- 1National Biopesticide Engineering Research Centre, Hubei Biopesticide Engineering Research Centre, Hubei Academy of Agricultural Sciences, Wuhan, China
- 2College of Food Science and Technology, Huazhong Agricultural University, Wuhan, China
The quality of fresh tea leaves is crucial to the final product, and maintaining microbial stability in tea plantations is essential for optimal plant growth. Unique microbial communities play a critical role in shaping tea flavor and enhancing plant resilience against biotic stressors. Tea production is frequently challenged by pests and diseases, which can compromise both yield and quality. While biotic stress generally has detrimental effects on plants, it also activates defense metabolic pathways, leading to shifts in microbial communities. Microbial biocontrol agents (MBCAs), including entomopathogenic and antagonistic microorganisms, present a promising alternative to synthetic pesticides for mitigating these stresses. In addition to controlling pests and diseases, MBCAs can influence the composition of tea plant microbial communities, potentially enhancing plant health and resilience. However, despite significant advances in laboratory research, the field-level impacts of MBCAs on tea plant microecology remain insufficiently explored. This review provides insights into the interactions among tea plants, insects, and microorganisms, offering strategies to improve pest and disease management in tea plantations.
1 Introduction
Tea is one of the three major non-alcoholic beverages in the world. Global tea demand has increased significantly during the COVID-19 pandemic (Castellana et al., 2021), a trend that may be closely related to the potential benefits of bioactive substances in tea (Gilbert, 2019; Bag et al., 2022b). Reports from the International Tea Committee (ITC) and the Food and Agriculture Organization of the United Nations (FAO) indicated that in 2022, the global annual value of tea production exceeded $17 billion, with the world tea trade amounting to approximately $9.5 billion (FAO, 2022). The tea industry is among the key agricultural activities in many countries, which is vital in sustaining rural economies (Jayasinghe and Kumar, 2021; Bermúdez et al., 2024). To ensure the production of high-quality tea, tea farmers and researchers have long been committed to these pursuits. Tea production primarily relies on tea trees (Camellia sinensis), and the quality of fresh tea leaves forms the foundation for high-quality finished tea. The growth conditions of tea plants, including growth environment, soil quality, climate, and cultivation practices, significantly impact the quality of fresh tea leaves. However, during the growth process, tea plants frequently face threats from pests and diseases.
Biotic stress factors such as tea blister blight (caused by Exobasidium vexans Massee), tea anthracnose (caused by Colletotrichum spp.), tea looper (Ectropis oblique), and tea red spider mite (Oligonychus coffeae) have long threatened tea yield and quality. If not effectively controlled, these pests and diseases can lead to production losses of up to 55% (Hazarika et al., 2009). Although precise data on economic losses is difficult to determine, it is estimated that these impacts may amount to billions of dollars annually. In recent years, climate change has increased the risk of new pathogens and pests in tea plantations. Rising temperatures, altered rainfall patterns, and extreme weather have expanded the range of these threats, enabling them to thrive in previously unsuitable areas (Pandey et al., 2021b). Additionally, Biotic stress activates the expression of the defense genes in tea plants such as caffeine, catechins, L-theanine, and volatile compounds (Zeng et al., 2024), thereby impacting its flavor and health benefits (Berg and Smalla, 2009). Traditionally, chemical pesticides have long been the primary method for controlling these pests and diseases in tea plantations. However, many studies have demonstrated that the application of synthetic pesticides directly affects environmental microorganisms (Wang and Cernava, 2020; Win et al., 2021) and may also indirectly influence them by interfering with plant metabolic pathways (Liu et al., 2018). For instance, glyphosate can severely weaken the defense mechanisms of glyphosate-sensitive plants (including tea plants) against microbial diseases by disrupting the shikimate pathway, thereby significantly enhancing its efficacy as an herbicide (Martinez et al., 2018).
In contrast, microbial control methods have increasingly shown advantages in tea plantations (Ahirwar et al., 2020; Idris et al., 2020). Microbial biocontrol agents (MBCAs) primarily consist of entomopathogenic and antagonistic microorganisms, which specifically target pests and pathogens while minimizing harm to non-target organisms and the environment (Roy and Muraleedharan, 2014). Compared to chemical pesticides, MBCAs offer safer and more sustainable pest management solutions. However, since MBCAs contain large quantities of exogenous microorganisms, their impact extends beyond just pest and disease control, influencing the broader tea plant ecosystem (Grosch et al., 2012). Microorganisms play a crucial role in tea plant growth by forming symbiotic relationships within and on plant surfaces, contributing to a balanced and dynamic ecosystem (Trivedi et al., 2020; Tan et al., 2022; Jibola-Shittu et al., 2024). These microbial communities primarily influence the plant’s metabolism or produce bioactive compounds, affecting the plant’s characteristic metabolites (Liao et al., 2022; Wu et al., 2024a). These interactions shape the community structure and succession of tea plant microorganisms, which are intricately linked to plant health, resilience, and overall growth (Hu et al., 2018; Xie et al., 2020; Hazarika et al., 2021; Bag et al., 2022a). For example, Xin et al (Xin et al., 2024)identified specific microorganisms in the root microbiomes of high-theanine and low-theanine tea varieties that may regulate theanine levels by influencing nitrogen metabolism. Similarly, Sun et al (Sun et al., 2019). isolated an endophytic bacterium (Luteibacter spp.) from tea seedlings, which exhibited biocatalytic activity by converting glutamine and ethylamine into theanine. The stability of these microbial communities is affected by various factors (Gong et al., 2024), such as pesticide application (Duke, 2018; Hage-Ahmed et al., 2019), biotic stress (Huang et al., 2023), abiotic stress (Zeng et al., 2019a; Bora et al., 2022b), etc. MBCAs act directly on the microbial community within the tea plant ecosystem and may indirectly influence tea quality by altering the structure of the microbial community (Cernava et al., 2019). Bora et al (Bora et al., 2022a). found that using microbial consortia to combat grey blight disease (caused by Pseudopestalotiopsis curvatispora Petch) not only suppressed the pathogens effectively but also increased rhizosphere microbial diversity and enhanced leaf nutrient content. Certain MBCAs may also induce systemic resistance in tea plants and improve their nutritional status as biofertilizers. Nevertheless, current research on commercially MBCAs has predominantly focused on the initial screening of biocontrol strains, with relatively less attention given to their practical impacts on ecological microorganisms and tea plant metabolism.
In light of these considerations, this review aims to provide a concise overview of the main types and mechanisms of commercially available or promising MBCAs used in tea plantations, their colonization efficacy, and their impact on the tea plant’s microbial community. Additionally, this review explores the influence of biotic stress and exogenous microorganisms on tea plant metabolism, while briefly introducing newly discovered microorganisms and their potential applications in the outlook section. The interactions between the main factors discussed in this review are illustrated in Figure 1. By offering new insights into the interactions among tea plants, insects, and microorganisms, this review aims to advance practical pest and disease management strategies in tea plantations and support the biocontrol agent industry.
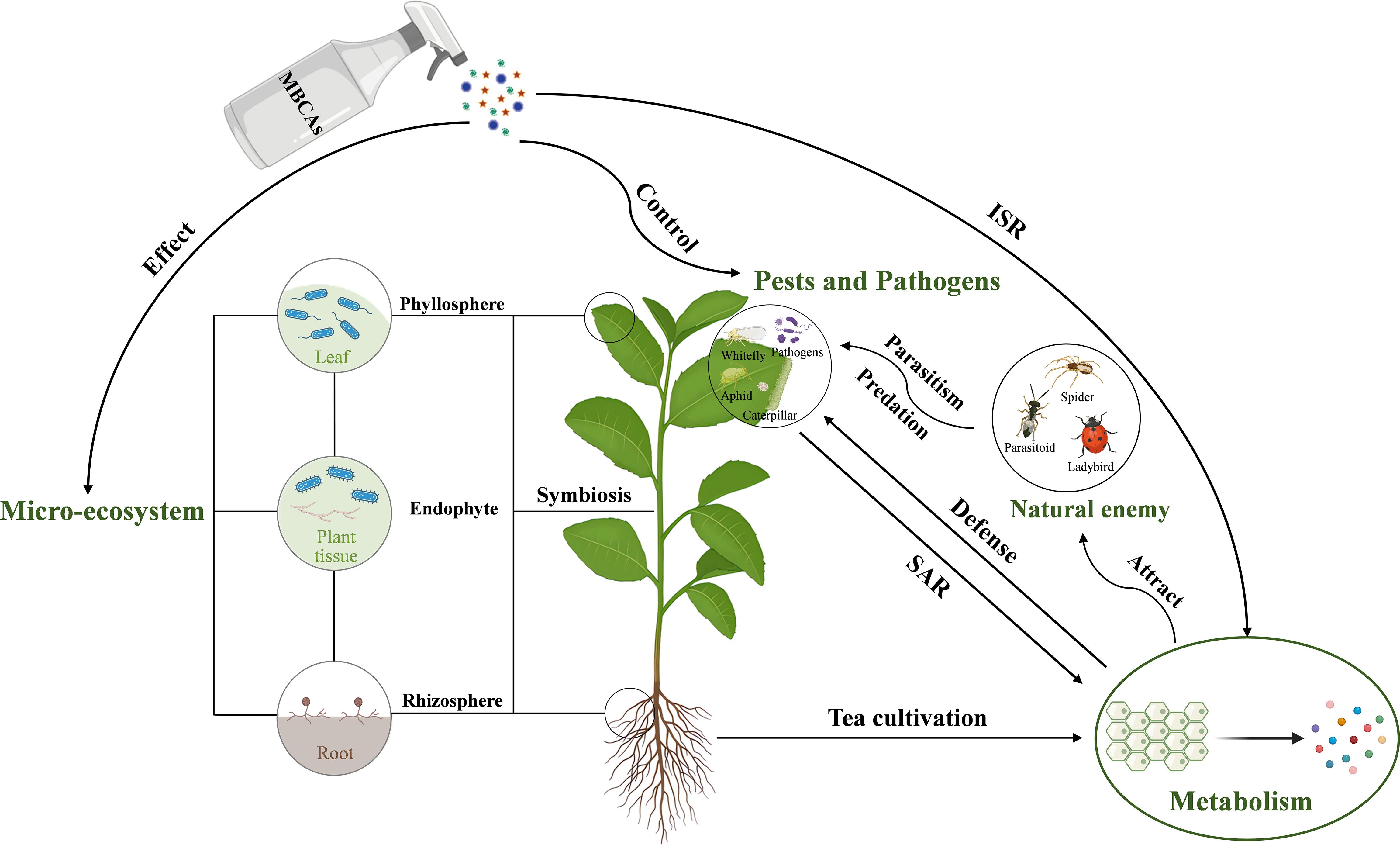
Figure 1. The interactions between MBCAs, tea plant microorganisms, insects, tea plant pathogens, and tea plant metabolism. MBCAs, microbial biocontrol agents; ISR, induced systemic resistance; SAR, systemic acquired resistance.
2 Types and mechanisms of microbial biocontrol agents applied in pests and diseases control of tea
To understand the impact of MBCAs on the micro-ecosystem of tea plantations and the metabolism of tea plants, it is essential first to identify the types of biocontrol agents used and their underlying mechanisms. Some microbial resources discovered in tea plantations exhibit specific activity against pests and pathogens through unique mechanisms, highlighting their significant potential for development. Therefore, in addition to presenting MBCAs that are already widely commercialized in tea plantations, this review also introduces promising biocontrol agents with potential for future application. The MBCAs targeting major pests and pathogens in tea plantations are listed in Tables 1, 2, respectively.
2.1 Entomopathogenic microorganisms
Entomopathogenic microorganisms predominantly consist of bacteria, fungi, and viruses (Deka et al., 2022b). Bacterial entomopathogens primarily achieve insecticidal effects by secreting toxic proteins that inhibit pest growth (Pan et al., 2024). Research indicates that almost all insect-pathogenic bacteria isolated from tea pests belong to Bacillus thuringiensis (Bt) (Ye et al., 2014). The insecticidal mechanism of Bt involves the production of insecticidal crystal proteins (ICPs) within the sporangium during the sporulation phase, which exert stomach poisoning and contact toxicity effects on pests. These ICPs exhibit high specificity, becoming toxic only upon activation within specific host insects (Azizoglu et al., 2023). In the 1970s and 1980s, China employed Bt to manage Lepidopteran pests, achieving control efficacy rates of over 95% (Barthakur, 2011). With the continuous discovery of new strains, Bt has demonstrated effective control against other common tea plantation pests, such as tea thrips (Scirtothrips dorsalis) (Gurusubramanian et al., 2008), red slug caterpillar (Eterusia magnifica) (Mukhopadhyay et al., 2010), and tea termite (Microterms obesi) (Singha et al., 2010). Not only in tea plantations, but Bt has become the most widely used commercial bacterial insecticide worldwide (Bravo et al., 2011). Current research suggests that Bt’s effectiveness in tea plantation ecosystems could be further enhanced through the use of highly virulent strains (Banik et al., 2019), new delivery systems (Pan et al., 2024), or combined use with entomopathogenic fungi (Vimala Devi et al., 2020).
Apart from Bt, several other bacteria have shown potential in controlling tea plantation pests. Damayanti et al (De et al., 2008). isolated Enterobacter sp. from Caloptilia theivora, which exhibited an LC50 value of 363.1 μg/ml (bacterial weight/volume of water) against C.theivora larvae. Additionally, Wang et al (Ye et al., 2014). discovered that Serratia marcescens showed pathogenicity against two scale insects, Paralepidosaphes tubulorum Ferris and Chrysomphalus ficus (Roobak Kumar et al., 2011). Popy et al (Bora et al., 2023)found that Pseudomonas fluorescens and Bacillus velezensis exhibited lethal activity against the Oligonychus coffeae by secreting hydrolytic enzymes and secondary metabolites. However, most of these bacteria, aside from Bt, face significant challenges such as development difficulties, high costs, and unstable activity, which limit their extensive application in tea plantations.
Entomopathogenic fungi (EPF) thrive in ecosystems with humidity above 80%, making valuable for pest control in the hot, humid climates of tropical and subtropical tea plantations (Ye et al., 2014). The potential of Beauveria bassiana as a non-chemical pest control agent was first reported in 1837, leading to increased attention on EPF (Bhattacharyya et al., 2022). The main genera involved include Beauveria, Metarhizium, Paecilomyces, Hirsutella, and Nomuraea (Saito et al., 2012; Roy and Muraleedharan, 2014; Cheramgoi et al., 2016; Abdel-Raheem et al., 2020; Canassa et al., 2020). Notably, B.bassiana and Metarhizium anisopliae have been registered as biopesticides and are extensively employed for managing tea pests in regions including China, India, and Sri Lanka (Idris et al., 2020; McGuire and Northfield, 2020). Unlike bacteria, fungi infect insects primarily by spreading spores. The fungal hyphae penetrate the insect cuticle through mechanical pressure and the action of hydrolytic enzymes, thereby exerting a contact insecticidal effect (Wang et al., 2023; Shang et al., 2024). For example, B.bassiana has shown significant effectiveness in controlling various pests in tea plantations, including the tea looper (Buzura suppressaria) (Ghatak and Reza, 2007), false-eye leafhopper (Empoasca vitis) (Feng et al., 2004), tea weevil (Myllocerinus aurolineatus) (Pandey et al., 2021a), wood-eating tea termite (Microtermes obesi) (Singha et al., 2011), and tea mosquito bug (Helopeltis theivora) (Deka et al., 2021). Additionally, a study conducted in Japan demonstrated that a concentration of 1×107 conidia/ml of Paecilomyces cinnamomeus led to a 90% infection rate of whiteflies (Aleurocanthus camphalus), which was significantly higher than other fungal insecticides available on the market (Saito et al., 2012). The spore suspension of M.anisopliae can achieve a mortality rate of 78% in O.coffeae (Deka et al., 2022a). However, most entomopathogenic fungi have poor compatibility with chemical pesticides (Joshi et al., 2018), while rainfall can promote the extensive growth of these pathogens in insects.
