- 1College of Forestry, Northwest A&F University, Yangling, Shaanxi, China
- 2State Key Laboratory of Soil Erosion and Dryland Farming on the Loess Plateau, Northwest A&F University, Yangling, Shaanxi, China
Introduction: It is unclear whether plants and microorganisms achieve niche complementarity by taking up different inorganic nitrogen (N) forms to alleviate N competition, particularly in N–limited regions.
Methods: This paper conducted a 15-day 15N tracer study (15NH4NO3 or 15NH4NO3) in situ to quantitatively calculate the uptake rates of plants and microorganisms in four stands (pure Hippophae rhamnoides L, pure Pinus tabuliformis Carrière, mixed H. rhamnoides–P. tabuliformis, and Artemisia gmelinii Weber ex Stechm grassland) in the forest–grassland transition zone on the Loess Plateau during the growing season. Among them, H. rhamnoides and P. tabuliformis can associated with arbuscular mycorrhizal and ectomycorrhizal, respectively.
Results: The results indicated that H. rhamnoides in the pure stand and A. gmelinii preferred to take up 15NO3–, whereas P. tabuliformis in the pure stand preferred 15NH4+. Compared to pure stands, mixed afforestation decreased the NH4+ and NO3– uptake rate of H. rhamnoides by 87% and 70%, respectively, but did not alter the N preference of plants. Plants and microorganisms differed in their N preferences in the pure stand, whereas this was not the case in the mixed stand. The proportional similarity index between H. rhamnoides and P. tabuliformis (0.90 ± 0.01) was higher than that between plants and microorganisms in forest stands, except for P. tabuliformis and microorganisms in the mixed stand (0.90 ± 0.02).
Discussion: Those results indicated that niche complementarity by preferring different N forms can alleviate N competition. This study helped to gain a deeper understanding of the plasticity of N uptake patterns by plants and microorganisms in the forest–grassland transition zone, and provides theoretical support for vegetation restoration during the implementation of the Grain for Green program on the Loess Plateau.
1 Introduction
Soil inorganic nitrogen (N), NH4+ and NO3–, is the primary N source for the majority of plants and is often considered a limiting factor for ecosystem productivity (Andersen and Turner, 2013; Cui et al., 2017; Zhou et al., 2020; He et al., 2022). Previous studies demonstrated that N demand may lead to competition for NH4+ and NO3– among different plants and between plants and microorganisms under N limiting conditions (Kuzyakov and Xu, 2013; Song et al., 2017; Hu et al., 2021). Coexisting species and microorganisms take up different N forms, i. e. niche complementarity, which has been considered the main mechanism of alleviating soil N limitation (Miller et al., 2007; Ashton et al., 2010; Liu et al., 2020). Human activities have significantly affected the chemical forms of atmospheric inorganic N deposition over recent decades (Fowler et al., 2013; Ackerman et al., 2018; Yu et al., 2019; Gurmesa et al., 2022). Therefore, understanding the inorganic N uptake patterns by plants and microorganisms is essential for optimizing resource utilization in N–limited ecosystems.
Soil N availability was acknowledged to influence N uptake by plants (Zhu et al., 2019; Hu et al., 2021). In general, plants prefer the most abundant form of N in the soil (Mckane et al., 2002; Kahmen et al., 2006; Cui et al., 2017; Liu et al., 2020). In addition, different mycorrhizal symbiosis types, such as arbuscular mycorrhizal (ACM) and ectomycorrhizal (ECM), have also been suggested as important factors affecting N uptake of plants (Liu et al., 2020). ACM increases N uptake by decomposing organic matter and generally acquires high mobility of NO3– faster than other mycorrhizal types (Xie et al., 2022). Conversely, plants associated with ECM take up more NH4+ compared to NO3– due to the high assimilation of NH4+ by extraradical mycelium (Javelle et al., 2008; Phillips et al., 2013; Zhang et al., 2019). However, it is still unclear whether the plant N preferences are influenced by different root associations with mycorrhizal types under similar soil N availabilities.
Competition among plants and between plants and microorganisms in N–limited regions have been reported to exert impacts on plant N uptake rate and preference (Kahmen et al., 2006; Andersen et al., 2017; Hu et al., 2021). Liu et al. (2021) demonstrated that plants dependent on ACM (Michelia maudiae and Schima superba) exhibit inhibitory effects on inorganic N uptake of two ECM plants (Pinus massoniana and Pinus elliottii) in mixed forests. In addition, microorganisms are often considered the main competitors of plants for soil N, as they grow rapidly and have a high surface area–to–volume ratio (Hodge et al., 2000; Harrison et al., 2007; Andersen et al., 2017). Traditionally, it was assumed that microorganisms are more inclined to prefer NH4+ due to its lower energy costs (Fraterrigo et al., 2011; Wang and Macko, 2011; Liu et al., 2016). However, some experiments indicated that microorganisms can take up NO3– as efficiently as NH4+ (Inselsbacher et al., 2009; Kaštovská and Šantrůčková, 2011), presumably due to the higher NO3– mobility in soils (Hodge et al., 2000; Song et al., 2007; Inselsbacher et al., 2009). Currently, most studies use roots or seedlings in hydroponic and pot experiments to investigate plant N preferences (Templer and Dawson, 2004; Li et al., 2016; He et al., 2022). The results, however, could not take into account the impact of N competition among plants and between plants and microorganisms on the N uptake of plants (Uscola et al., 2017; Zhou et al., 2020). Therefore, quantifying the degree of competition among plants and between plants and microorganisms is recommended in characterizing plant N uptake patterns in N–limited conditions.
Severe soil erosion on the Loess Plateau has led to a lack of soil N in the region (Chen et al., 2021; Yan et al., 2022; Tang et al., 2024). To enhance soil and water conservation, policies related to “Grain for Green” have been implemented (Fu et al., 2016; Tang et al., 2019). However, the lack of N during vegetation reconstruction also poses a challenge to plant growth (Gao et al., 2018). Yu et al. (2019) found that the amount of inorganic N deposited has increased, and NHx was slightly higher than NOy over the past decade on the Loess Plateau. Studies have revealed that Hippophae rhamnoides L and Pinus tabuliformis Carrière can associate with ACM and ECM, respectively (Zhang et al., 2017; Tang et al., 2019), which are ecological and economic plants in the region (Wang et al., 2022; Ning et al., 2023). Artemisia gmelinii Weber ex Stechm is a typical herbaceous plant species in this forest–grassland transition zone with a wide coverage area (Li et al., 2022). Additionally, mixed plantations of H. rhamnoides with P. tabuliformis have been widely used on the Loess Plateau due to the high soil conservation capacity (Tang et al., 2019). Therefore, understanding the N uptake patterns of plants and microorganisms is crucial to improving the available N utilization efficiency under soil N–limited conditions on the Loess Plateau.
This study conducted in situ 15N labeling experiments (NH4+ and NO3–) in four stands (H. rhamnoides, P. tabuliformis, H. rhamnoides–P. tabuliformis mixed stand, and A. gmelinii) in the forest–grassland transition zone of the Loess Plateau. The main intentions of this research include: (1) examining the NH4+ or NO3– preferences of H. rhamnoides, P. tabuliformis, and A. gmelinii; and (2) assessing the degree of interspecific plant competition and competition between plants and microorganisms for soil N in different stands. Based on previous studies, the following hypotheses were made: (1) H. rhamnoides and P. tabulaeformis maytake up NO3– and NH4+, respectively, due to differences in the association of their roots with mycorrhizal types; (2) A. gmelinii prefer to take up the dominant N forms in the soil; (3) microorganisms primarily take up NH4+ with low energy efficiency.
2 Materials and methods
2.1 Study area
This research was performed in Ansai ecological station of the Chinese Academy of Sciences (109.3137°E, 36.8723°N, 1230–1237 m above sea level, Supplementary Figure S1), in the forest–grassland transition zone on the Loess Plateau. The area with a temperate semi–arid climate (Tang et al., 2018a). The average annual air temperature is 10.7 ± 0.1°C and the average annual precipitation is 456.9 ± 5.7 mm (2000–2021, mean ± SE). Three forest stands planted in 2004 and one naturally growing grassland were selected as the study sites, i.e., pure H. rhamnoides stand, pure P. tabuliformis stand, mixed H. rhamnoides–P. tabuliformis stand, and A. gmelinii stand. The soil in this area was divided into silt loam soil depending on the USDA soil taxonomy classification with 65% silt, 22% sand, and 13% clay (Tang et al., 2024). The detailed data of soil has been shown in Table 1. According to Güsewell (2004) and Sorrell et al. (2011) description, the N: P ratio of plant leaves in this area ranges from 11.26 (P. tabuliformis (p)) to 15.65 (H. rhamnoides (p)) (Table 2), which belongs to the N-limited zone. Three replicated plots were selected for each forest (20 m × 20 m) and grassland (10 m × 10 m) stands. The understory grasses were Glycyrrhiza uralensis and Bothriochloa ischaemum in forest stands (Tang et al., 2018b). The original tree density for H. rhamnoides and P. tabuliformis in the pure stands (H. rhamnoides (p) and P. tabuliformis (p), respectively) were 3025 trees ha–1, with a spacing of 2 m between each plant. The H. rhamnoides (1650 trees ha–1) and P. tabuliformis (1375 trees ha–1) in the mixed stand (H. rhamnoides (m) and P. tabuliformis (m), respectively) have six and five rows, respectively. P. tabuliformis were planted in a 4 m gap between rows of H. rhamnoides. The vegetation coverage rates were 80.72%, 74.76%, 87.01%, and 85.64% in the H. rhamnoides pure stand, P. tabuliformis pure stand, mixed stand, and grassland, respectively. The coverage rates of H. rhamnoides and P. tabuliformis in the mixed stand were 47.43% and 63.30%, respectively. Tree crown size of H. rhamnoides (p), P. tabuliformis (p), H. rhamnoides (m) and P. tabuliformis (m) were 2.18 m2, 4.22 m2, 1.92 m2, and 4.73 m2 (Table 2).
