- Virginia Tech School of Plant and Environmental Sciences, Alson H. Smith Jr. Agricultural Research and Extension Center, Winchester, VA, United States
Ethylene is an important phytohormone that orchestrates a multitude of physiological and biochemical processes regulating fruit ripening, from early maturation to post-harvest. This review offers a comprehensive analysis of ethylene’s multifaceted roles in climacteric fruit ripening, characterized by a pronounced increase in ethylene production and respiration rates. It explores potential genetic and molecular mechanisms underlying ethylene’s action, focusing on key transcription factors, biosynthetic pathway genes, and signal transduction elements crucial for the expression of ripening-related genes. The varied sensitivity and dependency of ripening traits on ethylene are elucidated through studies employing genetic mutations and ethylene inhibitors such as AVG and 1-MCP. Additionally, the modulation of ripening traits by ethylene is influenced by its interaction with other phytohormones, including auxins, abscisic acid, gibberellins, jasmonates, brassinosteroids, and salicylic acid. Pre-harvest fruit drop is intricately linked to ethylene, which triggers enzyme activity in the abscission zone, leading to cell wall degradation and fruit detachment. This review also highlights the potential for applying ethylene-related knowledge in commercial contexts to enhance fruit quality, control pre-harvest drop, and extend shelf life. Future research directions are proposed, advocating for the integration of physiological, genetic, biochemical, and transcriptional insights to further elucidate ethylene’s role in fruit ripening and its interaction with other hormonal pathways.
Highlight
● This review explores the ethylene-dependent and independent regulation of fruit ripening traits like color, firmness, starch metabolism, aroma, and fruit drop at both biochemical and molecular levels.
Introduction
Ethylene is a crucial natural plant hormone that plays a pivotal role in regulating fruit ripening by initiating and coordinating various physiological and biochemical changes from early maturity to post-harvest. It modulates numerous ripening-related attributes such as color (Whale and Singh, 2007), firmness (Tonutti et al., 1996), total soluble solids (TSS) (Singh and Janes, 2001), aroma (Lalel et al., 2003a), respiration (Bower et al., 2002), and storage and shelf life (Xu et al., 2019). Climacteric fruits, including apples, peaches, bananas, and tomatoes, exhibit a substantial increase in ethylene production and respiration rate during ripening (Barry and Giovannoni, 2007). The autocatalytic nature of ethylene production (Yang et al., 1986) in these fruits means that once ethylene synthesis commences, it leads to a self-amplifying increase in production, thereby accelerating the ripening process. Consequently, these fruits can continue to ripen after being harvested. In contrast, non-climacteric fruits such as grapes and strawberries do not display a climacteric rise in ethylene production or respiration (Symons et al., 2012; Pérez-Llorca et al., 2019). These fruits must be harvested at full ripeness, as they do not continue to ripen post-harvest. The ripening of non-climacteric fruits is governed by plant hormones like abscisic acid (ABA), rather than ethylene (Li et al., 2022a).
Ethylene is synthesized from the amino acid methionine through a series of enzymatic reactions involving ACC (1-aminocyclopropane-1-carboxylic acid) synthase (ACS) and ACC oxidase (ACO) (Figure 1). ACS converts S-adenosyl-L-methionine (SAM) into ACC, which is subsequently converted to ethylene gas by ACO (Adams and Yang, 1979; Boller et al., 1979). The increased expression and activity of ACS and ACO genes result in higher ethylene production, thereby initiating and accelerating the ripening process. Ethylene can induce its own synthesis in a positive feedback loop, known as autocatalytic ethylene (Abeles et al., 2012). Ethylene perception occurs at the endoplasmic reticulum membrane, where it initiates a signal transduction cascade that elicits diverse physiological responses (Ju and Chang, 2015). The ethylene signaling pathway involves various components, including ethylene receptors such as ETR1 (Ethylene Response 1), protein kinase CTR1 (Constitutive Triple Response 1), EIN2 (Ethylene Insensitive 2), transcription factors like EIN3 (Ethylene Insensitive 3) and its homologs EILs (Ein3-Like), and ERFs (Ethylene Response Factors) (Figure 1). These components collectively modulate the plant’s response to ethylene and trigger downstream transcriptional reprogramming (Wang et al., 2002; Binder et al., 2004; Merchante et al., 2013). Moreover, mapping the biosynthetic and signal transduction pathways of ethylene provides a critical framework for understanding the multi-layered and complex regulation of ripening traits. This pathway mapping enables researchers to uncover points of interaction and regulation, which are essential for both basic scientific understanding and the development of applied strategies particularly in pre- and post-harvest fruit management.
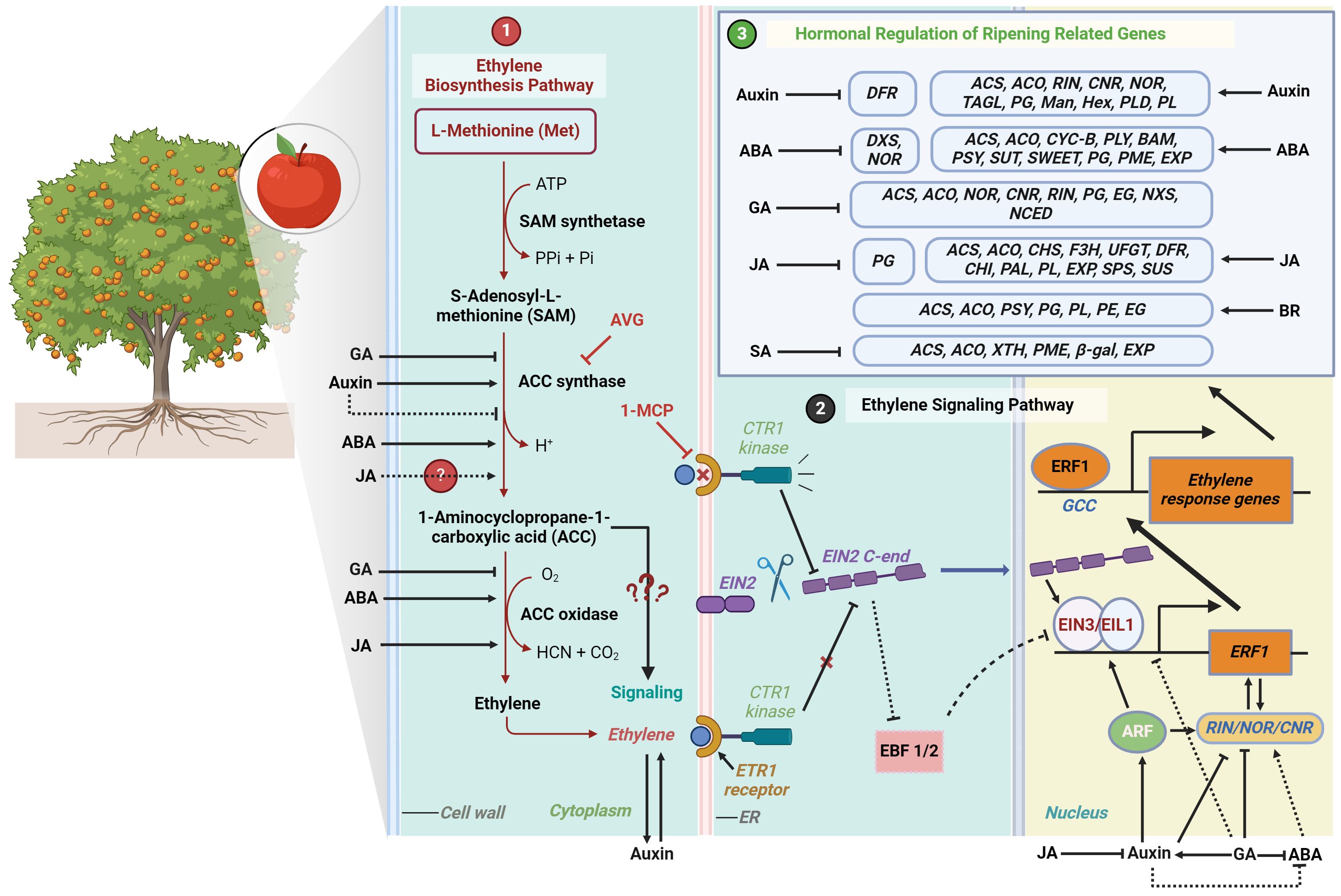
Figure 1. The diagram illustrates the ethylene biosynthesis and signaling pathways in plants, with an emphasis on fruit ripening and related traits modulating genes those further regulated by other hormones. 1) Ethylene biosynthesis starts with L-Methionine, which is converted to S-Adenosyl-L-methionine (SAM) by SAM synthetase using ATP. SAM is then converted to 1-Aminocyclopropane-1-carboxylic acid (ACC) by ACC synthase, a process regulated positively by abscisic acid (ABA) and auxins, and negatively by gibberellins (GA). In the next step, ACC is oxidized by ACC oxidase to produce ethylene, with its production being influenced by ABA and jasmonic acid (JA). 2) Ethylene is perceived by the ETR1 receptor on the endoplasmic reticulum (ER) membrane, initiating a signaling cascade involving CTR1 kinase and EIN2. Cleaved EIN2 translocates to the nucleus, where it stabilizes EIN3/EIL1, leading to the activation of Ethylene Response Factor 1 (ERF1). ERF1 then binds to the GCC box in ethylene response genes, regulating physiological responses such as color changes, softening, sugar accumulation, aroma production, and abscission, mediated by various hormones. ACC production can be inhibited by the application of aminoethoxyvinylglycine (AVG), which suppresses ACC synthase, while the ethylene receptor ETR1 is blocked by 1-Methylcyclopropene (1-MCP), halting ethylene signaling. 3) Other hormones like ABA, GA, Auxin, JA, BR and SA have divergent regulatory effects on ripening and related genes. A solid arrow indicates positive regulation, a solid inhibitor line indicates negative regulation, and a dotted line represents potential interaction.
The role of ethylene in the development of various fruit ripening traits has been elucidated not only through ethylene application and quantification but also through genetic mutations and the use of ethylene inhibitors. Key transcription factors, such as Ripening Inhibitor (RIN), Colorless Non-Ripening (CNR), and Non-Ripening (NOR), play crucial roles in fruit ripening. These transcription factors regulate the expression of genes involved in ripening, and their activity is influenced by ethylene signaling (Liu et al., 2016; Mou et al., 2016). For example, studies using RIN tomato mutants demonstrated that these mutated fruits do not exhibit the typical climacteric rise in ethylene and show delayed ripening (Herner and Sink, 1973). Ethylene can initiate the ripening of mature green fruit and upregulate RIN expression (Li et al., 2020), but RIN-deficient fruit never fully ripens, even with exogenous ethylene, indicating that both RIN and ethylene are required for complete ripening. On the other hand, ethylene inhibitors such as aminoethoxyvinylglycine (AVG) and 1-methylcyclopropene (1-MCP) play negative roles in modulating the ripening process of climacteric fruits. AVG directly inhibits ACC synthase activity, resulting in lower ethylene production in treated fruits (Amrhein and Wenker, 1979; Yu et al., 1979; Yang and Hoffman, 1984) (Figure 1). In contrast, 1-MCP does not directly inhibit ethylene biosynthesis but prevents the action of ethylene by binding to its receptors (Sisler and Serek, 1997; Sisler et al., 1999; Blankenship and Dole, 2003) (Figure 1). Consequently, while ethylene may still be produced in 1-MCP-treated fruits, its effects on ripening are nullified.
The influence of ethylene on various ripening-related traits frequently suggests the hypothesis that ethylene-dependent characteristics may cluster or segregate in a distinct manner during the ripening process. These traits typically exhibit a coordinated sequence of changes, although the degree and nature of their clustering can vary. In the early ripening phase, increased ethylene synthesis initiates changes in cell wall structure, beginning with the solubilization of pectin (Rose et al., 1998; Ponce et al., 2010). Simultaneously, significant chlorophyll degradation and enhanced pigment synthesis result in visible color changes (Whale and Singh, 2007; Su et al., 2015; Chen et al., 2022). During the mid-ripening stage, further breakdown of cell wall components leads to noticeable fruit softening and at the same time aroma volatile compounds starts to synthesize (El Hadi et al., 2013; Tucker et al., 2017; Wang et al., 2018b). Later, cell wall degradation reaches its peak, rendering the fruit very soft, while the characteristic aroma fully develops, and starches are completely converted to sugars (Farcuh et al., 2018), resulting in the fruit’s sweetest phase.
However, ethylene-dependent traits do not always segregate uniformly; they can exhibit distinct patterns influenced by the interplay of other hormones, genetic factors, environmental conditions, and developmental stages (Stepanova et al., 2005; Barry and Giovannoni, 2007; Klee and Giovannoni, 2011; Sobolev et al., 2014; Liu et al., 2020). For instance, in seminal studies by Pech et al. (2008) and Johnston et al. (2009), the authors explored the effects of silencing the ACO gene on ethylene production and ripening in melon and apple, respectively. By reducing ethylene synthesis, they could examine ripening traits without ethylene interference. Both investigations concluded that individual ripening traits, such as color change, volatile production, and softening, exhibit varying sensitivities to and dependencies on ethylene (Pech et al., 2008; Johnston et al., 2009). Specifically, Johnston et al. (2009) demonstrated that early ripening traits, like starch conversion to sugars, showed low ethylene dependency but high sensitivity to low ethylene concentrations. In contrast, traits such as flesh softening and volatile production displayed high ethylene dependency but require higher ethylene concentrations to elicit responses. Not only can ethylene-dependent ripening traits segregate non-uniformly, but research conducted on tomatoes (Murray et al., 1993), bananas (Golding et al., 1998), and melons (Hadfield et al., 2000) using genetic mutations in ethylene biosynthesis and employing ethylene inhibitors has also demonstrated that both ethylene-dependent and ethylene-independent pathways exist for the regulation of ripening traits in climacteric fruits.
In this review, we aim to provide a comprehensive analysis of the multifaceted roles that ethylene plays during the ripening of climacteric fruits. Emphasis will be placed on the diverse fruit ripening traits, elucidating their dependence or independence on ethylene, and exploring the roles of other plant hormones in the ripening process and their potential interactions. We will examine these elements from physiological, genetic, biochemical, and transcriptional perspectives, highlighting the utility of genetic mutations and ethylene inhibitors in addressing key questions. Finally, this article will discuss how this knowledge can be translated into commercial applications and explore future perspectives in this field of research.
Ethylene regulation of fruit ripening traits from molecular, biochemical and physiological perspectives
The correlation between ethylene levels and ripening traits is a fundamental aspect of fruit physiology and post-harvest management. Understanding the biochemical correlation between ethylene and ripening traits involves delving into the molecular and biochemical mechanisms underlying this process. This section discusses how ethylene affects different ripening traits.
Ethylene regulation of fruit coloration
The alteration in fruit coloration is a pivotal indicator of ripeness, predominantly governed by heightened ethylene production in various climacteric fruits. In exemplars such as apples and tomatoes, ethylene production intensifies red pigmentation, whereas in avocados, it transits the green hue to a purple or black due to the augmented concentration of anthocyanins, particularly cyanidin 3-O-glucoside (Dong et al., 1995; Cox et al., 2004; Whale and Singh, 2007). In mangoes, ethylene facilitates chlorophyll degradation by accelerating the activities of chlorophyllase and Mg-dechelatase, and it enhances anthocyanin content by regulating a series of enzymes including phenylalanine ammonia-lyase (PAL), chalcone isomerase (CHI), dihydroflavonol 4-reductase (DFR), and UDP-glucose: flavonoid 3-O-glucosyl transferase (UFGT) (Chen et al., 2022). This leads to the manifestation of the characteristic red, yellow, or orange colors in ripe mangoes. Conversely, in fruits such as plums, skin color development is not directly influenced by ethylene; rather, ethylene acts as a catalyst, expediting and synchronizing pigment production and chlorophyll degradation (Abdi et al., 1998). The intensity and timing of these color changes are modulated by ethylene signaling associated with system-2 ethylene, known for its autocatalytic nature (Huang et al., 2022).
At the transcriptional level, ethylene significantly influences the expression of genes responsible for the biochemical transformations that lead to color change in fruits. In apples, ethylene enhances anthocyanin biosynthesis and fruit coloration by inducing the expression of MYB transcription factors. Specifically, MdMYB1 is crucial in regulating anthocyanin biosynthesis and accumulation. MdEIL1 directly activates MdMYB1 expression, which in turn promotes anthocyanin accumulation (An et al., 2018). MdMYB1 also activates MdERF3, further enhancing ethylene production (An et al., 2018). This regulatory loop is modulated by MdMYB17, which represses MdMYB1 and MdEIL1 expression (Wang et al., 2022b). Additionally, ethylene-activated MdPUB24 ubiquitinates MdBEL7, thereby upregulating the expression of chlorophyll catabolic genes such as MdCLH (Chlorophyllase), MdPPH2 (Pheophytinase), and MdRCCR2 (Red Chl Catabolite Reductase), leading to chlorophyll degradation (Wei et al., 2021). The never-ripe (Nr) tomato mutant, which is insensitive to ethylene due to a mutation in the ethylene receptor gene (ETR1), provides further evidence of ethylene’s role in color change, as both coloration and softening are impaired in this mutant (Wilkinson et al., 1995).
Investigations utilizing ethylene inhibitors, such as AVG and 1-MCP, provide robust evidence on ethylene’s regulation of chlorophyll degradation and the synthesis of pigments like carotenoids and anthocyanins in various fruits (Bangerth, 1978; Clayton et al., 2000; Feng et al., 2000; Phan-Thien et al., 2004; Whale et al., 2008; Lee et al., 2010; Lv et al., 2020; Zhang et al., 2020). For instance, 1-MCP treatment in Chinese pears delays chlorophyll degradation by inhibiting ethylene production and suppressing the expression of genes such as Pheophorbide A Oxygenase (PAO), Non-Yellow Coloring (NYC), Nyc1-Like (NOL), and Stay-Green1 (SGR1) (Cheng et al., 2012). Similarly, in plums, 1-MCP delays anthocyanin accumulation by suppressing the expression of biosynthesis-related genes, including Phenylalanine Ammonia-Lyase (PsPAL), Chalcone Synthase (PsCHS), Chalcone Isomerase (PsCHI), Flavanone 3-Hydroxylase (PsF3H), Dihydroflavonol 4-Reductase (PsDFR), Anthocyanidin Synthase (PsANS), Udp-Glucose: Flavonoid 3-O-Glucosyl Transferase (PsUFGT), and the transcription factor PsMYB10 (Xu et al., 2020). Moreover, AVG delays red coloration in apples, with its effect being dose-dependent and influenced by climatic conditions (Greene, 2005; Yildiz et al., 2012; Liu et al., 2022). Collectively, these findings underscore ethylene’s critical role as a regulator of color change in climacteric fruits, orchestrating complex biochemical pathways and gene expression mechanisms. Genetic mutations, biochemical analyses, gene expression studies, and the application of ethylene inhibitors all illuminate the multifaceted role of ethylene in this essential ripening trait.
