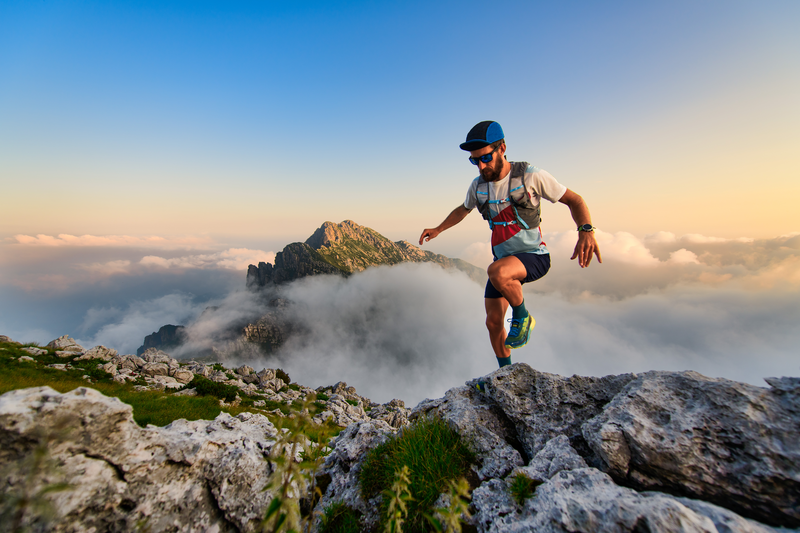
95% of researchers rate our articles as excellent or good
Learn more about the work of our research integrity team to safeguard the quality of each article we publish.
Find out more
ORIGINAL RESEARCH article
Front. Plant Sci. , 27 November 2024
Sec. Plant Nutrition
Volume 15 - 2024 | https://doi.org/10.3389/fpls.2024.1492137
The imbalanced fertilization and the consequential deterioration on the rhizosphere microbial community (RMC) were two potential reasons for the quick yielding degradation of Phyllostachys violascens (Lei-bamboo), a high-value shoot-oriented bamboo. However, most research only focused on nitrogen, phosphorus, and potassium; the studies on the dynamics of other nutrients, such as calcium and magnesium; and their driving mechanisms, lags far behind. Thus, Lei-bamboo fields of different mulching and recovery ages were selected to investigate the dynamics of calcium and magnesium in both soil and bamboo tissue, and to explore their relationship to RMC composition and network patterns. The results showed that mulching increased the content of soil acidification, total organic carbon, alkali-hydrolysable nitrogen, available phosphorus, and available potassium but reduced soil exchangeable magnesium and calcium in soil as well as the magnesium and calcium content in rhizome, stem, and leaf of Lei-bamboo, which indicated an increased relative limitation on magnesium and calcium. Mulching also enhanced the α-diversity and reshaped the composition of RMC, which had a close link to Mg rather than nitrogen, phosphorus, and potassium. As the mulching years increased, the RMC network became bigger and more complex, and the magnesium and calcium gradually appeared in the network center, which further support the magnesium and calcium deficiency to RMC. Nearly all the variation mentioned above could be revered after the removing of mulching. Structural equation modeling showed two main pathways that mulching leads to magnesium and calcium deficiency in Lei-bamboo, one is directly by lowering soil magnesium and calcium content, the other one is indirectly by improving RMC network interactions, a sign of weakened mutualism between RMC and plant roots that hampering the uptake of nutrients. This research highlights the quick magnesium and calcium deficiency caused by mulching in Lei-bamboo forest and the contribution of RMC in amplify the effects of soil magnesium and calcium deficiency, which offers valuable information on balancing fertilization pattern for future sustainable Lei-bamboo cultivation.
● Mulching induced magnesium and calcium deficiency in soil and plant tissue.
● Mulching enhanced rhizosphere microbial network interactivity.
● Microbial network focus more on magnesium and calcium as the mulching age increases.
● Increased network interactivity might amplify the effects of soil magnesium and calcium limitation.
Phyllostachys violascens (Lei-bamboo) is a high-value and food-oriented bamboo species that exhibits a high potential for enhancing the local people’s incomes (Gui et al., 2013; Chen et al., 2015). Most farmers adopt intensive cultivation pattern to ensure the yielding and income from Lei-bamboo forest (Wei et al., 1994; Gui et al., 2018). The intensive cultivation of Lei-bamboo includes not only classic intensive measures, such as high-quantity fertilization with nitrogen, phosphorus, and potassium, but also with organic material-based mulching, a special intensive measure of Lei-bamboo cultivation (Wei et al., 1994; Gui et al., 2013; Chen et al., 2015). For about 4 months, beginning in November, the soil is covered with a mixed layer of straw and rice husks, 20–30 cm thick, to increase the soil temperature and stimulate the sprouting of shoots, thereby increasing shoot yield (Wei et al., 1994). Mulching can potentially increase yield by as much as 3 times and raise the yield to as high as 375,00 kg ha−1 (Gui et al., 2013), the harvest income can reach nearly USD 30,000 ha−1 in certain regions (Chen et al., 2015). However, after several years of mulching, the yield will sharply decrease, especially after the third year (Gui et al., 2013), and even if fertilization is doubled, the yield could still be decreased by as much as 50% (Wang et al., 2017). Only after 2 years of recovery cultivation, with no mulching, can Lei-bamboo again achieve a considerable yield using the mulching technique (Wang et al., 2017). Moreover, after long-term intensive cultivation (usually over 15 years), Lei-bamboo is incapable of yield recovery through mulch removal. Full recovery can only be achieved by exchange of the soil to a depth of more than 1 m and replanting of the bamboo (Zhou et al., 2017). The undesirable effects caused by mulching not only reduce the potential yield but also increase management costs, in particular, the rapid yield reduction after just 3 years of mulching has become a core problem to be resolved in Lei-bamboo intensive cultivation (Wang et al., 2017; Zhou et al., 2017). Unfortunately, the detailed degradation mechanisms for mulched Lei-bamboo forests were still unclarified.
Empirically, mulching-induced impairment of soil nutrient cycling and subsequent deterioration of the rhizosphere microbial environment have been hypothesized to be factors for the short-term degradation of Lei-bamboo (Jiang et al., 2000; Dai et al., 2018; Das et al., 2021). However, most studies have only focused on the nutrient cycling and microbial processes of nitrogen, phosphorus, and potassium applied under mulching and on their relationship to yield degradation (Wei et al., 1994; Gui et al., 2013; Chen et al., 2015), with the variation of the content and stoichiometric of other elements in soil and Lei-bamboo tissue, such as magnesium and calcium, scarcely discussed. It seems that it had been tacitly assumed that magnesium and calcium were relatively adequate and less affected by mulching. Magnesium and calcium play important roles in photosynthetic (Jing et al., 2024) and organic matter transportation and storage for plants (Farhat et al., 2016). Magnesium and calcium can also influence the soil biogeochemical processes and the effects of nitrogen and phosphorus fertilizer and their. The application of magnesium and calcium were reported to save half nitrogen fertilizer in a consecutively cultivated peanut field (Gao et al., 2024b) and can reduce the N2O emission and nitrate leaching so that to enhance nitrogen fertilizer efficiency in a paddy soil (He et al., 2023). Hence, the magnesium and calcium limitation could both directly and indirectly contribute to the short-term degradation of Lei-bamboo and lower the yielding.
Yet, the dynamic of magnesium and calcium through mulching years and whether they were limiting factors of mulched Lei-bamboo forests are still open questions, as mulching may increase or decrease the availability of soil magnesium and calcium (Clark et al., 2005; Yang et al., 2010; Prietzel et al., 2021). The availability of soil magnesium and calcium was determined by mineralization and leaching, two processes mainly regulated by microbial activity and soil acidity. Mulching could offer controversial influences on these two processes. Mulching vegetable plots with straw, for example, releases organic matter that adsorbs free protons from the soil and subsequently mitigates soil acidification (Guan et al., 2022; Le et al., 2022), while some studies have found that mulching materials can release organic acids and accelerate soil acidification (Gui et al., 2018). Mulching may introduce anaerobic stress (Qian et al., 2022) and aluminum toxicity stress (Beeckman et al., 2018; Zhao et al., 2020), reducing the activity of microbial communities and their ability to turn over nutrients, but mulching also releases organic matters that promote microbial community activity and subsequently accelerate mineralization (Guan et al., 2022; Le et al., 2022). Thus, it’s necessary to systematically investigate the dynamic of magnesium and calcium along a mulching cycle of Lei-bamboo and introduce stoichiometry to test their relative limitation to shoot yields. Stoichiometry ratio is a commonly used tool to evaluate the relative limitation of certain nutrients, the increase and decrease of the ratio indicate an uplifting limitation on denominator nutrient and numerator nutrient, respectively (Martiny et al., 2013).
The root is the core plant organ for magnesium and calcium assimilation, and the rhizosphere soils are thus more sensitive to nutrient limitation variation. Meanwhile, rhizosphere is the location of a highly active microbial community, named the rhizosphere microbial community (RMC) (Ling et al., 2022). The RMC receives organic exudates from their host plants and, in return, aides the host with nutrient assimilation (Pausch et al., 2024), pathogen defense (Trivedi et al., 2020), and stress tolerance (Aslam et al., 2022). As the bridge linking host plant and bulk soil, the properties of the RMC, including richness, composition (Vorholt et al., 2017), and network interactions (Jiang et al., 2017), are regulated by both the physiological signals of host plants (e.g., certain nutrient deficiencies) (Venturi and Keel, 2016) and the soil physiochemical properties (e.g., nutrient contents and their limitations) (Garbeva et al., 2008). The RMC is sensitive to environmental change and can quickly reshape richness, composition, and network interactions accordingly, and it can drive host plant community variation (Zhou et al., 2021, 2022). The RMC community structure is largely regulated by the most critical limiting factors, and the network interactions will also tend to be structured around these factors (Zhou et al., 2021, 2022). Thus, changes in Lei-bamboo RMC may indicate variations in both soil physiochemical properties and plant nutrient physiological status of magnesium and calcium, which possibly offers a deeper explanation of the interactive mechanisms between the latter two (Jiang et al., 2017; Vorholt et al., 2017).