2.2 Experimental design
The experiment was set up in June and August 2022. To analyze the inorganic N uptake patterns of plants and microorganisms, there were two types of 15N labeling solutions, 15NH4NO3 (99.12 atom%) and NH415NO3 (99.21 atom%) were employed. A solution with the same amount of unlabeled NH4NO3 was used as the control (Kaštovská and Šantrůčková, 2011). To avoid N fertilization effects, 15N was added at a low rate of 0.441 g m–2, which was equal to 8% of local atmospheric N deposition in the study region (20 kg N ha–1 year–1) (Guo et al., 2020). Adjacent H. rhamnoides and P. tabuliformis received the same labeling solutions simultaneously in the mixed stand. Therefore, each plant can accept the same molar concentration of NH4+–N and NO3––N. The total amount of added N did not cause additional disturbance of the different N pools in the soil (including total N, NH4+, and NO3–; P > 0.05) (Supplementary Table S1). In addition, ceramic soil suction samplers were installed in each plot to collect soil solution at a depth of 80 cm. A subplot (2 m × 2 m) was randomly selected within each plot to collect surface runoff.
The labeling experiment was conducted on 25 July 2022. Three subplots of 2.5 m × 2.5 m and 1 m × 1 m were established in each of the forest and grassland plots to receive three N forms. Three replicates per treatment were established in each plot. To exclude competition effects on N uptake patterns from other plants, the grasses in each forest plot were cut manually by clipping stems 10 cm above the ground (Zhu et al., 2021). In each forest and grassland subplot, the tracers were dissolved in 31.25 L and 5 L of pure water (approximately equal to 5 mm precipitation), respectively, which was approximately 1% of the local rainfall. Two backpack sprayers were used to spray the solution evenly and directly on the soil surface to avoid cross contamination (Zhou et al., 2020). The litter in the sample subplot was removed before 15N labeling to prevent the litter retaining 15N tracers and then returned to its original location after 15N labeling. Several rainfall events occurred after 15N labeling, with daily precipitation ranging from 0.0 to 24.2 mm (Supplementary Figure S2), which promoted infiltration of the 15N tracer. Furthermore, leachate in the 80 cm soil layer and lateral surface runoff were not observed at any sampling times. This result indicated that most of the labeled solution was retained in the 0–50cm soil layer. If the δ15N by plant organs and whole plant in the NO3– labeled treatment were more than in the NH4+ labeled treatment, then the plants preferred NO3–, and vice versa (Wang and Macko, 2011; Zhou et al., 2020).
The plant organs and soil samples in each subplot were collected at 3 (28 July), 7 (1 August), and 15 days (9 August) after 15N tracer application. The organs of trees were divided into leaves, branches, and roots. The organs of grass were divided into leaves and root. Fine roots (< 2 mm) were manually selected from the soil sample. To determine mycorrhizal colonization rates, additional fine roots were collected from each of the four cardinal directions (north, south, east, west) from trees in the control subplot before labeling (n = 9). Roots were then extracted from blocks by washing with water and maintained at 4 °C. The soil samples were collected using a 6 cm diameter spiral drill and divided into two layers (0–20 and 20–50 cm). More than 75% of the fine roots were concentrated within those layers (Supplementary Figure S3). All samples from each subplot were pooled into one composite sample. The soil sample was divided into two portions. One portion was stored at −20°C until subsequent microbial determination. The other portion was used to determine the soil properties, including total carbon content (TC), total N content (TN), total phosphorus (TP), available phosphorus (AP), pH, and the concentration of NO3– and NH4+. Plant samples were also transferred to the laboratory for determination of the total N concentrations and δ15N. An in situ soil core cultivation method was used to determine the net N conversion rate (Xu et al., 2007). Two polyvinyl chlorid cylinders were driven into the soil on each of 3, 7, and 15 days after labeling. The soil in one of the polyvinyl chlorid tubes was extracted to determine the initial concentrations of inorganic N, while the other was incubated in the soil for 15 days before determining inorganic N.
2.3 Chemical analyses
All plant leaves, branches, and root samples were cleaned using deionized water and then dried to a constant weight at 75°C. Soil sample, after air drying, was sieved through a 2 mm sieve and then maintained at 4°C until measurements. Plants and soil samples were ball–milled and the TC, TN, and δ15N were analyzed by elemental analyzer–isotope ratio mass spectrometry (Finnigan MAT253, Thermo Finnigan, Germany). TP and AP concentration of the soil were analyzed by the HClO4–H2SO4 colorimetric (Wang et al., 2012). The concentration of inorganic N, extracted by 2 mol L–1 potassium chloride, was measured using a high–performance flow injection auto–analyzer (AA3, SEAL, Germany) (Zhou et al., 2020). A pH meter (Sartorius PB-10, Gottingen, Germany) was used to gauge soil pH in 1:2.5 (soil/solution ratio) water extract. To measure the soil moisture, partial soil samples were dried at 105°C for 2 d. Microbial biomass N and δ15N were determined using the chloroform fumigation–extraction technique according to Andersen et al. (2017) and Ma et al. (2021). Briefly, a portion of fresh soil was treated with chloroform fumigation for 24 h, and the fumigated sample and another portion of fresh soil were removed using 0.5 mol L–1 potassium sulfate. Subsequently, the solutions were filtered, freeze–dried, and analyzed for N and δ15N concentrations. The enzyme–linked immunosorbent assay kit was used to determine the activities of plant root nitrate reductase (NR) and glutamine synthetase (GS) (JLC455621 and JLC455326, Jining Industrial Co., Ltd, Shanghai) (Ji et al., 2024). The 5% acetic acid ink and 0.05% trypan blue methods were used to detect the ACM and ECM infection rates of plant roots, respectively (Phillips and Hayman, 1970; Vierheilig et al., 1998).
The 15N uptake rate (µg 15N g–1 d–1) was estimated using the following formulas (Templer and Dawson, 2004):
where APE represents the atom% excess; atom%labeled and atom%control represent the atom% in the labeled and unlabeled organs, respectively; N concentrations represent the dry masses of N in the N pool (leaves, branches, roots, or microorganisms, µg g–1); and atom%15N tracer is the atomic percentage of applied 15N–labeled N (99.12 atom% for 15NH4NO3 and 99.21 atom% for NH415NO3). The N uptake rate of whole plants was calculated by summing the weighs of the N concentrations of the different organs.
The net mineralization (Rmineralization), nitrification (Rnitrification), and ammonification (Rammonification) rates were calculated using the following formulas (Xu et al., 2007):
where t1 and t2 represent the initial and after incubation time, respectively; c is the mean N concentration.
The proportional similarity (PS) index was used to evaluate the degree of N competition between plants and microorganisms in the four stands, and between plants in the mixed stand. PS was calculated as follows (Hoekstra et al., 2014):
where P1i and P2i represent the same form of N uptake proportion (NH4+ or NO3–) of the plants and microorganisms in each stand, respectively, or different plants in the mixed H. rhamnoides–P. tabuliformis stand. The PS index ranged from 0 to 1, and the niche overlap of the N uptake patterns increased as the PS index increased (Phillips and Gregg, 2003; Hoekstra et al., 2014).
2.4 Statistical analyses
To test for normality, the Kolmogorov–Smirnov test was applied, and Levene’s test was conducted to assess the homogeneity of variances before analyzing the data. If the normality assumption was not met, the data were log–transformed. However, the soil properties (TC, TN, TP, AP, pH, moisture, net N conversion rate, NH4+ concentration, NO3– concentration, and NH4+:NO3– ratio) among the four stands and in plant root–related variables (mycorrhizal colonization rate, NR, and GS activity) among plants were still not normally distributed after transformation. Therefore, the Kruskal–Wallis test and Dunn’s test were used to examine the differences among these parameters (Zhang et al., 2018). The 15N uptake rates of plants and microorganisms were analyzed using the repeated measurements method with sample time as a repeated variable, and N forms as factors (Zhang et al., 2019). If the interaction between N forms and sample time was significant, one–way analysis of variance (ANOVA) was conducted to analyze the 15N uptake rate differences of plants and microorganisms for a specific sampling date (at 3, 7, and 15 days after labeling) (Wang et al., 2020). The differences 15N uptake rates for the above–ground parts of plants and whole plants between the different plants, and for microorganisms between the four stands were analyzed using one–way ANOVA followed by least significant difference tests. The same method was performed to analyze the differences in PS index between different plants in the mixed stand and between plants and microorganisms among the four stands. All statistical tests were conducted using SPSS 26.0 (IBM Corp, Armonk, NY, USA). Graphs were prepared in Origin 2023b software.
3 Results
3.1 Soil and plant properties
Ammonium was the main form of inorganic soil N in all stands (Figure 1), with concentrations ranging from 1.71 to 2.37 mg N kg–1, which was 2.02 to 3.21 times that of NO3– in the 0–50 cm soil (Table 1). Furthermore, the highest inorganic N concentrations were found in the 0–20 cm soil layer of the H. rhamnoides pure stand (P< 0.05) (Figure 1A). In addition, the NH4+:NO3– ratio for the P. tabuliformis pure stand (3.33 ± 0.48) was higher than that of the other stands. However, the H. rhamnoides pure stand had the highest Rnitrification (0.49 ± 0.18 mg kg–1 d–1; P< 0.05) (Table 1). The soil pH was 8.86, 8.90, 8.84, and 8.94 in the H. rhamnoides stand, P. tabuliformis stand, H. rhamnoides–P. tabuliformis mixed stand, and grassland, respectively. Average soil moisture ranged from 14.22% to 15.48% and there were no significant differences in pH and soil moisture among the four stands (P > 0.05) (Table 1).
