- 1Center of Molecular Ecophysiology (CMEP), College of Resources and Environment, Southwest University, Chongqing, China
- 2College of Resources and Environment, Southwest University, Chongqing, China
- 3Interdisciplinary Research Center for Agriculture Green Development in Yangtze River Basin, Southwest University, Chongqing, China
Introduction: Groundcover management plays a crucial role in improving water retention and soil nutrition in orchard systems, thereby preventing environmental constrains by non-point source pollution. However, effectiveness of groundcover management in citrus orchards developed on sloping farmland with eroded purple soil has not been studied in detail. In particular, information on the soil nutrient losses, e.g., nitrogen (N) and phosphorus (P), through interflow and its effects on growth and nutrition of citrus plants has not been reported.
Methods: The present study evaluated the effects of different cover crops, i.e., Lolium perenne L. (Lolium), Vicia villosa Roth (Vicia) and Orychophragmus violaceus (Ory), on nutrition and growth of citrus trees as well as water, soil and nutrient retention in an orchard developed in sloping farmland during two consecutive years.
Results and discussion: The results show that the groundcover species Lolium and Vicia mediated nursing effects on nutrition and growth of citrus trees. These nursing effects included enhanced foliar levels of carbon(C), N and P as well as increased tree height, stem diameter, and crown width. Groundcover management generally reduced the annual surface runoff, interflow, soil loss, total N loss and total P loss. Among the cover crop species studied, Lolium and Vicia were overall more efficient than Ory in this context. Lolium reduced the average annual total loss of N and P by 42.53% and 49.23%, respectively, compared with clean tillage. The estimated annual reduction potentials of soil, N and P losses in Southwestern China were 16.3, 3.4 and 8.5 million tons yr-1, respectively. Obviously, Lolium and Vicia provide highly beneficial ground coverage on sloping farmland and, thus, can be used for future sustainable development of citrus orchards.
1 Introduction
Land degradation by erosion is a critical environmental problem worldwide that threatens global soil fertility and sustainable agriculture (Prosdocimi et al., 2016; Xiong et al., 2018; Poesen, 2018). This issue is especially significance in Southwest China, where poor agricultural practices under extreme rainfall have caused widespread soil erosion and nutrient leaching (Su et al., 2022). Nitrogen (N) and phosphorus (P) losses via runoff are major contributors to agricultural non-point source pollution, environment, and human health (Bowles et al., 2018; Liu et al., 2021). Particularly, orchards grown on farmland slopes are among the most severe areas to these losses (Wang et al., 2018; Du et al., 2021).
Farmland slopes on purple soil in Southwest China have been facing severe soil, water, and nutrient losses due to hilly terrain, high precipitation, and unsustainable cultivation practices. These farmland slopes cover 7.67 million ha, accounting for ca. 32.0% of sloping land nationwide (Zhang et al., 2016; Dai et al., 2018). Effective land management, such as groundcover, is essential to control runoff, reduce erosion, and improve nutrient status on degraded and/or marginal farmland (Zhang et al., 2016). Moreover, interflow is a major pathway for N and P losses from these systems, making it crucial to manage nutrient leaching and promote sustainable orchard management.
Citrus orchards on purple soil farmland slopes are economically important in Southwest China. However, the space between rows of citrus trees was usually bare due to herbicide application as a common farming practice, thus lacking surface vegetation cover. This is of particular significance for these farming systems, because purple soil in this area is characterized by steep slopes, a shallow soil layer, high gravel content, weak water and fertilizer retention (Wang et al., 2020a; Liang et al., 2021). The dual structure of rock and soil aggravates the risk of soil, water and fertilizer loss and intense nutrient leaching in orchards on these farmland slopes (Zhang et al., 2016; Zhong et al., 2019; Wang et al., 2021). Groundcover is a promising strategy to prevent soil, water, and fertilizer losses in orchard ecosystems worldwide. Compared with conventional clean tillage, groundcover has numerous advantages for nutrient cycling and sustainable development of orchard ecosystems. These advantages include: improved soil physical and chemical properties and nutrient preservation (Liu et al., 2021; de Torres et al., 2021); increased soil organic carbon content and fertility (Zheng et al., 2021; Reis et al., 2024); increased soil microbial diversity to promote soil nutrient cycling (Wang et al., 2020b; Wu et al., 2021); and improved yield and quality of fruit tree products (Tu et al., 2021; Panigrahi et al., 2021). Additionally, groundcover has been shown to significantly reduce soil erosion and nutrient losses in orchards compared to conventional clean tillage (Martinez-Mena et al., 2020; Garcia et al., 2018).
Although in previous studies, efficiency of groundcover management in orchard ecosystems in controlling soil, water and nutrient losses were reported, such results were either not generated in studies on purple soil, and/or not on the degraded sloping farmland systems. For instance, on yellow cinnamon soil slopes of reservoir area of central China, runoff was reduced by 31% and soil loss was reduced by 20% through groundcover (Liu et al., 2012). In an avocado orchard on Calcisol soil of Southeast Spain, groundcover reduced soil loss and runoff by 56% and 62%, respectively (Almagro et al., 2016). Research on red soil citrus orchards of Southeast China indicated that groundcover reduced runoff and soil loss by 89% and 99%, respectively (Mo et al., 2019). Thus, published information indicates that the effect of groundcover on runoff and nutrient losses differs significantly between soil types. In addition, previous studies on the characteristics of nutrient output from sloping land mainly focused on surface flow, but information on nutrient loss through interflow is lacking (Zheng et al., 2017). Additionally, while interflow is predicted to be a significant source of N losses in purple soil, a comprehensive investigation comparing nutrient losses via surface runoff and interflow has yet to be conducted (Han et al., 2010; Song et al., 2017).
The effects of groundcover on orchard tree growth and nutrition are economically and ecologically important but underexplored, especially in citrus orchards on purple soil. For instance, milk vetch cultivation in Southern China paddy fields significantly improved soil quality and rice yield (Zhou et al., 2020), while groundcover on North China’s brown soils enhanced soil conditions and the growth of Juglans regia (walnut) trees (Dong et al., 2021). However, cover crops can also compete with trees for nutrients and water, as observed in a Abies fraseri (Fraser fir) plantation in Michigan, USA, where groundcover reduced leaf N and P content in trees (Nikiema et al., 2012). The effects of groundcover on growth and nutrition in citrus orchards on purple soil of degraded sloping farmland have not been reported. In this context, legumes are particularly interesting as groundcover species. Since symbiosis of legume roots with rhizobia mediates the use of atmospheric N2 by biological nitrogen fixation (BNF) as N source for plant growth and development (Frungillo, 2022), groundcover by legumes may constitute a cost-effective and attractive alternative to fertilization for the improvement of N and P availability in the groundcover-orchard tree-soil system (Hao et al., 2014). Such nursery effects of legumes are of particular significance for the successful establishment and growth of non-N2-fixing tree species in mixed cultivation on N depleted soil (Hu et al., 2017).
In the present study, we conducted a 2-years consecutive field experiment aimed at comparing the effects of different groundcover management (i.e., clean tillage, coverage with Lolium perenne L., Vicia villosa Roth, or Orychophragmus violaceus) on citrus tree nutrition and growth as well as surface runoff and interflow with particular emphasis on soil erosion, water retention as well as N and P losses in citrus orchards on sloping farmland of the purple soil regions in Southwest China. The objectives of this study were to (i) elucidate the effects of different groundcover species on nutrition and growth of citrus trees; (ii) quantify the efficiency of groundcover species in reducing soil erosion, water runoff, N and P losses; and (iii) select a ground cover crop, suitable for maintaining growth and nutrition of citrus trees and maximizing the reduction of runoff, soil and nutrient losses. Based on these objectives, we hypothesized that (i) groundcover improves nutrition and growth of citrus trees; and (ii) reduces soil, water, N and P losses, and the need for fertilizer application; (iii) the losses of N and P in interflow are much higher than those in surface runoff; and (iv) these effects of groundcover management highly depend on the cover crop species selected.
2 Materials and methods
2.1 Background of study area
The present field experiment was conducted in the runoff monitoring field of the National Monitoring Base for Purple Soil Fertility and Fertilizer Efficiency, which is located in Beibei, Chongqing, China (106°26′33″E, 29°48′36″N; altitude 266.3 m) (Figure 1A). This region has a subtropical monsoon climate with an average annual temperature of 18.3°C. The mean annual precipitation is 1115.3 mm, which is distributed unevenly through the year with April to August accounting for more than 80% of the total precipitation. The annual sunshine duration reaches 1276.7 h at an average annual frost-free period of 317 days (Zhang et al., 2021). The study region is dominated by purple soil formed in the purple sandy shale, classified as Entisol according to the USDA soil taxonomy. The physicochemical properties of the top soil layer were: pH 5.6, total N 1.2 g kg-1, total P 0.7 g kg-1, total potassium (K) 22.3 g kg-1, available N 98.4 mg kg-1, available P 55.8 mg kg-1, available K 90.7 mg kg-1, soil bulk density 1.22 g cm-3, and soil porosity 53.96%.
A total of 12 experimental plots were built on February 28, 2018 on a slope (Figures 1B, C). The size of each plot is 12 m (length) × 4 m (width) × 0.6 m (depth), with a slope of 15°, which allows the simultaneous monitoring of surface runoff and interflow (at 60 cm underground). The sidewalls and floors of the compartments were made of reinforced concrete to interrupt the connection of contiguous plots and to avoid hydrological interferences. The soil layers of each plot from top to bottom were 20 cm of surface-cultivated mellow soil, 20 cm of plow pan soil, and 20 cm of clastic purple shale parent materials, respectively, which originated from Ziyuntai, Beibei, Chongqing. The trench for the collection of surface runoff, sediment and interflow was placed at the bottom of each plot, and PVC pipes were used to connect each groove to the surface runoff and interflow collection barrel (Figure 1C).
In each runoff plot, four annual citrus seedlings of the Citrus reticulata ‘Ai Yuan 38’ were transplanted in April 2018 into the central axis of each plot with a plant spacing of 3 m. The variety exhibits a moderate degree of polyembryony, and the seedlings are carefully selected for transplantation from uniformly sized seedlings grown under the same environmental conditions (Figures 1B, C). The fertilization level of each experimental plot was the same and amounted to 273 g N, 151 g P2O5, and 299 g K2O in 2018-2019, and 249 g N, 104 g P2O, and 178 g K2O in 2019-2020. The fertilizer was applied using the trenching method, where a shallow trench was dug around the drip line of the citrus trees, ensuring even nutrient distribution to the root zone. Soil nutrient analysis conducted at the end of the first year showed that residual nutrient levels were sufficient to support the continued growth of citrus trees (Chen et al., 2010). Additionally, as the trees matured, their nutrient requirements decreased compared to the seedling stage in the first year (Zhang et al., 2017). Furthermore, following local agricultural guidelines for citrus cultivation, fertilizer application rates were reduced in subsequent years to prevent excessive fertilization and minimize nutrient runoff. Therefore, the amount of fertilizer used in the second year was lower than in the first year. The selection of fertilization rates was based on local soil nutrient conditions and citrus tree growth stages, following standard agronomic practices. The prevention and control of citrus diseases and insect pests were carried out according to routine practices and the management of each plot was consistent (Lin et al., 2019).
2.2 Experimental design and collection of runoff samples
The field experiment was arranged in a randomized complete block design with four treatments, including: i) clean tillage as control (CK), which represents the habit of fruit farmers for keeping the ground bare by manual weeding or spraying herbicides; ii) coverage with Lolium perenne L. (Lolium), a gramineous grass; iii) coverage with Vicia villosa Roth (Vicia), a leguminous species; iv) coverage with Orychophragmus violaceus (Ory), a cruciferous species. Each treatment was set up in three replicates to ensure statistical validity. The Lolium perenne L., Vicia villosa Roth and Orychophragmus violaceus seeds were sown inside the plots in the middle of September 2018 and 2019 at rates of 22.5, 45.0, and 22.5 kg ha-1, respectively.