Insect viruses are classified into six major categories: nuclear polyhedrosis viruses (NPV), granulosis viruses (GV), entomopoxviruses, iridoviruses, ascoviruses, and picornaviruses. As pathogenic natural enemies, these viruses exhibit a high degree of specificity towards their target pests (Sood et al., 2019). They infect hosts by penetrating the body, replicating and producing specific proteins that disrupt host cells, ultimately causing insect mortality (Payne, 1982).In the 1990s, Kagoshima Prefecture in Japan used a mixture of GV for the biological control of pests Homona magnanima and Adoxophyes sp., achieving pest mortality rates of 60-75% (Sato et al., 1986). To date, 82 viruses associated with tea plant insects have been identified and reported in China. Among these, the Ectropis obliqua nuclear polyhedrosis virus (EcobNPV) and Euproctis pseudoconspersa nuclear polyhedrosis virus (EpNPV) have been registered as biopesticides in China and commercialized (Ye et al., 2014). However, viral biopesticides are currently used sparingly in tea plantations, primarily due to the narrow host ranges. Moreover, the term “virus” may negatively impact consumer and farmer perceptions.
2.2 Pathogen antagonistic microorganisms
In tea plantations, where fungal pathogens are the primary cause of diseases, research has predominantly focused on fungal antagonistic microorganisms. Antagonistic microorganisms inhibit pathogenic growth through mechanisms such as competition, siderophore production, antibiotic secretion, and quorum sensing (Fenta et al., 2023), with their effects often being complex and multifaceted. Some plant growth-promoting rhizobacteria (PGPR) not only exhibit growth-promoting characteristics but also possess antibacterial, insecticidal, and plant resistance-inducing properties (Khoso et al., 2024). In this context, they are discussed as MBCAs. For instance, Serratia marcescens ETR17, isolated from the rhizosphere of tea plants, demonstrated in vitro antagonistic activity against nine tea plant pathogens (Dhar Purkayastha et al., 2018). This antagonism was primarily achieved through the secretion of various hydrolytic enzymes (chitinase, protease, lipase, cellulase) and antibiotics (pyrrolnitrin and prodigiosin). Additionally, ETR17 also produced the plant hormone indole-3-acetic acid (IAA) and siderophore. This suggests that MBCAs may simultaneously play a role in both biocontrol and improving the quality of tea.
Bacillus species are known for their rapid growth, production of various antimicrobial metabolites and enzymes, and widespread application in plant disease management. Yuan et al (He et al., 2024). isolated Bacillus velezensis CSUFT-BV4 from healthy oil tea (Camellia oleifera) leaves, which exhibited up to 73.2% inhibition against five pathogens causing tea oil anthracnose. Among the Actinobacteria members, Streptomyces are recognized for their abilities to produce antifungal, insecticidal, and growth-promoting metabolites (Borah et al., 2022; Das et al., 2024). Marimuthu et al (Marimuthu et al., 2020). identified Streptomyces sp. SLR03 from river soil samples, which demonstrated effective antagonistic activity against the tea pathogen Pestalotiopsis theae. Studies have demonstrated that internal control mechanisms are more effective than external applications, highlighting endophytes as a valuable source of biocontrol agents. Thoudam et al (Thoudam and Dutta, 2012). evaluated the efficacy of several epiphytic fungi on tea plants for controlling Corticium theae Bernard, which causes black rot. Their findings indicated that Aspergillus niger exhibited the most effective inhibition, followed by Trichoderma atroviride and Trichoderma cithnoviride.
Trichoderma species are considered among the most promising antagonistic fungi (Thambugala et al., 2020). Research has demonstrated that T.atroviride, T. asperellum, and T. harzianum effectively control the wilt pathogen Fusarium solani, with inhibition rates ranging from 64.6% to 71.7%. Additionally, these fungi significantly promote the development of new shoots in tea plants (Kumhar et al., 2020). Kolandasamy et al (Kolandasamy et al., 2023). reported that Bacillus subtilis VBS3 and Trichoderma viride VTV7, isolated from tea rhizospheres, effectively inhibited the mycelial growth and spore germination of Phomopsis theae by producing high levels of chitinase and β-1,3-glucanase. Shang et al (Shang et al., 2020). found that T. asperellum TC01 significantly reduced the severity of tea anthracnose caused by Colletotrichum gloeosporioides, with a reduction rate of 58.37%. Pandey et al (Pandey et al., 2022, 2023) observed that the strain Trichoderma reesei TRPATH01 exhibited antagonistic activities of 81.3% and 82.6% against the pathogens Pseudopestalotiopsis theae and F.solani, respectively on tea plants, through the production of inhibitory metabolites. Furthermore, Avascular Mycorrhizal Fungi (AMF) can also exhibit antagonistic activity. Rhizophagus intraradices BGC JX04B were able to reduce lesions caused by Colletotrichum camelliae in tea plants by 35.29% (Chen et al., 2022).
Fungal viruses have also shown potential in managing fungal diseases in tea plantations. For instance, Pestalotiopsis theae chrysovirus-1 (PtCV1), a fungal virus isolated from the tea pathogen P.theae, belongs to the family Chrysoviridae and the genus Alphachrysovirus. This virus significantly reduces the growth rate and virulence of its host fungus, effectively converting it into a non-pathogenic endophyte on tea leaves (Zhou et al., 2021). Furthermore, research indicates that plants can participate in the spread of fungal viruses (Hai et al., 2024). For example, after infection with Sclerotinia sclerotiorum, the concentration of proline in the plant significantly increases. This rise in proline weakens the fungus’s non-self-recognition response, thereby facilitating the spread of fungal viruses within the plant.
Despite the significant success of MBCAs in managing pests and diseases, their effectiveness still falls short compared to some chemical formulations. The efficacy of MBCAs in field conditions largely depends on factors such as their intrinsic activity, interactions with the natural microbiome, colonization ability within the ecosystem, and environmental adaptability (do Nascimento et al., 2022). Combining MBCAs with chemical treatments may offer effective pest and disease control while reducing the reliance on chemical agents (Jeyaraman and Robert, 2017). Additionally, the development of synthetic metal nanoparticles with biological activity represents an emerging and promising direction (Mythili Gnanamangai et al., 2017). However, it is essential to consider the broader ecological impacts of introducing exogenous microorganisms into tea plantations. Beyond their role in controlling pests and pathogens, studies have shown that some MBCAs can promote tea plant growth and enhance tea quality (Dhar Purkayastha et al., 2018; Bhattacharyya et al., 2020; Kumhar et al., 2020; Bora et al., 2022a; Pandey et al., 2022; He et al., 2024). Despite these findings, the underlying mechanisms remain largely unexplored. It is hypothesized that these effects may result from the complex interactions between the microorganisms and the tea plants. Therefore, further research is needed to elucidate the impact of MBCAs on the microecological dynamics of tea plantations and the metabolic processes of tea plants, ensuring a comprehensive understanding of their potential benefits and limitations.
3 Comprehensive impact of microbial biocontrol agents in tea plantations
3.1 Colonization efficacy of microbial biocontrol agents in tea plantations
Tea grower generally expect MBCAs to fulfill two key criteria: effective suppression of pathogens or pests and sustained survival in the environment. When conditions are favorable, MBCAs can be released as inoculants and remain in the microecosystem to prevent pest outbreaks or inhibit pathogen proliferation (McGuire and Northfield, 2020). One example is that MBCAs products containing Pseudomonas fluorescens in the U.S. often failed because these non-sporulating bacteria cannot survive long-term in natural environments (Choudhary and Johri, 2009). But studies have shown that sporulating Bt also exhibits poor colonization ability on plant leaves (Pedersen et al., 1995), likely due to its limited competitive ability in foliar environments (Maduell et al., 2008). The colonization of MBCAs on host plants is influenced by multiple factors, including inoculation methods, microbial species, crop types, growth conditions, and carriers (Bamisile et al., 2018), cannot be attributed to a single factor. Research on the colonization efficacy of microbial agents on tea plants is crucial for advancing microbial control strategies.
Nutritional supplements may aid in enhancing the survival of MBCAs under adverse conditions (Lin et al., 2023a). Certain strains adapted to tea plantation environments, such as Streptomyces sannanensis, are particularly suited for tea cultivation due to their ability to thrive in acidic soils (Gnanamangai and Ponmurugan, 2012). Furthermore, due to the saprophytic nature of Trichoderma harzianum, Gliocladium virens, P.fluorescens, and Trichoderma atroviride, they can occupy advantageous ecological niches in both soil and tea leaves, thereby altering the ecological functions of microbial communities (Sanjay et al., 2008; Manjukarunambika et al., 2013). These biocontrol agents also form biofilms and produce various cell wall-degrading enzymes upon colonizing host plants, thereby serving as effective barriers against pathogen invasion.
3.2 Impact on tea plant growth and tea quality
The ultimate goal of employing various management strategies in tea plantations is to enhance tea quality and mitigate adverse factors affecting. Key metabolic compounds in tea are critical indicators of quality. For instance, caffeine and catechins jointly influence the color and bitterness of tea. Non-protein amino acids, particularly theanine, are closely associated with the freshness and sweetness of tea. Volatile compounds such as linalool and cis-3-hexenol determine the aroma of tea (Zhang et al., 2020b). Stress from pests and pathogens, along with various exogenous factors, can affect the metabolic responses of tea plants, thereby altering the types and concentrations of metabolites in the tea leaves (Liao et al., 2019). Some MBCAs, in addition to their insecticidal and antimicrobial properties, have been shown to improve tea quality (Afridi et al., 2024). For example, growth-promoting effects associated with endophytic colonization by B.bassiana (Sánchez-Rodríguez et al., 2018) and Metarhizium species (Ahmad et al., 2020) have been observed in various plants. The growth-promoting mechanisms of MBCAs encompass the synthesis of phytohormones and siderophore, phosphate solubilization, potassium release, and nitrogen fixation (Barelli et al., 2020).
In tea plants, the antagonistic microorganism Streptomyces sannanensis has been shown to significantly improve both tea yield and quality parameters (Gnanamangai and Ponmurugan, 2012). Similarly, Ochrobactrum anthropi BMO‐111 has been reported to significantly increase the levels of chlorophyll, polyphenols, and catechins in tea buds. However, the observed increase in these indicators may be a result of the plant’s recovery from pathogen infection (Sowndhararajan et al., 2013). In another study, the rhizosphere strain O. anthropi TRS-2 demonstrated phosphate solubilization, iron carrier production, and IAA synthesis in vitro, confirming its plant growth-promoting mechanisms (Chakraborty et al., 2009). Huang et al (Huang et al., 2022). found that foliar application of Bacillus amyloliquefaciens to tea plants resulted in a decrease in the ratio of tea polyphenols to amino acids (TP/AA), catechin, and caffeine content, while the theanine content increased, with catechin reduction being associated with the biosynthesis pathway of flavonoids. Moreover, treatments with T.asperellum TC01 (Shang et al., 2020) and T.reesei TRPATH01 (Pandey et al., 2023) significantly enhanced parameters such as shoot height, stem diameter, weight of buds and roots in tea plants. These studies indicate that exogenous MBCAs may have a positive impact on tea quality. However, is this impact always beneficial? Although no direct reports have been found indicating adverse effects of MBCAs on tea plant growth, similar outcomes have been observed in other crops. For example, Pseudomonas chlororaphis IDV1 and Pseudomonas putida RA2, which antagonize the tomato pathogen Ralstonia solanacearum, exhibited good survival in maize rhizospheres but caused slight inhibition of maize growth (Kozdrój et al., 2004). Whether such effects arise from changes in the plant’s microecological structure, direct interference with plant metabolic processes, or other biological factors remains unclear.
3.3 Effects on non-target organisms and safety evaluation
As biological control agent use expands, it is essential to assess their potential impacts on non-target organisms in tea plantations. Non-target organisms, including non-tea plants, soil microbes, and wildlife. These organisms play significant roles within ecosystems, and disturbances to them could lead to ecological imbalances. Research has identified harmful effects of certain biocontrol agents on non-target species. For example, B.bassiana has been shown to have detrimental effects on some natural enemies (Li et al., 2024), while Bt exhibits toxicity to bees (Borges et al., 2021) and silkworms (Sandeep Kumar et al., 2016), which limits its application in sericulture countries such as India (Dashora et al., 2017). However, Bt proteins, particularly the ICPs, are highly specific and fully biodegradable, with no observed risk of toxic accumulation in the environment (Brar et al., 2007). Additionally, studies have indicated that injecting Bt crystal proteins into mice does not produce toxic effects (Román Calderón et al., 2007). Nevertheless, another study found that Bt inoculation might inhibit the root colonization of AMF by releasing suppressive compounds (Ferreira et al., 2003).
Plant-derived insecticides such as sophoridine and neem extract have not shown significant impacts on the populations of ladybugs, spiders, or parasitic wasps in tea plantations (Tian et al., 2020). However, neem extract has demonstrated adverse effects on the rhizosphere microbial communities of leguminous plants, resembling the effects of chemical pesticides (Singh et al., 2015a, 2015b). Notably, research has shown that bacterial inoculants can mitigate such negative impacts (Singh et al., 2022). Additionally, some Gram-negative bacteria used in biological control, such as O. anthropi strains, have potential as human pathogens. However, in murine models, the pathogenicity of O. anthropi strain BMO-111 was not observed, even with high inoculum concentrations (0.5 ml of 1 × 107 CFU ml−1) orally administered to mice (Sowndhararajan et al., 2013).
4 Impact of microbial biocontrol methods on tea plant microbial communities
Microbial communities associated with tea plants can be broadly categorized into three main types: phyllosphere, rhizosphere, and endophytic microorganisms. Each of these groups plays a unique role in the plant’s microecology and overall health. Under-standing their characteristics and interactions is crucial for effectively applying microbial biocontrol methods to improve tea plant resilience and productivity.