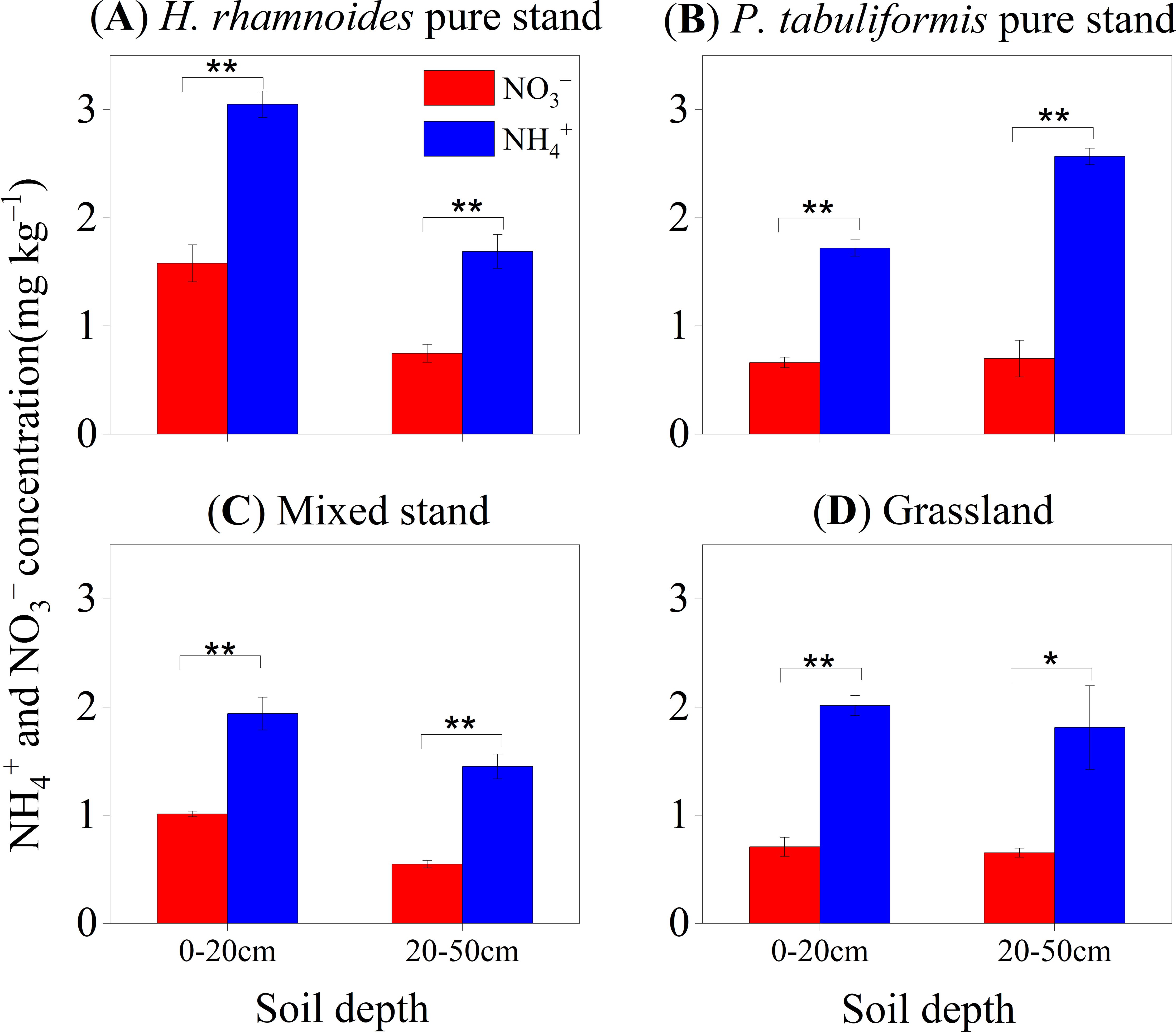
Figure 1. Concentrations of NH4+ and NO3– in different soil layers in the four stands of (A) H. rhamnoides, (B) P. tabuliformis, (C) Mixed, and (D) Grassland (mean ± SE, n = 3). Error bars provide ± SE. Asterisks indicate significant differences in concentration between the two N forms in each soil layer of individual stands (*, P< 0.05; **, P< 0.01).
Leaf N concentrations significantly differed among the three plants. The highest and lowest leaf N concentrations occurred in H. rhamnoides and P. tabuliformis (p), respectively (P< 0.01) (Table 2). The mycorrhizal colonization rate ranged from 43.31% to 52.92%, but there was no significant difference between plants (P > 0.05). Moreover, there were significant differences in the NR and GS activities among the plant roots (P< 0.05). The range of NR and GS activity in plant roots were 43.81 U L–1 to 58.04 U L–1 and 18.90 U L–1 to 34.23 U L–1, respectively. The NR and GS activities in H. rhamnoides (p) were lower than those in H. rhamnoides (m). The roots of H. rhamnoides (p) also had the lowest GS activity among the three plants (P< 0.05) (Table 2).
3.2 Changes in δ15N and 15N uptake rates
After labeling with the 15N tracer, the δ15N in all plant organs significantly increased over the experimental period (P< 0.01) (Supplementary Figures S4, S6). Repeated measurement ANOVA showed that the δ15N values in plant organs with 15NO3– labeling were higher than those with 15NH4+ labeling for H. rhamnoides and A. gmelinii (Supplementary Figures S4A, B, S6A). Conversely, the organs of P. tabuliformis exhibited higher δ15N values for 15NH4+ than for 15NO3– labeling (Supplementary Figures S4C, D).
The average uptake rate of 15NH4+ and 15NO3– by trees were 0.304 ± 0.024 and 0.398 ± 0.066 µg 15N g–1 d–1, respectively (Figures 2A–D). The 15NO3– uptake rates for H. rhamnoides (p) and H. rhamnoides (m) were significantly higher than those for 15NH4+ (P< 0.01). Conversely, P. tabuliformis had higher 15N uptake rates for 15NH4+ than for 15NO3– (P< 0.01) in the pure and mixed stands (Figure 2). The A. gmelinii 15N uptake rate for 15NH4+ was significantly lower than that for 15NO3– (P< 0.01) (Figure 2E). After 15N labeling, the N uptake rates of the whole plant and specific organs were significantly decreased for 15NH4+ and 15NO3– labeled in all sample stands (Supplementary Figures S5, S6).
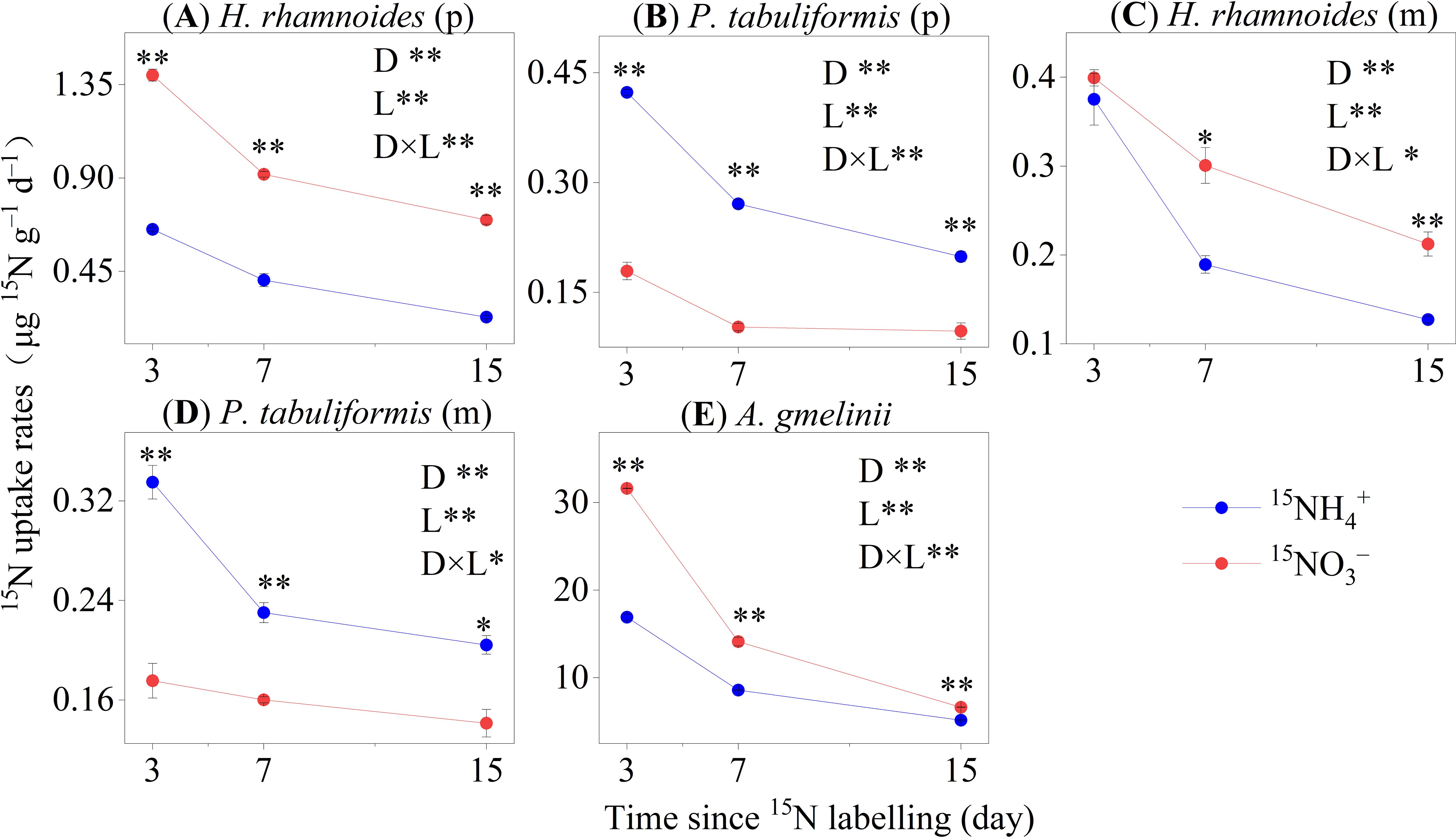
Figure 2. The 15N uptake rates of whole plants in the four studied stands of (A, C) H. rhamnoides, (B, D) P. tabuliformis and (E) A. gmelinii (means ± SE, n = 3). Error bars provide ± SE. Asterisks indicate significant differences in the 15N uptake rates between N forms within each stand (*, P< 0.05; **, P< 0.01). D: Time since 15N labeling (day); L: The form of applied 15N labeled N. (p) and (m) represent plants in pure and mixed stands, respectively.
