- 1Laboratory for Phytopathology, Small Grains Department, Institute of Field and Vegetable Crops, Novi Sad, Serbia
- 2John Innes Centre, Norwich Research Park, Norwich, United Kingdom
Wheat yellow (stripe) rust, caused by the fungus Puccinia striiformis f.sp. tritici (Pst), is a devastating disease of wheat worldwide. The success of Pst is largely due to the pathogens ability to rapidly overcome host resistance, generating new races that are easily dispersed between territories through wind-borne transmission of Pst urediniospores. Thus, first signs of entry of new Pst races into a region is usually captured by changes in disease severity. To examine any alterations of winter wheat variety response to Pst infection in Serbia, we analyzed yield and Pst disease severity in field trials conducted in 2014, 2021, and 2023. We specifically focused on analyzing Pst disease severity at growth stages related to yield. Associations between qualitative variables (variety, year) and quantitative variables (yield in untreated plots, yield loss, and disease index (DI) of Pst infection) were analyzed using Principal Component Analysis with mixed data. A General Linear Model was used to investigate the most influential factors on yield, yield loss, and Pst infection. The results indicated that yellow rust disease severity increased over the past decade, suggesting a potential recent change in the Pst population in Serbia. Comparative population genetic analysis of Pst samples from the 2023 wheat season and those collected in Serbia in 2014 confirmed a potential change in the Pst population. In addition, we found that yield losses across wheat varieties varied independently of Pst infection levels, indicating that wheat varieties differ in their ability to overcome damage caused by high levels of Pst infection. Given that the level of pathogen pressure triggering susceptibility reactions is cultivar-specific, our study highlights the need for a deeper focus on the mechanisms underlying these differences. Expanding our understanding of the interactions between pathogens, plant defense responses, and the ability of cultivars to mitigate yield losses will better equip us to predict and prevent potential yield losses in commercial wheat varieties due to yellow rust in the future.
1 Introduction
Puccinia striiformis f.sp. tritici (Pst) is the causal agent of wheat yellow (stripe) rust, that is one of the most devastating diseases of wheat worldwide and is largely dispersed by air currents that transmit asexual urediniospores over long distances (Zadoks, 1961; Hovmøller et al., 2011). Wheat yellow rust is not transmitted through seed although under high infection intensities Pst urediniospores can be observed on host plant ears (Chen et al., 2014). Changing climatic conditions drive the continuous breeding of new varieties for increased fitness in response to these rapidly shifting environments. However, new Pst races also regularly emerge that can overcome resistance in introduced wheat varieties making the control of yellow rust challenging. Furthermore, recent shifts in the geographic distribution of Pst, particularly in North America, have also been linked to temperature-specific adaptation (Lyon and Broders, 2017), reflecting the high adaptability of Pst to environmental pressures. Consequently, changes in pathogens populations affected by changes in climatic conditions, selection pressure of resistant varieties and applied pesticides (Ash and Brown, 1990; Pritsch et al., 2000; Ahmad et al., 2010; Ma et al., 2013; Xi et al., 2015; El Jarroudi et al., 2017; Carmona et al., 2019; Chen, 2020) makes yellow rust control challenging (Chen, 2005; Ali et al., 2014; Hovmøller et al., 2016; Bueno-Sancho et al., 2017).
The potential for pathogens to better adapt to changing environmental conditions are also influenced by the type of reproduction, giving high adaptation abilities to pathogens with sexual reproduction stages in their life cycles. Pst is heteroecious, needing two hosts to complete its life cycle; it reproduces asexually on wheat and sexually on Berberis spp (Jin et al., 2010). Pst diversity is centered in the Himalayan region, where sexual reproduction and thus significant genetic variation occurs, in contrast to the predominant clonal population structure found in Europe, America, and Australia (Hovmøller et al., 2011; Ali et al., 2014). While mutation is known to serve as a major source of genetic variation, generating new alleles and genotypes, sexual, parasexual, and somatic recombination also influence genotype diversity (Hovmøller et al., 2011).