In this study, the rhizosphere soil physiochemical properties, plant nutrient content, and RMC properties of Lei-bamboo with different mulching and recovery ages were investigated to explore the dynamics of magnesium and calcium in soil and plant tissue caused by mulching and to resolve the relevant mechanisms. Given the fact that the 20–30 cm thick organic mulching layer would deliver large amounts of organic matter to the soil, we hypothesized that mulching would increase soil pH and increase soil available magnesium and calcium content. However, because a large amount of nitrogen, phosphorus, and potassium was applied before mulching, we hypothesized that the relative limitation of magnesium and calcium in both soil and Lei-bamboo tissue would increase, indicated by an increase in the corresponding stoichiometry (e.g., N:Mg), which would lead the RMC networks under mulching focused more on magnesium and calcium rather than on N, P, and K. The mulching should offer a more hospitable environment for microorganisms, as it introduces a considerable quantity of foreign organic matters, which would not only enhance interactions among microbes but also weaken the functional link between root and RMC. Thus, we hypothesized that mulching would increase both RMC diversity and network interactivity. Last, the increased relative limitation on soil calcium and magnesium and the weakened cooperation between root and RMC combined to induce calcium and magnesium deficiency in Lei-bamboo under mulching conditions. This research further highlighted the importance of magnesium and calcium in Lei-bamboo cultivation and offered new sight to protect mulched Lei-bamboo forest from short-term degradation.
The target Lei-bamboo fields were in Hongxi Farm of Fuzhou city, Jiangxi province, China (E 116°20′37.17″, N 28°27′03.46″). The site is a mid-subtropical zone, with a mean annual temperature of 19.3°C and mean annual precipitation of 1,742 mm. The bamboo was planted from 2014 to 2019, and samples were taken on 5 March 2023 (the mulching materials had recently been removed), when the farm possessed a bamboo field that would be mulched in the next year (M0, planted in the winter of 2019), a field that had been mulched once (M1, planted in the winter of 2018), a field that had been mulched for two consecutive years (M2, planted in the winter of 2017), a field that had been mulched for three consecutive years (M3, planted in the winter of 2016), a field that had recovered for one year (R1, planted in the winter of 2014), and a field that had recovered for 2 years (R2, planted in the winter of 2015). Every field was large enough (over 6,660 m2) to ensure representativity. All fields were selected from the same farm to reduce variations introduced by climate conditions and soil parent materials. Mulching operations are initiated in early November when a mixture of rice husks (200t hm−2) and straw pieces (50t hm−2), with a thickness of over 25 cm, is placed over the bamboo growing beds. The mixture contains about 0.06% nitrogen, 0.015% phosphorus, 0.12% potassium, 0.24% calcium, and 0.14% magnesium. This mulch is removed approximately 4 months later. About 7.5 kg year−1 m−2 organic fertilizer was applied to the fields in the middle of November, and the middle of March, respectively. About 0.188 kg year−1 m−2 chemical fertilizer (N:P:K = 10:3:7) was applied to the fields in early March, early June, early September and mid-November. The soil belongs to subtropic soils (Latosol), which developed from river sediments, the bulk density of the field soil is about 1.29 g cm−3.
During sampling, eight 5 m × 5 m plots were set in each field, the fine roots of the rhizomes within the square were dug up and bulk soil removed by shaking, then the adhering rhizosphere soil (mainly 30 cm deep topsoil of where roots were distributed) was collected by brushing. After all the rhizosphere soil in the square was collected, 100 g were retained for analysis. The rhizomes, stems, and leaves in the square were respectively collected and crushed, and approximately 1 kg was retained for analysis. The samples were transported to the lab on ice. 5 g of each soil sample were stored at −80°C for molecular analysis. The remaining soil was air dried, grounded to pass through a 0.2-mm sieve, and stored at −80°C until measurement of physiochemical properties. The plant tissue samples were dried to a constant weight at 100°C, then the tissue was powdered to pass a 0.2-mm sieve, and approximately 100 g were stored in a drying dish at room temperature.
The total organic carbon (TOC) was measured by a TOC analyzer (Elementar, Berlin, Germany). 0.1g sample was weighed and then burned at 950°C with gas flow 250 ml min−1 (Contreras et al., 2018).
The available phosphorus (AP) was determined by the Mo-Sb-Vc method. 2.5 g soil sample was placed in a 50-ml centrifuge tube, to which 18 ml extraction solution (0.03 mol L−1 NH4F and 0.025 mol L−1 HCl) was added. The mixture was then shaken at 300 rpm for 1.5 min. 5–10 ml of the supernatant was transferred to another bottle, to which 5 ml Mo-Sb-Vc solution (100 ml 5 g L−1 C8H4K2OSb2, 450 ml 22.22 g L−1 (NH4)2MoO4, 10.5 g Vitamin C, and 153 ml concentrated sulfuric acid) was added and deionized water to adjust the total volume to 50 ml. The solution was incubated at 25°C for 30 min, and the 880 nm luminous absorbance value was then measured by a spectrophotometer (Simatsu-UV1900, Tokyo, Japan). The AP content was subsequently calculated (Huang et al., 2020).
The available potassium (AK), exchangeable magnesium (Mg), and exchangeable calcium (Ca) were measured by an atomic spectrum spectrophotometer (Spectrum-3803AA, Shanghai, China). A 2.0 g soil sample was placed into a 50 ml centrifuge tube, and extraction solution (1 mol L−1 NH4Ac, pH = 7.0) was added. Next, the solution was shaken at 300 rpm for 30 min, and the supernatant was collected. AK, magnesium, and calcium were determined at 766.5 nm, 285.2 nm, and 422.7 nm, respectively. The burning gas was ethyne (purity > 99.99%) (Kurokawa et al., 2020).
Alkali-hydrolysable nitrogen (AN) was measured by diffusion method (Chen et al., 2016). The pH was determined by a pH meter (Leici-PHS3G, Shanghai, China), where 5 g of soil was mixed with 12.5 ml of water, and the mixture was used for measurement (Zhou et al., 2022).
For nitrogen content measurement, 1 g tissue powder was placed in a digesting tube, to which 1 g accelerator (K2SO4:CuSO4:Se = 100:10:1, thoroughly powdered and mixed) and 5 ml concentrated sulfuric acid were added. Measurement was performed using a Kjeldahl Nitrogen Analyser (Hanon K9860, Jinan, China) (Chen et al., 2016).
For measurement of phosphorus, potassium, calcium, and magnesium, 0.2 g plant tissue powder were placed in a digestion tube, and 8 ml of concentrated sulfuric acid was added. The mixture was allowed to sit for 12 h, after which it was digested at 250°C for 10 min, then heated to 380°C and 2 ml 30% H2O2 was added, the solution was digested until it became clear. After cooling to room temperature, the solution was transferred to a centrifuge tube, and water was added until the total volume reached 50 ml. Phosphorus content was determined by Mo-Sb-Vc method, and the potassium, calcium, and magnesium were measured by an atomic spectrum spectrophotometer (same as Section 2.2).
Total soil DNA was extracted by FastDNA-SPIN Kit for Soil (MP Biomedicals, Santa Ana, CA) according to the manufacturer’s instructions. The PCR primer pairs used were 338F-(5’-ACTCCTACGGGAGGCAGCA-3’) and 806R-(5’-GGACTACHVGGGTWTCTAAT-3’, targeting the bacterial 16S V3-V4 region (Liu et al., 2018), and ITS1F-(5’-CTTGGTCATTTAGAGGAAGTAA-3’) and ITS2R-(5’-GCTGCGTTCTTCATCGATGC-3’), targeting the Fungal ITS2 region (Adams et al., 2013). The PCR system was 50 μl, and included 1–10 ng DNA template, 1 μl forward primers and 1 μl reverse primers, 25 μl 2×Premix Taq, and enough water to bring the volume to 50 μl. The PCR program was 95°C pre-denaturing for 3 min, 40 cycles of (95°C denaturing for 30 s, 58°C annealing for 30 s, 72°C elongating for 30 s), 72°C for 8 min, then held at 4°C.
Sequencing was conducted using an Illumina Novaseq6000 (Guangdong Magigene Biotechnology Co., Ltd. Guangdong, China). Using a sliding window (-W4-M20), the quality control on raw sequencing data was done by FASTP v0.14.1. Then the primers were removed by the CUTADAPT software program. Paired-end clean reads were combined using the usearch-fast_mergepairs command according to the overlapped reads. Chimeric sequences were removed using the usearch-cluster_otus command. Sequences with at least 16 bp overlap reads and less than five mismatch sites were merged. The merged sequences with similarity > 97% were defined as belonging to the same OTU (Edgar, 2013). The bacterial and fungal OTUs were annotated using Silva-138 (Prüsse et al., 2011) and Unite-v8.0 (Abarenkov et al., 2010), respectively. The OTU tables were resampled to account for sequencing depth, with the bacterial OTU table containing 45,326 reads for each sample and the fungal table containing 19,344 reads, and the resampled OTU tables were used for subsequent analyses.
The calculation of relative abundance (RA), α- and β-diversity, and the redundancy analysis/ canonical correspondence analysis (RDA/CCA) and analysis of similarities (ANOSIM) analyses (Bray-Curtis distance based) were all conducted using an online platform (http://cloud.magigene.com/, accessed on 14 February 2024). Chao1 and Shannon index were chose to assess alpha diversity. The difference in the microbiome composition was calculated using redundancy analysis/classical canonical analysis based on Bray-Curtis distances.
The network analysis was conducted using an online platform (http://ieg4.rccc.ou.edu/MENA, accessed on 16 February 2024); the network construction parameters were set as majority=6;missing_fill=fill_paired(0.01);logarithm=n;similarity=spearman2, the difference of cutoff values among networks was less than 0.01 (Deng et al., 2012). Four parameters were calculated to quantify the structural variations of the network: the average degree (avgK), which indicates the network interactivity; the geographical distance (GD), which indicates the length of the interaction chain; the average clustering coefficient (avgCC), which indicates the network clustered state; and the connectedness, which indicates the number degree of the nodes connected (Deng et al., 2012). The identity of key nodes, including module hubs, the nodes that were potential functional centers of a module (Zi > 2.5), and connectors, the nodes that were key for interaction among modules (Pi > 0.625), were determined (Deng et al., 2012). The figures were drawn by Origin 8.0, and the networks were visualized by Cytoscape v3.3.0.