Among the four stands, the average 15NH4+ and 15NO3– uptake rates by microorganisms were 0.014 ± 0.002 and 0.009 ± 0.001 µg 15N g–1 d–1, respectively (Figures 3A–D). In the H. rhamnoides pure stand, mixed stand, and grassland, the 15NH4+ uptake rates for microorganisms were significantly higher than those for 15NO3–, while the opposite result was observed in the P. tabuliformis pure stand (P< 0.01) (Figure 3B). In addition, the microorganisms in the P. tabuliformis pure stand had the lowest 15NH4+ uptake rate (P< 0.01) (Figures 4D, H).
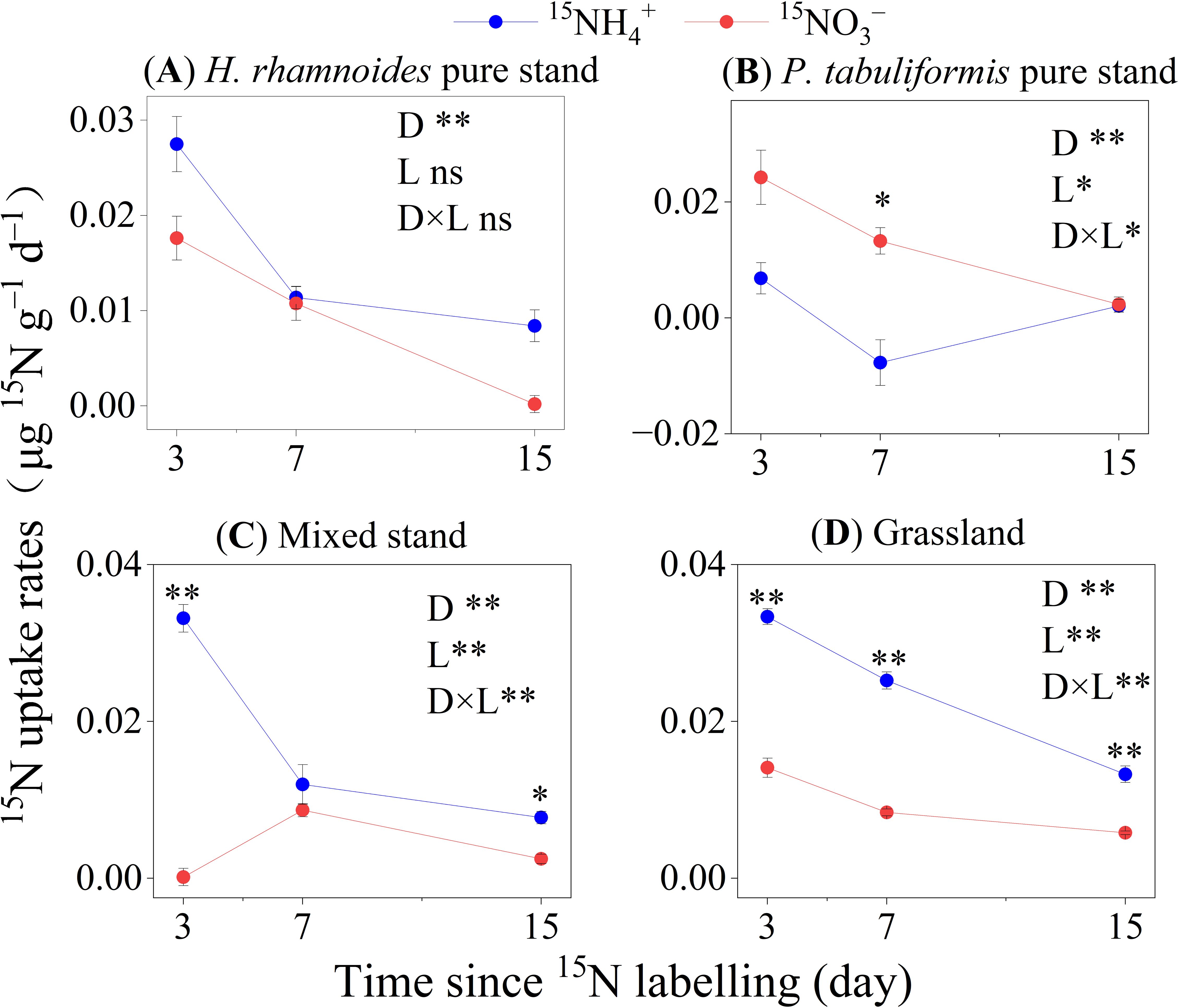
Figure 3. The 15N uptake rates of microorganisms in the four studied stands of (A) H. rhamnoides, (B) P. tabuliformis, (C) Mixed, and (D) Grassland (means ± SE, n =3). Error bars provide ± SE. Asterisks indicate significant differences in the 15N uptake rates between N forms within each stand (*, P< 0.05; **, P< 0.01; ns, no significant difference, p>0.05). D: Time since 15N labeling (day); L: The form of applied 15N–labeled N.
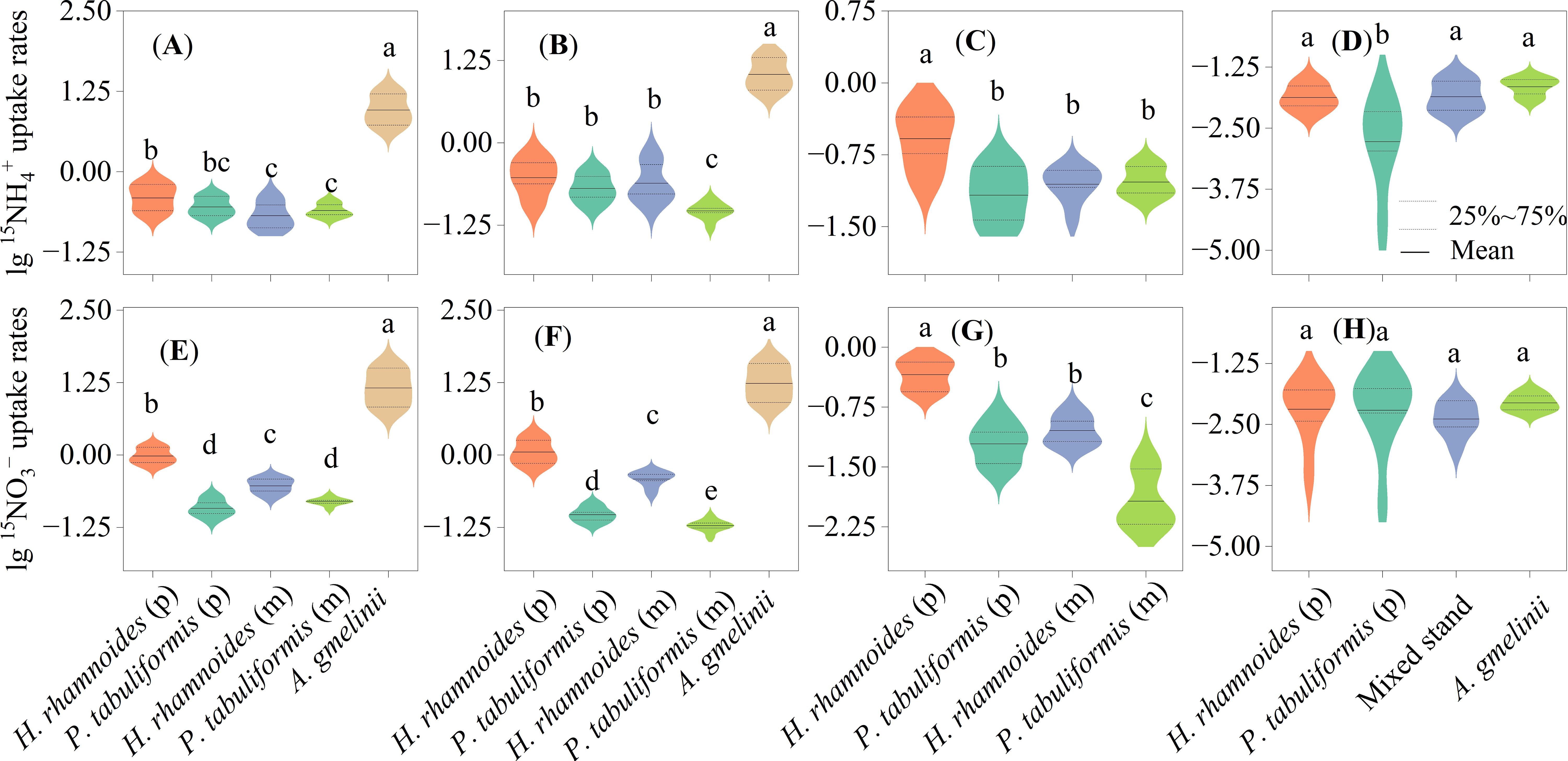
Figure 4. Uptake rates of 15N tracer of 15NH4+ (A–D) and 15NO3– (E–H) in the whole (A, E), leaves (B, F), branches (C, G) of plants and microorganisms (D, H) in the four studied stands. Different letters indicate significant differences in N uptake rates between sample stands. (P) and (M) represent plants in and mixed stands, respectively.