4.1 Phyllosphere microorganisms
Phyllosphere microorganisms refer to the microbial communities associated with the aerial parts of plants (Xu et al., 2022a). The phyllosphere functions as an open system, making it susceptible to external factors such as ultraviolet radiation, air pollution, and microbial inoculation. Plant genotypes play a key role, shaping tissue structure and secondary metabolites (Ohler et al., 2022). In tea plants, the phyllosphere microbial community predominantly consists of Proteobacteria, Actinobacteria, Bacteroidetes, and Firmicutes at the phylum level. At the genus level, the core microbial taxa include bacteria such as Herbaspirillum, Massilia, Methylobacterium, Pantoea, Pseudomonas, and Sphingomonas. The diversity of phyllosphere fungi is generally lower than that of bacteria. These fungi can inhabit the phyllosphere in either epiphytic or endophytic forms, with predominant taxa including Basidiomycota, Ascomycota, and Mortierella (Xu et al., 2023b). Fungi and bacteria in the phyllosphere can collaborate in the metabolic processes of tea plants, with this interaction being more pronounced in young leaves (Lin et al., 2022).
The assembly of phyllosphere microbial communities and their interactions with host metabolites are crucial for the health of tea plants. Xu et al (Xu et al., 2022b). demonstrated that the phyllosphere microbiota varies with the metabolic products of tea leaves at different developmental stages, although a stable core microbial community exhibits antagonistic effects against various pathogens (Xu et al., 2022b). Microorganisms such as Sphingomonas, Herbaspirillum, and Massilia can migrate from the soil to the leaves and maintain phyllosphere homeostasis (Xu et al., 2023b). Additionally, Bt is also a common component of many plant phyllosphere microbiota, where it can interact with and kill herbivorous insect larvae (Uribe et al., 2003; Collier et al., 2005). Key drivers of microbial community assembly in tea plants include metabolites like caffeine and epigallocatechin gallate (Xu et al., 2022b), suggesting that tea plants may recruit beneficial microorganisms by secreting specific metabolites under biotic stress. Similarly, Xie et al (Xie et al., 2022). found that tea plants influence rhizosphere bacterial diversity, community structure, and nitrogen cycling-related gene abundance through the secretion of L-theanine into the rhizosphere. This indicates the presence of complex interactions among insects, microorganisms, and tea plants.
The use of chemical agents in agriculture can disrupt phyllosphere microbiota (Chen et al., 2021). For example, a study on wheat found that while the herbicide S-metolachlor did not significantly affect plant physiology, it reduced the diversity of the phyllosphere microbiota, indicating that microbial communities are sensitive indicators of short-term plant stress (Xu et al., 2020). MBCAs have shown significant impacts on the microbiota of various crops (Zhang et al., 2008; Sylla et al., 2013; Perazzolli et al., 2014; Wang et al., 2014; Qin et al., 2019; Hao et al., 2021), though some studies have reached the opposite conclusion (Wei et al., 2016). For instance, Bt does not significantly alter the bacterial community on the leaves of cabbage (Brassica oleracea) (Russell et al., 1999) or the phyllosphere bacterial community of Oryza sativa (Wang et al., 2014), likely due to Bt’s weak competitive ability (Maduell et al., 2008). Evidence also suggests that combined microbial inoculants have a more pronounced effect on microbial community structure compared to single-agent applications (Xu and Jeger, 2013), and repeated application of MBCAs may increase opportunities for establishing active populations (Wei et al., 2016). However, microbial combinations can lead to competitive or antagonistic relationships, necessitating careful consideration. While research on MBCAs in tea plant phyllosphere microbiota is limited, studies on other crops indicate that MBCAs may influence tea plants. Further research is needed to understand the specific mechanisms by which MBCAs affect tea plant phyllosphere microbiota.
4.2 Rhizosphere microorganisms
In the rhizosphere of tea plants, AMF and various other microorganisms play key roles in processes such as phosphate solubilization, nitrogen fixation, iron chelation, stress tolerance, and auxin production (Chen et al., 2023). In tea plantations, soil fungi predominantly belong to Ascomycota, Mortierellomycota, and Basidiomycota, while the dominant bacterial phyla are Acidobacteria, Actinobacteria, and Proteobacteria (Jibola-Shittu et al., 2024). Research suggests that more complex soil ecological networks may contribute to the suppression of tobacco wilt disease (Yang et al., 2017). Additionally, rhizosphere bacteria from tea plantations, such as Burkholderia sp. AULS-B3, has been found to both metabolize the pesticide endosulfan and promote tea plant growth (Huidrom and Sharma, 2024).
The stability of tea plant rhizosphere microbial communities is shaped by agronomic practices (Zou et al., 2022; Zhang et al., 2023). Lin et al (Tan et al., 2019). observed that organic tea plantations (OTP) exhibit significantly higher alpha diversity and Chao1 indices than conventional tea plantations (CTP) (Sang and Kim, 2012). Exogenous microbial applications can also influence native rhizosphere microbiome, though the effects may be ambiguous. For instance, lower concentrations of Beauveria bassiana spore suspensions improved paddy soil yields by affecting microbial community structure and enzyme activity (Du et al., 2013). In soybeans (Glycine max), Bt did not alter cultivable heterotrophic bacteria or saprophytic fungi in the rhizosphere (Ferreira et al., 2003). Similarly, applying Pseudomonas fluorescens DR54 to barley (Hordeum vulgare) temporarily shifted the rhizosphere microbiome (Johansen and Olsson, 2005) due to competition with native microbes, causing DR54 decline. This phenomenon explains why most MBCAs rapidly decline in number after soil introduction, and microbial communities tend to recover quickly.
The relationship between rhizosphere microorganisms and their host plants is highly intricate. For instance, the soil-dwelling insect-pathogenic fungus Metarhizium robertsii possesses two adhesin genes: MAD1, which facilitates attachment to the insect cuticle, and MAD2, which aids in adhesion to plants, highlighting its association with plant hosts (Sasan and Bidochka, 2012). Similar to the phyllosphere, plant roots release signaling molecules into the soil under stress, recruiting beneficial microorganisms to alleviate various environmental pressures. These signaling molecules are likely plant hormones or other secondary metabolites. For example, research by Berendsen et al (Berendsen et al., 2018). showed that plants can attract beneficial microorganisms by regulating hormone levels when infected by pathogens. Studies suggest that pathogen-infected roots secrete more amino acids, nucleic acids, and long-chain organic acids, which alter the root microbiome and enhance disease resistance (Yuan et al., 2018). The application of MBCAs can also change plant hormone levels, indicating that these microbial shifts are the result of multiple interacting factors, often regulated by the plant itself. This underscores the mutualistic relationship between plants and microorganisms, suggesting that selecting beneficial MBCAs could promote an ideal symbiosis.
4.3 Endophytic microorganisms
Endophytic microorganisms, which reside within plant tissues, have a particularly close relationship with their host plants (Wang and Cernava, 2020). Research highlights their critical role in promoting plant growth and health (Bastias et al., 2017). For instance, endophytic bacteria can enhance plant resistance to herbivorous insects through jasmonic acid (JA)-mediated defense pathways (Bastias et al., 2017). Most isolated endophytes, primarily from the Bacillus genus, exhibit plant growth-promoting abilities. However, the evaluation of MBCAs often neglects the impact on endophytes (Kabir et al., 2023). This omission may be due to the cryptic endophytic environment, the diversity and heterogeneous distribution of endophytes, which pose significant research challenges. Because endophytes are frequently grouped together with phyllosphere microorganisms in studies. While this simplifies experimental design, it overlooks the unique functions of endophytes. Future research should emphasize the interactions between tea plant microbiota and MBCAs, particularly in diverse ecological systems and management practices. Understanding how these interactions alter microbial communities is essential for developing beneficial microecologies that enhance tea plant resistance and quality.
5 Insects, microorganisms, and tea plant metabolism
The intricate interplay between insects, microorganisms, and tea plant metabolism significantly influences the overall health and productivity of tea plants. Understanding tea plant metabolic responses to pest and disease stress is crucial for developing effective management strategies. Additionally, MBCAs not only control pests and diseases but also influence tea plant metabolism, enhancing resilience and quality. This section examines into the response mechanisms of tea plant metabolism under stress and explores the role of MBCAs in mediating these metabolic processes (Figure 2).
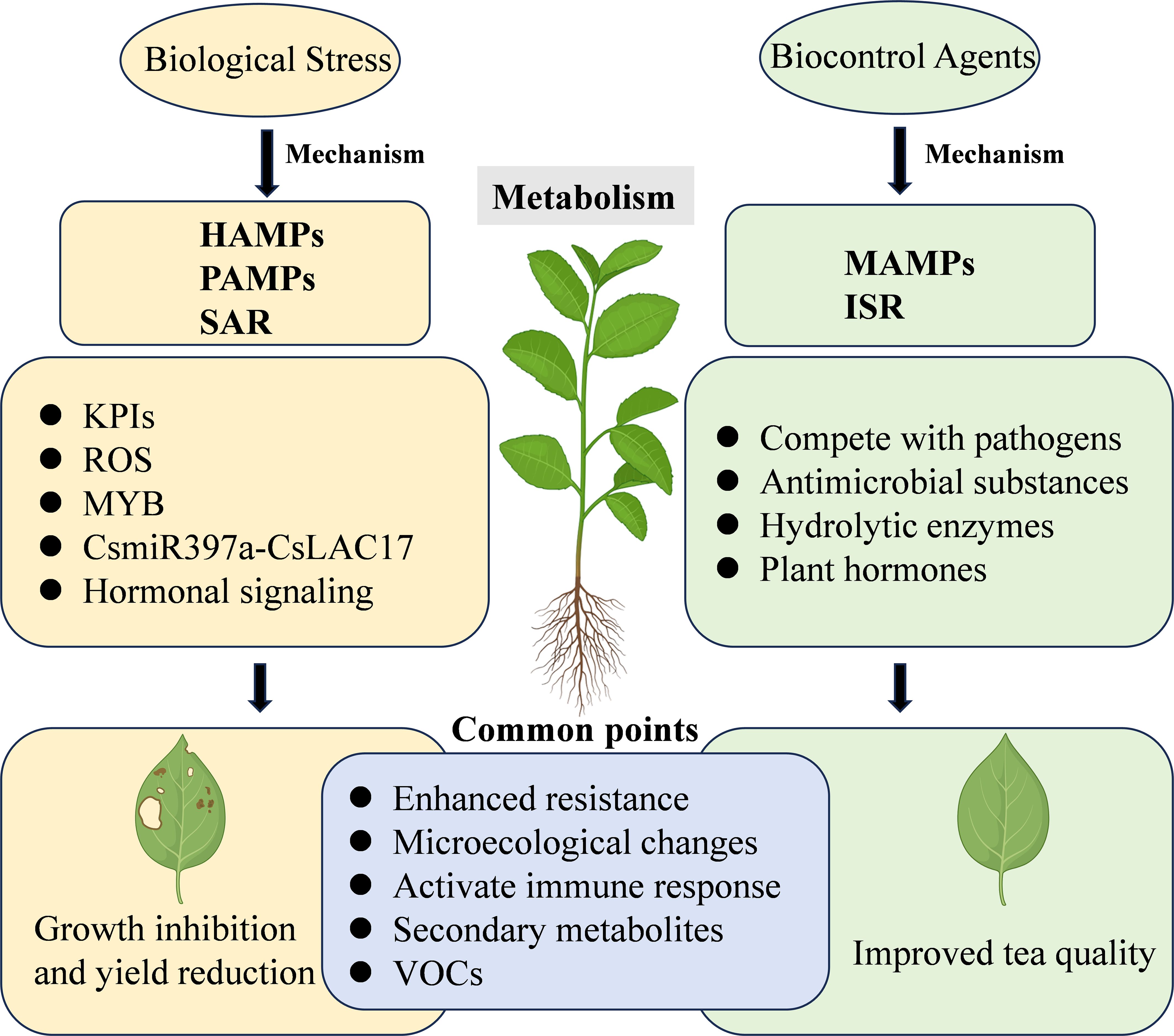
Figure 2. The respective impacts of MBCAs and biological stress factors on the metabolic mechanisms of tea plants. HAMPs, herbivore-associated molecular patterns; PAMPs, pathogen-associated molecular patterns; MAMPs, microbe-associated molecular patterns; SAR, systemic acquired resistance; ISR, induced systemic resistance; KPIs, proteinase inhibitors; ROS, reactive oxygen species; MYB, MYB transcription factors; VOCs, volatile organic compounds.
5.1 Response mechanisms of tea plant metabolism under pest and disease stress
Tea plants have evolved complex metabolic pathways and defense mechanisms. Pathogen attacks trigger systemic acquired resistance (SAR) in uninfected tissues, providing broad-spectrum resistance (Jeyaraj et al., 2017; Hilleary and Gilroy, 2018; Naskar et al., 2021; Zhang et al., 2024). Local responses triggered by herbivore-associated molecular patterns (HAMPs) and pathogen-associated molecular patterns (PAMPs) are rapid and intense, while systemic responses involve secondary metabolites, proteinase inhibitors (KPIs), reactive oxygen species (ROS), and plant hormones to combat further assaults (Fu and Dong, 2013; Naskar et al., 2021; Jomova et al., 2024). Under stress from pests and pathogens, tea plants also exhibit significant changes in primary metabolic pathways. Primary metabolites such as carbohydrates, amino acids, and organic acids are not only fundamental to tea plant growth and development but also play crucial roles in its defense mechanisms (Zhou et al., 2015).
For example, tea looper (Ectropis obliqua) feeding induces systemic carbon and nitrogen redistribution to bolster secondary metabolism for defense (Huang et al., 2018; Yang et al., 2019; Li et al., 2020). During this process, L-theanine is primarily synthesized by CsTSI in the roots and transported through the vascular system to new tea shoots (Lin et al., 2023b), while caffeine (1,3,7-trimethylxanthine) is predominantly synthesized in chloroplasts (Xia et al., 2017; Liao et al., 2022). This coordinated metabolic reorganization helps tea plants maintain a balance between growth and defense when facing external pressures. RNA sequencing (RNA-Seq) analysis has revealed 1,859 differentially expressed genes (949 upregulated and 910 downregulated) in tea plants subjected to E. oblique infestation compared to controls. These genes are involved in signal transduction, insect defense response transcription factors, phenylpropanoid pathways, herbivory-induced plant volatiles (HIPVs), and caffeine biosynthesis (Wang et al., 2016b). Similarly, Exobasidium vexans infection revealed 149 defense-related genes in resistant tea genotypes, including defense-related enzymes, resistance genes, multidrug-resistant transporters, transcription factors, retrotransposons, metacaspases, and chaperones (Jayaswall et al., 2016). Beyond local responses, tea plants also activate defense-related genes in adjacent leaves through signaling mechanisms (Zhou et al., 2020).
