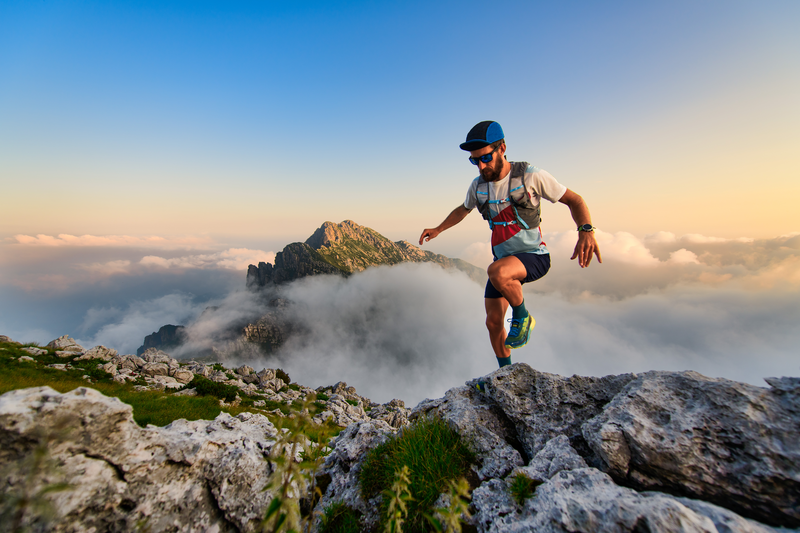
95% of researchers rate our articles as excellent or good
Learn more about the work of our research integrity team to safeguard the quality of each article we publish.
Find out more
EDITORIAL article
Front. Plant Sci. , 21 August 2024
Sec. Plant Metabolism and Chemodiversity
Volume 15 - 2024 | https://doi.org/10.3389/fpls.2024.1477551
This article is part of the Research Topic Plant Secondary Metabolite Biosynthesis View all 12 articles
Editorial on the Research Topic
Plant secondary metabolite biosynthesis
Plant secondary metabolites (PSM) are a diverse group of compounds that contribute to many important biological and ecological functions. They are synthesized by plants to interact with the biotic and abiotic environments, playing roles in plant defense, growth, and development (Erb and Kliebenstein, 2020). Additionally, PSM have widespread applications in human industries, including food additives, cosmetics, dyes, insecticides, and drugs. The biosynthesis of PSM is complex and dynamic, with more than one million PSM estimated to be present in terrestrial and aquatic plants (Afendi et al., 2012). Despite their diversity, plants produce limited quantities of PSM in a metabolic cost-saving way. This has limited their commercial production, and the overexploitation of source plants has raised concerns about their sustainability and highlighted the need for advanced research.
Recent advances in genomics (Siadjeu and Pucker, 2023), transcriptomics (Voelckel et al., 2017), metabolomics (Li et al., 2024), and other omics technologies have revolutionized our understanding of plant biology, enabling the discovery of new PSM and their biosynthetic pathways. Functional genomics approaches, such as genome-wide association studies (Luo et al., 2020), transcriptome analysis (Liu et al., 2021), and gene editing (Nasti and Voytas, 2021), have facilitated the identification and characterization of genes involved in the biosynthesis of PSM. Metabolic engineering and synthetic biology approaches have enabled the manipulation of plant secondary metabolism to improve the yield and quality of specific metabolites of interest or to produce them in heterologous cultures (Zhang et al., 2022). These advances have created new opportunities for the sustainable production and utilization of PSM.
This Research Topic on Plant Secondary Metabolite Biosynthesis illustrates a comprehensive and up-to-date view of the biosynthesis, regulation, and biotechnological production of PSM, and to promote interdisciplinary and cross-disciplinary research collaborations in this field for sustainable and efficient utilization of these valuable compounds.