The inorganic N uptake rates of A. gmelinii were significantly higher than those for the two trees (P< 0.05) (Figures 4A, E). The highest N uptake rates of leaves and branches appear in A.gmelinii and H. rhamnoides (p) (P<0.01) (Figures 4B, C, F, G). Pinus tabuliformis had the lowest 15NO3– uptake rate in the pure and mixed stands (P< 0.05) (Figure 4E). The 15NO3– uptake rates for the three plants were in the order: H. rhamnoides (p) > H. rhamnoides (m) and P. tabuliformis (m) > P. tabuliformis (p) (P< 0.05) (Figure 4E).
3.3 Differences in PS index among the four stands
The PS index between P. tabuliformis and microorganisms in the pure stand was significantly lower than that in the mixed stand, and was the lowest among the four stands (0.74 ± 0.04; P< 0.05) (Table 3). Conversely, there was no significant difference in the PS index between H. rhamnoides and microorganisms in the pure and mixed stands. In addition, the PS index between H. rhamnoides and P. tabuliformis was higher than that between plants and microorganisms, except for P. tabuliformis and microorganisms in the mixed stand (Table 3).
4 Discussion
4.1 Uptake of different N forms by the three plants
A major aim of this study was to explore the N preferences of two dominant afforestation tree species and grass in the forest–grassland transition zone on the Loess Plateau. The results showed that H. rhamnoides (p) associated with ACM had a strong preference for NO3–, although NH4+ was the main N form in the soil (Figures 2A, 5), which supports the first hypothesis. There may be two possible reasons for the preference for NO3–. First, soil NO3– has a higher fluidity and solubility than NH4+, and thus, can more easily transport to the root surface (Zhu et al., 2019; Hu et al., 2021), which would promote H. rhamnoides uptake of NO3–. In contrast, NH4+ is easily adsorbed by microorganisms and organic matter in the soil due to enzyme production based on their needs and the availability of substrates (Geisseler et al., 2010; Zhou et al., 2020). Second, the higher uptake rates for NO3– than NH4+ might be related to different uptake systems. NR converts NO3– into NO2–, which is the first and rate–limiting step in nitrate assimilation (Zhou et al., 2020). However, NH4+ must be converted into an organic form by GS before it can be transported throughout the plant (Zhu et al., 2019). Thus, the higher NR activity and lower GS activity (Table 2) of H. rhamnoides (p) may have promoted the uptake of NO3– by plants compared to that observed in the other three stands (Meng et al., 2016; Hu et al., 2021).
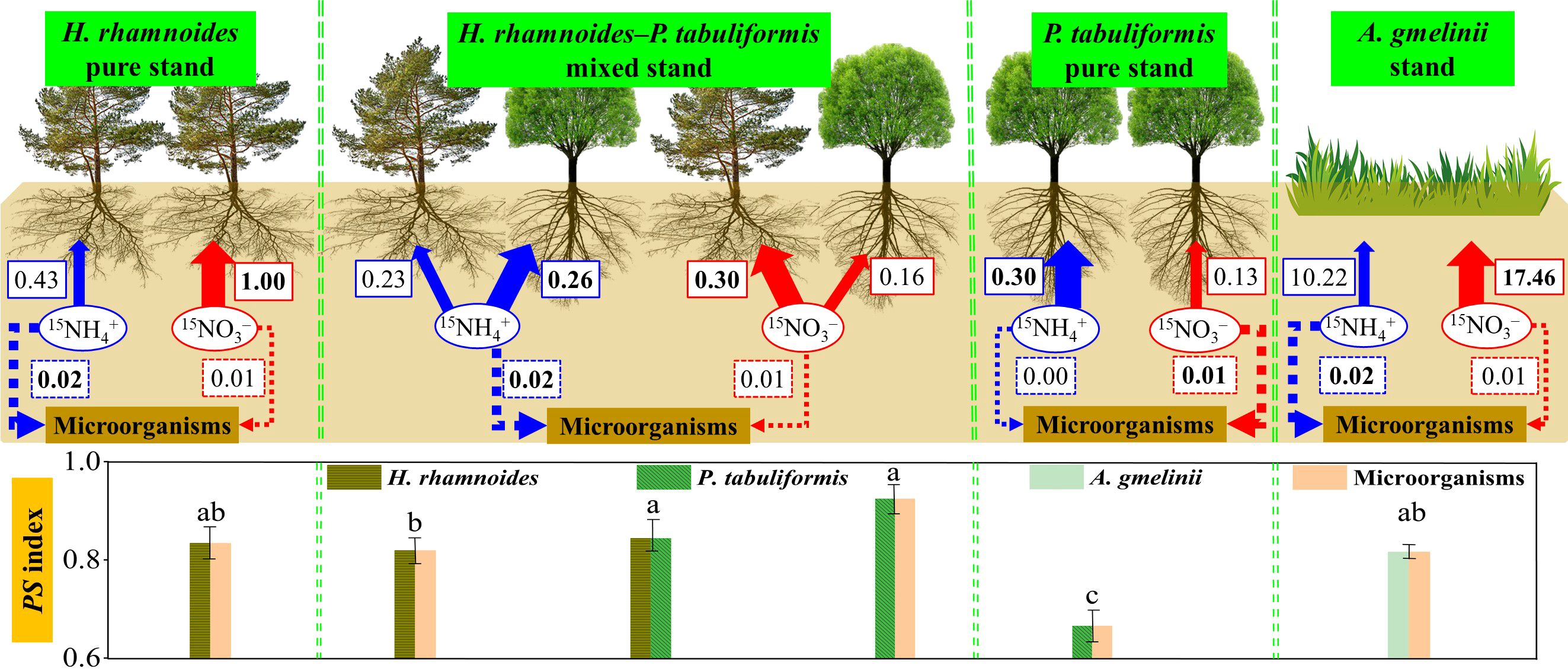
Figure 5. The schematic diagram of this study. The solid and dashed arrows represent the N uptake by plants and microorganisms in the four stands, respectively. The blue and red letters in the boxes represent the average uptake rates of NH4+ or NO3– in each stand, respectively (µg 15N g–1 d–1). Different letters indicated significant differences of PS index among the four stands (P< 0.05). Bold numbers and lines represent the main forms of inorganic N preferred by plants and microorganisms.
Pinus tabuliformis (p) took up more NH4+ compared to NO3– during the 15 day experimental period (Figures 2B, 5; Supplementary Figures S7, S8B). That finding confirmed that conifer trees were more inclined to take up NH4+ compared to NO3– (Gruffman et al., 2014; Castro–Rodríguez et al., 2017; Gurmesa et al., 2022). ECM symbiosis may be a potential mechanism for the high NH4+ uptake rate because of the assimilation by extraradical mycelium and high-affinity NH4+ transport genes (Andersen et al., 2017; Castro–Rodríguez et al., 2017). In addition, the highest soil NH4+:NO3– ratios in the P. tabuliformis pure stand among the four stands may also contribute to the uptake of NH4+ over NO3– by P. tabuliformis (p) (Cui et al., 2017; Hong et al., 2018). Overall, the combination of ECM and the highest NH4+: NO3– ratios resulted in a higher NH4+ but a lower NO3– uptake by P. tabuliformis compared to H. rhamnoides (Table 1).
Inconsistent with the second hypothesis, A. gmelinii had a higher uptake rate of NO3– than NH4+ (Figures 2E, 5; Supplementary Figures S7, S8C). A. gmelinii has a shorter lifespan and faster growth rates, and therefore, the preference for NO3– could be attributed to the high mobility of NO3–, which allows the N demand of A. gmelinii to be met (Xiong et al., 2021; Yuan et al., 2024). This conclusion is supported by the significantly higher uptake rate of NO3– by A. gmelinii leaves than NH4+ (Supplementary Figure S5). In addition, the higher root NR activity (Table 2) in grassland may also promote the uptake of NO3– by A. gmelinii (Meng et al., 2016; Hu et al., 2021).
The two added N forms (15NH4+ and 15NO3–) are labeled with the same molar, but due to the imbalance in soil NH4+ and NO3– concentrations, the tracers were diluted to varying degrees (Buchmann et al., 1995). Taking the dilution effect into account, the relative proportion of NO3– to total 15N tracer uptake ranged from 57.23% to 77.98% for the complete plant by H. rhamnoides (p) and A. gmelinii (at days 3, 7,15; Supplementary Table S2). The highest proportion of 15NH4+ occurred in P. tabuliformis (p), accounting for 75.06%, 77.93%, and 73.56% of total N uptake at days 3, 7,15 (Supplementary Table S2). This study also supports the conclusion of Zhu et al. (2021) that tracers with high enrichment can reduce the dilution of the inorganic soil N pool. Therefore, the dilution effect did not affect the preferences of plants for different N forms in this study.
