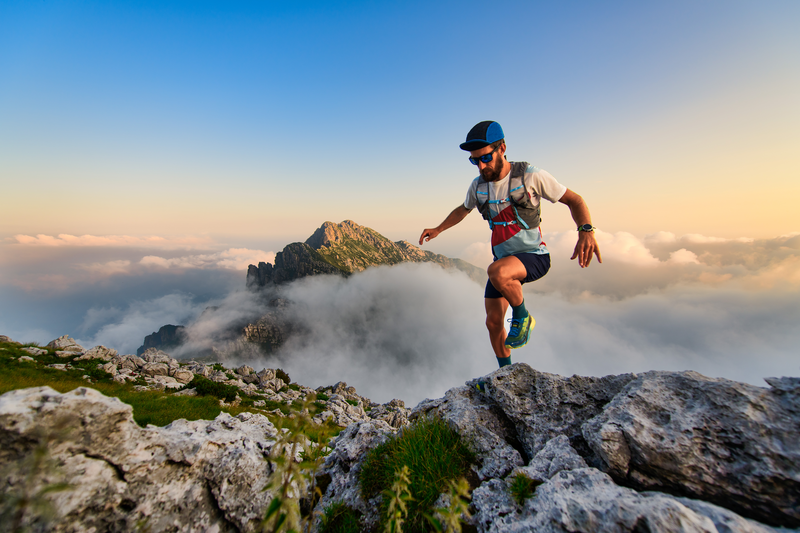
95% of researchers rate our articles as excellent or good
Learn more about the work of our research integrity team to safeguard the quality of each article we publish.
Find out more
ORIGINAL RESEARCH article
Front. Plant Sci. , 28 January 2025
Sec. Plant Breeding
Volume 15 - 2024 | https://doi.org/10.3389/fpls.2024.1501661
Powdery mildew (PM) caused by Erysiphie pisi Syd. is the most devastating disease of pea, affecting fresh pea production as well as the quality of the marketable harvest worldwide. The efforts were made to develop PM-resistant mutants of popular pea varieties “Lincoln” and “Azad P-1” through induced mutations by following gamma irradiation (300, 400, 500, and 600 Gy) and chemical mutagenesis, i.e., ethyl methane sulfonate (EMS) (0.3% and 0.4%). The screening of 13,868 M2 progenies at Kukumseri (summer season) followed by M3 generation at Palampur (winter season) resulted in the isolation of six putative PM-resistant mutants. The rigorous evaluation of these progenies under in vivo (field screening) and in vitro (artificial screening under greenhouse conditions and using the detached leaf assay method) conditions over the years resulted in the isolation of three PM-resistant mutants, viz., L-40-1014, L-0.3-139, and AP-0.3-129. SSR markers “PSMPSAD60 d” and “PSMPA5 c” linked to the er-1 gene indicated the presence of the “er1” gene in the mutant L-0.3-139 while the er-2 gene-linked SCAR marker “ScX171400” and SSR marker “AD141” indicated the probability of the “er-2” gene in mutant L-40-1014. The known markers linked to PM resistance genes could not be validated in the mutant AP-0.3-129, suggested to identify new markers linked to PM resistance. These PM-resistant mutants can be promising candidates as the new source of resistance for future pea breeding programs.
Garden pea (Pisum sativum L.) is an important leguminous crop worldwide belonging to the family Fabaceae and is cultivated for its fresh-shelled green seeds. The use of green shelled seeds in canned, frozen, or dehydrated products signifies its coveted position in the processing industry (Sharma et al., 2022). It is a rich source of nutrients such as proteins, vitamins, minerals, and lysine (an essential amino acid lacking in cereals), and its consumption, therefore, help to maintain human health (Sharma et al., 2020). In addition, fresh pea pods are exceptionally good sources of folic acid, vitamin C, vitamin K, and β-sitosterol (Rana et al., 2021). Its nutritional advantages are furthermore supported by its antidiabetic, antibacterial, antifungal, anti-inflammatory, anti-hypercholesterolemic, and antioxidant properties (Rungruangmaitree and Jiraungkoorskul, 2017). Apart from this, pea makes a substantial contribution in sustainable agriculture by improving soil health on account of its ability to fix atmospheric nitrogen and fits well in different crop rotation/cropping sequences, being a short duration crop.
Garden pea is grown commercially during winter season while the northwestern Himalayan region of India provides conditions for year-round cultivation as an off-season crop, i.e., in low and mid hills during winter season, and in high hills during summer season. India is the second-largest producer of fresh peas worldwide after China. Globally, pea occupies an area of 2.59 million ha with a production of 20.53 million tonnes (Anonymous, 2021). India occupies an area of 608.96 thousand ha with a production of 6631.29 thousand metric tonnes and average productivity of 10.89 metric tonnes ha−1 (Anonymous, 2024).
The major objectives for garden pea improvement are the development of high yielding varieties with desirable pod characteristics, i.e., long and dark green pods containing 8–10 seeds, sweet, and have high shelling besides carrying resistance to pests and diseases (Rana et al., 2021). Among the pea diseases, powdery mildew (PM; Erysiphie pisi Syd.) is the most devastating, causing very heavy yield losses to the extent of 25%–50% and thereby hampers the productivity and quality of produce (Fondevilla and Rubiales, 2012; Rana et al., 2023). The yield losses and green pod quality degradation are more conspicuous in the varieties of garden pea. In India, consumers prefer sweet, long, and dark green pods of garden pea that put Azad P-1 as the most preferred choice among different varieties, though it is highly susceptible to PM disease (Sharma et al., 2013). Inversely, PM-resistant varieties are generally carrying medium-sized pods with light-green color. It is, therefore, imperative to develop PM resistance varieties keeping in view the consumers’ preference for pod attributes without compromising yield. Genetic control is the most effective approach that is eco-friendly, cost-effective, and healthy that keeps green pods free from fungicide residues (Sharma et al., 2010).
