.3389/fpls.2024.1504135/full#supplementary-material
References
Aktaruzzaman, M., Afroz, T., Lee, Y. G., Kim, B. S. (2018). Post-harvest anthracnose of papaya caused by Colletotrichum truncatum in Korea. Eur. J. Plant Pathol. 150, 259–265. doi: 10.1007/s10658-017-1265-y
Cai, L., Hyde, K. D., Taylor, P., Weir, B. S., Waller, J. M., Abang, M. M., et al. (2009). A polyphasic approach for studying Colletotrichum. Fungal Divers. 39, 183–204.
Carbone, I., Kohn, L. M. (1999). A method for designing primer sets for speciation studies in filamentous ascomycetes. Mycologia 91, 553–556. doi: 10.1080/00275514.1999.12061051
Dean, R., Van Kan, J. A. L., Pretorius, Z. A., Hammond-Kosack, K. E., Di Pietro, A., Spanu, P. D., et al. (2012). The Top 10 fungal pathogens in molecular plant pathology. Mol. Plant Pathol. 13, 414–430. doi: 10.1111/j.1364-3703.2011.00783.x
Dowling, M., Peres, N., Villani, S., Schnabel, G. (2020). Managing Colletotrichum on fruit crops: a “complex challenge. Fungal Divers. 104, 2301–2316. doi: 10.1094/PDIS-11-19-2378-FE
Fu, M., Crous, P. W., Bai, Q., Zhang, P. F., Xiang, J., Guo, Y. S., et al. (2019). Colletotrichum species associated with anthracnose of Pyrus spp. in China. Persoonia: Mol. Phylogeny Evol. Fungi 42, 1–35. doi: 10.3767/persoonia.2019.42.01
Garibaldi, A., Gilardi, G., Frati, S., Gullino, M. L. (2007). First report of leaf spot caused by Alternaria alternata on Hydrangea macrophylla in Italy. Plant Dis. 91, 767. doi: 10.1094/PDIS-91-6-0767A
Garibaldi, A., Gilardi, G., Minerdi, D., Gullino, M. L. (2006). First report of leaf spot caused by Phoma exigua on Hydrangea macrophylla in Italy. Plant Dis. 90, 1113. doi: 10.1094/PD-90-1113B
Glass, N. L., Donaldson, G. C. (1995). Development of primer sets designed for use with the PCR to amplify conserved genes from filamentous ascomycetes. Appl. Environ. Microb. 61, 1323–1330. doi: 10.1128/aem.61.4.1323-1330.1995
Guan, Y. M., Zhang, L. L., Ma, Y. Y., Zhang, Y., Zhang, S. N. (2021). First report of anthracnose of American ginseng caused by Colletotrichum sojae in northeast China. Plant Dis. 105, 3755. doi: 10.1094/PDIS-11-20-2440-PDN
Guarnaccia, V., Groenewald, J. Z., Polizzi, G., Crous, P. W. (2017). High species diversity in Colletotrichum associated with citrus diseases in Europe. Persoonia 39, 32–50. doi: 10.3767/persoonia.2017.39.02
Guarnaccia, V., Martino, I., Gilardi, G., Garibaldi, A., Gullino, M. L. (2021). Colletotrichum spp. causing anthracnose on ornamental plants in northern Italy. J. Plant Pathol. 103, 127–137. doi: 10.1007/s42161-020-00684-2
Guo, Z., Luo, C. X., Wu, H. J., Peng, B., Kang, B. S., Liu, L. M., et al. (2022). Colletotrichum species associated with anthracnose disease of watermelon (Citrullus lanatus) in China. J. Fungi 8, 790. doi: 10.3390/jof8080790