Plant hormones play a crucial regulatory role in the defense responses of tea plants (Liao et al., 2019, 2022; Yang et al., 2019). Jasmonic acid (JA) and salicylic acid (SA) are key signaling molecules that activate defense pathways (Zhang et al., 2020a; Hou and Tsuda, 2022), JA is primarily involved in tea plant responses to folivorous insects, while SA predominantly mediates immune responses to microbial pathogens (Zhu et al., 2019; Hu et al., 2022; Sultana et al., 2024). Studies have shown that JA and SA play important roles in shaping the root microbiome (Lebeis et al., 2015). Additionally, other plant hormones such as ethylene (ET), abscisic acid (ABA), auxin (indole-3-acetic acid, IAA), cytokinins (CTK), and gibberellins (GA) are required to ensure proper coordination between growth and defense (Bigeard et al., 2015; Ye et al., 2021). Although JA and SA are generally considered antagonistic (Jiao et al., 2022), studies have shown that they can also act synergistically (Liu et al., 2016). For instance, attacks by both piercing-sucking insects like the tea green leafhopper (Empoasca onukii) and chewing insects like tea looper (Ectropis grisescens) lead to increased levels of both JA and SA (Jin et al., 2020). Notably, E. onukii attack also raises ABA levels, which may relate to the insect’s mode of injury (Liao et al., 2019). Conversely, simulated attacks by piercing-sucking insects alone elevate JA and ABA levels in tea leaves, with no significant change in SA levels. This raises the question of whether SA variation might be related to insect salivary effectors or microbial interactions (Xu et al., 2019; Smets and Koskella, 2020). Furthermore, Zhao et al (Zhao et al., 2020b). found that invasion by E. onukii upregulated genes related to the biosynthesis of phenylpropanoids and flavonoids and induced the synthesis of cuticular waxes, leading to increased levels of C29 alkanes. Tea plants infected with Pseudopestalotiopsis camelliae-sinensis may enhance lignin content in young tea shoots through the CsmiR397a-CsLAC17 module, thereby reducing stem tenderness and increasing resistance to tea leaf spot disease (Yang et al., 2024). The dynamic adjustment of these metabolic pathways not only enhances tea plant survival but also impacts the flavor and aroma of the tea.
As previously discussed, characteristic metabolites in tea plants, such as terpenoids, phenolic compounds (flavonoids, anthocyanins, lignins, and tannins), and nitrogen-containing compounds (such as alkaloids and non-protein amino acids), not only protect the tea plant but also determine the overall flavor and health benefits of tea (Hilleary and Gilroy, 2018; Yu and Yang, 2020; Zhao et al., 2020a, 2023b; Zeng et al., 2021; Li et al., 2022). It has been demonstrated that catechins and caffeine can inhibit pathogen growth in vitro (Bansal et al., 2012). For instance, infestations by E.oblique and Colletotrichum fructicola increase the biosynthesis of flavonoids and caffeine in tea leaves, accompanied by elevated expression of related synthesis genes (Wang et al., 2016a). Interestingly, certain Pseudomonas species in the gut microbiota of the coffee borer beetle (Hypothenemus hampei) use caffeine as their sole carbon and nitrogen source (Ceja-Navarro et al., 2015), suggesting that some insects may have evolved adaptive detoxification systems for caffeine, which could be relevant for research on tea plants as well. Additionally, changes in metabolite levels evidently influence microbial communities. For example, after infection with E.vexans, the dominant endophytic fungal community in tea plants shifted from Ascomycota to Basidiomycota, while the relative abundance of Actinobacteria in bacteria increased (Cao et al., 2023).
Tea plant volatile organic compounds (VOCs) play a dual role in both aromatic and defensive functions (Zeng et al., 2019b). They can repel pests (Jing et al., 2021), attract natural enemies (Zeng et al., 2019b), and trigger immune responses in neighboring plants (Bouwmeester et al., 2019). For instance, after feeding by E.oblique, tea plants release compounds such as S-linalool and β-ocimene, which significantly attract the parasitic wasp Parapanteles hyposidrae (Liu et al., 2024a). Due to the aromatic properties of VOCs, the impact of pest infestations on tea quality is not always negative. For example, feeding by the green leafhopper (Jacobiasca formosana) increases the release of (S)-linalool and geraniol, and induces the production of diene alcohol I. This process contributes to the Oriental Beauty Oolong tea, which is known for its distinctive aroma (Cho et al., 2007).
Overall, tea plants exhibit a sophisticated metabolic regulatory system in response to pest and pathogen pressures. These mechanisms encompass not only the accumulation of plant hormones and secondary metabolites but also the regulation of carbon and nitrogen metabolism, the generation and scavenging of reactive oxygen species, the release of volatile organic compounds, and systemic defense responses in adjacent leaves. These insights offer new possibilities for pest and disease management in tea cultivation. For example, exogenous application of JA and SA can enhance both the quantity and quality of volatile compounds (Chen et al., 2020). Additionally, inducing tea plant disease resistance genes through external agents may improve the plant’s disease resistance (Senthilkumar et al., 2012; Lu et al., 2019). Future research could further elucidate the specific regulatory mechanisms of these metabolic pathways, potentially providing novel strategies for genetic improvement and integrated pest and disease management in tea cultivation.
5.2 Microbial biocontrol agents mediating tea plant metabolism
In tea plants, inoculation with weak pathogens or non-pathogenic microbial agents can trigger an enhanced resistance state known as induced systemic resistance (ISR) (Pieterse et al., 2014). Typically, plant immune responses are triggered by microbe-associated molecular patterns (MAMPs), leading to microbe-associated molecular pattern-triggered immunity (MTI). MTI functions by recognizing pathogen-associated molecular patterns and activating defense signaling pathways. However, this defense response often leads to a reallocation of resources, consequently inhibiting plant growth (Shang et al., 2020; Ma et al., 2021). Unlike MTI, ISR regulates the expression of defense genes through the jasmonic acid (JA) and ethylene signaling pathways, promoting the accumulation of defense metabolites and protecting distal tissues. While both MTI and ISR enhance disease resistance, ISR also has the potential to promote plant growth, providing additional growth advantages alongside conventional immune defenses via MTI (Kusajima et al., 2018; Yu et al., 2022). However, this advantage may come with potential trade-offs due to differences in resource allocation.
In studies conducted in India, the application of Pseudomonas fluorescens and Pseudomonas aeruginosa strains RRLJ 134 and RRLJ 04, respectively, has been shown to activate systemic resistance in tea plants against Fomes lamoensis and Ustulina zonata (Mishra et al., 2014). These treatments, along with antagonistic microorganisms Bacillus altitudinis GS-16 and T. reesei TRPATH01, significantly enhanced key defense enzyme activities in tea plants (Pandey et al., 2022; Wu et al., 2024b). Additionally, antagonistic fungi Trichoderma asperellum TC01 treatment activated the expression of genes associated with flavonoids, phenylpropanoids, jasmonic acid, and ethylene (Shang et al., 2020). Treatment with the AMF Rhizophagus intraradices BGC JX04B resulted in a significant increase in superoxide anion levels, as well as the activities of catalase and peroxidase in young tea seedlings. This response is likely mediated through pathways involved in plant hormone signaling, mitogen-activated protein kinase (MAPK) signaling, and phenylpropanoid biosynthesis (Chen et al., 2022).
In other crops, a method analogous to the use of “inactivated vaccines” in medicine involves the application of fungal pathogen Stemphylium lycopersici mycelial suspensions, which have been attenuated by infection with Stemphylium lycopersici Alternavirus 1 (SlAV1), to enhance plant resistance against virulent strains and convert pathogenic fungi into biocontrol agents (Liu et al., 2022). Overall, MBCAs have a significant impact on the metabolism of tea plants, with some changes in metabolic pathways resembling the plant’s response to pathogen and pest stress. As shown in Table 3, both stress and the application of MBCAs can enhance the resistance of tea plants.
6 Conclusions and future perspectives
This review primarily summarizes the potential of microbial biocontrol agents (MBCAs) in enhancing both the management of tea tree diseases and the quality of tea leaves. The use of MBCAs represents a shift from merely targeting and killing pests or pathogens to protecting tea trees and improving their overall health. Integrating MBCAs into tea plantation management practices can bolster the natural defenses of tea trees and improve the overall quality of tea by fostering beneficial microbial communities. This approach also provides a valuable model for the application of microbial biocontrol agents in other perennial crop systems. However, existing research faces challenges such as limited effectiveness, stability issues, high costs, and gaps in understanding the molecular mechanisms of MBCAs. Future research directions should focus on:
1. Development and Application of Novel Microbial Biocontrol Agents: Future studies should aim to discover and develop more effective MBCAs, especially those targeting specific diseases or pests affecting tea trees. Additionally, research should explore the integration of MBCAs with tea plantation management practices such as fertilization, irrigation, and crop rotation. Optimizing these integrated management strategies could lead to a comprehensive microbial biocontrol approach for tea plantation management.
2. Mechanisms of MBCAs on Tea Quality: Understanding how MBCAs improve tea quality is essential. Research should focus on how these agents influence the metabolic pathways of tea trees, especially in terms of nutrient uptake, stress responses, and the production of secondary metabolites that contribute to tea flavor and aroma. Investigating how MBCAs improve tea quality by modulating the metabolic pathways of tea trees and promoting the establishment of beneficial microbial communities is essential. Understanding these mechanisms will help establish the relationship between tea tree microecology and tea quality, providing a scientific basis for optimizing ecological pest control in tea plantations and enhancing tea quality.
3. Addressing Limitations and Enhancing Efficiency of MBCAs: Current challenges with MBCAs include issues related to their stability, limited range of effectiveness, and high costs. Future research could focus on developing new carriers to maintain microbial activity, combining MBCAs with other control methods to enhance efficacy, and optimizing fermentation processes to reduce production and application costs. These efforts aim to make the use of MBCAs in tea plantation management more economically viable and effective.
Author contributions
YX: Methodology, Writing – original draft. CC: Funding acquisition, Methodology, Project administration, Writing – review & editing. DH: Software, Visualization, Writing – review & editing. YG: Conceptualization, Funding acquisition, Methodology, Resources, Supervision, Writing – review & editing. BW: Conceptualization, Funding acquisition, Methodology, Writing – original draft.
Funding
The author(s) declare that financial support was received for the research, authorship, and/or publication of this article. This research was funded by the National Natural Science Foundation of China (32302449), and Science and Youth Science Fund Project of Hubei Academy of Agricultural Sciences (2023NKYJJ27), Hubei Provincial Key Research and Development Project (2023BBB148).
Conflict of interest
The authors declare that the research was conducted in the absence of any commercial or financial relationships that could be construed as a potential conflict of interest.
Publisher’s note
All claims expressed in this article are solely those of the authors and do not necessarily represent those of their affiliated organizations, or those of the publisher, the editors and the reviewers. Any product that may be evaluated in this article, or claim that may be made by its manufacturer, is not guaranteed or endorsed by the publisher.
References
Abdel-Raheem, M., Youssif, M., Helaly, S. (2020). Use of Verticillium lecanii and Beauveria bassiana against Tomato leaf miner, Tuta absoluta (Meyrick) and Bemisia tabaci (Genn.) in Tomato Crop. Plant Arch. 20, 479–482.
Afridi, M. S., Kumar, A., Javed, M. A., Dubey, A., de Medeiros, F. H. V., Santoyo, G. (2024). Harnessing root exudates for plant microbiome engineering and stress resistance in plants. Microbiol. Res. 279, 127564. doi: 10.1016/j.micres.2023.127564
Ahirwar, N. K., Singh, R., Chaurasia, S., Chandra, R., Ramana, S. (2020). Effective role of beneficial microbes in achieving the sustainable agriculture and eco-friendly environment development goals: a review. Front. Microbiol. 5, 111–123. doi: 10.11648/j.fem.20190506.12
Ahmad, I., Jiménez-Gasco, M., del, M., Luthe, D. S., Shakeel, S. N., Barbercheck, M. E. (2020). Endophytic Metarhizium robertsii promotes maize growth, suppresses insect growth, and alters plant defense gene expression. Biol. Control 144, 104167. doi: 10.1016/j.biocontrol.2019.104167
Azizoglu, U., Salehi Jouzani, G., Sansinenea, E., Sanchis-Borja, V. (2023). Biotechnological advances in Bacillus thuringiensis and its toxins: Recent updates. Rev. Environ. Sci. Biotechnol. 22, 319–348. doi: 10.1007/s11157-023-09652-5
Bag, S., Mondal, A., Banik, A. (2022a). Exploring tea (Camellia sinensis) microbiome: Insights into the functional characteristics and their impact on tea growth promotion. Microbiological Res. 254, 126890. doi: 10.1016/j.micres.2021.126890
Bag, S., Mondal, A., Majumder, A., Banik, A. (2022b). Tea and its phytochemicals: Hidden health benefits & modulation of signaling cascade by phytochemicals. Food Chem. 371, 131098. doi: 10.1016/j.foodchem.2021.131098
Bamisile, B. S., Dash, C. K., Akutse, K. S., Keppanan, R., Afolabi, O. G., Hussain, M., et al. (2018). Prospects of endophytic fungal entomopathogens as biocontrol and plant growth promoting agents: An insight on how artificial inoculation methods affect endophytic colonization of host plants. Microbiol. Res. 217, 34–50. doi: 10.1016/j.micres.2018.08.016
Banik, A., Chattopadhyay, A., Ganguly, S., Mukhopadhyay, S. K. (2019). Characterization of a tea pest specific Bacillus thuringiensis and identification of its toxin by MALDI-TOF mass spectrometry. Ind. Crops Products 137, 549–556. doi: 10.1016/j.indcrop.2019.05.051
Bansal, S., Syan, N., Mathur, P., Choudhary, S. (2012). Pharmacological profile of green tea and its polyphenols: a review. Med. Chem. Res. 21, 3347–3360. doi: 10.1007/s00044-011-9800-4
Barelli, L., Waller, A. S., Behie, S. W., Bidochka, M. J. (2020). Plant microbiome analysis after Metarhizium amendment reveals increases in abundance of plant growth-promoting organisms and maintenance of disease-suppressive soil. PloS One 15, e0231150. doi: 10.1371/journal.pone.0231150
Barthakur, B. K. (2011). Recent approach of tocklai to plant protection in tea in Northeast India. Sci. Cult. 77, 381–384.
Bastias, D. A., Martínez-Ghersa, M. A., Ballaré, C. L., Gundel, P. E. (2017). Epichloë Fungal endophytes and plant defenses: not just alkaloids. Trends Plant Sci. 22, 939–948. doi: 10.1016/j.tplants.2017.08.005
Berendsen, R. L., Vismans, G., Yu, K., Song, Y., de Jonge, R., Burgman, W. P., et al. (2018). Disease-induced assemblage of a plant-beneficial bacterial consortium. ISME J. 12, 1496–1507. doi: 10.1038/s41396-018-0093-1
Berg, G., Smalla, K. (2009). Plant species and soil type cooperatively shape the structure and function of microbial communities in the rhizosphere. FEMS Microbiol. Ecol. 68, 1–13. doi: 10.1111/j.1574-6941.2009.00654.x
Bermúdez, S., Voora, V., Larrea, C., Luna, E. (2024). Global Market Report: Tea prices and sustainability. Available online at: https://www.iisd.org/publications/report/2024-global-market-report-tea (Accessed April 9, 2024).