Ethylene regulation of fruit softening and firmness
Ethylene plays a crucial role in fruit softening and firmness by activating cell wall-degrading enzymes, such as polygalacturonase, which hydrolyze pectin in the cell walls, leading to fruit softening. For instance, the stony hard (hd) peach mutant, which lacks ethylene production likely due to allelic variation within the first intron of the PpYUC11-like gene (a YUCCA-like auxin-biosynthesis gene), maintains firm flesh but softens when treated with exogenous ethylene (Hayama et al., 2006; Cirilli et al., 2018). Similarly, kiwifruit lines with suppressed ethylene production due to the knockdown of the ACO1 gene retain their firmness but soften upon exogenous ethylene treatment (Atkinson et al., 2011). In tomatoes, an abnormally ripening ‘Alcobaca’ mutant that carries a third allele at the nor locus, characterized by reduced ethylene production, shows increased storability and slower softening during storage (Lobo et al., 1984; Mutschler, 1984; Lu et al., 1993). Consequently, ethylene negatively impacts fruit firmness and shelf life, with ethylene-suppressed fruits being firmer and having a longer shelf life (Dandekar et al., 2004).
Ethylene also regulates enzymes responsible for the catabolism of cellulose and pectin, resulting in the dismantling of polysaccharide networks (Prasanna et al., 2007). Various cell wall-related genes, such as Polygalacturonase1 (PG1), β-Galactosidase1 (BGAL1), Xyloglucan Endotransglucosylase/hydrolase1/2 (XTH1/2), and Expansins (EXPs), exhibit differential sensitivities to and dependencies on ethylene (Johnston et al., 2009; Ireland et al., 2014). For example, the expression of PG genes, which are responsible for cell wall degradation and fruit softening, is regulated by both ethylene-dependent and ethylene-independent factors (Oeller et al., 1991; Pech et al., 2008).
Ethylene biosynthetic inhibitors, such as AVG, delay softening in various climacteric fruits, including peaches (Hayama et al., 2008), apples (Kon et al., 2023), pears (He et al., 2023), and nectarines (McGlasson et al., 2005). Conversely, exogenous ethylene promotes the expression of cell wall-degrading genes, particularly PG in apples (Tacken et al., 2010), and activity of enzymes like PG, pectin esterase (PE), and cellulase in guavas (Abu-Goukh and Bashir, 2003), which reduce firmness. In apple and peach, AVG-treated fruits retain firmness longer by reducing PG activity in fruit abscission zones (Wang and Dilley, 2001; Belding and Lokaj, 2002; Zhu et al., 2008). Similarly, apple fruits treated with 1-MCP remain firmer during storage and transportation (Watkins et al., 2000; Win et al., 2019, 2021). Additionally, in papaya, 1-MCP treatment delays ripening and maintain normal characteristics when applied for a short-term (Zhu et al., 2019).
Ethylene’s influence on sugar metabolism and accumulation
The role of ethylene in sugar metabolism and accumulation is multifaceted and varies among different types of fruits, exhibiting diverse regulatory mechanisms. In Japanese plums, banana and blueberry, ethylene significantly influences sugar metabolism by reducing sucrose catabolism and promoting sucrose biosynthesis (Farcuh et al., 2018; Cordenunsi-Lysenko et al., 2019; Wang et al., 2020b). Conversely, antisense ACO melons demonstrate higher soluble solids content despite a lower ripeness index (Martínez-Madrid et al., 2002). These melons exhibit suppressed ethylene biosynthesis, show reduced ethylene production by up to 97.7% compared to wild-type fruit (Martínez-Madrid et al., 2002). Similarly, in tomatoes, the metabolism of citrate, malate, and starch operates independently of ethylene (Jeffery et al., 1984). In kiwifruit, low temperatures during storage can stimulate sugar accumulation without triggering ethylene and ethylene-dependent aroma volatiles, indicating that ethylene signaling is inactive during this process (Mitalo et al., 2019). The transcript levels of the MADS-box transcription factor AcMADS2, which regulates the starch degradation gene β-Amylase2 (Acβ-AMY2) and the sucrose metabolism gene Invertase (AcINV3-1), increased only in response to low temperature (5°C). Treatment with propylene, an ethylene analog, did not affect these transcript levels (Mitalo et al., 2019).
Ethylene plays a critical role in the conversion of starches to sugars in fruits, whereas ethylene inhibitors tend to delay this process, resulting in higher starch content and a slower increase in sugars (Yuan and Carbaugh, 2007; Yildiz et al., 2012; Brackmann et al., 2015; Malladi et al., 2023). Specifically, amylase activity in AVG-treated apples is reduced during the initial stages of starch mobilization, while starch phosphorylase activity significantly increases during the later stages and remains unaffected (Silverman et al., 2004). Similarly, 1-MCP-treated apples experience slower starch breakdown, enabling delayed harvesting without compromising quality (Tomala et al., 2020). In bananas, the levels of β-amylase protein, responsible for hydrolyzing the α-1,4-glucosidic linkages in starch, are nearly undetectable in 1-MCP-treated fruit (do Nascimento et al., 2006). Conversely, AVG does not alter the levels of amylopectin, fructose, malate, ascorbate, and citrate in apples (Silverman et al., 2004), while 1-MCP increases the activity of α-1,4-glucan-phosphorylase in bananas. This enzyme, essential for starch synthesis and degradation, suggests that the absence of ethylene perception positively affects phosphorylase activity (Mainardi et al., 2006). Collectively, these findings indicate that ethylene is not the sole regulator of carbohydrate metabolism during fruit ripening; other pathways may also play a significant role in starch degradation and sugar accumulation.
Ethylene regulation of aroma development in fruits
Aroma development is a critical trait influenced by ethylene and intricately linked to ethylene levels. Elevated ethylene production enhances the synthesis of aroma volatile compounds (Zhang and Jia, 2005). Post-harvest applications of acetaldehyde and ethanol, both associated with ethylene, improve the sensory quality of tomatoes and pears (Paz et al., 1982). Further research confirms that ethylene-dependent processes significantly contribute to aroma production, as observed in Charentais cantaloupe melons (Pech et al., 2001; Bauchot et al., 2002). In these melons, reduced ethylene production via ACO antisense mRNA expression delays ripening and decreases total volatile composition (Bauchot et al., 1998). Similarly, genetic modifications to suppress ethylene biosynthesis by silencing ACS and ACO genes in apples result in substantial reductions in aroma volatile esters (Dandekar et al., 2004). In Greensleeves apples with high suppression of ethylene biosynthesis, Defilippi et al. (2005) found that levels of aroma volatile-related enzymes, including alcohol acyltransferase (AAT), alcohol dehydrogenase (ADH), and lipoxygenase (LOX), along with amino acids and fatty acids as aroma volatile precursors, varied between peel and flesh tissues. Generally, volatile production, enzyme activity levels, and precursor availability were higher in the peel than in the flesh and were differentially affected by ethylene regulation. AAT enzyme activity showed a clear pattern consistent with ethylene regulation. However, it should be noted that some upstream steps in the aroma biosynthetic pathway, such as the production of alcohols and aldehydes, remained unaffected in the study by Dandekar et al. (2004), indicating that ethylene does not control every aspect of flavor production. Additionally, Tsantili and Knee (1991) found that storing apples in low-ethylene conditions for extended periods did not impair the synthesis of aroma volatiles, suggesting that ethylene may not be the sole contributor to the production of these compounds.
Given that ethylene promotes the biosynthesis of aroma volatile compounds, ethylene inhibitors can significantly impact this process. Fruits treated with 1-MCP demonstrated inhibited production of volatile compounds such as 2-methylbutyl acetate, butyl acetate, hexyl acetate, and butanol, which contribute to flavor in apples (Fan and Mattheis, 2001; Marin et al., 2009). At the transcriptional level, ethylene treatment upregulates the expression of genes involved in volatile biosynthesis in apple fruit, including branched-chain amino acid aminotransferase (BCAT), aromatic amino acid aminotransferase (ArAT), amino acid decarboxylases (AADC), and various enzymes related to fatty acid synthesis and metabolism. Conversely, treatment with 1-MCP generally produces opposite effects on these genes, providing further evidence that their regulation is ethylene-dependent (Yang et al., 2016).
Ethylene regulation of respiration and shelf life in climacteric fruits
Changes in respiration rate and ethylene production in climacteric fruits follow a typical pattern, with the peak of ethylene production coinciding with an increase in respiration (Abbas and Fandi, 2002). The interaction between ethylene and respiration significantly impacts the storage and shelf life of fruits. High ethylene levels induced by substances like ethephon can accelerate the respiration rate and ethylene production during ripening (Lalel et al., 2003a). Maintaining lower ethylene levels can lead to more uniform ripening in fruits such as plums (Rozo-Romero et al., 2015). However, in mangoes, research has shown that respiration can remain relatively constant regardless of the absence or low levels (0.005 μL/L) of endogenous ethylene when treated with short-term ultraviolet-C light (180-280 nm) during storage (Pristijono et al., 2019). Although the molecular links between ethylene and respiration have not been extensively examined, recent studies suggest that the alternative oxidase (AOX) respiratory pathway may play a crucial role in mediating the crosstalk between ethylene response, carbon metabolism, ATP production, and reactive oxygen species (ROS) signaling during climacteric ripening (Hewitt and Dhingra, 2020). Furthermore, in tomatoes, the relationship between respiration rate and ethylene production varies among different fruit sizes and cultivars, with small-fruited types generally exhibiting higher respiration rates and ethylene production compared to medium and large-fruited varieties (Ustun et al., 2023).
Controlled ethylene levels can reduce respiration rates, further delaying starch breakdown, resulting in firmer and less sweet fruits during storage, thereby extending shelf life (Thammawong and Arakawa, 2007). Paull and Chen (1983) found a positive correlation between polygalacturonase and xylanase activity and respiration in papaya. Inhibiting ethylene biosynthesis with compounds like AVG can reduce the climacteric rise in respiration associated with ripening, thereby slowing down the ripening process (Whale et al., 2008; Çetinbaş et al., 2012). Similarly, post-harvest treatments such as controlled atmosphere storage in low O2 concentration (0.5-1.0%) and the use of ethylene inhibitors like 1-MCP are employed to manipulate ethylene levels and prolong the shelf life of pears (Chen et al., 1981; Argenta et al., 2003). In apples, 1-MCP effectively delays the climacteric peak of respiration and ethylene production (Lv et al., 2020). Additionally, the application of chlorine dioxide (ClO2) effectively reduces respiration rates in ‘Hami’ melon fruit, combined with a downregulation of ethylene-biosynthesis genes such as CmACS2, CmACO1, and CmACO3 (Guo et al., 2013). These studies indicate that controlling ethylene levels with inhibitors can be effective in extending the shelf life of fruits by reducing respiration rates.
Roles of other plant hormones affecting ripening
Ethylene has historically been recognized as the principal regulator of ripening in climacteric fruits. However, over the past two decades, there has been a significant accumulation of knowledge regarding the intricate interplay between ethylene and other phytohormones during the ripening process. This section delves into the influence of additional plant hormones such as auxin, ABA, gibberellins (GA), and jasmonates (JA) on various ripening characteristics.
Auxins
Auxin, particularly indole-3-acetic acid (IAA), plays a pivotal role in the initial stages of fruit development. Auxin biosynthesis and signaling pathways were reviewed by Gomes and Scortecci (2021). In climacteric fruits such as apples and tomatoes, auxin is essential for early fruit development and fruit set (Shin et al., 2016; Zhang et al., 2021). During the transition to ripening, auxin levels rise in climacteric fruits, thereby initiating the ripening processes (Trainotti et al., 2007). By the late stages of ripening, auxin concentrations diminish, facilitating the complete maturation and senescence of the fruit, as observed in peaches (Trainotti et al., 2007). Auxin is also known to enhance ethylene production in numerous horticultural crops. Indeed, the promoter regions of several auxin response factors (ARFs) contain cis-regulatory elements for both auxin and ethylene, indicating that these ARFs may be regulated by both hormones (Muday et al., 2012; Zouine et al., 2014). Recent research has also shown that auxin induces ethylene biosynthesis in apple fruits by activating the expression of the auxin response factor MdARF5 (Figure 1). MdARF5 binds to the promoter of MdERF2, which encodes a transcription factor in the ethylene signaling pathway, as well as to the promoters of two ACC synthase genes, MdACS3a and MdACS1, and an ACC oxidase gene, MdACO1 (Yue et al., 2020). Similarly, in tomatoes, Auxin Response Factor2 (SlARF2) functions as a positive regulator of ripening, despite being a transcriptional repressor (Hao, 2014). Fruits with reduced expression of SlARF2 produce less climacteric ethylene and exhibit down-regulation of key ripening genes such as RIN, CNR, NOR, and TAGL1 (Tomato Agamous-Like 1) (Hao et al., 2015). Mechanistically, the ethylene response factor SlERF.D7 directly targets and positively regulates SlARF2A and SlARF2B, thereby integrating auxin and ethylene signaling pathways in the control of ripening (Gambhir et al., 2022). Additionally, silencing Auxin Response Factor4 (ARF4), which is strongly expressed in tomato pericarp, resulted in increased accumulation of starch and chlorophyll content. This finding suggests that auxin signaling is involved in the regulation of chloroplastic activity and sugar metabolism in the fruit (Jones et al., 2002; Sagar et al., 2013). The elevated starch content in developing fruits of SlARF4 down-regulated lines correlated with the up-regulation of genes and enzyme activities involved in starch biosynthesis, implying negative regulation by SlARF4 (Sagar et al., 2013). Notably, the application of exogenous auxin has been found to influence the expression levels of genes such as PpPG2, thereby reducing fruit firmness in peach fruits (Tatsuki et al., 2013).
Auxin has also been observed to exert negative effects on various ripening traits. For example, auxins tend to suppress the climacteric rise in respiration rate by downregulating numerous genes involved in the tricarboxylic acid (TCA) cycle in tomatoes (Frenkel and Dyck, 1973; Li et al., 2016; Yue et al., 2020). Additionally, auxin inhibits the synthesis of pigments such as anthocyanin in apples, with the degree of inhibition increasing with higher doses (Ji et al., 2015). (Wang et al., 2018a) further elucidated that the auxin response factor MdARF13 acts as a negative regulator of anthocyanin biosynthesis via the Aux/IAA–ARF signaling pathway, directly binding to the promoter of MdDFR, a structural gene in the anthocyanin biosynthetic pathway in apples. Similarly, Wu et al. (2018a) discovered that exogenous auxin negatively impacts the accumulation of aroma volatiles during tomato fruit ripening. In a separate study on Japanese plums (P. salicina L.), it was found that auxin advances ripening independently of ethylene action by modulating cell wall genes encoding α-Mannosidase (α-Man), β-d-N-Acetylhexosaminidase (β-Hex), Phospholipase d-α (PLD), and Pectate Lyase (PL) (El-Sharkawy et al., 2016) (Figure 1). These studies collectively suggest that auxin’s role in regulating the ripening of climacteric fruits can be mediated through the induction of ethylene production, but it may also function independently to regulate specific ripening traits, particularly during the later stages of fruit ripening.
Abscisic acid
Abscisic acid (ABA) has been demonstrated to promote fruit ripening, enhancing color change and accelerating sugar accumulation in fruits such as date palm and banana (Jiang et al., 2000; Elbar et al., 2023). In kiwifruit, ABA treatment increases the production of esters and other aroma compounds by enhancing the activity of related enzymes, particularly alcohol acyltransferase (AAT), branched amino acid transaminase (BCAT), and hydroperoxide lyase (HPL) (Han et al., 2022). ABA accelerates both ethylene production and respiration in bananas (Jiang et al., 2000), and the application of ABA accelerates ethylene biosynthesis by promoting the activities of ethylene biosynthesis enzymes, ACC synthase and ACC oxidase, and the accumulation of ACC in mango and tomato (Zhang et al., 2009; Zaharah et al., 2013) (Figure 1). At the genetic level, overexpressing the persimmon gene DkBG1, which encodes β-glucosidase 1 responsible for cleaving ABA-glucose esters to release active ABA, in tomato plants hastened fruit ripening by 3-4 days (Liang et al., 2020). Furthermore, these transgenic tomatoes exhibited modified expression of the ripening regulator gene, NOR, and its downstream targets, resulting in noticeable changes in fruit coloration by downregulating the expression of 1-Deoxy-D-Xylulose-5-Phosphate Synthase (DXS1) and Phytoene Synthase (PSY1), while significantly upregulating the expression of lycopene β-Cyclase (CYC-B) (Liang et al., 2020) (Figure 1). The interaction between ethylene and ABA during tomato fruit ripening is supported by the finding that SlAREB1, an ABA-responsive transcription factor, directly activates NOR (Mou et al., 2018). Additionally, transient overexpression of SlAREB1 in tomato fruits results in increased levels of several downstream ethylene biosynthetic genes, such as SlACS2, SlACS4, and SlACO1, thereby linking ABA signaling to ethylene production and ripening (Mou et al., 2018). Abscisic acid (ABA) biosynthesis and signaling mechanisms were reviewed by Hauser et al. (2011).
The role of ABA in ripening is contingent upon the induction of various transcription factors and is frequently modulated by interactions with inhibitors. In mango, (Wu et al., 2022) discovered that ABA is closely linked to ripening, where ABA-responsive transcription factors such as long hypocotyl5 (MiHY5) directly regulate ripening-related genes like Pectate Lyase (MiPLY8), Beta-Amylase (MiBAM9), and Phytoene Synthase (MiPSY) (Figure 1). Another study revealed that the ABA-responsive transcription factor MdAREB2 directly activates the expression of sugar transporter genes, specifically Tonoplast Monosaccharide Transporters MdTMT1 and Sucrose Transporter MdSUT2, to enhance soluble sugar accumulation in apple (Ma et al., 2017). Additionally, the transcription factor MdWRKY9, induced by ABA, positively regulates the expression of the apple SWEET gene MdSWEET9b, a sucrose transporter gene, thereby promoting sugar accumulation in apple fruits (Zhang et al., 2023a).
