- College of Horticulture, Sichuan Agricultural University, Chengdu, China
The phenylacetone pathway, which encompasses flavonoids, lignin, and other compounds, is of paramount importance in determining the quality of pear fruit. Nevertheless, the precise regulatory functions of R2R3-MYB transcription factors in the metabolic pathways that regulate pear color changes remain unclear. In this study, we isolated an R2R3-PbMYB5(PbMYB5) transcription factor from ‘Red Zaosu’ pears and demonstrated that it influenced the expression of several genes, including PbCAD1, PbF5H, PbLAR, PbANR, and PbUFGT. The overexpression of PbMYB5 resulted in a notable elevation in anthocyanin concentration within the pear epidermis. Further research has shown that PbMYB5 is able to bind to PbANS and also has interactions with PbbHLH3 and PbbHLH33.We proposed that PbMYB5 forms a complex with PbbHLH3, PbbHLH33, and PbWD40 to activate PbANS and promote anthocyanin accumulation. This study offers new insights into the regulation of various metabolic pathways that impact fruit coloration.
1 Introduction
As a global fruit, pears (Pyrus spp.) have great economic and food value due to their unique flavor, rich nutritional value, and diverse pigmentation (Wu et al., 2013). Anthocyanosides are important flavonoids that are not only strong antioxidants or free radical scavengers in plants that resist adversity and aid in plant reproduction but may also be important in improving pear fruit quality (Shang et al., 2011; Wen et al., 2020; Yousuf et al., 2016; Zhai et al., 2014). Anthocyanins and PAs, the main components of anthocyanidins, significantly influence the color formation of the pear skin. Moreover, lignin, a compound of the phenylpropane pathway, plays an important role in pear hardness, crispness and flavour formation. However, it is noteworthy that there is substrate competition between lignin, PAs, and anthocyanin biosynthesis. Therefore, exploring the homeostatic equilibrium of metabolite synthesis via different pathways is a priority for research on pear color formation and germplasm innovation.
Most of the biosynthesis through the phenylpropanoid pathway is well understood, and many substrates are common to the lignin, anthocyanin, and PAs biosynthetic pathways. Cinnamoyl-coenzyme A reductase (CCR) and chalcone synthase (CHS) catalyze the movement of the common substrate p-coumaroyl-coenzyme A into the lignin, PA, and anthocyanidin pathways, respectively. Anthocyanins are synthesized by anthocyanin synthase (ANS) and UDP-glucoflavonoid-3-glucosyltransferase (UFGT), and PAs are synthesized by color less anthocyanin reductase (LAR) and anthocyanin reductase (ANR) (Zhang et al., 2020). Different biosynthetic pathways are regulated by structural gene expression and the MBW ternary complex composed of R2R3-MYB, bHLH, and WD40 transcription factors (TFs), with R2R3-MYB TFs playing a central role (Jiang et al., 2023). PyMYB10 and PyMYB10.1 not only activate the AtDFR promoter but also have the ability to bind to bHLH cofactors such as PybHLH, MrbHLH1, MrbHLH2, or AtbHLH2. This interaction forms a complex that further boosts AtDFR promoter activation, ultimately leading to the positive regulation of anthocyanin biosynthesis in pear (Jiang et al., 2023). The downregulation of PyMYB114 has been demonstrated to impede the synthesis of anthocyanin in red-skinned pears. Moreover, evidence suggests that the ERF/AP2 transcription factor PyERF3 interacts with PyMYB114 and its partner PybHLH3, thereby regulating anthocyanin production. Furthermore, the concomitant expression of PyMYB114 and PyMYB10 has been observed to enhance anthocyanin synthesis. PbMYB10b and PbMYB9 can regulate anthocyanins and PAs; MYB17 directly activates the structural genes involved in anthocyanin synthesis to regulate anthocyanin accumulation (Premathilake et al., 2020; Wang et al., 2020; Wang et al., 2017). VvMYB5a has been shown to activate the promoters of key genes in the general flavonoid pathway, such as VvANS, VvF3′5′H, and VvCHI, as well as the catechin-specific gene VvLAR1 (Deluc et al., 2006). Interestingly, it does not affect the promoter of the VvANR gene. When VvMYB5b was overexpressed in tobacco plants, it led to the increased expression of genes involved in the flavonoid pathway, resulting in higher levels of anthocyanins and PAs (Deluc et al., 2008). This demonstrates the ability of both VvMYB5a and VvMYB5b to activate the promoters of various structural genes in the grapevine flavonoid pathway (Deluc et al., 2006, 2008). R2R3-FaMYB5 has been proven to enhance the accumulation of anthocyanin and PA by activating F3’H and LAR. Additionally, it interacts with FaEGL3 and FaLWD1/FaLWD1-like to create the MYB-bHLH-WD40 complex (MBW), which enhances regulatory effectiveness (Jiang et al., 2023). The R2R3 MYB transcription factor, MYB6, promoted the biosynthesis of burlap anthocyanins and PAs, but inhibited burlap secondary cell wall formation (Wang et al., 2019a).
The analysis revealed that MYB5 is involved in the synthesis of multiple pathways in different species. Simultaneously, during the ‘Red Zaosu’ pear fruit development process, there is a difference in peel color changes (As the fruit matures, a distinct yellow-green hue emerges on the surface of the pericarp, gradually becoming more pronounced over time.). An R2R3-MYB-mediated homeostatic regulation of lignin, PAs, and anthocyanin biosynthesis was not identified. Therefore, we proposed to investigate: (1) The color change in the pericarp of ‘Red Zaosu’ pears is a result of disruptions in the multi-pathway metabolism caused by competition between lignin, anthocyanin, and PA for substrates. (2) The transcription factor MYB5 in the pericarp of ‘Red Zaosu’ pears plays a role in regulating the multi-pathway metabolism, and the interconnected regulation influences the color change in the pericarp. Based on this, we used the ‘Red Zaosu’ pear pericarp as the research object, MYB5 identified as the target transcription factor, and forward and reverse genetics techniques to reveal the above problems and provide a theoretical foundation for pear color research and molecular breeding.
2 Materials and methods
2.1 Plant material
The ‘Red Zaosu’ and ‘Zaosu’ pear trees were planted in the Pear Germplasm and Innovative Resources Nursery at the Chongzhou Modern Agriculture R&D Base of Sichuan Agricultural University, located at 103°64’52’’ E and 30°55’67’’ N. The trees were of similar age, growth potential, and management level. Tobacco (Nicotiana benthamiana) was cultured under controlled environmental conditions in the Materials Room of the Plant Molecular Genetic Breeding and Biotechnology Laboratory, College of Horticulture, Sichuan Agricultural University, China, for subcellular localisation, dual luciferase experiments, and BiFC tests. The temperature was maintained at 23 ± 2°C, with a relative humidity of 80%–90% and a light-dark cycle of 16/8 h. The light intensity was 220 μmol m-2s-1. ‘Red Zaosu’ stems, leaves, buds, flowers, fruits, seeds at various developmental stages, and pear pericarp samples were collected at 25, 45, 65, 85, 105, and 115 Day after anthesis (DAF). The pear fruits were harvested, and the epidermis was removed using a scalpel. All samples were immediately frozen in liquid nitrogen and stored at –80°C. The samples were then sorted by tissue type. The ‘Zaosu’ and ‘Red Zaosu’ pears were bagged 15 d after flowering. The fruit was then collected at 30 and 90 d after bagging (DAB) for the instantaneous injection test.
2.2 RNA extraction and RNA sequencing
The epidermis of different regions of the fruit at 65, 85 and 105 DAF was used as the material, total RNA was extracted using the CTAB-based method (Gambino and Gribaudo, 2012) and transcribed into cDNA. The quality of the total RNA was assessed, and BioMarker company (Qingdao, China) was used to construct and sequence the libraries. Each sample included three biological replicates. The sequencing reads were analyzed using our previously described procedures (www.biocloud.net).
2.3 Gene cloning and molecular bioinformatics analysis
The primers listed in Supplementary Table S1 were used to amplify and clone the genes into the pBlunt vector (Yeasen Biotechnology, Shanghai) using the mined gene sequences. The sequence information was determined by comparing and analyzing the cloned sequences in the Genome Database for Rosaceae (GDR) and National Center For Biotechnology Information databases (NCBI). The MYB structural domains were analyzed using the Pfam (pfam.xfam.org) and ESPript 3.0 online websites (Jiang et al., 2023). The ClustalW program in the MEGA 6.06 package was used for multiple sequence comparisons. A phylogenetic tree was constructed using the neighbor-joining method with 1000 bootstrap repeats.
