- 1Entomology Section, Council for Scientific and Industrial Research, Savanna Agricultural Research Institute, Tamale, Ghana
- 2Intitute for Agricultural Research, Ahmadu Bello University, Zaria, Nigeria
- 3Council for Scientific and Industrial Research, Savanna Agricultural Research Institute, Tamale, Ghana
- 4Commonwealth Scientific and Industrial Research Organisation, Agriculture and Food, Canberra, ACT, Australia
- 5Institute for International Crop Improvement, Donald Danforth Plant Science Center, St. Louis, MO, United States
Introduction: The cowpea weevil, Callosobruchus maculatus Fab., is the most economically important storage pest of cowpeas, causing up to 100 percent grain losses within six months of storage. To sustainably resist weevil damage, the cowpea cultivar IT86D-1010 was genetically modified via Agrobacterium-mediated transformation to produce event CSI-32, which expresses the kidney bean alpha-amylase inhibitor 1 (αAI-1) protein exclusively in the seed, providing suppression of weevil development.
Methods: Trials were conducted in Ghana and Nigeria during the 2023 growing season to assess the performance in the field and in post-harvest storage of this transgenic event (CSI-32) and of four check lines: the non-transformed parental line (IT86D-1010) and three released varieties (SAMPEA 7, SAMPEA 20-T and IT13K-1070-2). Data collected from the field trials comprised plant growth parameters, pest infestations and damage, and grain yield. Harvested grain from each replicated entry was used in a storage assessment of bruchid resistance following artificial infestation with laboratory-reared cowpea weevils. Data were collected on egg oviposition, adult emergence, and grain damage as well as computation of median development period and Dobie’s susceptibility index for each entry.
Results and discussion: The agronomic performance and phenotypic characteristics of event CSI-32 were very similar to its parental counterpart and the other compared varieties. However, event CSI-32 exhibited complete suppression of weevil emergence and resistance to seed damage over the four-month period of the post-harvest study.
Conclusions: This work represents the first field study of genetically modified cowpea expressing the αAI-1 protein. It demonstrates how a biotechnology solution to mitigate significant economic losses during cowpea storage, offers great potential for cowpea improvement programs.
1 Introduction
Cowpea, Vigna unguiculata (L.) Walp., is a drought-tolerant and warm weather leguminous crop that is well adapted to the drier regions of the tropics (Singh, 2005). The grains of this legume are widely consumed and serve as an essential source of plant protein to millions of people in sub-Saharan Africa (SSA) and other parts of the world (Bolarinwa et al., 2022; Ige et al., 2011; Nwagboso et al., 2024). The grains are severely damaged during storage by a bruchid, the cowpea weevil, Callosobruchus maculatus Fab. (Coleoptera: Chrysomelidae) (Hajam and Kumar, 2022; Devi and Devi, 2014). Infestation starts on the field when females get into damaged or shattered pods and lay their eggs on the seeds. Under favourable environmental conditions in storage, these eggs hatch and the weevil population builds up rapidly leading to both qualitative and quantitative grain losses (Singh et al., 1985; Kpoviessi et al., 2019; Torres et al., 2016). Weevil damage is manifested as reductions in seed weight and germination ability, reduced nutritional value, and adverse effects on the appearance of cowpeas, rendering them unfit for consumption and commercialization (Torres et al., 2016). In general, losses due to cowpea weevil infestation can be as high as 100 percent within six months of storage of untreated cowpea (Mkenda et al., 2014).
To effectively mitigate losses due to this pest, the use of hermetic technology, such as the Purdue Improved Crop Storage (PICS) bags, has proved to be an environmentally-friendly strategy (da Silva et al., 2018). Thermal disinfection has also been reported to be effective at managing eggs, larvae, pupae, and adults of this insect although the different stages require different temperatures and different times of exposure (Loganathan et al., 2011). Other environmentally benign strategies include the use of botanicals (Eugenia aromatica (Baill.), Cymbopogon citratus (lemon grass), Citrus sinensis (orange peel) and Azadirachta indica (neem) (Ofuya et al., 2010; Ojebode et al., 2016; Kusuma et al., 2014), Spinosad sprays (Sanon et al., 2010), vegetable oils (Khan, 2011), and microbial pesticide formulations containing Bacillus thuringiensis (Malaikozhundan and Vinodhini, 2018). These management approaches, however, are not widely adopted by resource-poor farmers and others in the cowpea value chain because of limited access to these protection agents and the high cost of some of these management options. For botanical extracts, the labour associated with their preparations often limit their adoption in SSA, while the price of those industrially prepared and marketed ones are prohibitive.
Hence. most farmers, grain merchants and seed producers rely on synthetic insecticides, such as carbamates (propoxur), organochlorines (lindane), organophosphates (e.g., acephate, diazinon, dichlorvos, dursban, malathion, pirimiphos-methyl), synthetic pyrethroids (permethrin), phosphine and phostoxin, methyl bromide, and iodofenphos, to mitigate losses due to the cowpea weevil (Ekoja et al., 2021; Hajam and Kumar, 2022). Insecticide misuse to control bruchids can be the main cause of harmful pesticide residues often found in marketed cowpea grains (Olufade et al., 2014; Olutona and Aderemi, 2019). Residues of these insecticides in the grains, and the development of insect resistance to the active ingredients in their formulations are major concerns. There is therefore a need to increase the quality of stored cowpea by exploring more effective, economic, and environmentally friendly cowpea weevil management alternatives. The development of varieties with resistance to infestation and damage by the cowpea weevil is the most viable cost-effective method of overcoming this damaging storage pest and increasing access to the grains of this nutritious legume (Singh et al., 1985; Kpoviessi et al., 2019; Nyarko et al., 2022). However, to date there is no known conventionally bred cowpea variety with an adequate level of protection from infestation and damage by this weevil. Only a few varieties with modest resistance under low insect pressure have been identified, and this is usually influenced by physical seed attributes such as surface area, smoothness, and curvature (Adam and Baidoo, 2008; Ajayi and Lale, 2001; Musa and Adeboye, 2017), as well as chemical traits such as production of trypsin, arcelin, and p-aminophenylalanine (Amusa et al., 2014; Badii et al., 2013; Onigbinde and Oigiangbe, 1996; Tengey et al., 2023).
In the absence of adequate sources of resistance in conventional breeding programs, biotechnological approaches are needed to introduce effective and durable protection from cowpea weevil infestation and damage. Starch digestion involves the breakdown by α-amylase to small linear and branched malto-oligosaccharides, which are in turn hydrolyzed to glucose by α-glucosidases. Several insects, especially bruchids that feed on starchy seeds during larval stages, depend on their α-amylases for survival. The α-amylase inhibitor 1 (αAI-1) protein produced in the common bean (Phaseolus vulgaris) binds irreversibly to the enzyme active site and inhibits the activity of porcine, human, and insect α-amylases (Altabella and Chrispeels, 1990) and is thus toxic to bruchids in adzuki beans (Ishimoto et al., 1996), peas (Schroeder et al., 1995; Shade et al., 1994), chickpeas (Sarmah et al., 2004), and cowpeas (Solleti et al., 2008; Lüthi et al., 2013). Cowpea line IT86D-1010 was genetically modified via Agrobacterium-mediated transformation to create transgenic event CSI-32, which expresses the kidney bean αAI-1 protein exclusively in the seed as a means of controlling post-harvest damage due to the bruchid weevil.
The current study evaluated the phenotypic characteristics and post-harvest resistance to weevil damage of CSI-32 cowpea in comparison with its non-transformed parental line, IT86D-1010, and three released cowpea cultivars, including SAMPEA 7, SAMPEA 20-T and IT13K-1070-2. The purpose of the field phase was to assess whether there were any adverse effects on agronomic performance or plant phenotype arising from the genetic modification resulting in event CSI-32, while the storage study on harvested grain was intended to evaluate the efficacy of the introduced weevil resistance trait.
2 Materials and methods
2.1 Plant materials
Event CSI-32 was produced as described by Higgins et al. (2012) at the Commonwealth Scientific and Industrial Research Organisation (CSIRO) in 2006. This was done by transforming cultivar IT86D-1010 with a binary vector carrying the kidney bean αAI-1 gene under the control of the seed-specific phytohemagglutinin PHA-L (dlec2) promoter (Higgins et al., 2012). Homozygous T10 seeds were multiplied in Canberra, Australia, in 2023 and sent to Ghana and Nigeria for the field trials. The check lines used in these trials were the non-transformed parental line, IT86D-1010, which is derived as a cross between Tvx4659-03E × IT82E-60. The other lines were SAMPEA 20-T (IT97K-499-35 containing transformation event AAT-709AA-4), IT13K-1070-2 (moderately resistant to bruchids), and SAMPEA 7 (highly susceptible to bruchids). Detailed descriptions of the plant material are presented in Table 1. Apart from IT86D-1010, which was obtained from CSIRO, the other lines were sourced from the Institute for Agricultural Research (IAR), Ahmadu Bello University (ABU) Zaria, Nigeria.