The PM-resistant pea varieties can be developed through hybridization by involving the available resistance sources in the germplasm, which broadly indicated monogenic recessive inheritance (Sharma, 2003). The recessive “er1” gene imparts complete resistance against PM by restricting its penetration in the host and, therefore, “Mexique 4” and “S143” possessing “er1” gene were used extensively in a pea breeding program (Janila and Sharma, 2004; Ek et al., 2005). Another recessive gene, “er2”, was identified in “JI 2480”, which imparts resistance through post-penetration cell death (Fondevilla et al., 2006) and was also involved in PM resistance breeding. Apart from recessive genes, the dominant “Er3” gene was identified in Pisum fulvum, which is not temperature-dependent for its expression like “er2” (Fondevilla et al., 2007a; Bobkov and Selikhova, 2021). All the three resistance sources have been identified in pulse-type pea, and hence, their use as a donor for PM resistance is associated with traits like tall growth habit, and small and yellowish green pods with a high starch content that are undesirable in garden pea. Therefore, the pea varieties that are developed by involving the existing PM resistance sources lack consumers’ acceptance due to the light green/yellowish green and medium-sized pods. The regular use of these resistance sources to breed PM-resistant varieties also resulted in the narrow genetic base of modern cultivars along with compromised pod quality (Pandey et al., 2022).
The mutation breeding to some extent may help to resolve these issues besides help to identify new sources of resistance by rectifying a single undesirable trait while keeping all the other desirable traits intact as it does not involve hybridization (Bhat et al., 2001). The development of the “mlo” gene in 1942, conferring resistance to all forms of PM in barley, is a significant illustration of induced mutation (Dreiseitl, 2024). The application of induced mutation is the most appropriate in garden pea to create genetic variability because of its low chromosomal count (2n = 14) and short life cycle (Kalapchieva and Tomlekova, 2016). Pereira and Leitao (2010) identified and isolated two PM resistance mutants in pea. The natural loss of function of the PM-susceptible gene “PsMLO1” was identified as “er1” resistance gene that encouraged the mutation breeding for PM resistance in pea (Humphry et al., 2011; Pavan et al., 2011). Therefore, the present study was carried out for the development of PM-resistant mutants in popular pea varieties, viz., “Lincoln” and “Azad P-1” using physical (gamma irradiation) and chemical [ethyl methane sulfonate (EMS)] mutagenesis followed by their evaluation and screening under in vivo and in vitro conditions along with validation for PM-resistant genes “er1” and “er2” using known markers linked to PM resistance genes.
Experimental material consisted of genetically pure seed of two popular varieties of garden pea, viz., Azad P-1 and Lincoln. Both the varieties are high yielding vis-a-vis possessing green and medium-sized pods containing eight seeds with a sweet taste but are highly susceptible to PM disease (Table 1).
Four sets of 5,000 seeds of both the varieties with a uniform size and 10%–12% moisture content were irradiated with 300-, 400-, 500-, and 600-Gy doses of gamma rays in gamma cells (source: CO60) at Nuclear Research Laboratory, IARI, New Delhi.
Another set of 2,500 pre-soaked seeds of each variety were treated with 0.3% and 0.4% EMS for 8 h at 30 ± 1°C with intermittent shaking in a gyratory shaker. The EMS-treated seeds were thoroughly washed in running water for 8 h to leach out excess EMS.
The research project on the development of PM resistance lines through induced mutation in garden pea was carried out in Research Farms of Chaudhary Sarwan Kumar Himachal Pradesh Agricultural University at two diverse locations, i.e., Palampur and Kukumseri (Lahaul and Spiti). Palampur is situated at 1,290.8 m above mean sea level, 32°6′ N latitude and 76°3′ E longitude with a humid temperate climate (annual rainfall of 2,500 mm). On the other hand, Kukumseri Farm is located at an elevation of 2,672 m above mean sea level with latitudes of 31°44′ N and 76°41′ E, 360 km away from Palampur. It has a dry temperate climate with an annual precipitation of 250 mm and is the most suitable for shuttle breeding by advancing generations in off-season during summer vis-à-vis hot spot for screening genetic material for PM disease reaction.
Both irradiated and EMS-treated seeds were sown in the field at Vegetable Research Farm, Palampur to raise M1 generation during winter 2011–2012. All surviving M1 plants of the respective treatments of both the varieties were harvested individually that constitute 7,092 and 6,776 M2 progenies of Azad P-1 and Lincoln, respectively.
The plant-to-row M2 progenies derived from Azad P-1 (7,092) and Lincoln (6,776) were raised at Kukumseri to isolate PM disease-resistant mutants along with mutants for important morphological traits during summer 2012. The M2 population was carefully observed throughout the growth period and putative mutants were identified based on visual observations in comparison to susceptible parent varieties as control/check.
In the following winter 2012–2013, plant to row M3 progenies of two PM-resistant putative mutant of Lincoln vis-a-vis 142 mutant progenies identified for morphological traits of Lincoln (72) and Azad P-1 (70) were grown along with check varieties Lincoln and Azad P-1 at Palampur. The observations on PM disease incidence were recorded following the (0–4) scale of Mains and Dietz (1930) (Table 2) to identify and isolate PM-resistant mutants.
The resistant mutant lines along with check varieties were evaluated for yield attributes by raising them in a randomized complete block design, replicated thrice at Kukumseri and Palampur from 2014 onwards. Each mutant with check was grown in two rows of 3 m length over the replications with an inter- and intra-row spacing of 45 cm and 7.5–10 cm, respectively. The observations were recorded on 10 randomly selected plants of each genotype in each replication for first flowering node, days to first picking, number of branches plants−1, internode length (cm), nodes plant−1, plant height (cm), pod length (cm), seeds pod−1 (number), shelling percentage, pods plant−1 (number), pod yield plant−1 (g), average pod weight (g), and harvest duration (days). The data on days to 50% flowering were recorded on a plot basis when 50% of the plants of the respective progenies had at least one flower opened.
The data were analyzed using analysis of variance (ANOVA) following Gomez and Gomez (1983) for the randomized complete block design and the significance of mean sum of squares for each trait was tested using the F-test at 5% level while critical difference (CD) using t-test was used for the comparison of means of different traits.