The correlation analysis and between group differences analyses (one-way analysis of variance, Tukey’s HSD) were performed using IBM SPSS Statistics 27. The structural equation modeling (SEM) was performed by Amos v2.0 compounded in SPSS. During the reduction of data dimension for SEM, the main axis was significantly related to the factor’s axis (each coefficient higher than 0.8). The composition of the SEM was adjusted until the goodness of fit index (GFI) was over 0.9, the comparative fit index (CFI) was over 0.9, the root-mean-square error of approximation (RMSEA) was less than 0.05, the χ2/df was less than 2, and the P-value was over 0.05, and then chose the models of the best parameters (Weston and Gore, 2006).
The results showed that soil pH, TOC, AN, TP, AK, AP, exchangeable magnesium, and calcium were all significantly changed with the increasing mulching ages (Figure 1). TOC, AN, AP, and AK showed an increasing trend that the value in M3 was nearly double of that in M0 (Figures 1B–E). But soil pH exchangeable magnesium and calcium lead a decreasing trend (Figures 1A, F, G). The exchangeable Mg decreased from M0 (46.56 ± 5.07 mg kg−1) to M3 (27.39 ± 5.00 mg kg−1) (Figure 1F) and exchangeable Ca similarly decreased from 64.25 ± 9.48 mg kg−1 (M0) to 31.65 ± 5.29 mg kg−1 (M3), (Figure 1G). Most of properties showed a partial recovery after the removal of mulching, except AP and AK (Figures 1A–G). Pearson correlation analysis found the factors were all significantly correlated (Figure 1H).
Figure 1. The dynamics of soil physiochemical properties and their relationship analysis. The lowercase letters in subfigures (A–G) indicate significant differences at the P < 0.05 level (Tukey’s HSD), the * and ** in subfigure (H) indicate significant correlation at the levels of P < 0.05 and P < 0.01, respectively. The Ca, Mg, AK, AP, and AN in subfigure (H) indicate exchangeable calcium, exchangeable magnesium, available potassium, available phosphorus and alkali-hydrolysable nitrogen, respectively. The M0 to M3, respectively, indicate mulched for 0–3 years and R1 and R2, respectively, indicate recovered for 1 and 2 years.
The most prominent feature of soil nutrient stoichiometry dynamic was that the relative limitations of magnesium and calcium (C:Mg, N:Mg, P:Mg, K:Mg, C:Ca, N:Ca, P:Ca, and K:Ca) increased during mulching and partially recovered after mulch removal, which indicated the increased relative limitation of magnesium and calcium were induced by mulching (Figures 2D, E, H, I, K–N). As the ratios of P:Mg and P:Ca showed the greatest variance in magnitude, increasing from 3.97 ± 1.14 and 4.70 ± 0.66 (M0) to 14.76 ± 2.66 and 21.24 ± 3.64 (M3), respectively. The C:N, C:P, and C:K significantly increased in the first year of mulching, reaching 121.0 ± 12.13, 260.7 ± 74.77, and 197.3 ± 41.12, respectively. They then subsequently decreased until reaching the values of 69.10 ± 9.81, 114.8 ± 66.36, and 94.24 ± 29.65, respectively, in R2 (Figures 2A–C). P:K showed an increasing trend during the mulching period, with highest and lowest values of 0.909 ± 0.204 (M3) and 0.689 ± 0.077 (M0), respectively (Figure 2J). There were no significant variations in N:P and during the mulching period, the value of N:P was higher during M0–M3 than in the M3 to R2 stage (Figure 2F). No regular trends were observed for N:K (Figure 2G) and Mg: Ca (Figure 2O).
Figure 2. The subfigure (A–O) depicts the dynamics of soil nutrient stoichiometry. The lowercase letters indicate significant differences at the level of P<0.05 (Tukey’ HSD). The M0 to M3 respectively indicate mulched for 0 to 3 years and R1 and R2 respectively indicate recovered for 1 and 2 years.
The contents of nitrogen, phosphorus, potassium, calcium, and magnesium in Lei-bamboo tissue were significantly altered by mulching, and nearly all of them showed at least partially recovery (except for phosphorus in the rhizome) after mulch removal (Figure 3). The TP and TK in rhizome, leaf, and stem showed an increasing trend with mulching ages while the magnesium and calcium showed a decreasing trend (Figures 3D–O), which was similar to what happened in soil (Figure 1). However, the TN decreased during mulching years, which was opposite to its trend in soil (Figures 1, 3A–C). The nitrogen content of rhizome, stem, and leaf significantly decreased from 15.06 ± 0.14 g kg−1 (M0) to 12.00 ± 0.37 g kg−1 (M3), from 13.67 ± 0.20 g kg−1(M0) to 11.84 ± 0.21 g kg−1 (M3) and from 27.57 ± 0.47 g kg−1 g kg−1 (M0) to 25.05 ± 0.43 g kg−1 (M3), respectively, and then recovered to 13.21 ± 0.35 g kg−1, 12.92 ± 0.19 g kg−1, and 26.43 ± 0.59 g kg−1 (R2), respectively (Figures 3A–C). Instead, Mg in rhizome, stem, and leaf were, respectively, 1.56 ± 0.12 g kg−1, 1.87 ± 0.06 g kg−1, and 2.44 ± 0.080 g kg−1 (M0), but the values in M3 decreased to only 34%, 60.7%, and 75.5% that of M0 before recovering to 1.251 ± 0.131 g kg−1, 1.582 ± 0.099 g kg−1, and 2.311 ± 0.146 g kg−1 (R2) (Figures 3M–O).
Figure 3. The subfigure (A–O) depicts dynamic of plant tissue nutrient. The lowercase letters indicate significant differences at the level of P<0.05 (Tukey’ HSD). The M0 to M3 respectively indicate mulched for 0 to 3 years and R1 and R2 respectively indicate recovered for 1 and 2 years.
The results of plant tissue nutrients stoichiometry variation highlighted the magnified limitations of calcium and, especially, magnesium caused by mulching, and the greater magnitude of in variation in rhizome than stem and leaf (Figure 4). First, N:Ca, P:Ca, K:Ca, N:Mg, P:Mg and K:Mg of leaf, stem, and rhizome (except rhizome N:Ca) were increased by mulching, and decreased during the recovery period (Figures 4C, D, F–I). Rhizome P:Mg and K:Mg varied the most, increasing by nearly 4 and 3.5 times from M0 (1.54 ± 0.14 and 3.00 ± 0.25) to M3 (7.26 ± 0.57 and 13.34 ± 1.46), respectively (Figures 4F, H). Yet, mulching reduced N:P and N:K (Figures 4A, B). For instance, leaf N:P was 24.70 ± 0.60 in M0 and decreased to 19.39 ± 0.88 in M3, before increasing to 22.25 ± 0.24 in R2 (Figures 4A, B). The Mg: Ca was also decreased by mulching (Figure 4J). Second, the stoichiometry in rhizome was highest in rhizome, then stem and leaf (Figure 4). For example, the P:Mg of leaf and stem were only increased by 0.55 and 1.1 times, respectively, while K:Mg only increased by 0.54 and 1.0 times (Figures 4F, H), which was significantly lower than rhizome.
Figure 4. The subfigure (A–J) depicts dynamics of plant tissue nutrients stoichiometry. The lowercase letters indicate significant differences among the stages at the level of P<0.05 (Tukey’ HSD). The uppercase letters indicate significant differences among leaf, stem and rhizome at the level of P<0.05 (Tukey’ HSD). The M0 to M3 respectively indicate mulched for 0 to 3 years and R1 and R2 respectively indicate recovered for 1 and 2 years.
Chao and Shannon indices of bacterial and fungal community in rhizosphere were both enhanced by mulching, but they were not significantly reduced after mulching removal. The Chao and Shannon indices of the bacterial community were lowest in M1 (2113 ± 223 and 2.20 ± 0.11, respectively). The Chao and Shannon indices increased to maximums of 2758 ± 390 and 2.47 ± 0.19 in M3 and then weakly fall back to 2325 ± 345 and 2.30 ± 0.22 in R2, respectively (Figures 5A, B). The Chao and Shannon indices of the fungal community were 288 ± 94 and 0.98 ± 0.37 in M0 before significantly increasing to 482 ± 91 and 1.67 ± 0.15 (M1) and declining back to 384 ± 108 and 1.32 ± 0.33 (R2), respectively (Figures 5C, D).
Figure 5. The α-diversity index and the β-diversity dynamics of the rhizosphere bacterial and fungal communities. Subfigures (A–D) depict the α-diversity index variation. Subfigures (E–P) depict the relative abundance variation of major bacterial phyla and fungal orders. Subfigures (Q–V) depict the RDA/CCA analyses of the bacterial and fungal community compositions at OTU level, respectively. The lowercase letters in subfigures indicate significant differences at the level of P < 0.05 (Tukey’s HSD). The M0 to M3 respectively indicate mulched for 0 to 3 years and R1 and R2, respectively, indicate recovered for 1 and 2 years. The TOC, Ca, Mg, AK, AP, and AN in subfigures (Q, T) indicate total organic carbon, exchangeable calcium, exchangeable magnesium, available potassium, available phosphorus and alkali-hydrolysable nitrogen, respectively.