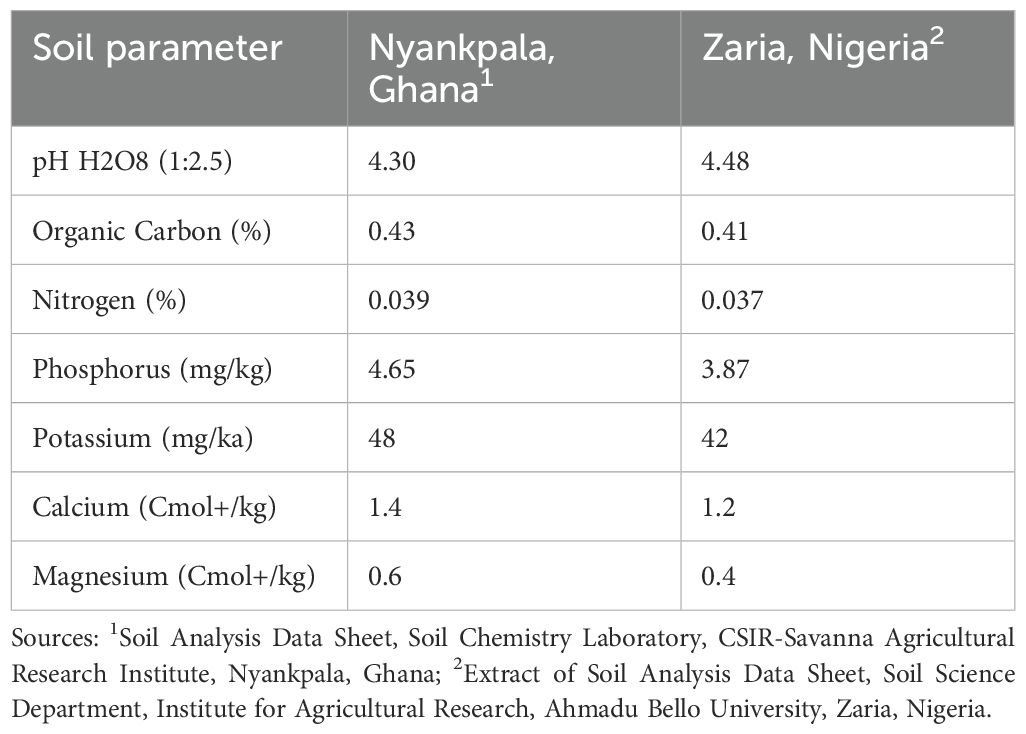
Table 1. Summary of the chemical properties of soils at the confined field trial sites at Nyankpala, Ghana and Zaria, Nigeria.
2.2 Field studies
2.2.1 Ecology of the study areas
Regulated confined field trials (CFTs) were conducted at the authorized CFT sites at CSIR-SARI, Nyankpala, and IAR, Zaria (Figure 1). The CFT sites at Nyankpala and Zaria are classified as “Aw” (tropical wet and dry or savanna) climate under the Köppen-Geiger climate classification (Geiger, 1961). Both sites are characterized by unimodal rainfall pattern with the rainfall in Nyankpala commencing in April and ending in October, while that of Zaria starts in June and ends in October. The mean annual rainfall at Nyankpala ranges between 1000 mm and 1200 mm while that of Zaria ranges from 1200 mm to 1600 mm. The mean annual temperature during the growing season ranges from 23°C to 35°C for Nyankpala and 20°C to 36°C for Zaria.
The soil at Nyankpala is sandy loam and it developed from the Voltaian sandstones known as the Nyankpala series (Serno and van de Weg, 1985) (see Table 1). In contrast, Zaria soils are leached ferruginous tropical soils with high clay content and overlying drift materials, classified as Typic Haplustalf or Alfisol in the United States Department of Agriculture system (Baillie, 2001; Bekele and Birhan, 2021) (see Table 1).
2.2.2 Trial layout and management
At both trial sites, the fields were tractor-ploughed followed by harrowing to obtain a fine soil tilth. The prepared fields were laid out as randomized complete block design (RCBD) after which ridges were manually constructed on each plot at a spacing of 75 cm. The cowpea entries (Table 2) were then randomly assigned to the plots in each block. There were six blocks of each entry. At Nyankpala, plots comprised five rows of cowpea planted on ridges that were four meters long, while at Zaria, plots consisted of four rows of cowpea planted on ridges that were five meters long. The intra-row spacing was 20 cm and there were two plants per hill. Plots within a block were separated by 1.5 m wide alleys while blocks were two meters apart.
A starter dose of NPK (15:15:15) fertilizer was applied at one week after planting at a rate of 40 kg/ha (Table 1). Weed control was conducted manually at two weeks after planting and at podding. At the early vegetative growth stage, a single round of insecticide [Lambda-Cyhalothrin (15g/l) + Acetamiprid (20g/l)] spray was applied on 6th August to manage aphid infestation in Ghana. In contrast, a systemic and contact fungicide (Carbendazim 12% + Mancozeb 63% W.P.) spray was applied on 15th August to mitigate foliar disease infection at the vegetative growth stage in Nigeria. Afterwards, insecticide sprays were applied weekly from flower bud initiation until pod filling using Lamsate® (Lambda-Cyhalothrin 15g/l + Dimethoate 300 g/l) at a rate of 0.5 l/ha (Table 3). These insecticide sprays targeted vegetative stage (Bemisia tabaci Genn, Empoasca, Aphis craccivora Koch), flowering (Megalurothrips sjostedti Try., Maruca vitrata F.) and podding stage pests such as the pod-sucking bugs complex (Clavigralla tomentosicollis Stal, Anoplocnemis curvipes (F.), Riptortus dentipes (F.), Nezara viridula L., Thyanta custator (F.). and Aspavia armigera L.).
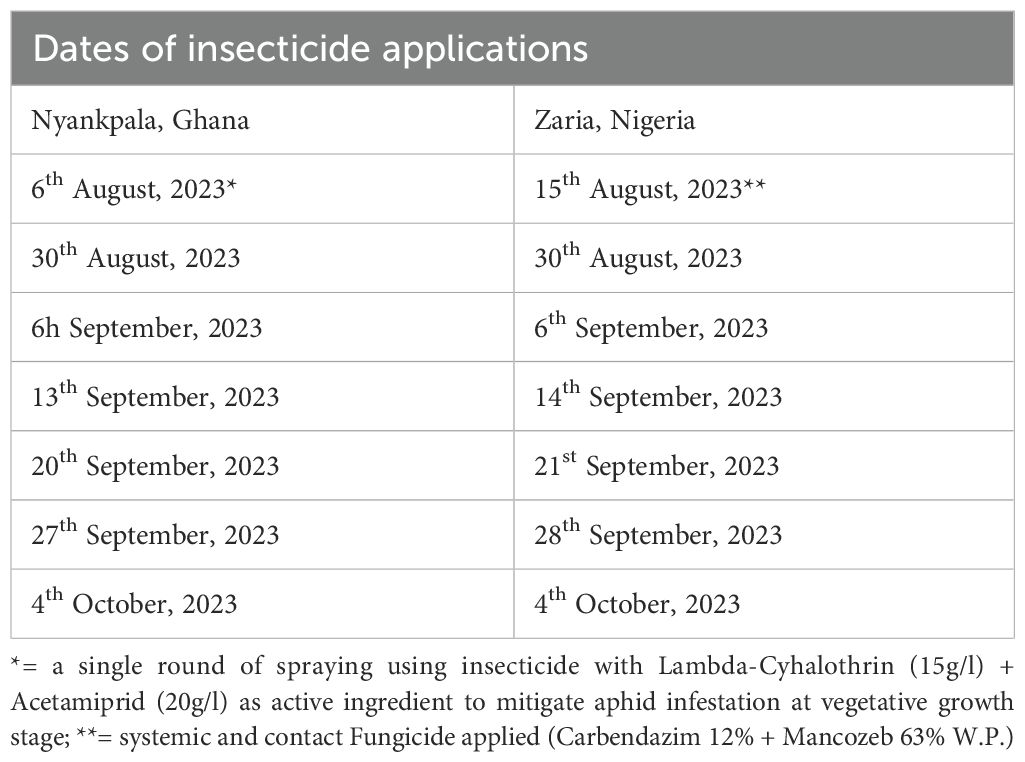
Table 3. Dates of application of insecticide with active ingredients comprising Lambda-Cyhalothrin (15g/l) and Dimethoate (300 g/l) at the confined field trial sites at Nyankpala, Ghana and Zaria, Nigeria.