Runoff water samples were collected after natural rainfall events from September 2018 to September 2020. The water level in each container were measured using a ruler after each rainfall event to calculate the runoff flux. Subsequently, the runoff samples mixed with sediment were collected in a plastic bottle and dried in an oven to constant weight at 105°C to measure the sediment content (Ma et al., 2016). The product of runoff volume and sediment content was used to calculate the soil loss for each rainfall event. If soil was deposited in the confluence ditch, it was included in each treatment separately (Ma et al., 2016; Dai et al., 2018). Another runoff sample was collected in a 500 ml plastic bottle, immediately taken to the laboratory, and stored at 4°C for further chemical analyses. The main measurement parameters were various forms of nitrogen, i.e. total nitrogen (TN), soluble total nitrogen (DN), nitrate-nitrogen (NO3–N), ammonium nitrogen (NH4+-N), and various forms of phosphorus, i.e. total phosphorus (TP), soluble total phosphorus (DP), phosphate (PO4+-P). A 200 mL unfiltered aliquot was used to analyze the TN and TP content in each runoff sample, using alkaline potassium persulfate oxidation UV spectrophotometry and ammonium molybdate spectrophotometric, respectively (Ma et al., 2016; Xiong et al., 2022). The other runoff samples were filtered through a 0.45 μm membrane to analyze the concentrations of DN, NO3–N, NH4+-N, DP, and PO4+-P by a flow auto analyzer AA3 (Bran and Lubbe, Norderstedt, Germany) (Tian et al., 2017). The following formulas were used to determine particulate nitrogen (PN) and particulate phosphorus (PP): PN = TN - DN and PP= TP - DP (Li et al., 2017). Ground coverage by cover crops was quantified by photographing the cover crops at regular intervals, followed by digital image analysis to assess the extent of coverage (Bousselot et al., 2010). Meteorological data from 2018 to 2020 were acquired from the meteorological station of the National Monitoring Base for Purple Soil Fertility and Fertilizer Efficiency, approximately 50 m from the experimental site.
For each rainfall event, nutrient losses were calculated by multiplication of the runoff volume and nutrient content. Total annual nutrient losses were computed by the addition of these rainfall event values. The fluxes of loss of a certain form of N or P in a single rainfall runoff event were calculated as follows:
The annual flux of N or P loss (Q) under the rainfall events was calculated as follows (Zhang et al., 2016):
Where Qi (g ha-1) represents the flux of loss of a certain form of N or P in surface runoff or interflow in the i-th rainfall, Ci (mg L−1) represents the contents of various forms of N and P in surface runoff and interflow water, qi (L) represents the surface runoff and interflow volume in a single event, S (m2) represents the area of the runoff plot, 10 represents the unit conversion factor (i = 1 to n, the number of runoff flow events during the year).
2.3 Determination of citrus growth parameters and collection of plant samples
Citrus tree height, stem diameter (near the ground surface) and crown width were recorded with a measuring tape and a vernier caliper on September 2018 (Prior to commencement of monitoring), 2019 (at the end of one year of monitoring) and 2020 (at the end of two years of monitoring). Changes in citrus tree parameters were obtained by subtraction. Mature leaves of spring shoots of citrus trees were collected from four directions (east, west, south, and north) in mid-May 2019 and 2020. The leaf dry weight was measured by heat treatment of leaves at 105°C for 15 minutes to inactivate the enzyme and then drying at 65°C for 72 h. The dried leaves were ground into powder by a grinder (JX-CL, Jingxin Ltd., Shanghai, China). Aliquots of 1.5 mg to 2.0 mg of dried powdered plant material were weighted into tin capsules combusted in an elemental analyzer (Flash EA, Thermo Fisher Scientific, Massachusetts, USA) connected to an isotope ratio mass spectrometer (253plus, Thermo Fisher Scientific, Massachusetts, USA) through a Conflo IV multi-purpose interface for C, N, and stable isotope natural abundance analysis, according the method described by Simon et al. (2011). Total P was determined by the molybdenum blue test with a spectrophotometer (UV-1800, AOE Instruments, Shanghai, China) as previously described (Netzer et al., 2017).
2.4 Collection of soil samples
In September 2019 and 2020, mixed soil samples (0-20 cm) from five spots in each plot were obtained using an auger after the removal of impurities on the surface. The collected soil samples were air-dried, ground, and then partly passed through 2.0 mm sieve and partly through a 0.25 mm sieve. The sieved and dried soil samples were analyzed for total N (TN), total phosphorus (TP) and organic matter (OM) contents as previously described (Cheng et al., 2019; Lu, 2000). Soil available N and available P were determined according to Olsen et al. (1982).
2.5 Statistical analysis
The raw data were first tested for normal distribution by Shapiro-Wilk tests. Where necessary, data were transformed using either log- or square-root transformation to satisfy the assumptions of normality and variance. One-way analysis of variance (ANOVA) followed by Duncan post hoc tests were employed to test the significances of difference between leaf nutrition, citrus growth and runoff, soil, and nutrient losses under the experimental treatments. All statistical tests were performed using with the SPSS 17.0 software (SPSS Inc., Chicago, USA). Differences were considered significant at P< 0.05. All the figures were generation of OriginPro 2016 (OriginLab Inc., Northampton, USA) and GraphPad Prism 6.0 (San Diego, CA, USA).
3 Results
3.1 Effectiveness of groundcover on nutrition and growth of citrus trees
Groundcover species mediated annual nursing effects on foliar nutrient contents of citrus trees (Figures 2A, B). In addition, different groundcovers significantly enhanced citrus tree growth and improve leaf nutrient (Figure 2B, Table 1). Specifically, significant positive effects on height, stem diameter and crown width of citrus trees were observed with the Vicia covering treatment within the first year of observation, but no significant effects on the number of mature leaves of spring shoots. After two consecutive years of groundcover management, Lolium and Vicia both significantly improved the growth of citrus trees, with increased height, stem diameter, and crown width by +19.92, +32.51, and +19.41%, (east-west direction by14.88% and north-south direction by 23.94%), and 37.20, 41.50, and 12.35% (east-west direction by17.74% and north-south direction by 6.95%), respectively compared to the CK treatment. The number of mature leaves of spring shoots was decreased, whereas total nitrogen (N), phosphorus (P) and carbon (C) contents of mature citrus leaves on spring shoots were increased at Lolium and Vicia ground cover. In addition, the total N and P contents of citrus spring leaves in 2020 were significantly higher than those in 2019, while the C/N and C/P ratios were reduced. In addition, groundcover significantly reduced the δ15N natural abundance levels in mature leaves of citrus spring shoots compared to CK. In 2020 the foliar δ15N natural abundance was more negative than in 2019 (Figure 2B). Groundcover had no significant effects on δ13C natural abundance of citrus leaves (Figure 2B).
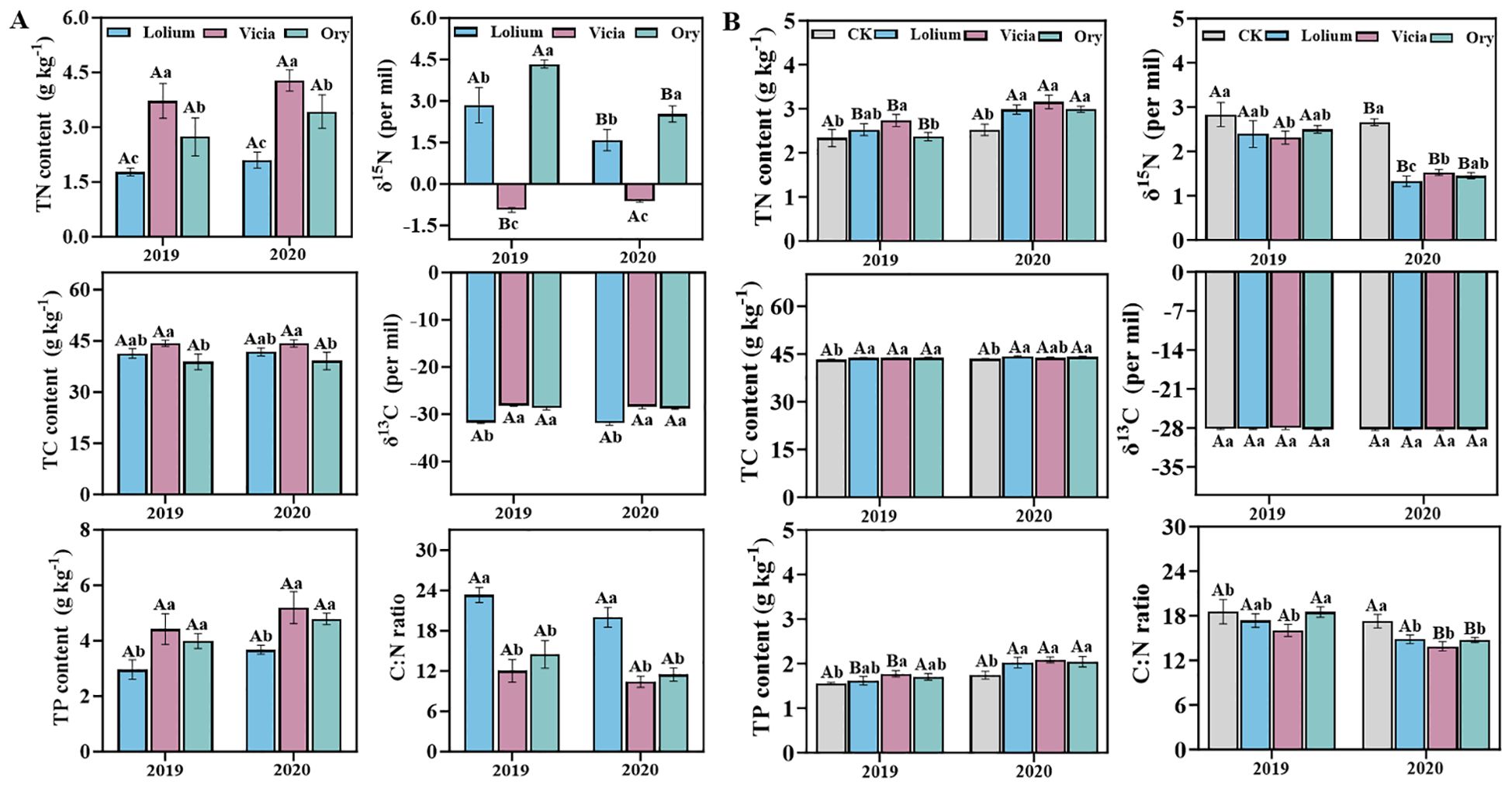
Figure 2. Total nitrogen content (TN), total carbon content (TC), total phosphorus content (TP) as well as C and N stable isotope signatures in (A) different groundcover species, and (B) the mature leaves of spring shoots of citrus trees as affected by different groundcover species. CK, clean tillage as control; Lolium, coverage with Lolium perenne L.; Vicia, coverage with Vicia villosa Roth Ory, coverage with Orychophragmus violaceus. Different lower-case letters (a-c) indicate significant differences between CK and groundcover management of the same experimental period. Different capital letters (A, B) indicate significant differences between the same treatment in different experimental periods.