ABA also influences fruit firmness by differentially affecting the expression of cell wall-related genes such as PG, PME, EXP1, and EXP2, thereby modulating the ripening process depending on the developmental stage (Soto et al., 2013) (Figure 1). In tomatoes, the key enzyme 9-cis-epoxycarotenoid dioxygenase1 (LeNCED1), which initiates ABA biosynthesis at the onset of fruit ripening, acts as a potential inducer, and treatment with the ABA inhibitor nordihydroguaiaretic acid delayed fruit ripening and softening (Zhang et al., 2009). Interestingly, the ABA-induced tomato ripening was not observed in fruits treated with 1-MCP, indicating that ABA’s stimulation of ripening progress is at least partially dependent on ethylene signaling (Mou et al., 2016). Overall, most studies on the role of ABA in the ripening of climacteric fruits suggest that ABA acts as a positive regulator of many ripening traits, primarily through its interaction with ethylene.
Gibberellins
Gibberellins (GA) play a negative role in fruit ripening, delaying the ripening process by inhibiting ethylene production (Chen et al., 2020) and regulating the expression of ripening related genes NOR, CNR and RIN (Li et al., 2019) (Figure 1). GA biosynthesis and its regulation were reviewed by Hedden and Thomas (2012). In mango and nectarine, GA3 is responsible for the delay in chlorophyll degradation and decreases the total carotenoid content including anthocyanin during ripening and storage (Khader, 1991; Zilkah et al., 1997). Gibberellins can also delay the increase in TSS by prolonging the fruit development stage. In banana, GA3 slows down the onset of starch degradation by delaying starch phosphorylase activity and delays sucrose accumulation affecting sucrose phosphate synthase activity by at least 2 days (da Mota et al., 2002; Rossetto et al., 2003). GA3 also delays cell wall modification related to fruit softening. These changes involve inhibition of the breakdown of the middle lamella, separation of the plasmalemma from the cell wall, increased structural integrity of the primary cell wall, decreased solubilization of pectic polymers, loss of neutral sugars (arabinose and galactose), and lower activities of enzymes PG and endo-1,4-β-glucanase (EG) (Ben-Arie et al., 1996). Moreover, gibberellins delay the climacteric rise by maintaining a lower respiration rate (Nakano et al., 1997).
The role of gibberellins in ripening further explored by Wu et al. (2024) by overexpressing the gibberellin synthesis gene SlGA3ox2 specifically in fruit tissues. The transgenic tomato fruits exhibited delayed fruit ripening, whereas treatment with the GA biosynthesis inhibitor paclobutrazol (PAC) accelerated their fruit ripening (Wu et al., 2024). The involvement of GA in the repression of fruit ripening may occur through the modulation of auxin metabolism and/or signal transduction since many genes related to auxin biosynthesis or signaling pathways were altered in GA-treated fruits (Wu et al., 2024). This hypothesis was previously investigated by Park and Malka (2022) where they showed that GA delays metabolic shifts during fruit ripening by inducing auxin signaling in tomato. However, the effect of auxin on GA-responses seems to be species/organ/developmental stage-dependent (Sun and Gubler, 2004). Moreover, in tomato fruits, GAs downregulate Neoxanthine Synthase (NXS) and 9-Cis-Epoxycarotenoid Dioxygenase (NCED), ACS and ACO genes encoding the biosynthesis of ABA and ethylene (Obroucheva, 2014) further underscored it’s negative role in ripening (Figure 1).
Jasmonates
Jasmonates (JAs), key phytohormones associated with stress responses, exhibit a multifaceted role in fruit ripening, acting as either promoters or inhibitors depending on the context and fruit type. JAs are known to activate ethylene biosynthesis genes, thereby enhancing the climacteric rise in ethylene production (Kondo et al., 2007; Li et al., 2017). The application of n-propyl dihydrojasmonate (PDJ), a JA derivative, has been shown to increase the expression of ACS1 and ACO1 genes in the skin of tomatoes, apples, and pears at the pre-climacteric stage, while decreasing ACS1 mRNA accumulation during the climacteric stage (Fan et al., 1998; Kondo et al., 2007; Kondo, 2010). Additionally, exogenous JAs promote color change in a concentration- and time-dependent manner during the onset of ripening in apples. For ‘Cripps Pink’ apples, a single pre-harvest spray of methyl jasmonate (MeJA), another derivative of JA, at the optimal time is more effective than multiple applications in enhancing red blush and export-grade fruit quality (Shafiq et al., 2013). Similarly, PDJ application in ‘Gala’ and ‘Braeburn’ apples shows optimal effects on coloration when applied about two weeks before harvest (Atay, 2015). JA may act also as an inhibitor. While auxin acts as a positive regulator of ripening, exogenous JA generally down-regulated genes associated with auxin signal transduction in apples (Feng et al., 2017). A review by Han (2017) provides an in-depth examination of the biosynthesis and signaling pathways of JAs.
At the transcriptional level, MeJA enhances the expression of several structural genes involved in anthocyanin biosynthesis, including PpCHS, PpUFGT, PpF3H, PpDFR, PpCHI, and PpPAL, as well as the transcription factor PpMYB10 during peach fruit ripening (Wei et al., 2017) (Figure 1). In red Chinese pear fruits, JAs have been found to induce anthocyanin accumulation through the regulation of transcription factors such as PpMYB10, PpMYB114, and a basic helix-loop-helix (bHLH), independent of ethylene (Ni et al., 2020).
JAs also regulate other fruit quality attributes such as fruit firmness, soluble solids content, flavor, and aroma. In peaches, MeJA increases the expression of cell wall-associated genes, such as PpPL, PpEXP1, and Ppcel1 (Cellulase), while decreasing the expression and enzymatic activity of the PpPG gene (Ziosi et al., 2008a; Wei et al., 2017) (Figure 1). Conversely, in apples, the expression of both MdPL and MdPG was significantly downregulated when treated with MeJA, leading to prolonged firmness (Ding et al., 2024). Jasmonates influence TSS by modulating sugar metabolism and transport. JA-treated Japanese plums and tomatoes exhibited higher TSS levels, total antioxidant activity, and total phenolics (Khan and Singh, 2007; Kucuker et al., 2014; Tao et al., 2022). In tomatoes, JA treatment increased the expression of genes related to sugar metabolism, such as Amylase, Sucrose Phosphate Synthase (SPS), and Sucrose Synthase (SUS), thereby enhancing sugar accumulation at optimal concentrations (Li et al., 2022b). The application of MeJA resulted in higher levels of fatty acids and a notable increase in total aroma volatiles, including monoterpenes, sesquiterpenes, aromatics, norisoprenoids, alcohols, and esters in the pulp of mango fruit (Lalel et al., 2003b). Lalel et al. (2003b) also found that MeJA increased the respiration rate at the beginning of ripening but did not affect the respiration rate at the climacteric stage in mango. In contrast, in apples, MeJA reduced the respiration rate during the later stages of storage (Ding et al., 2024).
Aminocyclopropane carboxylic acid
Aminocyclopropane carboxylic acid (ACC) is a direct precursor of ethylene. Although it was first identified in plants in the 1950s (Burroughs, 1957), recent evidence has shown that ACC functions as a signaling molecule in plants independently of ethylene (Polko and Kieber, 2019). ACC affects early vegetative development and root elongation even when ethylene signaling is blocked by 1-MCP (Tsang et al., 2011; Vanderstraeten et al., 2019). In tomato, ACC promotes pollen tube growth independently of ethylene receptors, as demonstrated using the ethylene-insensitive Never Ripe mutant and 1-MCP treatment (Althiab-Almasaud et al., 2021). ACC’s independent activity is also evident when ethylene biosynthesis is inhibited by AVG (Tsang et al., 2011; Althiab-Almasaud et al., 2021).
In Arabidopsis, the ein3 eil1 mutant is completely insensitive to ethylene and shows no response to ACC (Alonso et al., 2003). However, Tsang et al. (2011) observed that short-term ACC treatment induced the shortening of trichoblasts in ein3 eil1 mutants in Arabidopsis roots. This growth inhibition was absent when combined with α-(phenylethyl-2-one)-indole-3-acetic acid (PEO-IAA), an auxin receptor antagonist, indicating that ACC signaling inhibits root elongation independently of ethylene, and auxin signaling is necessary for ACC signaling. Additionally, previous research indicated that ACC synthase is upregulated earlier in fruit ripening compared to ACC oxidase (Sitrit et al., 1986), suggesting that ACC may play a role in initiating the ripening process independently of ethylene. Due to the close relationship between ACC and ethylene, the exact extent to which their signaling pathways overlap or diverge remains to be fully elucidated (Figure 1).
Brassinosteroids
Brassinosteroids (BRs) are steroid plant hormones that play key roles in plant development and defense. Regulation of brassinosteroid signaling and biosynthesis pathways were reviewed by Ye et al. (2011). BRs significantly promotes ethylene-mediated fruit ripening by upregulating ethylene biosynthesis genes in various fruits such as tomato and persimmon. In tomatoes, treatment with brassinolide (BL), the most active BR, increases the expression of ethylene biosynthesis genes ACS2, ACS4, ACO1, ACO4, and the key carotenoid biosynthesis gene PSY1 (Zhu et al., 2015). Delving deeper, the overexpression of the Brassinazole Resistant1 (BZR1) gene, a downstream transcription factor of BR signaling, in tomato enhances fruit lycopene content by directly activating the transcription of ACO1, ACO3, and PSY1 genes (Sang et al., 2022; Meng et al., 2023). Conversely, knocking out BZR1 inhibits ripening through transcriptome reprogramming at the onset of ripening (Meng et al., 2023).
The story doesn’t end there. Knocking out Brassinosteroid-Insensitive2 (SlBIN2), which acts as a negative regulator upstream of SlBZR1 in BR signaling, accelerates fruit ripening and boosts carotenoid accumulation (Meng et al., 2023). Additionally, overexpression of the BR synthesis gene Dwarf (DWF), which plays a key role in catalyzing bioactive BRs like castasterone (CS), results in accelerated fruit softening, increased lycopene synthesis, and elevated ethylene levels in DWF-OE transgenic tomato plants (Sang et al., 2022). Furthermore, treating tomato plants with exogenous BR or enhancing endogenous BR levels by overexpressing the BR biosynthetic gene SlCYP90B3 leads to an ethylene production surge, propelling fruit ripening forward, while the application of 1-MCP stifles this carotenoid accumulation (Sang et al., 2022; Hu et al., 2020).
In persimmon, the application of the brassinosteroid 24-epibrassinolide (EBR) increases the transcripts of DkACO2, DkACS1, and DkACS2, and upregulates several cell wall-modifying genes like DkPG1, DkPL1, DkPE2, and DkEG1, while treatment with brassinazole (Brz), a BR biosynthesis inhibitor, delays ripening (He et al., 2018). These findings underscore the pivotal role of BRs in regulating fruit ripening by acting through ethylene biosynthesis and signaling pathways.
Salicylic acid
Salicylic acid (SA), a phytohormone well-known for inducing immune responses to pathogen attacks in plants, also plays a crucial role in inhibiting fruit ripening processes. In bananas, SA treatment results in reduced sugar content, lower invertase activity, decreased respiration rate, and less fruit softening. This is particularly evident in the reduced activity of major cell wall-degrading enzymes such as cellulase, polygalacturonase, and xylanase (Srivastava and Dwivedi, 2000). Additionally, acetylsalicylic acid (ASA), a derivative of SA, has been found to inhibit the rise in LOX activity and superoxide free radical production (Zhang et al., 2003; Mo et al., 2008). The positive correlation between lipoxygenase (LOX) activity and ethylene production in fruit tissue has already been demonstrated (Marcelle, 1991). Moreover, ASA reduces ACS and ACO activities in kiwifruit, leading to decreased ethylene biosynthesis, thereby delaying ethylene production and ripening (Zhang et al., 2003). Aghdam et al. (2010) also demonstrated that MeSA, another derivative of SA, inhibits ethylene production, decreases total soluble solids (TSS), and prevents fruit flesh softening of kiwifruits, thereby maintaining firmness and ascorbic acid content during storage. SA biosynthesis and signaling mechanisms were reviewed by Peng et al. (2021).
A recent study on pears (Pyrus pyrifolia Nakai) a transcriptomic analysis of SA-treated fruits showed the downregulation of genes such as XTH, PME, β-gal, and EXP, which inhibits firmness loss and cell wall degradation (Zhang et al., 2023b)(Figure 1). Along the same vein, another study on pears revealed that SA downregulated the expression of ethylene-responsive EIN3a but upregulated it in response to ethylene, auxin, and glucose. SA treatment increased the levels of both free and conjugated SA while dramatically reducing ethylene and auxin (indole-3-acetic acid, IAA) content during fruit senescence (Xu et al., 2023).
The effect of SA can vary widely based on concentration and timing of application. For example, in tomatoes, Ding and Wang (2003) found that low concentrations of methyl salicylate (MeSA), such as 0.1 mM at the mature green stage and 0.01 mM at the breaker stage, enhanced color development, ethylene production, and respiration. However, a higher concentration (0.5 mM) of MeSA inhibited these processes by reducing ACS2 and ACS4 transcript accumulation and delaying ACO1 accumulation (Ding and Wang, 2003). These findings highlight the nuanced role of SA and its derivatives in modulating fruit ripening, offering potential strategies for managing fruit quality and extending shelf life.
Relationship between ethylene and pre-harvest fruit drop
The relationship between fruit ripening and pre-harvest drop is deeply rooted in enzymatic actions, genetic factors, and genomic adaptations. During the ripening of climacteric fruits, enzymes such as expansin, cellulase and PG become more active. These enzymes degrade the cell walls within the abscission zone- a specific layer of cells where the fruit attaches to the stem. The activity of these enzymes increases as the fruit approaches ripeness (Yu-fa et al., 1982; Kondo and Takahashi, 1989; Malladi, 2020). This increase is correlated with a higher incidence of fruit softening and pre-harvest drop (Bonghi et al., 1992; Wakasa et al., 2003; Li and Yuan, 2008). Expansins modulate the loosening of the cell wall by disrupting non-covalent bonds between cellulose and hemicellulose, allowing other enzymes to access and degrade these components more effectively (Cosgrove, 2000). PGs break down pectin, a polysaccharide that acts as a glue holding cells together. The degradation of pectin facilitates cell separation at the abscission zone, enhancing the likelihood of fruit drop (Hadfield et al., 1998; Bonnin and Pelloux, 2020). Cellulases hydrolyze cellulose, a major component of the cell wall. In the abscission zone, increased cellulase activity results in the degradation of cell wall cellulose (Abeles, 1969; Tonutti et al., 1995; Li et al., 2010; Wang et al., 2022c). This degradation leads to the weakening of the fruit’s attachment, facilitating its drop.
Ethylene acts as a key hormonal signal that initiates and regulates the complex biochemical and physiological changes associated with pre-harvest drop. It activates the enzymatic breakdown mechanisms at the abscission zone (Brady and Speirs, 1991; Brown, 1997). Ethylene serves as a key signal molecule in initiating the transcription of several genes like PGs, cellulases, expansins (Li and Yuan, 2008; Eccher et al., 2015; Sriskantharajah et al., 2021). In apple, the expression of MdPG1, MdEG1 (Endo- β-1,4-Glucanase) and MdEXP3 increases in the fruit cortex and abscission zone during ripening, with a higher ERS/ETR ratio at transcript level indicating increased sensitivity to ethylene (Wakasa et al., 2003; Cin et al., 2005; Li and Yuan, 2008; Li et al., 2010). Ethylene signaling also interacts with other hormonal pathways (e.g. auxin, JA, and ABA), which can modulate the expression of abscission-related genes (Li and Yuan, 2008; Tadiello et al., 2016; Bai et al., 2021; Hu et al., 2022; Wang et al., 2022a). These findings suggest that ethylene directly or indirectly promotes ripening related pre-harvest fruit drop in climacteric fruits.
The application of ethylene inhibitors through pre-harvest sprays has been shown to effectively reduce fruit drop in climacteric fruits such as apples, thereby extending the harvest window (Bangerth, 1978; Greene and Schupp, 2004). These inhibitors increase fruit removal force, and inhibit ethylene biosynthesis, which are all critical factors responsible for reducing fruit drop (Bangerth, 1978). Pre-harvest application of AVG, 1-MCP, and Naphthaleneacetic acid (NAA), whether used in combination or alone, has proven to reduce premature fruit drop (Bangerth, 1978; Yuan and Carbaugh, 2007; Villalobos-Acuña et al., 2010; Yildiz et al., 2012). The effectiveness of AVG applications is particularly influenced by the timing and concentration used. Studies have shown that lower concentrations (150-300 mg/L) and application at 1 month before commercial harvest date are more effective than applying earlier or later in reducing fruit drop (Greene and Schupp, 2004; Schupp and Greene, 2004; Ozturk et al., 2015). Liu et al. (2022) found that a single application of AVG applied three weeks before the anticipated harvest (WBAH) is more effective than applications made at 1 WBAH. Moreover, the combination of AVG+NAA accompanied by 1-MCP has been found to more effectively delay pre-harvest fruit drop compared to the use of AVG or NAA alone (Yuan and Li, 2008). This synergistic effect suggests that a strategic approach, utilizing multiple ethylene inhibitors, can enhance the mitigation of fruit drop.
Predicting fruit drop in the climacteric fruit is a continuous challenge due to the intricate maturation processes that involve various physiological changes. One primary indicator of fruit drop is the change in fruit firmness and texture, which directly impacts the pre-harvest fruit drop and postharvest life of the product (Infante, 2012; Arseneault and Cline, 2016). Since direct measurement of flesh firmness before harvest is destructive and impractical for large-scale application, non-destructive technologies have been developed (Fathizadeh et al., 2021). Remote sensing technology like laser doppler vibrometer is a promising tool for determining fruit firmness and for evaluations of maturity (Muramatsu et al., 2000). In particular, optical properties near the chlorophyll absorbance range, have proven to be highly correlated with the flesh firmness (Infante, 2012). The Index of Absorbance Difference (IAD) has also shown strong correlations with chlorophyll degradation and flesh firmness that allows for continuous monitoring of on-tree fruit ripening, facilitating accurate determination of the optimal harvest time (Ziosi et al., 2008b). In addition to firmness and color, biochemical markers such as starch and sugar concentrations play a vital role in predicting fruit drop. In fruits like apples, a high starch concentration in the early stages indicates immaturity, while elevated levels of soluble sugars signal readiness for harvest maturity (Smith et al., 1979; Reid et al., 1982; Brookfield et al., 1997). Moreover, the loss of xylem functionality has been identified as an indicator of preharvest fruit drop potential. Due to its lignification, xylem is believed to be the last tissue to break down in the fruit pedicel, leading to preharvest fruit drop (Larson et al., 2023). There is a linear decrease in xylem functionality with rising internal ethylene content, correlating with increased preharvest fruit drop and higher expression levels of genes such as MdEG1 and MdPG2 in the fruit pedicel (Larson et al., 2023).