Hu, Y. F., Luo, X. X., Xu, Z. H., Wang, Y. Q., Cui, R. Q., Zhang, L. H., et al. (2023). First report of anthracnose caused by Colletotrichum siamense on Hydrangea macrophylla in China. Plant Dis. 107, 2853. doi: 10.1094/PDIS-02-23-0328-PDN
Jiang, J. J., Zhai, H. Y., Li, H. N., Wang, Z. H., Chen, Y. S., Hong, N., et al. (2014). Identification and characterization of Colletotrichum fructicola causing black spots on young fruits related to bitter rot of pear (Pyrus bretschneideri Rehd.) in China. Crop Prot. 58, 41–48. doi: 10.1016/j.cropro.2014.01.003
Kim, C. H., Hassan, O., Chang, T. (2020). Diversity, pathogenicity, and fungicide sensitivity of Colletotrichum species associated with apple anthracnose in South Korea. Plant Dis. 104, 2866–2874. doi: 10.1094/PDIS-01-20-0050-RE
Kongtragoul, P., Imamoto, K., Ishii, H. (2020). Resistance to quinone-outside inhibitor (QoI) fungicides in Colletotrichum species isolated from anthracnose disease occurring in Thailand. Curr. Appl. Sci. Technol. 20, 79–89. doi: 10.14456/cast.1477.8
Li, C. X., Cao, P., Du, C. J., Xu, X., Xiang, W. S., Wang, X. J., et al. (2021). First report of leaf spot caused by Cladosporium tenuissimum on Panicle hydrangea (Hydrangea paniculate) in China. Plant Dis. 105, 33822661. doi: 10.1094/PDIS-12-20-2640-PDN
Liu, F., Ma, Z. Y., Hou, L. W., Diao, Y. Z., Wu, W. P., Damm, U., et al. (2022). Updating species diversity of Colletotrichum, with a phylogenomic overview. Stud. Mycol. 101, 1–56. doi: 10.3114/sim.2022.101.01
Liu, Z. H., Song, B., Fu, Y. P., Li, Y. (2017). First report of Alternaria alternata causing brown leaf spot disease on Panicle hydrangea (Hydrangea paniculata) in China. Plant Dis. 101, 2147–2148. doi: 10.1094/PDIS-03-17-0350-PDN
Maheswari, C. U., Sharma, R. K., Prabakaran, N., Nallathambi, P. (2015). New host record of Colletotrichum dematium on Hydrangea macrophylla. Ann. Plant Prot. Sci. 23, 183–185.
Mmbaga, M. T., Kim, M. S., Mackasmie, L., Li, Y. H. (2012). Evaluation of Hydrangea macrophylla for resistance to leaf-spot diseases. J. Phytopathol. 160, 88–97. doi: 10.1111/j.1439-0434.2011.01862.x
O’Donnell, K., Cigelnik, E. (1997). Two divergent intragenomic rDNA ITS2 types within a monophyletic lineage of the fungus Fusarium are nonorthologous. Mol. Phylogenet. Evol. 7, 103–116. doi: 10.1006/mpev.1996.0376
Qi, H. X., Li, S. M., Yu, J. Y., Liu, R., Yang, W. L. (2008). Pathogen identification and biological characteristics of anthracnose in Hydrangea macrophylla Ser. Nonwood For. Res. 26, 84–87. (in Chinese).
Shi, N. N., Ruan, H. C., Gan, L., Dai, Y. L., Yang, X., Du, Y., et al. (2020). Evaluating the sensitivities and efficacies of fungicides with different modes of action against Phomopsis asparagi. Plant Dis. 104, 448–454. doi: 10.1094/PDIS-05-19-1040-RE
Swofford, D. L. (2002). PAUP*: Phylogenetic analysis using parsimony (*and other methods), version 4.0b10. 4 ed (Inc., Publishers, Sunderland: Sinauer Associates).