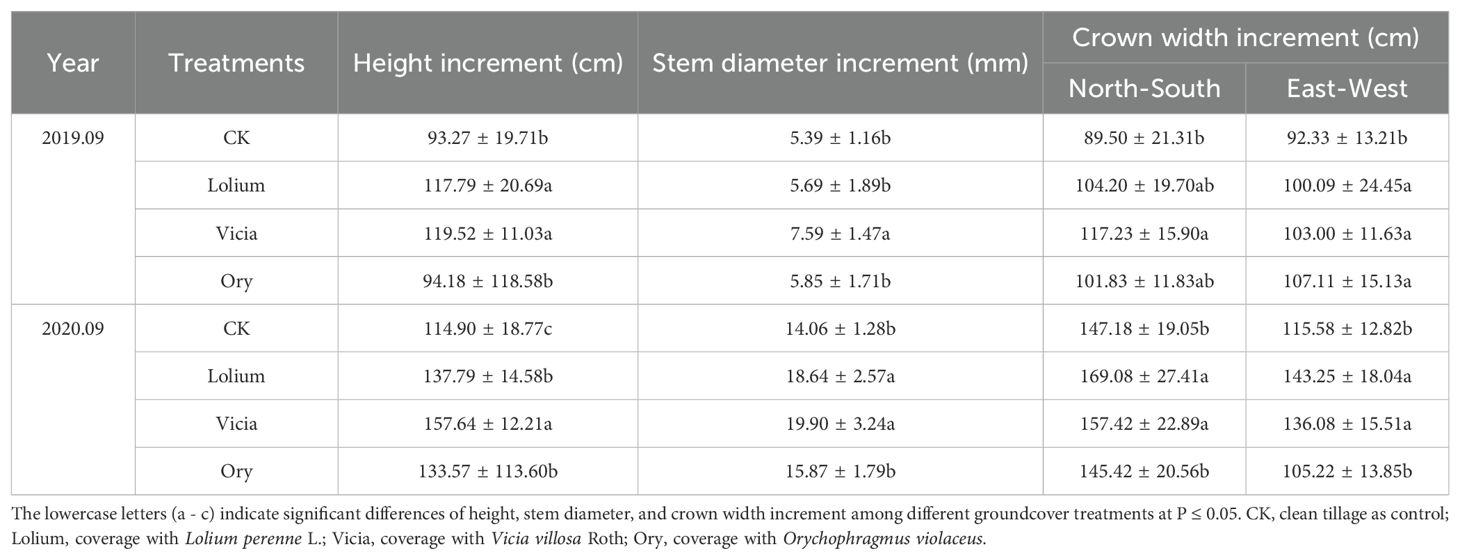
Table 1. Height, stem diameter and crown width increment of citrus tree as affected by different groundcover management.
3.2 Effectiveness of different groundcover species on soil nutrient contents
The present results showed that groundcover had no obvious effects on soil N and P nutrient contents after the first year (at the end of 2019), but at the end of the second year (2020), all groundcover treatments significantly increased total N (TN), total P (TP), available N (AN), available P (AP), and organic matter (OM) contents of the surface soil of the citrus orchard compared to CK by +26.35-51.10%, +22.56-32.36%, +21.57-54.72%, +9.26-28.68% and +10.77-18.57%, respectively (Table 2). In particularly, TN and AN contents of surface soil in the Vicia treatment were significantly higher than in the Lolium and Ory treatments (Table 2).
3.3 Rainfall distribution and vegetation cover change
During the study period from September 2018 to September 2020, rainfall at the experimental site was mainly concentrated from May to July (Figure 3A). The total rainfall of the experimental plots between September 2018 and October 2019 was 980.66 mm and 75.05% of it was noted in May to July. The rainfall in May was highest, amounting to 276.90 mm and representing 28.25% of the total annual precipitation (Figure 3A). The total rainfall between September 2019 and September 2020 was 1,084.00 mm and 69.74% of it was noted in June to July. During this period, rainfall in July was highest, amounting to 451.00 mm and representing 41.61% of the total annual precipitation (Figure 3A). Cover crop type is a crucial factor controlling runoff and soil loss. During the study period, Lolium and Vicia had greater coverage on the ground, with Vicia reaching 60-100% coverage from November to March, while from April to July, Lolium had approached 95% coverage, greater than Vicia (Figure 3B). Ory came into full flowering in March with 50% ground cover, but from April to October with less than 10% ground cover (Figure 3B).
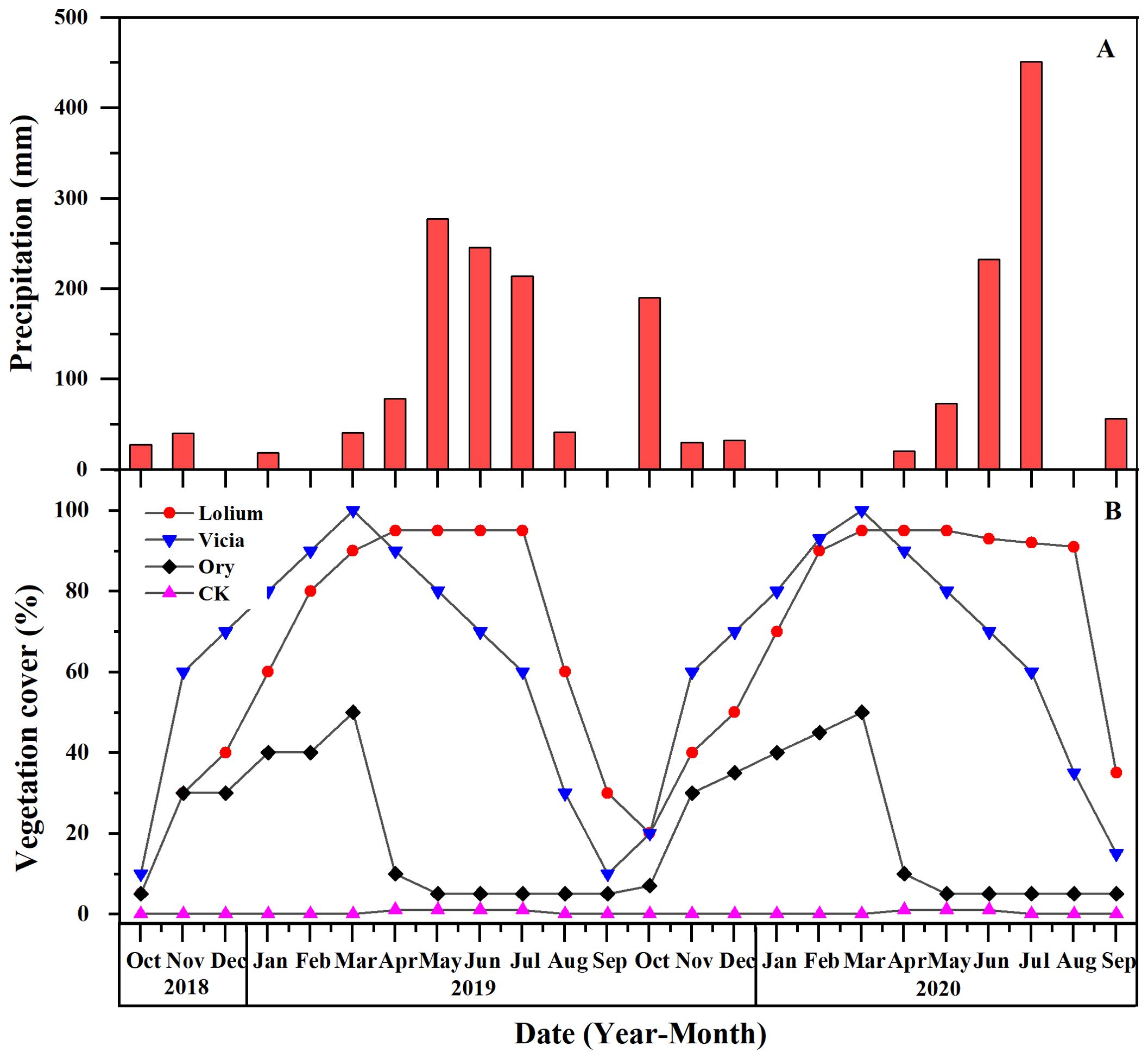
Figure 3. Monthly precipitation (A) and vegetation cover (B) of the soil during the monitoring period.
3.4 Surface runoff, interflow, and soil loss in the citrus orchard
Surface runoff, and interflow were collected to compare impacts of groundcover on these parameters. A total of 40 natural rainfall events generating surface runoff and 39 natural rainfall events producing interflow were measured during the period of the study (Figure 4). The amount of surface runoff and interflow of different treatments increased with precipitation. In the early stage of the experiment (September to October), there was no significant difference in surface runoff and interflow among the treatments. Since November, surface runoff from the groundcover treatments (especially Lolium and Vicia treatments) was smaller than that from the CK treatment. From April to July, the Lolium treatment had a better interception effect on the surface runoff, the interception effect was best in June and amounted to 25.41% - 37.69% less than in the CK treatment. The volumes of surface runoff collected from the Ory treatment were similar to the CK treatment. The interflow volume from the Lolium treatment was considerably smaller from April to May, but close to or greater than the CK treatment from June to July (Figure 4). Surface runoff and interflow peaked on July 15, 2020. Compared with the CK treatment, the surface runoff of Lolium, Vicia, and Ory treatments decreased by 25.51%, 14.61% and 10.18%, respectively, while the interflow increased by -0.29%, -0.34% and -0.31%, respectively (Figure 4).
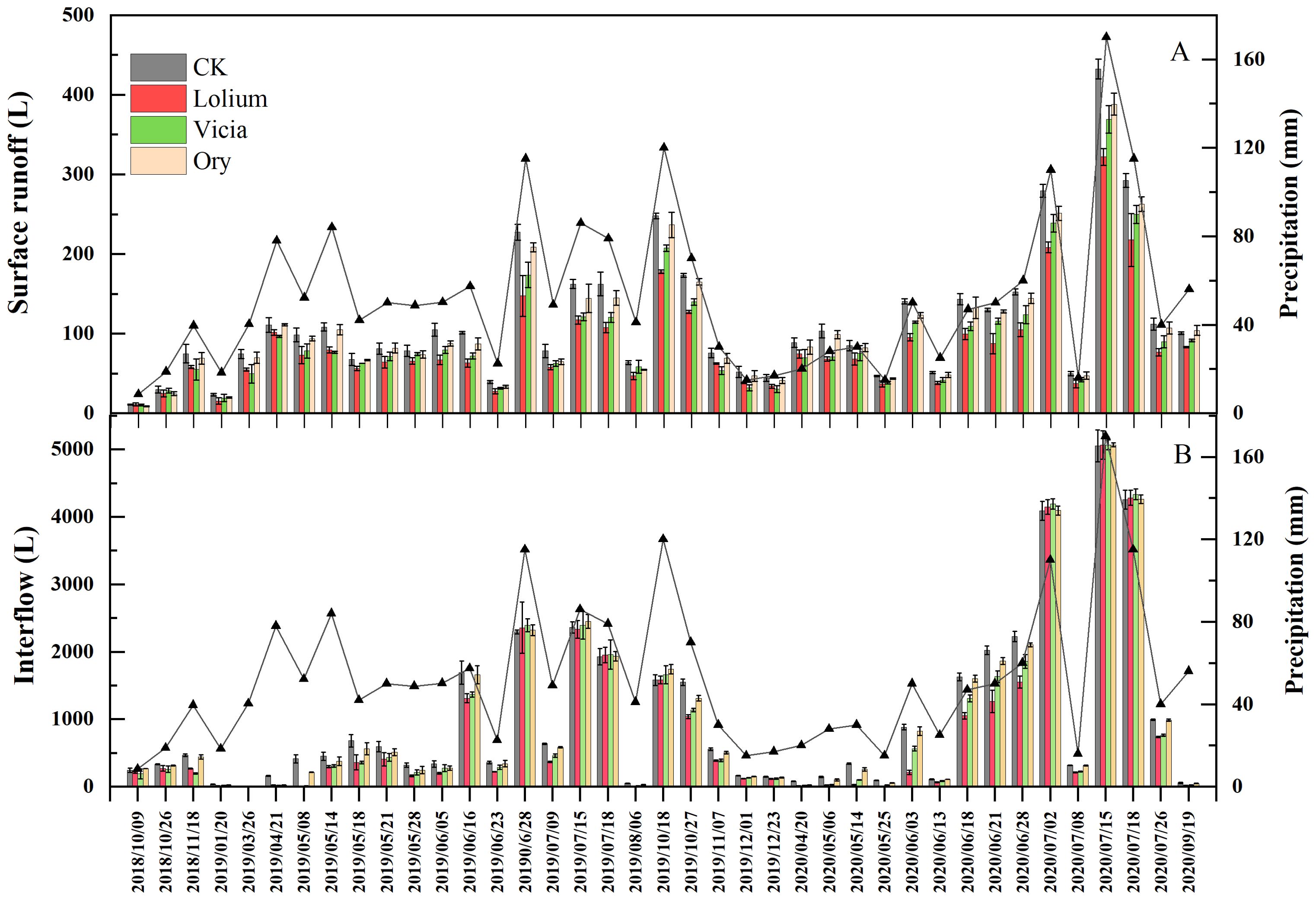
Figure 4. Dynamic changes of surface runoff (A) and interflow (B) with different groundcover management under rainfall events. Note: CK, clean tillage as control; Lolium, coverage with Lolium perenne L.; Vicia, coverage with Vicia villosa Roth; Ory, coverage with Orychophragmus viola.