Commercial applications
Commercial applications of hormones or plant growth regulators (PGRs) are pivotal in optimizing the ripening of climacteric fruits in commercial settings. Strategic use of PGRs allows for precise control of the ripening process, thereby enhancing market quality, extending the harvest season and shelf life, and ensuring uniform ripening stages to effectively meet consumer demands.
Ethylene-based management of ripening
Commercial growers utilize ethylene as a key tool in managing fruit ripening to meet market demands for ripe and high-quality produce. Ethylene is widely used in commercial settings to stimulate and induce the ripening of various fruits such as bananas, avocados, tomatoes, and mangos (Zhang et al., 2016). While ethylene itself does not initiate ripening in immature fruits, exposure to ethylene accelerates the onset of maturity, leading to earlier ripening (Kevany et al., 2007). The application of ethylene-releasing agents like ethephon has been shown to effectively accelerate ripening, particular by increasing skin color and TSS (Table 1). This method allows growers to manage the ripening process efficiently, ensuring that fruits are ready for market at the desired stage of ripeness.
To delay ripening and mitigate preharvest fruit drop, growers frequently utilize ethylene inhibitors. These inhibitors function either by delaying maturity, as seen with AVG and 1-MCP, or by inhibiting the production of cell hydrolysis enzymes in the fruit pedicel, such as NAA (Larson et al., 2023). Specifically, AVG and 1-MCP are employed to reduce preharvest fruit drop and delay fruit softening and harvesting, particularly in apples (Table 1). The application of 1-MCP followed by low-temperature storage (8-10°C) effectively extends the shelf life of various fruits, including mangoes, tomatoes, and plums, while minimizing economic losses during storage (Taye et al., 2019; Xu et al., 2020; Fayek et al., 2022). A significant advantage of ethylene biosynthesis inhibitors is their reversibility through exogenous ethylene treatment. For example, AVG application maintains higher flesh firmness, greener peel color, and reduced ethylene production (Steffens et al., 2006; Kang et al., 2007). However, AVG can also impede red color development in apples (Steffens et al., 2006). To counteract this, combining AVG with ethephon enhances red color development without sacrificing the maturation-delaying benefits of AVG. This combined treatment improves color development while preserving superior fruit quality during storage compared to untreated apples (Wang and Dilley, 2001; Steffens et al., 2006; Kang et al., 2007).
Similarly, the combined application of 1-MCP and ethephon has shown promise in extending the shelf life of bananas while maintaining normal ripening. Studies have demonstrated that 1-MCP (400 nL/L) combined with ethephon (50 μL/L) prolongs green-life during cold storage and induces uniform ripening during shelf-life (Satekge and Magwaza, 2020). This treatment effectively delays ripening without the uneven ripening often associated with 1-MCP alone (Botondi et al., 2014). Fruit size also influences 1-MCP efficacy, with medium and large fruit responding better to the treatment than small fruit. However, the combined 1-MCP and ethephon treatment shows consistent results across all fruit sizes, suggesting its potential as a beneficial postharvest treatment for bananas (Satekge and Magwaza, 2020).
The benefits of using ethylene inhibitors come with some trade-offs. For instance, while AVG treatments have been effective in delaying preharvest fruit drop, they have consistently been associated with a reduction in red skin color in apples (Wang and Dilley, 2001; Greene, 2005; Steffens et al., 2006; Liu et al., 2022). This reduction in red coloration due to AVG treatments can compromise the visual appeal of fruits, potentially leading to a decrease in market value. Similarly, application of 1-MCP for longer time increases storage flesh disorders. For instance, while short-term 1-MCP treatment delays ripening in papaya, long-term treatment can lead to ripening disorders, including a “rubbery” texture (Zhu et al., 2019). This disorder is associated with accelerated lignin accumulation and delayed cellulose degradation during ripening (Zhu et al., 2019).
In addition to AVG and 1-MCP, hydrogen sulfide (H2S) has emerged as a novel gaseous signaling molecule with the capacity to delay fruit ripening. It plays a complex regulatory role, often acting as an antagonist to ethylene, thereby slowing or inhibiting the ripening process (Yao et al., 2020). For example, H2S has been shown to reduce the activity of ACC oxidase. Specifically, treatments with H2S have been reported to suppress the expression of SlACO1, SlACO3, and SlACO4 in tomatoes (Hu et al., 2019). Additionally, H2S may modulate ethylene receptors through post-translational modifications, such as S-sulfhydration, which reduces their sensitivity to ethylene, thereby weakening the signaling cascade that initiates ripening (Aroca et al., 2015; Ge et al., 2017; Jia et al., 2018). Another critical function of H2S in fruit ripening involves its capacity to scavenge reactive oxygen species (ROS), helping to maintain fruit quality post-harvest by preserving cellular integrity and delaying senescence. This has been observed in apples, tomatoes, and kiwifruits (Gao et al., 2013; Zheng et al., 2016; Yao et al., 2018; Ziogas et al., 2018).
Use of JA and ABA for enhancing desired traits
In addition to ethylene, other hormones such as JA and ABA have been identified as key regulators of fruit ripening related traits including fruit coloration and anthocyanin accumulation. For instance, the JA derivative PDJ was found to significantly enhances color development in apples and other fruits by promoting anthocyanin accumulation. Specifically, PDJ application fosters the formation of red color in apples, pears, and peaches (Atay, 2015; Wang et al., 2020a; Tang et al., 2022). In apples, PDJ treatment is most efficacious when administered approximately two weeks before harvest, although the optimal timing may vary slightly among different cultivars (Atay, 2015). Notably, endogenous JA concentrations in apple pulp are high during the early stages of growth, decrease as the fruit develops, and then rise again during maturation (Kondo et al., 2000). In peaches, treatment with MeJA or PDJ positively influences red color formation due to an approximate 120% increase in anthocyanin accumulation. This enhancement is attributed to increased enzyme activities and elevated transcript levels of genes involved in anthocyanin biosynthesis induced by MeJA or PDJ (Tang et al., 2022). Similarly, Preharvest PDJ treatments markedly improve color development in red pears without adversely affecting other quality parameters such as total soluble solids and fruit acidity. PDJ treatments, particularly at a concentration of 100 mg/L, enhance the levels of anthocyanins and flavonols in the peel, while the concentrations of hydroxycinnamates and flavanols decrease (Wang et al., 2020a).
The levels of cyanidin 3-O-glucoside and cyanidin 3-O-rutinoside, the primary anthocyanins in fig fruit, increase with ABA treatment, while the expression of anthocyanin biosynthesis genes is downregulated by the ABA inhibitors nordihydroguaiaretic acid (NDGA) and fluridone (Lama et al., 2020). In mangoes, ABA treatment results in a yellower flesh color and upregulation of the carotenoid biosynthesis gene MiPSY, whereas fluridone inhibits carotenoid accumulation (Wu et al., 2022). Similarly, exogenous ABA accelerates carotenoid accumulation and chlorophyll degradation in cherry tomatoes (Wu et al., 2018b). Additionally, ABA application induces color change and softening in bananas, enhancing their visual appeal (Jiang et al., 2000). When ABA-treated bananas (265 ppm) were exposed to ethylene (100 ppm) for 24 hours, there was a notable increase in ethylene production and respiration, which further enhanced skin color changes and fruit softening beyond the effects of ethylene alone (Jiang et al., 2000).
ABA treatment also significantly promotes starch degradation and increases levels of soluble sugars (glucose and sucrose) in apples, accompanied by the induction of amylase genes and genes responsible for glucose and sucrose transport (Ma et al., 2017). Moreover, ABA treatment elevates the TSS content in mangoes, an effect inhibited by fluridone (Wu et al., 2022). In peaches, ABA maintains soluble sugar content during cold storage by enhancing the activities of sucrose phosphate synthase and sucrose synthase (Zhao et al., 2022).
Auxin applications in ripening control
Auxin and its inhibitors hold significant practical implications for growers and the commercial sector in modifying fruit ripening traits. Understanding the interplay between auxin and ethylene allows growers to manipulate the ripening process, thereby extending the harvest season and enhancing both fruit quality and shelf life. The application of synthetic auxin or auxin-like compounds such as NAA has proven effective in delaying ripening in climacteric fruits, underscoring the utility of auxin manipulation. Research shows that NAA applications can significantly reduce preharvest fruit drop in apples, extending fruit retention on trees by approximately 14 days (Rehman et al., 2017). However, alternative treatments like AVG could be more effective than NAA in preventing fruit drop and delaying maturity (Fallahi, 2007). The effectiveness of reducing fruit drop by applying NAA could also be heightened when used in combination with AVG, particularly when applied 2-3 weeks before harvest (Yuan and Carbaugh, 2007; Arseneault and Cline, 2018).
NAA treatments have also been found to maintain Apple fruit firmness at harvest compared to untreated fruits (Ozturk et al., 2019). However, during extended cold storage, NAA-treated fruits may exhibit reduced flesh firmness (Ozturk et al., 2019). Additionally, NAA applications can enhance fruit color development, as indicated by decreased L* and hue angle values during storage (Ozturk et al., 2019). While NAA treatments may slightly increase fruit weight in later harvests compared to other treatments (Fallahi, 2007), they generally result in lower soluble solids content (SSC) (Ozturk et al., 2019). Furthermore, NAA-treated fruits tend to experience faster starch degradation (Ozturk et al., 2019).
Future prospects
The future of fruit ripening management promises transformative advancements in our comprehension and control of this complex phenomenon. While the pivotal role of ethylene in climacteric fruit color change is well-documented, emerging research must probe the nuanced interactions between ethylene and other hormones and signaling molecules. For instance, investigating how JA, ABA, and auxin collaborate with ethylene to regulate ripening traits could unveil new dimensions in fruit development. Additionally, understanding these hormones’ multifaceted interactions with ethylene encompassing plant-microbe interactions, abiotic stress responses, and developmental cues may facilitate more optimized conditions that reconcile fruit ripening with other vital pathways. For example, auxin’s influence on xylem differentiation and tissue flexibility could be leveraged to mitigate physiological disorders, such as bitter pit in apples, that are manifested during ripening and post-storage (Griffith and Einhorn, 2023). However, the use of synthetic auxins like NAA might inadvertently affect ripening speed and fruit drop. Similarly, investigating the synergistic relationship between ethylene and JA and the antagonistic relationship between JA and SA in plant defense mechanisms against necrotrophic and biotrophic pathogens, respectively, (Phuong et al., 2020) alongside the above-mentioned roles of these hormones and their synthetic analogs in fruit ripening, can pave the way for informed spray applications and pest management strategies that account for these intricate hormonal interactions. By understanding these complex cross-talks, we can develop targeted approaches to enhance plant resilience and optimize fruit quality.
A promising avenue for future research involves examining ACC beyond its ethylene-related functions. Unraveling how ACC operates as an independent signaling molecule may reveal novel pathways and mechanisms influencing ripening and other physiological processes, potentially leading to precise ripening control. Preliminary findings from our group (unpublished) suggest that combining ACC with AVG may more effectively balance pre-harvest drop control and color development in apples compared to using these PGRs individually. Nonetheless, AVG’s role as an ACS enzyme inhibitor necessitates further elucidation of the molecular and biochemical mechanisms that could explain the observed effects.
Examining environmental conditions, genetic determinants, and crop load is crucial for elucidating their effects on ethylene production and sensitivity. Such analysis could provide valuable insights into pre-harvest fruit abscission and overall yield. Research should focus on how varying crop loads influence ethylene dynamics and fruit retention mechanisms, potentially uncovering strategies to enhance yield. Although there is a well-established correlation between fruit ripening and pre-harvest fruit drop, the precise nature of this relationship especially concerning skin color development and starch conversion as predictive indicators of pre-harvest drop remains ambiguous. Moreover, despite extensive investigations into ethylene mutants regarding the uniform segregation of ethylene-dependent ripening traits, the interplay between ripening characteristics and fruit abscission represents a compelling research frontier with potentially significant practical implications.
Addressing these research areas could lead to the development of more effective and sustainable fruit ripening management strategies. Such advancements will enhance fruit quality, reduce post-harvest losses, and improve the efficiency of fruit production and distribution, ultimately fulfilling consumer demands and fortifying the resilience of the agricultural industry.
Author contributions
MT: Writing – review & editing, Writing – original draft. SS: Writing – review & editing, Writing – original draft.
Funding
The author(s) declare that financial support was received for the research, authorship, and/or publication of this article. This project was partially funded by Virginia Department of Agriculture and Consumer Services (420698) and the Virginia’s Apple Research Program (469021).
Conflict of interest
The authors declare that the research was conducted in the absence of any commercial or financial relationships that could be construed as a potential conflict of interest.
The author(s) declared that they were an editorial board member of Frontiers, at the time of submission. This had no impact on the peer review process and the final decision.
Publisher’s note
All claims expressed in this article are solely those of the authors and do not necessarily represent those of their affiliated organizations, or those of the publisher, the editors and the reviewers. Any product that may be evaluated in this article, or claim that may be made by its manufacturer, is not guaranteed or endorsed by the publisher.
References
Abbas, M. F., Fandi, B. S. (2002). Respiration rate, ethylene production and biochemical changes during fruit development and maturation of jujube (Ziziphus mauritiana Lamk). J. Sci. Food Agric. 82, 1472–1476. doi: 10.1002/jsfa.v82:13
Abdi, N., McGlasson, W. B., Holford, P., Williams, M., Mizrahi, Y. (1998). Responses of climacteric and suppressed-climacteric plums to treatment with propylene and 1-methylcyclopropene. Postharvest Biol. Technol. 14, 29–39. doi: 10.1016/S0925-5214(98)00031-3
Abeles, F. B. (1969). Abscission: role of cellulase. Plant Physiol. 44, 447–452. doi: 10.1104/pp.44.3.447
Abeles, F. B., Morgan, P. W., Saltveit, M. E., Jr. (2012). Ethylene in Plant Biology (San diego, California, USA: Academic Press).
Abu-Goukh, A.-B. A., Bashir, H. A. (2003). Changes in pectic enzymes and cellulase activity during guava fruit ripening. Food Chem. 83, 213–218. doi: 10.1016/S0308-8146(03)00067-0
Adams, D. O., Yang, S. F. (1979). Ethylene biosynthesis: Identification of 1-aminocyclopropane-1-carboxylic acid as an intermediate in the conversion of methionine to ethylene. Proc. Natl. Acad. Sci. 76, 170–174. doi: 10.1073/pnas.76.1.170
Aghdam, M. S., Motallebiazar, A., Mostofi, Y., Moghaddam, J. F., Ghasemnezhad, M. (2010). Effects of mesa vapor treatment on the postharvest quality of ‘hayward’ kiwifruit. Acta Hortic. 877, 743–748. doi: 10.17660/ActaHortic.2010.877.98
Alonso, J. M., Stepanova, A. N., Solano, R., Wisman, E., Ferrari, S., Ausubel, F. M., et al. (2003). Five components of the ethylene-response pathway identified in a screen for weak ethylene-insensitive mutants in Arabidopsis. Proc. Natl. Acad. Sci. 100, 2992–2997. doi: 10.1073/pnas.0438070100
Althiab-Almasaud, R., Sallanon, H., Chang, C., Chervin, C. (2021). 1-Aminocyclopropane-1-carboxylic acid stimulates tomato pollen tube growth independently of ethylene receptors. Physiologia Plantarum 173, 2291–2297. doi: 10.1111/ppl.13579
Amrhein, N., Wenker, D. (1979). Novel inhibitors of ethylene production in higher plants. Plant Cell Physiol. 20, 1635–1642. doi: 10.1093/oxfordjournals.pcp.a075966
An, J.-P., Wang, X.-F., Li, Y.-Y., Song, L.-Q., Zhao, L.-L., You, C.-X., et al. (2018). EIN3-LIKE1, MYB1, and ETHYLENE RESPONSE FACTOR3 act in a regulatory loop that synergistically modulates ethylene biosynthesis and anthocyanin accumulation. Plant Physiol. 178, 808–823. doi: 10.1104/pp.18.00068
Argenta, L. C., Fan, X., Mattheis, J. P. (2003). Influence of 1-methylcyclopropene on Ripening, Storage Life, and Volatile Production by d’Anjou cv. Pear Fruit. J. Agric. Food Chem. 51, 3858–3864. doi: 10.1021/jf034028g
Aroca, Á., Serna, A., Gotor, C., Romero, L. C. (2015). S-sulfhydration: A cysteine posttranslational modification in plant systems. Plant Physiol. 168, 334–342. doi: 10.1104/pp.15.00009
Arseneault, M. H., Cline, J. A. (2016). A review of apple preharvest fruit drop and practices for horticultural management. Scientia Hortic. 211, 40–52. doi: 10.1016/j.scienta.2016.08.002
Arseneault, M. H., Cline, J. A. (2018). AVG, NAA, boron, and magnesium influence preharvest fruit drop and fruit quality of ‘Honeycrisp’ apples. Can. J. Plant Sci. 98, 741–752. doi: 10.1139/cjps-2017-0170
Atay, E. (2015). Effect of application time of prohydrojasmon on fruit coloration of gala and braeburn appleS. Bulgarian J. Agric. Sci. 21, 1242–1247.
Atkinson, R. G., Gunaseelan, K., Wang, M. Y., Luo, L., Wang, T., Norling, C. L., et al. (2011). Dissecting the role of climacteric ethylene in kiwifruit (Actinidia chinensis) ripening using a 1-aminocyclopropane-1-carboxylic acid oxidase knockdown line. J. Exp. Bot. 62, 3821–3835. doi: 10.1093/jxb/err063
Bai, Q., Huang, Y., Shen, Y. (2021). The physiological and molecular mechanism of abscisic acid in regulation of fleshy fruit ripening. Front. Plant Sci. 11. doi: 10.3389/fpls.2020.619953
Bangerth, F. (1978). The effect of a substituted amino acid on ethylene biosynthesis, respiration, ripening and preharvest drop of apple fruits. J. Am. Soc. Hortic. Sci. 103, 401–404. doi: 10.21273/JASHS.103.3.401
Barry, C. S., Giovannoni, J. J. (2007). Ethylene and fruit ripening. J. Plant Growth Regul. 26, 143–159. doi: 10.1007/s00344-007-9002-y
Bauchot, A. D., Mottram, D. S., Dodson, A. T., John, P. (1998). Effect of aminocyclopropane-1-carboxylic acid oxidase antisense gene on the formation of volatile esters in cantaloupe charentais melon (Cv. Védrandais). J. Agric. Food Chem. 46, 4787–4792. doi: 10.1021/jf980692z
Bauchot, A. D., Mottram, D. S., John, P. (2002). “Aroma Volatiles in Fruits in Which Ethylene Production Is Depressed by Antisense Technology,” in Analysis of Taste and Aroma. Eds. Jackson, J. F., Linskens, H. F. (Springer, Berlin, Heidelberg), 159–172.