2.2.3 Data collection and statistical analysis
Agronomic and phenotypic characteristics (Table 4) were recorded for each cowpea entry within all blocks at each site and were subjected to linear mixed model analysis using R, including the “lmerTest” package (Bates, 2014). The mean sample size for each agronomic parameter was five (5) per trial location and a total of 10 for the two locations. For a given agronomic parameter, data were analyzed using the following linear mixed model:
Where μi denotes the mean of the ith entry (fixed effect), lj denotes the effect of the jth site (fixed effect), rk(j) denotes the effect of the kth block within the jth site (random effect), (μl)ij denotes the interaction between the entries and sites, and ϵijk denotes the effect of the plot assigned the ith entry in the kth block of the jth site (random effect or residual) (Bates, 2014).
The “lmer” procedure from the “lmerTest” package was used to fit the linear mixed model and to generate estimates of variance components and p-values. For each agronomic parameter, the least square (LS) mean value across sites was estimated from the corresponding statistical model for each entry using the “emmeans” package (Lenth, 2013) where there was no significant site effect.
2.3 Post-harvest storage studies
2.3.1 Laboratory rearing of weevils
Cowpea weevils were reared at the Entomology Laboratories of CSIR–SARI and IAR using the methodology described by Swella and Mushobozy (2007). The cowpea seeds used for the rearing were obtained from the Cowpea Improvement Programmes of CSIR–SARI and IAR. These were prepared by freezing at -4°C for 48 h followed by oven drying at 60°C for 24 h. A total of 500 g of the resulting seed were weighed into individual clean Kilner jars followed by infesting the seed with 50 unsexed adult weevils. These adult weevils were obtained from weevil colonies maintained by the research institutes. After seven days, the adults were sieved out and the jars containing cowpeas with eggs were incubated at a temperature of 27 ± 3°C and relative humidity (RH) of 50–70%. At approximately 21 days after infestation (DAI), the newly emerged cowpea weevil cohorts were sieved out and used to infest seed samples for the trial. The peak of female egg laying occurs about 3–4 days after emergence begins and thus, all insects used for the tests were not older than 48 hr.
2.3.2 Storage trial establishment and management
The methodology described by Tengey et al. (2023) with slight modifications was used in this study. Briefly, a total of 250 g of harvested seed from each field trial plot was sterilized by freezing at -4°C for 48 h followed by oven drying at 60°C for 24 h. The oven-dried samples were allowed to cool overnight and then sorted to remove insect and mechanically damaged grains, as well as seeds that had egg deposits on them. Afterwards, 200 g of the clean seed for each test entry were weighed into individual 500 ml Kilner jars. Each jar was labelled with information on the identity of its test entry, plot number, and the field trial block from which it was collected. A total of 100 randomly selected seeds were removed from each jar and weighed to obtain the initial 100-seed weight for each entry. These were returned back to the jars after which 10 randomly selected seeds of CSI-32 were removed from jars of each plot and placed in labelled plastic bags for quantitative analysis of αAI-1 content.
The jars were then infested with 50 adult cowpea weevils (25 males and 25 females) and covered with clean cheesecloth and then tightened with a perforated lid to allow for ventilation. They were then arranged in a completely randomized design (CRD) on a laboratory bench in the trial rooms at the testing Entomology Laboratories. The temperature and RH in these trial rooms were maintained at 27 ± 3°C and 50–70%, respectively.
2.3.3 Data collection and statistical analysis
The storage trial was conducted for a period of 120 days, sufficient for the emergence of four filial generations (F1 through F4) of emerged weevils, during which time the following data were collected and/or computed:
2.3.3.1 Number of eggs per 100 seeds
On the 8th DAI, the adult weevils were sieved out with the aid of a fine-mesh sieve followed by randomly selecting 100 seeds from each entry and counting the number of eggs per seed as described by Lambert et al. (1985). All grains were placed back into their respective jars afterwards, and jars were then returned to the storage trial room.
2.3.3.2 Adult emergence
From the 12th DAI onwards, the experimental setups were examined daily and the days to first adult emergence (DFE) were recorded. Afterwards, the number of weevils emerging in each jar were recorded daily until the nth day after first emergence when there was no change in the numbers counted (i.e., emergence was completed), which marked the end of a generation (F). This process was repeated until completion of the F4 generation. To count weevils, each experimental unit was sieved with a fine mesh sieve into a large container and the sieved weevils were frozen for three to five minutes to immobilize them for easy counting. The immobilized weevils were divided into approximately 10 equal portions and counted three times, after which they were placed back into their respective jars. The mean values were added to obtain the total weevil population.
2.3.3.3 Seed weight loss and percent seed damage
Seed weight loss was measured at the end of the F4 generation by randomly selecting 100 seeds from each entry and recording the weight in comparison to the initial weight measured at the start of the trial. The percent weight loss was calculated using the formula:
At the end of each filial generation, the seeds from each entry were sorted into damaged and undamaged seed, followed by counting the number in each category. Damaged seeds were those that had at least one exit hole on the seed surface while undamaged ones had no exit hole. The percent seed damage was calculated as:
2.3.3.4 Median development period
The MDP is the number of days taken for 50% of the adults to emerge, was calculated using the following formula (Howe, 1971; Nyarko et al., 2022);
Where d1 = day at which adults started emerging and a1 = number of adults emerged on d1th day, and so on until the end of adult emergence (dnan).
2.3.3.5 Dobie susceptibility index
The DSI (Dobie, 1974; Dobie and Kilminster, 1978) was calculated for each genotype using the number of adults that emerged at the end of the F1 generation and the MDP using the equation:
The DSI was used in categorizing the cowpea genotypes into resistant or susceptible classes, where 1−5 = resistant; 6−10 = moderately resistant; 11−15 = susceptible; and >16 = highly susceptible (Chakraborty et al., 2015).
Data on adult emergence, percent seed damage, and computed parameters MDP and DSI, were subjected to linear mixed model analysis as previously described (section 2.2.3).
2.3.4 Quantification of αAI-1 content in event CSI-32 seed
Concentrations of αAI-1 protein in seed samples from each replicated plot of event CSI-32 grown at Nyankpala were determined by enzyme linked immunosorbent assay (ELISA) using a mouse monoclonal antibody (MAb) produced against a synthetic peptide sequence (NDIKSVPWDVHDYDG) derived from the β-chain of αAI-1. Seed samples were ground to a crude powder with a mortar and pestle and then homogenized with 50 mM sodium carbonate pH 9.6 at a ratio of 100 mg/ml using a bead mill homogenizer. The crude homogenate was centrifuged (14,000 rpm × 4 min), diluted 1:10,000 with carbonate buffer, and 100 μl aliquots were dispensed into EIA microtiter plate wells (Nunc, MaxiSorp®, Thermo Fisher) and incubated overnight at 4°C. For calibration, αAI-1 was purified from CSI-32 seed essentially as described by Marshall and Lauda (1975) and 100 μl volumes of a dilution series ranging from 1–250 ng/ml were dispensed into microtiter plate wells to construct a standard curve. Following plate washing with TBST (Tris buffered saline, 0.05% Tween 20, pH 7.6) and blocking (TBST + 1% bovine serum albumin), bound αAI-1 protein was detected using horseradish peroxidase (HRP)-conjugated MAb DDP2-MM05 followed by substrate development using 1-Step™ Ultra TMB substrate (Thermo Scientific) and measurement of the absorbance at 660 nm.
3 Results
3.1 Agronomic and phenotypic characteristics
In the combined-sites analysis, there were small but statistically significant differences between many of the entries for most of the agronomic and phenotypic parameters (Table 5). Not surprisingly, entries with different germplasm backgrounds exhibited differences in parameters associated with growth and development (e.g., days to flowering and to first pod of maximum length, plant height, pods per plant, and pod length). Insect damage by pod borers and sucking insects was generally similar across the different entries, except for SAMPEA 20-T, which showed significantly reduced numbers of Maruca damaged pods and seed. However, a statistically significant increase in sucking insect damaged pods was observed for SAMPEA 20-T relative to the other entries. Except for IT13K-1070-2, which showed higher yield, the grain yields among the remaining four entries were not significantly different.