Combining bioinformatics method, gene quantitative analysis, and evolutionary analysis, Wang et al. retrieve 103 BAHD genes from the Ginseng Genome Data resource. Phylogenetic analysis indicates that these BAHD genes are clustered in three clades. Most PgBAHDs contain cis-acting elements associated with abiotic stress response and plant hormone response. Among them, 34 PgBAHDs are clustered with genes that display malonyl transferase activity. Seven PgBAHDs (PgBAHD4, PgBAHD45, PgBAHD65, PgBAHD74, PgBAHD90, PgBAHD97, and PgBAHD99) are designated as the potential candidates involved in the biosynthesis of malonyl ginsenosides based on co-expression analysis. To fully elucidate the biosynthetic pathways of orobanchol derivatives in Fabaceae plants, Homma et al. probe the metabolomic pathways downstream of orobanchol in cowpea, barrel medic, and pea via substrate feeding experiment after different enzyme inhibitor (fluridone, uniconazole-P, and prohexadione) treatment. Subsequently, DOX and BAHD acyltransferases responsible for converting orobanchol to their derivatives are mined from the public dataset and screened using co-expression analysis. Enzymatic assays of heterologously expressed proteins reveal that the DOX in barrel medic converts orobanchol to medicaol, the DOX and BAHD acyltransferase in pea convert orobanchol to fabacol and acetylated fabacol, the favacol acetyltransferase and its homolog in cowpea acetylate orobanchol. These findings shed light on the molecular mechanisms underlying the structural diversity of strigolactones.
Hendrickson et al. characterize series of terpene synthases (TPS) from Medicago truncatula, a model legume. Thirty-nine MtTPS candidates are obtained from the Mt4.0v1 genome in Phytozome (tps://phytozome-next.jgi.doe.gov). They assess the MtTPS activity using a modular metabolic engineering system in E. coli and characterize the resultant metabolite using GC-MS. Analyses of the resultant metabolite reveal the production of an assortment of sesquiterpenes. This work also establishes the gene-to-metabolite relationships for sesquiterpene synthase in M. truncatula. To explore the glycosylation step for rutin biosynthesis in Solanum melongena, Gan et al. identify 195 UDP-glycosyltransferase (UGT) candidate genes from the S. melongena genome V4.0 (ttps://solgenomics.net). These UGT genes are classified into 17 subgroups and the members of Groups A, B, D, E, and L are associated with flavonol biosynthesis. A hierarchical clustering analysis of gene expression profiles reveals that the expression patterns of SmUGT genes in Clusters 7-10 are closely related to those of rutin biosynthetic pathway genes. Among them, SmUGT89B2 is verified to encode the final enzyme in rutin biosynthesis via virus-induced gene silencing and transient overexpression assay in eggplant. Rosmarinic acid (RA) is one of the major bioactive components of Prunella vulgaris. Yan et al. identified 51 RA biosynthesis-related genes from the chromosome-level genome of P. vulgaris. Bioinformatic analyses and gene expression pattern indicate that 17 of them could be involved in RA biosynthesis. In vitro enzymatic assay reveals that PvRAS3 catalyzes the condensation of p-coumaroyl-CoA and caffeoyl-CoA with pHPL and DHPL, and PvRAS4 only catalyzes the condensation of p-coumaroyl-CoA with pHPL and DHPL. Generation of pvras3 homozygous mutants through CRISPR/Cas9 technology and subsequent chemical compound analysis confirm that PvRAS3 is the main enzyme catalyzing the condensation of acyl donors and acyl acceptors during RA biosynthesis in P. vulgaris. This work supports the existence of at least four RA biosynthetic pathways, with the role of PvRAS4 appears minor in this plant.
Zheng et al. assemble a high-quality genome of Fagopyrum dibotrys. Based on evolutionary relationship analysis, the authors speculate that F. dibotrys has originated in the high-altitude Tibetan Plateau region. The homologues of genes involved in the biosynthesis of flavonoids are annotated. This study could reveal the genetic background and facilitate the cultivation of high-yielding F. dibotrys varieties. Li et al. employ single-cell RNA sequencing to profile the transcriptomes of protoplasts derived from Gynostemma pentaphyllum shoot apexes and leaves. Examining gene expression patterns across various cell types reveal that genes related to gypenoside biosynthesis are predominantly expressed in mesophyll cells. They also explore the impact of transposable elements (TE) on G. pentaphyllum transcriptomic landscapes. This study not only provides new insights into the spatiotemporal organization of gypenoside biosynthesis and TE activity in shoot apexes and leaves, but also offers valuable cellular and genetic resources for improving the yield of gypenoside in G. pentaphyllum.