2.4 Transient expression and stable transformation assays
The CDS sequence of PbMYB5 was cloned into the expression vector pCAMBIA-35SN (35S::PbMYB5), and the RNA interference (RNAi) vector pRNAi-35SN (RNAi::PbMYB5). Transient overexpression and silencing were performed in pear fruit per the described protocol (Cong et al., 2021; Ni et al., 2023; Yao et al., 2017; Zhai et al., 2016). Each replicate included at least 10 fruits (30 and 90 DAB), with three biological replicates. The injected fruits were harvested after seven days, and the peel of the injected area was scraped using a scalpel. The harvested peel was stored at –80°C before use. For stable genetic transformation of ‘Red zaosu’ and ‘Zaosu’ healing tissues, the 35S::PbMYB5 and RNAi::PbMYB5 constructs were transformed into Agrobacterium tumefaciens GV3101. Pear healing tissues were infiltrated following the previously described method (Ni et al., 2023). Anthocyanoside synthesis was induced by culturing positive healing tissues in the dark for two weeks, followed by continuous light treatment for 96 h.
2.5 RT-qPCR assay
SYBR Green (TaKaRa, Dalian, China) was used to detect the PCR products on a CFX96 real-time reaction system (Bio-Rad). The housekeeping gene JN684184 (Actin) was selected and analyzed using the 2-ΔΔCt method (Bai et al., 2019). The qPCR primers are listed in Supplementary Table S2.
2.6 Anthocyanin and PAs detection
The total anthocyanin content was determined using the pH difference method (Ni et al., 2023; Yao et al., 2017). PAs were detected by reaction with p-dimethylaminocinnamaldehyde (DMACA) solution (0.1% [w/v] DMACA, 90% [v/v] ethanol, and 10% [w/v] HCl) to produce a blue color, and their concentrations were determined through full-wavelength zymography using the DMACA assay, as previously described (Zhang et al., 2022). Positive healing tissues were stained for PA using DMACA solution (0.2% [w/v] DMACA, methanol: 6M HCL = 1:1 [v/v]), immersed for 48 h, rinsed with distilled water, dried and observed (Zhang et al., 2022).
2.7 Subcellular localization and bimolecular fluorescence complementation assays
PbMYB5 was used to the N-terminus of eGFP in the pCAMBIA-35 S-eGFP vector (PbMYB5-eGFP) by homologous recombination. For BiFC, the target genes were cloned into vectors modified with pSAT1-nEYFP-C1 and pSAT1-cEYFP-N1 to produce fused YFP target proteins (Citovsky et al., 2006). The vector was transformed into the Agrobacterium strain GV3101. The cells were incubated overnight at 28°C in a YEP medium supplemented with the appropriate antibiotics. After centrifugation, the collected cells were resuspended in MMA solution (10 mM MES, 10 mM MgCl2, and 500 μM acetosyringone) and incubated at room temperature for 1 h on a shaker. The bacteria were suspended in fresh buffer and adjusted to a final density of OD 600 = 1. The suspension was injected into the dorsal axial lateral chloroplasts of the tobacco leaves using a syringe. The treated plants were kept in a greenhouse for 2–3 d and then observed under a confocal microscope (Olympus FV1000). The primers used to construct the vectors are listed in Supplementary Table S1.
2.8 Yeast two-hybrid analysis
Two target genes were cloned into the pGADT7 and pGBKT7 vectors, and positive interactions were detected using the SD/-Trp/-Leu and SD/-Trp/-Leu/-Ade/-His/+ X-α-gal/+3-AT medium. Supplementary Table S1 lists all primers used for vector construction.
2.9 Yeast one-hybrid analysis
Genes were cloned into the pGADT7 vectors, target gene promoter cloning in pHIS vector, and positive interactions were detected using the SD/-Trp, and SD/-His/-Trp + 3AT, and SD/-Trp/-Leu/-His + 3-AT medium. Supplementary Table S1 lists all primers used for vector construction.
2.10 Dual luciferase assay
To construct the reporter gene, promoter sequences of CHS, CHI, F3H, DFR, LAR, ANR, and UFGT were cloned from the ‘Red Zaosu’ pericarp and inserted into the pGreenII0800-LUC vector. 35S::PbMYB5 was used as an effector. Empty pCAMBIA-35SN was used as a negative control. The vector was transformed into Agrobacterium GV3101 (pSoup-p19). Dual-luciferase transient expression assays were performed as described (Liu et al., 2008). Firefly luciferase (LUC) and Renilla luciferase (REN) were detected using the Dual-Luciferase Reporter Gene Assay Kit (Yeasen Biotechnology, Shanghai) according to the manufacturer’s instructions. Supplementary Table S1 lists all primers used for vector construction.
2.11 Statistical analysis
Unless otherwise noted, all data were analyzed using the IBM SPSS Statistics 23 software. Statistically significant differences between samples were determined using Student’s t-test (P < 0.05).
3 Results
3.1 Bioinformatic identification and analysis of the R2R3 transcription factor PbMYB5
The transcriptome of ‘Red Zaosu’ pear epidermis was sequenced in the red region (RS) and in the red-yellow-green gradient region (GS) at 65, 85 and 105 d after anthesis, and KEGG and GO enrichment analyses showed that differentially differentiated genes were enriched in the phenylpropanoid pathway, with one of the genes 2459, having a significantly higher abundance in the RS than in the GS region at 65 d after anthesis and no significant difference in its abundance at 85 and 105 d (Supplementary Figure S1). By searching the GDR database, we found that the sequence of gene 2459 was highly similar to that of rna3106-v1.1-pbr and rna18247-v1.1-pbr in white pear, located on chromosome 3 and chromosome 11, with amino acid sequence lengths of 373 aa and 375 aa, respectively. Further sequence comparison showed that the sequence of gene 2459 had the highest homology with rna3106-v1.1-pbr (Supplementary Figure S2). The gene was therefore cloned from the peel of the ‘Red Zaosu’ pear and compared with the NCBI database, and it was found that several genes with high sequence similarity in different species belonged to the MYB family. Subsequent amino acid sequence conservation analyses showed that the ‘Red Zaosu’ pear skin gene 2459, like other MYB genes, has a complete R2R3 conserved structural domain (Figure 1A). Furthermore, a phylogenetic tree demonstrated that this gene was closely related to the predicted transcription repressor factor MYB5 in ‘Hongxiangsu’ pear and MdMYB5 in apple, support level of up to 99 (Figure 1B). Consequently, it was designated PbMYB5. Bioinformatics analysis revealed that the CDS sequence of PbMYB5 was 1122 bp long and encoded a 373 amino acid protein. The amino acid sequence contained a highly conserved bHLH interaction motif (DLx2Rx3Lx6Lx3R) in the R3 structural domain, and no anthocyanin characterization motifs (ANDV and KPRPRS/TF) or inhibition motifs (LxLxL and TLLLFR) were found (Stracke et al., 2001). PbMYB5 belongs to a remote clade with PbMYB1, PbMYB10, and PbMYB114. Quantitative gene expression analysis revealed that PbMYB5 expression was higher in the leaves, buds, and flowers (Figure 1D). In the pericarp at different developmental stages, the highest expression of PbMYB5 was observed at 45 DAF by 105 and 115 DAF (Figure 1C). The subcellular localisation analysis revealed that PbMYB5 was present in both the nucleus and cytoplasm (Figure 1E).