Belding, R. D., Lokaj, G. R. W. (2002). Aminoethoxyvinylglycine treatment of peach fruit reduces ethylene production and softening. HortScience 37, 1065–1068. doi: 10.21273/HORTSCI.37.7.1065
Ben-Arie, R., Saks, Y., Sonego, L., Frank, A. (1996). Cell wall metabolism in gibberellin-treated persimmon fruits. Plant Growth Regul. 19, 25–33. doi: 10.1007/BF00024399
Binder, B. M., Mortimore, L. A., Stepanova, A. N., Ecker, J. R., Bleecker, A. B. (2004). Short-term growth responses to ethylene in arabidopsis seedlings are EIN3/EIL1 independent. Plant Physiol. 136, 2921–2927. doi: 10.1104/pp.104.050393
Blankenship, S. M., Dole, J. M. (2003). 1-methylcyclopropene: a review. Postharvest Biol. Technol. 28, 1–25. doi: 10.1016/S0925-5214(02)00246-6
Boller, T., Herner, R. C., Kende, H. (1979). Assay for and enzymatic formation of an ethylene precursor, 1-aminocyclopropane-1-carboxylic acid. Planta 145, 293–303. doi: 10.1007/BF00454455
Bonghi, C., Rascio, N., Ramina, A., Casadoro, G. (1992). Cellulase and polygalacturonase involvement in the abscission of leaf and fruit explants of peach. Plant Mol. Biol. 20, 839–848. doi: 10.1007/BF00027155
Bonnin, E., Pelloux, J. (2020). “Pectin Degrading Enzymes,” in Pectin: Technological and Physiological Properties. Ed. Kontogiorgos, V. (Springer International Publishing, Cham), 37–60.
Botondi, R., De Sanctis, F., Bartoloni, S., Mencarelli, F. (2014). Simultaneous application of ethylene and 1-MCP affects banana ripening features during storage. J. Sci. Food Agric. 94, 2170–2178. doi: 10.1002/jsfa.2014.94.issue-11
Bower, J., Holford, P., Latché, A., Pech, J.-C. (2002). Culture conditions and detachment of the fruit influence the effect of ethylene on the climacteric respiration of melon. Postharvest Biol. Technol. 26, 135–146. doi: 10.1016/S0925-5214(02)00007-8
Brackmann, A., Thewes, F. R., Anese R de, O., Both, V., Linke Junior, W., Schultz, E. E. (2015). Aminoethoxyvinylglycine: isolated and combined with other growth regulators on quality of ‘Brookfield’ apples after storage. Scientia Agricola 72, 221–228. doi: 10.1590/0103-9016-2014-0099
Brady, C. J., Speirs, J. (1991). “Ethylene in Fruit Ontogeny and Abscission,” in The Plant Hormone Ethylene (Boca Raton, Florida, USA: CRC Press).
Brookfield, P., Murphy, P., Harker, R., MacRae, E. (1997). Starch degradation and starch pattern indices; interpretation and relationship to maturity. Postharvest Biol. Technol. 11, 23–30. doi: 10.1016/S0925-5214(97)01416-6
Brown, K. M. (1997). Ethylene and abscission. Physiologia Plantarum 100, 567–576. doi: 10.1111/j.1399-3054.1997.tb03062.x
Burroughs, L. F. (1957). I-aminocyclopropane-I-carboxylic acid: A new amino-acid in perry pears and cider apples. Nature 179, 360–361. doi: 10.1038/179360a0
Çetinbaş, M., Butar, S., Onursal, C. E., Koyuncu, M. A. (2012). The effects of pre-harvest ReTain [aminoethoxyvinylglycine (AVG)] application on quality change of ‘Monroe’ peach during normal and controlled atmosphere storage. Scientia Hortic. 147, 1–7. doi: 10.1016/j.scienta.2012.08.025
Chen, M., Gu, H., Wang, L., Shao, Y., Li, R., Li, W. (2022). Exogenous ethylene promotes peel color transformation by regulating the degradation of chlorophyll and synthesis of anthocyanin in postharvest mango fruit. Front. Nutr. 9. doi: 10.3389/fnut.2022.911542
Chen, P. M., Spotts, R. A., Mellenthin, W. M. (1981). Stem-end decay and quality of low oxygen stored ‘d’Anjou’ Pears. Journal of the American Society for Horticultural Science 106, 695–698. doi: 10.21273/JASHS.106.6.695
Chen, S., Wang, X.-J., Tan, G.-F., Zhou, W.-Q., Wang, G.-L. (2020). Gibberellin and the plant growth retardant Paclobutrazol altered fruit shape and ripening in tomato. Protoplasma 257, 853–861. doi: 10.1007/s00709-019-01471-2
Cheng, Y., Dong, Y., Yan, H., Ge, W., Shen, C., Guan, J., et al. (2012). Effects of 1-MCP on chlorophyll degradation pathway-associated genes expression and chloroplast ultrastructure during the peel yellowing of Chinese pear fruits in storage. Food Chem. 135, 415–422. doi: 10.1016/j.foodchem.2012.05.017
Cin, V. D., Danesin, M., Boschetti, A., Dorigoni, A., Ramina, A. (2005). Ethylene biosynthesis and perception in apple fruitlet abscission (Malus domestica L. Borck). J. Exp. Bot. 56, 2995–3005. doi: 10.1093/jxb/eri296
Cirilli, M., Giovannini, D., Ciacciulli, A., Chiozzotto, R., Gattolin, S., Rossini, L., et al. (2018). Integrative genomics approaches validate PpYUC11-like as candidate gene for the stony hard trait in peach (P. persica L. Batsch). BMC Plant Biol. 18, 88. doi: 10.1186/s12870-018-1293-6
Clayton, M., Biasi, W. V., Southwick, S. M., Mitcham, E. J. (2000). ReTain™ Affects maturity and ripening of `Bartlett’ Pear. HortScience 35, 1294–1299. doi: 10.21273/HORTSCI.35.7.1294
Cordenunsi-Lysenko, B. R., Nascimento, J. R. O., Castro-Alves, V. C., Purgatto, E., Fabi, J. P., Peroni-Okyta, F. H. G. (2019). The starch is (Not) just another brick in the wall: the primary metabolism of sugars during banana ripening. Front. Plant Sci. 10. doi: 10.3389/fpls.2019.00391
Cosgrove, D. J. (2000). Loosening of plant cell walls by expansins. Nature 407, 321–326. doi: 10.1038/35030000
Cox, K. A., McGhie, T. K., White, A., Woolf, A. B. (2004). Skin colour and pigment changes during ripening of ‘Hass’ avocado fruit. Postharvest Biol. Technol. 31, 287–294. doi: 10.1016/j.postharvbio.2003.09.008
Dal Cin, V., Danesin, M., Botton, A., Boschetti, A., Dorigoni, A., Ramina, A. (2008). Ethylene and preharvest drop: the effect of AVG and NAA on fruit abscission in apple (Malus domestica L. Borkh). Plant Growth Regul. 56, 317–325. doi: 10.1007/s10725-008-9312-5
da Mota, R. V., Cordenunsi, B. R., do Nascimento, J. R., Purgatto, E., Rosseto, M. R., Lajolo, F. M. (2002). Activity and expression of banana starch phosphorylases during fruit development and ripening. Planta 216, 325–333. doi: 10.1007/s00425-002-0858-6
Dandekar, A. M., Teo, G., Defilippi, B. G., Uratsu, S. L., Passey, A. J., Kader, A. A., et al. (2004). Effect of down-regulation of ethylene biosynthesis on fruit flavor complex in apple fruit. Transgenic Res. 13, 373–384. doi: 10.1023/B:TRAG.0000040037.90435.45
Defilippi, B. G., Dandekar, A. M., Kader, A. A. (2005). Relationship of ethylene biosynthesis to volatile production, related enzymes, and precursor availability in apple peel and flesh tissues. J. Agric. Food Chem. 53, 3133–3141. doi: 10.1021/jf047892x
Ding, C.-K., Wang, C. Y. (2003). The dual effects of methyl salicylate on ripening and expression of ethylene biosynthetic genes in tomato fruit. Plant Sci. 164, 589–596. doi: 10.1016/S0168-9452(03)00010-4
Ding, X., Wang, B., Gong, Y., Yan, X., Chen, X., Zhong, Y., et al. (2024). Exogenous methyl jasmonate (MeJA) improves ‘Ruixue’ Apple fruit quality by regulating cell wall metabolism. Foods 13, 1594. doi: 10.3390/foods13111594
do Nascimento, J. R. O., Júnior, A. V., Bassinello, P. Z., Cordenunsi, B. R., Mainardi, J. A., Purgatto, E., et al. (2006). Beta-amylase expression and starch degradation during banana ripening. Postharvest Biol. Technol. 40, 41–47. doi: 10.1016/j.postharvbio.2005.11.008
Dong, Y., Mitra, D., Kootstra, A., Lister, C., Lancaster, J. (1995). Postharvest stimulation of skin color in royal gala apple. J. Am. Soc. Hortic. Sci. 120, 95–100. doi: 10.21273/JASHS.120.1.95
Eccher, G., Begheldo, M., Boschetti, A., Ruperti, B., Botton, A. (2015). Roles of ethylene production and ethylene receptor expression in regulating apple fruitlet abscission. Plant Physiol. 169, 125–137. doi: 10.1104/pp.15.00358
Elbar, S., Maytal, Y., David, I., Carmeli-Weissberg, M., Shaya, F., Barnea-Danino, Y., et al. (2023). Abscisic acid plays a key role in the regulation of date palm fruit ripening. Front. Plant Sci. 13. doi: 10.3389/fpls.2022.1066142
El Hadi, M. A. M., Zhang, F.-J., Wu, F.-F., Zhou, C.-H., Tao, J. (2013). Advances in fruit aroma volatile research. Molecules 18, 8200–8229. doi: 10.3390/molecules18078200
El-Sharkawy, I., Sherif, S., Qubbaj, T., Sullivan, A. J., Jayasankar, S. (2016). Stimulated auxin levels enhance plum fruit ripening, but limit shelf-life characteristics. Postharvest Biol. Technol. 112, 215–223. doi: 10.1016/j.postharvbio.2015.09.012
Fallahi, E. (2007). Influence of 1-aminoethoxyvinylglycine hydrochloride and α-naphthalene acetic acid on fruit retention, quality, evolved ethylene, and respiration in apples. Int. J. Plant Production 1, 53–61. doi: 10.22069/ijpp.2012.525
Fan, X., Mattheis, J. P. (2001). 1-Methylcyclopropene and storage temperature influence responses of ‘Gala’ apple fruit to gamma irradiation. Postharvest Biol. Technol. 23, 143–151. doi: 10.1016/S0925-5214(01)00119-3
Fan, X., Mattheis, J. P., Fellman, J. K. (1998). A role for jasmonates in climacteric fruit ripening. Planta 204, 444–449. doi: 10.1007/s004250050278
Farcuh, M., Rivero, R. M., Sadka, A., Blumwald, E. (2018). Ethylene regulation of sugar metabolism in climacteric and non-climacteric plums. Postharvest Biol. Technol. 139, 20–30. doi: 10.1016/j.postharvbio.2018.01.012
Fathizadeh, Z., Aboonajmi, M., Hassan-Beygi, S. R. (2021). Nondestructive methods for determining the firmness of apple fruit flesh. Inf. Process. Agric. 8, 515–527. doi: 10.1016/j.inpa.2020.12.002
Fayek, M. A., Ahmed, D. M., El-Shenawy, G. I., Khoudair, A. A. (2022). Pre storage application of 1- methylcyclopropane (1-MCP) on quality and extending storability of tommy atkins mango fruits. Egyptian J. Chem. 65, 111–118. doi: 10.21608/ejchem.2021.96899.4535
Feng, X., Apelbaum, A., Sisler, E. C., Goren, R. (2000). Control of ethylene responses in avocado fruit with 1-methylcyclopropene. Postharvest Biol. Technol. 20, 143–150. doi: 10.1016/S0925-5214(00)00126-5
Feng, S., Sun, J., Sun, S., Wang, Y., Tian, C., Sun, Q., et al. (2017). Transcriptional profiles underlying the effects of methyl jasmonate on apple ripening. J. Plant Growth Regul. 36, 271–280. doi: 10.1007/s00344-016-9636-8
Flores, N. R., Veloz, C. S., Reyes, D. S., Jiménez, A. L., Guadarrama, S. V., Guzmán, M. E. R., et al. (2021). Postharvest ripening of Hass and Méndez avocado fruit cultivars treated with ethephon. Trends Horticulture 4, 103–111. doi: 10.24294/th.v4i1.1805
Frenkel, C., Dyck, R. (1973). Auxin inhibition of ripening in bartlett pears. Plant Physiol. 51, 6–9. doi: 10.1104/pp.51.1.6
Gambhir, P., Singh, V., Parida, A., Raghuvanshi, U., Kumar, R., Sharma, A. K. (2022). Ethylene response factor ERF.D7 activates auxin response factor 2 paralogs to regulate tomato fruit ripening. Plant Physiol. 190, 2775–2796. doi: 10.1093/plphys/kiac441
Gao, S.-P., Hu, K.-D., Hu, L.-Y., Li, Y.-H., Han, Y., Wang, H.-L., et al. (2013). Hydrogen sulfide delays postharvest senescence and plays an antioxidative role in fresh-cut kiwifruit. HortScience 48, 1385–1392. doi: 10.21273/HORTSCI.48.11.1385
Ge, Y., Hu, K.-D., Wang, S.-S., Hu, L.-Y., Chen, X.-Y., Li, Y.-H., et al. (2017). Hydrogen sulfide alleviates postharvest ripening and senescence of banana by antagonizing the effect of ethylene. PloS One 12, e0180113. doi: 10.1371/journal.pone.0180113
Golding, J. B., Shearer, D., Wyllie, S. G., McGlasson, W. B. (1998). Application of 1-MCP and propylene to identify ethylene-dependent ripening processes in mature banana fruit. Postharvest Biol. Technol. 14, 87–98. doi: 10.1016/S0925-5214(98)00032-5
Gomes, G. L. B., Scortecci, K. C. (2021). Auxin and its role in plant development: structure, signalling, regulation and response mechanisms. Plant Biol. 23, 894–904. doi: 10.1111/plb.13303
Greene, D. W. (2005). Time of aminoethoxyvinylglycine application influences preharvest drop and fruit quality of ‘McIntosh’ Apples. HortScience 40, 2056–2060. doi: 10.21273/HORTSCI.40.7.2056
Greene, D. W., Schupp, J. R. (2004). Effect of aminoethoxyvinylglycine (AVG) on preharvest drop, fruit quality, and maturation of `McIntosh’ Apples. II. Effect of timing and concentration relationships and spray volume. HortScience 39, 1036–1041. doi: 10.21273/HORTSCI.39.5.1036
Griffith, C., Einhorn, T. C. (2023). The effect of plant growth regulators on xylem differentiation, water and nutrient transport, and bitter pit susceptibility of apple. Scientia Hortic. 310, 111709. doi: 10.1016/j.scienta.2022.111709
Guo, Q., Lv, X., Xu, F., Zhang, Y., Wang, J., Lin, H., et al. (2013). Chlorine dioxide treatment decreases respiration and ethylene synthesis in fresh-cut ‘Hami’ melon fruit. Int. J. Food Sci. Technol. 48, 1775–1782. doi: 10.1111/ijfs.2013.48.issue-9
Hadfield, K. A., Dang, T., Guis, M., Pech, J.-C., Bouzayen, M., Bennett, A. B. (2000). Characterization of ripening-regulated cDNAs and their expression in ethylene-suppressed charentais melon fruit. Plant Physiol. 122, 977–984. doi: 10.1104/pp.122.3.977
Hadfield, K. A., Rose, J. K. C., Yaver, D. S., Berka, R. M., Bennett, A. B. (1998). Polygalacturonase gene expression in ripe melon fruit supports a role for polygalacturonase in ripening-associated pectin disassembly. Plant Physiol. 117, 363–373. doi: 10.1104/pp.117.2.363
Han, G.-Z. (2017). Evolution of jasmonate biosynthesis and signaling mechanisms. J. Exp. Bot. 68, 1323–1331. doi: 10.1093/jxb/erw470
Han, X., Wang, X., Shen, C., Mo, Y., Tian, R., Mao, L., et al. (2022). Exogenous ABA promotes aroma biosynthesis of postharvest kiwifruit after low-temperature storage. Planta 255, 82. doi: 10.1007/s00425-022-03855-w
Hao, Y. (2014). Auxin-mediated fruit development and ripening : new insight on the role of ARFs and their action mechanism in tomato (S. lycopersicum). Toulouse, France: Institut National Polytechnique de Toulouse - INPT.