Mentha spicata (spearmint) possesses peltate glandular trichomes (PGT) where valuable essential oils are produced. Reddy et al. identify a novel non-canonical Aux/IAA gene, MsIAA32, from spearmint, which lacks the TIR1-binding domain and regulates the development of PGT. Using yeast two-hybrid studies, two canonical Aux/IAAs (MsIAA3, MsIAA4) and an ARF (MsARF3) are identified as the preferred binding partners of MsIAA32. The possible role of MsIAA32 in non-glandular trichome formation is confirmed by ectopic expression in Arabidopsis. Undoubtedly, identifying new gene targets controlling PGT numbers in spearmint will provide new ways to increase volatile/scented PSM production. Dendrobine, a noteworthy alkaloid found in Dendrobium nobile, possesses valuable pharmaceutical potential. Sarsaiya et al. develop innovative approaches to enhance dendrobine production by utilizing endophytic fungi. Using test bottles (EGTB), the experimental group (12-month-old D. nobile seedling), co-cultured with Trichoderma longibrachiatum (MD33), displays a 2.6-fold denrobine increase (1804.23 ng/ml) compared to the control group (685.95 ng/ml). Co-culturing D. nobile seedlings with T. longibrachiatum MD33 in the temporary immersion bioreactor systems (EGTIBS) leads to a substantial 9.7-fold dendrobine increase (4415.77 ng/ml) compared to the control (454.01 ng/ml) after 7 days. Scaling up the TIBS approach for commercial dendrobine production could provide an accessible platform for dendrobine production.
Phoebe zhennan is widely cultivated in China and the price of nanmu wood is expensive. Yang et al. explore the composition and content of essential oil and fragrance compounds in P. zhennan wood at different tree ages. The yield of essential oil from 30a wood is significantly greater than that from 10a and 80a wood. In total, 596 (LC) and 76 (GC) features are annotated using chromatography-coupled mass spectrometry in the essential oil and wood. This research determines that the main components of the wood fragrance are sesquiterpenoids. The types and relative contents of sesquiterpenoids from wood increase with tree age. These results suggest that choosing wood from trees of a suitable age will significantly improve the yield of essential oil. Qin et al. successfully improve the seed tocopherol concentration by altering chlorophyll metabolism. They find that RNAi suppression of CHLSYN coupled with homogentisate phytyltransferase (HPT) overexpression increase tocopherol concentration by more than two-fold in Arabidopsis seeds. Additional three-fold increase in seed tocopherol are observed by engineering homogentisate production via overexpression of bacterial TyrA, which encodes chorismate mutase/prephenate dehydrogenase. They further increase total tocochromanol concentration by overexpression of the gene for hydroxyphenylpyruvate dioxygenase. These biofortification approaches shift metabolism towards increased amounts of tocotrienols relative to tocopherols. This study provides a theoretical basis for genetic improvement of total tocopherol concentrations in green oilseeds.
The number of PSM is still expanding due to the diversity of plant species and the rapid advances in analytical technology. Undoubtedly, PSM has served as a natural compound resource for human industries. But there is a contradiction between the insatiable human needs for PSM and the existing chemical-based production methods, such as plant source-dependent extraction and sophisticated chemical synthesis. Metabolic engineering and synthetic biology approaches have been verified as one of the best ways for PSM manufacture. These innovative biotechnological techniques depend on fully elucidating the biosynthetic pathway and biochemically charactering the gene elements responsible for PSM biosynthesis one by one. In recent years, characterization and heterologous reconstitution of the biosynthetic pathway for several valuable PSM including artemisinic acid, thebaine, colchicine, and baccatin III have been reported. These findings are intriguing and encouraging for researchers focusing on PSM biosynthesis. However, there is still a long way to go. A huge number of biosynthetic steps for PSM remain unknown. Thousands of gene elements responsible for PSM biosynthesis remain uncharacterized. Modern omics technologies, novel gene manipulation approaches, and artificial intelligence technology has expanded our knowledge on PSM biosynthesis and will facilitate the biomanufacture of valuable PSM.
XP: Writing – original draft. NK: Writing – review & editing. CR-L: Writing – review & editing. SC: Writing – review & editing.
The author(s) declare financial support was received for the research, authorship, and/or publication of this article. XP received funding from the Department of Science and Technology of Sichuan Province, PR China (2021ZYD0059; 2022NSFC1551) and the National Natural Science Foundation of China (21708028). CR-L received funding from Tecnológico de Monterrey Challenge-Based Research Funding Program.