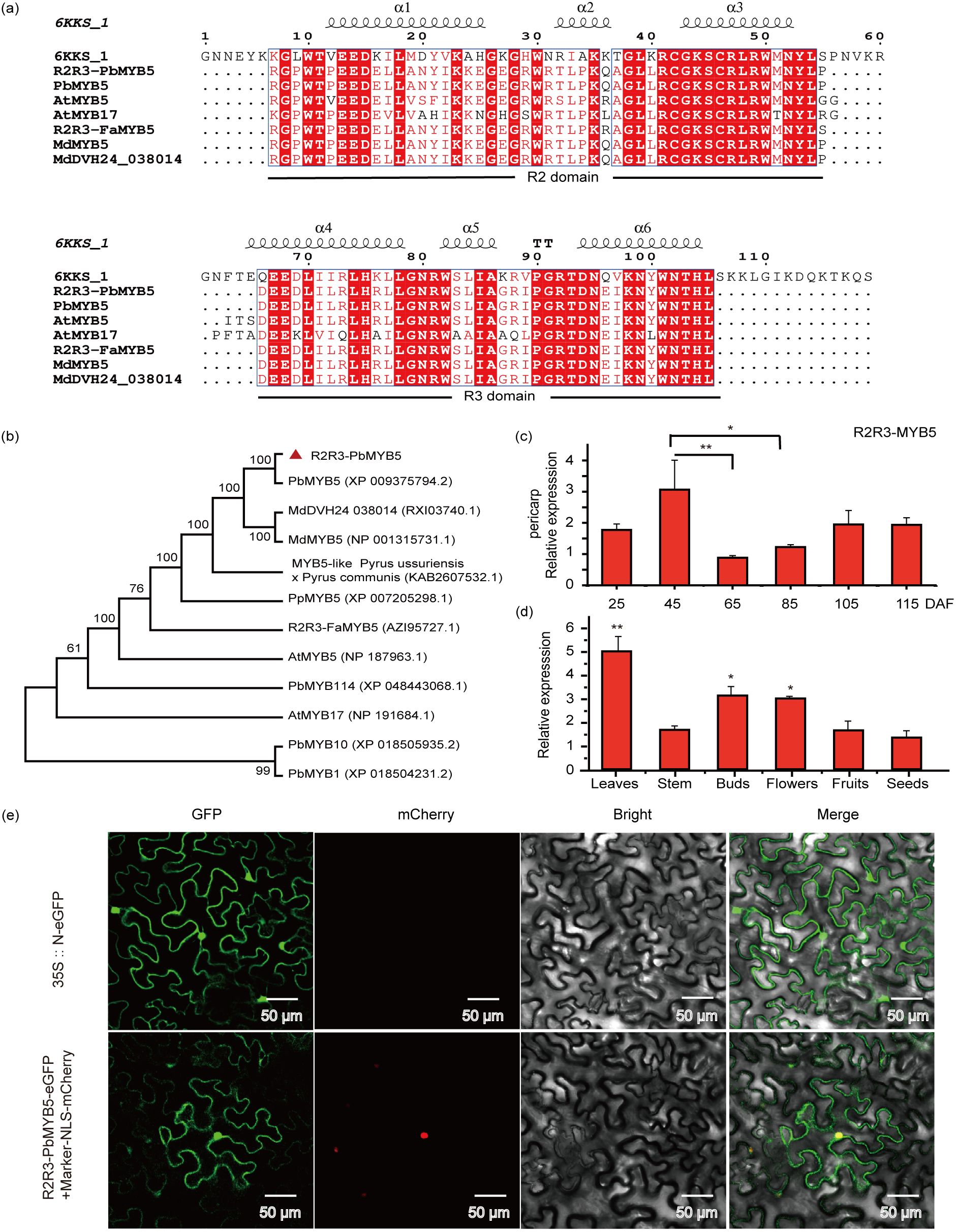
Figure 1. Bioinformatic analysis and subcellular localization of PbMYB5. (A) Amino acid sequence comparison and analysis of conserved structural domains. Multiple sequence alignments of MYB5 were performed through ClustalW and the phylogenetic tree was constructed using the neighbor-joining method with 1,000 bootstrap replicates. Accession IDs (GenBank): PbMYB5 (Pyrus x bretschneideri), XP_009375794.2; MdDVH24_038014 (Malus domestica), RXI03740.1; MdMYB5 (Malus domestica), NP_001315731.1; MYB5-like (Pyrus ussuriensis x Pyrus communis), KAB2607532.1; PpMYB5 (Prunus persica), XP_007205298.1; VvMYB5a (Vitis vinifera), RVW48712.1; VvMYB5b (Vitis vinifera), NP_001267854.1; R2R3-FaMYB5 (Fragaria x ananassa), AZI95727.1; PtMYB5 (Populus tomentosa), KAG6787562.1; PtMYB6 (Populus tomentosa), AHH34325.1; AtMYB5 (Arabidopsis thaliana), NP_187963.1; AtMYB17 (Arabidopsis thaliana), NP_191684.1; PbMYB114 (Pyrus x bretschneideri), XP_048443068.1; PbMYB10 (Pyrus x bretschneideri), XP_018505935.2; PbMYB1 (Pyrus x bretschneideri), XP_018504231.2. (B) Phylogenetic analysis of PbMYB5 with different species. (C) Expression of the PbMYB5 gene in various tissues. (D) Expression of the PbMYB5 gene in the pericarp was measured at various developmental stages. (E) Subcellular localization of PbMYB5. *p<0.05, **p<0.01.
3.2 PbMYB5 inhibits lignin and PAs synthesis and promotes anthocyanin synthesis in pear pericarp
The 35S::PbMYB5 overexpression vector and 35S::N empty vector were transiently injected into ‘Zaosu’ pear fruits using Agrobacterium transformation. Injections were administered 30 and 90 d after bagging, respectively. After one week of exposure, significant red accumulations were observed in both injected 35S::N. After one week of treatment, significant red accumulation was observed in all regions injected with 35S::PbMYB5, whereas no red color accumulation was observed after 35S::N injection (Figure 2A). Examination of the epidermis injected with 35S::PbMYB5 and 35S::N vectors revealed that 35S::PbMYB5 increased the expression of PbMYB5. Expression was higher at 30 d after bagging than at 90 d (Figure 2B). Additionally, the expression of lignin synthesis genes PbCAD1 and PbF5H, as well as the PAs synthesis gene PbLAR, was significantly lower than that of 35S::N during both stages. Conversely, after the injection of 35S::PbMYB5, the expression of the PbUFGT was significantly higher than that of 35S::N (Figure 2C). The results of the total anthocyanin and PAs content of the injected pericarp revealed that the anthocyanin content was higher than 35S::N and the PAs content was lower than 35S::N after injection of 35S::PbMYB5 at 30 and 90 DAB, respectively (Figure 2D, E).
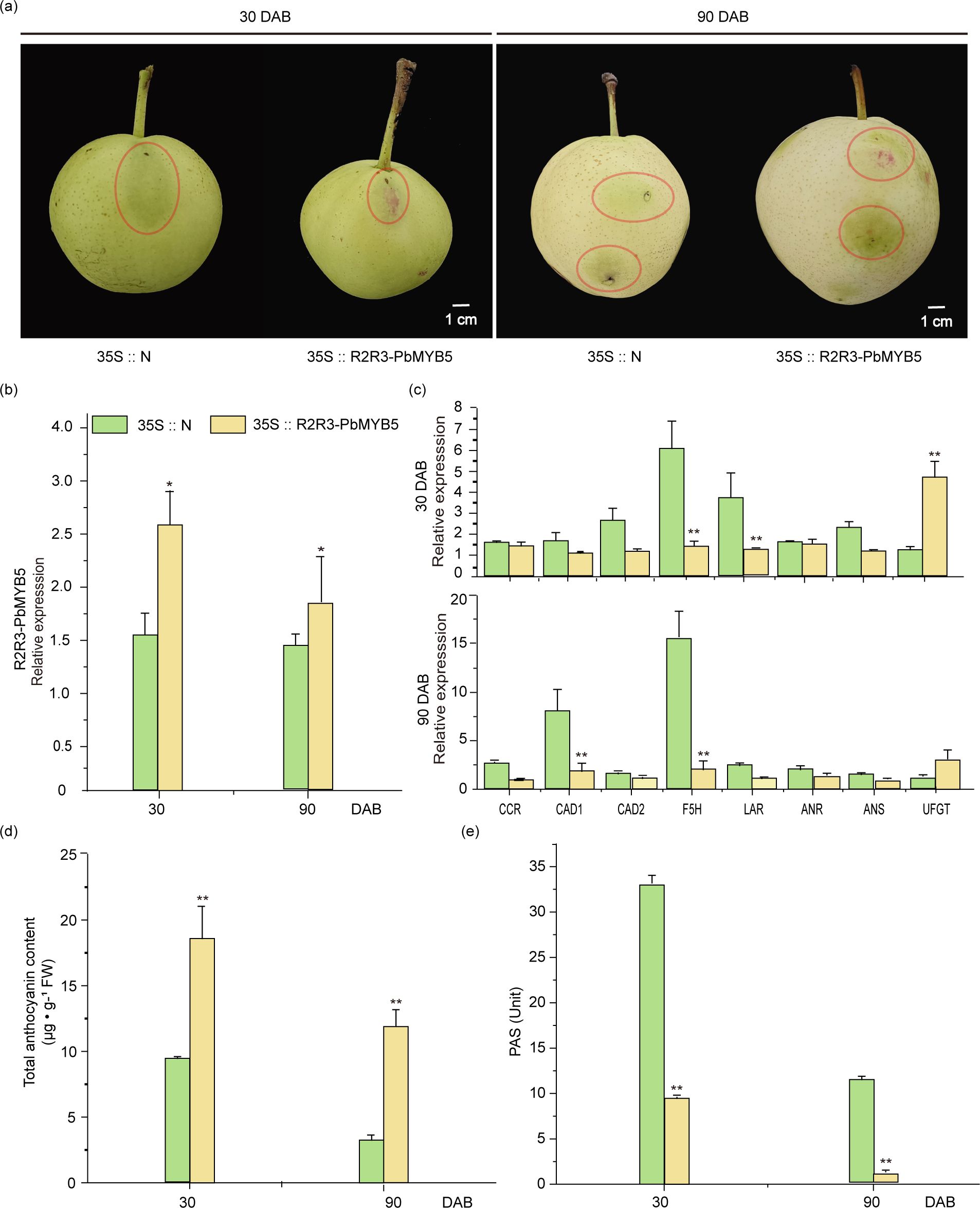
Figure 2. Phenotypes and associated gene expression after 35S::PbMYB5 injection in ‘Zaosu’ pears at different time points. (A) Overexpression of PbMYB5 at different times and transient injection into ‘Zaosu’ pear fruit. The area where the injection is to be administered is indicated by the circle. (B) Lignin, PA and anthocyanin-related gene expression after overexpression of PbMYB5. (C) Expression of lignin, PAs and anthocyanin-related genes after overexpression of PbMYB5. (D) Anthocyanins after overexpression of PbMYB5. (E) PAs after overexpression of PbMYB5. *p<0.05, **p<0.01.