Hao, Y., Hu, G., Breitel, D., Liu, M., Mila, I., Frasse, P., et al. (2015). Auxin response factor slARF2 is an essential component of the regulatory mechanism controlling fruit ripening in tomato. PloS Genet. 11, e1005649. doi: 10.1371/journal.pgen.1005649
Hauser, F., Waadt, R., Schroeder, J. I. (2011). Evolution of abscisic acid synthesis and signaling mechanisms. Curr. Biol. 21, R346–R355. doi: 10.1016/j.cub.2011.03.015
Hayama, H., Tatsuki, M., Ito, A., Kashimura, Y. (2006). Ethylene and fruit softening in the stony hard mutation in peach. Postharvest Biol. Technol. 41, 16–21. doi: 10.1016/j.postharvbio.2006.03.006
Hayama, H., Tatsuki, M., Nakamura, Y. (2008). Combined treatment of aminoethoxyvinylglycine (AVG) and 1-methylcyclopropene (1-MCP) reduces melting-flesh peach fruit softening. Postharvest Biol. Technol. 50, 228–230. doi: 10.1016/j.postharvbio.2008.05.003
He, J., Feng, Y., Cheng, Y., Guan, J. (2023). Effects of preharvest aminoethoxyvinylglycine (AVG) treatment on fruit ripening, core browning and related gene expression in ‘Huangguan’ Pear (Pyrus bretschneideri rehd.). Horticulturae 9, 179. doi: 10.3390/horticulturae9020179
He, Y., Li, J., Ban, Q., Han, S., Rao, J. (2018). Role of brassinosteroids in persimmon (Diospyros kaki L.) fruit ripening. J. Agric. Food Chem. 66, 2637–2644. doi: 10.1021/acs.jafc.7b06117
Hedden, P., Thomas, S. G. (2012). Gibberellin biosynthesis and its regulation. Biochem. J. 444, 11–25. doi: 10.1042/BJ20120245
Herner, R. C., Sink, K. C., Jr. (1973). Ethylene production and respiratory behavior of the rin tomato mutant. Plant Physiol. 52, 38–42. doi: 10.1104/pp.52.1.38
Hewitt, S., Dhingra, A. (2020). Beyond ethylene: new insights regarding the role of alternative oxidase in the respiratory climacteric. Front. Plant Sci. 11. doi: 10.3389/fpls.2020.543958
Hu, S., Liu, L., Li, S., Shao, Z., Meng, F., Liu, H., et al. (2020). Regulation of fruit ripening by the brassinosteroid biosynthetic gene SlCYP90B3 via an ethylene-dependent pathway in tomato. Horticulture Res. 7, 163. doi: 10.1038/s41438-020-00383-0
Hu, Y., Sun, H., Han, Z., et al. (2022). ERF4 affects fruit ripening by acting as a JAZ interactor between ethylene and jasmonic acid hormone signaling pathways. Hortic. Plant J. 8, 689–699. doi: 10.1016/j.hpj.2022.01.002
Hu, K.-D., Zhang, X.-Y., Wang, S.-S., Tang, J., Yang, F., Huang, Z-Q., et al. (2019). Hydrogen sulfide inhibits fruit softening by regulating ethylene synthesis and signaling pathway in tomato (Solanum lycopersicum). HortScience 54, 1824–1830. doi: 10.21273/HORTSCI14283-19
Huang, W., Hu, N., Xiao, Z., Qiu, Y., Yang, Y., Yang, J., et al. (2022). A molecular framework of ethylene-mediated fruit growth and ripening processes in tomato. Plant Cell 34, 3280–3300. doi: 10.1093/plcell/koac146
Infante, R. (2012). Harvest maturity indicators in the stone fruit industry. Stewart Postharvest Rev. 8, 1–6. doi: 10.2212/spr.2012.1.3
Ireland, H. S., Gunaseelan, K., Muddumage, R., Tacken, E. J., Putterill, J., Johnston, J. W., et al. (2014). Ethylene regulates apple (Malus × domestica) fruit softening through a dose × Time-dependent mechanism and through differential sensitivities and dependencies of cell wall-modifying genes. Plant Cell Physiol. 55, 1005–1016. doi: 10.1093/pcp/pcu034
Jeffery, D., Smith, C., Goodenough, P., Prosser, I., Grierson, D. (1984). Ethylene-independent and ethylene-dependent biochemical changes in ripening tomatoes. Plant Physiol. 74, 32–38. doi: 10.1104/pp.74.1.32
Ji, X.-H., Wang, Y.-T., Zhang, R., Wu, S.-J., An, M.-M., Li, M., et al. (2015). Effect of auxin, cytokinin and nitrogen on anthocyanin biosynthesis in callus cultures of red-fleshed apple (Malus sieversii f.niedzwetzkyana). Plant Cell Tissue Organ Culture (PCTOC) 120, 325–337. doi: 10.1007/s11240-014-0609-y
Jia, H., Chen, S., Liu, D., Liesche, J., Shi, C., Wang, J., et al. (2018). Ethylene-induced hydrogen sulfide negatively regulates ethylene biosynthesis by persulfidation of ACO in tomato under osmotic stress. Front. Plant Sci. 9. doi: 10.3389/fpls.2018.01517
Jiang, Y., Joyce, D. C., Macnish, A. J. (2000). Effect of abscisic acid on banana fruit ripening in relation to the role of ethylene. J. Plant Growth Regul. 19, 106–111. doi: 10.1007/s003440000011
Johnston, J. W., Gunaseelan, K., Pidakala, P., Wang, M., Schaffer, R. J. (2009). Co-ordination of early and late ripening events in apples is regulated through differential sensitivities to ethylene. J. Exp. Bot. 60, 2689–2699. doi: 10.1093/jxb/erp122
Jones, B., Frasse, P., Olmos, E., Zegzouti, H., Li, Z. G., Latché, A., et al. (2002). Down-regulation of DR12, an auxin-response-factor homolog, in the tomato results in a pleiotropic phenotype including dark green and blotchy ripening fruit. Plant J. 32, 603–613. doi: 10.1046/j.1365-313X.2002.01450.x
Ju, C., Chang, C. (2015). Mechanistic insights in ethylene perception and signal transduction. Plant Physiol. 169, 85–95. doi: 10.1104/pp.15.00845
Kang, I.-K., Byun, J.-K., Kweon, H.-J., Kim, M.-J., Kwon, S.-I., Park, M.-Y., et al. (2007). Effects of aminoethoxyvinylglycine on preharvest drop, fruit color, and quality of ‘Tsugaru’ Apples. HORTICULTURE Environ. Biotechnol. 48, 159–164.
Kevany, B. M., Tieman, D. M., Taylor, M. G., Cin, V. D., Klee, H. J. (2007). Ethylene receptor degradation controls the timing of ripening in tomato fruit. Plant J. 51, 458–467. doi: 10.1111/j.1365-313X.2007.03170.x
Khader, S. E. S. A. (1991). Effect of preharvest application of GA3 on postharvest behaviour of mango fruits. Scientia Hortic. 47, 317–321. doi: 10.1016/0304-4238(91)90014-P
Khan, A. S., Singh, Z. (2007). Methyl jasmonate promotes fruit ripening and improves fruit quality in Japanese plum. J. Hortic. Sci. Biotechnol. 82, 695–706. doi: 10.1080/14620316.2007.11512293
Klee, H. J., Giovannoni, J. J. (2011). Genetics and control of tomato fruit ripening and quality attributes. Annu. Rev. Genet. 45, 41–59. doi: 10.1146/annurev-genet-110410-132507
Kon, T. M., Clavet, C. D., Clarke, G. G. (2023). Organic aminoethoxyvinylglycine is an effective alternative for reducing apple preharvest drop. HortScience 58, 733–738. doi: 10.21273/HORTSCI17115-23
Kondo, S. (2010). Roles of jasmonates in fruit ripening and environmental stress. Acta Hortic. 884, 711–716. doi: 10.17660/ActaHortic.2010.884.96
Kondo, S., Takahashi, Y. (1989). Relation between early drop of apple fruit and ethylene evolution under high night-temperature conditions. J. Japanese Soc. Hortic. Sci. 58, 1–8. doi: 10.2503/jjshs.58.1
Kondo, S., Tomiyama, A., Seto, H. (2000). Changes of endogenous jasmonic acid and methyl jasmonate in apples and sweet cherries during fruit development. J. American Soc. Hortic. Sci. 125, 282–287. doi: 10.21273/JASHS.125.3.282
Kondo, S., Yamada, H., Setha, S. (2007). Effect of jasmonates differed at fruit ripening stages on 1-aminocyclopropane-1-carboxylate (ACC) synthase and ACC oxidase gene expression in pears. J. Am. Soc. Hortic. Sci. 132, 120–125. doi: 10.21273/JASHS.132.1.120
Kucuker, E., Ozturk, B., Celik, S. M., Aksit, H. (2014). Pre-harvest spray application of methyl jasmonate plays an important role in fruit ripening, fruit quality and bioactive compounds of Japanese plums. Scientia Hortic. 176, 162–169. doi: 10.1016/j.scienta.2014.07.007
Lacap, A. T., Bayogan, E. R. V., Secretaria, L. B., Tac-an, M. I. A., Lubaton, C. D. S. (2021). Ethephon as an Alternative Ripening Agent for ‘Cardaba’ Banana (Musa acuminata x M. balbisiana). Mindanao J. Sci. Technol. 19, 40–58. doi: 10.61310/mndjstecbe.0909.21
Lalel, H. J. D., Singh, Z., Tan, S. C. (2003a). The role of ethylene in mango fruit aroma volatiles biosynthesis. J. Hortic. Sci. Biotechnol. 78, 485–496. doi: 10.1080/14620316.2003.11511653
Lalel, H. J. D., Singh, Z., Tan, S. C. (2003b). The role of methyl jasmonate in mango ripening and biosynthesis of aroma volatile compounds. J. Hortic. Sci. Biotechnol. 78, 470–484. doi: 10.1080/14620316.2003.11511652
Lama, K., Harlev, G., Shafran, H., Peer, R., Flaishman, M. A. (2020). Anthocyanin accumulation is initiated by abscisic acid to enhance fruit color during fig (Ficus carica L.) ripening. J. Plant Physiol. 251, 153192. doi: 10.1016/j.jplph.2020.153192
Larson, J. E., Malladi, A., Kon, T. M. (2023). Xylem functionality is not a direct indicator of apple preharvest fruit drop. J. American Soc. Hortic. Sci. 148, 117–125. doi: 10.21273/JASHS05302-23
Lee, Y.-S., Chung, D.-S., Harte, B. R., Shin, J.-M. (2010). Effect of 1-methylcyclopropene (1-MCP) treatment on the quality characteristics and pigmentation of tomato fruit (Lycopersicon esculentum mill.). Hortic. Sci. Technol. 28, 600–608.
Li, B.-J., Grierson, D., Shi, Y., Chen, K.-S. (2022a). Roles of abscisic acid in regulating ripening and quality of strawberry, a model non-climacteric fruit. Horticulture Res. 9, uhac089. doi: 10.1093/hr/uhac089
Li, X., Liu, P., Zhou, J., Su, M., Ma, Y., Jia, H., et al. (2021). Effects of exogenous application of GA4 + 7 and NAA on sugar accumulation and related gene expression in peach fruits during developing and ripening stages. J. Plant Growth Regul. 40, 962–973. doi: 10.1007/s00344-020-10150-z
Li, J., Min, D., Li, Z., Fu, X., Zhao, X., Wang, J., et al. (2022b). Regulation of sugar metabolism by methyl jasmonate to improve the postharvest quality of tomato fruit. J. Plant Growth Regul. 41, 1615–1626. doi: 10.1007/s00344-021-10415-1
Li, J., Tao, X., Li, L., Mao, L., Luo, Z., Khan, Z. U., et al. (2016). Comprehensive RNA-seq analysis on the regulation of tomato ripening by exogenous auxin. PloS One 11, e0156453. doi: 10.1371/journal.pone.0156453
Li, H., Wu, H., Qi, Q., Li, H., Li, Z., Chen, S., et al. (2019). Gibberellins play a role in regulating tomato fruit ripening. Plant Cell Physiol. 60, 1619–1629. doi: 10.1093/pcp/pcz069
Li, T., Xu, Y., Zhang, L., Ji, Y., Tan, D., Yuan, H., et al. (2017). The jasmonate-activated transcription factor mdMYC2 regulates ETHYLENE RESPONSE FACTOR and ethylene biosynthetic genes to promote ethylene biosynthesis during apple fruit ripening. Plant Cell 29, 1316–1334. doi: 10.1105/tpc.17.00349
Li, J., Yuan, R. (2008). NAA and ethylene regulate expression of genes related to ethylene biosynthesis, perception, and cell wall degradation during fruit abscission and ripening in ‘Delicious’ Apples. J. Plant Growth Regul. 27, 283–295. doi: 10.1007/s00344-008-9055-6
Li, S., Zhu, B., Pirrello, J., Xu, C., Zhang, B., Bouzayen, M., et al. (2020). Roles of RIN and ethylene in tomato fruit ripening and ripening-associated traits. New Phytol. 226, 460–475. doi: 10.1111/nph.v226.2
Li, J., Zhu, H., Yuan, R. (2010). Profiling the expression of genes related to ethylene biosynthesis, ethylene perception, and cell wall degradation during fruit abscission and fruit ripening in apple. J. Am. Soc. Hortic. Sci. 135, 391–401. doi: 10.21273/JASHS.135.5.391
Liang, B., Zheng, Y., Wang, J., Zhang, W., Fu, Y., Kai, W., et al. (2020). Overexpression of the persimmon abscisic acid β-glucosidase gene (DkBG1) alters fruit ripening in transgenic tomato. Plant J. 102, 1220–1233. doi: 10.1111/tpj.v102.6
Liu, M., Gomes, B. L., Mila, I., Purgatto, E., Peres, L. E. P., Frasse, P., et al. (2016). Comprehensive profiling of ethylene response factor expression identifies ripening-associated ERF genes and their link to key regulators of fruit ripening in tomato. Plant Physiol. 170, 1732–1744. doi: 10.1104/pp.15.01859
Liu, J., Islam, M. T., Sherif, S. M. (2022). Effects of aminoethoxyvinylglycine (AVG) and 1-methylcyclopropene (1-MCP) on the pre-harvest drop rate, fruit quality, and stem-end splitting in ‘Gala’ Apples. Horticulturae 8, 1100. doi: 10.3390/horticulturae8121100
Liu, Y., Tang, M., Liu, M., Su, D., Chen, J., Gao, Y., et al. (2020). The molecular regulation of ethylene in fruit ripening. Small Methods 4, 1900485. doi: 10.1002/smtd.201900485
Lobo, M., Bassett, M. J., Hannah, L. C. (1984). Inheritance and characterization of the fruit ripening mutation in ‘Alcobaca’ Tomato. J. American Soc. Hortic. Sci. 109, 741–745. doi: 10.21273/JASHS.109.5.741
Lu, C., Xu, H., Zhao, Y. (1993). Physiological analysis on long shelf-life in ripening mutants (rin, nor, alc) tomato and their F1. J. Jiangsu Agric. College/Jiangsu Nongxueyuan Xuebao 14, 61–66.
Lv, J., Zhang, M., Bai, L., Han, X., Ge, Y., Wang, W., et al. (2020). Effects of 1-methylcyclopropene (1-MCP) on the expression of genes involved in the chlorophyll degradation pathway of apple fruit during storage. Food Chem. 308, 125707. doi: 10.1016/j.foodchem.2019.125707
Ma, Q.-J., Sun, M.-H., Lu, J., Liu, Y.-J., Hu, D.-G., Hao, Y.-J. (2017). Transcription factor AREB2 is involved in soluble sugar accumulation by activating sugar transporter and amylase genes. Plant Physiol. 174, 2348–2362. doi: 10.1104/pp.17.00502
Mainardi, J. A., Purgatto, E., Vieira, A., Bastos, W. A., Cordenunsi, B. R., Oliveira do Nascimento, J. R., et al. (2006). Effects of ethylene and 1-methylcyclopropene (1-MCP) on gene expression and activity profile of α-1,4-glucan-phosphorylase during banana ripening. J. Agric. Food Chem. 54, 7294–7299. doi: 10.1021/jf061180k
Malladi, A. (2020). “Molecular Physiology of Fruit Growth in Apple,” in Horticultural Reviews (Hoboken, New Jersey, USA: John Wiley & Sons, Ltd), 1–42.