The PM disease reaction in the mutant progenies was recorded by raising them under field (Figure 1) and in vitro conditions, i.e., in pots under naturally ventilated polyhouse conditions (Figure 2) at peak harvest of fresh pods (second/third picking) and at the seed maturity stage. The individual plants were categorized into different classes of disease severity following the (0–4) scale of Mains and Dietz (1930).
Figure 1. Comparative powdery mildew disease reaction. (A, B) L-50-1113 (M2 and M4), (C) Lincoln (highly susceptible), (D) Azad P-1 (highly susceptible reaction), (E, F) isolation of resistant mutant L-40-1014 (M3), (G, H) resistant mutants (AP-0.3-129 and L-0.3-139) under field conditions at Kukumseri.
Figure 2. In vitro screening for powdery mildew disease reaction under nethouse conditions. (A) General view of the experiment; (B) L-0.4-43-1 (powdery growth on lower leaves); (C, D) L-50-1113-1 and L-03-139 (resistant reaction); (E) L-40-1014 (resistant) vs. Lincoln (susceptible); (F, G) susceptible check varieties Lincoln and Azad P-1; (H, I) L-40-1014 and AP-0.3-129 (resistant reaction).
To eliminate chances of disease escape, in vitro multiplied conidial inoculums of the disease were dusted on the plants with a camel hair brush for uniform development of disease infestation to facilitate effective screening of mutants for resistance under greenhouse conditions at Palampur (Figure 3B).
Figure 3. In vitro screening. (A) Detached leaf technique. (B) Isolation chamber with artificial inoculation.
In addition to field and greenhouse screening, the detached leaf method was used to screen the resistant progenies under laboratory conditions (Banyal and Tyagi, 1999). Detached leaf assay of resistant mutants was conducted by floating the leaves of 3- to 4-week-old seedlings on the water solution in Petri dishes under controlled conditions in a plant growth chamber at Palampur (Figure 3A) by keeping temperature at 23 ± 2°C, 50%–70% humidity, and a photoperiod of 16 h light/8 h darkness. The disease inoculum was dusted on the leaves for further development. Observations on the disease development were studied for macroscopic and microscopic density of mycelia and sporulation at 9 days interval (Banyal et al., 2005).
The selection/screening process was carried out until stable lines are attained, i.e., uniform disease reaction across environments and other economic traits. The fixed lines (no variation in genetic makeup) with morphological attributes and PM disease resistance were maintained through evaluation under PM epiphytic conditions continuously until winter 2023–2024 to eliminate the chance of reverse mutation.
Eighteen primers (Supplementary Table S1), i.e., 11 markers (5 SSR and 6 SCAR) linked to er1, 4 markers (3 SSR and 1 SCAR) linked to er2, and 3 markers (2 SSR and 1 SCAR) linked to the Er3 gene of PM resistance, were used for the validation of the resistant gene present in the respective mutants.
The total genomic DNA was extracted from PM-resistant mutant lines, susceptible checks (Lincoln and Azad P-1), and er1 and er2 harboring lines (JI-1559 and JI-2480) followed by polymerase chain reaction (PCR) amplification using er1- and er2-linked markers. DNA was isolated from young leaf tissue by using the CTAB method (Murray and Thompson, 1980). The extracted DNA samples of all genotypes were loaded on 0.8% agarose gel (1 g/100 ml 1× TAE buffer) and run at 90 V for 40 min to determine the quality and quantity of DNA. Furthermore, the Nanodrop 2000c (Thermo Scientific) was used to verify the quantity and purity of the isolated DNA.
DNA amplification was carried out in a 12.5 µL reaction volume containing 20 ng of template DNA, 0.2 mM of each dNTP, 0.2 µM of each primer, 1.5 mM MgCl2, 1× PCR buffer (10 mM Tris-HCl and 50 mM KCl, pH 8.3) and 1 U Taq polymerase. PCR amplification for all primers was carried out in a thermocycler (Make ProFlex) using initial denaturation at 94°C for 5 min followed by 39 cycles at 94°C for 30 s, 50°C–60°C for 30 s, 72°C for 1 min, and a final extension at 72°C for 5 min followed by rapid cooling at 4°C.
Each PCR product (10 µL) was mixed with 3 µL of 6× gel loading dye (0.25% bromophenol blue and 40% sucrose) and amplified DNA fragments were separated on 4% agarose gel prepared in 1× TAE buffer and ethidium bromide (0.5 µg/mL). The gels were run at a constant voltage of 120 V for 1.5 h. The DNA profile was visualized by using the Gel Documentation system (Labnet, ENDURO™ GDS, Aplegen). The presence of appropriate size product of the markers linked to PM-resistant genes was recorded for putative mutant lines, susceptible checks, and er1 and er2 harboring lines by using 50 bp and a 1-kb ladder.
Germination was reduced significantly in all the mutagenic treatments of both varieties (Table 3). Of the total 20,000 irradiated seeds each of Azad P-1 and Lincoln at 300, 400, 500, and 600 Gy that were sown to raise M1 population, a total of 4,853 and 5,570 individual plants that constitute only 24% and 28% of Lincoln and Azad P-1 were harvested, respectively, to raise plant-to-row M2 progenies. Similarly, 19% and 15% of plants were obtained from 10,000 EMS-treated seeds of the respective varieties at 0.3% and 0.4% EMS, i.e., 1,923 and 1,522 M1 plants. The resultant 13,868 M1 plant progenies of irradiated and EMS-treated seeds were harvested separately to raise M2 generation at Kukumseri.
Table 3. PM-resistant progenies isolated in M2 and M3 generations from different gamma rays irradiated and EMS-treated seeds of garden pea varieties Lincoln and Azad P-1.
Plant-to-row 13,868 M2 progenies were raised that resulted in the development of 34,845 M2 plants. Among this large population, only two putative mutant plants of “Lincoln” were isolated that carry a resistant reaction to PM disease and were designated as L-50-1113-1 (500 Gy) and L-0.4-43-1 (0.4% EMS) (Table 3; Figure 1). In addition, agronomically superior plants for various traits, viz., flower color, plant height, vigorous growth, and long pods, were also selected in M2 generation and were harvested separately to raise M3 generation.