The results showed that mulching altered the RA of several taxa, but the effects were not consistent, and most of them were not recovered after mulching removal (Figure 5). The RA of several taxa was significantly changed after the first year but did not significantly change during the subsequent stages. These taxa included Acidobacteria (RA from M0 33.15 ± 9.00% to M1 52.48 ± 8.30%), Chloroflexi (RA from M0 17.60 ± 5.47% to M1 6.85 ± 1.36%), Unclassified Bacteria (RA from M0 6.94 ± 3.85% to M1 3.48 ± 0.75%) and Sordariomycetes (RA from M0 5.73 ± 3.33% to M1 26.72 ± 14.57%) (Figures 5E–H). The RA of Actinobacteria and WPS-2 decreased during the mulching and recovery periods, from 2.80 ± 0.62% and 2.24 ± 1.22% (M0) to 1.52 ± 0.46% and 0.67 ± 0.57% (R2), respectively (Figures 5I, J). The RA of Bacteroidetes increased from nearly 5% (M0 and M1) to nearly 10% (M2 and M3), then decreased slightly to 8.34 ± 3.77% and 9.21 ± 3.39% in R1 and R2, respectively (Figure 5K). The RA of Proteobacteria also increased from 19.60 ± 6.78% (M0) to 25.73 ± 5.23% (M3), before decreasing slightly to about 23% (R1 and R2) (Figure 5L). The RA of Unclassified Fungi gradually increased from 8.27 ± 5.13% (M0) to 12.41 ± 8.13% (R1), with a decrease in R2; however, no significant differences were observed among any of the stages (Figure 5M). Several taxa displayed no regular dynamic during mulching and recovery (Figures 5N–P). The maximum and minimum RA of Agaricomycetes were 41.21 ± 12.13% (M1) and 20.58 ± 5.81% (M2), respectively (Figure 5N). The RA of Eurotiomycetes was nearly same for every stage (about 10%) except R1 (5.35 ± 2.13%) (Figure 5O). The RA of Planctomycetes was lowest in M1 (4.25 ± 0.96%) and highest in R1 (8.95 ± 1.92%) (Figure 5P).
RDA analysis showed that bacterial OTU-level composition was significantly altered by mulching, especially from M0 to M1 (Figure 5Q), as the PC1 varied significantly from −0.257 ± 0.035 (M0) to −0.011 ± 0.039 (M1) (Figure 5R), but no significant differences were found among M3, R1, and R2, for both PC1 and PC2 (Figures 5R, S). The ANOSIM analysis also found that the P-values for between M3 and R1 and for between R1 and R2 were 0.05 and 0.144, respectively (Supplementary Table S1). These indicated that the composition of the rhizosphere bacterial community had not recovered after mulch removal. The magnitudes of contribution made by the environmental factors to bacterial composition was pH > TOC > Mg > AK > AN > Ca > AP. (Figure 5Q) Fungal community composition at the OTU level was also significantly shaped by mulching (Figure 5T). The compositional variation of fungi was greater than that for bacteria in the first year, as not only did PC1 significantly vary, from −1.991 ± 0.236 (M0) to −0.374 ± 0.275 (M1), but PC2 also varied significantly, from 0.288 ± 0.599 (M0) to −1.193 ± 0.826 (M1) (Figures 5U, V). After mulch removal, PC1 did not recover, but PC2 showed a partial recovery, to 1.224 ± 0.728 (R2), which was significantly higher than in M3. ANOSIM analysis found that the P-value for between M3 and R1 and for between R1 and R2 were, respectively, 0.025 and 0.016, both higher than 0.01 (Supplementary Table S1). This indicated that the fungal community was only weakly recovered. The magnitude of contribution by environmental factors to fungal composition was pH > Mg > TOC > AP > Ca > AK > AN (Figure 5T).
The network became more clustered and interactive with increasing mulching age and looser during recovery. Moreover, AP, AK, and AN were found in the core interactive area of the M0 network, while AP and Ca were found in the core interactive area of M2, and magnesium and calcium were found in the core interactive area of M3. The results indicated that mulching enhanced the complexity of the rhizosphere microbial network and led microbes to focus more on magnesium and calcium (Figure 6).
Figure 6. The co-occurrence networks during the mulching and recovery periods. The red, blue, and black dots indicate bacterial, fungal, and environmental members, respectively. The red and blue lines indicate positive and negative interactions, respectively. The shaded areas indicate the collection of core interactive modules. The subfigures M0 to M3, respectively, indicate mulched for 0 to 3 years, and R1 and R2, respectively, indicate recovered for 1 and 2 years. The TOC, Ca, Mg, AK, AP, and AN indicate total organic carbon, exchangeable calcium, exchangeable magnesium, available potassium, available phosphorus and alkali-hydrolysable nitrogen, respectively.
The number of network nodes gradually increased from 349 (bacterial 302 and fungal 47 in M0) to 466 (bacterial 376 and fungal 90 in M3), then decreased to 375 (bacterial 307 and fungal 68 in R2). The fungal nodes had a higher increment proportion than bacterial nodes (Figure 7A). The link number was also enhanced by mulching, increasing from 478 (140 negative and 338 positive in M0) to 793 (228 negative and 565 positive in M3), before decreasing to 404 (115 negative and 289 positive in R2) (Figure 7D). The avgK, GD, avgCC, and connectedness also increased with mulching age, respectively, reaching maximums of 3.734, 20.31, 0.285, 0.458 in M3, which was about 1.38, 1.35, 3.17, and 2.22 times that of M0 (Figures 7B, C, E, F). The recovery period decreased network parameters to a level that were sometimes even lower than that of stage M0. The avgK and connectedness in R2 were, respectively, 2.126 and 0.053, which was lower than in M0 (2.693 and 0.206). This indicated that the size and the complexity of the network were enhanced by mulching. Also, the number of key nodes in stages M0 to R2 were 3, 2, 3, 6, 2, and 1, and the proportion of Proteobacteria in key nodes increased with mulching, indicating a potential shift in network functional preference (Supplementary Figure S1).
Figure 7. The parameters of co-occurrence networks during the mulching and recovery periods. The M0 to M3, respectively, indicate mulched for 0 to 3 years, and R1 and R2, respectively, indicate recovered for 1 and 2 years. The blue and red part in subfigure (A) indicate fungal and bacterial node numbers, respectively. The blue and red parts in subfigure (D) indicate negative and positive links, respectively. The subfigure (B, C, E, F) depicts the average degree, the average path distance, the average clustering coefficient and the connectedness of networks, respectively.
Both mulching and fertilization influenced soil exchangeable magnesium and calcium content, mulching offered 0.603 standard total effects (STEs), which was nearly 2.4 times the magnitude of fertilization (−0.247) (Figure 8D). Mulching offered −0.742 STEs to microbial community composition, which was about 5.2 times higher than fertilization (0.119). The exchangeable calcium and magnesium offered the greatest STEs to microbial richness (−0.527), while mulching and fertilization offered only −0.318 and 0.24, respectively. Mulching offered −0.887 STEs to network complexity, which was higher than calcium and magnesium (−0.543) and microbial composition (−0.409) offered. These results showed that mulching had a greater impact on the soil exchangeable calcium and magnesium and microbial community properties than fertilization (Figure 8D).
Figure 8. The structural equation model resolving the mechanisms of mulching on Lei-bamboo tissue calcium and magnesium limitation. (A–C) Explain rhizome, stem, and leaf, respectively. Subfigure (D) shows the effects offered by the factors. The solid and dashed lines indicate negative and positive correlations, respectively. The *, **, and *** indicate significant correlations at the level of P < 0.05, P < 0.01, and P < 0.001, respectively. The TOC, Ca, Mg, AK, AP, and AN indicate total organic carbon, exchangeable calcium, exchangeable magnesium, available potassium, available phosphorus, and alkali-hydrolysable nitrogen, respectively. The avgK, GD, avgCC, and con indicate the average degree, the geographic distance, the average clustering coefficient, and connectedness of the structure equation modeling, respectively. B-PC1 and F-PC1 indicate the first axis coordinate of bacterial and fungal community composition of RDA/PCA analysis, respectively. B-Chao and F-Chao indicate bacterial and fungal Chao index, respectively.
The mulching offered, respectively, −0.56, −0.556, and −0.606 STEs to the calcium and magnesium limitation of rhizome, stem, and leaf, and soil calcium and magnesium respectively offered −0.612, −0.505, and −0.45 STEs, while fertilization only offered 0.38, 0.397, and 0.247 STEs, respectively. To the biotic factors, network complexity offered 0.459, 0.484, and 0.549 STEs, respectively, to the calcium and magnesium limitation on rhizome, stem, and leaf, which was much higher than composition and richness offered (Figure 8D). The results indicated that mulching, rather than fertilization, was more influential on the calcium and magnesium limitation in Lei-bamboo tissue, mainly by changing soil Ca and Mg content and by regulating network interactions of the rhizosphere microbial community (not composition or richness).
Soil TOC and acidification were enhanced by mulching (Figures 1A, B), and TOC and pH were highly correlated (Figure 1A). During Lei-bamboo mulching, the soil is covered by a layer of wet organic material more than 25 cm thick, that includes straw and rice husks, to enhance the soil temperature via the metabolic heat released by microbes within the mulch (Wei et al., 1994; Wang et al., 2017). Due to the considerable thickness and water content of the materials, anaerobic fermentation will occur in the anoxic ozone (Qian et al., 2022; Mbukwa et al., 2023), leading to the production of organic acids (Lim et al., 2008; Dionisi and Silva, 2016; Li et al., 2023). The downward transportation of these organic acids via rainfall or irrigation will lower soil pH. Excessive fertilizer, such as urea, will also lead lower soil pH, because of the free protons released when ammonia is oxidized to nitrate (Wang et al., 2023c). Thus, the over application of fertilizer may contribute to the soil acidification of intensively cultivated Lei-bamboo forests (Figures 1C–E). Meanwhile, in the current study, soil pH was partially recovered after removal of the mulch layer, which indicated that the organic acid released by mulching, rather than fertilization, was the main cause of soil acidification.