Bhattacharyya, C., Banerjee, S., Acharya, U., Mitra, A., Mallick, I., Haldar, A., et al. (2020). Evaluation of plant growth promotion properties and induction of antioxidative defense mechanism by tea rhizobacteria of Darjeeling, India. Sci. Rep. 10, 15536. doi: 10.1038/s41598-020-72439-z
Bhattacharyya, P. N., Sarmah, S. R., Roy, S., Sarma, B., Nath, B. C., Bhattacharyya, L. H. (2022). Perspectives of Beauveria bassiana, an entomopathogenic fungus for the control of insect-pests in tea [Camellia sinensis (L.) O. Kuntze]: opportunities and challenges. Int. J. Trop. Insect Sci. 43, 1–19. doi: 10.1007/s42690-022-00932-1
Bigeard, J., Colcombet, J., Hirt, H. (2015). Signaling mechanisms in pattern-triggered immunity (PTI). Mol. Plant 8, 521–539. doi: 10.1016/j.molp.2014.12.022
Bora, P., Chandra Bora, L., Bhuyan, R. P., Hashem, A., Fathi Abd-Allah, E. (2022a). Bioagent consortia assisted suppression in grey blight disease with enhanced leaf nutrients and biochemical properties of tea (Camellia sinensis). Biol. Control 170, 104907. doi: 10.1016/j.biocontrol.2022.104907
Bora, P., Gogoi, S., Deshpande, M. V., Garg, P., Bhuyan, R. P., Altaf, N., et al. (2023). Rhizospheric Bacillus spp. Exhibit Miticidal Efficacy against Oligonychus coffeae (Acari: Tetranychidae) of Tea. Microorganisms 11, 2691. doi: 10.3390/microorganisms11112691
Bora, S. S., Hazarika, D. J., Gogoi, R., Dullah, S., Gogoi, M., Barooah, M. (2022b). Long-term pruning modulates microbial community structure and their functional potential in Tea (Camellia sinensis L.) soils. Appl. Soil Ecol. 176, 104483. doi: 10.1016/j.apsoil.2022.104483
Borah, A., Hazarika, S. N., Thakur, D. (2022). Potentiality of actinobacteria to combat against biotic and abiotic stresses in tea [Camellia sinensis (L) O. Kuntze. J. Appl. Microbiol. 133, 2314–2330. doi: 10.1111/jam.15734
Borges, S., Alkassab, A. T., Collison, E., Hinarejos, S., Jones, B., McVey, E., et al. (2021). Overview of the testing and assessment of effects of microbial pesticides on bees: strengths, challenges and perspectives. Apidologie 52, 1256–1277. doi: 10.1007/s13592-021-00900-7
Bouwmeester, H., Schuurink, R. C., Bleeker, P. M., Schiestl, F. (2019). The role of volatiles in plant communication. Plant J. 100, 892–907. doi: 10.1111/tpj.14496
Brar, S. K., Verma, M., Tyagi, R. D., Surampalli, R. Y., Barnabé, S., Valéro, J. R. (2007). Bacillus thuringiensis proteases: Production and role in growth, sporulation and synergism. Process Biochem. 42, 773–790. doi: 10.1016/j.procbio.2007.01.015
Bravo, A., Likitvivatanavong, S., Gill, S. S., Soberón, M. (2011). Bacillus thuringiensis: A story of a successful bioinsecticide. Insect Biochem. Mol. Biol. 41, 423–431. doi: 10.1016/j.ibmb.2011.02.006
Canassa, F., Esteca, F. C. N., Moral, R. A., Meyling, N. V., Klingen, I., Delalibera, I. (2020). Root inoculation of strawberry with the entomopathogenic fungi Metarhizium robertsii and Beauveria bassiana reduces incidence of the twospotted spider mite and selected insect pests and plant diseases in the field. J. Pest Sci. 93, 261–274. doi: 10.1007/s10340-019-01147-z
Cao, R., Dong, X., Zhao, Y., Yin, J. (2023). Effects of blister blight disease on endophytic microbial diversity and community structure in tea (Camellia sinensis) leaves. 3 Biotech. 13, 421. doi: 10.1007/s13205-023-03846-y
Castellana, F., De Nucci, S., De Pergola, G., Di Chito, M., Lisco, G., Triggiani, V., et al. (2021). Trends in coffee and tea consumption during the COVID-19 pandemic. Foods 10, 2458. doi: 10.3390/foods10102458
Ceja-Navarro, J. A., Vega, F. E., Karaoz, U., Hao, Z., Jenkins, S., Lim, H. C., et al. (2015). Gut microbiota mediate caffeine detoxification in the primary insect pest of coffee. Nat. Commun. 6, 7618. doi: 10.1038/ncomms8618
Cernava, T., Chen, X., Krug, L., Li, H., Yang, M., Berg, G. (2019). The tea leaf microbiome shows specific responses to chemical pesticides and biocontrol applications. Sci. Total Environ. 667, 33–40. doi: 10.1016/j.scitotenv.2019.02.319
Chakraborty, U., Chakraborty, B., Basnet, M. (2006). Plant growth promotion and induction of resistance in Camellia sinensis by Bacillus megaterium. J. Basic Microbiol. 46, 186–195. doi: 10.1002/jobm.200510050
Chakraborty, U., Chakraborty, B. N., Basnet, M., Chakraborty, A. P. (2009). Evaluation of Ochrobactrum anthropi TRS-2 and its talc based formulation for enhancement of growth of tea plants and management of brown root rot disease. J. Appl. Microbiol. 107, 625–634. doi: 10.1111/j.1365-2672.2009.04242.x
Chen, Y., Fu, W., Xiao, H., Zhai, Y., Luo, Y., Wang, Y., et al. (2023). A review on rhizosphere microbiota of tea plant (Camellia sinensis L): recent insights and future perspectives. J. Agric. Food Chem. 71, 19165–19188. doi: 10.1021/acs.jafc.3c02423
Chen, X., Wicaksono, W. A., Berg, G., Cernava, T. (2021). Bacterial communities in the plant phyllosphere harbour distinct responders to a broad-spectrum pesticide. Sci. Total Environ. 751, 141799. doi: 10.1016/j.scitotenv.2020.141799
Chen, W., Ye, T., Sun, Q., Niu, T., Zhang, J. (2022). Arbuscular mycorrhizal fungus alleviates anthracnose disease in tea seedlings. Front. Plant Sci. 13. doi: 10.3389/fpls.2022.1058092
Chen, S., Zhang, L., Cai, X., Li, X., Bian, L., Luo, Z., et al. (2020). (E)-Nerolidol is a volatile signal that induces defenses against insects and pathogens in tea plants. Horticulture Res. 7, 52. doi: 10.1038/s41438-020-0275-7
Cheramgoi, E., Wanjala, F., Sudoi, V., Kanyiri, W., Mwamburi, L., Nyukuri, R. (2016). Efficacy and mode of application of local Beauveria bassiana isolates in the control of the tea weevil. Annu. Res. Rev. Biol. 10, 1–8. doi: 10.9734/ARRB/2016/23235
Cho, J.-Y., Mizutani, M., Shimizu, B., Kinoshita, T., Ogura, M., Tokoro, K., et al. (2007). Chemical profiling and gene expression profiling during the manufacturing process of Taiwan oolong tea “Oriental beauty. Biosci. Biotech. Bioch 71, 1476–1486. doi: 10.1271/bbb.60708
Choudhary, D. K., Johri, B. N. (2009). Interactions of Bacillus spp. and plants – With special reference to induced systemic resistance (ISR). Microbiol. Res. 164, 493–513. doi: 10.1016/j.micres.2008.08.007
Collier, F. A., Elliot, S. L., Ellis, R. J. (2005). Spatial variation in Bacillus thuringiensis/cereus populations within the phyllosphere of broad-leaved dock (Rumex obtusifolius) and surrounding habitats. FEMS Microbiol. Ecol. 54, 417–425. doi: 10.1016/j.femsec.2005.05.005
Das, R., Bharadwaj, P., Thakur, D. (2024). Insights into the functional role of Actinomycetia in promoting plant growth and biocontrol in tea (Camellia sinensis) plants. Arch. Microbiol. 206, 65. doi: 10.1007/s00203-023-03789-1
Dashora, K., Roy, S., Nagpal, A., Roy, S. M., Flood, J., Prasad, A., et al. (2017). Pest management through Bacillus thuringiensis (Bt) in a tea-silkworm ecosystem: status and potential prospects. Appl. Microbiol. Biotechnol. 101, 1795–1803. doi: 10.1007/s00253-017-8113-z
De, D., Mukhopadhyay, A., Chakraborty, R. (2008). A novel bacterial pathogen (Enterobacter sp.) isolated from the leaf roller, Caloptilia theivora of tea of Darjeeling foothills. World J. Microbiol. Biotechnol. 24, 2727–2729. doi: 10.1007/s11274-008-9760-0
Deka, B., Babu, A., Pandey, A. K., Kumhar, K. C., Rajbongshi, H., Dey, P., et al. (2022a). Potential of the entomopathogenic fungus, Metarhizium anisopliae s.l. for control of red spider mite, Oligonychus coffeae Nietner on tea crop. Int. J. Acarol. 48, 121–129. doi: 10.1080/01647954.2022.2041089
Deka, B., Babu, A., Peter, A. J., Pandey, A. K., Kumhar, K. C., Sarkar, S., et al. (2021). Beauveria bassiana: as a potential microbial biocontrol agent for tea mosquito bug, Helopeltis theivora Waterhouse (Hemiptera: Miridae) in Dooars and Darjeeling, India. Egypt J. Biol. Pest Control 31, 134. doi: 10.1186/s41938-021-00478-3
Deka, B., Babu, A., Sarkar, S., Kuriakose, B., Thomas, G. (2023). Hyposidra talaca NPV (HytaNPV): a potential baculovirus for efficient control of the black inch worm, Hyposidra talaca Walker (Lepidoptera: Geometridae), a major pest of tea Camellia sinensis (Ericales: Theaceae (L.) O. Kuntze). Egypt J. Biol. Pest Co 33, 116. doi: 10.1186/s41938-023-00760-6
Deka, B., Sarkar, S., Modak, D., Babu, A. (2022b). Significance of microbes and their role in pest management in tea ecosystem. IJTS 16, 16–21. doi: 10.20425/ijts1614
Dhar Purkayastha, G., Mangar, P., Saha, A., Saha, D. (2018). Evaluation of the biocontrol efficacy of a Serratia marcescens strain indigenous to tea rhizosphere for the management of root rot disease in tea. PloS One 13, e0191761. doi: 10.1371/journal.pone.0191761
do Nascimento, J., Cristina Goncalves, K., Pinto Dias, N., Luiz de Oliveira, J., Bravo, A., Antonio Polanczyk, R. (2022). Adoption of Bacillus thuringiensis-based biopesticides in agricultural systems and new approaches to improve their use in Brazil. Biol. Control 165, 104792. doi: 10.1016/j.biocontrol.2021.104792
Du, W., Zhou, J., Jiang, P., Yang, T., Bu, Y. Q., Liu, C. H., et al. (2013). Effects of Beauveria bassiana and acephate on enzyme activities and microbial diversity in paddy soil. Plant Soil Environ. 59, 562–567. doi: 10.17221/447/2013-PSE
Duke, S. O. (2018). Interaction of chemical pesticides and their formulation ingredients with microbes associated with plants and plant pests. J. Agric. Food Chem. 66, 7553–7561. doi: 10.1021/acs.jafc.8b02316
FAO (2022). International tea market: market situation, prospects and emerging issues. Available online at: https://openknowledge.fao.org/handle/20.500.14283/cc0238en (Accessed April 16, 2024).
Feng, M.-G., Pu, X.-Y., Ying, S.-H., Wang, Y.-G. (2004). Field trials of an oil-based emulsifiable formulation of Beauveria bassiana conidia and low application rates of imidacloprid for control of false-eye leafhopper Empoasca vitis on tea in southern China. Crop Prot. 23, 489–496. doi: 10.1016/j.cropro.2003.10.004
Fenta, L., Mekonnen, H., Kabtimer, N. (2023). The exploitation of microbial antagonists against postharvest plant pathogens. Microorganisms 11, 1044. doi: 10.3390/microorganisms11041044
Ferreira, L. H. P. L., Molina, J. C., Brasil, C., Andrade, G. (2003). Evaluation of Bacillus thuringiensis bioinsecticidal protein effects on soil microorganisms. Plant Soil 256, 161–168. doi: 10.1023/A:1026256700237
Fu, Z. Q., Dong, X. (2013). Systemic acquired resistance: turning local infection into global defense. Annu. Rev. Plant Biol. 64, 839–863. doi: 10.1146/annurev-arplant-042811-105606
Fu, N., Wang, T., Li, Q., Luo, Z., Li, Z., Bian, L., et al. (2023). Identification and biocontrol potential evaluation of a naturally occurring Metarhizium pingshaense isolate infecting tea weevil Myllocerinus aurolineatus Voss (Coleoptera: Curculionidae). Egypt J. Biol. Pest Co 33, 101. doi: 10.1186/s41938-023-00749-1
Ghatak, S. S., Reza, M. W. (2007). Bio-efficacy of Beauveria bassiana against tea looper caterpillar, Buzura suppressaria Guen.(Lepidopera: Geometridae). Acta Entomologica Sinica. 50, 962–966. doi: 10.16380/J.KCXB.2007.09.014
Gilbert, N. (2019). The science of tea’s mood-altering magic. Nature 566, S8–S9. doi: 10.1038/d41586-019-00398-1
Gnanamangai, B. M., Ponmurugan, P. (2012). Evaluation of various fungicides and microbial based biocontrol agents against bird’s eye spot disease of tea plants. Crop Prot. 32, 111–118. doi: 10.1016/j.cropro.2011.10.001
Gong, F., He, C., Xianen, L., Wang, K., Li, M., Zhou, X., et al. (2024). Impacts of fertilization methods on Salvia miltiorrhiza quality and characteristics of the epiphytic microbial community. Front. Plant Sci. 15. doi: 10.3389/fpls.2024.1395628
Grosch, R., Dealtry, S., Schreiter, S., Berg, G., Mendonça-Hagler, L., Smalla, K. (2012). Biocontrol of Rhizoctonia solani: complex interaction of biocontrol strains, pathogen and indigenous microbial community in the rhizosphere of lettuce shown by molecular methods. Plant Soil 361, 343–357. doi: 10.1007/s11104-012-1239-y
Gurusubramanian, G., Rahman, A., Sarmah, M., Ray, S., Bora, S. (2008). Pesticide usage pattern in tea ecosystem, their retrospects and alternative measures. J. Environ. Biol. 29, 813–826.