The runoff and soil losses from orchards with groundcover were significantly smaller compared to the CK treatment (Table 3). The overall effect on the amount of surface runoff, interflow, and soil loss was Lolium > Vicia > Ory. The Lolium and Vicia plots showed a reduction of 27.17% and 18.94% in surface runoff, 18.50% and 13.60% in interflow, and 58.34% and 47.65% in soil loss (Table 3). In addition, the interflow was much higher than the surface runoff under the different treatments, and the average annual interflow amounted to 89.55% to 90.60% of the total annual runoff (Table 3).
3.5 Nitrogen losses by surface runoff and interflow
For the annual N loss dynamics, most of the results showed that the Lolium treatment reduced N losses more than the other groundcover treatments, followed by the Vicia treatment, whereas the effect of Ory treatment was minute (Figure 5). The loss of N in surface runoff was highest from April to June, while the loss of N in interflow was the highest from May to July (Figure 5).
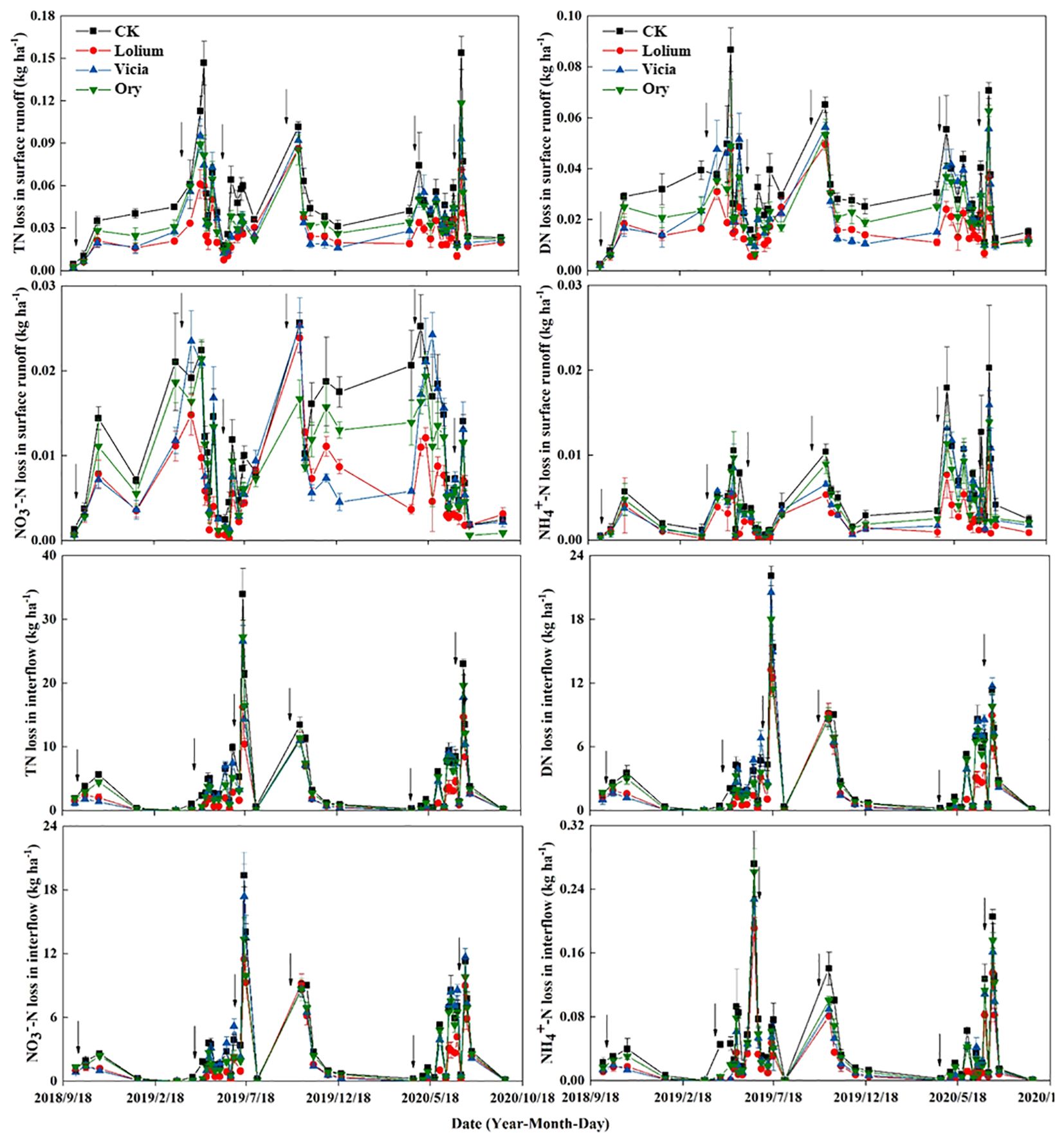
Figure 5. Dynamic changes of N loss in surface runoff and interflow with different groundcover management under rainfall events. CK, clean tillage as control; Lolium, coverage with Lolium perenne L.; Vicia, coverage with Vicia villosa Roth Ory, coverage with Orychophragmus violaceus. The vertical arrow indicates the fertilization time.
The different groundcover treatments significantly reduced the annual loss of various forms of N in surface runoff and interflow compared to CK (Table 4). N losses via interflow were higher than those via surface runoff, averaging to 59.53-103.48 kg ha-1 per year, with significant differences in total N losses between groundcover treatments. In the Lolium treatment, surface runoff of TN, DN, NO3–N, NH4+-N and PN was reduced by 50.63%, 46.99%, 49.51%, 48.09%, and 56.18%, respectively, whereas the interflow was reduced by 50.51%, 46.40%, 50.50%, 50.93%, and 62.54%, respectively (Table 4). Furthermore, different groundcover treatments significantly reduced N fertilizer loss (Table 4). Compared with the CK treatment, Lolium, Vicia, and Ory treatment reduced the annual average N fertilizer loss by 42.74%, 14.10%, and 19.60%, respectively. There was no significant difference between the Vicia and Ory treatments. Overall, the Lolium treatment was most productive in decreasing the amount of N in surface runoff and interflow (Table 4).
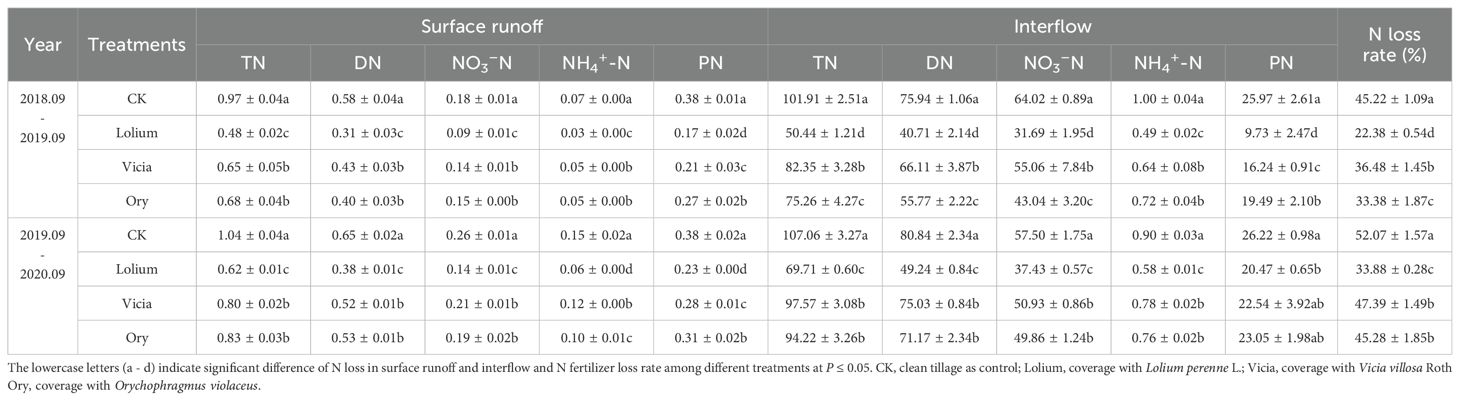
Table 4. Forms of N loss (kg ha-1) in surface runoff and interflow and N fertilizer loss rate from different groundcover management.
N was lost mainly in the form of DN (Supplementary Table S1). In the surface runoff, DN accounted for 59.69% - 66.99% of TN, and PN accounted for 33.01% - 40.31% of TN. In DN, NO3–N was the main component, accounting for 19.13% - 25.65% of TN (Supplementary Table S1). The DN in the interflow accounted for 66.02% - 80.74% of TN, and NO3–N accounted for 52.24% - 66.62% of TN. The Lolium and Vicia treatments significantly increased the loss of DN relative to PN. The Ory treatment did not significantly change the form of N lost in surface runoff and interflow (Supplementary Table S1).
3.6 Phosphorus losses in surface runoff and interflow
The interception effects of different treatments on the annual P loss in surface runoff and interflow differed between seasons (Figure 6). From October to March, most of the interception effects were in the order: Vicia > Lolium > Ory >CK. But from April to July, most of these effects were in the order: Lolium > Vicia >Ory >CK. At the initial stage of the experiment (October 2018), there was no significant difference in P loss among different treatments. In July, P loss was greater than that in other months (Figure 6). The losses of TP, DP, and PO4+-P in surface runoff and interflow were lowest under the Lolium treatment (Figure 6). The amount of TP, DP, and PO4+-P losses in surface runoff were 76.55, 29.87, and 12.34 g ha-1 for the Lolium treatment, respectively, with a reduction of 68.88%, 59.04%, and 53.60%, respectively, compared with CK (Figure 6). The amount of TP, DP, and PO4+-P losses in interflow was 1.20, 0.50, and 0.22 kg ha-1 for the Lolium treatment, respectively, with a reduction of 45.98%, 30.66%, and 37.00%, respectively, compared with CK treatment. Peak losses occurred from June to August (Figure 6).