3.3 RNAi of PbMYB5 promotes lignin and PA synthesis while suppressing anthocyanin synthesis in the pericarp of ‘Red Zaosu’
The PbMYB5 silencing vector (RNAi::PbMYB5) and the silencing null vector (RNAi::N) were transiently injected using Agrobacterium transformation into ‘Red Zaosu’ fruits at 30 and 90 d after bagging, respectively. After one week of exposure treatment, it was observed that none of the injected areas showed significant red accumulation after injection of RNAi::PbMYB5. There was no significant red accumulation in any of the injected areas and no inhibition of red accumulation in the vicinity of the RNAi::N injection (Figure 3A). Examination of the epidermis after RNAi::PbMYB5 and RNAi::N injections revealed that both RNAi::PbMYB5 phases inhibited PbMYB5 expression compared to RNAi::N (Figure 3B). Moreover, expression of lignin synthesis genes (PbCAD1, PbF5H) and PAs genes (PbLAR and PbANR) was higher than that of 35S::N, while the expression of anthocyanidin synthesis and transporter gene PbUFGT was significantly lower than that of 35S::N, after injection of RNAi::PbMYB5 (Figure 3C). Results of the total anthocyanin and PAs content of the injected pericarp revealed that the anthocyanin content was lower than that of RNAi::N and the PAs content was higher than 35S::N after injection of RNAi::PbMYB5 at 30 and 90 d after bagging, respectively (Figures 3D, E).
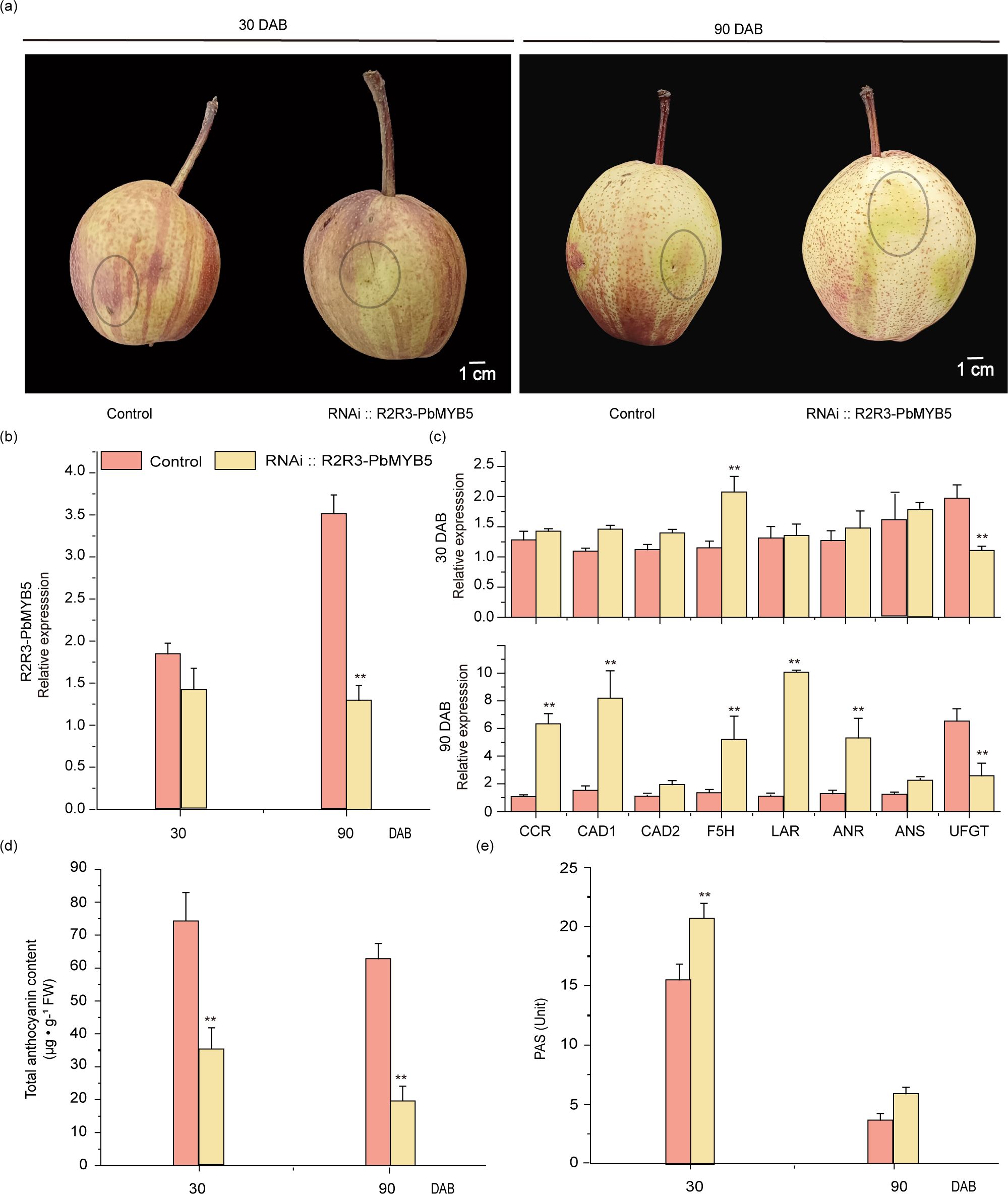
Figure 3. Phenotypes and associated gene expression after RNAi::PbMYB5 injection in ‘RedZaosu’ pears at different time points. (A) Silencing of PbMYB5 at different times for transient injection into ‘Red Zaosu’ pear fruit. The area where the injection is to be administered is indicated by the circle. (B) Gene expression after silencing PbMYB5. (C) Expression of lignin, PAs and anthocyanin-related genes after silencing of PbMYB5. (D) Anthocyanins content after silencing PbMYB5. (E) PAs after silencing PbMYB5. *p<0.05, **p<0.01.
3.4 Transformation of PbMYB5 and its effect on flavonoids in the pericarp of ‘Zaosu’ and ‘Red Zaosu’ pear during wound healing
The PbMYB5 overexpression vector (35S::PbMYB5) and the blank control overexpression vector (35S::N) were transformed into ‘Zaosu’ pear healing tissues. The positive healing tissue analysis revealed that 35S::PbMYB5 promoted PbMYB5 expression comparedto 35S::N (Figure 4B). Moreover, DMACA staining revealed that 35S::PbMYB5 suppressed the expression of proanthocyanidins (Figure 4A) and related genes PbLAR and PbANR, while promoted the expression of PbANS and PbUFGT (Figure 4C). The PbMYB5 silencing vector (RNAi::PbMYB5) and blank control silencing vector (RNAi::N) were transformed into ‘Red Zaosu’ pear healing tissues. Analysis of positive healing tissues showed that RNAi::PbMYB5 was able to repress PbMYB5 expression compared to control. (Figure 4E). Moreover, DMACA staining revealed that RNAi::PbMYB5 promoted the expression of PAs (Figure 4D) and related genes PbLAR and PbANR, in addition to promoting the expression of lignin synthesis genes PbCCR, PbCAD1, PbCAD2, and PbF5H, and reducing the expression of anthocyanin synthesis gene PbANS (the difference was not statistically significant) (Figure 4F).