Hage-Ahmed, K., Rosner, K., Steinkellner, S. (2019). Arbuscular mycorrhizal fungi and their response to pesticides. Pest Manage. Sci. 75, 583–590. doi: 10.1002/ps.5220
Hai, D., Li, J., Jiang, D., Cheng, J., Fu, Y., Xiao, X., et al. (2024). Plants interfere with non-self recognition of a phytopathogenic fungus via proline accumulation to facilitate mycovirus transmission. Nat. Commun. 15, 4748. doi: 10.1038/s41467-024-49110-6
Hao, L., Zhang, Z., Hao, B., Diao, F., Zhang, J., Bao, Z., et al. (2021). Arbuscular mycorrhizal fungi alter microbiome structure of rhizosphere soil to enhance maize tolerance to La. Ecotoxicol Environ. Saf. 212, 111996. doi: 10.1016/j.ecoenv.2021.111996
Hazarika, L. K., Bhuyan, M., Hazarika, B. N. (2009). Insect pests of tea and their management. Annu. Rev. Entomology 54, 267–284. doi: 10.1146/annurev.ento.53.103106.093359
Hazarika, S. N., Saikia, K., Borah, A., Thakur, D. (2021). Prospecting endophytic bacteria endowed with plant growth promoting potential isolated from Camellia sinensis. Front. Microbiol. 12. doi: 10.3389/fmicb.2021.738058
He, Y., Miao, X., Xia, Y., Chen, X., Liu, J., Zhou, G. (2024). The Research of Antagonistic Endophytic Bacterium Bacillus velezensis CSUFT-BV4 for Growth Promotion and Induction of Resistance to Anthracnose in Camellia oleifera. Microorganisms 12, 763. doi: 10.3390/microorganisms12040763
Hilleary, R., Gilroy, S. (2018). Systemic signaling in response to wounding and pathogens. Curr. Opin. Plant Biol. 43, 57–62. doi: 10.1016/j.pbi.2017.12.009
Hou, S., Tsuda, K. (2022). Salicylic acid and jasmonic acid crosstalk in plant immunity. Essays Biochem. 66, 647–656. doi: 10.1042/EBC20210090
Hu, L., Robert, C. A. M., Cadot, S., Zhang, X., Ye, M., Li, B., et al. (2018). Root exudate metabolites drive plant-soil feedbacks on growth and defense by shaping the rhizosphere microbiota. Nat. Commun. 9, 2738. doi: 10.1038/s41467-018-05122-7
Hu, Y., Zhang, M., Lu, M., Wu, Y., Jing, T., Zhao, M., et al. (2022). Salicylic acid carboxyl glucosyltransferase UGT87E7 regulates disease resistance in Camellia sinensis. Plant Physiol. 188, 1507–1520. doi: 10.1093/plphys/kiab569
Huang, X., Tang, Q., Li, Q., Lin, H., Li, J., Zhu, M., et al. (2022). Integrative analysis of transcriptome and metabolome reveals the mechanism of foliar application of Bacillus amyloliquefaciens to improve summer tea quality (Camellia sinensis). Plant Physiol. Bioch 185, 302–313. doi: 10.1016/j.plaphy.2022.06.016
Huang, H., Yao, Q., Xia, E., Gao, L. (2018). Metabolomics and transcriptomics analyses reveal nitrogen influences on the accumulation of flavonoids and amino acids in young shoots of tea plant (Camellia sinensis L.) associated with tea flavor. J. Agric. Food Chem. 66, 9828–9838. doi: 10.1021/acs.jafc.8b01995
Huang, X., Zheng, Y., Li, P., Cui, J., Sui, P., Chen, Y., et al. (2023). Organic management increases beneficial microorganisms and promotes the stability of microecological networks in tea plantation soil. Front. Microbiol. 14. doi: 10.3389/fmicb.2023.1237842
Huidrom, P., Sharma, G. D (2024). Role of rhizobacteria of tea garden in bioremediation of pesticide residues in tea soil. Int. J. Sci. Res. (IJSR) 13, 1019–1023. doi: 10.21275/SR24314152107
Idris, A. L., Fan, X., Muhammad, M. H., Guo, Y., Guan, X., Huang, T. (2020). Ecologically controlling insect and mite pests of tea plants with microbial pesticides: a review. Arch. Microbiol. 202, 1275–1284. doi: 10.1007/s00203-020-01862-7
Islam, M. S., Ahmad, I., Ali, M. (2018). Biocontrol studies on rizpspheric microorganisms against black rot disease of tea caused by Corticium theae Bernard. Bangladesh J. Bot. 47, 985–991. doi: 10.3329/bjb.v47i4.47399
Jayasinghe, S. L., Kumar, L. (2021). Potential impact of the current and future climate on the yield, quality, and climate suitability for tea [Camellia sinensis (L.) O. Kuntze]: A systematic review. Agronomy 11, 619. doi: 10.3390/agronomy11040619
Jayaswall, K., Mahajan, P., Singh, G., Parmar, R., Seth, R., Raina, A., et al. (2016). Transcriptome Analysis Reveals Candidate Genes involved in Blister Blight defense in Tea (Camellia sinensis (L) Kuntze). Sci. Rep. 6, 30412. doi: 10.1038/srep30412
Jeyaraj, A., Liu, S., Zhang, X., Zhang, R., Shangguan, M., Wei, C. (2017). Genome-wide identification of microRNAs responsive to Ectropis oblique feeding in tea plant (Camellia sinensis L.). Sci. Rep. 7, 13634. doi: 10.1038/s41598-017-13692-7
Jeyaraman, M., Robert, P. S. A. (2017). Integrated management of branch canker disease (Macrophoma sp.) in tea under field level. J. Plant Dis. Prot 124, 115–119. doi: 10.1007/s41348-017-0072-1
Jeyaraman, M., Robert, P. S. A. (2018). Bio efficacy of indigenous biological agents and selected fungicides against branch canker disease of (Macrophoma theicola) tea under field level. BMC Plant Biol. 18, 222. doi: 10.1186/s12870-018-1445-8
Jiao, L., Bian, L., Luo, Z., Li, Z., Xiu, C., Fu, N., et al. (2022). Enhanced volatile emissions and anti-herbivore functions mediated by the synergism between jasmonic acid and salicylic acid pathways in tea plants. Hortic. Res-england 9, uhac144. doi: 10.1093/hr/uhac144
Jibola-Shittu, M. Y., Heng, Z., Keyhani, N. O., Dang, Y., Chen, R., Liu, S., et al. (2024). Understanding and exploring the diversity of soil microorganisms in tea (Camellia sinensis) gardens: toward sustainable tea production. Front. Microbiol. 15. doi: 10.3389/fmicb.2024.1379879
Jin, S., Ren, Q., Lian, L., Cai, X., Bian, L., Luo, Z., et al. (2020). Comparative transcriptomic analysis of resistant and susceptible tea cultivars in response to Empoasca onukii (Matsuda) damage. Planta 252, 10. doi: 10.1007/s00425-020-03407-0
Jing, T., Qian, X., Du, W., Gao, T., Li, D., Guo, D., et al. (2021). Herbivore-induced volatiles influence moth preference by increasing the β-Ocimene emission of neighbouring tea plants. Plant Cell Environ. 44, 3667–3680. doi: 10.1111/pce.14174
Johansen, A., Olsson, S. (2005). Using phospholipid fatty acid technique to study short-term effects of the biological control agent pseudomonas fluorescens DR54 on the microbial microbiota in barley rhizosphere. Microb. Ecol. 49, 272–281. doi: 10.1007/s00248-004-0135-2
Jomova, K., Alomar, S. Y., Alwasel, S. H., Nepovimova, E., Kuca, K., Valko, M. (2024). Several lines of antioxidant defense against oxidative stress: antioxidant enzymes, nanomaterials with multiple enzyme-mimicking activities, and low-molecular-weight antioxidants. Arch. Toxicol. 98, 1323–1367. doi: 10.1007/s00204-024-03696-4
Joshi, M., Gaur, N., Pandey, R. (2018). Compatibility of entomopathogenic fungi Beauveria bassiana and Metarhizium anisopliae with selective pesticides. J. Entomology Zoology Stud. 6, 867–872. doi: 10.22271/j.ento.2020.v8.i6u.8040
Kabir, M. H., Unban, K., Kodchasee, P., Govindarajan, R. K., Lumyong, S., Suwannarach, N., et al. (2023). Endophytic Bacteria Isolated from Tea Leaves (Camellia sinensis var. assamica) Enhanced Plant-Growth-Promoting Activity. Agriculture 13, 533. doi: 10.3390/agriculture13030533
Khoso, M. A., Wagan, S., Alam, I., Hussain, A., Ali, Q., Saha, S., et al. (2024). Impact of plant growth-promoting rhizobacteria (PGPR) on plant nutrition and root characteristics: Current perspective. Plant Stress 11, 100341. doi: 10.1016/j.stress.2023.100341
Kim, G.-H., Lim, M.-T., Hur, J.-S., Yum, K.-J., Koh, Y.-J. (2009). Biological control of tea anthracnose using an antagonistic bacterium of bacillus subtilis isolated from tea leaves. Plant Pathol. J. 25, 99–102. doi: 10.5423/PPJ.2009.25.1.099
Kolandasamy, M., Mandal, A. K. A., Balasubramanian, M. G., Ponnusamy, P. (2023). Multifaceted plant growth-promoting traits of indigenous rhizospheric microbes against Phomopsis theae, a causal agent of stem canker in tea plants. World J. Microbiol. Biotechnol. 39, 237. doi: 10.1007/s11274-023-03688-z
Kozdrój, J., Trevors, J., Van Elsas, J. (2004). Influence of introduced potential biocontrol agents on maize seedling growth and bacterial community structure in the rhizosphere. Soil Biol. Biochem. 36, 1775–1784. doi: 10.1016/j.soilbio.2004.04.034
Kumhar, K. C., Babu, A., Arulmarianathan, J. P., Deka, B., Bordoloi, M., Rajbongshi, H., et al. (2020). Role of beneficial fungi in managing diseases and insect pests of tea plantation. Egypt J. Biol. Pest Co 30, 78. doi: 10.1186/s41938-020-00270-9
Kusajima, M., Shima, S., Fujita, M., Minamisawa, K., Che, F.-S., Yamakawa, H., et al. (2018). Involvement of ethylene signaling in Azospirillum sp. B510-induced disease resistance in rice. Biosci. Biotech. Bioch 82, 1522–1526. doi: 10.1080/09168451.2018.1480350
Lebeis, S. L., Paredes, S. H., Lundberg, D. S., Breakfield, N., Gehring, J., McDonald, M., et al. (2015). Salicylic acid modulates colonization of the root microbiome by specific bacterial taxa. Science 349, 860–864. doi: 10.1126/science.aaa8764
Li, L., Li, T., Jiang, Y., Yang, Y., Zhang, L., Jiang, Z., et al. (2020). Alteration of local and systemic amino acids metabolism for the inducible defense in tea plant (Camellia sinensis) in response to leaf herbivory by Ectropis oblique. Arch. Biochem. Biophys. 683, 108301. doi: 10.1016/j.abb.2020.108301
Li, X., Zhang, J., Li, D., Cai, X., Qi, Y., Lu, Y. (2024). Toxicity of Beauveria bassiana to Bactrocera dorsalis and effects on its natural predators. Front. Microbiol. 15. doi: 10.3389/fmicb.2024.1362089
Li, X., Zhang, J., Lin, S., Xing, Y., Zhang, X., Ye, M., et al. (2022). (+)-Catechin, epicatechin and epigallocatechin gallate are important inducible defensive compounds against Ectropis grisescens in tea plants. Plant Cell Environ. 45, 496–511. doi: 10.1111/pce.14216
Liao, Y., Yu, Z., Liu, X., Zeng, L., Cheng, S., Li, J., et al. (2019). Effect of major tea insect attack on formation of quality-related nonvolatile specialized metabolites in tea (Camellia sinensis) leaves. J. Agric. Food Chem. 67, 6716–6724. doi: 10.1021/acs.jafc.9b01854
Liao, Y., Zhou, X., Zeng, L. (2022). How does tea (Camellia sinensis) produce specialized metabolites which determine its unique quality and function: a review. Crit. Rev. Food Sci. Nutr. 62, 3751–3767. doi: 10.1080/10408398.2020.1868970
Lin, S., Chen, Z., Chen, T., Deng, W., Wan, X., Zhang, Z. (2023b). Theanine metabolism and transport in tea plants (Camellia sinensis L.): advances and perspectives. Crit. Rev. Biotechnol. 43, 327–341. doi: 10.1080/07388551.2022.2036692
Lin, H., Liu, C., Peng, Z., Tan, B., Wang, K., Liu, Z. (2022). Distribution pattern of endophytic bacteria and fungi in tea plants. Front. Microbiol. 13. doi: 10.3389/fmicb.2022.872034
Lin, F., Mao, Y., Zhao, F., Idris, A. L., Liu, Q., Zou, S., et al. (2023a). Towards sustainable green adjuvants for microbial pesticides: recent progress, upcoming challenges, and future perspectives. Microorganisms 11, 364. doi: 10.3390/microorganisms11020364
Liu, Y., Li, Y., Hua, X., Müller, K., Wang, H., Yang, T., et al. (2018). Glyphosate application increased catabolic activity of gram-negative bacteria but impaired soil fungal community. Environ. Sci. pollut. Res. Int. 25, 14762–14772. doi: 10.1007/s11356-018-1676-0
Liu, L., Sonbol, F.-M., Huot, B., Gu, Y., Withers, J., Mwimba, M., et al. (2016). Salicylic acid receptors activate jasmonic acid signalling through a non-canonical pathway to promote effector-triggered immunity. Nat. Commun. 7, 13099. doi: 10.1038/ncomms13099
Liu, G., Wang, Q., Chen, H., Wang, Y., Zhou, X., Bao, D., et al. (2024a). Plant-derived monoterpene S-linalool and β-ocimene generated by CsLIS and CsOCS-SCZ are key chemical cues for attracting parasitoid wasps for suppressing Ectropis obliqua infestation in Camellia sinensis L. Plant Cell Environ. 47, 913–927. doi: 10.1111/pce.14803
Liu, H., Wang, H., Liao, X. L., Gao, B., Lu, X., Sun, D., et al. (2022). Mycoviral gene integration converts a plant pathogenic fungus into a biocontrol agent. Proc. Natl. Acad. Sci. 119, e2214096119. doi: 10.1073/pnas.2214096119
Liu, Y., Yan, Y., Ma, L., Cao, D., Jin, X. (2024b). Physiological and metabolomic analyses reveal the resistance response mechanism to tea aphid infestation in new shoots of tea plants (Camellia sinensis). Plant Stress 13, 100545. doi: 10.1016/j.stress.2024.100545
Lu, C., Liu, H., Jiang, D., Wang, L., Jiang, Y., Tang, S., et al. (2019). Paecilomyces variotii extracts (ZNC) enhance plant immunity and promote plant growth. Plant Soil 441, 383–397. doi: 10.1007/s11104-019-04130-w
Ma, K.-W., Niu, Y., Jia, Y., Ordon, J., Copeland, C., Emonet, A., et al. (2021). Coordination of microbe–host homeostasis by crosstalk with plant innate immunity. Nat. Plants 7, 814–825. doi: 10.1038/s41477-021-00920-2
Maduell, P., Armengol, G., Llagostera, M., Orduz, S., Lindow, S. (2008). B. thuringiensis is a poor colonist of leaf surfaces. Microb. Ecol. 55, 212–219. doi: 10.1007/s00248-007-9268-4
Manjukarunambika, K., Ponmurugan, P., Marimuthu, S. (2013). Efficacy of various fungicides and indigenous biocontrol agents against red root rot disease of tea plants. Eur. J. Plant Pathol. 137, 67–78. doi: 10.1007/s10658-013-0217-4
Marimuthu, S., Karthic, C., Mostafa, A. A., Mohammed Al-Enazi, N., Abdel-Raouf, N., Nageh Sholkamy, E. (2020). Antifungal activity of Streptomyces sp. SLR03 against tea fungal plant pathogen Pestalotiopsis theae. J. King Saud Univ. - Sci. 32, 3258–3264. doi: 10.1016/j.jksus.2020.08.027
Martinez, D. A., Loening, U. E., Graham, M. C. (2018). Impacts of glyphosate-based herbicides on disease resistance and health of crops: a review. Environ. Sci. Eur. 30, 2. doi: 10.1186/s12302-018-0131-7
McGuire, A. V., Northfield, T. D. (2020). Tropical Occurrence and Agricultural Importance of Beauveria bassiana and Metarhizium anisopliae. Front. Sustain Food S 4. doi: 10.3389/fsufs.2020.00006
Mishra, A. K., Morang, P., Deka, M., Nishanth Kumar, S., Dileep Kumar, B. S. (2014). Plant growth-promoting rhizobacterial strain-mediated induced systemic resistance in tea (Camellia sinensis (L.) O. Kuntze) through defense-related enzymes against brown root rot and charcoal stump rot. Appl. Biochem. Biotechnol. 174, 506–521. doi: 10.1007/s12010-014-1090-0
Mukhopadhyay, A., De, D., Khewa, S. (2010). Exploring the biocontrol potential of naturally occurring bacterial and viral entomopathogens of defoliating lepidopteran pests of tea plantations. J. Biopesticides 3, 117.