Fortunately, out of 142 progenies carrying variable morphological traits, 4 progenies showed segregation for PM disease reaction under field conditions (in vivo) at Palampur during 2012–2013 and were designated as L-40-1014 (Lincoln at 400 Gy), L-0.3-139 (Lincoln at 0.3% EMS), AP-0.3-439, and AP-0.3-129 (Azad P-1 at 0.3% EMS) (Table 3). The M3 generation of L-50-1113-1 and L-0.4-43-1 was raised in progeny rows constituting 19 and individual plants of resistant progenies of both PM-resistant mutants and other desirable traits were raised at Palampur during winter 2012–2013. All 19 M3 progenies of L-50-1113-1 showed a resistant reaction whereas 23 progenies of L-0.4-43-1 showed susceptible reaction (Table 4). The resistant plants of these progenies were harvested individually and were further evaluated at Kukumseri during summer 2013 for validation of resistance.
Table 4. Powdery mildew disease reaction of putative mutants in M3 and M4 generation in polyhouse and field conditions during 2012–2013 at Palampur.
All individual plant progenies of L-50-1113-1, L-40-1014-1, L-0.3-139, AP-0.3-439, and AP-0.3-129 carrying resistance to powdery disease reaction were evaluated separately in the following years along with pod and plant characters. The plant-to-row progenies of these five mutants that showed segregation or inferiority for pod attributes were discarded and only those with uniform pod and plant characteristic were selected for evaluating them for yield attributes, e.g., seven of L-50-1113 (-1, -2, -3, -4, -6, -8, and -10), two each of L-40-1014 (-1 and -6) and L-0.3-139 (-1 and -7), one of AP-0.3-439-1, and three of AP-0.3-129 (-1, -2, and -3) at Kukumseri during 2014 (Table 5; Figure 1). Majority of the plant-to-row progenies of the isolated mutants showed resistant reaction (1) with powdery growth in traces only on leaves at the end of vegetative growth while pods and stem remained free except AP-0.3-439-1 with a highly susceptible reaction (Table 5). The putative mutant progenies selected within the same group performed almost similar for PM and accordingly only one progeny in each set was retained with better performance for pod and plant attributes for further evaluation that constitute four mutants, namely, L-50-1113-1-6, L-40-1014-1-1, L-0.3-139-1-7, and AP-0.3-129-2. The mutant lines L-50-1113-1-6 and L-40-1014-1-1 recorded pod yields of 58.33 and 58.63 g plant−1, respectively, which were significantly better than check variety Lincoln (43.33 g) and Azad P-1 (40.67 g), and at par with Pb-89 (58.0 g).
Table 5. Performance of PM-resistant mutants in M4 generation for pod yield and related traits during summer 2014 at Kukumseri.
Furthermore, all these mutant progenies showed resistant reaction at Palampur during winter 2014–2015 under field conditions and using the detached leaf assay method (Table 6) along with a desirable performance for yield and its attributes, namely, pod length, seeds/pod, and shelling (%). Lines L-50-1113-1-6 and L-40-1014-1-1 produced pod yields of 93.20 and 94.25g plant−1, respectively, which were at par with check variety Lincoln and Azad P-1. These mutant progenies were finally designated as “L-50-1113”, “L-40-1014”, “L-0.3-139-1”, and “AP-0.3-129” for further evaluation/screening.
Table 6. Performance of PM-resistant mutants in M5 generation for pod yield, related traits, and disease reaction during winter 2014–2015 at Palampur.
The four resistant mutant progenies raised in pots under greenhouse conditions during 2014–2015 at Palampur by providing favorable conditions of temperature and humidity along with dusting of PM inoculum at the seedling stage showed resistance only in three mutants, i.e., L-40-1014, L-0.3-139-1, and AP-0.3-129, while L-50-1113 showed susceptible reaction. The further evaluation of these three resistant lines followed the same trend for disease reaction under in vivo and in vitro conditions over the years, i.e., at Kukumseri during 2017 and at Palampur for four consecutive years (2018–2019 to 2021–2022) under field conditions (Table 7), and net house/polyhouse (Figure 2) for six consecutive years (2018–2019 to 2023–2024) along with isolation chamber (Figure 3) at Palampur (2018–2019), which was further validated by the detached leaf assay method (Table 8, Figure 3). These three resistant lines L-40-1014, L-0.3-139, and AP-0.3-129 were further validated for the PM-resistant genes er1, er2, and Er3 by using linked molecular markers.
Only two SSR markers “PSMPSAD60” and “PSMPA5” linked to the er-1 gene differentiated the susceptible check, resistant checks, and mutants from one another (Figure 4). The primer “PSMPA5” linked to the er1 gene showed that mutant L-0.3-139 derived from Lincoln had the same size amplification (340 bp) as that of resistant parent JI1559 indicating the possibility of resistance in L-0.3-139 due to the er1 gene. The other SSR marker “PSMPSAD60” also validated resistance in L-0.3-139 due to the er1 gene as it showed the same size amplification (225 bp) as that of JI1559 (source of the er1 gene) but the susceptible parent Azad P-1 also showed the band at the same position as in the resistant parent JI1559.
Figure 4. Agarose gel showing the presence/absence/polymorphism of amplification products of er-1-linked primers (PSMPSAD60 d, PSMPSAA374 e, and PSMPA5 c) and er-2-linked primers (AC30, ScX171400, and AD141) in the susceptible checks (Lincoln and Azad P-1), resistant checks (JI1559: source of er-1 gene, JI2480: source of er-2), and putative mutated lines (L-40-1014, L-0.3-139, and AP-0.3-129); M1 = 50 bp DNA Ladder, 1 = JI1559 in the first lane and JI2480 in the second lane, 2 = Azad P-1, 3 = AP-0.3-129, 4 = Lincoln, 5 = L-0.3-139, 6 = L-40-1014(1), 7 = L-40-1014(2), M2 = 1 Kb DNA Ladder.