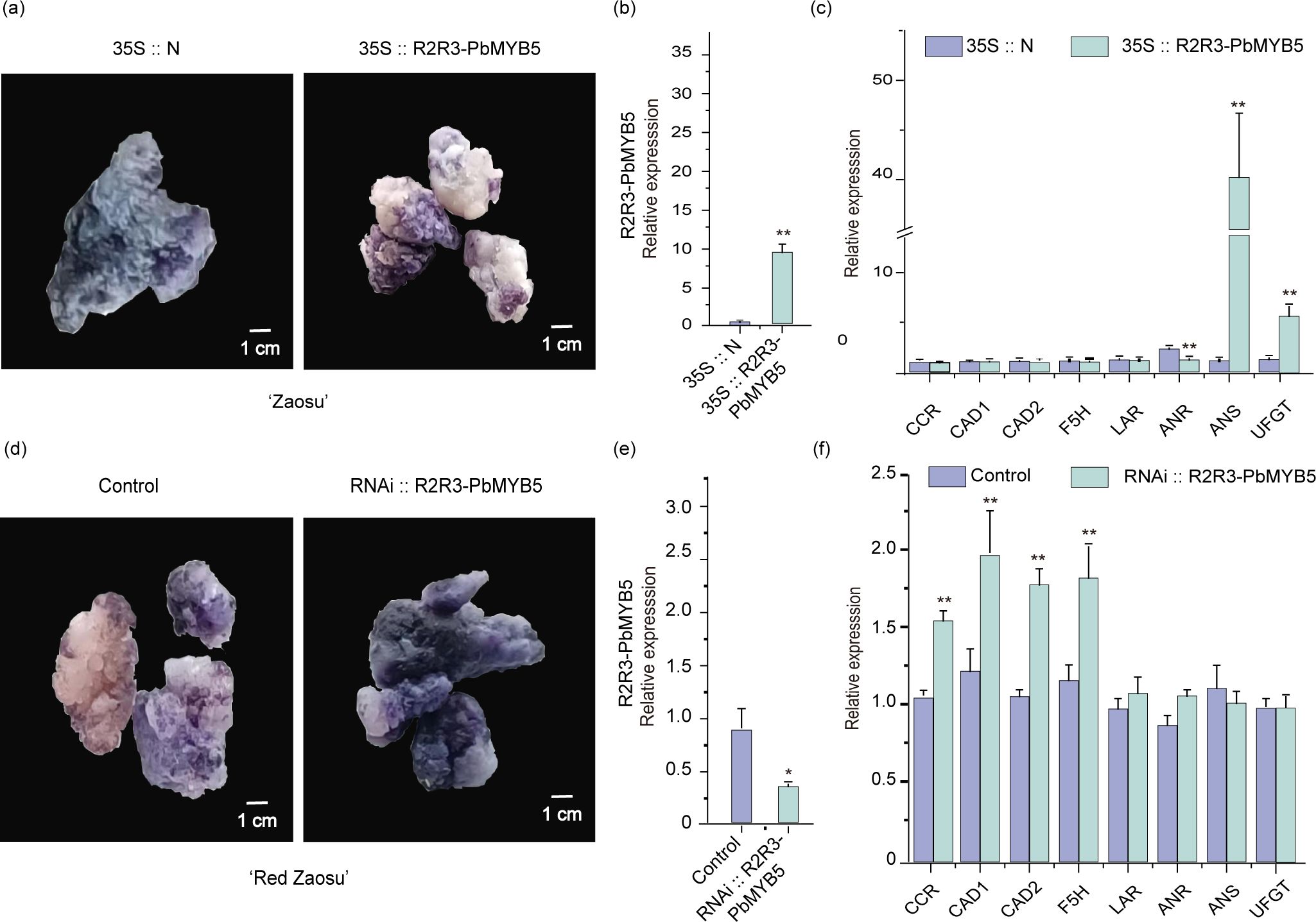
Figure 4. PbMYB5 transformation of ‘Zaosu’ and ‘Red Zaosu’ pears, healing, and DMACA staining. (A) PbMYB5 overexpression, ‘Zaosu’ pear healing DMACA staining phenotype. (B) Gene expression after PbMYB5 overexpression. (C) Lignin, PAs, and anthocyanin-related gene expression after PbMYB5 overexpression. (D) Silencing PbMYB5, ‘Red Zaosu’ pear healing DMACA staining phenotype. (E) Gene expression after silencing PbMYB5, (F) Lignin, PAs, and anthocyanin-related gene expression after silencing PbMYB5. *p < 0.05, **p < 0.01.
3.5 PbMYB5 forms a novel complex with bHLH3/bHLH33 for regulation of flavonoid metabolism
The yeast two-hybrid results (Figure 5) revealed that PbMYB5 can bind to PbHLH3/PbHLH33 and does not directly interact with PbWD40. The BiFC assay revealed that PbMYB5 interacts with PbbHLH3/PbbHLH33 in the nuclei of tobacco cells. This suggests that PbMYB5 forms a functional complex with PbbHLH3/PbbHLH33 and forms an MYB-bHLH-WD40 (MBW) complex with the stabilizing protein WD40, which in turn regulates the structural genes involved in the anthocyanin pathway and regulates the metabolic flux and accumulation of anthocyanins and PAs.
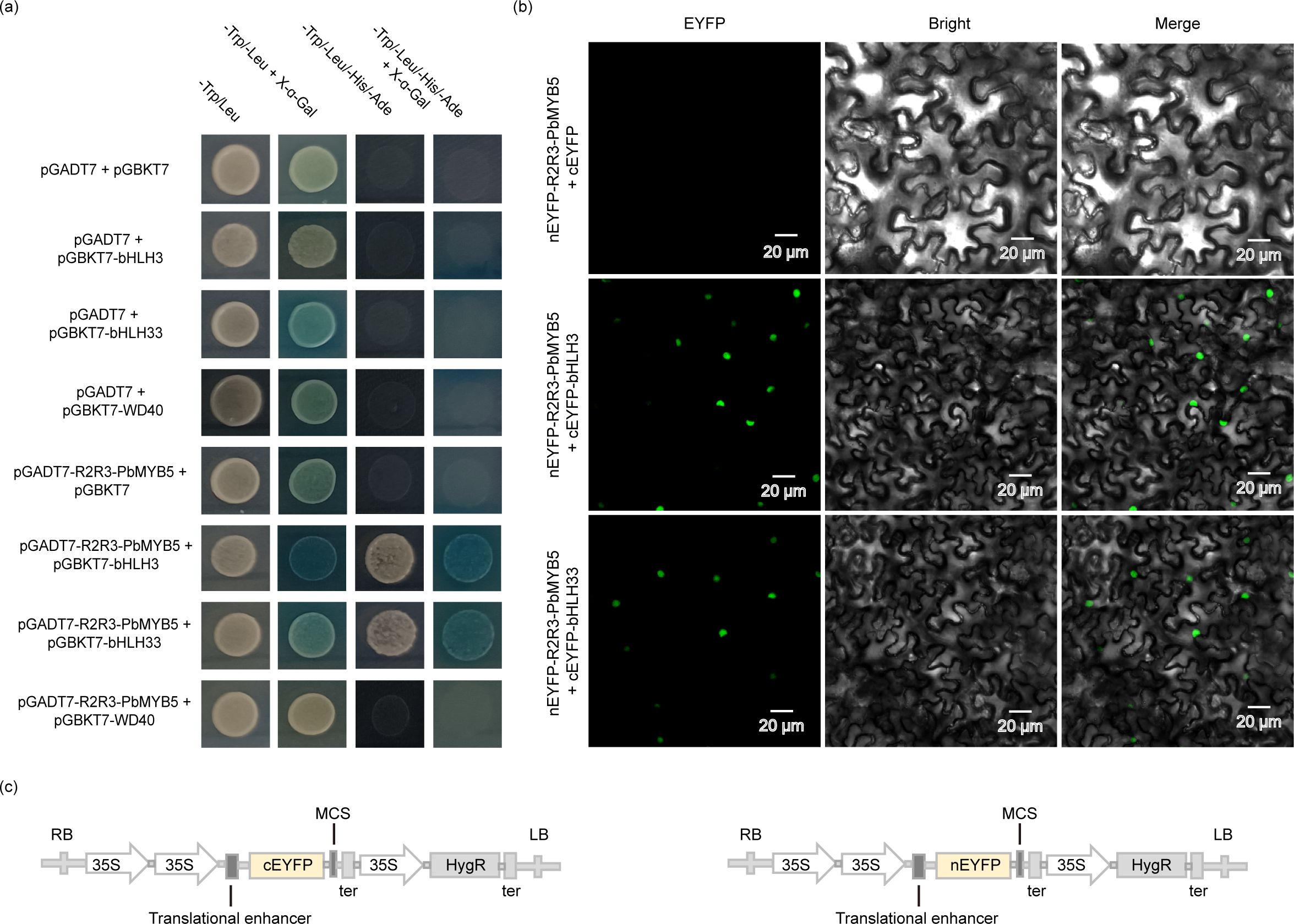
Figure 5. Validation of Yeast two-hybrid and BiFC interactions. (A) PbMYB5, bHLH, WD40 yeast two-hybrid validation. (B) BiFC experimental validation. (C) Schematic representation of BiFC vector expression pattern.
3.6 PbMYB5 has been demonstrated to bind to the PbANS promoter, thereby regulating anthocyanin metabolismPbMYB5
The Dual luciferase assay showed that PbMYB5 was not able to effectively activate the promoter activity of structural enzyme genes involved in the anthocyanin synthesis pathway (Figures 6A, B). The interaction between PbMYB5 and pro-PbANS was observed in a yeast one-hybrid assay (Figure 6C), suggesting that PbMYB5 may lack transcriptional activation abilities. To further support this idea, yeast self-activation assays were performed on PbMYB5, bHLH3/33, and WD40. It was found that, unlike bHLH33, PbMYB5, bHLH3, and WD40 did not exhibit self-activating properties (Figure 7).
