- 1Julius Kühn-Institut (JKI), Federal Research Centre for Cultivated Plants, Institute for Breeding Research on Fruit Crops, Dresden, Germany
- 2Julius Kühn-Institut (JKI), Federal Research Centre for Cultivated Plants, Institute for Resistance Research and Stress Tolerance, Quedlinburg, Germany
The bacterial pathogen Erwinia amylovora causes fire blight on rosaceous plants, including apples and their wild relatives. The pathogen uses the type III secretion pathogenicity island to inject effector proteins, such as Eop1, into host plants, leading to disease phenotypes in susceptible genotypes. In contrast, resistant genotypes exhibit quantitative resistance associated with genomic regions and/or R-gene-mediated qualitative resistance to withstand the pathogen. In Malus, strong resistance is observed in some wild species accessions, for example, in Malus xarnoldiana accession MAL0004. The resistance locus FB_Mar12, previously identified on linkage group 12 (LG12) of MAL0004, is one of two gene loci in Malus proven to withstand highly virulent North American strains of E. amylovora. This suggests the influence of a major gene, with a few candidate genes proposed within the FB_Mar12 region. In this report, we provide evidence that this gene locus is completely broken down by a mutant strain of the E. amylovora effector protein Eop1 (Δeop1) following artificial shoot inoculations of an ‘Idared’ × MAL0004 F1 progeny set, indicating a gene-for-gene interaction. Interestingly, Δeop1 does not overcome the resistance of the FB_Mar12 donor MAL0004 itself, but only the QTL on LG12, an indication that other resistance factors, possibly QTLs/genes are contributing to the fire blight resistance of MAL0004.
Introduction
Fire blight is the most destructive bacterial disease of apples (Malus domestica Borkh.) and other rosaceous plants, causing huge economic losses (Norelli et al., 2003; Hasler et al., 2002). The causal pathogen, Erwinia amylovora (Burrill) (Winslow et al., 1920), enters hosts through flowers or wounds on vegetative tissues and deposits effectors via the hypersensitive response and pathogenicity (hrp) type III secretion system (T3SS), resulting in disease in susceptible hosts (Oh et al., 2005; Oh and Beer, 2005; Yuan et al., 2021). Effector proteins secreted and translocated by E. amylovora via the T3SS include DspA/E, AvrRpt2EA, HopPtoCEA, Eop1, and Eop3 (Oh and Beer, 2005; Zhao, 2014; McNally et al., 2015) among other virulence factors and helper proteins (Piqué et al., 2015; Yuan et al., 2021). From the host perspective, genomic regions associated with fire blight resistance have been described in both wild and cultivated apple genotypes (Peil et al., 2021). However, wild apple genotypes exhibit the strongest resistance effects against E. amylovora in Malus, with candidate resistance genes underlying these regions identified only in wild species (Emeriewen et al., 2019). For example, resistance has been associated with linkage group 3 (LG3) of Malus xrobusta 5 (Mr5) (Peil et al., 2007; Fahrentrapp et al., 2013), on LG12 of the ornamental cultivar ‘Evereste’ (Durel et al., 2009; Parravicini et al., 2011), on LG10 of Malus fusca MAL0045 (Emeriewen et al., 2014, 2018, 2022), and on LG12 of Malus xarnoldiana MAL0004 (Emeriewen et al., 2017, 2021).
Furthermore, resistance to E. amylovora is strain-dependent (Vogt et al., 2013; Wöhner et al., 2018). Vogt et al. (2013) demonstrated that strains with a single nucleotide polymorphism (SNP) at position 156 of the amino acid sequence of the E. amylovora effector AvrRpt2EA differ in virulence on Mr5. For example, Ea222, which carries cysteine at this position, is avirulent on Mr5, whereas Ea3049, which carries serine, is virulent and can break down the resistance of Mr5. Peil et al. (2011) also showed that Ea3049 completely broke down the resistance QTL on LG3 of Mr5. Similarly, the deletion of the E. amylovora effector gene avrRpt2EA in a wild-type strain, Ea1189 (ΔavrRpt2EA), led to the breakdown of Mr5 resistance (Vogt et al., 2013) and the resistance gene FB_MR5, which underlies the resistance region on LG3 of Mr5 (Broggini et al., 2014). This provided the first evidence of a gene-for-gene relationship between a Malus host and the E. amylovora pathosystem (Vogt et al., 2013). Furthermore, Wöhner et al. (2018) demonstrated that the wild-type strain Ea1189 did not lead to disease symptoms on ‘Evereste’, M. floribunda 821 (Mf821), and M. xarnoldiana MAL0004—three donors of fire blight resistance that map to the distal end of LG12 (Durel et al., 2009; Emeriewen et al., 2017). Nevertheless, the deletion of the E. amylovora effector gene eop1 (Δeop1) in this wild-type strain led to considerable disease symptoms on ‘Evereste’ and Mf821, but not on M. xarnoldiana MAL0004. This suggests gene-for-gene relationships between eop1 of E. amylovora and the fire blight resistance genes of ‘Evereste’ and Mf821, respectively (Wöhner et al., 2018).