SCAR markerScX171400 linked to the er2 gene showed the specific band at 1,400 bp in the resistant parent JI2480 (source of er2) and mutant L-40-1014 (Figure 4), indicating the possibility of the er2 gene governing resistance in mutant L-40-1014. Similar results were recorded with the SSR primer AD141 as the resistant parent JI2480 and mutant L-40-1014 revealed the bands at the same position (200 bp) harboring the resistance governed by the er2 gene. Er3-linked primers could not validate the presence in the mutants that may be due to the presence of recessive gene of PM resistance in the putative mutants.
The artificial mutagenesis led to the introduction of innovative genetic variations that result in the development of new cultivars, functional description of genes, and the conception of gene–trait associations in many crops. Induced mutagenesis increases the frequency and range of mutations in the desired plant characteristics. The PM is one of the economically significant diseases of garden pea that has a marked effect on the quality and quantity of production. The management of disease through fungicides has severe consequences on human health and environment, and therefore, genetic resistance is ideal and sustainable. The management of PM in pea through resistance breeding has been the subject of several research investigations to date (Heringa et al., 1969; Tiwari et al., 1997; Fondevilla et al., 2007b; Katoch et al., 2010; Pavan et al., 2010; Sharma et al., 2013). Genetic resistance in plants has the advantage to boost productivity and handle the situation for a long period (Nigussie et al., 2008). Keeping in view the significance of pea and disease, we undertook the study on mutagenesis using both physical and chemical mutagens. The Mn generations were developed with an aim to isolate PM disease-resistant mutants.
We raised 13,868 M2 progenies consisting of 34,845 plants of two mutagenized populations of Lincoln and Azad P-1, both gamma irradiated and EMS treated for screening against PM disease infection. The screening was primarily undertaken at Highland Agricultural Research and Extension Centre, Kukumseri, Lahaul, and Spiti having a dry temperate climate and is situated in the northwestern Himalayas during the off-season in summer and we were able to isolate only two resistant plants that were designated as “L-50-1113-1” and “L-0.4-43-1”(Table 3; Figure 1). The very low frequency was obvious because the northwestern Himalayas are the hot spot for PM disease development on account of favorable environmental conditions for disease growth (Rana et al., 2013). They reported the evidence of existence of pathogenic virulence of E. pisi in the Himalayas because the cleistothecia (reproductive stage) of pathogen are usually established exclusively in the dry temperate zone. Furthermore, a more virulent race of pathogen identified as “Kukumseri” showed more disease pressure and severe illness development that substantiate the area as the hot spot for PM disease. Therefore, Kukumseri was preferred for M2 screening based on the high level of discrimination in the population. Simultaneously, 142 progenies were selected based on plant and pod characteristics. The 142 M3 plants were grown in plant-to row progenies for further selection at Palampur during 2012–2013 out of which four resistant progenies were isolated, i.e., L-40-1014, L-0.3-139, AP-0.3-439, and AP-0.3-129 (Table 3; Figure 1). The rigorous evaluation/screening of plant-to-row M4 and M5 progenies of these six mutants at Kukumseri and Palampur, respectively, resulted in the identification of four mutants, which were finally designated as “L-50-1113”, “L-40-1014”, “L-0.3-139-1”, and “AP-0.3-129”. The evaluation was continued over the years (2017 to 2024) under in vivo conditions at Palampur and Kukumseri (Table 7) along with in vitro conditions at Palampur (greenhouse/isolation chamber/natural ventilated polyhouse and detached leaf assay) (Table 8; Figures 2, 3), which resulted in the identification of three mutants with a PM score of 1 (resistant), viz., “L-40-1014”, “L-0.3-139-1” and “AP-0.3-129”. In vitro testing with disease pathogen inoculation validates the presence of resistance in the cultivars. Line L-40-1014 also showed better performance for pod yield and other important attributes that can be utilized for commercial cultivation after multi-location testing.
In our current study, the resistant plants showed some patches of PM growth on the leaves at the end of the vegetation period especially at Kukumseri, suggesting some kind of quantitative expression of the resistance. Pereira and Leitao (2010) reported that this type of quantitative expression is misleading, and a simple, but rigorous screening of individual plants will permit its qualitative classification in clearly distinct classes, either resistant or susceptible. The strong qualitative expression of the resistance can be recorded at the end of the vegetative period where the disease infestation profoundly appears on pods, causing deterioration of pod quality and making them unfit for consumption. In contrast, the designated resistant plants showed no spots of infection on pods and resulted in unequivocally healthy pods. In other words, categorization of PM resistance can be established during the vegetation stage based on inclusive plant appearance and pod infection (Sharma, 2003). Based on multiple experiments on PM resistance, it was concluded that the resistant plants can be qualitatively distinguished based on infection on the stem, peduncles, and pods, while ignoring the fungal growth on the foliage whatever its intensity.
Three putative resistant lines L-40-1014, L-0.3-139-1, and AP-0.3-129 identified from the present study have the PM resistance potential and can be further used as a resistant source in pea breeding programs. These three putative resistant lines were further validated for the PM resistance gene by linked molecular markers. Only two er1 and er2 recessive genes and one dominant Er-3 gene have been identified to provide resistance to E. pisi (Harland, 1948; Heringa et al., 1969; Fondevilla et al., 2007a). It was found that the bulk of E. pisi-resistant pea germplasm has the er1 recessive gene, and it confers long-term broad-spectrum resistance to PM disease (Tiwari et al., 1997). Researchers found different markers linked to the resistance genes er1 and er2 and the markers were placed at varied genetic distances in different mapping populations. Ek et al. (2005) found five SSR markers (PSMPSA5, PSMPSAD51, PSMPSAD60, PSMPSAA374e, and PSMPSAA369) linked to the er1 gene with PSMPSAD60 being the most closely associated marker, at 10.4 cM distance from the er1 locus, and PSMPA5 c at 14.9 cM. They are flanked markers for the er1 gene. These markers showed nearly the same size amplification in the mutant L-0.3-139 as that of the resistant parent JI1559, indicating the possibility of resistance to be governed by the er1 gene. Pereira et al. (2010) identified two altered genes, er1 mut1 and er1 mut-2, by using ethyl nitrosourea mutagenesis, which leads to the development of PM-resistant pea mutants, and the SSR marker PSMPSA5 was found to be related to the er1 mut-2 locus at a greater distance. Thus, our findings suggest that the mutation has affected the er1 locus, which is frequently found in naturally occurring resistances for PM. It was also observed that mutations are more frequent in the er1 locus as compared to the er2 locus (Pereira and Leitao, 2010). The er1 gene was placed on linkage group VI (Timmerman et al., 1994) and the er2 gene was located on linkage group III (Katoch et al., 2010). Molecular markers found closely linked to the resistance locus will be beneficial for marker-assisted breeding that exploits the mutant gene to create new PM-resistant cultivars. SCAR marker ScX171400 (Katoch et al., 2010) linked to the er2 gene clearly validated the er2 gene in mutant L-40-1014 by showing the specific band of 1,400 bp in a resistant parent (JI 2480) and mutant L-40-1014. Similarly, the SSR marker AD141 at 9.3 cM linked to er2 gene/allele (Gupta et al., 2023) also validated this gene in the resistant parent and mutant L-40-1014 by depicting a specific band of 200 bp.