Mythili Gnanamangai, B., Ponmurugan, P., Jeeva, S. E., Manjukarunambika, K., Elango, V., Hemalatha, K., et al. (2017). Biosynthesised silver and copper nanoformulation as foliar spray to control bird’s eye spot disease in tea plantations. IET Nanobiotechnol 11, 917–928. doi: 10.1049/iet-nbt.2017.0023
Naskar, S., Roy, C., Ghosh, S., Mukhopadhyay, A., Hazarika, L. K., Chaudhuri, R. K., et al. (2021). Elicitation of biomolecules as host defense arsenals during insect attacks on tea plants (Camellia sinensis (L.) Kuntze). Appl. Microbiol. Biotechnol. 105, 7187–7199. doi: 10.1007/s00253-021-11560-z
Ohler, L.-M., Seeleitner, S., Haselberger, S., Kraushaar, S., Otto, J.-C., Mitter, B., et al. (2022). Manipulation of phyllosphere bacterial communities reversibly alters the plant microbiome and leaf traits in the field. Alpine Bot. 132, 301–314. doi: 10.1007/s00035-021-00273-8
Pan, X., Cao, F., Guo, X., Wang, Y., Cui, Z., Huang, T., et al. (2024). Development of a safe and effective bacillus thuringiensis -based nanobiopesticide for controlling tea pests. J. Agric. Food Chem. 72, 7807–7817. doi: 10.1021/acs.jafc.4c00833
Pandey, A. K., Deka, B., Varshney, R., Cheramgoi, E. C., Babu, A. (2021a). Do the beneficial fungi manage phytosanitary problems in the tea agro-ecosystem? BioControl 66, 445–462. doi: 10.1007/s10526-021-10084-9
Pandey, A. K., Kumar, A., Samota, M. K., Tanti, A. (2022). Trichoderma reesei as an elicitor triggers defense responses in tea plant and delays gray blight symptoms. Pestic Biochem. Physiol. 188, 105279. doi: 10.1016/j.pestbp.2022.105279
Pandey, A. K., Samota, M. K., Tanti, A. J., Babu, A. (2023). Trichoderma reesei induces defense-related biochemical markers associated with resistance to Fusarium dieback in tea crop. Biol. Control 180, 105200. doi: 10.1016/j.biocontrol.2023.105200
Pandey, A. K., Sinniah, G. D., Babu, A., Tanti, A. (2021b). How the global tea industry copes with fungal diseases – challenges and opportunities. Plant Dis. 105, 1868–1879. doi: 10.1094/PDIS-09-20-1945-FE
Payne, C. C. (1982). Insect viruses as control agents. Parasitology 84, 35–77. doi: 10.1017/S0031182000053609
Pedersen, J. C., Hansen, B. M., Damgaard, P. H., Eilenberg, J. (1995). Dispersal of Bacillus thuringiensis var. kurstaki in an experimental cabbage field. Can. J. Microbiol. 41, 118–125. doi: 10.1139/m95-016
Perazzolli, M., Antonielli, L., Storari, M., Puopolo, G., Pancher, M., Giovannini, O., et al. (2014). Resilience of the natural phyllosphere microbiota of the grapevine to chemical and biological pesticides. Appl. Environ. Microbiol. 80, 3585–3596. doi: 10.1128/AEM.00415-14
Pieterse, C. M. J., Zamioudis, C., Berendsen, R. L., Weller, D. M., Wees, S. C. M. V., Bakker, P. A. H. M. (2014). Induced systemic resistance by beneficial microbes. Annu. Rev. Phytopathol. 52, 347–375. doi: 10.1146/annurev-phyto-082712-102340
Qin, C., Tao, J., Liu, T., Liu, Y., Xiao, N., Li, T., et al. (2019). Responses of phyllosphere microbiota and plant health to application of two different biocontrol agents. AMB Express 9, 42. doi: 10.1186/s13568-019-0765-x
Román Calderón, M. E., Alcocer González, J. M., Franco Molina, M. A., Tamez Guerra, R. S., Padilla, C. R. (2007). Adjuvant effects of crystal proteins from a Mexican strain of Bacillus thuringiensis on the mouse humoral response. Biologicals 35, 271–276. doi: 10.1016/j.biologicals.2007.01.006
Roobak Kumar, A., Rahman, V. J., Vasantha Kumar, D., Babu, A., Subramaniam, M. S. R. (2011). Utilization of the bacterium, Pseudomonas putida as a potential biocontrol agent against red spider mite, Oligonychus coffeae, (Acari: Tetranychidae) infesting tea. J. Plantation Crops (India) 39, 236–238.
Roy, S., Muraleedharan, N. (2014). Microbial management of arthropod pests of tea: current state and prospects. Appl. Microbiol. Biotechnol. 98, 5375–5386. doi: 10.1007/s00253-014-5749-9
Russell, D., Chard, J., McKinlay, R. (1999). Effect of Bacillus thuringiensis and a pyrethroid insecticide on the leaf microflora of Brassica oleracea. Lett. Appl. Microbiol. 28, 359–362. doi: 10.1046/j.1365-2672.1999.00548.x
Saito, T., Takatsuka, J., Shimazu, M. (2012). Characterization of Paecilomyces cinnamomeus from the camellia whitefly, Aleurocanthus camelliae (Hemiptera: Aleyrodidae), infesting tea in Japan. J. Invertebrate Pathol. 110, 14–23. doi: 10.1016/j.jip.2012.01.011
Sánchez-Rodríguez, A. R., Raya-Díaz, S., Zamarreño, Á.M., García-Mina, J. M., del Campillo, M. C., Quesada-Moraga, E. (2018). An endophytic Beauveria bassiana strain increases spike production in bread and durum wheat plants and effectively controls cotton leafworm (Spodoptera littoralis) larvae. Biol. Control 116, 90–102. doi: 10.1016/j.biocontrol.2017.01.012
Sandeep Kumar, D., Tarakeswari, M., Lakshminarayana, M., Sujatha, M. (2016). Toxicity of Bacillus thuringiensis crystal proteins against eri silkworm, Samia cynthia ricini (Lepidoptera: Saturniidae). J. Invertebrate Pathol. 138, 116–119. doi: 10.1016/j.jip.2016.06.012
Sang, M. K., Kim, K. D. (2012). Plant growth-promoting rhizobacteria suppressive to Phytophthora blight affect microbial activities and communities in the rhizosphere of pepper (Capsicum annuum L.) in the field. Appl. Soil Ecol. 62, 88–97. doi: 10.1016/j.apsoil.2012.08.001
Sanjay, R., Ponmurugan, P., Baby, U. I. (2008). Evaluation of fungicides and biocontrol agents against grey blight disease of tea in the field. Crop Prot. 27, 689–694. doi: 10.1016/j.cropro.2007.09.014
Sasan, R. K., Bidochka, M. J. (2012). The insect-pathogenic fungus Metarhizium robertsii (Clavicipitaceae) is also an endophyte that stimulates plant root development. Am. J. Bot. 99, 101–107. doi: 10.3732/ajb.1100136
Sato, T., Oho, N., Kodomari, S. (1986). Utilization of granulosis viruses for controlling leafrollers in tea fields. JARQ. 19, 271–275.
Senthilkumar, P., Thirugnanasambantham, K., Mandal, A. K. A. (2012). Suppressive subtractive hybridization approach revealed differential expression of hypersensitive response and reactive oxygen species production genes in tea (Camellia sinensis (L.) O. Kuntze) leaves during Pestalotiopsis thea infection. Appl. Biochem. Biotechnol. 168, 1917–1927. doi: 10.1007/s12010-012-9907-1
Shang, J., Hong, S., Wang, C. (2024). Fights on the surface prior to fungal invasion of insects. PloS Pathog. 20, e1011994. doi: 10.1371/journal.ppat.1011994
Shang, J., Liu, B., Xu, Z. (2020). Efficacy of Trichoderma asperellum TC01 against anthracnose and growth promotion of Camellia sinensis seedlings. Biol. Control 143, 104205. doi: 10.1016/j.biocontrol.2020.104205
Singh, S., Gupta, R., Kumari, M., Sharma, S. (2015a). Nontarget effects of chemical pesticides and biological pesticide on rhizospheric microbial community structure and function in Vigna radiata. Environ. Sci. pollut. Res. Int. 22, 11290–11300. doi: 10.1007/s11356-015-4341-x
Singha, D., Singha, B., Dutta, B. (2010). In vitro pathogenicity of Bacillus thuringiensis against tea termites. J Biol Control. 24, 279–281.
Singh, S., Gupta, R., Sharma, S. (2015b). Effects of chemical and biological pesticides on plant growth parameters and rhizospheric bacterial community structure in Vigna radiata. J. Hazard Mater 291, 102–110. doi: 10.1016/j.jhazmat.2015.02.053
Singh, U., Roy, P., Sharma, S. (2022). Bacterial inoculants as effective agents in minimizing the non-target impact of azadirachtin pesticide and promoting plant growth of Vigna radiata. Arch. Microbiol. 204, 555. doi: 10.1007/s00203-022-03162-8
Singha, D., Singha, B., Dutta, B. K. (2011). Potential of Metarhizium anisopliae and Beauveria bassiana in the control of tea termite Microtermes obesi Holmgren in vitro and under field conditions. J. Pest Sci. 84, 69–75. doi: 10.1007/s10340-010-0328-z
Smets, W., Koskella, B. (2020). Microbiome: insect herbivory drives plant phyllosphere dysbiosis. Curr. Biol. 30, R412–R414. doi: 10.1016/j.cub.2020.03.039
Sood, P., Choudhary, A., Prabhakar, C. S. (2019). “Granuloviruses in Insect Pest Management,” in Microbes for Sustainable Insect Pest Management : An Eco-friendly Approach, vol. 1 . Eds. Khan, M., Ahmad, W. (Springer International Publishing, Cham), 275–298. doi: 10.1007/978-3-030-23045-6_10
Sowndhararajan, K., Marimuthu, S., Manian, S. (2013). Integrated control of blister blight disease in tea using the biocontrol agent Ochrobactrum anthropi strain BMO-111 with chemical fungicides. J. Appl. Microbiol. 114, 1491–1499. doi: 10.1111/jam.12159
Sultana, R., Imam, Z., Kumar, R. R., Banu, V. S., Nahakpam, S., Bharti, R., et al. (2024). Signaling and defence mechanism of jasmonic and salicylic acid response in pulse crops: role of WRKY transcription factors in stress response. J. Plant Growth Regul., 1–17. doi: 10.1007/s00344-023-11203-9
Sun, J., Chang, M., Li, H., Zhang, Z., Chen, Q., Chen, Y., et al. (2019). Endophytic bacteria as contributors to theanine production in Camellia sinensis. J. Agric. Food Chem. 67, 10685–10693. doi: 10.1021/acs.jafc.9b03946
Sylla, J., Alsanius, B. W., Krüger, E., Reineke, A., Strohmeier, S., Wohanka, W. (2013). Leaf microbiota of strawberries as affected by biological control agents. Phytopathology 103, 1001–1011. doi: 10.1094/PHYTO-01-13-0014-R
Tan, L., Gu, S., Li, S., Ren, Z., Deng, Y., Liu, Z., et al. (2019). Responses of microbial communities and interaction networks to different management practices in tea plantation soils. Sustainability 11, 4428. doi: 10.3390/su11164428
Tan, X., Xie, H., Yu, J., Wang, Y., Xu, J., Xu, P., et al. (2022). Host genetic determinants drive compartment-specific assembly of tea plant microbiomes. Plant Biotechnol. J. 20, 2174–2186. doi: 10.1111/pbi.13897
Thambugala, K. M., Daranagama, D. A., Phillips, A. J. L., Kannangara, S. D., Promputtha, I. (2020). Fungi vs. Fungi in biocontrol: an overview of fungal antagonists applied against fungal plant pathogens. Front. Cell. Infect. Microbiol. 10. doi: 10.3389/fcimb.2020.604923
Thoudam, R., Dutta, B. K. (2012). Control of black rot disease of tea, Camellia sinensis (L.) O. Kuntze with mycoflora isolated from tea environment and phyllosphere. J. Biol. Control 50, 205–210. doi: 10.18311/jbc/2012/3479
Tian, Y., Chen, Z., Huang, X., Zhang, L., Zhang, Z. (2020). Evaluation of botanicals for management of piercing-sucking pests and the effect on beneficial arthropod populations in tea trees Camellia sinensis (L.) O. Kuntze (Theaceae). J. Insect Sci. 20, 27. doi: 10.1093/jisesa/ieaa101
Trivedi, P., Leach, J. E., Tringe, S. G., Sa, T., Singh, B. K. (2020). Plant–microbiome interactions: from community assembly to plant health. Nat. Rev. Microbiol. 18, 607–621. doi: 10.1038/s41579-020-0412-1
Uribe, D., Martinez, W., Cerón, J. (2003). Distribution and diversity of cry genes in native strains of Bacillus thuringiensis obtained from different ecosystems from Colombia. J. Invertebrate Pathol. 82, 119–127. doi: 10.1016/S0022-2011(02)00195-7
Vimala Devi, P. S., Duraimurugan, P., Poorna Chandrika, K. S. V., Vineela, V., Hari, P. P (2020). Novel formulations of camellia sinensis var. kurstaki: an eco-friendly approach for management of lepidopteran pests. World J. Microbiol. Biotechnol. 36, 78. doi: 10.1007/s11274-020-02849-8
Wang, M., Cernava, T. (2020). Overhauling the assessment of agrochemical-driven interferences with microbial communities for improved global ecosystem integrity. Environ. Sci. Ecotechnology 4, 100061. doi: 10.1016/j.ese.2020.100061
Wang, H., Lu, Z., Keyhani, N. O., Deng, J., Zhao, X., Huang, S., et al. (2023). Insect fungal pathogens secrete a cell wall-associated glucanase that acts to help avoid recognition by the host immune system. PloS Pathog. 19, e1011578. doi: 10.1371/journal.ppat.1011578