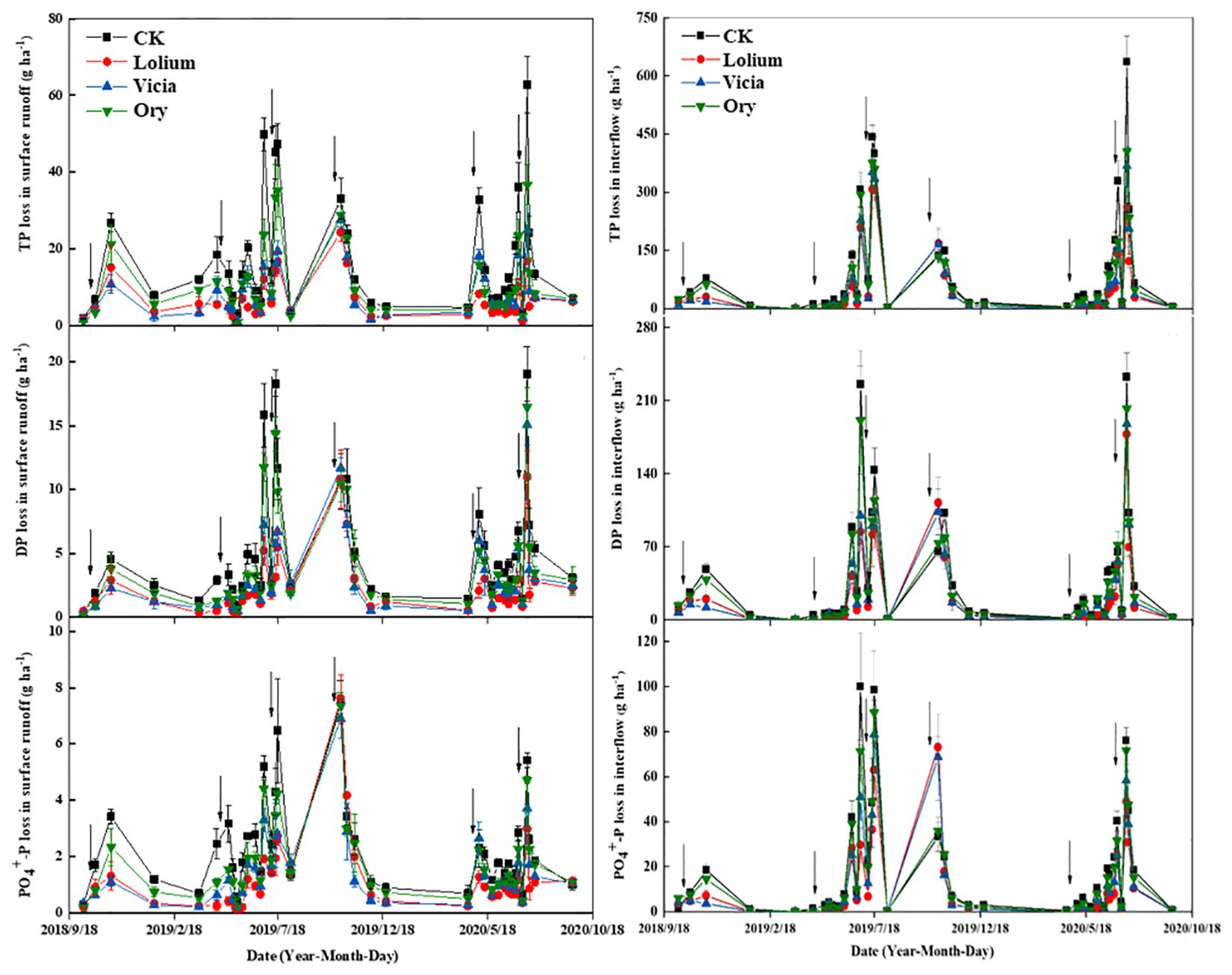
Figure 6. Dynamic changes of P loss in surface runoff and interflow with different groundcover management under rainfall events. CK, clean tillage as control; Lolium, coverage with Lolium perenne L.; Vicia, coverage with Vicia villosa Roth Ory, coverage with Orychophragmus violaceus. The vertical arrow indicates the fertilization time.
Compared with the CK treatment, different groundcover treatments significantly reduced the loss of P in surface runoff and interflow (Table 5). The effects of the Lolium treatment on TP, DP, PO4+-P and PP in surface runoff and interflow were most significant (Table 5). The surface runoff of TP, DP, PO4+-P and PP was reduced by 61.52%, 55.03%, 48.16%, and 64.43%, respectively. The interflow of TP, DP, PO4+-P and PP was reduced by 46.09%, 41.54%, 39.35 and 48.16%, respectively (Table 5). Furthermore, different groundcover treatments significantly reduced the P fertilizer loss. Compared with the CK treatment, Lolium, Vicia, and Ory treatments reduced the annual average P fertilizer loss by 48.46%, 37.89%, and 23.42%, respectively (Table 5).
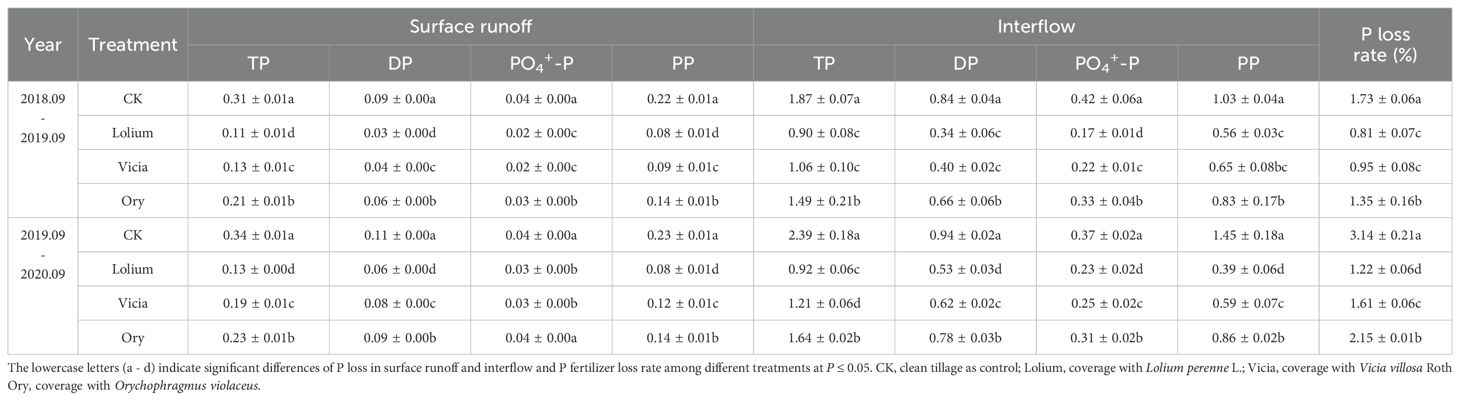
Table 5. Annual P loss (kg ha-1) in surface runoff and interflow and P fertilizer loss rate from groundcover management.
The loss of P was mainly in the form of PP (Supplementary Table S2). In surface runoff, PP accounted for 57.63% - 71.77% of TP, and DP accounted for 28.23% - 42.37% of TP, while PO4+-P only accounted for 12.66% - 21.10% of P loss. The PP in interflow accounted for 43.06% - 63.07% of P loss by TP (Supplementary Table S2). The proportion of PP loss in interflow was less than that in surface runoff (Supplementary Table S2). Groundcover significantly increased the contribution of DP and decreased the contribution of PP to P loss in the second year of the study (September 2019 - September 2020) (Supplementary Table S2).
4 Discussion
4.1 Groundcover improves nutrition and growth of citrus trees
The present study shows that groundcover can greatly improve soil nutrient contents and growth of citrus trees in orchards and can enhance the foliar nutrient content of citrus trees (Figure 2, Tables 1, 2). This result is consistent with our hypothesis (i) and previous reports showing that groundcover can improve soil properties (Zhou et al., 2020; Tu et al., 2021). However, information on the consequences of groundcover for growth and nutrition of citrus trees has so far not been provided. After two consecutive years of groundcover, the legume species (Vicia villosa Roth) showed greater effectiveness than the non-legume species (Lolium perenne and Orychophragmus violaceus) in improving soil properties and foliar nutrient contents of citrus trees. This may be due to biological N2 fixation (BNF) by the legumes, which in turn promoted the growth of citrus trees. Also in previous studies on other species it has been reported that BNF by co-cultivated legumes can promote nutrient availability and growth of non-N2-fixing species, especially when soil N availability is limited. Co-cultivation N2-fixing Robinia pseudoacacia and non-N2-fixing Juglans regia in a mixed stand significantly improved soil nutrient availability and in turn increased N and P content as well as root biomass in non-N2-fixing Juglans regia (Hu et al., 2017; Du et al., 2019). Similarly, Sayyad et al. (2006) also reported a significant increase in foliar N concentration for a non-N2-fixing Populus deltoides in mixed cultivation with N2-fixing Alnus subcordata. δ15N abundance is closely correlated to BNF, with lower δ15N abundances in N2-fixing than in non-N2-fixing plants (Hu et al., 2017). In this study the δ15N abundance was more negative in the legume crop (Vicia villosa Roth) compared to the non-legume cover crops investigated (Lolium perenne L. and Orychophragmus violaceus), which coincided with enhanced total N content in both, the legume crop tissues and citrus tree leaves (Figure 2). These results indicate that N2 fixed by Vicia villosa Roth contributed to the N nutrition the citrus trees. This conclusion is supported by the positive correlation of soil and foliar δ15N, indicating that increased soil N and enhanced N nutrition of the citrus trees. However, Nikiema et al. (2012) showed that in a newly established Abies fraseri (Fraser fir) plantation cover crops competed with the major tree species for nutrients and water. As a consequence, the N and P contents of Fir leaves were reduced after 2 years of cover management. This difference to the present field study can be attributed to differences in management, climate or water availability (Cherr et al., 2006). Apparently, efficient nutrient uptake by the fir trees was prevented by dry weather conditions in the study by Nikiema et al. (2012).
4.2 Effects of groundcover on surface runoff, interflow, and soil loss
Our results indicate that groundcover on purple soil land slopes can significantly reduce surface runoff and soil loss (Table 3). This result is consistent with our hypothesis (ii) and previous results of Liu et al. (2012) obtained in a citrus orchard on yellow cinnamon soil in a reservoir area of central China. In agreement with these findings, also previous reports on other soil types and/or production systems indicated that the application of groundcover management in the inter rows is one of the most effective soil management practice to prevent soil erosion in sloping orchards (Morvan et al., 2014; Ferreira et al., 2018; Bagagiolo et al., 2018), thereby improving also other ecosystem services, such as rainwater interception and absorption of runoff energy by the soil surface (Montanaro et al., 2017; Feng et al., 2018; Winter et al., 2018). In addition, Almagro et al. (2016) reported that groundcover reduced runoff and soil loss by 62.3% and 56.3%, respectively, in an avocado orchard on Calcisol soil on a farmland slope of southeastern Spain while research of citrus orchards in a red soil region of southern China, revealed 89.4% and 99.7% reduction in runoff and soil loss (Mo et al., 2019). Such differences between different field studies may be associated with local climate conditions, soil properties, landscape structure (plain and sloping farmland with different inclination and slopes), and growth of different groundcover species (Deng et al., 2019a; Martinez-Mena et al., 2020). The selection of crop species in the present experiment was based on the previous study by Yang (2020) showing the potential of Lolium perenne L. (Lolium), Vicia villosa Roth (Vicia) and Orychophragmus violaceus (Ory) in citrus orchards for runoff and nutrient interception. As expected by our hypothesis (iv), the variations between groundcover systems in the present study were closely related to growth, development, and the degree of coverage of the crop species applied. Compared to Lolium and Vicia the Ory treatment had a poorer interception effect on surface runoff and soil loss (Figure 4, Table 3). This was probably due to the long surface coverage period, higher amounts of surface coverage and growth conditions of Lolium that reduced runoff and soil erosion. Additionally, Vicia began to decompose in April, and the surface coverage decreased to about 60% in July, whilst Ory had higher requirements for soil fertility and had the lowest surface coverage of about 40% to 50% in March, and less than 10% after April among three crop species studied (Figure 3B). Other investigations also highlight that more vegetation coverage can intercept higher amounts of runoff and enhance the infiltration of rainwater into the ground, effectively reduce the splashing of rain on the soil surface, and that parts of the sediment particles carried by the runoff could be intercepted by grass stems, thus restricting soil erosion (Deng et al., 2019b; Zhu et al., 2021; Liu et al., 2021). The results of Wang et al. (2019a) also demonstrated that the energy of rainfall was influenced by differences in vegetation coverage, which was the main factor affecting soil loss. In this context, compared to Vicia, Lolium was a more effective buffer at the soil surface due to its higher density of stems and larger biomass at enhanced surface coverage (Figure 3), thereby decreasing the speed and energy of surface runoff and further reducing soil erosion.