Before 2011, the population of Pst in Europe was mainly clonal, relying on mutations with minimal influence from sexual recombination (Hovmøller et al., 2002). Consequently, new virulent Pst strains arose due to stepwise mutation within these clonal populations (Linde et al., 2002; de Vallavieille-Pope et al., 2012). However, this situation abruptly changed in 2011, with the discovery of two new Pst races, termed ‘Warrior’ and ‘Kranich’, that were detected across numerous European countries and had greater genetic variability than previous clonal populations (Hovmøller et al., 2016). These new exotic Pst lineages then rapidly replaced the previously known Pst population in Europe (Hubbard et al., 2015) and harbored virulence against varieties that were previously resistant to the prevailing Pst races, making rust control much more challenging (Sørensen et al., 2014). The Warrior race has also spread to North Africa since its appearance in Europe, challenging wheat production (Ali et al., 2014; Hovmøller et al., 2016; Shahin, 2020). These findings underscore the reality that, despite ongoing efforts to develop wheat varieties resistant to existing Pst populations, the emergence of new Pst races can trigger outbreaks of epidemic proportions, even on a continental scale (Brown and Hovmøller, 2002).
The first observations of yellow rust in Serbia occurred at Rimski Šančevi in 1997 within a genetic collection comprising 630 genotypes of wheat and wild relatives (Jevtić et al., 1997). The first warning of its heightened threat was issued in 2007 as part of the ADAGIO Project (Adaptation of Agriculture in European Regions at Environmental Risk Under Climate Change) (Jevtić et al., 2009; Mihailovic et al., 2009; Jevtić and Župunski, 2023). This increase in yellow rust infections in 2007 was associated with increased drought and heat likely due to climate change and coincided with expanded geographic distribution of Pst. However, until 2014, the related wheat leaf rust pathogen, Puccinia triticina, remained the predominant wheat rust species in Serbia. Yet, during the 2013/2014 production season, winter temperatures exceeded average temperatures since 1964 leading to yellow rust predominance over leaf rust and jeopardizing wheat production (Jevtić et al., 2017, 2020). The outbreak of yellow rust in Serbia in 2014 was attributed to influx of the Pst Warrior race (Jevtić et al., 2017, 2020), with disease indices (DI) ranging from 40% to 60% (Jevtić et al., 2020).
The studies primarily focus on monitoring shifts in the yellow rust population and its effects on wheat production, but the number of model genotypes used per study to assess the impact of yellow rust on agronomic traits is usually limited to a few well-known cultivars categorized as either susceptible or resistant. However, the variation in responses among different susceptible cultivars to yellow rust infection is often overlooked, as is the combined effect of biotic and abiotic stressors on disease occurrence (White et al., 2011; Juroszek and von Tiedemann, 2013). The lack of information on factors that affect diversity of reactions of susceptible varieties to yellow rust infection, and how they compensate for yield losses makes predictions of yellow rust occurrence more challenging and limits the effectiveness of methods aimed at preventing agronomic losses.
Considering the ability of Pst to rapidly overcome host plant resistance, and the complexity of signal cross-talk controlling plant responses to abiotic and biotic environmental factors, we set out to examine the yield response of winter wheat varieties to Pst infection. In particular, we decided to assess how different levels of Pst infection affect yield responses of commercial winter wheat varieties, and the potential for different host genotypes to compensate for yield losses after delayed fungicide application under high established Pst infection levels. Our results, indicate that the level of pathogen pressure that triggers susceptible reactions is cultivar-specific, with diversity in the ability for commercial winter wheat varieties to overcome damage caused by high levels of Pst infection. Furthermore, through population genetic analysis we also uncovered a potential recent change in the Pst population in Serbia that could have an impact on disease severity in the region.
2 Materials and methods
2.1 Wheat field trials
Field trials were conducted in 2014, 2021, and 2023 at Rimski šančevi (45°19′47.30″ N, 19°50′44.00″ E, at an altitude of 85.7 m), in Vojvodina, the northern province of Serbia to assess resistance/susceptibility of commercial winter wheat varieties to Pst. Trials were undertaken in a slightly carbonated loamy chernozem soil with reduced cultivation practices. A total of 17 and 89 commercial varieties in 2014 and 2023 were assessed, respectively. To track the yield potential of commercial varieties in the absence of Pst infection, data from 2021 were also considered in the study, since no yellow rust was reported in 2021 due to unfavorable climatic conditions.