Malladi, A., Tonapi, K. V., Kon, T. M. (2023). Aminoethoxyvinylglycine reduces preharvest fruit drop and fruit ethylene evolution in ‘Red delicious’ Apple but affects fruit size and quality inconsistently. HortScience 58, 1410–1417. doi: 10.21273/HORTSCI17287-23
Marcelle, R. D. (1991). Relationships between mineral content, lipoxygenase activity, levels of 1-aminocyclopropane-1-carboxylic acid and ethylene emission in apple fruit flesh disks (cv. Jonagold) during storage. Postharvest Biol. Technol. 1, 101–109. doi: 10.1016/0925-5214(91)90001-R
Marin, A. B., Colonna, A. E., Kudo, K., Kupferman, E. M., Mattheis, J. P. (2009). Measuring consumer response to ‘Gala’ apples treated with 1-methylcyclopropene (1-MCP). Postharvest Biol. Technol. 51, 73–79. doi: 10.1016/j.postharvbio.2008.06.008
Martínez-Madrid, M. C., Flores, F., Romojaro, F. (2002). Behaviour of abscisic acid and polyamines in antisense ACC oxidase melon (Cucumis melo) during ripening. Funct. Plant Biol. 29, 865–872. doi: 10.1071/PP01164
McGarry, R., Ozga, J. A., Reinecke, D. M. (2005). The effects of ethephon on saskatoon (Amelanchier alnifolia nutt.) fruit ripening. J. American Soc. Hortic. Sci. 130, 12–17. doi: 10.21273/JASHS.130.1.12
McGlasson, W. B., Rath, A. C., Legendre, L. (2005). Preharvest application of aminoethoxyvinylglycine (AVG) modifies harvest maturity and cool storage life of ‘Arctic Snow’ nectarines. Postharvest Biol. Technol. 36, 93–102. doi: 10.1016/j.postharvbio.2004.10.006
Meng, F., Liu, H., Hu, S., Jia, C., Zhang, M., Li, S., et al. (2023). The brassinosteroid signaling component SlBZR1 promotes tomato fruit ripening and carotenoid accumulation. J. Integr. Plant Biol. 65, 1794–1813. doi: 10.1111/jipb.13491
Merchante, C., Alonso, J. M., Stepanova, A. N. (2013). Ethylene signaling: simple ligand, complex regulation. Curr. Opin. Plant Biol. 16, 554–560. doi: 10.1016/j.pbi.2013.08.001
Mitalo, O. W., Tokiwa, S., Kondo, Y., Otsuki, T., Galis, I., Suezawa, K., et al. (2019). Low temperature storage stimulates fruit softening and sugar accumulation without ethylene and aroma volatile production in kiwifruit. Front. Plant Sci. 10. doi: 10.3389/fpls.2019.00888
Mo, Y., Gong, D., Liang, G., Han, R., Xie, J., Li, W. (2008). Enhanced preservation effects of sugar apple fruits by salicylic acid treatment during post-harvest storage. J. Sci. Food Agric. 88, 2693–2699. doi: 10.1002/jsfa.v88:15
Mou, W., Li, D., Bu, J., Jiang, Y., Khan, Z. U., Luo, Z., et al. (2016). Comprehensive analysis of ABA effects on ethylene biosynthesis and signaling during tomato fruit ripening. PloS One 11, e0154072. doi: 10.1371/journal.pone.0154072
Mou, W., Li, D., Luo, Z., Li, L., Mao, L., Ying, T. (2018). SlAREB1 transcriptional activation of NOR is involved in abscisic acid-modulated ethylene biosynthesis during tomato fruit ripening. Plant Sci. 276, 239–249. doi: 10.1016/j.plantsci.2018.07.015
Muday, G. K., Rahman, A., Binder, B. M. (2012). Auxin and ethylene: collaborators or competitors? Trends Plant Sci. 17, 181–195. doi: 10.1016/j.tplants.2012.02.001
Muramatsu, N., Sakurai, N., Wada, N., Yamamoto, R., Tanaka, K., Asakura, T., et al. (2000). Remote sensing of fruit textural changes with a laser doppler vibrometer. J. American Soc. Hortic. Sci. 125, 120–127. doi: 10.21273/JASHS.125.1.120
Murray, A. J., Hobson, G. E., Schuch, W., Bird, C. R. (1993). Reduced ethylene synthesis in EFE antisense tomatoes has differential effects on fruit ripening processes. Postharvest Biol. Technol. 2, 301–313. doi: 10.1016/0925-5214(93)90035-2
Mutschler, M. A. (1984). Ripening and storage characteristics of the ‘Alcobaca’ Ripening mutant in tomato. J. Am. Soc. Hortic. Sci. 109, 504–507. doi: 10.21273/JASHS.109.4.504
Nakano, R., Yonemori, K., Sugiura, A., Kataoka, I. (1997). Effect of gibberellic acid and abscisic acid on fruit respiration in relation to final swell and maturation in persimmon. Acta Hortic. 436, 203–214. doi: 10.17660/ActaHortic.1997.436.23
Ni, J., Zhao, Y., Tao, R., Yin, L., Gao, L., Strid, Å., et al. (2020). Ethylene mediates the branching of the jasmonate-induced flavonoid biosynthesis pathway by suppressing anthocyanin biosynthesis in red Chinese pear fruits. Plant Biotechnol. J. 18, 1223–1240. doi: 10.1111/pbi.13287
Obroucheva, N. V. (2014). Hormonal regulation during plant fruit development. Russian J. Dev. Biol. 45, 11–21. doi: 10.1134/S1062360414010068
Oeller, P. W., Lu, M.-W., Taylor, L. P., Pike, D. A., Theologis, A. A. (1991). Reversible inhibition of tomato fruit senescence by antisense RNA. Science 254, 437–439. doi: 10.1126/science.1925603
Ozturk, B., Karakaya, O., Ozkan, Y., Yildiz, K., Medeni, K., Koç Güler, S. (2019). The effects of pre-harvest naphthalene acetic acid (NAA) treatments on fruit quality attributes of braeburn apples during cold storage. J. Exp. Agric. Int. 33, 1–7. doi: 10.9734/jeai/2019/v33i630159
Ozturk, B., Yildiz, K., Ozkan, Y., Kilic, K. (2015). Effects of aminoethoxyvinylglycine treatments on pre-harvest fruit drop and fruit quality of Braeburn apples. Bangladesh J. Bot. 44, 299–307. doi: 10.3329/bjb.v44i2.38520
Park, M.-H., Malka, S. K. (2022). Gibberellin delays metabolic shift during tomato ripening by inducing auxin signaling. Front. Plant Sci. 13. doi: 10.3389/fpls.2022.1045761
Paull, R. E., Chen, N. J. (1983). Postharvest Variation in Cell Wall-Degrading Enzymes of Papaya (Carica papaya L.) during Fruit Ripening. Plant Physiol. 72, 382–385. doi: 10.1104/pp.72.2.382
Paz, O., Janes, H. W., Prevost, B. A., Frenkel, C. (1982). Enhancement of fruit sensory quality by post-harvest applications of acetaldehyde and ethanol. J. Food Sci. 47, 270–273. doi: 10.1111/j.1365-2621.1982.tb11076.x
Pech, J. C., Bouzayen, M., Latché, A. (2008). Climacteric fruit ripening: Ethylene-dependent and independent regulation of ripening pathways in melon fruit. Plant Sci. 175, 114–120. doi: 10.1016/j.plantsci.2008.01.003
Pech, J.-C., El-Yahyaoui, F., Bernadac, A., Latché, A., de Billerbeck, G., Ambid, C., et al. (2001). “Role of ethylene on various ripening pathways and on the development of sensory quality of Charentais cantaloupe melons,” in 2nd International Symposium on Cucurbits, Tsukuba, Japan. 1–9.
Peng, Y., Yang, J., Li, X., Zhang, Y. (2021). Salicylic acid: biosynthesis and signaling. Annu. Rev. Plant Biol. 72, 761–791. doi: 10.1146/annurev-arplant-081320-092855
Pérez-Llorca, M., Muñoz, P., Müller, M., Munné-Bosch, S. (2019). Biosynthesis, metabolism and function of auxin, salicylic acid and melatonin in climacteric and non-climacteric fruits. Front. Plant Sci. 10. doi: 10.3389/fpls.2019.00136
Phan-Thien, K.-Y., Wargo, J. M., Mitchell, L. W., Collett, M. G., Rath, A. C. (2004). Delay in ripening of Gala and Pink Lady apples in commercial orchards following pre-harvest applications of aminoethoxyvinylglycine. Aust. J. Exp. Agric. 44, 807–812. doi: 10.1071/EA03136
Phuong, L. T., Fitrianti, A. N., Luan, M. T., Matsui, H., Noutoshi, Y., Yamamoto, M., et al. (2020). Antagonism between SA- and JA-signaling conditioned by saccharin in Arabidopsis thaliana renders resistance to a specific pathogen. Journal of General Plant Pathology. 86, 86–99. doi: 10.1007/s10327-019-00899-x
Polko, J. K., Kieber, J. J. (2019). 1-aminocyclopropane 1-carboxylic acid and its emerging role as an ethylene-independent growth regulator. Front. Plant Sci. 10. doi: 10.3389/fpls.2019.01602
Ponce, N. M. A., Ziegler, V. H., Stortz, C. A., Sozzi, G. O. (2010). Compositional Changes in Cell Wall Polysaccharides from Japanese Plum (Prunus salicina Lindl.) during Growth and On-Tree Ripening. J. Agric. Food Chem. 58, 2562–2570. doi: 10.1021/jf9039099
Prasanna, V., Prabha, T. N., Tharanathan, R. N. (2007). Fruit ripening phenomena–an overview. Crit. Rev. Food Sci. Nutr. 47, 1–19. doi: 10.1080/10408390600976841
Pristijono, P., Golding, J. B., Bowyer, M. C. (2019). Postharvest UV-C treatment, followed by storage in a continuous low-level ethylene atmosphere, maintains the quality of ‘Kensington pride’ Mango fruit stored at 20°C. Horticulturae 5, 1. doi: 10.3390/horticulturae5010001
Rehman, M. U., Rather, G. H., Gull, Y., Mir, M. M., Umar, I. (2017). Effect of 1-naphthaleneacetic acid and calcium chloride on preharvest drop and quality of ‘Red Delicious’ apples. Can. J. Plant Sci. 97, 902–905. doi: 10.1139/cjps-2016-0359
Reid, M. S., Padfield, C. A. S., Watkins, C. B., Harman, J. E. (1982). Starch iodine pattern as a maturity index for Granny Smith apples: 1. Comparison with flesh firmness and soluble solids content. New Z. J. Agric. Res. 25, 229–237. doi: 10.1080/00288233.1982.10420918
Rose, J. K. C., Hadfield, K. A., Labavitch, J. M., Bennett, A. B. (1998). Temporal sequence of cell wall disassembly in rapidly ripening melon fruit. Plant Physiol. 117, 345–361. doi: 10.1104/pp.117.2.345
Rossetto, M. R. M., Purgatto, E., do Nascimento, J. R. O., Lajolo, F. M., Cordenunsi, B. R. (2003). Effects of gibberellic acid on sucrose accumulation and sucrose biosynthesizing enzymes activity during banana ripening. Plant Growth Regul. 41, 207–214. doi: 10.1023/B:GROW.0000007508.91064.8c
Rozo-Romero, L. X., Álvarez-Herrera, J. G., Balaguera-López, H. E. (2015). Ethylene and changes during ripening in ‘Horvin’ plum (Prunus salicina Lindl.) fruits. Agronomía Colombiana 33, 228–237. doi: 10.15446/agron.colomb.v33n2.49856
Sagar, M., Chervin, C., Mila, I., Hao, Y., Roustan, J. P., Benichou, M., et al. (2013). SlARF4, an auxin response factor involved in the control of sugar metabolism during tomato fruit development. Plant Physiol. 161, 1362–1374. doi: 10.1104/pp.113.213843
Sang, K., Li, J., Qian, X., Yu, J., Zhou, Y., Xia, X. (2022). The APETALA2a/DWARF/BRASSINAZOLE-RESISTANT 1 module contributes to carotenoid synthesis in tomato fruits. Plant J. 112, 1238–1251. doi: 10.1111/tpj.v112.5
Satekge, T. K., Magwaza, L. S. (2020). The combined effect of 1-methylcyclopropene (1-MCP) and ethylene on green-life and postharvest quality of banana fruit. Int. J. Fruit Sci. 20, S1539–S1551. doi: 10.1080/15538362.2020.1818162
Schultz, E. E., Mallmann Wendt, L., Ludwig, V., Thewes, F. R., Pasquetti Berghetti, M. R., Brackmann, A., et al. (2023). Aminoethoxyvinylglycine, naphthalene acetic acid and ethephon: impacts on pre-harvest fruit drop, volatile compounds profile, and overall quality of ‘Galaxy’ Apples. Erwerbs-Obstbau 65, 7–23. doi: 10.1007/s10341-022-00691-w
Schupp, J. R., Greene, D. W. (2004). Effect of aminoethoxyvinylglycine (AVG) on preharvest drop, fruit quality, and maturation of `McIntosh’ Apples. I. Concentration and timing of dilute applications of AVG. HortScience 39, 1030–1035. doi: 10.21273/HORTSCI.39.5.1030
Shafiq, M., Singh, Z., Khan, A. S. (2013). Time of methyl jasmonate application influences the development of ‘Cripps Pink’ apple fruit colour. J. Sci. Food Agric. 93, 611–618. doi: 10.1002/jsfa.2013.93.issue-3
Shin, S., Lee, J., Rudell, D., Evans, K., Zhu, Y. (2016). Transcriptional regulation of auxin metabolism and ethylene biosynthesis activation during apple (Malus × domestica) fruit maturation. J. Plant Growth Regul. 35, 655–666. doi: 10.1007/s00344-015-9568-8
Silverman, F. P., Petracek, P. D., Noll, M. R., Warrior, P. (2004). Aminoethoxyvinylglycine effects on late-season apple fruit maturation. Plant Growth Regul. 43, 153–161. doi: 10.1023/B:GROW.0000040113.05826.d2
Singh, Z., Janes, J. (2001). Effects of postharvest application of ethephon on fruit ripening, quality and shelf life of mango under modified atmosphere packaging. Acta Hortic. 553, 599–602. doi: 10.17660/ActaHortic.2001.553.141
Sisler, E. C., Serek, M. (1997). Inhibitors of ethylene responses in plants at the receptor level: Recent developments. Physiologia Plantarum 100, 577–582. doi: 10.1111/j.1399-3054.1997.tb03063.x
Sisler, E. C., Serek, M., Dupille, E., Goren, R. (1999). Inhibition of ethylene responses by 1-Methylcyclopropene and 3-Methylcyclopropene. Plant Growth Regul. 27, 105–111. doi: 10.1023/A:1006153016409
Sitrit, Y., Riov, J., Blumenfeld, A. (1986). Regulation of ethylene biosynthesis in avocado fruit during ripening. Plant Physiol. 81, 130–135. doi: 10.1104/pp.81.1.130
Smith, R. B., Lougheed, E. C., Franklin, E. W., Mcmillan, I. (1979). The starch iodine test for determining stage of maturation in apples. Can. J. Plant Sci. 59, 725–735. doi: 10.4141/cjps79-113
Sobolev, A. P., Neelam, A., Fatima, T., Shukla, V., Handa, A. K., Mattoo, A. K. (2014). Genetic introgression of ethylene-suppressed transgenic tomatoes with higher-polyamines trait overcomes many unintended effects due to reduced ethylene on the primary metabolome. Front. Plant Sci. 5. doi: 10.3389/fpls.2014.00632
Soto, A., Ruiz, K. B., Ravaglia, D., Costa, G., Torrigiani, P. (2013). ABA may promote or delay peach fruit ripening through modulation of ripening- and hormone-related gene expression depending on the developmental stage. Plant Physiol. Biochem. 64, 11–24. doi: 10.1016/j.plaphy.2012.12.011
Sriskantharajah, K., El Kayal, W., Torkamaneh, D., Ayyanath, M. M., Saxena, P. K., Sullivan, A. J., et al. (2021). Transcriptomics of improved fruit retention by hexanal in ‘Honeycrisp’ Reveals hormonal crosstalk and reduced cell wall degradation in the fruit abscission zone. Int. J. Mol. Sci. 22, 8830. doi: 10.3390/ijms22168830
Srivastava, M. K., Dwivedi, U. N. (2000). Delayed ripening of banana fruit by salicylic acid. Plant Sci. 158, 87–96. doi: 10.1016/S0168-9452(00)00304-6
Steffens, C. A., Guarienti, A. J. W., Storck, L., Brackmann, A. (2006). Maturation of the ‘Gala’ apple with preharvest sprays of aminoethoxyvynilglycine and ethephon. Ciec. Rural 36, 434–440. doi: 10.1590/S0103-84782006000200012
Stepanova, A. N., Hoyt, J. M., Hamilton, A. A., Alonso, J. M. (2005). A link between ethylene and auxin uncovered by the characterization of two root-specific ethylene-insensitive mutants in arabidopsis. Plant Cell 17, 2230–2242. doi: 10.1105/tpc.105.033365
Stover, E., Fargione, M. J., Watkins, C. B., Iungerman, K. A. (2003). Harvest management of marshall `McIntosh’ Apples: effects of AVG, NAA, ethephon, and summer pruning on preharvest drop and fruit quality. HortScience 38, 1093–1099. doi: 10.21273/HORTSCI.38.6.1093
Su, L., Diretto, G., Purgatto, E., Danoun, S., Zouine, M., Li, Z., et al. (2015). Carotenoid accumulation during tomato fruit ripening is modulated by the auxin-ethylene balance. BMC Plant Biol. 15, 114. doi: 10.1186/s12870-015-0495-4
Sun, T., Gubler, F. (2004). Molecular mechanism of gibberellin signaling in plants. Annu. Rev. Plant Biol. 55, 197–223. doi: 10.1146/annurev.arplant.55.031903.141753
Symons, G. M., Chua, Y.-J., Ross, J. J., Quittenden, L. J., Davies, N. W., Reid, J. B. (2012). Hormonal changes during non-climacteric ripening in strawberry. J. Exp. Bot. 63, 4741–4750. doi: 10.1093/jxb/ers147
Tacken, E., Ireland, H., Gunaseelan, K., Karunairetnam, S., Wang, D., Schultz, K., et al. (2010). The role of ethylene and cold temperature in the regulation of the apple POLYGALACTURONASE1 gene and fruit softening. Plant Physiol. 153, 294–305. doi: 10.1104/pp.109.151092
Tadiello, A., Longhi, S., Moretto, M., Ferrarini, A., Tononi, P., Farneti, B., et al. (2016). Interference with ethylene perception at receptor level sheds light on auxin and transcriptional circuits associated with the climacteric ripening of apple fruit (Malus x domestica Borkh.). Plant J. 88, 963–975. doi: 10.1111/tpj.2016.88.issue-6
Tang, T., Zhou, H., Wang, L., Zhao, J., Ma, L., Ling, J., et al. (2022). Post-harvest application of methyl jasmonate or prohydrojasmon affects color development and anthocyanins biosynthesis in peach by regulation of sucrose metabolism. Front. Nutr. 9. doi: 10.3389/fnut.2022.871467
Tao, X., Wu, Q., Li, J., Huang, S., Cai, L., Mao, L., et al. (2022). Exogenous methyl jasmonate regulates phenolic compounds biosynthesis during postharvest tomato ripening. Postharvest Biol. Technol. 184, 111760. doi: 10.1016/j.postharvbio.2021.111760
Tatsuki, M., Nakajima, N., Fujii, H., Shimada, T., Nakano, M., Hayashi, K., et al. (2013). Increased levels of IAA are required for system 2 ethylene synthesis causing fruit softening in peach (Prunus persica L. Batsch). J. Exp. Bot. 64, 1049–1059. doi: 10.1093/jxb/ers381
Taye, A. M., Tilahun, S., Seo, M. H., Park, D. S., Jeong, C. S. (2019). Effects of 1-MCP on quality and storability of cherry tomato (Solanum lycopersicum L.). Horticulturae 5, 29. doi: 10.3390/horticulturae5020029
Thammawong, M., Arakawa, O. (2007). Starch degradation of detached apple fruit in relation to ripening and ethylene. J. Japanese Soc. Hortic. Sci. 76, 345–350. doi: 10.2503/jjshs.76.345
Tomala, K., Grzęda, M., Guzek, D., Głąbska, D., Gutkowska, K. (2020). The effects of preharvest 1-methylcyclopropene (1-MCP) treatment on the fruit quality parameters of cold-stored ‘Szampion’ Cultivar apples. Agriculture 10, 80. doi: 10.3390/agriculture10030080
Tonutti, P., Bonghi, C., Ramina, A. (1996). Fruit firmness and ethylene biosynthesis in three cultivars of peach (Prunus persica L. Batsch). J. Hortic. Sci. 71, 141–147. doi: 10.1080/14620316.1996.11515390
Tonutti, P., Cass, L. G., Christoffersen, R. E. (1995). The expression of cellulase gene family members during induced avocado fruit abscission and ripening. Plant Cell Environ. 18, 709–713. doi: 10.1111/j.1365-3040.1995.tb00573.x
Trainotti, L., Tadiello, A., Casadoro, G. (2007). The involvement of auxin in the ripening of climacteric fruits comes of age: the hormone plays a role of its own and has an intense interplay with ethylene in ripening peaches. J. Exp. Bot. 58, 3299–3308. doi: 10.1093/jxb/erm178
Tsang, D. L., Edmond, C., Harrington, J. L., Nühse, T. S. (2011). Cell wall integrity controls root elongation via a general 1-aminocyclopropane-1-carboxylic acid-dependent, ethylene-independent pathway. Plant Physiol. 156, 596–604. doi: 10.1104/pp.111.175372
Tsantili, E., Knee, M. (1991). Ripening of Gloster 69 apples after low-ethylene storage. J. Sci. Food Agric. 54, 579–586. doi: 10.1002/jsfa.2740540409
Tucker, G., Yin, X., Zhang, A., Wang, M., Zhu, Q., Liu, X., et al. (2017). Ethylene† and fruit softening. Food Qual. Saf. 1, 253–267. doi: 10.1093/fqsafe/fyx024
Ustun, H., Dogan, A., Peker, B., Ural, C., Cetin, M., Ozyigit, Y., et al. (2023). Determination of the relationship between respiration rate and ethylene production by fruit sizes of different tomato types. J. Sci. Food Agric. 103, 176–184. doi: 10.1002/jsfa.v103.1
Vanderstraeten, L., Depaepe, T., Bertrand, S., van der Straeten, D. (2019). The ethylene precursor ACC affects early vegetative development independently of ethylene signaling. Front. Plant Sci. 10. doi: 10.3389/fpls.2019.01591
Villalobos-Acuña, M. G., Biasi, W. V., Flores, S., Mitcham, E. J., Elkins, R. B., Willits, N. H. (2010). Preharvest application of 1-methylcyclopropene influences fruit drop and storage potential of ‘Bartlett’ Pears. HortScience 45, 610–616. doi: 10.21273/HORTSCI.45.4.610
Wakasa, Y., Hatsuyama, Y., Takahashi, A., Sato, T., Niizeki, M., Harada, T. (2003). Divergent Expression of six Expansin Genes during Apple Fruit Ontogeny. Eur. J. Hortic. Sci. 68, 253–259.