4.2 Mixed afforestation did not alter the N uptake patterns by plants
Previous studies indicated that interspecific plant competition could affect the preference of N form by plants (Song et al., 2017). However, mixed afforestation did not alter the N preference by plants in this study, but decreased the N uptake rate of H. rhamnoides. This finding suggested that the N uptake patterns of plants mainly depended on species rather than soil N availability, which is also consistent with studies by Song et al. (2017) and Hu et al. (2021).
The strategy of niche complementarity has been proposed as a mechanism to alleviate soil N limitation and promote species coexistence (Ashton et al., 2010; Pang et al., 2019). This suggestion was supported by the results of the present study, which suggest that H. rhamnoides (m) and P. tabuliformis (m) tend to exploit different soil N forms to meet their N demands (Figures 2C, D, 5). The PS index also confirmed that the different N preferences of plants was an effective method to alleviate N limitation in the mixed stand. Additionally, the relatively higher level of NH4+ in the soil can also meet the needs of P. tabuliformis and microorganism growth (Figure 1; Table 1). The decrease in the 15NH4+ and 15NO3– uptake rate of H. rhamnoides may be linked to the limitation of light. Plants must allocate the C produced by photosynthesis to roots to obtain N from soil (Perkowski et al., 2021). However, the tree height and average crown diameter of P. tabulaeformis were larger than H. rhamnoides, and the average crown diameter of P. tabulaeformis was larger than the planted distance between them in the present study (Table 2). Therefore, the limitation of light by P. tabulaeformis led to a lower level of photosynthesis and N demand of H. rhamnoides in the mixed stand compared to that in the pure stand (Tang et al., 2019). Thus, H. rhamnoides had an insufficient C cost associated with obtaining N, resulting in a decrease in the N uptake rate. In addition, the relatively low Rmineralization and Rnitrification may limit N uptake of H. rhamnoides, which has a high N demand, compared to P. tabulaeformis (Van den Berg et al., 2016; Mao et al., 2021).
4.3 Competition for inorganic N between plants and soil microorganisms
Microorganisms preferred NH4+ over NO3– in the pure H. rhamnoides, mixed, and grasslands stands, despite the dilution impact being considered (Figures 3, 5; Supplementary Figure S9). This result was consistent with previous studies indicated that microorganisms preferred NH4+ due to the lower energy costs associated with NH4+ uptake (Berger et al., 2008; Fraterrigo et al., 2011; Liu et al., 2016). In addition, the relatively high NH4+:NO3– ratio in soil may meet the N demands of microorganisms(Figure 1; Table 1), which further promotes N uptake.
The finding that microorganisms exhibited a higher uptake rate for NO3– than for NH4+ in the P. tabuliformis plot was unexpected (Figures 3B, 5; Supplementary Figure S9B). A possible explanation may be related to the competition between plants and microorganisms for N (Harrison et al., 2007; Andersen et al., 2017). Previous study suggested that plants and microorganisms take up different forms of N to alleviate N competition (Kaštovská and Šantrůčková, 2011). Thus, microorganisms may mainly rely on NO3– uptake to alleviate competition with P. tabuliformis for NH4+ (Figure 3B; Table 3).
Prior studies also suggested that contrasting forms of N uptake between plants and microorganisms could largely result in niche complementarity (Ashton et al., 2010; Liu et al., 2022). As confirmed by this study, niche complementarity predicts that species can alleviate N competition by differing their preference for N forms (Ashton et al., 2010; Liu et al., 2020). The PS index between plants and microorganisms was lower by taking up different N forms than those taking up same N forms (Table 3). In addition, the PS index indicated that even plants take up different N forms, the competition between two plants tends to be higher than that between plants and microorganisms, which may be related to the duration of the experiment. Previous studies indicated that N uptake by plants significantly increased 24 h after adding tracers, while microbial N uptake was “saturated” within 24 h because of insufficient C availability (Inselsbacher et al., 2009). Meanwhile, the unidirectional N flux from soil to roots promotes N migration from microorganisms to plant roots, which enables plants to gain an advantage over microorganisms during long–term competition for N (Kaštovská and Šantrůčková, 2011; Kuzyakov and Xu, 2013). The competition between plants and soil microorganisms for inorganic N directly affects the ecosystem productivity and stability. Besides, differences in their preferences for inorganic N can enhance N available and reduce N leaching losses. Based on the spatiotemporal niche differentiation of N between plants and microorganisms, N supply can be effectively regulated. This allows for optimized fertilization and soil management strategies, which not only enhance ecosystem productivity but also contribute to sustainable land management and ecological restoration in N-limited areas.
5 Conclusion
This study demonstrated that the N preference of plants was influenced by different root mycorrhizal symbiosis types under similar soil N conditions. H. rhamnoides was associated with ACM preference for NO3–. Conversely, P. tabuliformis, which associated with ECM, exhibited the preference for NH4+ due to the high assimilation of NH4+ by extraradical mycelium. In addition, mixed afforestation did not alter the N preference of plants. A. gmelinii preferred to take up NO3–, which is highly mobile. The different N uptake forms preferred by plants and soil microorganisms resulted in a low PS index, indicating that niche complementarity may be a potential mechanism to decrease niche overlap and maintain community stability. Given that mycorrhiza is ubiquitous in majority plants, these findings may provide strong evidence for the importance of the mycorrhizal type in plant N uptake patterns.
Data availability statement
The original contributions presented in the study are included in the article/Supplementary Material. Further inquiries can be directed to the corresponding author.
Author contributions
LW: Conceptualization, Investigation, Methodology, Writing – original draft. XD: Investigation, Methodology, Writing – original draft. YZ: Investigation, Writing – original draft. XG: Investigation, Writing – original draft. ZZ: Investigation, Writing – original draft. YT: Conceptualization, Funding acquisition, Supervision, Writing – review & editing.
Funding
The author(s) declare financial support was received for the research, authorship, and/or publication of this article. This work was supported by the National Natural Science Foundation of China (41977425).
Conflict of interest
The authors declare that the research was conducted in the absence of any commercial or financial relationships that could be construed as a potential conflict of interest.
Publisher’s note
All claims expressed in this article are solely those of the authors and do not necessarily represent those of their affiliated organizations, or those of the publisher, the editors and the reviewers. Any product that may be evaluated in this article, or claim that may be made by its manufacturer, is not guaranteed or endorsed by the publisher.
Supplementary material
The Supplementary Material for this article can be found online at: https://www.frontiersin.org/articles/10.3389/fpls.2024.1480517/full#supplementary-material
References
Ackerman, D., Millet, D. B., Chen, X. (2018). Global estimates of inorganic nitrogen deposition across four decades. Global Biogeochem. Cycles. 33, 100–107. doi: 10.1029/2018GB005990
Andersen, K. M., Mayor, J. R., Turner, B. L. (2017). Plasticity in nitrogen uptake among plant species with contrasting nutrient acquisition strategies in a tropical forest. Ecology 98, 1388–1398. doi: 10.1002/ecy.2017.98.issue-5
Andersen, K. M., Turner, B. L. (2013). Preferences or plasticity in nitrogen acquisition by understorey palms in a tropical montane forest. J. Ecol. 101, 819–825. doi: 10.1111/jec.2013.101.issue-3
Ashton, I. W., Miller, A. E., Bowman, W. D., Suding, K. N. (2010). Niche complementarity due to plasticity in resource use: plant partitioning of chemical N forms. Ecology 91, 3252–3260. doi: 10.1890/09-1849.1
Berger, H., Basheer, A., Böck, S., Reyes–Dominguez, Y., Altmann, F., Strauss, J., et al. (2008). Dissecting individual steps of nitrogen transcription factor cooperation in the Aspergillus Nidulans nitrate cluster. Mol. Microbiol. 69, 1331–1579. doi: 10.1111/j.1365-2958.2008.06359.x