The present results indicated that interflow was one of the main pathways of runoff from purple soil and that the annual interflow accounts for 89.6% - 90.6% of total runoff (Figure 4, Table 3), in line with our hypothesis (iii) and Hua et al. (2014). It can be predicted that due to the dual geological structure of purple soil with surface soil and underlying rocks, affluent soil pores, intense penetration, and inefficient water holding capability may cause severe interflow losses under intensive rainfall and high temperatures (Qian et al., 2020; Khan et al., 2016), especially on farmland slopes (Xie et al., 2018). Our results show that on the early plant developmental stage, effects of different groundcover systems on surface runoff and interflow were similar to the control (Figure 4). However, with increasing time of groundcover cultivation, amounts of surface runoff and interflow changed oppositely (Figure 4). This is assumed to be due to the small amount and low intensity of rainfall in the early stage of plant development (September to April of the next year). Under this condition, the aboveground part of the vegetation could effectively intercept rainfall and reduce surface runoff (Liu et al., 2021). Previous studies have reported the root systems had a good winding and consolidation effect on the soil, by increasing soil porosity, reducing soil bulk density and, hence, enhancing the stability of soil aggregates and soil cohesion (Duan et al., 2020; Liu et al., 2021). This way, the ability of water retention and soil consolidation was enhanced, ultimately mediating high soil and water retention and reducing both, surface runoff and interflow. On the other hand, June to July is the annual rainy season in the local area with high amounts and intensity of rainfall. The highest individual rainfall observed was 170 mm, which led to soil saturation and increased interflow. Although the vegetation coverage significantly reduced the surface runoff, the interflow was increased due to the large root systems and cracks were easily generated in the soil to cause more interflow. Previous studies reported that rainwater produces surface runoff when its intensity exceeds the intensity of soil infiltration that is changed by the vegetation coverage (Huang et al., 2013). The stems and leaves of vegetation can intercept rainfall, while the roots form irregular soil voids, thereby increasing soil permeability (Zhang et al., 2020; Liu et al., 2021).
4.3 Groundcover reduces N and P losses in runoff and interflow
Nitrogen (N) and phosphorus (P) can move from soil to water by dissolving in surface runoff or interflow, thereby inducing non-point source pollution to the surrounding area (Cameron et al., 2013; Zhang et al., 2020). Groundcover could control N and P losses not only by minimizing the destruction of soil structure caused by raindrops and decreasing the surface runoff velocity (Yagioka et al., 2015; Keesstra et al., 2019), but also by efficient nutrient uptake by the root system of the cover crops (Solangi et al., 2019; Tran et al., 2021). Previous studies in grassland and farmland slopes established in other soil types reported that groundcover improved soil porosity, accelerates the formation of macro-aggregates (Villarreal et al., 2021), and decreased the risk of non-point source pollution (Griffith et al., 2020). The present results indicated that different groundcover in citrus orchards on farmland slopes on purple soil could significantly reduce N and P losses in the surface runoff and interflow, controlled fertilizer losses, and further improved soil fertility to promote the growth of young citrus trees as hypothesized (hypothesis i, ii) (Tables 1, 2, 4, 5). Groundcover improves nutrient use efficiency by root uptake and enhances soil retention (Liu et al., 2021). In addition, cover crops could also add organic compounds to the soil by root exudation that supports soil decomposition and mineralization processes (Zhang et al., 2020; Maurer et al., 2021). In agreement with our hypothesis (iv), the capacity of cover plants to decrease nutrient losses is highly dependent on the vegetation species selected. Regarding runoff, N and P losses, our results showed that the Lolium has the most positive effect, followed by Vicia (Tables 4, 5). This outcome may be due to the fact that leguminous (Vicia) could fix atmospheric N2 and thus induce a net gain of soil N (Xie et al., 2016). This conclusion is supported by the data on δ15N in plants and total N in soil in the present study. Apparently, in the present study, the legume plant (Vicia) had a more negative δ15N signal compared to the graminaceous plant (Lolium) and the cruciferous plant (Ory). In addition, the low C/N ratio of degrading plant material may result in rapid N decomposition, whereas the gramineous Lolium with a relative high C/N rate of degrading plant material may result in low N mineralization and slow N release to the soil (Zhou et al., 2020, Zhou et al., 2019). This partially explains a greater reduction of N and P losses with the gramineous Lolium from May to June. Although Vicia increased the loss of N and P during the decomposition period (from April to June) compared to the graminaceous plant, the total annual loss of N and P in the runoff was significantly lower than that of the clean tillage control treatment, because dead branches and residues of Vicia still seemed to effectively control runoff. Overall, Lolium is a beneficial cropping system of the present study to reduce soil erosion in citrus orchards on sloping land of purple soil. However, in terms of long-term orchard sustainable nutrient management systems, the legume Vicia has wider potential.
Our results indicated that different vegetation cover crops did not show preferable forms of N and P losses through runoff and interflow (Supplementary Tables S1, S2). Overall, total soluble nitrogen (DN) was the dominant form of N lost in surface runoff and interflow for all investigated cover crop species. Because nitrate-nitrogen (NO3–N) is the dominant form of DN, the loss of NO3–N was much higher than the loss of ammonium nitrogen (NH4+-N) (Supplementary Table S1). This finding is consistent with previous research on the effect of long-term fertilizer management on nutrient loss from purple soil slopes in Southwest China by Du et al. (2021) and on the mechanism of N and P losses from plain farmland (soil was mostly formed by alluvial flood, including tidal soil, meadow cinnamon soil, and calcareous cinnamon soil) in the North China Plain by Liu et al. (2014). Apparently, NH4+-N is readily absorbed by negatively charged particulates in the soil, whereas NO3–N is mostly present in soil solution and easily lost with runoff at heavy precipitation (Chen et al., 2012; Baptista et al., 2015). In addition, in the rhizosphere of citrus trees, microbial activities such as nitrification will rapidly transform reduced forms of N in the fertilizer into NO3–N that is easily absorbed and leached to lower layers of the soil profile (Chen et al., 2019).
In the present study, soluble P losses amounted to 28% - 57% of the total phosphorus (TP) losses (Supplementary Table S2), indicating that particulate P was the dominant form of P loss from the soil. This is due to the fact that phosphate has a high affinity to soil minerals and is readily enclosed and immobilized by soil particles (Wang et al., 2019b; Du et al., 2021). In addition, the amount of N and P losses in interflow accounts for a considerable share of surface runoff in steep slopes [Han et al., 2010; Tan and Zhang, 2011, hypothesis (ii)]. Therefore, for purple soil developed on farmland slopes, controlling runoff is an important means to reduce N and P losses, with the generation of interflow being particularly important to control nutrition losses.
5 Conclusion
The present study quantified the water, soil and nutrients retention, in particularly through both surface runoff and interflow pathways of soil by groundcover management on sloping farmland systems in purple soil regions. The presence of groundcover significantly reduced surface runoff, interflow, soil erosion as well as N and P losses compared to clean tillage. In addition, the enhanced growth and improved leaf N and P nutrient contents of citrus tree were observed with the groundcover systems. However, the effectiveness of such effects highly depended on the species of groundcover. The Lolium treatment was more effective in controlling soil and water losses and reducing N, P losses from both runoff and interflow than other treatments. Compared with clean tillage, ground coverage with Lolium can reduce soil, N and P losses by 216.46 kg ha-1, 44.87 kg ha-1 and 1.11 kg ha-1, respectively. From these results, it is estimated that the annual reduction potentials of soil, N and P losses that could be obtained by groundcover amounts to 16.3 million tons soil yr-1, 3.44 million tons N yr-1and 8.5 million tons P yr-1, respectively on average based on the areas of purple soil slope farmland in Southwestern China. For such reductions, Lolium constitutes the more beneficial coverage system to reduce soil, water, and nutrient losses from surface runoff and interflow in the citrus orchards. However, cultivation of the legume Vicia significantly enhanced soil nutrition status in citrus orchards and promoted leaf nutrients levels, which further promoted the growth of young citrus trees. In terms of a sustainable nutrient management system for long-term orchards, the leguminous Vicia also has the great potential to bring economic and ecological values for rehabilitation of the vulnerable and eroded sloping farmland systems in purple soil regions. Thus, the selection of appropriate groundcover to match the target of improved soil properties and/or facilitative effects on nutrient status of citrus trees is essential for the sustainable development of citrus orchard, particularly on sloping farmland systems. Further effects of groundcover management on the yield and fruit quality of citrus should be considered in future studies.
Data availability statement
The original contributions presented in the study are included in the article/Supplementary Material. Further inquiries can be directed to the corresponding author.
Author contributions
RL: Conceptualization, Formal analysis, Methodology, Visualization, Writing – original draft. YTZ: Formal analysis, Investigation, Supervision, Writing – review & editing. ZW: Formal analysis, Methodology, Writing – original draft. XZ: Formal analysis, Writing – original draft, Conceptualization. WX: Formal analysis, Writing – review & editing. JZ: Writing – review & editing, Methodology. YQZ: Writing – review & editing, Investigation, Supervision. BH: Writing – review & editing. XS: Writing – review & editing, Conceptualization, Formal analysis, Funding acquisition, Project administration, Resources, Validation. HR: Supervision, Validation, Writing – review & editing.
Funding
The author(s) declare financial support was received for the research, authorship, and/or publication of this article. This work was supported by China Agriculture Research System- Green Manure (No. CARS-22-G-13) and funding from the State Cultivation Base of Eco-agriculture for Southwest Mountainous Land of Southwest University.
Conflict of interest
The authors declare that the research was conducted in the absence of any commercial or financial relationships that could be construed as a potential conflict of interest.
Publisher’s note
All claims expressed in this article are solely those of the authors and do not necessarily represent those of their affiliated organizations, or those of the publisher, the editors and the reviewers. Any product that may be evaluated in this article, or claim that may be made by its manufacturer, is not guaranteed or endorsed by the publisher.
Supplementary material
The Supplementary Material for this article can be found online at: https://www.frontiersin.org/articles/10.3389/fpls.2024.1489693/full#supplementary-material
References
Almagro, M., Vente, J., Boix-Fayos, C., García-Franco, N., Aguilar, J. M. D., González, D., et al. (2016). Sustainable land management practices as providers of several ecosystem services under rainfed Mediterranean agroecosystems. Mitigation Adaptation Strategies Global Change. 21, 1029–1043. doi: 10.1007/s11027-013-9535-2
Bagagiolo, G., Biddoccu, M., Rabino, D., Cavallo, E. (2018). Effects of rows arrangement, soil management, and rainfall characteristics on water and soil losses in Italian sloping vineyards. Environ. Res. 166, 690–704. doi: 10.1016/j.envres.2018.06.048
Baptista, I., Ritsema, C., Geissen, V. (2015). Effect of integrated water-nutrient management strategies on soil erosion mediated nutrient loss and crop productivity in Cabo Verde drylands. PLoS One 10, e0134244. doi: 10.1371/journal.pone.0134244
Bousselot, J. M., Klett, J. E., Koski, R. D. (2010). Extensive green roof species evaluations using digital image analysis. HortScience horts 45, 1288–1292. Available at: https://journals.ashs.org/hortsci/view/journals/hortsci/45/8/article-p1288.xml.