Field trials were conducted using methodology recommended by CIMMYT (1979). Accordingly, each genotype was sown in one un-replicated 5 meter row or using smaller row lengths but with replicates so that the sum of all row lengths was equal to 5 meters. To enable assessment of yield in the presence and absence of yellow rust, varieties were sown in plots of 5 metre2 and divided into fungicide-untreated area (4 m2) and fungicide-treated area (1 m2). Fungicide treatments were applied at recommended dosage rates at growth stage BBCH 65. The active ingredients used were: 100 g/L (10.3% w/w) prothioconazole, 100 g/L (10.5% w/w) tebuconazole and 250 g/L (26.3% w/w) spiroxamine (2014) and 75 g/l benzovindiflupyr 150 g/l, prothioconazole (2023). Fungicides were applied using calibrated field crop sprayers with fan nozzles, at 300 kPa pressure, and 200 L of water per hectare. The mean sowing date for winter wheat was October 20 (optimal time of sowing), and the mean harvest date was June 30.
2.2 Disease severity assessments
Disease severity of obligate pathogens were made at the growth stage known to be highly related to yield (BBCH 71-73, kernel watery; early milk) (Wegulo et al., 2009). A modified Cobb’s scale was used for disease severity assessments (Peterson et al., 1948; Corazza and Islongo, 1987). Since yellow rust predominated over the rest of the obligate pathogens in 2014 and 2023, the disease indices (%) of yellow rust were calculated by taking into account disease incidence and average disease severity (Cao et al., 2014).
2.3 Wheat yield assessment
Wheat yield per cultivar was measured separately for each fungicide-treated and fungicide-untreated plot at 15% water content. Yield loss (%) was determined as the average reduction in yield in fungicide-untreated plots compared to the yield in fungicide-treated plots (Equation 1). Yield gain (%) was calculated by considering average yield in fungicide-untreated plots and average yield achievement in fungicide-treated plots (Equation 2).
Y1—Average grain yield in fungicide-treated plots.
Y2—Average grain yield in fungicide-untreated plots
2.4 Pst population genetic analysis
A total of 26 Pst-infected wheat samples were collected from the field trials in 2022-2023 and were subjected to RNA extraction using a Qiagen RNeasy Mini Kit (Qiagen, UK) (Hubbard et al., 2015; Boshoff et al., 2020) (Supplementary Table S1). Extracted total RNA were analyzed for quality and quantity using a Qubit fluorometer (Thermo Fisher Scientific, USA). Subsequently, cDNA libraries were prepared using an Illumina TruSeq RNA Sample Preparation Kit (Illumina, USA) and sequenced on an Illumina NovaSeq instrument by Azenta Life Sciences (USA). In addition, genomic or RNA-seq datasets for a total of 169 Pst isolates collected across 27 countries were obtained from public repositories (Hubbard et al., 2015; Boshoff et al., 2020) (Supplementary Table S1). Reads were aligned for each sample against the Pst reference genome (Pst isolate Pst104e; (Schwessinger et al., 2018) using the Burrows-Wheeler Alignment (BWA) tool, version 0.7.5 (Li and Durbin, 2009) for genomic reads, and the Star alignment tool, version 2.5 (Dobin et al., 2013) for RNA-seq reads. SAMtools version 0.1.19 (Li et al., 2009) was used to generate pileups and identify single nucleotide polymorphisms (SNPs). This information was utilized to create the consensus sequences for each gene per Pst isolate as described previously (Hubbard et al., 2015) Phylogenetic analysis was conducted using 4,682 gene models with coverage in at least 80% of the 195 Pst isolates using FastTree version 2.1 and default settings allowing inference of maximum-likelihood (Price et al., 2010).
2.5 Statistical analyses
Since principal component analysis (PCA) requires quantitative and multiple correspondence analysis (MCA) qualitative variables only, associations between qualitative variables (variety, year) and quantitative variables (yield in untreated plots, yield loss, and DI of yellow rust) were analyzed using PCA with mixed data (PCAmix). A general linear model (GLM) was used to investigate the most influencing factors on yield, yield loss, and Pst infection of commercial winter wheat varieties in 2014 and 2023. In GLM analysis, predictor variables for evaluating the influencing factors on yield in untreated plots and yield losses were year, variety, and their interaction, while Pst infection was considered as a covariate variable. The relationship between yield and yield losses with Pst infection in 2014 and 2023 was analyzed using Spearman’s coefficient of correlation. Tukey pairwise comparison was used to identify whether yield achievements in treated and untreated plots differed among growing seasons. Monthly averages of temperature, total rainfall, and humidity during the 2014, 2021, and 2023 growing seasons were recorded for the experimental site using the weather station of the Republic Hydrometeorological Institute of Serbia located at Rimski šančevi (RHMZ) (http://www.hidmet.gov.rs/). All statistical analysis was performed using Minitab 17 Statistical Software and XLSTAT in Microsoft Excel. The ‘ggplot2’ package in R software was used for the visualization of PCAmix analysis.