In this brief research report, we confirm that the deletion mutant strain, Δeop1, causes disease on Mf821 but not on MAL0004. However, we report that inoculating the F1 progeny of MAL0004, derived from crosses with the apple cultivar ‘Idared’ (Emeriewen et al., 2017), with Δeop1 leads to the complete breakdown of the resistance QTL of LG12 of MAL0004. We discuss the implications of these results.
Methods
Plant material
As previously reported, ‘Idared’ was crossed with MAL0004 to establish an F1 progeny designated as the 07240 population, which was used to identify the resistance region on LG12 associated with the fire blight resistance of MAL0004 (Emeriewen et al., 2017, 2021). This population, maintained in the orchard of the Julius Kühn Institute, Institute for Breeding Research on Fruit Crops in Dresden-Pillnitz (Germany), served as the basis for this study.
Artificial shoot inoculations
We inoculated the 07240 progeny with the same Δeop1-deletion mutant strain reported in Wöhner et al. (2018). Between six and 10 replicates of 102 individuals from the 07240 population were grafted on rootstock M111 and grown in the greenhouse under conditions of 25°C–27°C during the day, 20°C at night, and 85% air humidity, with normal day and night lighting conditions. Inoculation was performed on plants by cutting the youngest leaves with a pair of scissors dipped in an inoculum with a bacterial concentration of 109 cfu/ml. Both parents of the 07240 population, ‘Idared’ and MAL0004, as well as Mf821, were included as controls. Shoot length and lesion length (in cm) of the replicates for each genotype were measured 28 days postinoculation (dpi). The percent lesion length (PLL) per shoot was calculated from the data, and the average PLL for each genotype was determined for further analysis.
Mapping analyses
We employed the molecular marker data of the 07240 individuals for LG12 previously reported (Emeriewen et al., 2017, 2021) for mapping analyses. The genetic map of LG12 of MAL0004 was recreated with 114 F1 individuals using JoinMap 4.0 (Van Ooijen, 2018). The phenotypic data of these same individuals for the Δeop1 strain generated in this study and data for two other strains, Ea222 and Ea3049 (Emeriewen et al., 2017), as well as their LG12 marker data, were used for QTL analysis via Kruskal–Wallis analysis and interval mapping on MapQTL software 5 (Van Ooijen, 2004).
Results
Artificial shoot inoculations