Buchmann, N., Schulze, E. D., Gebauer, G. (1995). 15N-ammonium and 15N-nitrate uptake of a 15–year–old Picea abies plantation. Oecologia 102, 361–370. doi: 10.1007/BF00329803
Castro–Rodríguez, V., Canas, R. A., de la Torre, F. N., Pascual, M. B., Avila, C., Canovas, F. M. (2017). Molecular fundamentals of nitrogen uptake and transport in trees. J. Exp. Bot. 68, 2489–2500. doi: 10.1093/jxb/erx037
Chen, Y., Li, Y. Q., Wang, X. Y., Niu, Y. Y. (2021). Advances in ecological stoichiometry in typically and ecologically vulnerable regions of China. Acta Ecol. Sinica. 41, 4213–4225. doi: 10.5846/stxb202006131540
Cui, J. H., Yu, C. Q., Qiao, N., Xu, X. L., Tian, Y. Q., Ouyang, H. (2017). Plant preference for NH4+ versus NO3– at different growth stages in an alpine agroecosystem. Field Crops Res. 201, 192–199. doi: 10.1016/j.fcr.2016.11.009
Fowler, D., Coyle, M., Skiba, U., Sutton, M. A., Cape, J. N., Reis, S., et al. (2013). The global nitrogen cycle in the twenty–first century. Philos. Trans. R. Soc. London B. 368, 20130164. doi: 10.1098/rstb.2013.0164
Fraterrigo, J. M., Strickland, M. S., Keiser, A. D., Bradford, M. A. (2011). Nitrogen uptake and preference in a forest understory following invasion by an exotic grass. Oecologia 167, 781–791. doi: 10.1007/s00442-011-2030-0
Fu, B., Wang, S., Liu, Y., Liu, J. B., Liang, W., Miao, C. Y. (2016). Hydrogeomorphic ecosystem responses to natural and anthropogenic changes in the loess plateau of China. Annu. Rev. Earth Planet. Sci. 45, 223–243. doi: 10.1146/annurev-earth-063016-020552
Gao, X. D., Li, H. C., Zhao, X. N., Ma, W., Wu, P. T. (2018). Identifying a suitable revegetation technique for soil restoration on water–limited and degraded land: considering both deep soil moisture deficit and soil organic carbon sequestration. Geoderma 319, 61–69. doi: 10.1016/j.geoderma.2018.01.003
Geisseler, D., Horwath, W. R., Joergensen, R. G., Ludwig, B. (2010). Pathways of nitrogen utilization by soil microorganisms–a review. Soil Biol. Biochem. 42, 2058–2067. doi: 10.1016/j.soilbio.2010.08.021
Gruffman, L. D., Jämtgård, S., Näsholm, T. (2014). Plant nitrogen status and cooccurrence of organic and inorganic nitrogen sources influence root uptake by Scots pine seedlings. Tree Physiol. 34, 205–213. doi: 10.1093/treephys/tpt121
Guo, W. J., Zhang, Z. L., Liu, Q., Xiao, J., Yin, H. J. (2020). Seasonal variations in plant nitrogen acquisition in an ectomycorrhizal alpine forest on the eastern Tibetan Plateau, China. Plant Soil. 459, 79–91. doi: 10.1007/s11104-020-04644-8
Gurmesa, G. A., Wang, A., Li, S. L., Peng, S. S., de Vries, W., Gundersen, P., et al. (2022). Retention of deposited ammonium and nitrate and its impact on the global forest carbon sink. Nat. Commun. 13, 880–880. doi: 10.1038/s41467-022-28345-1
Güsewell, S. (2004). N:P ratios in terrestrial plants: variation and functional significance. New Phytol. 164, 243–266. doi: 10.1111/j.1469-8137.2004.01192.x
Harrison, K. A., Bol, R., Bardgett, R. D. (2007). Preferences for different nitrogen forms by coexisting plant species and soil microbes. Ecology 88, 989–999. doi: 10.1890/06-1018
He, X. X., Chi, Q. D., Meng, L., Zhao, C., He, M. Q., Dan, X. Q., et al. (2022). Plants with nitrate preference can regulate nitrification to meet their nitrate demand. Soil Biol. Biochem. 165, 108516. doi: 10.1016/j.soilbio.2021.108516
Hodge, A., Robinson, D., Fitter, A. (2000). Are microorganisms more effective than plants at competing for nitrogen? Trends Plant Sci. 5, 304–308. doi: 10.1016/S1360-1385(00)01656-3
Hoekstra, N. J., Finn, J. A., Hofer, D., Lüscher, A. (2014). The effect of drought and interspecific interactions on depth of water uptake in deep- and shallow-rooting grassland species as determined by δ18O natural abundance. Biogeosciences 11, 4493–4506. doi: 10.5194/bg-11-4493-2014
Hong, J. T., Ma, X. V., Yan, Y., Zhang, X. K., Wang, X. D. (2018). Which root traits determine nitrogen uptake by alpine plant species on the Tibetan Plateau? Plant Soil. 424, 63–72. doi: 10.1007/s11104-017-3434-3
Hu, X. F., Li, W. T., Liu, Q. H., Yin, C. Y. (2021). Interactions between species changes the uptake of ammonium and nitrate in Abies Faxoniana and Picea Asperata. Tree Physiol. 68, 2501–2512. doi: 10.1093/treephys/tpab175
Inselsbacher, E., Umana, H. N., Stange, F. C., Gorfer, M., Schüller, E., Ripka, K., et al. (2009). Short–term competition between crop plants and soil microbes for inorganic N fertilizer. Soil Biol. Biochem. 42, 360–372. doi: 10.1016/j.soilbio.2009.11.019
Javelle, A., Chalot, M., Brun, A., Botton, B. (2008). Nitrogen transport and metabolism in mycorrhizal fungi and mycorrhizas. Springer Berlin Heidelberg. 393–429. doi: 10.1007/978-3-540-74051-3_22
Ji, P. T., Peng, Y. J., Li, X. L., Wang, R., Tao, P. J., Zhang, Y. C. (2024). Polyaspartic acid urea increased maize yield by enhancing leaf N turnover efficiency and soil microbial diversity. Agronomy 14, 796. doi: 10.3390/agronomy14040796
Kahmen, A., Renker, C., Unsicker, S. B., Unsicker, S. B., Buchmann, N. (2006). Niche complementarity for nitrogen: an explanation for the biodiversity and ecosystem functioning relationship? Ecology 87, 1244–1255. doi: 10.1890/0012-9658(2006)87[1244:NCFNAE]2.0.CO;2
Kaštovská, E., Šantrůčková, H. (2011). Comparison of uptake of different N forms by soil microorganisms and two wet–grassland plants: A pot study. Soil Biol. Biochem. 43, 1285–1291. doi: 10.1016/j.soilbio.2011.02.021
Kuzyakov, Y., Xu, X. L. (2013). Competition between roots and microorganisms for nitrogen: mechanisms and ecological relevance. New phytol. 198, 656–669. doi: 10.1111/nph.2013.198.issue-3
Li, C., Li, Q., Qiao, N., Xu, X., Li, Q., Wang, H. (2016). Inorganic and organic nitrogen uptake by nine dominant subtropical tree species. Iforest Biogeosci. Forest. 9, 253–258. doi: 10.3832/ifor1502-008
Li, P. P., Wang, B., Yang, Y. F., Liu, G. B. (2022). Effects of vegetation near-soil-surface factors on runoff and sediment reduction in typical grasslands on the Loess Plateau, China. Journal of Arid Land. J. Arid Land. 14, 325–340. doi: 10.1007/s40333-022-0007-y
Liu, M., Xu, X. L., Yang, B., Zhang, N. L., Ma, Z. Q., Dam, N. M. V., et al. (2022). Niche partitioning in nitrogen uptake among subtropical tree species enhances biomass production. Sci. Total Environ. 823, 153716. doi: 10.1016/j.scitotenv.2022.153716
Liu, Q. Y., Qiao, N., Xu, X. L., Xin, X. P., Han, J. Y., Tian, Y. Q., et al. (2016). Nitrogen acquisition by plants and microorganisms in a temperate grassland. Sci. Rep. 6, 22642. doi: 10.1038/srep22642
Liu, Q. Y., Wang, H. M., Xu, X. L. (2020). Root nitrogen acquisition strategy of trees and understory species in a subtropical pine plantation in southern China. Eur. J. For. Res. 139, 1–14. doi: 10.1007/s10342-020-01284-6
Liu, Q. Y., Xu, M. J., Yuan, Y., Wang, H. M. (2021). Interspecific competition for inorganic nitrogen between canopy trees and underlayer-planted young trees in subtropical pine plantations. For. Ecol. Manage. 494, 119331. doi: 10.1016/j.foreco.2021.119331
Ma, L. N., Xu, X. F., Zhang, C. X., Lv, Y. X., Liu, G. F., Zhang, Q. B., et al. (2021). Strong non-growing season N uptake by deciduous trees in a temperate forest: A 15N isotopic experiment. J. Ecol. 109, 3752–3766. doi: 10.1111/1365-2745.13754
Mao, J. H., Mao, Q. G., Gundersen, P., Gurmesa, G. A., Zhang, W., Huang, J., et al. (2021). Unexpected high retention of 15N–labeled nitrogen in a tropical legume forest under long–term nitrogen enrichment. Global Change Biol. 28, 1529–1543. doi: 10.1111/gcb.16005
Mckane, R. B., Johnson, L. C., Shaver, G. R., Nadelhoffer, K. J., Rastetter, E. B., Fry, B., et al. (2002). Resource–based niches provide a basis for plant species diversity and dominance in arctic tundra. Nature 415, 68–71. doi: 10.1038/415068a
Meng, S., Zhang, C. X., Su, L., Li, Y. M., Zhao, Z. (2016). Nitrogen uptake and metabolism of Populus simonii in response to PEG–induced drought stress. Environ. Exp. Bot. 123, 78–87. doi: 10.1016/j.envexpbot.2015.11.005
Miller, A. E., Bowman, W. D., Suding, K. N. (2007). Plant uptake of inorganic and organic nitrogen: neighbor identity matters. Ecology 88, 1832–1840. doi: 10.1890/06-0946.1
Ning, P., Zhang, M., Bai, T. Y., Zhang, B. (2023). Dendroclimatic response of Pinus tabuliformis Carr. along an altitudinal gradient in the warm temperate region of China. Front. Plant sci. 14, 1147229. doi: 10.3389/fpls.2023.1147229
Pang, Z., Jiang, L. L., Wang, S. P., Xu, X. L., Rui, Y. C., Wang, Y. F. (2019). Differential response to warming of the uptake of nitrogen by plant species in non-degraded and degraded alpine grasslands. J. Soils Sedim. 19, 2212–2221. doi: 10.1007/s11368-019-02255-0