Systemic acquired resistance (SAR) plays a significant role in the genetic processes of pathogen resistance, which is related to the expression of resistance genes and the induction of the hypersensitive reaction (Pavan et al., 2010; Martins et al., 2020). This mechanism of resistance helps plants to deal with the presence of nonspecific and opportunistic infections on a regular basis. Susceptibility genes (S-genes) are the genes that encode special target proteins. Loss-of-function mutations in S-genes frequently result in broad-spectrum resistance to a specific pathogen (Eckardt, 2002; Pavan et al., 2010). In the mutant AP-0.3-129, none of the gene is confirmed for the resistance but Azad P-1 susceptible check showed a band pattern like the er1 gene. Thus, the resistance in the mutant AP-0.3-129 may be due to the loss-of-function mutations in S-genes. The gene er1 has been widely employed in pea breeding, although having the same source of resistance may increase the incidence of new races and eventually leads to resistance breakdown. Combining many key genes from diverse sources into a variety can result in long-lasting resistance and the mutants identified in the present study can be promising candidates for the new source of resistance for future pea breeding improvement. This breeding method should be supplemented using more accurate PM resistance-linked molecular markers. The key disadvantage is that such markers are obtained from genomic areas that play no causal role in the development of resistance and may show a similar pattern in both susceptible and resistant genotypes. As a result, gene-based markers resulting from functional polymorphisms within resistance genes must be utilized to screen the germplasm for target genes. The specific use of molecular markers in the improvement of pea breeding accentuates the development of PM resistance new gene-specific markers. The progress of high-throughput next-generation sequencing (NGS) and genotyping technologies has resulted in a significant decrease in the cost for developing molecular markers, which paves the way for a marker-assisted breeding approach as a more wide-ranging, useful, efficient, and cost-effective method in the future.
The current study resulted in the isolation of three new PM disease resistance lines, viz., L-40-1014, L-0.3-139, and AP-0.3-129, following induced mutagenesis, and these were validated for PM-resistant genes er1 and er2 by using linked molecular markers. SSR markers PSMPSAD60 d and PSMPA5 c linked to the er1 gene indicated the presence of this gene in the mutant L-0.3-139 while SCAR marker ScX171400 an SSR marker, AD141, linked to the er2 gene clearly validated the er-2 gene in mutant L-40-1014. The resistance in the mutant AP-0.3-129 was found to be governed by a different mechanism and may be due to the loss-of-function mutations in S-genes. Line L-40-1014 also showed promise for pod yield and other important pod and plant attributes. The identified mutants with PM resistance potential can be promising candidates as new sources of resistance for future pea breeding programs.
The original contributions presented in the study are included in the article/Supplementary Material. Further inquiries can be directed to the corresponding author.
AS: Conceptualization, Data curation, Funding acquisition, Investigation, Methodology, Project administration, Supervision, Validation, Visualization, Writing – original draft, Writing – review & editing. DB: Data curation, Investigation, Writing – review & editing. VD: Supervision, Writing – review & editing. B: Data curation, Writing – review & editing. RR: Writing – review & editing. RK: Writing – review & editing. PK: Conceptualization, Writing – review & editing. NK: Data curation, Writing – review & editing. S: Data curation, Writing – review & editing. AP: Writing – review & editing. VS: Writing – review & editing. AnS: Writing – review & editing.
The author(s) declare financial support was received for the research, authorship, and/or publication of this article. We are grateful to the Bhabha Atomic Research Centre (BARC) 2011/35/8/BRNS/1500 (Rs. 21,15,800/- or USD 32550), Trombay, Mumbai (India) for their financial grant to carry out the research. We acknowledge the funds provided by the National Agricultural Higher Education Project (NAHEP) F. No. NAHEPCAAST/2019-20;DY No. 642 NC(NAHEP) amount 3.14 million USD, Indian Council of Agricultural Research, New Delhi that helped to undertake molecular validation studies.
The authors declare that the research was conducted in the absence of any commercial or financial relationships that could be construed as a potential conflict of interest.
The author(s) declare that no Generative AI was used in the creation of this manuscript.
All claims expressed in this article are solely those of the authors and do not necessarily represent those of their affiliated organizations, or those of the publisher, the editors and the reviewers. Any product that may be evaluated in this article, or claim that may be made by its manufacturer, is not guaranteed or endorsed by the publisher.
The Supplementary Material for this article can be found online at: https://www.frontiersin.org/articles/10.3389/fpls.2024.1501661/full#supplementary-material
Anonymous (2021). Food and Agriculture organization of the United Nations (FAO). Available at: https://www.fao.org/statistics/en
Anonymous (2024). Area and Production of Horticulture crops for 2023-24 (3rd Advance Estimates). Department of Agriculture & Farmers Welfare, GOI, Ministry of Agriculture & Farmers' Welfare. Available online at: https://agriwelfare.gov.in/en/StatHortEst (Accessed January 15, 2025).