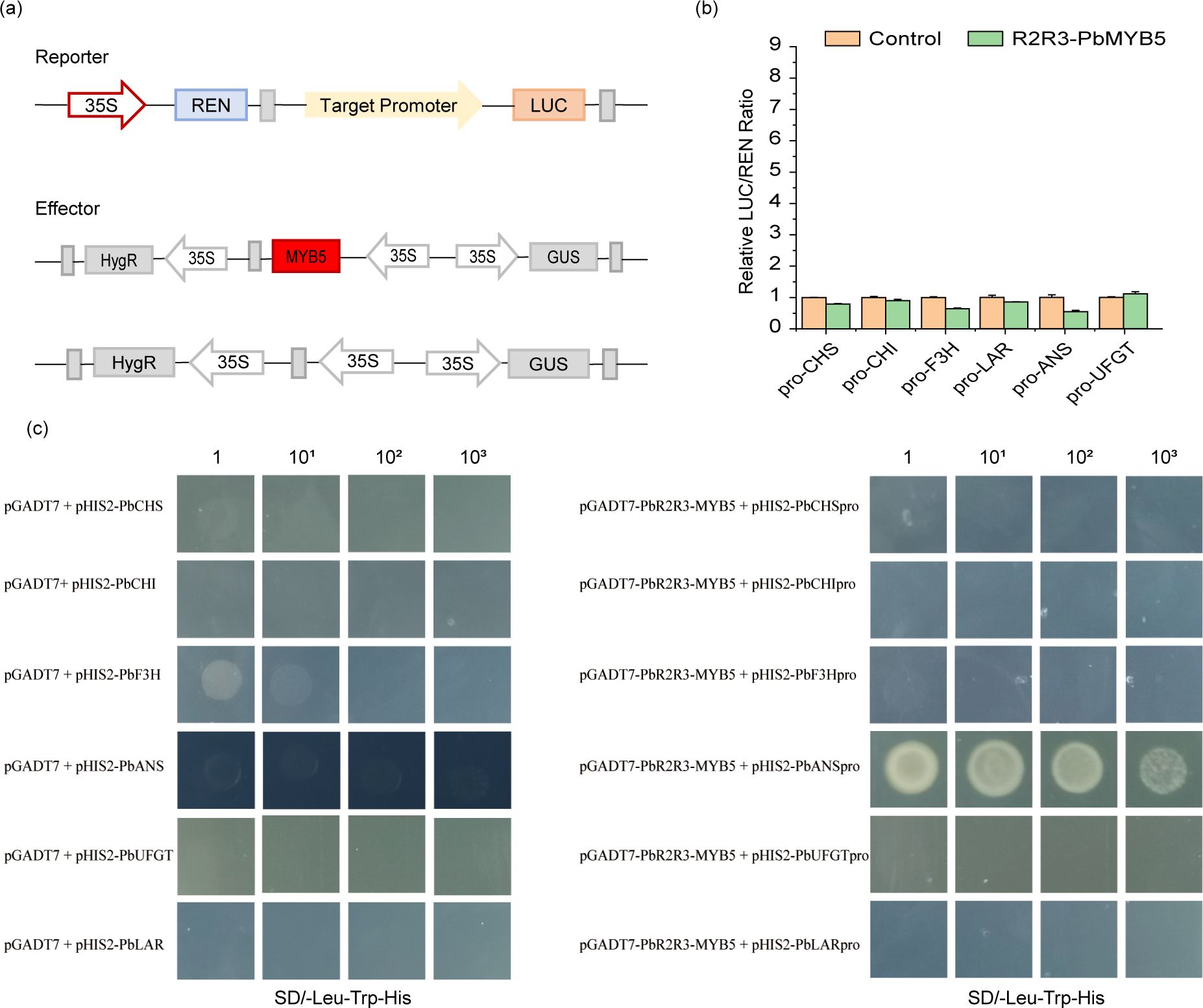
Figure 6. Complementary validation of the dual luciferase assay (A) Schematic representation of the PbMYB5 dual luciferase. (B) Validation of PbMYB5 interactions with the promoters of flavonoid pathway structural genes. (C) The objective of this study was to validate the interaction between PbMYB5 and the promoters of structural genes involved in flavonoid metabolism using a yeast one-hybrid system. 10X represents the number of dilutions of the bacterial solution.
4 Discussion
4.1 PbMYB5 affects pericarp coloration by regulating lignin and anthocyanoside metabolism
In the Pyrus bretschneideri genome (SAMN01797448 and SAMN12751541), PbMYB5 was predicted to be a transcriptional repressor. In this study, PbMYB5 was overexpressed in ‘Zaosu’ pear fruits and healing wounds and was found to promote reddening of the ‘Zaosu’ pear epidermis, promote anthocyanin accumulation, and regulate of PAs synthesis-related gene expression. Silencing the PbMYB5 gene in ‘Red Zaosu’ pear fruits and healing wounds inhibited reddening of the ‘Red Zaosu’ pear epidermis, suppressed anthocyanin accumulation. The PbMYB5 transcription factor promoted the accumulation of anthocyanins and regulated the accumulation of PAs in the pear epidermis, promoting the coloration of the pear epidermis. There are ongoing and well-defined studies on anthocyanin synthesis and metabolic regulation of fruit coloration. However, relatively little research has been done on transcriptional regulators that regulate crosstalk between different secondary metabolic pathways (e.g., anthocyanin, PAs, and lignin pathways). Photoinduction of apple pericarp promotes anthocyanin biosynthesis while reducing lignin production, indicating a negative correlation between anthocyanins and lignin and competition for metabolic flow (Hu et al., 2021). R2R3-MYB6 promotes the accumulation of anthocyanins and PAs and inhibits secondary metabolites like lignin during the growth and development of poplar trees (Wang et al., 2019a). The results of this study revealed that overexpression of PbMYB5 significantly increased the accumulation of red phenotypes in pear pericarp, decreasing the expression of the key enzyme genes for lignin synthesis, CCR, CAD1, and F5H and increasing the expression of the anthocyanin synthesis and transporter gene UFGT. Contrarily, silencing PbMYB5 prevented the accumulation of red phenotype in the pear pericarp. These results are consistent with those of MdMYB1, which promoted anthocyanin accumulation and inhibited lignin synthesis (Zhou et al., 2017).
VmMYBA1 and VmMYBA2 upregulated the expression of genes involved in the anthocyanin and PAs pathways in blueberries. Overexpression of MYB165 and MYB194 inhibited PAs and anthocyanin synthesis in poplar and regulated the synthesis and metabolism of other phenolic substances (Ma et al., 2018). Overexpression of VvMYC1 induced the accumulation of anthocyanins and PAs in grape berries. Furthermore, VvMYB5a overexpression increased the metabolism of anthocyanins, PAs, and lignin; VvMYB5b can induce the accumulation of anthocyanins and PAs derivatives, revealing a spatiotemporal specificity of VvMYB5a and VvMYB5b in regulating the accumulation of lignin, anthocyanins and PAs in grapevine fruits (Deluc et al., 2006, 2008). The R2R3-FaMYB5 TF regulates anthocyanin and PAs metabolism in strawberry fruit and transgenic tissues. The results of this study revealed that PbMYB5 expression was higher in pear fruits at 45 d after anthesis than at 105 and 115 d after anthesis and lower at 65 and 85 d after anthesis than at other times. These results are similar to those observed for grapes. PbMYB5 overexpression increased anthocyanin synthesis in pear pericarp, consistent with the results of MYB5 in strawberries and grapes. Nevertheless, in this study, PbMYB5 overexpression resulted in the suppression of lignin and PAs synthesis gene expression in pear pericarp, and a significant inhibition of PA synthesis in pear pericarp and healing tissues. These results revealed that PbMYB5 regulates the fluctuations of metabolic fluxes of different metabolic pathways and can affect pear skin color formation through metabolic flux competition.
4.2 PbMYB5 binds bHLH3/bHLH33 to form the MBW complex for the regulation of anthocyanin accumulation
Flavonoid pathways are generally influenced by the spatiotemporal expression specificity of their structural genes and, to a lesser extent, by regulation of the MBW complex (Gonzalez et al., 2008, 2010; Pu et al., 2021). In Arabidopsis, MYB113/114 forms a complex with TTG1 and bHLH to regulate the anthocyanin synthesis pathway in seedlings, whereas TT2-TT8-TTG1 regulates PAs in seeds (Gonzalez et al., 2008; Xu et al., 2013). R2R3-FaMYB5, FaEGL3, and FaLWD1/FaLWD1-like may form a new MBW complex that regulates flavonoid metabolism in strawberries, FaMYB9/FaMYB11-FabHLH3-FaTTG1 regulates the PAs pathway in fruits, and FaMYB10-FabHLH3/FabHLH33-FaTTG1 may regulate anthocyanin metabolism (Jiang et al., 2023). In apples, MdHB1 regulates anthocyanin synthesis by restricting MdMYB10, MdbHLH3, and MdTTG1 to the cytoplasm, indirectly repressing the transcription of MdDFR and MdUFGT (Jiang et al., 2017). A ternary complex formed by PyMYB10, PybHLH, and PyWD40 transcription factors regulates anthocyanin biosynthesis and accumulation in the ‘Yunnan Hong’ Pear (Cui et al., 2021). PpMYB114 and PpbHLH3 promote PpMYB114-induced PpUFGT expression and red color accumulation; anthocyanin accumulation is transcriptionally regulated by the MYB-bHLH-WD40 complex (Ni et al., 2019). In this study, we discovered that PbMYB5 could interact with bHLH3/bHLH33 but not with WD40. This result is consistent with previous reports that MYB transcription factors interact with bHLH3 to form MYB-bHLH and that WD40 acts as a stabilizing protein that interacts with bHLH, forming the MYB-bHLH-WD complex (Alabd et al., 2022; Cheng et al., 2014; Ni et al., 2021; Wang et al., 2019b; Ye et al., 2016). The spatiotemporal specificity of PbMYB5 contributes to the dynamics of PbMYB5-bHLH3/bHLH33-WD40 complex, which in turn inhibits the expression of lignogenic anthocyanin genes, reduces the flux of related metabolites, promotes the expression of anthocyanin-related genes and accumulation of anthocyanin glycosides, and influences the coloration of pear skin.