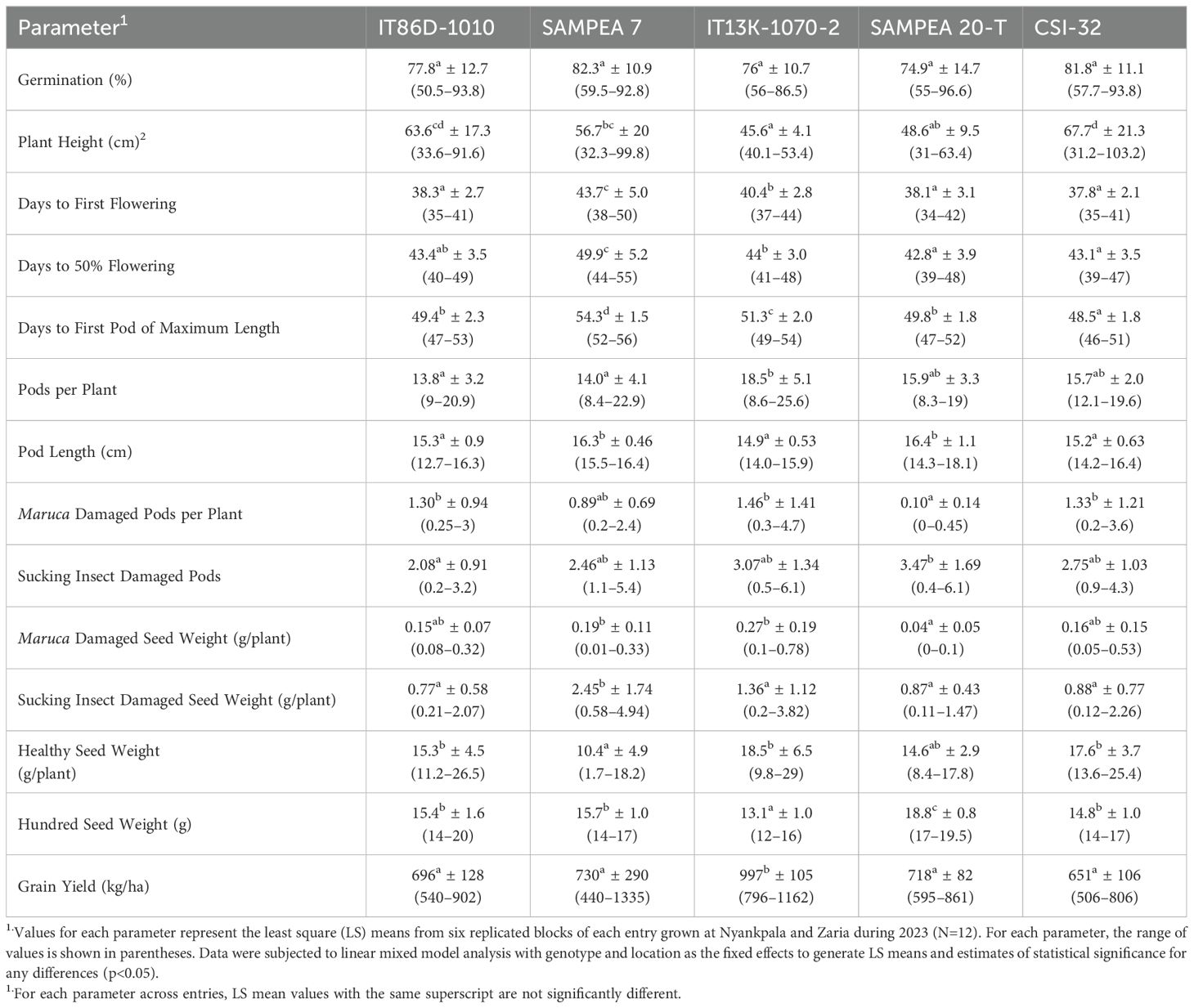
Table 5. Combined-sites analysis of phenotypic data for all entries grown at Nyankpala and Zaria during 2023.
In direct comparisons between event CSI-32 and its non-transformed conventional counterpart, IT86D-1010, the only statistically significant differences were the number of days to first pod of maximum length (FPML), which was approximately one day shorter for CSI-32 cowpea, and the number of sucking insect damaged pods per plant, which were 2.75 for event CSI-32 and 2.08 for the control line (Table 6). There were no differences noted between event CSI-32 and control IT86D-1010 cowpea with respect to plant vigour at either trial site, and at both locations, the transgenic and control entries exhibited normal growth and development with no significant differences in grain yield.
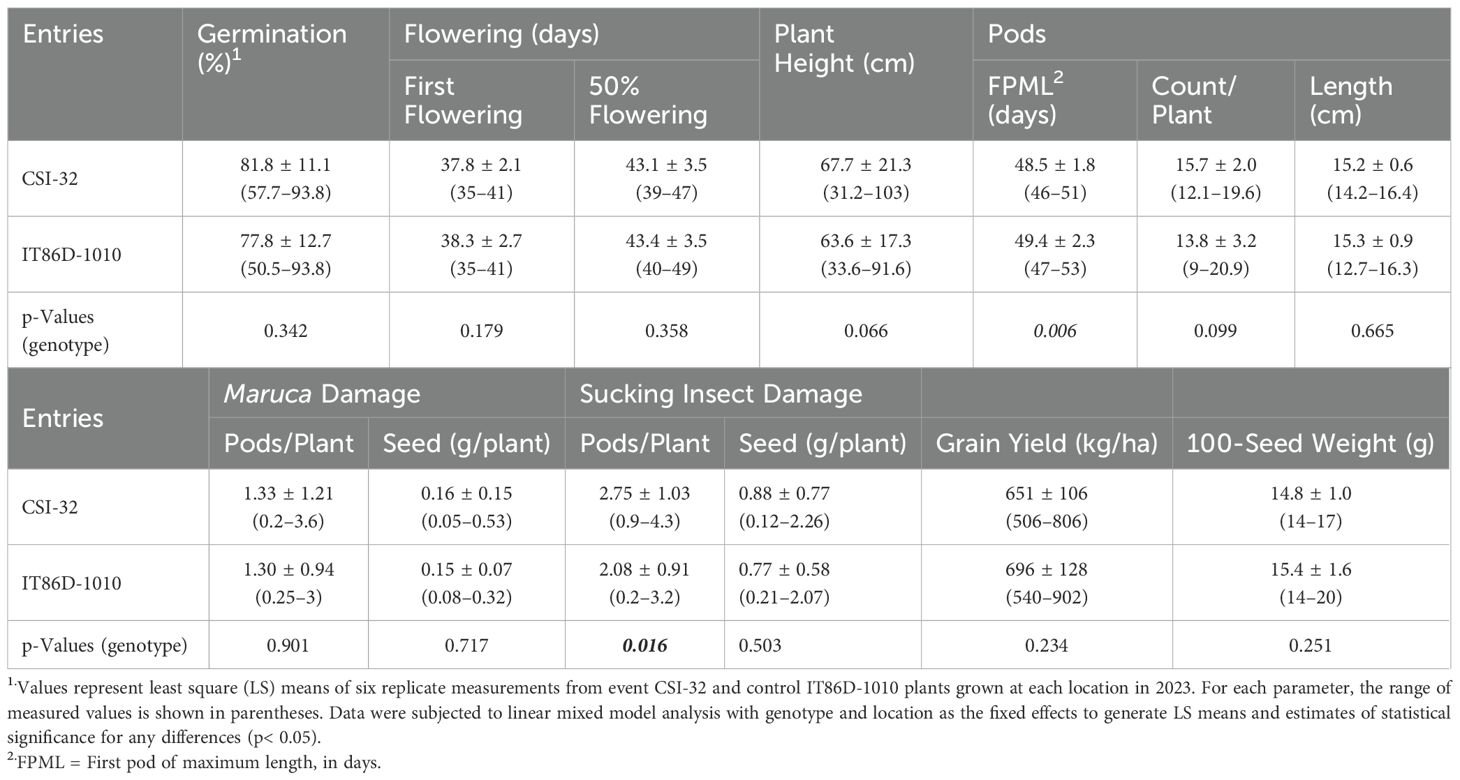
Table 6. Combined sites analysis of phenotypic data for CSI-32 and control cowpea grown at Nyankpala and Zaria during 2023.
3.2 Post-harvest storage trial
Bruchid emergence data were collected over four filial generations of cowpea weevils that were observed for every entry except event CSI-32. As evidenced from the combined sites analysis of F1 generation data (Table 7), there were no significant differences between IT86D-1010, SAMPEA 7, SAMPEA 20-T, or IT13K-1070-2 in days to first emergence (p=0.065), number of emerged adults (p=0.413), Dobie’s susceptibility index (p=0.651), and the percentage of damaged seed (p=0.434). While there were small, statistically significant differences between the four susceptible entries in median development period (p=0.009), the maximum difference between mean MDP values was only 0.5 days (ca. 2%). In the combined sites analysis, all four susceptible varieties were classified as highly susceptible based on mean DSI values >20 (Table 7).