We observed and recorded an average lesion length of 1.7% for MAL0004, the resistant parent, based on five replicates, which showed no disease symptoms and one replicate with disease symptoms of 10.4%. ‘Idared’, the susceptible parent, on the other hand, showed 90.9% average disease, with most replicates showing 100% lesions. The other control genotype, Mf821, showed 23.2% average disease. Figure 1A shows the phenotype distribution of 102 progeny of the 07240 population that were phenotyped with Δeop1. Of these individuals, only two displayed no disease symptoms, while the overall average PLL was 35.7. To compare the results of Δeop1 and two other strains (Ea222 and Ea3049) previously used to inoculate the progeny, we used 77 progeny that possessed phenotypic data for the three strains. The direct comparison showed that only one individual showed no symptom to Δeop1, whereas for Ea222 and Ea3049 (data from Emeriewen et al., 2017), 11 and seven individuals, respectively, showed no symptoms (Figure 1B). For these 77 individuals, the average PLL with Δeop1 was 35.9, whereas it was 32.0 and 69.9 for Ea222 and Ea3049, respectively (Figure 1B).
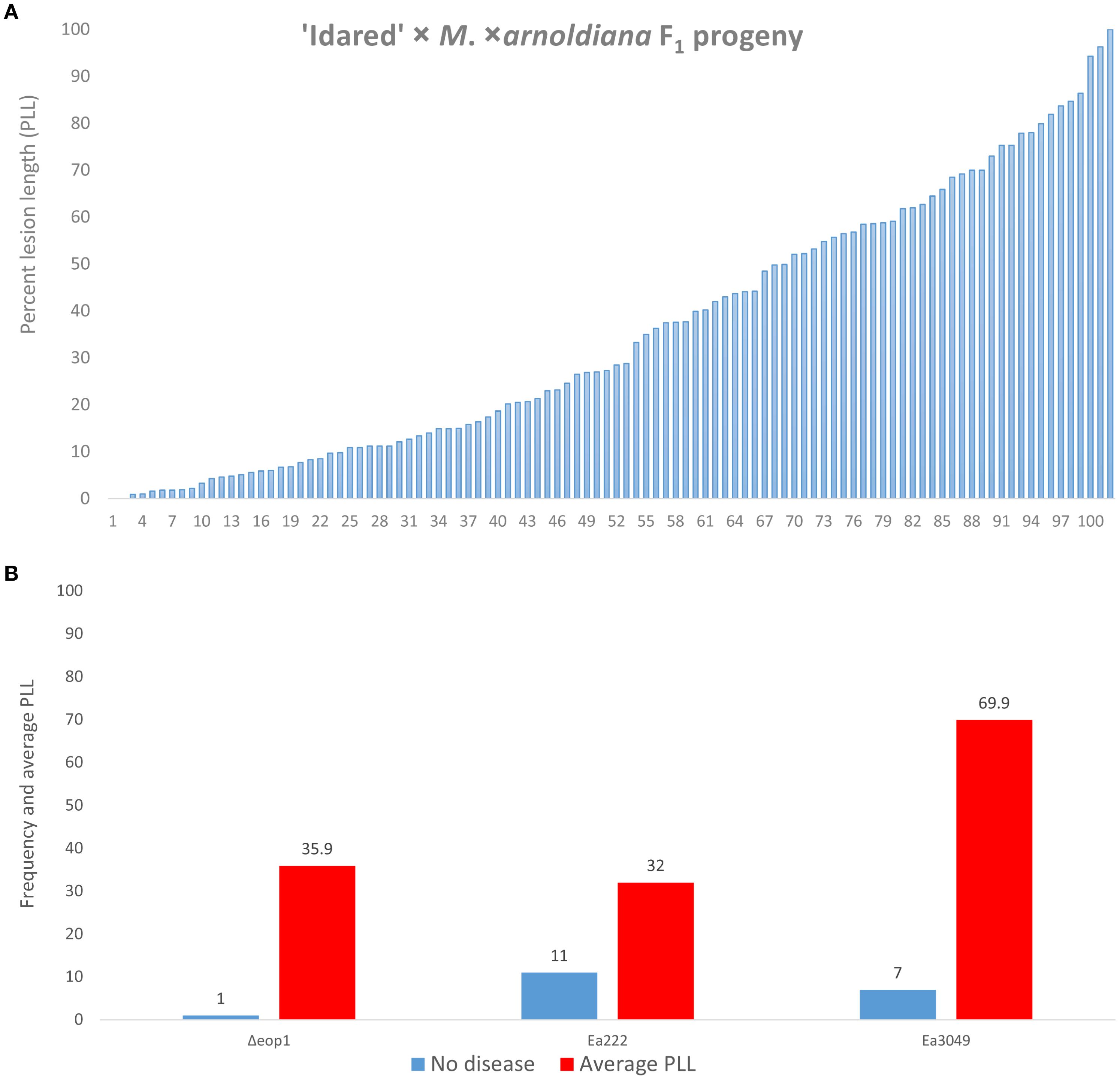
Figure 1. Distribution of resistance/susceptibility of 102 ‘Idared’ × M. xarnoldiana progeny inoculated with E. amylovora Δeop1 (A). A direct comparison of 77 progeny with phenotypic data for Δeop1 (current study) and Ea222 and Ea3049 (data from Emeriewen et al., 2017) showing the number of individuals exhibiting complete resistant phenotype (no disease) and the average percent lesion for these individuals (B).
Mapping analyses
The 14 markers that map to LG12 of MAL0004 (Emeriewen et al., 2017), along with two additional markers used for fine mapping the FB_Mar12 region (Emeriewen et al., 2021), totaled 16 markers for recreating the LG12 genetic map of MAL0004. The recreated genetic map measured 36.74 cM, compared to 34.29 cM reported by Emeriewen et al. (2017), attributed to the inclusion of the two additional markers and different progeny sizes used in the analyses. However, the order of the markers remained the same.
The genotypic data from the 114 individuals used to generate the map, along with the phenotypic data for 102 of these individuals that included Δeop1 data, as well as data for Ea222 and Ea3049 (Emeriewen et al., 2017), were used for marker-phenotype analyses and QTL mapping. The Kruskal–Wallis analysis (Table 1) revealed a significant correlation between the LG12 markers and resistance to Ea222 and Ea3049, but not to Δeop1. The strongest significance (K-value = 57.2) for Ea222 was observed for markers flanking and co-segregating with FB_Mar12, specifically CHFBE01, CHFBE02, and CHFBE08 (Emeriewen et al., 2021). The strength of the significance of these markers weakened but remained relevant with Ea3049 (K-value = 31.9) and completely disappeared for Δeop1 (K-value = 1.2).