The authors wish to thank to all the contributors and the FPS editorial team for their efficient work. We would like to express our deep gratitude to all reviewers for their valuable participation in assuring the scientific quality of this Research Topic.
The authors declare that the research was conducted in the absence of any commercial or financial relationships that could be construed as a potential conflict of interest.
All claims expressed in this article are solely those of the authors and do not necessarily represent those of their affiliated organizations, or those of the publisher, the editors and the reviewers. Any product that may be evaluated in this article, or claim that may be made by its manufacturer, is not guaranteed or endorsed by the publisher.
Afendi, F. M., Okada, T., Yamazaki, M., Hirai-Morita, A., Nakamura, Y., Nakamura, K., et al. (2012). KNApSAcK family databases: integrated metabolite–plant species databases for multifaceted plant research. Plant Cell Physiol. 53, e1. doi: 10.1093/pcp/pcr165
Erb, M., Kliebenstein, D. J. (2020). Plant secondary metabolites as defenses, regulators, and primary metabolites: the blurred functional trichotomy. Plant Physiol. 184, 39–52. doi: 10.1104/pp.20.00433
Li, Y. H., Hirai, M. Y., Nakamura, Y. (2024). Plant metabolomics. J. Exp. Bot. 75, 1651–1653. doi: 10.1093/jxb/erae047
Liu, M. Y., Yang, L., Cai, M. M., Feng, C., Zhao, Z. M., Yang, D. P., et al. (2021). Transcriptome analysis reveals important candidate gene families related to oligosaccharides biosynthesis in Morinda officinalis. Plant Physiol. Biochem. 167, 1061–1071. doi: 10.1016/j.plaphy.2021.09.028
Luo, T. T., Xia, W., Gong, S. F., Mason, A. S., Li, Z. Y., Liu, R., et al. (2020). Identifying vitamin E biosynthesis genes in Elaeis guineensis by genome-wide association study. J. Agric. Food Chem. 68, 678–685. doi: 10.1021/acs.jafc.9b03832
Nasti, R. A., Voytas, D. F. (2021). Attaining the promise of plant gene editing at scale. Proc. Natl. Acad. Sci. U.S.A. 118, e2004846117. doi: 10.1073/pnas.2004846117
Siadjeu, C., Pucker, B. (2023). Medicinal plant genomics. BMC Genomics 24, 429. doi: 10.1186/s12864-023-09542-8
Voelckel, C., Gruenheit, N., Lockhart, P. (2017). Evolutionary transcriptomics and proteomics: Insight into plant adaptation. Trends Plant Sci. 22, 462–471. doi: 10.1016/j.tplants.2017.03.001
Keywords: plant, secondary metabolite, biosynthetic pathway, gene characterization, synthetic biotechnology
Citation: Pu X, Kitaoka N, Rodríguez-López CE and Chen S (2024) Editorial: Plant secondary metabolite biosynthesis. Front. Plant Sci. 15:1477551. doi: 10.3389/fpls.2024.1477551
Received: 08 August 2024; Accepted: 12 August 2024;
Published: 21 August 2024.
Edited and Reviewed by:
Laigeng Li, Chinese Academy of Sciences (CAS), ChinaCopyright © 2024 Pu, Kitaoka, Rodríguez-López and Chen. This is an open-access article distributed under the terms of the Creative Commons Attribution License (CC BY). The use, distribution or reproduction in other forums is permitted, provided the original author(s) and the copyright owner(s) are credited and that the original publication in this journal is cited, in accordance with accepted academic practice. No use, distribution or reproduction is permitted which does not comply with these terms.
*Correspondence: Xiang Pu, cHV4aWFuZ0BzaWNhdS5lZHUuY24=; Shilin Chen, c2xjaGVuQGNkdXRjbS5lZHUuY24=
Disclaimer: All claims expressed in this article are solely those of the authors and do not necessarily represent those of their affiliated organizations, or those of the publisher, the editors and the reviewers. Any product that may be evaluated in this article or claim that may be made by its manufacturer is not guaranteed or endorsed by the publisher.
Research integrity at Frontiers
Learn more about the work of our research integrity team to safeguard the quality of each article we publish.