Wang, Y.-C., Qian, W.-J., Li, N.-N., Hao, X.-Y., Wang, L., Xiao, B., et al. (2016a). Metabolic Changes of Caffeine in Tea Plant (Camellia sinensis (L.) O. Kuntze) as Defense Response to Colletotrichum fructicola. J. Agric. Food Chem. 64, 6685–6693. doi: 10.1021/acs.jafc.6b02044
Wang, Y.-N., Tang, L., Hou, Y., Wang, P., Yang, H., Wei, C.-L. (2016b). Differential transcriptome analysis of leaves of tea plant (Camellia sinensis) provides comprehensive insights into the defense responses to Ectropis oblique attack using RNA-Seq. Funct. Integr. Genomics 16, 383–398. doi: 10.1007/s10142-016-0491-2
Wang, X., Xue, Y., Han, M., Bu, Y., Liu, C. (2014). The ecological roles of Bacillus thuringiensis within phyllosphere environments. Chemosphere 108, 258–264. doi: 10.1016/j.chemosphere.2014.01.050
Wei, F., Hu, X., Xu, X. (2016). Dispersal of Bacillus subtilis and its effect on strawberry phyllosphere microbiota under open field and protection conditions. Sci. Rep. 6, 22611. doi: 10.1038/srep22611
Win, P. M., Matsumura, E., Fukuda, K. (2021). Effects of pesticides on the diversity of endophytic fungi in tea plants. Microb. Ecol. 82, 62–72. doi: 10.1007/s00248-020-01675-7
Wu, W., Jiang, X., Zhu, Q., Yuan, Y., Chen, R., Wang, W., et al. (2024a). Metabonomics analysis of the flavor characteristics of Wuyi Rock Tea (Rougui) with “rock flavor” and microbial contributions to flavor. Food Chem 450, 139376. doi: 10.1016/j.foodchem.2024.139376
Wu, Y., Tan, Y., Peng, Q., Xiao, Y., Xie, J., Li, Z., et al. (2024b). Biocontrol potential of endophytic bacterium Bacillus altitudinis GS-16 against tea anthracnose caused by Colletotrichum gloeosporioides. Peerj 12, e16761. doi: 10.7717/peerj.16761
Xia, E. H., Zhang, H. B., Sheng, J., Li, K., Zhang, Q. J., Kim, C., et al. (2017). The tea tree genome provides insights into tea flavor and independent evolution of caffeine biosynthesis. Mol. Plant. 10, 866–877. doi: 10.1016/j.molp.2017.04.002
Xie, H., Chen, Z., Feng, X., Wang, M., Luo, Y., Wang, Y., et al. (2022). L-theanine exuded from Camellia sinensis roots regulates element cycling in soil by shaping the rhizosphere microbiome assembly. Sci. Total Environ. 837, 155801. doi: 10.1016/j.scitotenv.2022.155801
Xie, H., Feng, X., Wang, M., Wang, Y., Kumar Awasthi, M., Xu, P. (2020). Implications of endophytic microbiota in Camellia sinensis: a review on current understanding and future insights. Bioengineered 11, 1001–1015. doi: 10.1080/21655979.2020.1816788
Xin, W., Zhang, J., Yu, Y., Tian, Y., Li, H., Chen, X., et al. (2024). Root microbiota of tea plants regulate nitrogen homeostasis and theanine synthesis to influence tea quality. Curr. Biol. 34, 868–880.e6. doi: 10.1016/j.cub.2024.01.044
Xu, P., Fan, X., Mao, Y., Cheng, H., Xu, A., Lai, W., et al. (2022b). Temporal metabolite responsiveness of microbiota in the tea plant phyllosphere promotes continuous suppression of fungal pathogens. J. Advanced Res. 39, 49–60. doi: 10.1016/j.jare.2021.10.003
Xu, X.-M., Jeger, M. J. (2013). Theoretical modeling suggests that synergy may result from combined use of two biocontrol agents for controlling foliar pathogens under spatial heterogeneous conditions. Phytopathology® 103, 768–775. doi: 10.1094/PHYTO-10-12-0266-R
Xu, H.-X., Qian, L.-X., Wang, X.-W., Shao, R.-X., Hong, Y., Liu, S.-S., et al. (2019). A salivary effector enables whitefly to feed on host plants by eliciting salicylic acid-signaling pathway. Proc. Natl. Acad. Sci. U.S.A. 116, 490–495. doi: 10.1073/pnas.1714990116
Xu, N., Qu, Q., Zhang, Z., Yuan, W., Cui, H., Shen, Y., et al. (2020). Effects of residual S-metolachlor in soil on the phyllosphere microbial communities of wheat (Triticum aestivum L.). Sci. Total Environ. 748, 141342. doi: 10.1016/j.scitotenv.2020.141342
Xu, P., Stirling, E., Xie, H., Li, W., Lv, X., Matsumoto, H., et al. (2023b). Continental scale deciphering of microbiome networks untangles the phyllosphere homeostasis in tea plant. J. Advanced Res. 44, 13–22. doi: 10.1016/j.jare.2022.04.002
Xu, G., Ying, F., Wu, H., Tang, X. (2023a). Biocontrol potential of two deep-sea microorganisms against gray blight disease of tea. Egypt J. Biol. Pest Co 33, 53. doi: 10.1186/s41938-023-00701-3
Xu, N., Zhao, Q., Zhang, Z., Zhang, Q., Wang, Y., Qin, G., et al. (2022a). Phyllosphere microorganisms: sources, drivers, and their interactions with plant hosts. J. Agric. Food Chem. 70, 4860–4870. doi: 10.1021/acs.jafc.2c01113
Yang, H., Jia, X., Gao, T., Gong, S., Xia, L., Zhang, P., et al. (2024). The CsmiR397a-CsLAC17 module regulates lignin biosynthesis to balance the tenderness and gray blight resistance in young tea shoots. Horticulture Res. 11, uhae085. doi: 10.1093/hr/uhae085
Yang, H., Li, J., Xiao, Y., Gu, Y., Liu, H., Liang, Y., et al. (2017). An integrated insight into the relationship between soil microbial community and tobacco bacterial wilt disease. Front. Microbiol. 8. doi: 10.3389/fmicb.2017.02179
Yang, H., Wang, Y., Li, L., Li, F., He, Y., Wu, J., et al. (2019). Transcriptomic and Phytochemical Analyses Reveal Root-Mediated Resource-Based Defense Response to Leaf Herbivory by Ectropis oblique in Tea Plant (Camellia sinensis). J. Agric. Food Chem. 67, 5465–5476. doi: 10.1021/acs.jafc.9b00195
Ye, M., Liu, M., Erb, M., Glauser, G., Zhang, J., Li, X., et al. (2021). Indole primes defence signalling and increases herbivore resistance in tea plants. Plant Cell Environ. 44, 1165–1177. doi: 10.1111/pce.13897
Ye, G.-Y., Xiao, Q., Chen, M., Chen, X., Yuan, Z., Stanley, D. W., et al. (2014). Tea: Biological control of insect and mite pests in China. Biol. Control 68, 73–91. doi: 10.1016/j.biocontrol.2013.06.013
Yu, Y., Gui, Y., Li, Z., Jiang, C., Guo, J., Niu, D. (2022). Induced systemic resistance for improving plant immunity by beneficial microbes. Plants (Basel) 11, 386. doi: 10.3390/plants11030386
Yu, Z., Yang, Z. (2020). Understanding different regulatory mechanisms of proteinaceous and non-proteinaceous amino acid formation in tea (Camellia sinensis) provides new insights into the safe and effective alteration of tea flavor and function. Crit. Rev. Food Sci. Nutr. 60, 844–858. doi: 10.1080/10408398.2018.1552245
Yuan, J., Zhao, J., Wen, T., Zhao, M., Li, R., Goossens, P., et al. (2018). Root exudates drive the soil-borne legacy of aboveground pathogen infection. Microbiome 6, 156. doi: 10.1186/s40168-018-0537-x
Zeng, L., Jin, S., Xu, Y.-Q., Granato, D., Fu, Y.-Q., Sun, W.-J., et al. (2024). Exogenous stimulation-induced biosynthesis of volatile compounds: Aroma formation of oolong tea at postharvest stage. Crit. Rev. Food Sci. Nutr. 64, 76–86. doi: 10.1080/10408398.2022.2104213
Zeng, L., Wang, X., Xiao, Y., Gu, D., Liao, Y., Xu, X., et al. (2019a). Elucidation of (Z)-3-hexenyl-β-glucopyranoside enhancement mechanism under stresses from the oolong tea manufacturing process. J. Agric. Food Chem. 67, 6541–6550. doi: 10.1021/acs.jafc.9b02228
Zeng, L., Watanabe, N., Yang, Z. (2019b). Understanding the biosyntheses and stress response mechanisms of aroma compounds in tea (Camellia sinensis) to safely and effectively improve tea aroma. Crit. Rev. Food Sci. Nutr. 59, 2321–2334. doi: 10.1080/10408398.2018.1506907
Zeng, L., Zhou, X., Liao, Y., Yang, Z. (2021). Roles of specialized metabolites in biological function and environmental adaptability of tea plant (Camellia sinensis) as a metabolite studying model. J. Adv. Res. 34, 159–171. doi: 10.1016/j.jare.2020.11.004
Zhang, B., Bai, Z., Hoefel, D., Tang, L., Yang, Z., Zhuang, G., et al. (2008). Assessing the impact of the biological control agent Bacillus thuringiensis on the indigenous microbial community within the pepper plant phyllosphere. FEMS Microbiol. Lett. 284, 102–108. doi: 10.1111/j.1574-6968.2008.01178.x
Zhang, L., Cao, Q.-Q., Granato, D., Xu, Y.-Q., Ho, C.-T. (2020b). Association between chemistry and taste of tea: A review. Trends Food Sci. Tech 101, 139–149. doi: 10.1016/j.tifs.2020.05.015
Zhang, J., Yu, Y., Qian, X., Zhang, X., Li, X., Sun, X. (2024). Recent advances in the specialized metabolites mediating resistance to insect pests and pathogens in tea plants (Camellia sinensis). Plants 13, 323. doi: 10.3390/plants13020323
Zhang, Q., Zhang, Y., Wang, Y., Lin, S., Chen, M., Cheng, P., et al. (2023). Effects of pruning on tea tree growth, tea quality, and rhizosphere soil microbial community. Microbiol. Spectr. 11, e01601–e01623. doi: 10.1128/spectrum.01601-23
Zhang, J., Zhang, X., Ye, M., Li, X.-W., Lin, S.-B., Sun, X.-L. (2020a). The Jasmonic Acid Pathway Positively Regulates the Polyphenol Oxidase-Based Defense against Tea Geometrid Caterpillars in the Tea Plant (Camellia sinensis). J. Chem. Ecol. 46, 308–316. doi: 10.1007/s10886-020-01158-6
Zhao, J., Chen, Y., Keyhani, N. O., Wang, C., Li, Y., Pu, H., et al. (2023a). Isolation of a highly virulent Metarhizium strain targeting the tea pest, Ectropis obliqua. Front. Microbiol. 14. doi: 10.3389/fmicb.2023.1164511
Zhao, X., Chen, S., Wang, S., Shan, W., Wang, X., Lin, Y., et al. (2020b). Defensive responses of tea plants (Camellia sinensis) against tea green leafhopper attack: A multi-omics study. Front. Plant Sci. 10. doi: 10.3389/fpls.2019.01705
Zhao, S., Cheng, H., Xu, P., Wang, Y. (2023b). Regulation of biosynthesis of the main flavor-contributing metabolites in tea plant (Camellia sinensis): A review. Crit. Rev. Food Sci. Nutr. 63, 10520–10535. doi: 10.1080/10408398.2022.2078787
Zhao, J., Li, P., Xia, T., Wan, X. (2020a). Exploring plant metabolic genomics: chemical diversity, metabolic complexity in the biosynthesis and transport of specialized metabolites with the tea plant as a model. Crit. Rev. Biotechnol. 40, 667–688. doi: 10.1080/07388551.2020.1752617
Zhou, L., Li, X., Kotta-Loizou, I., Dong, K., Li, S., Ni, D., et al. (2021). A mycovirus modulates the endophytic and pathogenic traits of a plant associated fungus. ISME J. 15, 1893–1906. doi: 10.1038/s41396-021-00892-3
Zhou, S., Lou, Y.-R., Tzin, V., Jander, G. (2015). Alteration of plant primary metabolism in response to insect herbivory. Plant Physiol. 169, 1488–1498. doi: 10.1104/pp.15.01405
Zhou, Q., Zhao, S., Zhu, J., Li, F., Tong, W., Liu, S., et al. (2020). Transcriptomic analyses reveal a systemic defense role of the uninfested adjacent leaf in tea plant (Camellia sinensis) attacked by tea geometrids (Ectropis obliqua). Genomics 112, 3658–3667. doi: 10.1016/j.ygeno.2020.03.007
Zhu, J., He, Y., Yan, X., Liu, L., Guo, R., Xia, X., et al. (2019). Duplication and transcriptional divergence of three Kunitz protease inhibitor genes that modulate insect and pathogen defenses in tea plant (Camellia sinensis). Hortic. Res. 6, 126. doi: 10.1038/s41438-019-0208-5
Keywords: tea, biotic stress, biological control, metabolomics, tea quality
Citation: Xie Y, Cao C, Huang D, Gong Y and Wang B (2025) Effects of microbial biocontrol agents on tea plantation microecology and tea plant metabolism: a review. Front. Plant Sci. 15:1492424. doi: 10.3389/fpls.2024.1492424
Received: 06 September 2024; Accepted: 26 December 2024;
Published: 20 January 2025.
Edited by:
Ankush Prasad, Palacký University Olomouc, CzechiaReviewed by:
Sudipta Sankar Bora, Assam Agricultural University, IndiaChanglong Shu, Institute of Plant Protection (CAAS), China
Deepak Rathi, Palacký University, Czechia
Copyright © 2025 Xie, Cao, Huang, Gong and Wang. This is an open-access article distributed under the terms of the Creative Commons Attribution License (CC BY). The use, distribution or reproduction in other forums is permitted, provided the original author(s) and the copyright owner(s) are credited and that the original publication in this journal is cited, in accordance with accepted academic practice. No use, distribution or reproduction is permitted which does not comply with these terms.
*Correspondence: Yan Gong, Z29uZ3lhbkBuYmVyYy5jb20=; Beibei Wang, d2FuZ2JlaV96anVAMTYzLmNvbQ==