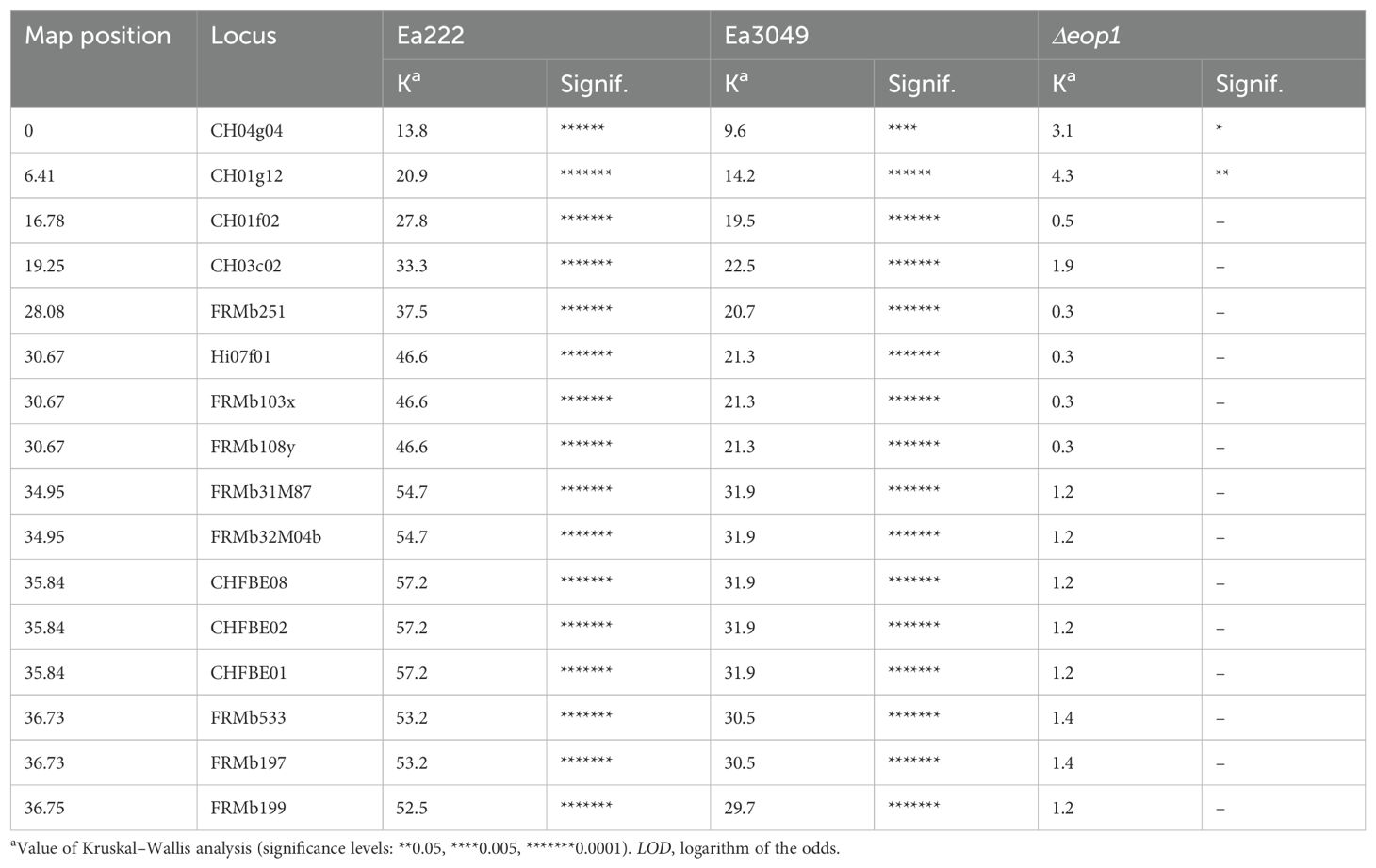
Table 1. Kruskal–Wallis analysis of linkage group 12 of Malus xarnoldiana using two wild-type Erwinia amylovora strains and the Δeop1 mutant.
QTL analysis via interval mapping (Figure 2) showed that the major QTL on LG12 of MAL0004 was detected using data from Ea222 and Ea3049, but not with Δeop1. The markers that significantly correlated with resistance to Ea222 and Ea3049 showed a LOD score of > 16 for both strains, while they showed almost zero for Δeop1. All markers on LG12, including those within the FB_Mar12 region, had < 1 LOD score (Figure 2), confirming the complete breakdown of the QTL and associated genes in this region by Δeop1.
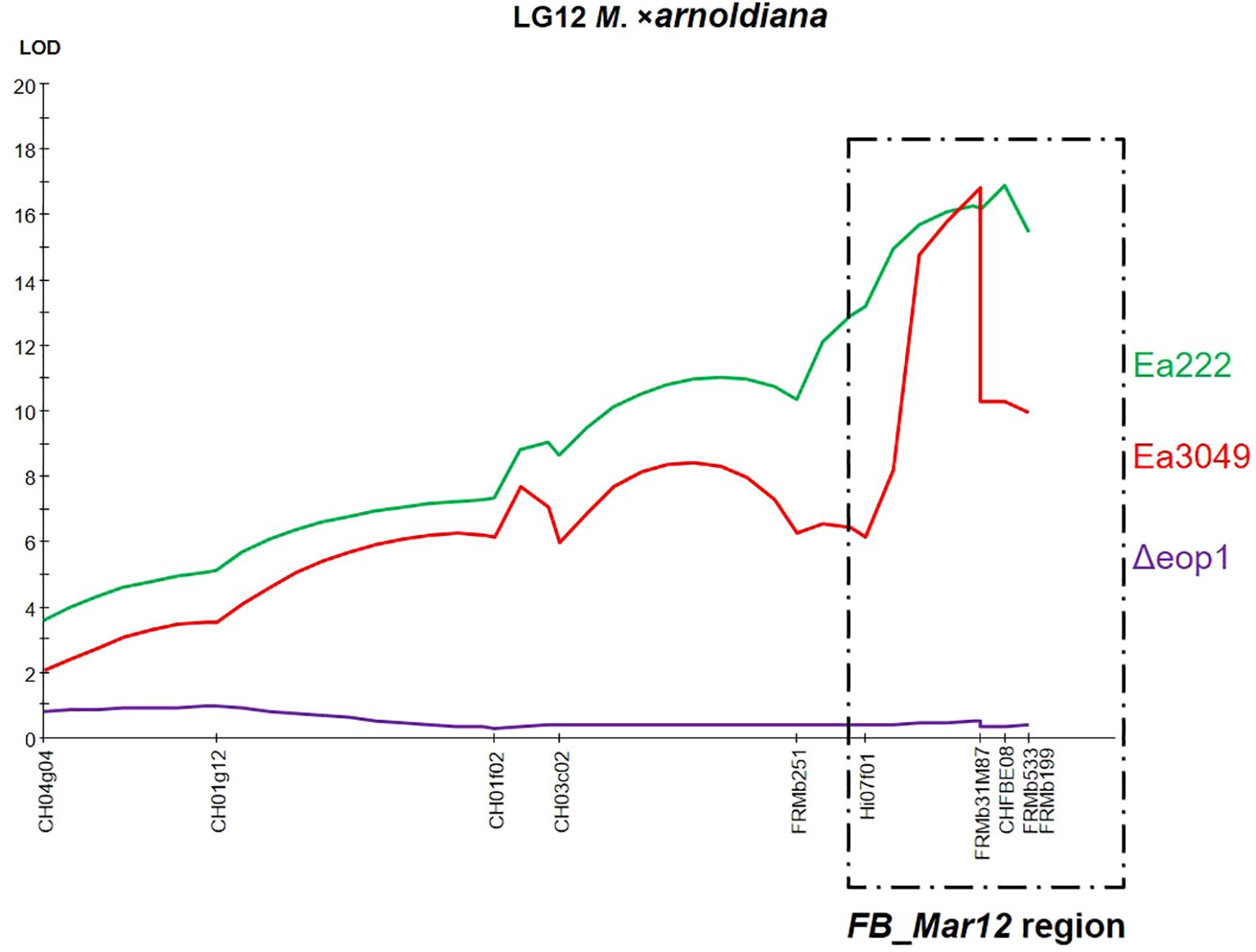
Figure 2. LOD score plot for the necrosis trait along LG12 of M. xarnoldiana, based on phenotypic data from the F1 progeny of ‘Idared’ × M. xarnoldiana MAL0004 inoculated with Ea222 and Ea3049 (Emeriewen et al., 2017), and Δeop1 (current study), as determined by interval mapping. The FB_Mar12 region is highlighted with a dotted box at the distal end of the linkage group.
Discussion
Inoculation of host plants with mutant strains of E. amylovora where T3SS effector genes are disrupted is an effective approach to determine several putative interactions between the pathogen and its hosts (Zhao et al., 2006; Vogt et al., 2013; Wöhner et al., 2014, 2018). The deletion of the entire T3SS in a wild-type strain of E. amylovora (ΔT3SS) proved its loss of function, as virulence was abolished in this mutant, resulting in no disease in known susceptible apple hosts and thereby confirming the T3SS as essential for E. amylovora pathogenicity (Wöhner et al., 2018). Similarly, the deletion of E. amylovora effector genes provides evidence of gene-for-gene relationships (Flor, 1971), as it reveals dominant avirulence genes in the pathogen that correspond to dominant resistance genes in the host. The absence of these