The contents of soil exchangeable Ca and Mg were significantly lowered by mulching (Figures 1F, G), which was closely related to the enhanced TOC and acidification. Ca and Mg are metals belonging to Group II of the periodic table, and exhibit similar chemical properties and geological processes in soil (Krám et al., 2019). Ca and Mg are mostly enriched in the early and middle stages of magma crystallization, and stored in soil parent materials in the form of metasilicate-type Ca-Mg salts, Ca-Mg olivines, and so forth (Su et al., 2019). Ca and Mg will be released into soil solution with the weathering of soil parent materials, and part of the Ca and Mg in solution will combine with the negatively charged surfaces of soil particles and organic matter, which then become the forms available to plants (Whittinghill and Hobbie, 2012). Climatic factors are determinative to the geochemical cycles of soil Ca and Mg (Boy and Wilcke, 2008; Whittinghill and Hobbie, 2012). In the dry areas, Ca and Mg are prone to form precipitates that are recalcitrant to leaching and weathering (Boy and Wilcke, 2008). While in wet and warm areas, like the locations where Lei-bamboo grow, soil Ca and Mg are vulnerable to leaching (Whittinghill and Hobbie, 2012), while the silica-aluminate parent materials there are recalcitrant to weathering for the release of new Ca and Mg, which led to a lower content of soil exchangeable Ca and Mg (Prietzel et al., 2021). The deficiency of Ca and Mg can be amplified by mulching. The organic matters produced by mulching materials absorb the dissolved Ca and Mg cations, as well as accelerate the leaching of Ca and Mg when they are transported downward by rainfall and irrigation (Adhikari et al., 2019). The increased levels of protons in soil solution compete with Ca and Mg ions for the same negatively charged positions on soil particles, which then force the originally attached Ca and Mg to release as free cations and become more vulnerable to leaching (Clarholm et al., 2015). Thus, through enhanced TOC and acidification, mulching accelerates the leaching of Ca and Mg in soil during intensive cultivation of Lei-bamboo forests, and further lowers soil exchangeable Ca and Mg content.
RMC composition was mainly altered by TOC and pH (Figures 5Q, T). Fermentation in the mulching layer releases intermediate metabolites, most of which are small molecular, labile organic carbon compounds that can be easily utilized by microbes. The enhanced labile organic carbon enriched r-strategy taxa, such as Proteobacteria (Wang et al., 2021) (Figure 5L), but lowered the RA of K-strategy taxa, such as Actinobacteria (Ho et al., 2017) (Figure 5I) and WPS-2 (Sheremet et al., 2020) (Figure 5J), which are capable of degrading recalcitrant organic carbon compounds. Lowered soil pH favors acidophilic groups, including the Acidobacteria (Rousk et al., 2010) (Figure 5E) and Sordariomycetes (Xing et al., 2022) (Figure 5H). It is unclear why community composition did not recover even when the soil physicochemical properties were partially ameliorated. This is possibly connected to the long-term degradation mechanisms of intensively cultivated Lei-bamboo. In contrast to short-term yield reduction, long-term reduction cannot be reversed by removing the mulching alone but requires complete removal and replacement of the soils and replanting with new bamboo, which is costly and, as such, has become a core problem of Lei-bamboo intensive cultivation (Wei et al., 1994). There are several possible reasons. First, the heavy metals that are potentially co-applied with the fertilizers may then accumulate in the soils and plants (Wei et al., 1994). Second, the release of labile organic carbon could induce a “priming effect,” reshaping the soil’s organic carbon composition as well as microbial community composition (Zhang et al., 2019; Shi et al., 2023). Third, long-term intensive cultivation likely caused the deficiency of certain functionally important micro-elements (Kumar et al., 2020). Last, while the α-diversity recovered, the β-diversity did not (Figure 5), which indicated that the contribution of rare species to the RMC composition had been weakened. These rare species may play key roles in certain ecological functions (Jousset et al., 2017; Wang et al., 2023a). Thus, rare species related compositional variation and functional disability might directly drive the long-term reduction in bamboo yield. The mechanisms mentioned above are potential factors that lead to long-term and irreversible changes in the soil microbial community as well as the degradation of Lei-bamboo cultivation but require further confirmation.
It is worth noting that Mg also contributed considerably to RMC composition, even more than AN, AP, and AK did (Figures 5Q, T), which indicated the undervalued importance of Mg in Lei-bamboo ecosystems. Mg could affect the RMC composition in both direct and indirect ways. On the one hand, Mg deficiency might directly enhance the RA of microbial taxa that possess high tolerance to this type of stress. For example, some microbes have a higher capability to solubilize Mg bearing minerals (Silverman and Munoz, 1980; Pokrovsky et al., 2021), others, can absorb the free Mg ions by zooglea, or can store Mg within cell compartments, such as the cell wall (Wang et al., 2019). On the other hand, Mg deficiency might offer indirect effects by altering the availability of other factors. For example, the application of Mg to acidic soil reduces the soil adsorption of phosphorus, thus lowering the relative limitation of phosphorus and altering microbial composition at the enzymic level (Lei et al., 2024). The addition of Mg to rice fields immobilizes Mn, As, and Cd in soil, lowering their potential toxicity to the microbial community (Zhang et al., 2023; Sun et al., 2024).
As mulching age increased, magnesium and calcium also shaped microbial interactions as they gradually appeared in the core interactive areas of the ecological network (Figure 6). The network was constructed from functional modules, which are organized by microbial taxa that possess higher functional connections, including nutrient mineralization and acquisition (Deng et al., 2012). The RMC can alter member interactions according to the variation of specific nutrient limitations, using the core module to remediate the most limiting nutrient, and thus meet the demands of the whole community or host plants (Deng et al., 2012; Zhou et al., 2022). The RMC often organize the network center around phosphorous when the host shrub has high phosphorus demands, but will organize around nitrogen when the host grass has high-nitrogen demands (Zhou et al., 2022). Hence, the data in this study support that the magnesium and calcium limitation of the rhizosphere led a higher microbe functional emphasis in relation to those elements.
Mulching also increased the complexity and interactivity of the RMC network (Figures 6, 7), which indicated a weakened functional dependency between RMC and roots. When facing stress, microbes tend to increase the expression of resistance related genes but decrease the expression of communication and interaction related genes, which reduce the number of interactions, leading to network simplification (Wang et al., 2018b; Zhou et al., 2021). The enhanced interactivity observed in the current study indicated that mulching introduced a more hospitable habitat rather than stresses to the RMC (Hernandez et al., 2021), even though it led to undesirable reduction in bamboo yield (Wei et al., 1994; Wang et al., 2017). Before mulching, the RMC received organic compounds from host plants and reciprocated with inorganic nutrients (Ling et al., 2022). However, the considerable labile organic carbon input from mulching reduced the RMC’s dependency on organic carbon exudates from the roots (Huang et al., 2014), which weakened the mutualistic interactions between microbes and host (Koide and Li, 1990; Hou et al., 2021). The RMC can release organic acids and chelating agents to improve the mobility of metal nutrients (Pokrovsky et al., 2021), and several taxa can use hyphae to break soil aggregates and expose the mineralized nutrients inside (Hou et al., 2021). Thus, the weakened mutualism in turn possibly hampered microbial assistance to host magnesium and calcium uptake.
Even though network parameters recovered after removal of the mulch (Figures 6, 7), the community composition did not (Figures 5R, S, U, V), which indicated that network complexity and interactivity, rather than community composition, may be a better indicator for host dominance. For example, Tricholoma matsutake was marginalized from its RMC network due to climate change, which reduced the yield of T. matsutake and lead to the risk of regional extinction (Zhou et al., 2021). In high richness systems with high functional redundancy (Louca et al., 2018), community members can change their interactive pattern to meet host demands (Averill et al., 2022), and a well-organized RMC network is highly associated with a better rhizosphere multifunctionality, including magnesium and calcium solubilization and assimilation (Chen et al., 2022; Wang et al., 2023b; Zhai et al., 2024). Thus, improving the network structure of the RMC is a potential method to alleviate magnesium and calcium limitations.
In this study, it was found that the magnesium and calcium limitations of Lei-bamboo were enhanced by mulching (Figures 4C, D, F–I). Mulching probably interferes with calcium and magnesium acquisition of Lei-bamboo in two ways. First, by reducing the content of exchangeable magnesium and calcium, which directly decreased the potential quantity available for assimilation (Tang and Luan, 2017) (Figure 8). Second, by altering RMC network interactivity and weakening the functional dependency between RMC and roots, which further enhanced the difficulties of Lei-bamboo to take up magnesium and calcium (Pokrovsky et al., 2021) (Figure 8).
The increasing magnitude of magnesium and calcium limitation in Lei-bamboo is rhizome > stem > leaf (Figure 4), which indicated that magnesium and calcium deficiency led to a physiological response by the plant. Plants will preferentially supply the leaves with limited nutrients to ensure the stability of photosynthesis, which can improve individual competence under nutrient deficiency (Farhat et al., 2016). Moreover, the nitrogen content in Lei-bamboo was reduced despite increased AN in the soil (Figure 3A), which supported that nitrogen assimilation might be hampered by magnesium and calcium limitation. Mg can reduce nitrogen loss through decreased N2O emissions and nitrate leaching, enhancing the available nitrogen pool for crops (He et al., 2023). Mg also mitigates alumina toxicity in acidic soils and improves crop nitrogen uptake by roots (Lyu et al., 2023). Mg accelerates the leaf nitrogen storage in the form of amino acids (He et al., 2023). The application of Mg fertilizer can even double the effects of nitrogen fertilizers in intensively cultivated peanut fields (Gao et al., 2024b). These studies show that Mg can affect crop nitrogen utilization through the regulation of the soil nitrogen cycle, root assimilation, and magnesium transportation and storage. Ca was reported to possess similar functions (Lian et al., 2022; Yang et al., 2023; Gao et al., 2024a). Thus, the limitation of magnesium and calcium can induce yield degradation by hampering the utilization of other nutrients.
Considering that while AN, AP, and AK increased with mulching age (Figures 1C–E), but yield decreased, we suggest the application of nitrogen, phosphorus, and potassium had far exceeded the true demands of Lei-bamboo. More attention should be paid to the dynamics and limitations of magnesium and calcium, as well as other medium-quantity nutrients and micronutrients (Kumar et al., 2020; Ahmed et al., 2024). Over fertilization reduces utilization efficiency, increases production cost, and leads to the pollution and eutrophication of underground water, rivers, and lakes (Wang et al., 2018a; Tian et al., 2022; Huang et al., 2023). Excessive nutrients give the wrong signals to roots, misleading them to believe that nutrient demands could be meet by fewer roots (Chen et al., 2023), resulting in ill-developed roots and RMCs that in turn impedes the uptake of magnesium and calcium (Gui et al., 2013, 2018).