Wang, X., Cao, X., Shang, Y., Bu, H., Wang, T., Lyu, D., et al. (2020a). Preharvest application of prohydrojasmon affects color development, phenolic metabolism, and pigment-related gene expression in red pear. J. Sci. Food Agric. 100, 4766–4775. doi: 10.1002/jsfa.v100.13
Wang, Z., Dilley, D. R. (2001). Aminoethoxyvinylglycine, combined with ethephon, can enhance red color development without over-ripening apples. HortScience 36, 328–331. doi: 10.21273/HORTSCI.36.2.328
Wang, K. L.-C., Li, H., Ecker, J. R. (2002). Ethylene biosynthesis and signaling networks. Plant Cell 14, S131–S151. doi: 10.1105/tpc.001768
Wang, S., Li, L.-X., Fang, Y., Li, D., Mao, Z., Zhu, Z., et al. (2022a). MdERF1B–MdMYC2 module integrates ethylene and jasmonic acid to regulate the biosynthesis of anthocyanin in apple. Horticulture Res. 9, uhac142. doi: 10.1093/hr/uhac142
Wang, S., Li, L.-X., Zhang, Z., Fang, Y., Li, D., Chen, X.-S., et al. (2022b). Ethylene precisely regulates anthocyanin synthesis in apple via a module comprising MdEIL1, MdMYB1, and MdMYB17. Horticulture Res. 9, uhac034. doi: 10.1093/hr/uhac034
Wang, Y., Wang, N., Xu, H., Jiang, S., Fang, H., Su, M., et al. (2018a). Auxin regulates anthocyanin biosynthesis through the Aux/IAA–ARF signaling pathway in apple. Horticulture Res. 5, 59. doi: 10.1038/s41438-018-0068-4
Wang, X., Wang, Y., Yan, S., Sun, X., Liu, H., Cheng, B., et al. (2022c). A multifaceted comparison between the fruit-abscission and fruit-retention cultivars in ornamental crabapple. Front. Plant Sci. 13. doi: 10.3389/fpls.2022.1013263
Wang, D., Yeats, T. H., Uluisik, S., Rose, J. K. C., Seymour, G. B. (2018b). Fruit softening: revisiting the role of pectin. Trends Plant Sci. 23, 302–310. doi: 10.1016/j.tplants.2018.01.006
Wang, S., Zhou, Q., Zhou, X., Zhang, F., Ji, S. (2020b). Ethylene plays an important role in the softening and sucrose metabolism of blueberries postharvest. Food Chem. 310, 125965. doi: 10.1016/j.foodchem.2019.125965
Watkins, C. B., Nock, J. F., Whitaker, B. D. (2000). Responses of early, mid and late season apple cultivars to postharvest application of 1-methylcyclopropene (1-MCP) under air and controlled atmosphere storage conditions. Postharvest Biol. Technol. 19, 17–32. doi: 10.1016/S0925-5214(00)00070-3
Wei, Y., Jin, J., Xu, Y., Liu, W., Yang, G., Bu, H., et al. (2021). Ethylene-activated MdPUB24 mediates ubiquitination of MdBEL7 to promote chlorophyll degradation in apple fruit. Plant J. 108, 169–182. doi: 10.1111/tpj.v108.1
Wei, J., Wen, X., Tang, L. (2017). Effect of methyl jasmonic acid on peach fruit ripening progress. Scientia Hortic. 220, 206–213. doi: 10.1016/j.scienta.2017.03.004
Whale, S. K., Singh, Z. (2007). Endogenous ethylene and color development in the skin of ‘Pink lady’ Apple. J. Am. Soc. Hortic. Sci. 132, 20–28. doi: 10.21273/JASHS.132.1.20
Whale, S. K., Singh, Z., Behboudian, M. H., Janes, J., Dhaliwal, S. S. (2008). Fruit quality in ‘Cripp’s Pink’ apple, especially colour, as affected by preharvest sprays of aminoethoxyvinylglycine and ethephon. Scientia Hortic. 115, 342–351. doi: 10.1016/j.scienta.2007.10.015
Wilkinson, J. Q., Lanahan, M. B., Yen, H. C., Giovannoni, J. J., Klee, H. J. (1995). An ethylene-inducible component of signal transduction encoded by never-ripe. Science 270, 1807–1809. doi: 10.1126/science.270.5243.1807
Win, N. M., Yoo, J., Kwon, S.-I., Watkins, C. B., Kang, I.-K. (2019). Characterization of fruit quality attributes and cell wall metabolism in 1-methylcyclopropene (1-MCP)-treated ‘Summer king’ and ‘Green ball’ Apples during cold storage. Front. Plant Sci. 10. doi: 10.3389/fpls.2019.01513
Win, N. M., Yoo, J., Naing, A. H., Kwon, J.-G., Kang, I.-K. (2021). 1-Methylcyclopropene (1-MCP) treatment delays modification of cell wall pectin and fruit softening in “Hwangok” and “Picnic” apples during cold storage. Postharvest Biol. Technol. 180, 111599. doi: 10.1016/j.postharvbio.2021.111599
Wu, M., Liu, K., Li, H., Li, Y., Zhu, Y., Su, D., et al. (2024). Gibberellins involved in fruit ripening and softening by mediating multiple hormonal signals in tomato. Horticulture Res. 11, uhad275. doi: 10.1093/hr/uhad275
Wu, Q., Tao, X., Ai, X., Luo, Z., Mao, L., Ying, T., et al. (2018a). Effect of exogenous auxin on aroma volatiles of cherry tomato (Solanum lycopersicum L.) fruit during postharvest ripening. Postharvest Biol. Technol. 146, 108–116. doi: 10.1016/j.postharvbio.2018.08.010
Wu, Q., Tao, X., Ai, X., Luo, Z., Mao, L., Ying, T., et al. (2018b). Contribution of abscisic acid to aromatic volatiles in cherry tomato (Solanum lycopersicum L.) fruit during postharvest ripening. Plant Physiol. Biochem. 130, 205–214. doi: 10.1016/j.plaphy.2018.06.039
Wu, S., Wu, D., Song, J., Zhang, Y., Tan, Q., Yang, T., et al. (2022). Metabolomic and transcriptomic analyses reveal new insights into the role of abscisic acid in modulating mango fruit ripening. Horticulture Res. 9, uhac102. doi: 10.1093/hr/uhac102
Xu, Y., Li, S., Huan, C., Jiang, T., Zheng, X., Brecht, J. K. (2020). Effects of 1methylcyclopropene treatment on quality and anthocyanin biosynthesis in plum (Prunus salicina cv. Taoxingli) fruit during storage at a non-chilling temperature. Postharvest Biol. Technol. 169, 111291. doi: 10.1016/j.postharvbio.2020.111291
Xu, Y., Huo, L., Zhao, K., Li, Y., Zhao, X., Wang, H., et al. (2023). Salicylic acid delays pear fruit senescence by playing an antagonistic role toward ethylene, auxin, and glucose in regulating the expression of PpEIN3a. Frontiers in Plant Science. 13. doi: 10.3389/fpls.2022.1096645
Xu, F., Liu, S., Xiao, Z., Fu, L. (2019). Effect of ultrasonic treatment combined with 1-methylcyclopropene (1-MCP) on storage quality and ethylene receptors gene expression in harvested apple fruit. J. Food Biochem. 43, e12967. doi: 10.1111/jfbc.12967
Yang, S. F., Hoffman, N. E. (1984). Ethylene biosynthesis and its regulation in higher plants. Annu. Rev. Plant Biol. 35, 155–189. doi: 10.1146/annurev.pp.35.060184.001103
Yang, S. F., Liu, Y., Lau, O. L. (1986). REGULATION OF ETHYLENE BIOSYNTHESIS IN RIPENING APPLE FRUITS. Acta Hortic. 179, 711–720. doi: 10.17660/ActaHortic.1986.179.123
Yang, X., Song, J., Du, L., Forney, C., Campbell-Palmer, L., Fillmore, S., et al. (2016). Ethylene and 1-MCP regulate major volatile biosynthetic pathways in apple fruit. Food Chem. 194, 325–336. doi: 10.1016/j.foodchem.2015.08.018
Yao, G.-F., Li, C., Sun, K.-K., Tang, J., Huang, Z-Q., Yang, F., et al. (2020). Hydrogen sulfide maintained the good appearance and nutrition in post-harvest tomato fruits by antagonizing the effect of ethylene. Front. Plant Sci. 11. doi: 10.3389/fpls.2020.00584
Yao, G.-F., Wei, Z.-Z., Li, T.-T., Tang, J., Huang, Z-Q., Yang, F., et al. (2018). Modulation of enhanced antioxidant activity by hydrogen sulfide antagonization of ethylene in tomato fruit ripening. J. Agric. Food Chem. 66, 10380–10387. doi: 10.1021/acs.jafc.8b03951
Ye, H., Li, L., Yin, Y. (2011). Recent advances in the regulation of brassinosteroid signaling and biosynthesis pathways. J. Integr. Plant Biol. 53, 455–468. doi: 10.1111/j.1744-7909.2011.01046.x
Yildiz, K., Ozturk, B., Ozkan, Y. (2012). Effects of aminoethoxyvinylglycine (AVG) on preharvest fruit drop, fruit maturity, and quality of ‘Red Chief’ apple. Scientia Hortic. 144, 121–124. doi: 10.1016/j.scienta.2012.07.005
Yu, Y.-B., Adams, D. O., Yang, S. F. (1979). 1-Aminocyclopropanecarboxylate synthase, a key enzyme in ethylene biosynthesis. Arch. Biochem. Biophysics 198, 280–286. doi: 10.1016/0003-9861(79)90420-X
Yuan, R., Carbaugh, D. H. (2007). Effects of NAA, AVG, and 1-MCP on ethylene biosynthesis, preharvest fruit drop, fruit maturity, and quality of ‘Golden supreme’ and ‘Golden delicious’ Apples. HortScience 42, 101–105. doi: 10.21273/HORTSCI.42.1.101
Yuan, R., Li, J. (2008). Effect of sprayable 1-MCP, AVG, and NAA on ethylene biosynthesis, preharvest fruit drop, fruit maturity, and quality of ‘Delicious’ Apples. HortScience 43, 1454–1460. doi: 10.21273/HORTSCI.43.5.1454
Yue, P., Lu, Q., Liu, Z., Lv, T., Li, X., Bu, H., et al. (2020). Auxin-activated MdARF5 induces the expression of ethylene biosynthetic genes to initiate apple fruit ripening. New Phytol. 226, 1781–1795. doi: 10.1111/nph.v226.6
Yu-fa, L., Liang-de, A. G., Wen-ji WM and, Y. (1982). The role of polygalacturonase (PG) in the ripening of apple fruits. J. Integr. Plant Biol. 24, 143–146.
Zaharah, S. S., Singh, Z., Symons, G. M., Reid, J. B. (2013). Mode of action of abscisic acid in triggering ethylene biosynthesis and softening during ripening in mango fruit. Postharvest Biol. Technol. 75, 37–44. doi: 10.1016/j.postharvbio.2012.07.009
Zhang, Y., Chen, K., Zhang, S., Ferguson, I. (2003). The role of salicylic acid in postharvest ripening of kiwifruit. Postharvest Biol. Technol. 28, 67–74. doi: 10.1016/S0925-5214(02)00172-2
Zhang, S., Gu, X., Shao, J., Hu, Z., Yang, W., Wang, L., et al. (2021). Auxin metabolism is involved in fruit set and early fruit development in the parthenocarpic tomato “R35-P. Front. Plant Sci. 12. doi: 10.3389/fpls.2021.671713
Zhang, X.-M., Jia, H.-J. (2005). Changes in aroma volatile compounds and ethylene production during ‘Hujingmilu’ peach (Prunus persica L.) fruit development. Zhi wu sheng li yu fen zi sheng wu xue xue bao = J. Plant Physiol. Mol. Biol. 31, 41–46.
Zhang, B., Luo, Y., Kanyuck, K., Bauchan, G., Mowery, J., Zavalij, P. (2016). Development of metal–organic framework for gaseous plant hormone encapsulation to manage ripening of climacteric produce. J. Agric. Food Chem. 64, 5164–5170. doi: 10.1021/acs.jafc.6b02072
Zhang, J., Ma, Y., Dong, C., Terry, L. A., Watkins, C. B., Yu, Z., et al. (2020). Meta-analysis of the effects of 1-methylcyclopropene (1-MCP) treatment on climacteric fruit ripening. Horticulture Res. 7, 208. doi: 10.1038/s41438-020-00405-x
Zhang, S., Wang, H., Wang, T., Zhang, J., Liu, W., Fang, H., et al. (2023a). Abscisic acid and regulation of the sugar transporter gene MdSWEET9b promote apple sugar accumulation. Plant Physiol. 192, 2081–2101. doi: 10.1093/plphys/kiad119
Zhang, J., Wen, M., Dai, R., Liu, X., Wang, C. (2023b). Comparative physiological and transcriptome analyses reveal mechanisms of salicylic-acid-reduced postharvest ripening in ‘Hosui’ Pears (Pyrus pyrifolia nakai). Plants 12, 3429. doi: 10.3390/plants12193429
Zhang, M., Yuan, B., Leng, P. (2009). The role of ABA in triggering ethylene biosynthesis and ripening of tomato fruit. J. Exp. Bot. 60, 1579–1588. doi: 10.1093/jxb/erp026
Zhao, Y., Tang, J., Brummell, D. A., Song, C., Qi, S., Lin, Q., et al. (2022). Abscisic acid alleviates chilling injury in cold-stored peach fruit by regulating the metabolism of sucrose. Scientia Hortic. 298, 111000. doi: 10.1016/j.scienta.2022.111000
Zheng, J.-L., Hu, L.-Y., Hu, K.-D., Wu, J., Yang, F., Zhang, H. (2016). Hydrogen sulfide alleviates senescence of fresh-cut apple by regulating antioxidant defense system and senescence-related gene expression. HortScience 51, 152–158. doi: 10.21273/HORTSCI.51.2.152
Zhu, H., Beers, E. P., Yuan, R. (2008). Aminoethoxyvinylglycine inhibits fruit abscission induced by naphthaleneacetic acid and associated relationships with expression of genes for ethylene biosynthesis, perception, and cell wall degradation in ‘Delicious’ Apples. J. American Soc. Hortic. Sci. 133, 727–734. doi: 10.21273/JASHS.133.6.727
Zhu, T., Tan, W.-R., Deng, X.-G., Zheng, T., Zhang, D.-W., Lin, H.-H. (2015). Effects of brassinosteroids on quality attributes and ethylene synthesis in postharvest tomato fruit. Postharvest Biol. Technol. 100, 196–204. doi: 10.1016/j.postharvbio.2014.09.016
Zhu, X., Ye, L., Ding, X., Gao, Q., Xiao, S., Tan, Q., et al. (2019). Transcriptomic analysis reveals key factors in fruit ripening and rubbery texture caused by 1-MCP in papaya. BMC Plant Biol. 19, 309. doi: 10.1186/s12870-019-1904-x
Zilkah, S., Lurie, S., Lapsker, Z., Zuthi, Y., David, I., Yesselson, Y., et al. (1997). The ripening and storage quality of nectarine fruits in response to preharvest application of gibberellic acid. J. Hortic. Sci. 72, 355–362. doi: 10.1080/14620316.1997.11515522
Ziogas, V., Molassiotis, A., Fotopoulos, V., Tanou, G. (2018). Hydrogen sulfide: A potent tool in postharvest fruit biology and possible mechanism of action. Front. Plant Sci. 9. doi: 10.3389/fpls.2018.01375
Ziosi, V., Bonghi, C., Bregoli, A. M., Trainotti, L., Biondi, S., Sutthiwal, S., et al. (2008a). Jasmonate-induced transcriptional changes suggest a negative interference with the ripening syndrome in peach fruit. J. Exp. Bot. 59, 563–573. doi: 10.1093/jxb/erm331
Ziosi, V., Noferini, M., Fiori, G., Tadiello, A., Trainotti, L., Casadoro, G., et al. (2008b). A new index based on vis spectroscopy to characterize the progression of ripening in peach fruit. Postharvest Biol. Technol. 49, 319–329. doi: 10.1016/j.postharvbio.2008.01.017
Keywords: ripening, ethylene, climacteric fruit, phytohormones, signal transduction, pre-harvest drop
Citation: Tipu MMH and Sherif SM (2024) Ethylene and its crosstalk with hormonal pathways in fruit ripening: mechanisms, modulation, and commercial exploitation. Front. Plant Sci. 15:1475496. doi: 10.3389/fpls.2024.1475496
Received: 04 August 2024; Accepted: 10 October 2024;
Published: 07 November 2024.
Edited by:
Lee Jeong Hwan, Jeonbuk National University, Republic of KoreaReviewed by:
Ke Wang, Anhui Agricultural University, ChinaYao Gaifang, Hefei University of Technology, China
Copyright © 2024 Tipu and Sherif. This is an open-access article distributed under the terms of the Creative Commons Attribution License (CC BY). The use, distribution or reproduction in other forums is permitted, provided the original author(s) and the copyright owner(s) are credited and that the original publication in this journal is cited, in accordance with accepted academic practice. No use, distribution or reproduction is permitted which does not comply with these terms.
*Correspondence: Sherif M. Sherif, c3NoZXJpZkB2dC5lZHU=