Bowles, T. M., Atallah, S. S., Campbell, E. E., Gaudin, A. C. M., Wieder, W. R., Grandy, A. S. (2018). Addressing agricultural nitrogen losses in a changing climate. Nat. Sustainability 1, 399–408. doi: 10.1038/s41893-018-0106-0
Cameron, K. C., Di, H. J., Moir, J. L. (2013). Nitrogen losses from the soil/plant system: a review. Ann. Appl. Biol. 162, 145–173. doi: 10.1111/aab.12014
Chen, F., Lu, S., Wang, X., Zhang, F. (2010). Nutrient uptake and residual effects of nitrogen and potassium fertilization in citrus trees. Soil Sci. Soc. America J. 74, 345–355. doi: 10.2136/sssaj2009.0115
Chen, Y. H., Wang, M. K., Wang, G., Chen, M. H., Luo, D., Li, R. (2012). Nitrogen runoff under simulated rainfall from a sewage-amended lateritic red soil in Fujian, China. Soil Tillage Res. 123, 35–42. doi: 10.1016/j.still.2012.03.007
Chen, J., Xiao, H., Li, Z., Liu, C., Wang, D., Wang, L., et al. (2019). Threshold effects of vegetation coverage on soil erosion control in small watersheds of the red soil hilly region in China. Ecol. Eng. 132, 109–114. doi: 10.1016/j.ecoleng.2019.04.010
Cheng, J. H., Tang, X. Y., Cui, J. F. (2019). Effect of long-term manure slurry application on the occurrence of antibiotic resistance genes in arable purple soil (entisol). Sci. Total Environment. 647, 853–861. doi: 10.1016/j.scitotenv.2018.08.028
Cherr, C., Scholberg, J., McSorley, R. (2006). Green manure approaches to crop production: a synthesis. Agron. J. 98, 302–319. doi: 10.2134/agronj2005.0035
Dai, C., Liu, Y., Wang, T., Li, Z., Zhou, Y. (2018). Exploring optimal measures to reduce soil erosion and nutrient losses in southern China. Agric. Water Manage. 210, 41–48. doi: 10.1016/j.agwat.2018.07.032
Deng, L. Z., Fei, K., Sun, T. Y., Zhang, L. P., Fan, X. J., Ni, L. (2019a). Characteristics of runoff processes and nitrogen loss via surface flow and interflow from weathered granite slopes of Southeast China. J. Mountain Sci. 16, 1048–1064. doi: 10.1007/s11629-018-5253-2
Deng, L., Kim, D. G., Li, M., Huang, C., Liu, Q., Cheng, M., et al. (2019b). Land-use changes driven by 'Grain for Green' program reduced carbon loss induced by soil erosion on the Loess Plateau of China. Global Planetary Change 177, 101–115. doi: 10.1016/j.gloplacha.2019.03.017
de Torres, M., Carbonell-Bojollo, R. M., Moreno-Garcia, M., Ordonez-Fernandez, R., Rodriguez-Lizana, A. (2021). Soil organic matter and nutrient improvement through cover crops in a Mediterranean olive orchard. Soil Tillage Res. 210, 104977. doi: 10.1016/j.still.2021.104977
Dong, N., Hu, G., Zhang, Y., Qi, J., Chen, Y., Hao, Y. (2021). Effects of green-manure and tillage management on soil microbial community composition, nutrients and tree growth in a walnut orchard. Sci. Rep. 11, 16882. doi: 10.1038/s41598-021-96472-8
Du, Y. N., Li, T. Y., He, B. H. (2021). Runoff-related nutrient loss affected by fertilization and cultivation in sloping croplands: An 11-year observation under natural rainfall. Agric. Ecosyst. Environ. 319, 107549. doi: 10.1016/j.agee.2021.107549
Du, B., Pang, J., Hu, B., Allen, D. E., Rennenberg, H. (2019). N2-fixing black locust intercropping improves ecosystem nutrition at the vulnerable semi-arid loess plateau region, China. Sci. Total Environ. 688, 333–245. doi: 10.1016/j.scitotenv.2019.06.245
Duan, J., Liu, Y. J., Yang, J., Tang, C. J., Shi, Z. H. (2020). Role of groundcover management in controlling soil erosion under extreme rainfall in citrus orchards of southern China. J. Hydrology 582, 124290. doi: 10.1016/j.jhydrol.2019.124290
Feng, T., Wei, W., Chen, L., Rodrigo-Comino, J., Die, C., Feng, X., et al. (2018). Assessment of the impact of different vegetation patterns on soil erosion processes on semiarid loess slopes. Earth Surface Processes Landforms 43, 1860–1870. doi: 10.1002/esp.4361
Ferreira, C. S. S., Keizer, J. J., Santos, L. M. B., Serpa, D., Silva, V., Cerqueira, M., et al. (2018). Runoff, sediment and nutrient exports from a Mediterranean vineyard under integrated production: An experiment at plot scale. Agric. Ecosyst. Environ. 256, 184–193. doi: 10.1016/j.agee.2018.01.015
Frungillo, L. (2022). Getting to the root of nodulation: how legumes and rhizobia use nitrate uptake to control symbiosis. Plant Cell 34, 1443–1444. doi: 10.1093/plcell/koac065
Garcia, L., Celette, F., Gary, C., Ripoche, A., Valdes-Gomez, H., Metay, A. (2018). Management of service crops for the provision of ecosystem services in vineyards: A review. Agric. Ecosyst. Environ. 251, 158–170. doi: 10.1016/j.agee.2017.09.030
Griffith, K. E., Young, E. O., Klaiber, L. B., Kramer, S. R. (2020). Winter rye cover crop impacts on runoff water quality in a northern new york (USA) tile-drained maize agroecosystem. Water Air Soil Pollut. 231, 84. doi: 10.1007/s11270-020-4443-z
Han, J., Li, Z., Li, P., Tian, J. L. (2010). Nitrogen and phosphorous concentrations in runoff from a purple soil in an agricultural watershed. Agric. Water Manage. 97, 757–762. doi: 10.1016/j.agwat.2010.01.007
Hao, X., Taghavi, S., Xie, P., Orbach, M. J., Alwathnani, H. A., Rensing, C., et al. (2014). Phytoremediation of heavy and transition metals aided by legume-rhizobia symbiosis. Int. J. Phytoremediation 16, 179–202. doi: 10.1080/15226514.2013.773273
Hu, B., Zhou, M. H., Dannenmann, M., Saiz, G., Simon, J., Bilela, S., et al. (2017). Comparison of nitrogen nutrition and soil carbon status of afforested stands established in degraded soil of the Loess Plateau, China. For. Ecol. Manage. 389, 46–58. doi: 10.1016/j.foreco.2016.12.011
Hua, K., Zhu, B., Wang, X. (2014). Dissolved organic carbon loss fluxes through runoff and sediment on sloping upland of purple soil in the Sichuan Basin. Nutrient Cycling Agroecosystems 98, 125–135. doi: 10.1007/s10705-014-9601-5
Huang, J., Wu, P. T., Zhao, X. N. (2013). Effects of rainfall intensity, underlying surface and slope gradient on soil infiltration under simulated rainfall experiments. Catena 104, 93–102. doi: 10.1016/j.catena.2012.10.013
Keesstra, S. D., Rodrigo-Comino, J., Novara, A., Gimenez-Morera, A., Pulido, M., Di Prima, S., et al. (2019). Straw mulch as a sustainable solution to decrease runoff and erosion in glyphosate-treated clementine plantations in Eastern Spain. Assess. using rainfall simulation experiments. Catena 174, 95–103. doi: 10.1016/j.scitotenv.2016.01.182
Khan, M. N., Gong, Y., Hu, T., Lal, R., Zheng, J., Justine, M. F., et al. (2016). Effect of slope, rainfall intensity and mulch on erosion and infiltration under simulated rain on purple soil of South-western sichuan province, China. Water 8, 528. doi: 10.3390/w8110528
Li, Z. G., Gu, C. M., Zhang, R. H., Ibrahim, M., Zhang, G. S., Wang, L., et al. (2017). The benefic effect induced by biochar on soil erosion and nutrient loss of slopping land under natural rainfall conditions in central China. Agric. Water Manage. 185, 145–150. doi: 10.1016/j.agwat.2017.02.018
Liang, K., He, X., He, B., Guo, X., Li, T. (2021). Runoff-associated nitrogen and phosphorus losses under natural rainfall events in purple soil area: the role of land disturbance and slope length. Water Supply 22, 1995–2007. doi: 10.2166/ws.2021.300
Lin, W. B., Wang, Y. S., Ji, C. Q. (2019). Pollution-free green prevention and control technology of citrus pests and diseases in taishun county. Hans J. Agric. Sci. 9. doi: 10.12677/HJAS.2019.98095
Liu, Y., Tao, Y., Wan, K. Y., Zhang, G. S., Liu, D. B., Xiong, G. Y., et al. (2012). Runoff and nutrient losses in citrus orchards on sloping land subjected to different surface mulching practices in the Danjiangkou Reservoir area of China. Agric. Water Manage. 110, 34–40. doi: 10.1016/j.agwat.2012.03.011
Liu, R., Thomas, B., Shi, X. J., Zhang, X. L., Wang, Z. C., Zhang, Y. T. (2021). Effects of ground cover management on improving water and soil conservation in tree crop systems: A meta-analysis. Catena 199, 105085. doi: 10.1016/j.catena.2020.105085
Liu, R. M., Wang, J. W., Shi, J. H., Chen, Y. X., Sun, C. C., Zhang, P. P., et al. (2014). Runoff characteristics and nutrient loss mechanism from plain farmland under simulated rainfall conditions. Sci. Total Environ. 468-469, 1069–1077. doi: 10.1016/j.scitotenv.2013.09.035
Lu, R. K. (2000). Analytical Methods of Soil Agricultural Chemistry (Beijing: China Agricultural Science and Technology Press).
Ma, X., Li, Y., Li, B., Han, W., Liu, D., Gan, X. (2016). Nitrogen and phosphorus losses by runoff erosion: Field data monitored under natural rainfall in Three Gorges Reservoir Area, China. Catena 147, 797–808. doi: 10.1016/j.catena.2016.09.004
Martinez-Mena, M., Carrillo-Lopez, E., Boix-Fayos, C., Almagro, M., Garcia Franco, N., Diaz-Pereira, E., et al. (2020). Long-term effectiveness of sustainable land management practices to control runoff, soil erosion, and nutrient loss and the role of rainfall intensity in Mediterranean rainfed agroecosystems. Catena 187, 104352. doi: 10.1016/j.catena.2019.104352
Maurer, D., Malique, F., Alfarraj, S., Albasher, G., Horn, M. A., Butterbach-Bahl, K., et al. (2021). Interactive regulation of root exudation and rhizosphere denitrification by plant metabolite content and soil properties. Plant Soil 467, 107–127. doi: 10.1007/s11104-021-05069-7
Mo, M., Liu, Z., Yang, J., Song, Y., Tu, A., Liao, K., et al. (2019). Water and sediment runoff and soil moisture response to grass cover in sloping citrus land, Southern China. Soil Water Res. 14, 10–21. doi: 10.17221/147/2017-swr
Montanaro, G., Xiloyannis, C., Nuzzo, V., Dichio, B. (2017). Orchard management, soil organic carbon and ecosystem services in Mediterranean fruit tree crops. Scientia Hortic. 217, 92–101. doi: 10.1016/j.scienta.2017.01.012
Morvan, X., Naisse, C., Issa, O. M., Desprats, J. F., Combaud, A., Cerdan, O. (2014). Effect of ground-cover type on surface runoff and subsequent soil erosion in Champagne vineyards in France. Soil Use Manage. 30, 372–381. doi: 10.1111/sum.12129
Netzer, F., Schmid, C., Herschbach, C., Rennenberg, H. (2017). Phosphorus-nutrition of European beech (Fagus sylvatica L.) during annual growth depends on tree age and P-availability in the soil. Environ. Exp. Bot. 137, 194–207. doi: 10.1016/j.envexpbot.2017.02.009
Nikiema, P., Nzokou, P., Rothstein, D. (2012). Effects of groundcover management on soil properties, tree physiology, foliar chemistry and growth in a newly established Fraser fir (Abies fraseri [Pursh] Poir) plantation in Michigan, United States of America. New Forests 43, 213–230. doi: 10.1007/s11056-011-9274-8
Olsen, S., Sommers, L., Page, A. (1982). Methods of soil analysis. Part 2. Chem. microbiological properties phosphorus. ASA Monogr. 9, 403–430.