In this study, the enhanced TOC and acidification induced by mulching were the fundamental reasons for Lei-bamboo magnesium and calcium limitation, with the deficiency of magnesium and calcium in soil as potential direct reasons, and the altered RMC network considerably magnifying the effects of soil magnesium and calcium deficiency (Figure 8). Hence, we suggest to find alternative mulching materials that will not release organic acids (Wei et al., 1994; Li et al., 2023), or the application of biochar to buffer the acidification and increase the number of potential binding sites for free magnesium and calcium ions (Zhao et al., 2020; Zhang et al., 2023). We strongly recommend the application of CaO (Li et al., 2019) and MgO (Zhang et al., 2022) immediately after mulching as this inexpensive method can sterilize the pathogens enriched during mulching, increase soil pH, and directly supply magnesium and calcium to soils. Last, an effective microbial fertilizer to prevent the weakening of functional mutualism between RMC and roots is also an ecofriendly method (Huang et al., 2014).
As the mulching age increased, the TOC, AN, AP, and AK were enhanced but the exchangeable magnesium and calcium were reduced, maybe due to the acidification caused by organic acid released from mulching layer. This was a direct reason for the increased Ca and Mg-related stoichiometry (N:Mg, etc) in soil and Lei-bamboo tissue. Meanwhile, mulching increased network interactivity of RMC, a sign of weakened mutualism between RMC and root, which possibly in turn hampered the uptake of magnesium and calcium in root and amplified the magnesium and calcium limitation on Lei-bamboo tissue. This research highlighted the potential limitation on magnesium and calcium rather than usually fertilized nitrogen, phosphorus and potassium, which offered practical information for balancing fertilization on Lei-bamboo mulching cultivation and might help preventing short-term yielding degradations and boosting sustainable development of Lei-bamboo forest.
The raw sequencing data are available in NCBI Sequence Read Archive of PRJNA1114674 (bacterial) and PRJNA1114879 (fungal).
HZ: Conceptualization, Data curation, Formal analysis, Funding acquisition, Investigation, Methodology, Project administration, Resources, Software, Supervision, Validation, Visualization, Writing – original draft, Writing – review & editing. SH: Data curation, Investigation, Methodology, Writing – review & editing. ZZ: Data curation, Formal analysis, Investigation, Methodology, Resources, Writing – review & editing. TL: Methodology, Project administration, Visualization, Writing – review & editing. YL: Investigation, Methodology, Visualization, Writing – review & editing. GZ: Writing – review & editing. GL: Writing – review & editing. BF: Supervision, Writing – review & editing. XK: Funding acquisition, Resources, Supervision, Writing – review & editing.
The author(s) declare financial support was received for the research, authorship, and/or publication of this article. The Young Scientist Program in Jiangxi Academy of Forestry, No.20230714A and The Post-Doctor Program in Jiangxi Academy of Forestry No.20220701A.
The authors declare that the research was conducted in the absence of any commercial or financial relationships that could be construed as a potential conflict of interest.
All claims expressed in this article are solely those of the authors and do not necessarily represent those of their affiliated organizations, or those of the publisher, the editors and the reviewers. Any product that may be evaluated in this article, or claim that may be made by its manufacturer, is not guaranteed or endorsed by the publisher.
The Supplementary Material for this article can be found online at: https://www.frontiersin.org/articles/10.3389/fpls.2024.1492137/full#supplementary-material
Abarenkov, K., Henrik Nilsson, R., Larsson, K.-H., Alexander, I. J., Eberhardt, U., Erland, S., et al. (2010). The UNITE database for molecular identification of fungi – recent updates and future perspectives. New Phytol. 186, 281–285. doi: 10.1111/j.1469-8137.2009.03160.x
Adams, R. I., Miletto, M., Taylor, J. W., Bruns, T. D. (2013). Dispersal in microbes: fungi in indoor air are dominated by outdoor air and show dispersal limitation at short distances. ISME J. 7, 1262–1273. doi: 10.1038/ismej.2013.28
Adhikari, D., Sowers, T., Stuckey, J. W., Wang, X., Sparks, D. L., Yang, Y. (2019). Formation and redox reactivity of ferrihydrite-organic carbon-calcium co-precipitates. Geochimica Cosmochimica Acta 244, 86–98. doi: 10.1016/j.gca.2018.09.026
Ahmed, N., Zhang, B., Chachar, Z., Li, J., Xiao, G., Wang, Q., et al. (2024). Micronutrients and their effects on Horticultural crop quality, productivity and sustainability. Scientia Hortic. 323, 112512. doi: 10.1016/j.scienta.2023.112512
Aslam, M. M., Okal, E. J., Idris, A. L., Qian, Z., Xu, W., Karanja, J. K., et al. (2022). Rhizosphere microbiomes can regulate plant drought tolerance. Pedosphere 32, 61–74. doi: 10.1016/S1002-0160(21)60061-9
Averill, C., Anthony, M. A., Baldrian, P., Finkbeiner, F., van den Hoogen, J., Kiers, T., et al. (2022). Defending Earth’s terrestrial microbiome. Nat. Microbiol. 7, 1717–1725. doi: 10.1038/s41564-022-01228-3
Beeckman, F., Motte, H., Beeckman, T. (2018). Nitrification in agricultural soils: impact, actors and mitigation. Curr. Opin. Biotechnol. 50, 166–173. doi: 10.1016/j.copbio.2018.01.014
Boy, J., Wilcke, W. (2008). Tropical Andean forest derives calcium and magnesium from Saharan dust. Global Biogeochemical Cycles 22, 1–11. doi: 10.1029/2007GB002960
Chen, S., Chen, S., Guo, Z. (2015). Effects of mulching management on the internal cycling of nutrient elements in the rhizomatous roots of Phyllostachys violascens. J. Acta Ecologica Sin. 35, 5788–5796.
Chen, Z., Wang, L., Cardoso, J. A., Zhu, S., Liu, G., Rao, I. M., et al. (2023). Improving phosphorus acquisition efficiency through modification of root growth responses to phosphate starvation in legumes 14, 1094157. doi: 10.3389/fpls.2023.1094157
Chen, W., Wang, J., Chen, X., Meng, Z., Xu, R., Duoji, D., et al. (2022). Soil microbial network complexity predicts ecosystem function along elevation gradients on the Tibetan Plateau. Soil Biol. Biochem. 172, 108766. doi: 10.1016/j.soilbio.2022.108766
Chen, B., Yang, H., Song, W., Liu, C., Xu, J., Zhao, W., et al. (2016). Effect of N fertilization rate on soil alkali-hydrolyzable N, subtending leaf N concentration, fiber yield, and quality of cotton. Crop J. 4, 323–330. doi: 10.1016/j.cj.2016.03.006
Clarholm, M., Skyllberg, U., Rosling, A. (2015). Organic acid induced release of nutrients from metal-stabilized soil organic matter – The unbutton model. Soil Biol. Biochem. 84, 168–176. doi: 10.1016/j.soilbio.2015.02.019
Clark, J. M., Chapman, P. J., Adamson, J. K., Lane, S. N. (2005). Influence of drought-induced acidification on the mobility of dissolved organic carbon in peat soils. Global Change Biol. 11, 791–809. doi: 10.1111/j.1365-2486.2005.00937.x
Contreras, S., Werne, J. P., Araneda, A., Urrutia, R., Conejero, C. A. (2018). Organic matter geochemical signatures (TOC, TN, C/N ratio, δ13C and δ15N) of surface sediment from lakes distributed along a climatological gradient on the western side of the southern Andes. Sci. Total Environ. 630, 878–888. doi: 10.1016/j.scitotenv.2018.02.225
Dai, Z., Su, W., Chen, H., Barberán, A., Zhao, H., Yu, M., et al. (2018). Long-term nitrogen fertilization decreases bacterial diversity and favors the growth of Actinobacteria and Proteobacteria in agro-ecosystems across the globe. Global Change Biol. 24, 3452–3461. doi: 10.1111/gcb.14163
Das, D., Dwivedi, B. S., Datta, S. P., Datta, S. C., Meena, M. C., Dwivedi, A. K., et al. (2021). Long-term differences in nutrient management under intensive cultivation alter potassium supplying ability of soils. Geoderma 393, 114983. doi: 10.1016/j.geoderma.2021.114983
Deng, Y., Jiang, Y.-H., Yang, Y., He, Z., Luo, F., Zhou, J. (2012). Molecular ecological network analyses. BMC Bioinf. 13, 113. doi: 10.1186/1471-2105-13-113
Dionisi, D., Silva, I. M. O. (2016). Production of ethanol, organic acids and hydrogen: an opportunity for mixed culture biotechnology? Rev. Environ. Sci. Bio/Technology 15, 213–242. doi: 10.1007/s11157-016-9393-y
Edgar, R. C. (2013). UPARSE: highly accurate OTU sequences from microbial amplicon reads. Nat. Methods 10, 996–998. doi: 10.1038/nmeth.2604
Farhat, N., Elkhouni, A., Zorrig, W., Smaoui, A., Abdelly, C., Rabhi, M., et al. (2016). Effects of magnesium deficiency on photosynthesis and carbohydrate partitioning. Acta Physiologiae Plantarum 38, 145. doi: 10.1007/s11738-016-2165-z
Gao, Y., Chen, S., Yang, M., Hao, Z., Wang, X., Shi, Y. (2024a). Nano calcium carbonate improves wheat nitrogen accumulation and grain yield by enhancing soil nitrogen supply and flag leaf photosynthetic characteristics. Field Crops Res. 310, 109341. doi: 10.1016/j.fcr.2024.109341
Gao, Y., Zeng, R., Yao, S., Wang, Y., Wang, J., Wan, S., et al. (2024b). Magnesium fertilizer application increases peanut growth and pod yield under reduced nitrogen application in southern China. Crop J. 12, 915–926. doi: 10.1016/j.cj.2024.03.008
Garbeva, P., van Elsas, J. D., van Veen, J. A. (2008). Rhizosphere microbial community and its response to plant species and soil history. Plant Soil 302, 19–32. doi: 10.1007/s11104-007-9432-0
Guan, P., Wang, R., Nkoh, J. N., Shi, R., Pan, X., Li, J., et al. (2022). Enriching organic carbon bioavailability can mitigate soil acidification induced by nitrogen fertilization in croplands through microbial nitrogen immobilization 86, 579–592. doi: 10.1002/saj2.20400
Gui, R., Sun, X., Zhuang, S. (2013). Soil acidification in Phyllostachys praecox f. preveynalis cultivation with intensive management. Commun. Soil Sci. Plant Anal. 44, 3235–3245. doi: 10.1080/00103624.2013.841915
Gui, R., Wu, W., Zhuang, S., Zhong, Z. (2018). Intensive management increases soil acidification and phytotoxic Al content in Phyllostachys praecox stands in Southeast China. J. Sustain. Forestry 37, 46–55. doi: 10.1080/10549811.2017.1405822
He, D., Chen, X., Zhang, Y., Huang, Z., Yin, J., Weng, X., et al. (2023). Magnesium is a nutritional tool for the yield and quality of oolong tea (Camellia sinensis L.) and reduces reactive nitrogen loss. Scientia Hortic. 308, 111590. doi: 10.1016/j.scienta.2022.111590
Hernandez, D. J., David, A. S., Menges, E. S., Searcy, C. A., Afkhami, M. E. (2021). Environmental stress destabilizes microbial networks. ISME J. 15, 1722–1734. doi: 10.1038/s41396-020-00882-x
Ho, A., Di Lonardo, D. P., Bodelier, P. L. E. (2017). Revisiting life strategy concepts in environmental microbial ecology. FEMS Microbiol. Ecol. 93, fix006. doi: 10.1093/femsec/fix006
Hou, L., Zhang, X., Feng, G., Li, Z., Zhang, Y., Cao, N. (2021). Arbuscular mycorrhizal enhancement of phosphorus uptake and yields of maize under high planting density in the black soil region of China. Sci. Rep. 11, 1100. doi: 10.1038/s41598-020-80074-x
Huang, X.-F., Chaparro, J. M., Reardon, K. F., Zhang, R., Shen, Q., Vivanco, J. M. (2014). Rhizosphere interactions: root exudates, microbes, and microbial communities. Botany 92, 267–275. doi: 10.1139/cjb-2013-0225
Huang, C., Fu, S., Ma, X., Ren, X., Tian, X., Tong, Y., et al. (2023). Long-term intensive management reduced the soil quality of a Carya dabieshanensis forest. Sci. Rep. 13, 5058. doi: 10.1038/s41598-023-32237-9
Huang, B., Yan, D., Ouyang, C., Zhang, D., Zhu, J., Liu, J., et al. (2020). Chloropicrin fumigation alters the soil phosphorus and the composition of the encoding alkaline phosphatase PhoD gene microbial community. Sci. Total Environ. 711, 135080. doi: 10.1016/j.scitotenv.2019.135080
Jiang, Y., Li, S., Li, R., Zhang, J., Liu, Y., Lv, L., et al. (2017). Plant cultivars imprint the rhizosphere bacterial community composition and association networks. Soil Biol. Biochem. 109, 145–155. doi: 10.1016/j.soilbio.2017.02.010
Jiang, P., Yu, Y., Jin, A., Wang, A., Yu, Q. (2000). Analysis on nutrients of soil under high-yield Phyllostachys praecox f. prevernalis forest. J. Bamboo Res. (in Chinese) 19, 50–53.