Banyal, D. K., Singh, A., Tyagi, P. D. (2005). Pathogenic variability in Erysiphe pisi causing pea powdery mildew. Himachal J. Agric. Res. 32, 87–92.
Banyal, D. K., Tyagi, P. D. (1999). Development of powdery mildew of pea in relation to different climatic condition in Himachal Pradesh. Plant Dis. Res. 13, 154–156.
Bhat, S. R., Haque, A., Chopra, V. L. (2001). Induction of mutations for cytoplasmic male sterility and some rare phenotypes in Indian mustard (Brassica juncea L.). Indian J. Genet. 61, 335–340. Available online at: https://www.isgpb.org/journal/index.php/IJGPB/article/view/1801
Bobkov, S. V., Selikhova, T. N. (2021). Introgession of powdery mildew resistance into cultural pea from wild accession of P. fulvum. IOP Conf. Ser. Earth Environ. Sci. 650, 12091. doi: 10.1088/1755-1315/650/1/012091
Dreiseitl, A. (2024). Mlo-mediated broad-spectrum and durable resistance against powdery mildews and its current and future applications. Plants 13, 138. doi: 10.3390/plants13010138
Eckardt, N. A. (2002). Plant disease susceptibility genes? Plant Cell 14, 1983–1986. doi: 10.1105/tpc.140910
Ek, M., Eklund, M., von Post, R., Dayteg, C., Henriksson, T., Weibull, P., et al. (2005). Microsatellite markers for powdery mildew resistance in pea (Pisum sativum L.). Hereditas 142, 86–91. doi: 10.1111/j.1601-5223.2005.01906.x
Fondevilla, S., Carver, T. L. W., Moreno, M. T., Rubiales, D. (2006). Macroscopic and histological characterisation of genes er1 and er2 for powdery mildew resistance in pea. Eur. J. Plant Pathol. 115, 309–321. doi: 10.1007/s10658-006-9015-6
Fondevilla, S., Carver, T. L. W., Moreno, M. T., Rubiales, D. (2007b). Identification and characterization of sources of resistance to Erysiphe pisi Syd. in Pisum spp. Plant Breed J. 126, 113–119. doi: 10.1111/j.1439-0523.2006.01312.x
Fondevilla, S., Rubiales, D. (2012). Powdery mildew control in pea: A review. Agron. Sustain Dev. 32, 401–409. doi: 10.1007/s13593-011-0033-1
Fondevilla, S., Torres, A. M., Moreno, M. T., Rubiales, D. (2007a). Identification of a new gene for resistance to powdery mildew in Pisum fulvum, a wild relative of Pea. Breed Sci. 57, 181–184. doi: 10.1270/jsbbs.57.181
Gomez, K. A., Gomez, A. A. (1983). Statistical Procedures for Agricultural Research (New York: John Wiley and Sons), 357–427.
Gupta, A., Singh, B., Kumar, M., Sirohi, U., Yadav, S. K., Sharma, V. R. (2023). Identification of SSR markers linked to powdery mildew resistance in table pea (Pisum sativum var. hortense L.). Legume Res. 47, 1032–1038. doi: 10.18805/LR-5085
Harland, S. C. (1948). Inheritance of immunity to mildew in Peruvian forms of Pisum sativum. Heredity 2, 263–269. doi: 10.1038/hdy.1948.15
Heringa, R. J., van Norel, A., Tazelaar, M. F. (1969). Resistance to powdery mildew (Erysiphe polygoni D. C.) in peas (Pisum sativum L.). Euphytica 18, 163–169. doi: 10.1007/BF00035687
Humphry, M., Reinstädler, A., Ivanov, S., Bisseling, T., Panstruga, R. (2011). Durable broad-spectrum powdery mildew resistance in pea er1 plants is conferred by natural loss-of-function mutations in PsMLO1. Mol. Plant Pathol. 12, 866–878. doi: 10.1111/j.1364-3703.2011.00718.x
Janila, P., Sharma, B. (2004). RAPD and SCAR markers for powdery mildew resistance gene er in pea. Plant Breed. 123, 271–274. doi: 10.1111/j.1439-0523.2004.00963.x
Kalapchieva, S., Tomlekova, N. (2016). Sensitivity of two garden pea genotypes to physical and chemical mutagens. J. Biosci. Biotechnol. 5, 167–171. Available online at: https://editorial.uni-plovdiv.bg/index.php/JBB/article/view/21/47
Katoch, V., Sharma, S., Pathania, S., Banayal, D. K., Sharma, S. K., Rathour, R. (2010). Molecular mapping of pea powdery mildew resistance gene er2 to pea linkage group III. Mol. Breed. 25, 229–237. doi: 10.1007/s11032-009-9322-7
Mains, E. B., Dietz, S. M. (1930). Physiologic form of barley, Erysiphe graminis hordei Marchal. Phytopathology 20, 229–239.
Martins, D., de Sousa Araujo, S., Rubiales, D., Patto, M. C. V. (2020). Legume crops and biotrophic pathogen interactions: A continuous cross-talk of a multilayered array of Défense mechanisms. Plants 9, 1460. doi: 10.3390/plants9111460
Murray, M. G., Thompson, W. F. (1980). Rapid isolation of high molecular weight plant DNA. Nucleic Acids Res. 8, 4321–4326. doi: 10.1093/nar/8.19.4321
Nigussie, T., Seid, A., Derje, G., Tesfaye, B., Chemeda, F., Adane, A., et al. (2008). “In: Increasing crop production through improved plant protection,” in Review of research on diseases food legumes, vol. 1. Ed. Tadesse, A., (Addis Ababa, Ethiopia: Plant Protection Society of Ethiopia), 85–124.