2.6 Data availability
Newly generated RNA-seq data was deposited in the European Nucleotide Archive (ENA) database under the accession number: PRJEB77002. Accession numbers for additional genomic and RNA-seq data used in the phylogenetic analysis is provided in Supplementary Table S1.
3 Results
3.1 Yellow rust severity increased over the past decade in Serbia
To assess any changes in yellow rust disease severity since 2014 in Serbia, we analyzed the average disease indexes (DI) in untreated plots in the 2014 and 2023 seasons. We found a significant increase in Pst infections, with an average severity of 31.2% for 17 cultivars analyzed in 2014 and 51.9% across the 89 cultivars analyzed in 2023 (p< 0.001). Although both 2014 and 2023 were favorable for Pst infection, we noted greater aggressiveness of Pst in untreated plots in 2023 (Figure 1A). Accordingly, in 2014, the majority of wheat genotypes (47.1%) were infected with a DI of 30%, and only 11.8% of genotypes were infected with yellow rust at 60% and above. Whereas in 2023, 50.5% of genotypes were infected with a DI of 60% and above (Figure 1A).
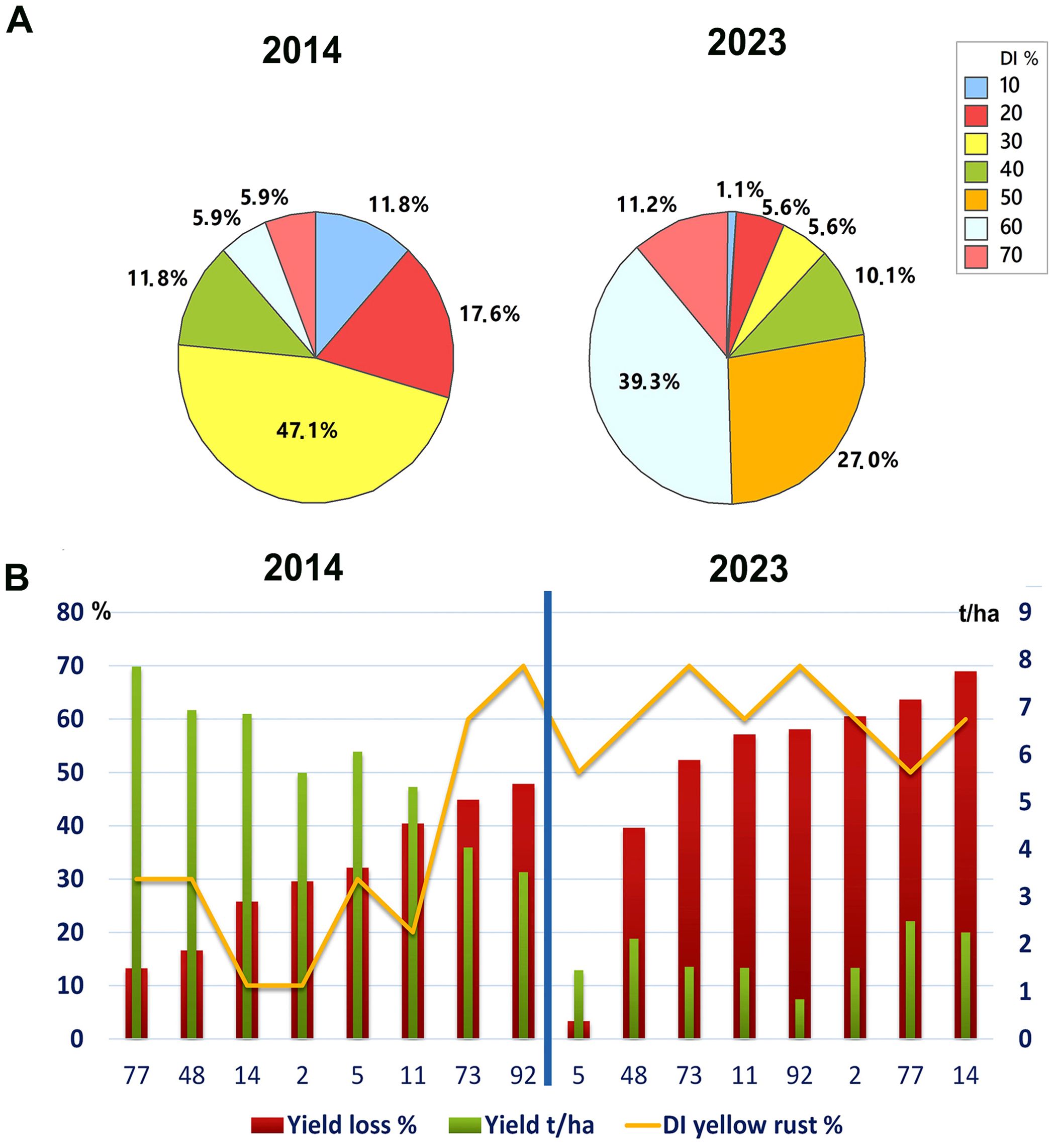
Figure 1. Yellow rust severity was much higher in the 2023 than the 2014 growth season. (A) The level of yellow rust disease incidence (DI) ranged from 10% to 70% in 2014 and 2023 across commercial wheat varieties grown in untreated plots at Rimski šančevi locality. A total of 17 and 89 commercial varieties in 2014 and 2023 were assessed for yellow rust severity at the growth stage known to be highly related to yield (BBCH 71-73, kernel watery; early milk). Disease indices (%) were calculated by taking into account yellow rust disease incidence and average disease severity. Significant increase in Pst infections, with an average severity of 31.2% for 17 cultivars analyzed in 2014 and 51.9% across