Jing, T., Li, J., He, Y., Shankar, A., Saxena, A., Tiwari, A., et al. (2024). Role of calcium nutrition in plant Physiology: Advances in research and insights into acidic soil conditions - A comprehensive review. Plant Physiol. Biochem. 210, 108602. doi: 10.1016/j.plaphy.2024.108602
Jousset, A., Bienhold, C., Chatzinotas, A., Gallien, L., Gobet, A., Kurm, V., et al. (2017). Where less may be more: how the rare biosphere pulls ecosystems strings. ISME J. 11, 853–862. doi: 10.1038/ismej.2016.174
Koide, R. T., Li, M. (1990). On host regulation of the vesicular-arbuscular mycorrhizal symbiosis. New Phytol. 114, 59–64. doi: 10.1111/j.1469-8137.1990.tb00373.x
Krám, P., Oulehle, F., Hruska, J., Veselovský, F., Čuřík, J., Myška, O., et al. (2019). Calcium and magnesium biogeochemistry in spruce catchments underlain by felsic, mafic, and ultramafic rocks. EDP Sciences 98, 1–6. doi: 10.1051/e3sconf/20199806007
Kumar, D., Patel, K. P., Ramani, V. P., Shukla, A. K., Meena, R. S. (2020). “Management of Micronutrients in Soil for the Nutritional Security,” in Nutrient Dynamics for Sustainable Crop Production. Ed. Meena, R. S. (Springer Singapore, Singapore), 103–134.
Kurokawa, K., Nakao, A., Wakabayashi, S., Fujimura, S., Eguchi, T., Matsunami, H., et al. (2020). Advanced approach for screening soil with a low radiocesium transfer to brown rice in Fukushima based on exchangeable and nonexchangeable potassium. Sci. Total Environ. 743, 140458. doi: 10.1016/j.scitotenv.2020.140458
Le, V. S., Herrmann, L., Hudek, L., Nguyen, T. B., Bräu, L., Lesueur, D. (2022). How application of agricultural waste can enhance soil health in soils acidified by tea cultivation: a review. Environ. Chem. Lett. 20, 813–839. doi: 10.1007/s10311-021-01313-9
Lei, J., Yin, J., Chen, S., Fenton, O., Liu, R., Chen, Q., et al. (2024). Understanding phosphorus mobilization mechanisms in acidic soil amended with calcium-silicon-magnesium-potassium fertilizer. Sci. Total Environ. 916, 170294. doi: 10.1016/j.scitotenv.2024.170294
Li, Y., Cui, S., Chang, S. X., Zhang, Q. (2019). Liming effects on soil pH and crop yield depend on lime material type, application method and rate, and crop species: a global meta-analysis. J. Soils Sediments 19, 1393–1406. doi: 10.1007/s11368-018-2120-2
Li, J., Ma, D., Tian, J., Sun, T., Meng, Q., Li, J., et al. (2023). The responses of organic acid production and microbial community to different carbon source additions during the anaerobic fermentation of Chinese cabbage waste. Bioresource Technol. 371, 128624. doi: 10.1016/j.biortech.2023.128624
Lian, H., Qin, C., Zhao, Q., Begum, N., Zhang, S. (2022). Exogenous calcium promotes growth of adzuki bean (Vigna angularis Willd.) seedlings under nitrogen limitation through the regulation of nitrogen metabolism. Plant Physiol. Biochem. 190, 90–100. doi: 10.1016/j.plaphy.2022.08.028
Lim, S.-J., Kim, B. J., Jeong, C.-M., Choi, J.-D.-R., Ahn, Y. H., Chang, H. N. (2008). Anaerobic organic acid production of food waste in once-a-day feeding and drawing-off bioreactor. Bioresource Technol. 99, 7866–7874. doi: 10.1016/j.biortech.2007.06.028
Ling, N., Wang, T., Kuzyakov, Y. (2022). Rhizosphere bacteriome structure and functions. Nat. Commun. 13, 836. doi: 10.1038/s41467-022-28448-9
Liu, T., Cui, C., He, J., Tang, J. (2018). Insights into the succession of the bacterial microbiota during biodrying of storage sludge mixed with beer lees: Studies on its biodiversity, structure, associations, and functionality. Sci. Total Environ. 644, 1088–1100. doi: 10.1016/j.scitotenv.2018.06.298
Louca, S., Polz, M. F., Mazel, F., Albright, M. B. N., Huber, J. A., O’Connor, M. I., et al. (2018). Function and functional redundancy in microbial systems. Nat. Ecol. Evol. 2, 936–943. doi: 10.1038/s41559-018-0519-1
Lyu, M., Liu, J., Xu, X., Liu, C., Qin, H., Zhang, X., et al. (2023). Magnesium alleviates aluminum-induced growth inhibition by enhancing antioxidant enzyme activity and carbon–nitrogen metabolism in apple seedlings. Ecotoxicology Environ. Saf. 249, 114421. doi: 10.1016/j.ecoenv.2022.114421
Martiny, A. C., Pham, C. T. A., Primeau, F. W., Vrugt, J. A., Moore, J. K., Levin, S. A., et al. (2013). Strong latitudinal patterns in the elemental ratios of marine plankton and organic matter. Nat. Geosci. 6, 279–283. doi: 10.1038/ngeo1757
Mbukwa, D., Gui, R., Deng, S. (2023). Effects of aeration treatments on root and rhizome growth parameters of Phyllostachys violascens (Lei bamboo) under intensive cultivation: A field study. Sci. Total Environ. 900, 165738. doi: 10.1016/j.scitotenv.2023.165738
Pausch, J., Holz, M., Zhu, B., Cheng, W. (2024). Rhizosphere priming promotes plant nitrogen acquisition by microbial necromass recycling. Plant Cell Environ. 47, 1987–1996. doi: 10.1111/pce.14858
Pokrovsky, O. S., Shirokova, L. S., Zabelina, S. A., Jordan, G., Bénézeth, P. (2021). Weak impact of microorganisms on Ca, Mg-bearing silicate weathering. NPJ Materials Degradation 5, 51. doi: 10.1038/s41529-021-00199-w
Prietzel, J., Klysubun, W., Hurtarte, L. C. C. (2021). The fate of calcium in temperate forest soils: a Ca K-edge XANES study. Biogeochemistry 152, 195–222. doi: 10.1007/s10533-020-00748-6
Prüsse, E., Quast, C., Yilmaz, P., Ludwig, W., Peplies, J., Glöckner, F. O. (2011). SILVA: Comprehensive databases for quality checked and aligned ribosomal RNA sequence data compatible with ARB. Nucleic Acid Research 35, 7188–7196.