Perkowski, E. A., Waring, E. F., Smith, N. G. (2021). Root mass carbon costs to acquire nitrogen are determined by nitrogen and light availability in two species with different nitrogen acquisition strategies. J. Exp. Bot. 72, 5766–5776. doi: 10.1093/jxb/erab253
Phillips, R. P., Brzostek, E., Midgley, M. G. (2013). The mycorrhizal–associated nutrient economy: a new framework for predicting carbon–nutrient couplings in temperate forests. New Phytol. 199, 41–51. doi: 10.1111/nph.2013.199.issue-1
Phillips, D. L., Gregg, J. W. (2003). Source partitioning using stable isotopes: coping with too many sources. Oecologia 136, 261–269. doi: 10.1007/s00442-003-1218-3
Phillips, J. M., Hayman, D. S. (1970). Improved procedures for clearing roots and staining parasitic and vesicular–arbuscular mycorrhizal fungi for rapid assessment of infection. Trans. Br. Mycol. Soc. 55, 158–161. doi: 10.1016/S0007-1536(70)80110-3
Song, M. H., Xu, X. L., Hu, Q. W., Tian, Y. Q., Ouyang, H., Zhou, C. P. (2007). Interactions of plant species mediated plant competition for inorganic nitrogen with soil microorganisms in an alpine meadow. Plant Soil. 297, 127–137. doi: 10.1007/s11104-007-9326-1
Song, M. Y., Yu, L., Jiang, Y. L., Lei, Y. B., Korpelainen, H., Li, C. Y., et al. (2017). Nitrogen-controlled intra- and interspecific competition between Populus purdomii and Salix rehderiana drive primary succession in the Gongga Mountain glacier retreat area. Tree Physiol. 37, 799–814. doi: 10.1093/treephys/tpx017
Sorrell, K. B., Chague-Goff, C., Basher, L. M., Partridge, T. R. (2011). N:P ratios, delta 15N fractionation and nutrient resorption along a nitrogen to phosphorus limitation gradient in an oligotrophic wetland complex. Aquat. Bot. 94, 93–101. doi: 10.1016/j.aquabot.2010.11.006
Tang, Y. K., Wang, L. N., Zhou, Y., Geng, X. Q., Deng, X., Zhang, Z. L. (2024). Drought decreases symbiotic nitrogen fixation and nitrogen transfer in a Populus tomentosa-Hippophae rhamnoides mixed plantation on the semiarid Loess Plateau, China. For. Ecol. Manage. 558, 121815. doi: 10.1016/j.foreco.2024.121815
Tang, Y. K., Wu, X., Chen, Y. M. (2018b). Sap flow characteristics and physiological adjustments of two dominant tree species in pure and mixed plantations in the semi-arid Loess Plateau of China. J. Arid Land. 10, 833–849. doi: 10.1007/s40333-018-0027-9
Tang, Y. K., Wu, X., Chen, C., Jia, C., Chen, Y. M. (2019). Water source partitioning and nitrogen facilitation promote coexistence of nitrogen–fixing and neighbor species in mixed plantations in the semiarid Loess Plateau. Plant Soil. 445, 289–305. doi: 10.1007/s11104-019-04301-9
Tang, Y. K., Wu, X., Chen, Y. M., Wen, J., Xie, Y. L., Lu, S. B. (2018a). Water use strategies for two dominant tree species in pure and mixed plantations of the semiarid Chinese Loess Plateau. Ecohydrology 11, e1943. doi: 10.1002/eco.v11.4
Templer, P. H., Dawson, T. E. (2004). Nitrogen uptake by four tree species of the catskill mountains, New York: implications for forest n dynamics. Plant Soil. 262, 251–261. doi: 10.1023/B:PLSO.0000037047.16616.98
Uscola, M., Villar–Salvador, P., Oliet, J., Warren, C. R. (2017). Root uptake of inorganic and organic N chemical forms in two coexisting Mediterranean forest trees. Plant Soil. 415, 387–392. doi: 10.1007/s11104-017-3172-6
Van den Berg, L. J. L., Jones, L., Sheppard, L. J., Smart, S. M., Bobbink, R., Dise, N. B. (2016). Evidence for differential effects of reduced and oxidised nitrogen deposition on vegetation independent of nitrogen load. Environ. Pollut. 208, 890–897. doi: 10.1016/j.envpol.2015.09.017
Vierheilig, H., Coughlan, A. P., Wyss, U., Piché, Y. (1998). Ink and vinegar, a simple staining technique for arbuscular–mycorrhizal fungi. Appl. Environ. Microbiol. 64, 5004–5007. doi: 10.1128/AEM.64.12.5004-5007.1998
Wang, X. Y., Gao, Y. Z., Zhang, H. L., Gao, Q. (2020). Enhancement of rhizosphere citric acid and decrease of NO3–/NH4+ ratio by root interactions facilitate N fixation and transfer. Plant and Soil. Int. J. Plant-Soil Relation. 447, 169–182. doi: 10.1007/s11104-018-03918-6
Wang, L. X., Macko, S. A. (2011). Constrained preferences in nitrogen uptake across plant species and environments. Plant Cell Environ. 34, 525–534. doi: 10.1111/j.1365-3040.2010.02260.x
Wang, B., Xue, S., Liu, B. G., Zhang, G. H., Li, G., Ren, Z. P. (2012). Changes in soil nutrient and enzyme activities under different vegetations in the Loess Plateau area, Northwest China. Catena 92, 186–195. doi: 10.1016/j.catena.2011.12.004
Wang, Z., Zhao, F. L., Wei, P. P., Chai, X. Y. (2022). Phytochemistry, health benefits, and food applications of sea buckthorn (Hippophae rhamnoides L.): A comprehensive review. Front. Nutr. 9, 1036295. doi: 10.3389/fnut.2022.1036295
Xie, K., Ren, Y. H., Chen, A., Yang, C. F., Zheng, Q. S., Chen, J., et al. (2022). Plant nitrogen nutrition: The roles of arbuscular mycorrhizal fungi. J. Plant Physiol. 269, 15391. doi: 10.1016/j.jplph.2021.153591
Xiong, J. L., Dong, L. W., Lu, J. L., Hu, W. G., Gong, H. Y., Xie, S. B., et al. (2021). Variation in plant carbon, nitrogen and phosphorus contents across the drylands of China. Funct. Ecol. 36, 174–186. doi: 10.1111/1365-2435.13937
Xu, Y. Q., Li, L. H., Wang, Q. B., Chen, Q. S., Cheng, W. X. (2007). The pattern between nitrogen mineralization and grazing intensities in an inner Mongolian typical steppe. Plant Soil. 300, 289–300. doi: 10.1007/s11104-007-9416-0
Yan, B. S., Duan, M. C., Wang, R. C., Li, J. J., Wei, F. R., Chen, J. R., et al. (2022). Planted forests intensified soil microbial metabolic nitrogen and phosphorus limitation on the Loess Plateau, China. Catena 211, 105982. doi: 10.1016/j.catena.2021.105982
Yu, G. R., Jia, Y. L., He, N. P., Zhu, J. X., Chen, Z., Wang, Q. F., et al. (2019). Stabilization of atmospheric nitrogen deposition in China over the past decade. Nat. Geosci. 12, 424–429. doi: 10.1038/s41561-019-0352-4
Yuan, Y., Wang, H., Dai, X. (2024). Aboveground and belowground biogeochemical niche separation between woody and herbaceous species explains their coexistence in subtropical plantations. Plant Soil. 501, 669–681. doi: 10.1007/s11104-024-06546-5
Zhang, J., Cai, Z., Müller, C. (2018). Terrestrial N cycling associated with climate and plant-specific N preferences: a review. Eur. J. Soil Sci. 69, 488–501. doi: 10.1111/ejss.2018.69.issue-3
Zhang, H. Q., Yu, H. X., Tang, M. (2017). Prior contact of Pinus tabulaeformis with ectomycorrhizal fungi increases plant growth and survival from damping-off. New Forest. 48, 855–866. doi: 10.1007/s11056-017-9601-9
Zhang, Z. L., Yuan, Y. S., Liu, Q., Yin, H. J. (2019). Plant nitrogen acquisition from inorganic and organic sources via root and mycelia pathways in ectomycorrhizal alpine forests. Soil Biol. Biochem. 136, 107517–107517. doi: 10.1016/j.soilbio.2019.06.013
Zhou, X. L., Wang, A., Hobbie, E. A., Zhu, F. F., Qu, Y. Y., Dai, L. M., et al. (2020). Mature conifers assimilate nitrate as efficiently as ammonium from soils in four forest plantations. New Phytol. 229, 3184–3194. doi: 10.1111/nph.v229.6
Zhu, F. F., Dai, L. M., Hobbie, E. A., Koba, K., Liu, X. Y., Gurmesa, G. A., et al. (2019). Uptake patterns of glycine, ammonium, and nitrate differ among four common tree species of Northeast China. Front. Plant Sci. 10, 799. doi: 10.3389/fpls.2019.00799
Keywords: forest–grassland transition zone, microorganisms, niche complementarity, 15N tracer, nitrogen uptake, plants
Citation: Wang L, Deng X, Zhou Y, Geng X, Zhang Z and Tang Y (2025) Different nitrogen uptake patterns of plant and soil microorganisms in the forest-grassland transition zone on the Loess Plateau. Front. Plant Sci. 15:1480517. doi: 10.3389/fpls.2024.1480517
Received: 14 August 2024; Accepted: 26 December 2024;
Published: 21 January 2025.
Edited by:
Anoop Kumar Srivastava, Central Citrus Research Institute (ICAR), IndiaReviewed by:
Kailou Liu, Jiangxi Institute of Red Soil, ChinaXianzhen Luo, Chinese Academy of Sciences (CAS), China
Copyright © 2025 Wang, Deng, Zhou, Geng, Zhang and Tang. This is an open-access article distributed under the terms of the Creative Commons Attribution License (CC BY). The use, distribution or reproduction in other forums is permitted, provided the original author(s) and the copyright owner(s) are credited and that the original publication in this journal is cited, in accordance with accepted academic practice. No use, distribution or reproduction is permitted which does not comply with these terms.
*Correspondence: Yakun Tang, eWt0YW5nQG53YWZ1LmVkdS5jbg==