the 89 cultivars analyzed in 2023 was detected. (B) Six out of eight cultivars, which had a moderate level of resistance to Pst in 2014, was highly susceptible in 2023, with reduced yield. This indicates that the aggressiveness of Pst races may have changed between 2014 and 2023. The yellow rust disease index, yield, and yield losses of 8 commercial winter wheat varieties were assessed in both 2014 and 2023 at the Rimski Šančevi locality. t/ha, tons per hectare.
Furthermore, comparison of 8 wheat genotypes cultivated in both 2014 and 2023 revealed that 6 out of 8 had lower Pst infection in 2014 than in 2023. Only 2 out of 8 of these common varieties had a consistent level of susceptibility in both years, with DI’s exceeding 60%. In addition, yields in untreated plots of these 2 varieties in 2014 reached 3.5 and 4 t/ha, but were below 1.5 t/ha in 2023 (Figure 1B). Tukey pairwise comparison showed that yields in untreated plots of the 8 varieties were significantly higher in 2014 (5.8 t/ha) than in 2023 (1.5 t/ha) (p< 0.001). This indicates that the aggressiveness of Pst races may have changed between 2014 and 2023, leading to an increase in DI in varieties previously shown to possess a moderate level of resistance to Pst.
3.2 Yield losses across wheat varieties varied independent of Pst infection levels
Across wheat varieties with similar levels of Pst infection we also noted a diversity in yield losses in both the 2014 and 2023 seasons. For instance, under a low level of yellow rust infection (20%), yields in untreated plots ranged from less than 3 t/ha (yield loss exceeding 50%) to over 4.5 t/ha (yield loss below 40%) (Figure 2A). Where high levels of Pst infection were recorded (exceeding 50%) we also recorded a variability in yield losses between genotypes, ranging from 3% to 80% in the same year (2023) (Figures 2A, B).