4.3 PbMYB5 induces the promoter activity of the anthocyanin structural gene and regulates the metabolism of anthocyanins
Changes in the expression of anthocyanin structural genes are controlled by transcriptional regulators. In strawberries, R2R3-FaMYB5 and FaMYB10 both promote the expression of most of the structural genes involved in the flavonoid biosynthetic pathway (PAL, C4H, 4CL-2, and F3’H), and R2R3-FaMYB5 can specifically regulate the PAs-associated gene LAR (Jiang et al., 2023). PpMYB17 positively regulates flavonoid biosynthesis in pear fruit by activating PpCHS, PpCHI, PpF3H, and PpFLS in the flavonoid biosynthetic pathway independently of the bHLH or WD40 cofactors in the MBW complex (Premathilake et al., 2020). Our findings suggest that PbMYB5 does not have inherent activation ability, but can specifically bind to the PbANS promoter to enhance anthocyanin production. It appears that bHLH33 may form complexes that activate PbMYB5 and promote PbANS gene expression. The slight discrepancy in results compared to previous studies may be due to the direct regulation of PbUFGT by the PbMYB5-bHLH-WD40 complex, as well as the transactivation of PbANS by PbMYB5 with the assistance of bHLH33, resulting in increased anthocyanidin synthesis and disruption of PbANS Regulation (Figure 8).
5 Conclusions
The study results show that PbMYB5 plays a role in enhancing anthocyanin accumulation and controlling the expression of genes related to lignin and PAs synthesis in pear skin. PbMYB5 forms a complex with bHLH3/bHLH33-WD40, which promotes anthocyanin accumulation in pears. Additionally, PbMYB5 can combine with PbANS, activating its promoter with bHLH33. These findings provide insights into metabolite regulation in pear skin and offer new strategies for molecular breeding and variety enhancement.
Data availability statement
The original contributions presented in the study are included in the article/Supplementary Material. Further inquiries can be directed to the corresponding authors.
Author contributions
SL: Data curation, Formal Analysis, Investigation, Methodology, Writing – original draft, Writing – review & editing. YY: Formal Analysis, Investigation, Writing – review & editing. ZZ: Formal Analysis, Investigation, Methodology, Writing – review & editing. XZ: Investigation, Methodology, Writing – review & editing. DL: Software, Writing – review & editing. RH: Methodology, Software, Writing – review & editing. YZ: Software, Writing – review & editing. JZ: Investigation, Writing – review & editing. YL: Methodology, Software, Writing – review & editing. YW: Resources, Writing – review & editing. ML: Resources, Writing – review & editing. WH: Software, Writing – review & editing. QC: Project administration, Writing – review & editing. YL: Visualization, Writing – review & editing. XW: Visualization, Writing – review & editing. HT: Funding acquisition, Supervision, Writing – review & editing. YZ: Conceptualization, Funding acquisition, Supervision, Writing – review & editing.
Funding
The author(s) declare financial support was received for the research, authorship, and/or publication of this article. This project was funded by ‘Sichuan Provincial Natural Science Foundation Upper-level Project’, No. 2024NSFSC0392. This work was supported by grants from the Science and Technology Plan Project of Sichuan Province (Grant No. 2021YFYZ0023-03) and the Double Support Project of Discipline Construction of Sichuan Agricultural University (03573134).
Acknowledgments
We thank all the members of the Laboratory of Plant Genetic Breeding and Biotechnology, College of Horticulture, Sichuan Agricultural University for their help during this experiment. Thanks are also due to Dr Leiyu Jiang, College of Horticulture and Landscape Architecture, Yangtze University, who provided us with significant intellectual and emotional support to complete this work. We are grateful to Dr. Yongqiang Liu in our laboratory, who provided important experimental technical support to complete this work.
Conflict of interest
The authors declare that the research was conducted in the absence of any commercial or financial relationships that could be construed as a potential conflict of interest.
Publisher’s note
All claims expressed in this article are solely those of the authors and do not necessarily represent those of their affiliated organizations, or those of the publisher, the editors and the reviewers. Any product that may be evaluated in this article, or claim that may be made by its manufacturer, is not guaranteed or endorsed by the publisher.
Supplementary material
The Supplementary Material for this article can be found online at: https://www.frontiersin.org/articles/10.3389/fpls.2024.1492384/full#supplementary-material
References
Alabd, A., Ahmad, M., Zhang, X., Gao, Y., Peng, L., Zhang, L., et al. (2022). PpMYB10Light-responsive transcription factor PpWRKY44 induces anthocyanin accumulation by regulating expression in pear. Horticulture Res. 9, uhac199. doi: 10.1093/hr/uhac199
Bai, S., Tao, R., Tang, Y., Yin, L., Ma, Y., Ni, J., et al. (2019). BBX16, a B-box protein, positively regulates light-induced anthocyanin accumulation by activating MYB10 in red pear. Plant Biotechnol. J. 17, 1985–1997. doi: 10.1111/pbi.v17.10
Cheng, Y., Zhu, Y., Chen, Y., Ito, S., Asami, T., Wang, X. (2014). Brassinosteroids control root epidermal cell fate via direct regulation of a MYB-bHLH-WD40 complex by GSK3-like kinases. eLife 3, e02525. doi: 10.7554/eLife.02525.022
Citovsky, V., Lee, L. Y., Vyas, S., Glick, E., Chen, M. H., Vainstein, A., et al. (2006). Subcellular localization of interacting proteins by bimolecular fluorescence complementation in planta. J. Mol. Biol. 362, 1120–1131. doi: 10.1016/j.jmb.2006.08.017
Cong, L., Qu, Y., Sha, G., Zhang, S., Ma, Y., Chen, M., et al. (2021). PbWRKY75 promotes anthocyanin synthesis by activating PbDFR, PbUFGT, and PbMYB10b in pear. Physiologia plantarum 173, 1841–1849. doi: 10.1111/ppl.v173.4
Cui, D., Zhao, S., Xu, H., Allan, A. C., Li, K. (2021). The interaction of MYB, bHLH and WD40 transcription factors in red pear (Pyrus pyrifolia) peel. Plant Mol. Biol. 106, 407–417. doi: 10.1007/s11103-021-01160-w
Deluc, L., Barrieu, F., Marchive, C., Lauvergeat, V., Decendit, A., Richard, T., et al. (2006). Characterization of a grapevine R2R3-MYB transcription factor that regulates the phenylpropanoid pathway. Plant Physiol. 140, 499–511. doi: 10.1104/pp.105.067231
Deluc, L., Bogs, J., Walker, A. R., Ferrier, T., Decendit, A., Merillon, J. M., et al. (2008). The transcription factor VvMYB5b contributes to the regulation of anthocyanin and proanthocyanidin biosynthesis in developing grape berries. Plant Physiol. 147, 2041–2053. doi: 10.1104/pp.108.118919
Gambino, G., Gribaudo, I. (2012). Genetic transformation of fruit trees: current status and remaining challenges. Transgenic Res. 21, 1163–1181. doi: 10.1007/s11248-012-9602-6
Gonzalez, A., Zhao, M., Leavitt, J., Lloyd, A. (2008). Regulation of the anthocyanin biosynthetic pathway by the TTG1/bHLH/Myb transcriptional complex in Arabidopsis seedlings. Plant journal: Cell Mol. Biol. 53, 814–827. doi: 10.1111/j.1365-313X.2007.03373.x
Gonzalez, A., Zhao, M., Leavitt, J. M., Lloyd, A. M. (2010). Regulation of the anthocyanin biosynthetic pathway by the TTG1/bHLH/Myb transcriptional complex in Arabidopsis seedlings. Plant J. 53, 814–827. doi: 10.1111/j.1365-313X.2007.03373.x
Hu, Y., Cheng, H., Zhang, Y., Zhang, J., Niu, S., Wang, X., et al. (2021). The MdMYB16/MdMYB1-miR7125-MdCCR module regulates the homeostasis between anthocyanin and lignin biosynthesis during light induction in apple. New Phytol. 231, 1105–1122. doi: 10.1111/nph.v231.3