Qian, Z., Zhuang, S., Gao, J., Tang, L., Harindintwali, J. D., Wang, F. (2022). Aeration increases soil bacterial diversity and nutrient transformation under mulching-induced hypoxic conditions. Sci. Total Environ. 817, 153017. doi: 10.1016/j.scitotenv.2022.153017
Rousk, J., Bååth, E., Brookes, P. C., Lauber, C. L., Lozupone, C., Caporaso, J. G., et al. (2010). Soil bacterial and fungal communities across a pH gradient in an arable soil. ISME J. 4, 1340–1351. doi: 10.1038/ismej.2010.58
Sheremet, A., Jones, G. M., Jarett, J., Bowers, R. M., Bedard, I., Culham, C., et al. (2020). Ecological and genomic analyses of candidate phylum WPS-2 bacteria in an unvegetated soil. Environ. Microbiol. 22, 3143–3157. doi: 10.1111/1462-2920.15054
Shi, J., Yang, L., Liao, Y., Li, J., Jiao, S., Shangguan, Z., et al. (2023). Soil labile organic carbon fractions mediate microbial community assembly processes during long-term vegetation succession in a semiarid region. iMeta 2, e142. doi: 10.1002/imt2.142
Silverman, M. P., Munoz, E. F. (1980). Microbial mobilization of calcium and magnesium in waterlogged soils. J. Environ. Qual. 9, 9–12. doi: 10.2134/jeq1980.00472425000900010003x
Su, B.-X., Hu, Y., Teng, F.-Z., Xiao, Y., Zhang, H.-F., Sun, Y., et al. (2019). Light Mg isotopes in mantle-derived lavas caused by chromite crystallization, instead of carbonatite metasomatism. Earth Planetary Sci. Lett. 522, 79–86. doi: 10.1016/j.epsl.2019.06.016
Sun, T., Li, G., Mazarji, M., Delaplace, P., Yang, X., Zhang, J., et al. (2024). Heavy metals drive microbial community assembly process in farmland with long-term biosolids application. J. Hazardous Materials 468, 133845. doi: 10.1016/j.jhazmat.2024.133845
Tang, R.-J., Luan, S. (2017). Regulation of calcium and magnesium homeostasis in plants: from transporters to signaling network. Curr. Opin. Plant Biol. 39, 97–105. doi: 10.1016/j.pbi.2017.06.009
Tian, K., Li, M., Hu, W., Fan, Y. N., Huang, B., Zhao, Y. (2022). Environmental capacity of heavy metals in intensive agricultural soils: Insights from geochemical baselines and source apportionment. Sci. Total Environ. 819, 153078. doi: 10.1016/j.scitotenv.2022.153078
Trivedi, P., Leach, J. E., Tringe, S. G., Sa, T., Singh, B. K. (2020). Plant–microbiome interactions: from community assembly to plant health. Nat. Rev. Microbiol. 18, 607–621. doi: 10.1038/s41579-020-0412-1
Venturi, V., Keel, C. (2016). Signaling in the rhizosphere. Trends Plant Sci. 21, 187–198. doi: 10.1016/j.tplants.2016.01.005
Vorholt, J. A., Vogel, C., Carlström, C. I., Müller, D. B. (2017). Establishing causality: opportunities of synthetic communities for plant microbiome research. Cell Host Microbe 22, 142–155. doi: 10.1016/j.chom.2017.07.004
Wang, F., Chen, S., Wang, Y., Zhang, Y., Hu, C., Liu, B. (2018a). Long-term nitrogen fertilization elevates the activity and abundance of nitrifying and denitrifying microbial communities in an upland soil: implications for nitrogen loss from intensive agricultural systems 9, 2424. doi: 10.3389/fmicb.2018.02424
Wang, T., Flint, S., Palmer, J. (2019). Magnesium and calcium ions: roles in bacterial cell attachment and biofilm structure maturation. Biofouling 35, 959–974. doi: 10.1080/08927014.2019.1674811
Wang, C., Guo, L., Shen, R. F. (2023a). Rare microbial communities drive ecosystem multifunctionality in acidic soils of southern China. Appl. Soil Ecol. 189, 104895. doi: 10.1016/j.apsoil.2023.104895
Wang, Z., Tao, T., Wang, H., Chen, J., Small, G. E., Johnson, D., et al. (2023c). Forms of nitrogen inputs regulate the intensity of soil acidification. Global Change Biol. 29, 4044–4055. doi: 10.1111/gcb.16746
Wang, S., Wang, X., Han, X., Deng, Y. (2018b). Higher precipitation strengthens the microbial interactions in semi-arid grassland soils. Global Ecol. Biogeography 27, 570–580. doi: 10.1111/geb.12718
Wang, H., Zeng, Q., Cheng, P., Yu, L. (2017). The current developing state and strategy for Lei bamboo economy in Jiangxi provicne. Communication World Bamboo Ratten (in Chinese) 15, 54–58.
Wang, X., Zhang, W., Liu, Y., Jia, Z., Li, H., Yang, Y., et al. (2021). Identification of microbial strategies for labile substrate utilization at phylogenetic classification using a microcosm approach. Soil Biol. Biochem. 153, 107970. doi: 10.1016/j.soilbio.2020.107970
Wang, X., Zhang, Q., Zhang, Z., Li, W., Liu, W., Xiao, N., et al. (2023b). Decreased soil multifunctionality is associated with altered microbial network properties under precipitation reduction in a semiarid grassland. iMeta 2, e106. doi: 10.1002/imt2.106
Wei, F., Junchao, H., Xueke, L., Jianhua, C. (1994). Cultivation teehniques of early shooting and high yielding for Lei bamboo sprout. J. Zhejiang A&F Univ. 11, 121–128.
Weston, R., Gore, P. A. (2006). A brief guide to structural equation modeling. Couns. Psychol. 34, 719–751. doi: 10.1177/0011000006286345
Whittinghill, K. A., Hobbie, S. E. (2012). Effects of pH and calcium on soil organic matter dynamics in Alaskan tundra. Biogeochemistry 111, 569–581. doi: 10.1007/s10533-011-9688-6
Xing, W., Lu, X., Ying, J., Lan, Z., Chen, D., Bai, Y. (2022). Disentangling the effects of nitrogen availability and soil acidification on microbial taxa and soil carbon dynamics in natural grasslands. Soil Biol. Biochem. 164, 108495. doi: 10.1016/j.soilbio.2021.108495
Yang, S.-X., Liao, B., Li, J.-t., Guo, T., Shu, W.-S. (2010). Acidification, heavy metal mobility and nutrient accumulation in the soil–plant system of a revegetated acid mine wasteland. Chemosphere 80, 852–859. doi: 10.1016/j.chemosphere.2010.05.055
Yang, S., Yang, L., Wen, D., Liu, L., Ni, K., Cao, J., et al. (2023). Soil calcium constrains nitrogen mineralization and nitrification rates in subtropical karst regions. Soil Biol. Biochem. 186, 109176. doi: 10.1016/j.soilbio.2023.109176
Zhai, C., Han, L., Xiong, C., Ge, A., Yue, X., Li, Y., et al. (2024). Soil microbial diversity and network complexity drive the ecosystem multifunctionality of temperate grasslands under changing precipitation. Sci. Total Environ. 906, 167217. doi: 10.1016/j.scitotenv.2023.167217
Zhang, T., Jiku, M. A. S., Li, L., Ren, Y., Li, L., Zeng, X., et al. (2023). Soil ridging combined with biochar or calcium-magnesium-phosphorus fertilizer application: Enhanced interaction with Ca, Fe and Mn in new soil habitat reduces uptake of As and Cd in rice. Environ. pollut. 332, 121968. doi: 10.1016/j.envpol.2023.121968
Zhang, W., Liu, Y., Muneer, M. A., Jin, D., Zhang, H., Cai, Y., et al. (2022). Characterization of different magnesium fertilizers and their effect on yield and quality of soybean and pomelo. Agronomy. 12, 2693. doi: 10.3390/agronomy12112693
Zhang, Z., Wang, W., Qi, J., Zhang, H., Tao, F., Zhang, R. (2019). Priming effects of soil organic matter decomposition with addition of different carbon substrates. J. Soils Sediments 19, 1171–1178. doi: 10.1007/s11368-018-2103-3
Zhao, W., Li, J., Deng, K., Shi, R., Jiang, J., Hong, Z., et al. (2020). Effects of crop straw biochars on aluminum species in soil solution as related with the growth and yield of canola (Brassica napus L.) in an acidic Ultisol under field condition. Environ. Sci. pollut. Res. 27, 30178–30189. doi: 10.1007/s11356-020-09330-x
Zhou, H., Ma, A., Liu, G., Zhou, X., Yin, J., Liang, Y., et al. (2021). Reduced interactivity during microbial community degradation leads to the extinction of Tricholomas matsutake. Land Degradation Dev. 32, 5118–5128. doi: 10.1002/ldr.4098
Zhou, H., Ma, A., Zhou, X., Chen, X., Zhang, J., Zhang, Q., et al. (2022). Phosphorus shapes soil microbial community composition and network properties during grassland expansion into shrubs in Tibetan dry valleys. Front. Plant Sci. 13. doi: 10.3389/fpls.2022.848691
Keywords: organic materials mulching, Lei-bamboo, rhizosphere microbial community, stoichiometry, magnesium, calcium
Citation: Zhou H, Huang S, Zhang Z, Li T, Li Y, Zhuang G, Liu G, Fu B and Kuang X (2024) Network and stoichiometry analysis revealed a fast magnesium and calcium deficiency of mulched Phyllostachys violascens. Front. Plant Sci. 15:1492137. doi: 10.3389/fpls.2024.1492137
Received: 06 September 2024; Accepted: 16 October 2024;
Published: 27 November 2024.
Edited by:
Bo Zhang, Chinese Academy of Sciences (CAS), ChinaReviewed by:
Tieguang He, Guangxi Academy of Agricultural Science, ChinaCopyright © 2024 Zhou, Huang, Zhang, Li, Li, Zhuang, Liu, Fu and Kuang. This is an open-access article distributed under the terms of the Creative Commons Attribution License (CC BY). The use, distribution or reproduction in other forums is permitted, provided the original author(s) and the copyright owner(s) are credited and that the original publication in this journal is cited, in accordance with accepted academic practice. No use, distribution or reproduction is permitted which does not comply with these terms.
*Correspondence: Bojie Fu, YmZ1QHJjZWVzLmFjLmNu; Xiaobao Kuang, a3Vhbmd4YjIwMjRAMTYzLmNvbQ==
Disclaimer: All claims expressed in this article are solely those of the authors and do not necessarily represent those of their affiliated organizations, or those of the publisher, the editors and the reviewers. Any product that may be evaluated in this article or claim that may be made by its manufacturer is not guaranteed or endorsed by the publisher.
Research integrity at Frontiers
Learn more about the work of our research integrity team to safeguard the quality of each article we publish.