Event CSI-32, with an average αAI-1 protein concentration of 21.0 ± 2.8 μg/mg in the seed as measured by quantitative ELISA of samples at Nyankpala, exhibited complete suppression of adult weevil emergence and absence of seed damage for the four-month duration of the study (120 days post initial infestation).
The days to first emergence increased with each generation (Figure 2A). The days to first emergence for the F4 generation was approximately 12 days later than for the F1 generation and this was not significantly different between the four susceptible cowpea varieties (p=0.391). The number of emerged adults was greatest for the F2 generation (Figure 2B) and declined in subsequent generations. The percentage of damaged seed increased with each generation of emerged weevils (Figure 2C). After the F4 generation, the percentage of damaged seeds was 43.4 percent, on average, with no significant differences between susceptible varieties (p=0.117).
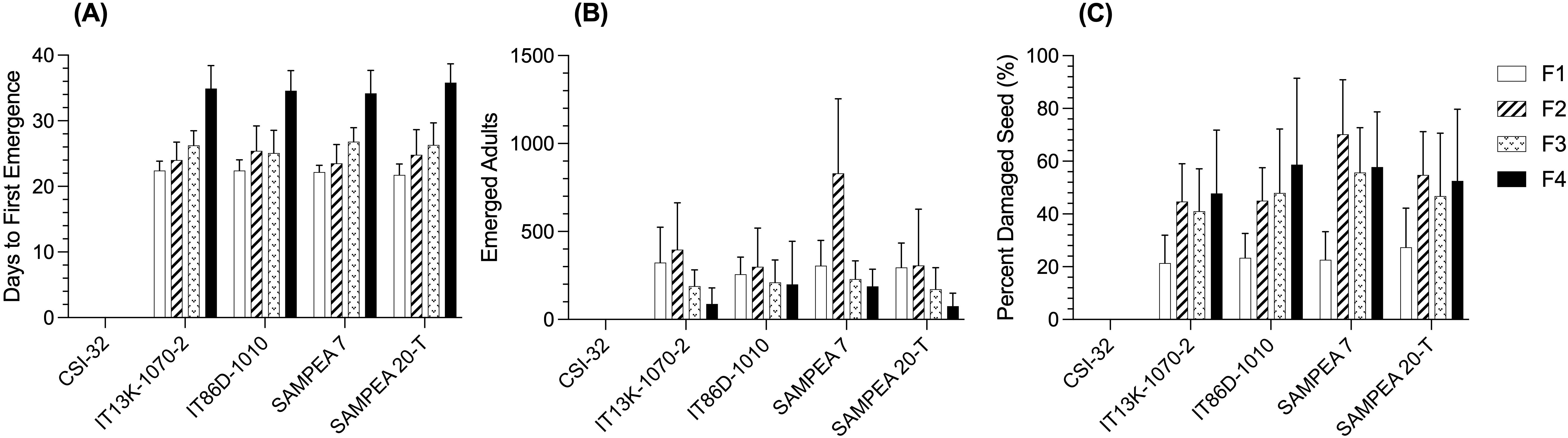
Figure 2. Comparisons of days to first emergence (A), numbers of emerged adults (B), and percent damaged seed (C) across the four filial generations (F1–F4) of emerged weevils for each of the test entries, CSI-32, IT13K-1070-2, IT86D-1010, SAMPEA 7, and SAMPEA 20-T. Bars represent least square (LS) mean values of six replicate samples from each location (N=12) and error bars are standard deviations. In the combined sites analysis, data were subjected to linear mixed model analysis with genotype and location as the fixed effects.
4 Discussion
Genetic variability among cowpea genotypes is crucial for the efficiency of cowpea improvements (Gerrano et al., 2015). These genetic differences account for observed variations in traits like plant height, flowering time, seeds per pod, productive branches, and seed yield (Shimelis and Shiringani, 2010). Therefore, the agronomic and phenotypic differences seen in the studied genotypes are expected. Similar variations in phenotypic traits among genetically diverse cowpea varieties are well documented (Animasaun et al., 2015; Agyeman et al., 2015; Belay et al., 2017; Nkoana et al., 2019).
The transgenic event, CSI-32, and its near-isogenic conventional comparator, IT86D-1010 exhibited normal growth and development when phenotypic parameters, including plant vigor were measured. The only difference detected between line CSI-32 and the control IT86D-1010 was the resistance to bruchid damage, which was the goal for the introduced trait. The findings suggest that the introduced αAI-1 gene and production of high levels of αAI-1 protein in the seed of transgenic event CSI-32 did not give rise to unintended phenotypic effects. Similar reports of a lack of significant difference in phenotypic characters between the transgenic Cry1Ab-expressing event, SAMPEA 20-T, and control cowpea entries were reported by Addae et al. (2020).
Damage to cowpea by the pod-sucking bug (PSB) complex (C. tomentosicollis, A. curvipes, R dentipes, N. viridula, T. custator and A. armigera) did not differ among the entries. To date, cowpea breeding programmes have not succeeded in developing varieties that are stably resistant to multiple damaging pests of the crop, including PSBs (Togola et al., 2017). This is because several minor genes (polygenic resistance) regulate the control of multiple insect pests’ attacks. Until recently, the introgression of these minor genes into new varieties was considered complex. In recent times however, advances in biotechnology makes it possible to introgress these genes into improved cowpea varieties (Sharma et al., 2002; Kogan, 1994). This notwithstanding, none of the cowpea entries used in this work were resistant to the PSB pest complex. Damage by these sucking pests probably accounted for the differences in the 100-seed weight at Nyankpala in particular.
Apart from SAMPEA 20-T, which expresses the Cry1Ab insecticidal protein conferring resistance to damage by Maruca pod borer (Addae et al., 2020), all the genotypes, including CSI-32, were susceptible to damage by this pest. The expression of αAI-1 protein in CSI-32 seed would not be expected to confer protection against pod borers and the in-field pest observations were consistent with the activity spectrum of αAI-1.
Cowpea yields are impacted by environmental variables (rainfall, temperature), genotypic potential, and pests and diseases (Cerıtoglu and Erman, 2020; Jackai and Daoust, 1986; Ibrahim et al., 2021; Karungi et al., 2000). In this study, a uniform spray regime was applied to all entries to control arthropod pests, and their exposure to elements of the weather were the same. Hence, grain yield was determined largely by the yield potential of the individual genotypes assessed. Insect damage did not significantly affect yield. Although genotype was a significant factor for yield (p<0.001), yields were very similar among four of the five entries, with only IT13K-1070-2 exhibiting higher yield at both locations.
Except landraces (Silva et al., 2021), many studies that have evaluated commercially cultivated improved cowpea varieties for weevil resistance, have been unsuccessful in identifying durable sources of resistance to this voracious pest (Badii et al., 2013; Deshpande et al., 2011; Kpoviessi et al., 2019; Amusa et al., 2018). Similarly, none of the commercial cowpea entries tested in this work were resistant to C. maculatus. The genotype, SAMPEA 7, which is reported to be susceptible to weevils (Azeez and Pitan, 2013; Ojumoola and Adesiyun, 2014; Ofuya and Credland, 1995) and IT13K-1070-2, which is reported to be moderately resistant to this pest (Tengey et al., 2023), were both found to be highly susceptible to weevils when they were subjected to an infestation pressure of 50 sexually matured insects per 200-g seed sample. Oviposition was similar across all the entries tested, including CSI-32, indicating that αAI-1 did not act as a deterrent to oviposition; however, it did act to completely suppress larval development and adult emergence during the 4-month duration of the study.
The median development period (MDP), adult emergence, and severity of grain damage are important determinants of ranking cowpea genotypes as being susceptible to cowpea weevils. Prolonged development time, low adult emergence, and low grain damage are key characteristics of resistant genotypes and vice versa (Amusa et al., 2018; Azeez and Pitan, 2013; Silva et al., 2021). Additionally, Badii et al. (2013) and Amusa et al. (2018) reported a difference in MDP of ca. 3 and ca 6 days, respectively, between susceptible and resistant cowpea genotypes. Here, we report ca. 0.5 days difference between the highest and lowest MDP, which is unlikely to be biologically meaningful. The genotypes, IT86D-1010, SAMPEA-7, IT13K-1070-2 and SAMPEA 20-T, were categorized as being highly susceptible based on mean Dobie’s Susceptibility Index (DSI) values >20 for each entry. Further, the survival and emergence of adults as well as the overall high levels of seed damage recorded for these genotypes confirmed their susceptibility.