Pandey, P. K., Bhowmik, P., Kagale, S. (2022). Optimized methods for random and targeted mutagenesis in field pea (Pisum sativum L.). Front. Plant Sci. 13. doi: 10.3389/fpls.2022.995542
Pavan, S., Jacobsen, E., Visser, R. G. F., Bai, Y. (2010). Loss of susceptibility as a novel breeding strategy for durable and broad-spectrum resistance. Mol. Breed. 25, 1–12. doi: 10.1007/s11032-009-9323-6
Pavan, S., Schiavulli, A., Appiano, M., Marcotrigiano, A. R., Cillo, F., Visser, R. G. F., et al. (2011). Pea powdery mildew er1 resistance is associated to loss-of-function mutations at a MLO homologous locus. Theor. Appl. Genet. 123, 1425–1431. doi: 10.1007/s00122-011-1677-6
Pereira, G., Leitao, J. (2010). Two powdery mildew resistance mutations induced by ENU in Pisum sativum L. affect the locus er1. Euphytica 171, 345–354. doi: 10.1007/s10681-009-0029-y
Pereira, G., Marques, C., Ribeiro, R., Formiga, S., Damaso, M., Sousa, T., et al. (2010). Identification of DNA markers linked to an induced mutated gene conferring resistance to powdery mildew in pea (Pisum sativum L.). Euphytica 171, 327–335. doi: 10.1007/s10681-009-0003-8
Rana, J. C., Banyal, D. K., Sharma, K. D., Sharma, M. K., Gupta, S. K., Yadav, S. K. (2013). Screening of pea germplasm for resistance to powdery mildew. Euphytica 189, 271–282. doi: 10.1007/s10681-012-0798-6
Rana, C., Sharma, A., Rathour, R., Bansuli, Banyal, D. K., Rana, R. S., et al. (2023). In vivo and in vitro validation of powdery mildew resistance in garden pea genotypes. Sci. Rep. 13, 2243. doi: 10.1038/s41598-023-28184-0
Rana, C., Sharma, A., Sharma, K. C., Mittal, P., Sinha, B. N., Sharma, V. K., et al. (2021). Stability analysis of garden pea (Pisum sativum L.) genotypes under North Western Himalayas using joint regression analysis and GGE biplots. Genet. Resour. Crop Evol. 68, 1–12. doi: 10.1007/s10722-020-01040-0
Rungruangmaitree, R., Jiraungkoorskul, W. (2017). Pea, Pisum sativum, and its anticancer activity. Pharmacogn. Rev. 11, 39–42. doi: 10.4103/phrev.phrev_57_16
Sharma, B. (2003). The Pisum genus has only one recessive gene for powdery mildew resistance. Pisum Genet. 35, 22–27. Available at: https://scholar.google.com/scholar_lookup?journal=Pisum%20Genet.&title=The%20Pisum%20genus%20has%20only%20one%20recessive%20gene%20for%20powdery%20mildew%20resistance&author=B.%20Sharma&volume=35&publication_year=2003&pages=22-27&
Sharma, A., Kapur, P., Katoch, V., Singh, Y., Sharma, J. D. (2013). Development of powdery mildew resistant genotypes in garden pea (Pisum sativum L.) through generation mean analysis approach. Indian J. Genet. 73, 371–377. doi: 10.5958/j.0975-6906.73.4.056
Sharma, A., Rathour, R., Plaha, P., Katoch, V., Khalsa, G. S., Patial, V., et al. (2010). Induction of Fusarium wilt (Fusarium oxysporum f. sp. pisi) resistance in garden pea using induced mutagenesis and in vitro selection techniques. Euphytica 173, 345–356. doi: 10.1007/s10681-009-0099-x
Sharma, A., Sekhon, B. S., Sharma, S., Kumar, R. (2020). Newly isolated intervarietal garden pea (Pisum sativum L.) progenies (F7) under north western Himalayan conditions of India. Exp. Agric. 56, 76–87. doi: 10.1017/S0014479719000115
Sharma, A., Sharma, S., Kumar, N., Rana, R. S., Sharma, P., Kumar, P., et al. (2022). Morphomolecular genetic diversity and population structure analysis in garden pea (Pisum sativum L.) genotypes using simple sequence repeat markers. PloS One 17, e0273499. doi: 10.1371/journal.pone.0273499
Timmerman, G. M., Frew, T. J., Weeden, N. F. (1994). Linkage analysis of er1, a recessive Pisum sativum gene for resistance to powdery mildew fungus (Erysiphe pisi D.C.). Theor. Appl. Genet. 88, 1050–1055. doi: 10.1007/BF00220815
Keywords: mutagenesis, garden pea, Erysiphe pisi Syd, resistance, screening, er genes, molecular markers
Citation: Sharma A, Banyal DK, Dhole VJ, Bansuli, Rana RS, Kumar R, Kumar P, Kumar N, Srishti, Prashar A, Singh V and Sharma A (2025) Development of new powdery mildew resistant lines in garden pea (Pisum sativum L.) using induced mutagenesis and validation of resistance for the er1 and er2 gene through molecular markers. Front. Plant Sci. 15:1501661. doi: 10.3389/fpls.2024.1501661
Received: 25 September 2024; Accepted: 31 December 2024;
Published: 28 January 2025.
Edited by:
Nicolas Rispail, Spanish National Research Council (CSIC), SpainReviewed by:
Vennampally Nataraj, ICAR Indian Institute of Soybean Research, IndiaCopyright © 2025 Sharma, Banyal, Dhole, Bansuli, Rana, Kumar, Kumar, Kumar, Srishti, Prashar, Singh and Sharma. This is an open-access article distributed under the terms of the Creative Commons Attribution License (CC BY). The use, distribution or reproduction in other forums is permitted, provided the original author(s) and the copyright owner(s) are credited and that the original publication in this journal is cited, in accordance with accepted academic practice. No use, distribution or reproduction is permitted which does not comply with these terms.
*Correspondence: Akhilesh Sharma, assharmaakhil1@gmail.com
Disclaimer: All claims expressed in this article are solely those of the authors and do not necessarily represent those of their affiliated organizations, or those of the publisher, the editors and the reviewers. Any product that may be evaluated in this article or claim that may be made by its manufacturer is not guaranteed or endorsed by the publisher.
Research integrity at Frontiers
Learn more about the work of our research integrity team to safeguard the quality of each article we publish.