avirulence genes is required for a compatible relationship between pathogen and host. For instance, the E. amylovora mutant strain ZYRKD3-1, with a disrupted avrRpt2EA effector gene, resulted in an average disease necrosis of 52.4% on Mr5, whereas a wild-type strain caused zero necrosis on Mr5 (Vogt et al., 2013). This breakdown of Mr5 resistance confirms a gene-for-gene relationship within the Mr5–E. amylovora pathosystem, with avrRpt2EA acting as the avirulence gene. Broggini et al. (2014) further validated this relationship by showing that transgenic ‘Gala’ plants overexpressing FB_MR5—the fire blight resistance gene of Mr5—were resistant to wild-type strains Ea222 and Ea1189 (with average necrosis between 0% and 4%), yet became susceptible to the avrRpt2EA effector mutant ZYRKD3-1 (average necrosis between 26.9% and 49.9%).
In a previous study, Wöhner et al. (2018) showed that the wild-type strain Ea1189 caused no disease on MAL0004 and Mf821, with both genotypes showing 0 and 0.3% average disease; however, an Δeop1 mutant of this same strain caused disease (35.1%) on Mf821 but not on MAL0004 (0.1%). Mf821 and MAL0004 are both donors of fire blight resistance QTLs located at the distal end of LG12 (Durel et al., 2009; Emeriewen et al., 2017). In the current study, we inoculated the 07240 F1 progeny of ‘Idared’ × MAL0004 with Δeop1 including both parents and Mf821 as controls. The results obtained confirmed the results of Wöhner et al. (2018) as Δeop1 caused disease on Mf821 but not on MAL0004. This confirms that the mechanism of fire blight resistance in both wild genotypes is different. Although MAL0004 was very resistant to Δeop1, only two individuals of the entire F1 progeny showed no disease symptoms (strong resistant phenotype) in comparison to inoculation results from this same F1 progeny with Ea222 and Ea3049, where 11 and seven individuals, respectively, showed no disease symptoms (Emeriewen et al., 2017).