This is the first study to examine both in-field agronomic performance and post-harvest storability of a transgenic cowpea expressing αAI-1 to control the cowpea weevil. The findings of the current study and earlier ones clearly demonstrate that developing cowpea varieties that express the αAI-1 protein presents a sustainable and cost-effective approach to mitigating damage by this pest. The technology to control the weevil is incorporated in the seed itself and protection is not reliant on the application of synthetic insecticides that have known adverse health and environmental consequences (Kolawole et al., 2014; Kpoviessi et al., 2019). Further, this technology could complement the use of hermetic sealed bags, especially for those who can afford the bags in Africa (Hajam and Kumar, 2022; Silva et al., 2018).
Genetic modification approaches have proven highly effective at separately controlling key field pests, such as M. vitrata, and as shown here, the post-harvest storage pest, C. maculatus. As discussed in Barrero et al. (2021), future work could include the development of novel cowpea varieties combining these two important insect resistance traits for the benefit of smallholder farmers and consumers in West Africa.
Data availability statement
The raw data supporting the conclusions of this article will be made available by the authors, without undue reservation.
Ethics statement
The manuscript presents research on animals that do not require ethical approval for their study.
Author contributions
JN: Conceptualization, Data curation, Investigation, Methodology, Writing – original draft, Writing – review & editing. MU: Data curation, Investigation, Methodology, Writing – review & editing. GA: Investigation, Methodology, Writing – original draft, Writing – review & editing. IU: Investigation, Methodology, Writing – original draft, Writing – review & editing. PA: Investigation, Writing – review & editing. FA: Investigation, Methodology, Writing – review & editing. MI: Data curation, Investigation, Methodology, Supervision, Writing – review & editing. JB: Conceptualization, Methodology, Supervision, Writing – original draft, Writing – review & editing. TH: Conceptualization, Data curation, Formal Analysis, Methodology, Writing – original draft, Writing – review & editing. DM: Conceptualization, Data curation, Formal Analysis, Methodology, Supervision, Writing – original draft, Writing – review & editing.
Funding
The author(s) declare financial support was received for the research, authorship, and/or publication of this article. This work was fully supported by Bill & Melinda Gates Agricultural Innovations (Gates Ag One).
Acknowledgments
The authors acknowledge the collective contributions of the Alliance for Cowpea Improvement in Africa partners: the Council for Scientific and Industrial Research - Savanna Agricultural Research Institute (Ghana), the Institute for Agricultural Research (Nigeria), the African Agricultural Technology Foundation (Kenya), the Commonwealth Scientific and Industrial Research Organisation (Australia), the Donald Danforth Plant Science Center (USA), and Bill & Melinda Gates Agricultural Innovations (USA).
Conflict of interest
The authors declare that the research was conducted in the absence of any commercial or financial relationships that could be construed as a potential conflict of interest.
Publisher’s note
All claims expressed in this article are solely those of the authors and do not necessarily represent those of their affiliated organizations, or those of the publisher, the editors and the reviewers. Any product that may be evaluated in this article, or claim that may be made by its manufacturer, is not guaranteed or endorsed by the publisher.
Author disclaimer
The conclusions and opinions expressed in this work are those of the author(s) alone and shall not be attributed to Bill & Melinda Gates Agricultural Innovations.
References
Adam, J. I., Baidoo, P. K. (2008). Susceptibility of five cowpea (Vigna unguiculata) cultivars to attack by Callosobruchus maculatus (FAB.) [Coleoptera: Bruchidae. J. Ghana Sci. Assoc. 10, 85–92. doi: 10.4314/jgsa.v10i2.18044
Addae, P. C., Ishiyaku, M. F., Tignegre, J. B., Ba, M. N., Bationo, J. B., Atokple, I. D., et al. (2020). Efficacy of a cry1Ab gene for control of Maruca vitrata (Lepidoptera: Crambidae) in cowpea (Fabales: Fabaceae). J. Economic Entomology 113, 974–979. doi: 10.1093/jee/toz367
Agyeman, K., Berchie, J. N., Osei-Bonsu, I., Fordjour, J. K. (2015). Seed yield and agronomic performance of seven improved cowpea (Vigna unguiculata L.) varieties in Ghana. Afr. J. Agric. Res. 10, 215–221. doi: 10.5897/AJAR2014.8835
Ajayi, F. A., Lale, N. S. F. (2001). Seed coat texture, host species and time of application affect the efficacy of essential oils applied for the control of Callosobruchus maculatus (F.) (Coleoptera: Bruchidae) in stored pulses. Int. J. Pest Manage. 47, 161–166. doi: 10.1080/09670870010004495
Altabella, T., Chrispeels, M. J. (1990). Tobacco plants transformed with the bean αAI gene express an inhibitor of insect α-amylase in their seeds. Plant Physiol. 93, 805–810. doi: 10.1104/pp.93.2.805
Amusa, O. D., Ogunkanmi, L. A., Adetumbi, J. A., Akinyosoye, S. T., Ogundipe, O. T. (2018). Genetics of bruchid (Callosobruchus maculatus Fab.) resistance in cowpea (Vigna unguiculata (L.) Walp.). J. Stored Products Res. 75, 18–20. doi: 10.1016/j.jspr.2017.11.004
Amusa, O. D., Ogunkanmi, L. A., Adetunbi, J. A., Akinyosoye, S. T., Bolarinwa, K. A., Ogundipe, O. T.. (2014). Assessment of bruchid (Callosobruchus maculatus) tolerance of some elite cowpea (Vigna unguiculata) varieties. Journal of Agriculture and Sustainability 6 (2), 164–178.
Animasaun, D. A., Oyedeji, S., Mustapha, O. T., Azeez, M. A. (2015). Genetic variability study mong ten cultivars of cowpea (Vigna unguiculata L. Walp) using morpho-agronomic traits and nutritional composition. J. Agric. Sci. 10, 119. doi: 10.4038/jas.v10i2.8057
Azeez, O. M., Pitan, O. O. R. (2013). Comparative seed resistance in eighty cowpea accessions to the seed bruchid,Callosobruchus maculatus(Fabricius) (Coleoptera: Bruchidae). Arch. Of Phytopathol. And Plant Prot. 47, 1806–1814. doi: 10.1080/03235408.2013.858426
Badii, K. B., Asante, S. K., Sowley, E. N. K. (2013). Varietal susceptibility of cowpea (Vigna unguiculata L.) to the storage beetle, Callosobruchus maculatus F.(Coleoptera: Bruchidae). Int. J. Sc. Technol Res 2 (4), 82–89.
Baillie, I. C. (2001). Soil survey staff, (1999). Soil Taxonomy: A basic system of soil classification for making and interpreting soil surveys, Agricultural Handbook 436, Natural Resources Conservation Service, USDA, Washington DC, USA, pp. 869. Soil Use Manage. 17, 57–60. doi: 10.1111/j.1475-2743.2001.tb00008.x
Barrero, J. M., Mackenzie, D. J., Goodman, R. E., Higgins, T. V. J. (2021). A biotech shield for cowpea against the major insect pests (Open Access Governmnet). Available at: https://www.openaccessgovernment.org/major-insect-pests/111590/.
Bates, D. (2014). Fitting linear mixed-effects models using lme4. arXiv preprint arXiv. 1406.5823. doi: 10.48550/arXiv.1406.5823
Bekele, D., Birhan, M. (2021). The characteristics, distribution and management of Alfisols. Int. J. Res. Stud. Agric. Sci. 7, 1–6. doi: 10.20431/2454-6224.0706001
Belay, F., Gebreslasie, A., Meresa, H. (2017). Agronomic performance evaluation of cowpea [Vigna unguiculata (L.) Walp] varieties in Abergelle District, Northern Ethiopia. J. Plant Breed. Crop Sci. 9, 139–143. doi: 10.5897/JPBCS2017.0640
Bolarinwa, K. A., Ogunkanmi, L. A., Ogundipe, O. T., Agboola, O. O., Amusa, O. D. (2022). An investigation of cowpea production constraints and preferences among small holder farmers in Nigeria. GeoJournal 87, 2993–3005. doi: 10.1007/s10708-021-10405-6
Cerıtoglu, M., Erman, M. (2020). Determination of some agronomic traits and their correlation with yield components in cowpea. Selcuk J. Agric. Food Sci. 34, 154–161.
Chakraborty, S., Mondal, P., Senapati, S. K. (2015). Evaluation of relative susceptibility of Callosobruchus chinensis Linn. on five different stored pulse seeds. Asian J. Plant Sci. Res. 39, 9–15.
da Silva, A. C., da Costa Santos, D., Junior, D. L. T., da Silva, P. B., dos Santos, R. C., Siviero, A. (2018). “Cowpea: A strategic legume species for food security and health,” in Legume seed nutraceutical research (London, United Kingdom: IntechOpen). doi: 10.5772/intechopen.79006
Deshpande, V. K., Makanur, B., Deshpande, S. K., Adiger, S., Salimath, P. M. (2011). Quantitative and qualitative losses caused by Callosobruchus maculatus in cowpea during seed storage. Plant Arch. 11, 723–731.