Panigrahi, P., Srivastava, A. K., Pradhan, S. (2021). Runoff and soil conservation effects in Nagpur mandarin orchard under a sub-humid tropical climate of central India. Agric. Water Manage. 258, 107185. doi: 10.1016/j.agwat.2021.107185
Poesen, J. (2018). Soil erosion in the Anthropocene: Research needs. Earth Surface Processes Landforms 43, 64–84. doi: 10.1002/esp.4250
Prosdocimi, M., Cerda, A., Tarolli, P. (2016). Soil water erosion on Mediterranean vineyards: A review. Catena 141, 1–21. doi: 10.1016/j.catena.2016.02.010
Qian, F., Dong, L., Liu, J., Sun, B., Liu, H., Huang, J., et al. (2020). Equations for predicting interrill erosion on steep slopes in the Three Gorges Reservoir, China. J. Hydrology Hydromechanics 68, 51–59. doi: 10.2478/johh-2019-0024
Reis, A., Duarte, B., Duarte, A. (2024). Effect of ground cover on soil carbon storage in a citrus orchard: challenges and preliminary results. Acta Hortic. 1399, 491–498. doi: 10.17660/ActaHortic.2024.1399.61
Sayyad, E., Hosseini, S. M., Mokhtari, J., Mahdavi, R., Jalali, S. G., Akbarinia, M., et al. (2006). Comparison of growth, nutrition and soil properties of pure and mixes stands of Populus deltoids and Alnus subcordata. Silva Fennica 40, 27–35. doi: 10.1080/02827580600717605
Simon, J., Dannenmann, M., Gasche, R., Holst, J., Mayer, H., Papen, H., et al. (2011). Competition for nitrogen between adult European beech and its offspring is reduced by avoidance strategy. For. Ecol. Manage. 262, 105–114. doi: 10.1016/j.foreco.2011.01.035
Solangi, F., Bai, J., Gao, S., Yang, L., Zhou, G., Cao, W. (2019). Improved accumulation capabilities of phosphorus and potassium in green manures and its relationship with soil properties and enzymatic activities. Agronomy 9, 708. doi: 10.3390/agronomy9110708
Song, X., Gao, Y., Green, S. M., Dungait, J. A. J., Peng, T., Quine, T. A. (2017). Nitrogen loss from karst area in China in recent 50 years: An in-situ simulated rainfall experiment's assessment. Ecol. Evol. 7, 10131–10142. doi: 10.1002/ece3.3502
Su, X. K., Shen, Y., Xiao, Y., Liu, Y. Q., Cheng, H., Wan, L. F., et al. (2022). Identifying ecological security patterns based on ecosystem services is a significative practice for sustainable development in Southwest China. Front. Ecol. Evol. 9, 810204. doi: 10.3389/fevo.2021.810204
Tan, C., Zhang, T. (2011). Surface runoff and sub-surface drainage phosphorus losses under regular free drainage and controlled drainage with sub-irrigation systems in southern Ontario. Can. J. Soil Sci. 91, 349–359. doi: 10.4141/cjss09086
Tian, L., Zhu, B., Akiyama, H. (2017). Seasonal variations in indirect N2O emissions from an agricultural headwater ditch. Biol. Fertility Soils 53, 651–662. doi: 10.1007/s00374-017-1207-z
Tran, C. T. K., Watts-Williams, S. J., Smernik, R. J., Cavagnaro, T. R. (2021). Root and arbuscular mycorrhizal effects on soil nutrient loss are modulated by soil texture. Appl. Soil Ecol. 167, 104097. doi: 10.1016/j.apsoil.2021.104097
Tu, A., Xie, S., Zheng, H., Li, H., Li, Y., Mo, M. (2021). Long-term effects of living grass mulching on soil and water conservation and fruit yield of citrus orchard in south China. Agric. Water Manage. 252, 106897. doi: 10.1016/j.agwat.2021.106897
Villarreal, R., Lozano, L. A., Melani, E. M., Polich, N. G., Salazar, M. P., Bellora, G. L., et al. (2021). First-year cover crop effects on the physical and hydraulic properties of the surface layer in a loamy soil. Soil Tillage Res. 213, 105141. doi: 10.1016/j.agwat.2021.107185
Wang, Y., Huang, Q., Liu, C., Ding, Y., Liu, L., Tian, Y., et al. (2020b). Mulching practices alter soil microbial functional diversity and benefit to soil quality in orchards on the Loess Plateau. J. Environ. Manage. 271, 110985. doi: 10.1016/j.jenvman.2020.110985
Wang, Z., Li, H., Bai, Y., Zhang, J., He, X. (2020a). Erosion process and soil nutrient loss of purple soil slope with rainfall and cultivation measures in southwestern China. Sci. Total Environ. 739, 139928. doi: 10.1016/j.scitotenv.2020.139928
Wang, Y., Ni, C., Wang, S., Xie, D., Ni, J. (2021). A reliable U-trough runoff collection method for quantifying the migration loads of nutrients at different soil layers under natural rainfall. Sustainability 13, 2050. doi: 10.3390/su13042050
Wang, M., Pendall, E., Fang, C., Li, B., Nie, M. (2018). A global perspective on agroecosystem nitrogen cycles after returning crop residue. Agric. Ecosyst. Environ. 266, 49–54. doi: 10.1016/j.agee.2018.07.019
Wang, F., Yang, Q., Liu, Z., Wu, F. (2019a). Influence of vegetation coverage on soil erosion and water retention in sloping lands of southwest China. Int. Soil Water Conserv. Res. 7, 229–236. doi: 10.1016/j.iswcr.2019.04.003
Wang, L., Zhao, X., Guo, J. X., Butterly, C. R., Chen, Q. H., Liu, M. Q., et al. (2019b). Effects of fertilizer types on nitrogen and phosphorous loss from rice-wheat rotation system in the Taihu Lake Region of China. Agric. Ecosyst. Environment. 285, 106605. doi: 10.1016/j.agee.2019.106605
Winter, S., Bauer, T., Strauss, P., Kratschmer, S., Paredes, D., Popescu, D., et al. (2018). Effects of vegetation management intensity on biodiversity and ecosystem services in vineyards: A meta-analysis. J. Appl. Ecol. 55, 2484–2495. doi: 10.1111/1365-2664.13124
Wu, Y., Wang, X., Hu, R., Zhao, J., Jiang, Y. (2021). Responses of soil microbial traits to ground cover in citrus orchards in central China. Microorganisms 9, 2507. doi: 10.3390/microorganisms9122507
Xie, Y., Liu, B., Wu, Y., Zhang, S. (2016). The effect of legumes on nitrogen fixation and nutrient cycling in agroecosystems. J. Agric. Environ. Sci. 5, 203–213. doi: 10.1016/j.jaes.2016.05.001
Xie, M. X., Zhang, Z. Y., Zhang, P. C., Chen, T. J. (2018). Nitrate nitrogen transport and leaching from sloping farmland of purple soil: experimental and modelling approaches. Fresenius Environ. Bull. 27, 1508–1521. Available online at: http://www.prt-parlar.de/download_feb_2018/.
Xiong, H. Y., Ma, H., Zhao, H. Y., Yang, L. S., Hu, B., Wang, J., et al. (2022). Integrated physiological, proteome and gene expression analyses provide new insights into nitrogen remobilization in citrus trees. Tree Physiol. 42, 1628–1645. doi: 10.1093/treephys/tpac024
Xiong, M. Q., Sun, R. H., Chen, L. D. (2018). Effects of soil conservation techniques on water erosion control: A global analysis. Sci. Total Environ. 645, 753–760. doi: 10.1016/j.scitotenv.2018.07.124
Yagioka, A., Komatsuzaki, M., Kaneko, N., Ueno, H. (2015). Effect of no-tillage with weed cover mulching versus conventional tillage on global warming potential and nitrate leaching. Agric. Ecosyst. Environ. 200, 42–53. doi: 10.1016/j.agee.2014.09.011
Yang, Y. H. (2020). Studies on the growth and development, nutrient accumulation and release characteristics of green manure in citrus orchard. (MA thesis). Southwest University, Chongqing, China. doi: 10.27684/d.cnki.gxndx.2020.003198
Zhang, Y., Cheng, D., Zhang, Y., Xie, J., Xiong, H., Wan, Y., et al. (2021). Soil type shapes the antibiotic resistome profiles of long-term manured soil. Sci. Total Environ. 786, 147361. doi: 10.1016/j.scitotenv.2021.147361
Zhang, Q., Liu, D., Cheng, S., Huang, X. (2016). Combined effects of runoff and soil erodibility on available nitrogen losses from sloping farmland affected by agricultural practices. Agric. Water Manage. 176, 1–8. doi: 10.1016/j.agwat.2016.05.018
Zhang, Y., Xie, D., Ni, J., Zeng, X. (2020). Conservation tillage practices reduce nitrogen losses in the sloping upland of the Three Gorges Reservoir area: No-till is better than mulch-till. Agric. Ecosyst. Environ. 300, 107003. doi: 10.1016/j.agee.2020.107003
Zhang, L., Xu, J., Liu, Y., Shen, Q. (2017). Nutrient uptake patterns and demand of citrus trees at different growth stages. J. Plant Nutr. 40, 1167–1183. doi: 10.1080/01904167.2016.1254635
Zheng, H., Liu, Z., Zuo, J. C., Wang, L. Y., Nie, X. F. (2017). Characteristics of nitrogen loss through surface-subsurface flow on red soil slopes of southeast China. Eurasian Soil Sci. 50, 1506–1514. doi: 10.1134/S1064229317130063
Zheng, J. Y., Zhao, J. S., Shi, Z. H., Wang, L. (2021). Soil aggregates are key factors that regulate erosion-related carbon loss in citrus orchards of southern China: bare land vs. grass-covered land. Agric. Ecosyst. Environ. 309, 107254. doi: 10.1016/j.agee.2020.107254
Zhong, S., Han, Z., Duo, J., Ci, E., Ni, J., Xie, D., et al. (2019). Relationships between the lithology of purple rocks and the pedogenesis of purple soils in the Sichuan Basin, China. Sci. Rep. 9, 13272. doi: 10.1038/s41598-019-49687-9
Zhou, G. P., Cao, W. D., Bai, J. S., Xu, C. X., Zeng, N. H., Gao, S. J., et al. (2019). Non-additive responses of soil C and N to rice straw and hairy vetch (Vicia villosa Roth L.) mixtures in a paddy soil. Plant Soil 436, 229–244. doi: 10.1007/s11104-018-03926-6
Zhou, G. P., Gao, S. J., Lu, Y. H., Liao, Y. L., Nie, J., Cao, W. D. (2020). Co-incorporation of green manure and rice straw improves rice production, soil chemical, biochemical and microbiological properties in a typical paddy field in southern China. Soil Tillage Res. 197, 104499. doi: 10.1016/j.still.2019.104499
Keywords: citrus orchard, leaf nutrients, tree growth, groundcover management, purple soil, runoff, soil and nutrient losses
Citation: Liu R, Zhang Y, Wang Z, Zhang X, Xu W, Zhang J, Zhang Y, Hu B, Shi X and Rennenberg H (2024) Groundcover improves nutrition and growth of citrus trees and reduces water runoff, soil erosion and nutrient loss on sloping farmland. Front. Plant Sci. 15:1489693. doi: 10.3389/fpls.2024.1489693
Received: 01 September 2024; Accepted: 15 October 2024;
Published: 06 November 2024.
Edited by:
Probir Kumar Pal, Institute of Himalayan Bioresource Technology (CSIR), IndiaReviewed by:
Amílcar Duarte, University of Algarve, PortugalRamesh Chauhan, Institute of Himalayan Bioresource Technology (CSIR), India
Copyright © 2024 Liu, Zhang, Wang, Zhang, Xu, Zhang, Zhang, Hu, Shi and Rennenberg. This is an open-access article distributed under the terms of the Creative Commons Attribution License (CC BY). The use, distribution or reproduction in other forums is permitted, provided the original author(s) and the copyright owner(s) are credited and that the original publication in this journal is cited, in accordance with accepted academic practice. No use, distribution or reproduction is permitted which does not comply with these terms.
*Correspondence: Xiaojun Shi, c2hpeGpAc3d1LmVkdS5jbg==