Interestingly, the Δeop1 strain resulted in the complete breakdown of the fire blight resistance QTL of MAL0004 on LG12, which was previously identified by Emeriewen et al. (2017) following artificial shoot inoculation of 116 F1 progeny with E. amylovora strains Ea222 and Ea3049. The QTL region was delimited from a 5.6 cM region to 0.67 cM in fine mapping studies using 892 progeny, leading to the identification of candidate genes within this locus, designated as FB_Mar12 (Emeriewen et al., 2021). Using data from Emeriewen et al. (2017), we detected the locus on LG12 with Ea222 and Ea3049 in 114 progeny in the current study. However, the complete breakdown of this locus by Δeop1 strongly indicates a gene-for-gene interaction between the Eop1 effector of E. amylovora and the resistance gene underlying the FB_Mar12 locus. In addition, the fact that Δeop1 does not overcome the resistance of MAL0004 itself, yet completely breaks down FB_Mar12, suggests that other resistance factors may play key and/or contributory roles in the resistance of MAL0004. This hypothesis is supported by the findings of Durel et al. (2009), who found a minor QTL on LG15 in addition to the major QTL on LG12 in ‘Evereste’. A genome-wide saturated genetic map of MAL0004 is required to further elucidate its fire blight resistance.
The putative gene-for-gene interaction identified in this study differs from that described between Mr5 and the avrRpt2EA effector gene of E. amylovora in that the resistance donor, Mr5, was also overcome, along with the responsible resistance gene (Vogt et al., 2013; Broggini et al., 2014). The situation with Mr5 provides a strong precedent, suggesting that since the resistance of Mf821 is broken down by Δeop1, as initially shown by Wöhner et al. (2018) and supported in the current study, it is highly probable that the responsible resistance gene locus on LG12 (Durel et al., 2009) could also be broken down. A similar situation may apply to the ornamental cultivar ‘Evereste’, whose resistance was also overcome by Δeop1 (Wöhner et al., 2018). This suggests that the resistance QTLs described in all three wild genotypes are overcome by Δeop1, raising the question of whether the QTLs on LG12 are the same or allelic. All three QTLs are located within the same region on LG12, below the SSR marker Hi07f01 (Figure 2), which is a common marker shared in their respective genetic maps (Durel et al., 2009; Emeriewen et al., 2017). In addition, FB_Mar12 co-segregates with CHFBE02, which also co-segregates with the ‘Evereste’ gene locus, FB_E, and is closely associated with CHFBE01 and CHFBE08 (Parravicini et al., 2011; Emeriewen et al., 2021). Mf821 possesses the same allele sizes as the alleles of the markers linked to resistance (data not shown). Therefore, it is plausible that MAL0004, ‘Evereste’, and Mf821 share the same resistance allele on LG12. However, our results clearly indicate that there is another resistance factor expressed in MAL0004 but not in Mf821 or Evereste, which makes MAL0004 itself resistant to Δeop1.
In summary, while we present strong evidence of a gene-for-gene interaction between the E. amylovora effector gene eop1 and FB_Mar12 on LG12, several missing links remain in fully elucidating the resistance mechanisms of M. xarnoldiana MAL0004 and the other donors of resistance at the distal end of LG12. Several open and interesting research questions remain concerning E. amylovora and host interactions (Rezzonico et al., 2024), not least the implications for the management of the disease and host resistance breeding (Zeng et al., 2024).
Data availability statement
The original contributions presented in the study are included in the article/supplementary material. Further inquiries can be directed to the corresponding author.
Author contributions
OFE: Conceptualization, Data curation, Formal analysis, Investigation, Methodology, Writing – original draft, Writing – review & editing. HZ: Formal analysis, Validation, Writing – review & editing. TWW: Conceptualization, Formal analysis, Writing – review & editing. HF: Conceptualization, Resources, Writing – review & editing. AP: Conceptualization, Data curation, Investigation, Resources, Validation, Writing – review & editing.
Funding
The author(s) declare that no financial support was received for the research, authorship, and/or publication of this article.
Acknowledgments
We are grateful to the orchard and greenhouse staff of JKI at the Dresden-Pillnitz and Quedlinburg locations for their technical assistance.
Conflict of interest
The authors declare that the research was conducted in the absence of any commercial or financial relationships that could be construed as a potential conflict of interest.
Publisher’s note
All claims expressed in this article are solely those of the authors and do not necessarily represent those of their affiliated organizations, or those of the publisher, the editors and the reviewers. Any product that may be evaluated in this article, or claim that may be made by its manufacturer, is not guaranteed or endorsed by the publisher.