Devi, M. B., Devi, N. V. (2014). Biology and morphometric measurement of cowpea weevil, Callosobruchus maculatus fabr.(Coleoptera: Chrysomelidae) in green gram. J. Entomology Zoology Stud. 2, 74–76.
Dobie, P. (1974). The laboratory assessment of the inherent susceptibility of maize varieties to post-harvest infestation by Sitophilus zeamais Motsch. (Coleoptera, Curculionidae). J. Stored Products Res. 10, 183–197. doi: 10.1016/0022-474X(74)90006-X
Dobie, P., Kilminster, A. M. (1978). The susceptibility of triticale to post-harvest infestation by Sitophilus zeamais Motschulsky, Sitophilus oryzae (L.) and Sitophilus granarius (L.). J. Stored Products Res. 14, 87–93. doi: 10.1016/0022-474X(78)90003-6
Ekoja, E. E., Onekutu, A., Ode, H. S. (2021). Control of Callosobruchus maculatus Fabricius (Coleoptera: Chrysomelidae: BruChinae) using Triglycerides from Animal Sources. Arch. Phytopathol. Crop Protection. 54, 516–553. doi: 10.1080/03235408.2020.1843324
Geiger, R. (1961). Überarbeitete Neuausgabe von Geiger, R. K€oppen-Geiger/Klima der Erde. (Wandkarte 1: 16 Mill.) (Klett-Perthes, Gotha).
Gerrano, A. S., Adebola, P. O., Jansen van Rensburg, W. S., Laurie, S. M. (2015). Genetic variability in cowpea (Vigna unguiculata (L.) Walp.) genotypes. South Afr. J. Plant Soil 32, 165–174. doi: 10.1080/02571862.2015.1014435
Hajam, Y. A., Kumar, R. (2022). Management of stored grain pest with special reference to Callosobruchus maculatus, a major pest of cowpea: A review. Heliyon 8 (1). 08703. doi: 10.1016/j.heliyon.2021.e08703
Higgins, T., Gollasch, S., Molvig, L., Moore, A., Popelka, C., Armstrong, J., et al. (2012). “Insect-protected cowpeas using gene technology,” in Fifth World Cowpea Conference on improving livlihoods in the cowpea value chain through advancement in science. Eds. Boukar, O., Coulibaly, O., Fatokun, C., Lopez, K., Tamo, M. (IITA, Saly, Senegal), 131–137.
Howe, R. W. (1971). A parameter for expressing the suitability of an environment for insect development. J. Stored Product Research. 7 (1), 63–65. doi: 10.1016/0022-474X(71)90039-7
Ibrahim, M., David, A. A., Mshelia, J. S., John, A. B., Sunday, B. A., Stephanie, S. (2021). Effect of climate variation on the yield of cowpea (Vigna unguiculata). Afr. J. Agric. Res. 17, 456–462. doi: 10.5897/AJAR2020.14960
Ige, O. E., Olotuah, O. F., Akerele, V. (2011). Floral biology and pollination ecology of cowpea (Vigna unguiculata L. Walp). Modern Appl. Sci. 5, 74–82. doi: 10.5539/mas.v5n4p74
Ishimoto, M., Sato, T., Chrispeels, M. J., Kitamura, K. (1996). Bruchid resistance of transgenic azuki bean expressing seed α-amylase inhibitor of common bean. Entomologia Experimentalis Applicata 79, 309–315. doi: 10.1111/j.1570-7458.1996.tb00838.x
Jackai, L. E., Daoust, R. A. (1986). Insect pests of cowpeas. Annu. Rev. entomology 31, 95–119. doi: 10.1146/annurev.en.31.010186.000523
Karungi, J., Adipala, E., Nampala, P., Ogenga-Latigo, M. W., Kyamanywa, S. (2000). Pest management in cowpea. Part 3. Quantifying the effect of cowpea field pests on grain yields in eastern Uganda. Crop Prot. 19, 343–347. doi: 10.1016/S0261-2194(00)00014-4
Khan, M. Z. (2011). Eco-friendly mana gement of pulse beetle, Callosobruchus chinensis Linn. using botanicals and fumigants on stored mungbean (doctoral dissertation, department of Entomology, Sher-e-Bangla Agricultural University, Dhaka-1207).
Kogan, M. (1994). “Plant resistance in pest management,” in Introduction to insect pest management, 3rd edn. Eds. Metcalf, R. L., Luckmann, W. H. (Wiley, New York).
Kolawole, A. O., Olajuyigbe, F. M., Ajele, J. O., Adedire, C. O. (2014). Activity of the antioxidant defense system in a typical bioinsecticide-and synthetic insecticide-treated cowpea storage beetle Callosobrochus maculatus F.(Coleoptera: Chrysomelidae). Int. J. Insect Sci. 6, IJIS–S19434. doi: 10.4137/IJIS.S19434
Kpoviessi, A., Agbahoungba, S., Agoyi, E., Chougourou, D., Assoghadjo, A. (2019). Resistance of cowpea to cowpea bruchid (Callosobruchus maculatus Fab.): Knowledge level on the genetic advances. J. Plant Breed. Crop Sci. 11, 185–195. doi: 10.5897/jpbcs2019.0818
Kusuma, I. W., Arung, E. T., Kim, Y. U. (2014). Antimicrobial and antioxidant properties of medicinal plants used by the Bentian tribe from Indonesia. Food Sci. Hum. Wellness 3, 191–196. doi: 10.1016/j.fshw.2014.12.004
Lale, N. E. S., Kolo, A. A. (1998). Susceptibility of eight genetically improved local cultivars of cowpea to Callosobruchus maculatus (F.) (Coleoptera: Bruchidae) in Nigeria. Int. J. Pest Manage. 44, 25–27. doi: 10.1080/096708798228482
Lambert, J. D. H., Gale, J., Amason, J. T., Philogene, B. J. R. (1985). Bruchid control with traditionally used insecticidal plants Hyptis spicigera and Cassia nigricans. Insect Sci. Its Appl. 6, 167–168. doi: 10.1017/S1742758400006573
Loganathan, M., Jayas, D. S., Fields, P. G., White, N. D. G. (2011). Low and high temperatures for the control of cowpea beetle, Callosobruchus maculatus (F.) (coleoptera: Bruchidae) in chickpeas. J. Stored Products Res. 47, 244–248. doi: 10.1016/j.jspr.2011.03.005
Lüthi, C., Álvarez-Alfageme, F., Ehlers, J. D., Higgins, T. J. V., Romeis, J. (2013). Resistance of αAI-1 transgenic chickpea (Cicer arietinum) and cowpea (Vigna unguiculata) dry grains to bruchid beetles (Coleoptera: Chrysomelidae). Bull. Entomological Res. 103, 373–381. doi: 10.1017/S0007485312000818
Malaikozhundan, B., Vinodhini, J. (2018). Biological control of the Pulse beetle, Callosobruchus maculatus in stored grains using the entomopathogenic bacteria, Bacillus thuringiensis. Microbial pathogenesis 114, 139–146. doi: 10.1016/j.micpath.2017.11.046
Marshall, J. J., Lauda, C. M. (1975). Purification and properties of phaseolamin, an inhibitor of alpha-amylase, from the kidney bean, Phaseolus vulgaris. Journal of Biological Chemistry. 250 (20), 8030–8037. doi: 10.1016/S0021-9258(19)40811-9
Mkenda, P. A., Mtei, K., Ndakidemi, P. A. (2014). Pesticidal efficacy of Tephrosia vogelii and Tithonia diversifolia against field insect pests of common beans (Phaseolus vulgaris L.) within African farming communities. Afr. J. Appl. Agric. Sci. Technol. 2, 9–26.
Musa, A. K., Adeboye, A. A. (2017). Susceptibility of some cowpea varieties to the seed beetle Callosobruchus maculatus (F.)(Coleoptera: Chrysomelidae). J. Agric. Sci. (Belgrade) 62, 351–360. doi: 10.2298/JAS1704351M
Nkoana, D. K., Gerrano, A. S., Gwata, E. T. (2019). Agronomic performance and genetic variability of cowpea (Vigna unguiculata) accessions. Legume Research-An Int. J. 42, 757–762. doi: 10.18805/LR-450
Nwagboso, C., Andam, K. S., Amare, M., Bamiwuye, T., Fasoranti, A. (2024). The Economic Importance of Cowpea in Nigeria Trends and Implications for Achieving Agri-Food System Transformation (Abuja, Nigeria: IFPRI Discussion Paper 02241), 30pp.