Jiang, Y., Liu, C., Yan, D., Wen, X., Liu, Y., Wang, H., et al. (2017). MdHB1 down-regulation activates anthocyanin biosynthesis in the white-fleshed apple cultivar ‘Granny Smith’. J. Exp. Bot. 68, 1055. doi: 10.1093/jxb/erx029
Jiang, L., Yue, M., Liu, Y., Zhang, N., Lin, Y., Zhang, Y., et al. (2023). A novel R2R3-MYB transcription factor FaMYB5 positively regulates anthocyanin and proanthocyanidin biosynthesis in cultivated strawberries (Fragaria × ananassa). Plant Biotechnol. J. 21, 1140–1158. doi: 10.1111/pbi.14024
Liu, H., Yu, X., Li, K., Klejnot, J., Lin, C. (2008). Photoexcited CRY2 interacts with CIB1 to regulate transcription and floral initiation in arabidopsis. Science 322, 1535–1539. doi: 10.1126/science.1163927
Ma, D., Reichelt, M., Yoshida, K., Gershenzon, J., Constabel, C. (2018). Two R2R3-MYB proteins are broad repressors of flavonoid and phenylpropanoid metabolism in poplar. Plant journal: Cell Mol. Biol. 96, 949–965. doi: 10.1111/tpj.2018.96.issue-5
Ni, J., Bai, S., Zhao, Y., Qian, M., Tao, R., Yin, L., et al. (2019). Ethylene response factors Pp4ERF24 and Pp12ERF96 regulate blue light-induced anthocyanin biosynthesis in ‘Red Zaosu’ pear fruits by interacting with MYB114. Plant Mol. Biol. 99, 67–68. doi: 10.1007/s11103-018-0802-1
Ni, J., Premathilake, A. T., Gao, Y., Yu, W., Tao, R., Teng, Y., et al. (2021). Ethylene-activated PpERF105 induces the expression of the repressor-type R2R3-MYB gene PpMYB140 to inhibit anthocyanin biosynthesis in red pear fruit. Plant journal: Cell Mol. Biol. 105, 167–181. doi: 10.1111/tpj.v105.1
Ni, J., Wang, S., Yu, W., Liao, Y., Pan, C., Zhang, M., et al. (2023). The ethylene-responsive transcription factor PpERF9 represses PpRAP2.4 and PpMYB114 via histone deacetylation to inhibit anthocyanin biosynthesis in pear. Plant Cell 35, 2271–2292. doi: 10.1093/plcell/koad077
Premathilake, A., Ni, J., Bai, S., Tao, R., Ahmad, M., Teng, Y. (2020). R2R3-MYB transcription factor PpMYB17 positively regulates flavonoid biosynthesis in pear fruit. Planta 252, 59. doi: 10.1007/s00425-020-03473-4
Pu, Z., Wei, G., Liu, Z., Chen, L., Zheng, Y. (2021). Selenium and anthocyanins share the same transcription factors R2R3MYB and bHLH in wheat. Food Chem. 356, 129699. doi: 10.1016/j.foodchem.2021.129699
Shang, Y. J., Venail, M., Bailey, P. C., Schwinn, K. E., Jameson, P. E. (2011). The molecular basis for venation patterning of pigmentation and its effect on pollinator attraction in flowers of Antirrhinum. New Phytol. 189, 602–615. doi: 10.1111/j.1469-8137.2010.03498.x
Stracke, R., Werber, M., Weisshaar, B. (2001). The R2R3-MYB gene family in Arabidopsis thaliana. Current opinion in plant biology 4 (5), 447–456. doi: 10.1016/s1369-5266(00)00199-0
Wang, Z., Du, H., Zhai, R., Song, L., Ma, F., Xu, L. (2017). Transcriptome Analysis Reveals Candidate Genes Related to Color Fading of 'Red Bartlett' (Pyrus communis L.). Frontiers in plant science. 8, 455. doi: 10.3389/fpls.2017.00455
Wang, L., Lu, W., Ran, L., Dou, L., Yao, S., Hu, J., et al. (2019a). R2R3-MYB transcription factor MYB6 promotes anthocyanin and proanthocyanidin biosynthesis but inhibits secondary cell wall formation in Populus tomentosa. Plant journal: Cell Mol. Biol. 99, 733–751. doi: 10.1111/tpj.14364
Wang, Z., Yang, Z., Li, F. (2019b). Updates on molecular mechanisms in the development of branched trichome in Arabidopsis and nonbranched in cotton. Plant Biotechnol. J. 17, 1706–1722. doi: 10.1111/pbi.13167
Wang, Y., Zhang, X., Zhao, Y., Yang, J., He, Y., Li, G., et al. (2020). Transcription factor PyHY5 binds to the promoters of PyWD40 and PyMYB10 and regulates its expression in red pear ‘Yunhongli No. 1’. Plant Physiol. Biochem. 154, 665–674. doi: 10.1016/j.plaphy.2020.07.008
Wen, W., Alseekh, S., Fernie, A. R. (2020). Conservation and diversification of flavonoid metabolism in the plant kingdom. Curr. Opin. Plant Biol. 55, 100–108. doi: 10.1016/j.pbi.2020.04.004
Wu, J., Wang, Z., Shi, Z., Zhang, S., Ming, R., Zhu, S., et al. (2013). The genome of the pear (Pyrus bretschneideri Rehd.). Genome Res. 23, 396–408. doi: 10.1101/gr.144311.112
Xu, W., Grain, D., Bobet, S., Le Gourrierec, J., Thévenin, J., Kelemen, Z., et al. (2013). Complexity and robustness of the flavonoid transcriptional regulatory network revealed by comprehensive analyses of MYB–bHLH–WDR complexes and their targets in Arabidopsis seed. New Phytol. 202(1), 132–144. doi: 10.1111/nph.12620
Yao, G., Ming, M., Allan, A., Gu, C., Li, L., Wu, X., et al. (2017). Map-based cloning of the pear gene MYB114 identifies an interaction with other transcription factors to coordinately regulate fruit anthocyanin biosynthesis. Plant journal: Cell Mol. Biol. 92, 437–451. doi: 10.1111/tpj.2017.92.issue-3
Ye, X., Tan, H., Ma, Z., Huang, J. (2016). DELLA Proteins Promote Anthocyanin Biosynthesis via Sequestering MYBL2 and JAZ Suppressors of the MYB/bHLH/WD40 Complex in Arabidopsis thaliana. Mol. Plant 9, 711–721. doi: 10.1016/j.molp.2016.01.014
Yousuf, B., Singh, P., Gul, K., Wani, A. A. (2016). Health benefits of anthocyanins and their encapsulation for potential use in food systems: A review. Crit. Rev. Food Sci. Nutr. 56, 2223–2230. doi: 10.1080/10408398.2013.805316
Zhai, R., Liu, X., Feng, W., Chen, S., Xu, L., Wang, Z., et al. (2014). Different biosynthesis patterns among flavonoid 3-glycosides with distinct effects on accumulation of other flavonoid metabolites in pears (Pyrus bretschneideri Rehd.). PloS One 9, e91945. doi: 10.1371/journal.pone.0091945
Zhai, R., Wang, Z., Zhang, S., Meng, G., Song, L., Wang, Z., et al. (2016). Two MYB transcription factors regulate flavonoid biosynthesis in pear fruit (Pyrus bretschneideri Rehd.). J. Exp. Bot. 67, 1275–1284. doi: 10.1093/jxb/erv524
Zhang, Z., Tian, C., Zhang, Y., Feng, S. (2020). Transcriptomic and metabolomic analysis provides insights into anthocyanin and procyanidin accumulation in pear. BMC Plant Biol. 20, 129. doi: 10.1186/s12870-020-02344-0
Zhang, B., Yang, H., Qu, D., Zhu, Z., Yang, Y., Zhao, Z. (2022). The MdBBX22-miR858-MdMYB9/11/12 module regulates proanthocyanidin biosynthesis in apple peel. Plant Biotechnol. J. 20, 1683–1700. doi: 10.1111/pbi.13839
Keywords: MYB transcription factor, lignin, anthocyanin, proanthocyanidins(PAs), coloration
Citation: Li S, Yang Y, Zhou Z, Zhou X, Lei D, He R, Zhang Y, Zhang J, Lin Y, Wang Y, Li M, He W, Chen Q, Luo Y, Wang X, Tang H and Zhang Y (2025) PbMYB5 transcription factor plays a role in regulating anthocyanin biosynthesis in pear (Pyrus bretschneideri Rehd) skin. Front. Plant Sci. 15:1492384. doi: 10.3389/fpls.2024.1492384
Received: 06 September 2024; Accepted: 10 December 2024;
Published: 14 January 2025.
Edited by:
Gabino Ríos, Valencian Institute for Agricultural Research (IVIA), SpainReviewed by:
Bhabesh Borphukan, Washington State University, United StatesYinan Yuan, Michigan Technological University, United States
Ji Tian, Beijing University of Agriculture, China
Copyright © 2025 Li, Yang, Zhou, Zhou, Lei, He, Zhang, Zhang, Lin, Wang, Li, He, Chen, Luo, Wang, Tang and Zhang. This is an open-access article distributed under the terms of the Creative Commons Attribution License (CC BY). The use, distribution or reproduction in other forums is permitted, provided the original author(s) and the copyright owner(s) are credited and that the original publication in this journal is cited, in accordance with accepted academic practice. No use, distribution or reproduction is permitted which does not comply with these terms.
*Correspondence: Haoru Tang, aHRhbmdAc2ljYXUuZWR1LmNu; Yong Zhang, emh5b25nQHNpY2F1LmVkdS5jbg==
†These authors have contributed equally to this work