References
Broggini, G. A., Wöhner, T., Fahrentrapp, J., Kost, T. D., Flachowsky, H., Peil, A., et al. (2014). Engineering fire blight resistance into the apple cultivar ‘Gala’ using the FB_MR5 CC-NBS-LRR resistance gene of Malus xrobusta 5. Plant Biotechnol. J. 12, 728–733. doi: 10.1111/pbi.12177
Durel, C. E., Denance, C., Brisset, M. N. (2009). Two distinct major QTL for resistance to fire blight co-localize on linkage group 12 in apple genotypes ‘Evereste’ and Malus floribunda clone 821. Genome 52, 139–147. doi: 10.1139/G08-111
Emeriewen, O., Richter, K., Killian, A., Zini, E., Hanke, M.-V., Malnoy, M., et al. (2014). Identification of a major quantitative trait locus for resistance to fire blight in the wild apple species Malus fusca. Mol. Breed. 34, 407–419. doi: 10.1007/s11032-014-0043-1
Emeriewen, O. F., Peil, A., Richter, K., Zini, E., Hanke, M.-V., Malnoy, M. (2017). Fire blight resistance of Malus ×arnoldiana is controlled by a quantitative trait locus located at the distal end of linkage group 12. Eur. J. Plant Pathol. 148, 1011–1018. doi: 10.1007/s10658-017-1152-6
Emeriewen, O. F., Piazza, S., Cestaro, A., Flachowsky, H., Malnoy, M., Peil, A. (2022). Identification of additional fire blight resistance candidate genes following MinION Oxford Nanopore sequencing and assembly of BAC clone spanning the Malus fusca resistance locus. J. Plant Pathol. 104, 1509–1516. doi: 10.1007/s42161-022-01223-x
Emeriewen, O. F., Richter, K., Flachowsky, H., Malnoy, M., Peil, A. (2021). Genetic analysis and fine mapping of the fire blight resistance locus of Malus ×arnoldiana on linkage group 12 reveal first candidate genes. Front. Plant Sci. 12, 667133. doi: 10.3389/fpls.2021.667133
Emeriewen, O. F., Richter, K., Piazza, S., Micheletti, D., Broggini, G. A. L., Berner, T., et al. (2018). Towards map-based cloning of FB_Mfu10: Identification of a receptor-like kinase candidate gene underlying the Malus fusca fire blight resistance locus on linkage group 10. Mol. Breed. 38, 106. doi: 10.1007/s11032-018-0863-5
Emeriewen, O. F., Wöhner, T., Flachowsky, H., Peil, A. (2019). Malus hosts–Erwinia amylovora interactions: strain pathogenicity and resistance mechanisms. Front. Plant Sci 10. doi: 10.3389/fpls.2019.00551
Fahrentrapp, J., Broggini, G. A. L., Kellerhals, M., Peil, A., Richter, K., Zini, E., et al. (2013). A candidate gene for fire blight resistance in Malus × robusta 5 is coding for a CC-NBS-LRR. Tree Genet. Genomes 9, 237–251. doi: 10.1007/s11295-012-0550-3
Flor, H. H. (1971). Current status of the gene-for-gene concept. Annu. Rev. Phytopathol. 9, 275–296. doi: 10.1146/annurev.py.09.090171.001423
Hasler, T., Schaerer, H. J., Holliger, E., Vogelsanger, J., Vignutelli, A., Schoch, B. (2002). Fire blight situation in Switzerland. Acta Hortic. 590, 73–79. doi: 10.17660/ActaHortic.2002.590.8
McNally, R. R., Zhao, Y., Sundin, G. W. (2015). Towards understanding fire blight: virulence mechanisms and their regulation in Erwinia amylovora. Bacteria-plant interactions: advanced Res. Future Trends, 61–82. doi: 10.21775/9781908230584
Norelli, J. L., Jones, A. L., Aldwinkle, H. S. (2003). Fire blight management in the twenty-first century – using new technologies that enhance host resistance in apple. Plant Dis. 87, 756–765. doi: 10.1094/PDIS.2003.87.7.756
Oh, C.-S., Beer, S. V. (2005). Molecular genetics of Erwinia amylovora involves in the development of fire blight. FEMS Microbio. Lett. 253, 185–192. doi: 10.1016/j.femsle.2005.09.051
Oh, C. S., Kim, J. F., Beer, S. V. (2005). The Hrp pathogenicity island of Erwinia amylovora and identification of three novel genes required for systemic infection. Mol. Plant Pathol. 6, 125–138. doi: 10.1111/j.1364-3703.2005.00269.x
Parravicini, G., Gessler, C., Denance, C., Lasserre-Zuber, P., Vergne, E., Brisset, M. N., et al. (2011). Identification of serine/threonine kinase and nucleotide-binding-site-leucine-rich repeat (NBS-LRR) genes in the fire blight resistance quantitative trait locus of apple cultivar ‘Evereste’. Mol. Plant Pathol. 12, 493–505. doi: 10.1111/j.1364-3703.2010.00690.x
Peil, A., Emeriewen, O. F., Khan, A., Kostick, S., Malnoy, M. (2021). Status of fire blight resistance breeding in Malus. J. Plant Pathol. 103, 3–12. doi: 10.1007/s42161-020-00581-8
Peil, A., Flachowsky, H., Hanke, M.-V., Richter, K., Rode, J. (2011). Inoculation of Malus ×robusta 5 progeny with a strain breaking resistance to fire blight reveals a minor QTL on LG5. Acta Hortic. 896, 357–362. doi: 10.17660/ActaHortic.2011.896.49
Peil, A., Garcia-Libreros, T., Richter, K., Trognitz, F. C., Trognitz, B., Hanke, M. V., et al. (2007). Strong evidence for a fire blight resistance gene of Malus robusta located on linkage group 3. Plant Breed. 126, 270–475. doi: 10.1111/j.1439-0523.2007.01408.x
Piqué, N., Miñana-Galbis, D., Merino, S., Tomás, J. M. (2015). Virulence factors of Erwinia amylovora: a review. Int. J. Mol. Sci. 16, 12836–12854. doi: 10.3390/ijms160612836
Rezzonico, F., Emeriewen, O. F., Zeng, Q., Peil, A., Smits, T. H., Sundin, G. W. (2024). Burning questions for fire blight research: I. Genomics and evolution of Erwinia amylovora and analyses of host-pathogen interactions. J. Plant Pathol. 106, 1–14. doi: 10.1007/s42161-023-01581-0
Van Ooijen, J. W. (2004). MapQTL® 5 Software for the mapping of quantitative trait loci in experimental populations (Wageningen, the Netherlands: Plant Research International).