Nyarko, J., Asare, A. T., Mensah, B. A., Adjei, F. (2022). Assessment of the response of fifteen cowpea [Vigna unguiculata L. (Walp.)] genotypes to infestation by Callosobruchus maculatus Fab. (Coloeptera: Bruchidae). Cogent Food Agric. 8 (1). doi: 10.1080/23311932.2022.2095713
Ofuya, T. I., Credland, P. F. (1995). Responses of three populations of the seed beetle, Callosobruchus maculatus (F.) (Coleoptera: Bruchidae), to seed resistance in selected varieties of cowpea, Vigna unguiculata (L.) Walp. J. Stored Products Res. 31, 17–27. doi: 10.1016/0022-474x(95)91807-d
Ofuya, T. I., Olotuah, O., Akinyoade, D. (2010). The effect of storage on the efficacy of Eugenia Aromatica (Baill.) in the control of Callosobruchus maculatus (Fabricius)(Coleoptera: Bruchidae) pest. J. Appl. Sci. Environ. Manage. 14 (1), 97–100. doi: 10.4314/jasem.v14i1.56509
Ojebode, M. E., Olaiya, C. O., Adegbite, A. E., Karigidi, K. O., Ale, T. O. (2016). Efficacy of some plant extracts as storage protectants against Callosobruchus maculatus. J. Biotechnol. Biomaterials 6, 1–4. doi: 10.4172/2155-952X.1000217
Ojumoola, A. O., Adesiyun, A. A. (2014). Effects of five improved cowpea seed genotypes on oviposition and progeny development of Callosobruchus maculatus (F.) (Coleoptera : Chrysomelidae). FUTA J. Res. Sci. 1, 84–90.
Olufade, Y. A., Sosan, M. B., Oyekunle, J. A. O. (2014). Levels of Organochlorine Insecticide Residues in Cowpea Grains and Dried Yam chips from markets in Ile-Ife, Southwestern Nigeria: A Preliminary Survey. Ife J. Sci. 16, 161–170.
Olutona, G. O., Aderemi, M. A. (2019). Organochlorine pesticide residue and heavy metals in leguminous food crops from selected markets in Ibadan, Nigeria. Legume Science. 1, e3. doi: 10.1002/leg3.v1.1
Onigbinde, A. O., Oigiangbe, N. O. (1996). The association between some physico-chemical characteristics and susceptibility of cowpea (Vigna unguiculata (L.) Walp) to Callosobruchus maculatus (F.). J. Stored Products Res. 32, 7–11. doi: 10.1016/0022-474X(96)00001-X
Sanon, A., Ba, N. M., Binso-Dabire, C. L., Pittendrigh, B. R. (2010). Effectiveness of spinosad (naturalytes) in controlling the cowpea storage pest, Callosobruchus maculatus (Coleoptera: Bruchidae). J. Economic Entomology 103, 203–210. doi: 10.1603/EC09093
Sarmah, B. K., Moore, A., Tate, W., Molvig, L., Morton, R. L., Rees, D. P., et al. (2004). Transgenic chickpea seeds expressing high levels of a bean a-amylase inhibitor. Mol. Breed. 14, 73–82. doi: 10.1023/B:MOLB.0000037996.01494.12
Schroeder, H. E., Gollasch, S., Moore, A., Tabe, L. M., Craig, S., Hardie, D. C., et al. (1995). Bean α-amylase inhibitor confers resistance to the pea weevil (Bruchus pisorum) in transgenic peas (Pisum sativum L.). Plant Physiol. 107, 1233–1239. doi: 10.1104/pp.107.4.1233
Serno, G., van de Weg, R. F. (1985). Preliminary assessment of the (available) existing soil information of Nyankpala Agricultural Experimental Station, Tamale, Ghana (Stiboka: Wageningen, The Netherlands).
Shade, R. E., Schroeder, H. E., Pueyo, J. J., Tabe, L. M., Murdock, L. L., Higgins, T., et al. (1994). Transgenic pea seeds expressing the α-amylase inhibitor of the common bean are resistant to bruchid beetles. Nat. Biotechnol. 12, 793–796. doi: 10.1038/nbt0894-793
Sharma, H. C., Crouch, J. H., Sharma, K. K., Seetharama N.and Hash, C. T. (2002). Applications of biotechnology for crop improvement: prospects and constraints. Plant Sci. 163, 381–395. doi: 10.1016/S0168-9452(02)00133-4
Shimelis, H., Shiringani, R. (2010). Variance components and heritabilities of yield and agronomic traits among cowpea genotypes. Euphytica 176, 383–389. doi: 10.1007/s10681-010-0222-z
Silva, A. M. C., da Matos, C. H. C., de Oliveira, C. R. F., da Silva, T. G. F. (2021). Resistance of landrace and commercial cowpea genotypes to Callosobruchus maculatus attack. J. Stored Products Res. 92, 101801. doi: 10.1016/j.jspr.2021.101801
Silva, M. G., Silva, G. N., Sousa, A. H., Freitas, R. S., Silva, M. S., Abreu, A. O. (2018). Hermetic storage as an alternative for controlling Callosobruchus maculatus (Coleoptera: Chrysomelidae) and preserving the quality of cowpeas. J. stored products Res. 78, 27–31. doi: 10.1016/j.jspr.2018.05.010
Singh, B. B. (2005). “Cowpea [Vigna unguiculata (L.) Walp.],” in Genetic resources, chromosome engineering and crop improvement, vol. 1 . Eds. Singh, R. J., Jauher, P. P. (CRC Press, Taylor & Francis Group, Singapore), 117–162.
Singh, B. B., Singh, S. R., Adjadi, O. (1985). Bruchid resistance in cowpea. Crop Sci. 25, 736–739. doi: 10.2135/cropsci1985.0011183x002500050004x
Solleti, S. K., Bakshi, S., Purkayastha, J., Panda, S. K., Sahoo, L. (2008). Transgenic cowpea (Vigna unguiculata) seeds expressing a bean α-amylase inhibitor 1 confer resistance to storage pests, bruchid beetles. Plant Cell Rep. 27, 1841–1850. doi: 10.1007/s00299-008-0606-x
Swella, G. B., Mushobozy, D. M. K. (2007). Evaluation of the efficacy of protectants against Cowpea bruchids (Callosobruchus maculatus (F.)) on Cowpea seeds (Vigna unguiculata (L.) Walp.). Plant Prot. Sci. 43, 68–72. doi: 10.17221/2256-pps
Tengey, T. K., Alidu, S. M., Moro, A., Nboyine, J., Affram, E. I. (2023). Resistance to Callosobruchus maculatus among cowpea (Vigna unguiculata (L.) Walp.) genotypes. J. Crop Improvement 37, 309–322. doi: 10.1080/15427528.2022.2092573
Togola, A., Boukar, O., Belko, N., Chamarthi, S. K., Fatokun, C., Tamo, M., et al. (2017). Host plant resistance to insect pests of cowpea (Vigna unguiculata L. Walp.): achievements and future prospects. Euphytica 213, 239. doi: 10.1007/s10681-017-2030-1
Torres, E. B., Nóbrega, R. S. A., Fernandes-Júnior, P. I., Silva, L. B., Carvalho, G., Marinho, R., et al. (2016). The damage caused by Callosobruchus maculatus on cowpea grains is dependent on the plant genotype. J. Sci. Food Agric. 96, 4276–4280. doi: 10.1002/jsfa.7639
Keywords: alpha-amylase inhibitor, bruchids, cowpea, genetic modification, storage losses, host plant resistance
Citation: Nboyine JA, Umar ML, Adazebra GA, Utono IM, Agrengsore P, Awuku FJ, Ishiyaku MF, Barrero JM, Higgins TJV and MacKenzie DJ (2024) Assessment of field performance and bruchid resistance during seed storage of a genetically modified cowpea expressing the alpha-amylase inhibitor 1 protein from common bean. Front. Plant Sci. 15:1478700. doi: 10.3389/fpls.2024.1478700
Received: 10 August 2024; Accepted: 28 October 2024;
Published: 22 November 2024.
Edited by:
Lorena Parra, Universitat Politècnica de València, SpainReviewed by:
Phatu William Mashela, University of Limpopo, South AfricaRasha Ezzat Mahdy, Assiut University, Egypt
Copyright © 2024 Nboyine, Umar, Adazebra, Utono, Agrengsore, Awuku, Ishiyaku, Barrero, Higgins and MacKenzie. This is an open-access article distributed under the terms of the Creative Commons Attribution License (CC BY). The use, distribution or reproduction in other forums is permitted, provided the original author(s) and the copyright owner(s) are credited and that the original publication in this journal is cited, in accordance with accepted academic practice. No use, distribution or reproduction is permitted which does not comply with these terms.
*Correspondence: Jerry A. Nboyine, bmJveWluZWplcnJ5QGdtYWlsLmNvbQ==