Templeton, M. D., Rikkerink, E., Solon, S. L., Crowhurst, R. N. (1992). Cloning and molecular characterization of the glyceraldehyde-3-phosphate dehydrogenase-encoding gene and cDNA from the plant pathogenic fungus Glomerella cingulata. Gene 122, 225–230. doi: 10.1016/0378-1119(92)90055-T
Vieira, W. A. S., Bezerra, P. A., Silva, A. C., Veloso, J. S., Câmara, M. P.S., Doyle, V. P. (2020). Optimal markers for the identification of Colletotrichum species. Mol. Phylogenet. Evol. 143, 106694. doi: 10.1016/j.ympev.2019.106694
Vieira, W. A. S., Michereff, S. J., Morais, M. A., Hyde, K. D., Camara, M. P. S. (2014). Endophytic species of Colletotrichum associated with mango in northeastern Brazil. Fungal Divers. 67, 181–202. doi: 10.1007/s13225-014-0293-6
Wang, Q. H., Fan, K., Li, D. W., Han, C. M., Qu, Y. Y., Qi, Y. K., et al. (2020). Identification, virulence and fungicide sensitivity of Colletotrichum gloeosporioides s. s. responsible for walnut anthracnose disease in China. Plant Dis. 104, 1358–1368. doi: 10.1094/PDIS-12-19-2569-RE
Wei, L. L., Chen, W. C., Zhao, W. C., Wang, J., Wang, B. R., Li, F. J., et al. (2020). Mutations and overexpression of CYP51 associated with DMI-resistance in Colletotrichum gloeosporioides from chili. Plant Dis. 104, 668–676. doi: 10.1094/PDIS-08-19-1628-RE
Weir, B., Johnston, P. R., Damm, U. (2012). The Colletotrichum gloeosporioides species complex. Stud. Mycol. 73, 115–180. doi: 10.3114/sim0011
White, T. J., Bruns, T., Lee, S., Taylor, J. (1990). "Amplification and direct sequencing of fungal ribosomal RNA genes for phylogenetics." in PCR protocols, A guide to methods and applications. ,eds. Innis, M. A., Gelfand, D. H., Sninsky, J. J., White, T. J. (New York: Academic Press), 315–322.
Wu, X. B., Hulse-Kemp, A. M., Wadl, P. A., Smith, Z., Mockaitis, K., Staton, M. E., et al. (2021). Genomic resource development for Hydrangea (Hydrangea macrophylla (Thunb.) Ser.) - A transcriptome assembly and a high-density genetic linkage map. Horticulturae 7, 25. doi: 10.3390/horticulturae7020025
Zhang, C., Diao, Y., Wang, W., Hao, J., Imran, M., Duan, H. X., et al. (2017). Assessing the risk for resistance and elucidating the genetics of Colletotrichum truncatum that is only sensitive to some DMI fungicides. Front. Microbiol. 8. doi: 10.3389/fmicb.2017.01779
Zhang, G. H., Gu, H. X., Si, S. L. (2016). Investigation and identification of plant diseases and insect pests in Kaili base of the national forest genetic resource platform. For. Pest Dis. 35, 6–8. (in Chinese).
Zhang, L. Q., Song, L. L., Xu, X. M., Zou, X. H., Duan, K., Gao, Q. H. (2020). Characterization and fungicide sensitivity of Colletotrichum species causing strawberry anthracnose in eastern China. Plant Dis. 104, 1960–1968. doi: 10.1094/PDIS-10-19-2241-RE
Zhang, N., Yuan, S. L., Zhu, Y. N., Wang, B. Y., Xu, Y. Y., Yang, W. X. (2022). First report of anthracnose on chili pepper caused by Colletotrichum sojae in Hebei province, China. Plant Dis. 107, 1633. doi: 10.1094/PDIS-03-22-0703-PDN
Keywords: Hydrangea macrophylla, anthracnose, Colletotrichum species, multi-loci phylogeny, fungicide sensitivity
Citation: Zhao J, Cheng Y, Liu Y, Shi X, Zhang T and Qin W (2025) Characterization and fungicides sensitivity of Colletotrichum species causing Hydrangea macrophylla anthracnose in Beijing, China. Front. Plant Sci. 15:1504135. doi: 10.3389/fpls.2024.1504135
Received: 30 September 2024; Accepted: 27 December 2024;
Published: 21 January 2025.
Edited by:
Abhay K. Pandey, North Bengal Regional R & D Center, IndiaReviewed by:
Julia Christine Meitz-Hopkins, Stellenbosch University, South AfricaZhengnan Li, Inner Mongolia Agricultural University, China
Copyright © 2025 Zhao, Cheng, Liu, Shi, Zhang and Qin. This is an open-access article distributed under the terms of the Creative Commons Attribution License (CC BY). The use, distribution or reproduction in other forums is permitted, provided the original author(s) and the copyright owner(s) are credited and that the original publication in this journal is cited, in accordance with accepted academic practice. No use, distribution or reproduction is permitted which does not comply with these terms.
*Correspondence: Wentao Qin, cWlud2VudGFvQGJhYWZzLm5ldC5jbg==