Van Ooijen, J. W. (2018). JoinMap ® 5, software for the calculation of genetic linkage maps in experimental populations of diploid species (Wageningen, Netherlands: Kyazma B.V).
Vogt, I., Wöhner, T., Richter, K., Flachowsky, H., Sundin, G. W., Wensing, A., et al. (2013). Gene-for-gene relationship in the host-pathogen system Malus xrobusta 5-Erwinia amylovora. New Phytol. 197, 1262–1275. doi: 10.1111/nph.12094
Winslow, C. E. A., Broadhurst, J., Buchanan, R. E., Krumwiede, C., Rogers, L. A., Smith, G. H. (1920). The families and genera of the bacteria. Final report of the Committee of the Society of American Bacteriologists on the characterization and classification of bacterial types. J. Bacteriol. 5, 191–229. doi: 10.1128/jb.5.3.191-229.1920
Wöhner, T. W., Flachowsky, H., Richter, K., Garcia-Libreros, T., Trognitz, F., Hanke, M. V., et al. (2014). QTL mapping of fire blight resistance in Malus× robusta 5 after inoculation with different strains of Erwinia amylovora. Mol. Breed. 34, 217–230. doi: 10.1007/s11032-014-0031-5
Wöhner, T., Richter, K., Sundin, G. W., Zhao, Y., Stockwell, V. O., Sellmann, J., et al. (2018). Inoculation of Malus genotypes with a set of Erwinia amylovora strains indicates a gene-for-gene relationship between the effector gene Eop1 and both Malus floribunda 821 and Malus ‘Evereste’. Plant Pathol. 67, 938–947 doi: 10.1111/ppa.12784
Yuan, X., Hulin, M. T., Sundin, G. W. (2021). Effectors, chaperones, and harpins of the Type III secretion system in the fire blight pathogen Erwinia amylovora: a review. J. Plant Pathol. 103, 25–39. doi: 10.1007/s42161-020-00623-1
Zeng, Q., Emeriewen, O. F., Rezzonico, F., Sundin, G. W., Peil, A. (2024). Burning questions for fire blight research: II. Critical next steps in disease management and in host resistance breeding of apple and pear. J. Plant Pathol 106, 811–822. doi: 10.1007/s42161-024-01678-0
Zhao, Y. (2014). “Genomics of Erwinia amylovora and related Erwinia species associated with pome fruit trees,” in Genomics of plant-associated bacteria (Springer Berlin Heidelberg, Berlin, Heidelberg), 1–36.
Keywords: apple wild species, fire blight, resistance QTL, resistance-breakdown, Malus hosts
Citation: Emeriewen OF, Zetzsche H, Wöhner TW, Flachowsky H and Peil A (2024) A putative gene-for-gene relationship between the Erwinia amylovora effector gene eop1 and the FB_Mar12 resistance locus of Malus ×arnoldiana accession MAL0004. Front. Plant Sci. 15:1472536. doi: 10.3389/fpls.2024.1472536
Received: 29 July 2024; Accepted: 30 October 2024;
Published: 04 December 2024.
Edited by:
Choong-Min Ryu, Korea Research Institute of Bioscience and Biotechnology (KRIBB), Republic of KoreaReviewed by:
Erik H. A. Rikkerink, The New Zealand Institute for Plant and Food Research Ltd, New ZealandHuamin Chen, Chinese Academy of Agricultural Sciences, China
Copyright © 2024 Emeriewen, Zetzsche, Wöhner, Flachowsky and Peil. This is an open-access article distributed under the terms of the Creative Commons Attribution License (CC BY). The use, distribution or reproduction in other forums is permitted, provided the original author(s) and the copyright owner(s) are credited and that the original publication in this journal is cited, in accordance with accepted academic practice. No use, distribution or reproduction is permitted which does not comply with these terms.
*Correspondence: Ofere Francis Emeriewen, b2ZlcmUuZW1lcmlld2VuQGp1bGl1cy1rdWVobi5kZQ==