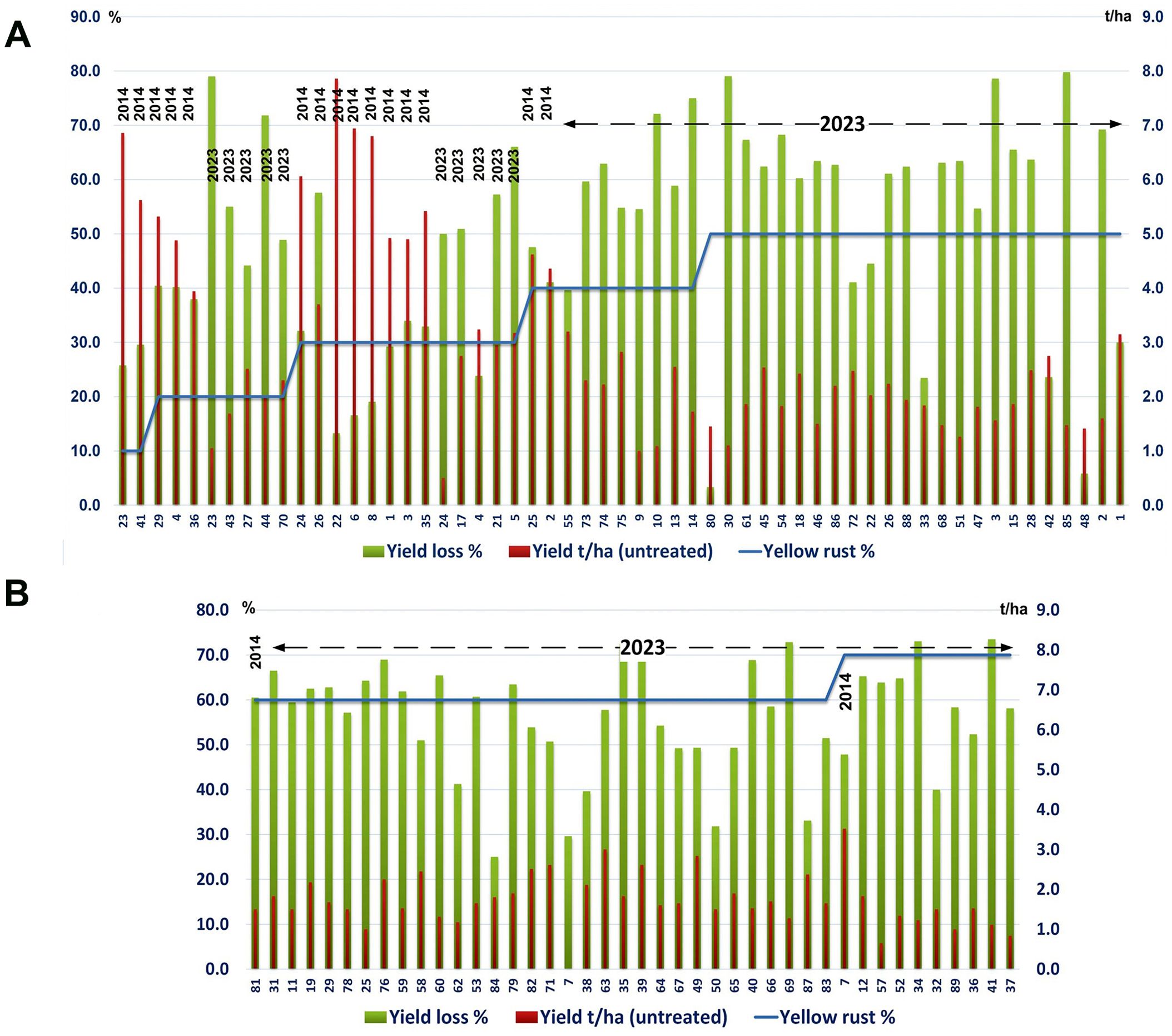
Figure 2. Yield losses across wheat varieties varied regardless of Pst infection levels. The yellow rust disease index, yield, and yield losses were assessed for 17 or 89 commercial winter wheat varieties in 2014 and 2023 respectively at the Rimski Šančevi locality. (A) Yield loss varied widely with varieties that had a low level of yellow rust infection (20%) in 2014, differing in yield loss in untreated plots from below 40% or well above 50%. Similarly, in untreated plots in 2023, where Pst infection levels exceeded 50%, yield losses also varied between genotypes, and ranged from 3% to 80%. (B) In untreated plots in 2023, where Pst infection levels exceeded 60%, yield losses also varied between genotypes, and ranged from 25% to 75%. Variability in yield losses under the same level of yellow rust infection indicate that for every variety, a specific level of yellow rust infection may need to be reached to trigger yield decrement. t/ha, tons per hectare.
To further examine any particular associations between yield in untreated plots, yield losses, Pst infection, and year, we conducted PCA mix analysis (Figure 3). Overall, data from the two years (2014 and 2023) were dispersed in the PCA analysis, with no notable association between yield and yield losses, indicating that they were affected by different factors. However, severity of Pst infection was positioned more closely to yield in untreated plots than to yield loss, indicating a potential relationship between yield loss and Pst infection. Further analysis of the Spearman coefficient of correlation indicated a significant low correlation between yield loss and Pst infection when considering both 2014 and 2023 (r = 0.227, p = 0.019). In contrast, Pst infection and yield in untreated plots had a negative moderate significant correlation (r = -0.510, p< 0.001). The squared cosine indicated that year, yield in untreated plots, and Pst infection severity had the highest degree of association with the first dimension (red color), while yield loss had a lower association with the first two dimensions (blue color) (Figure 3).
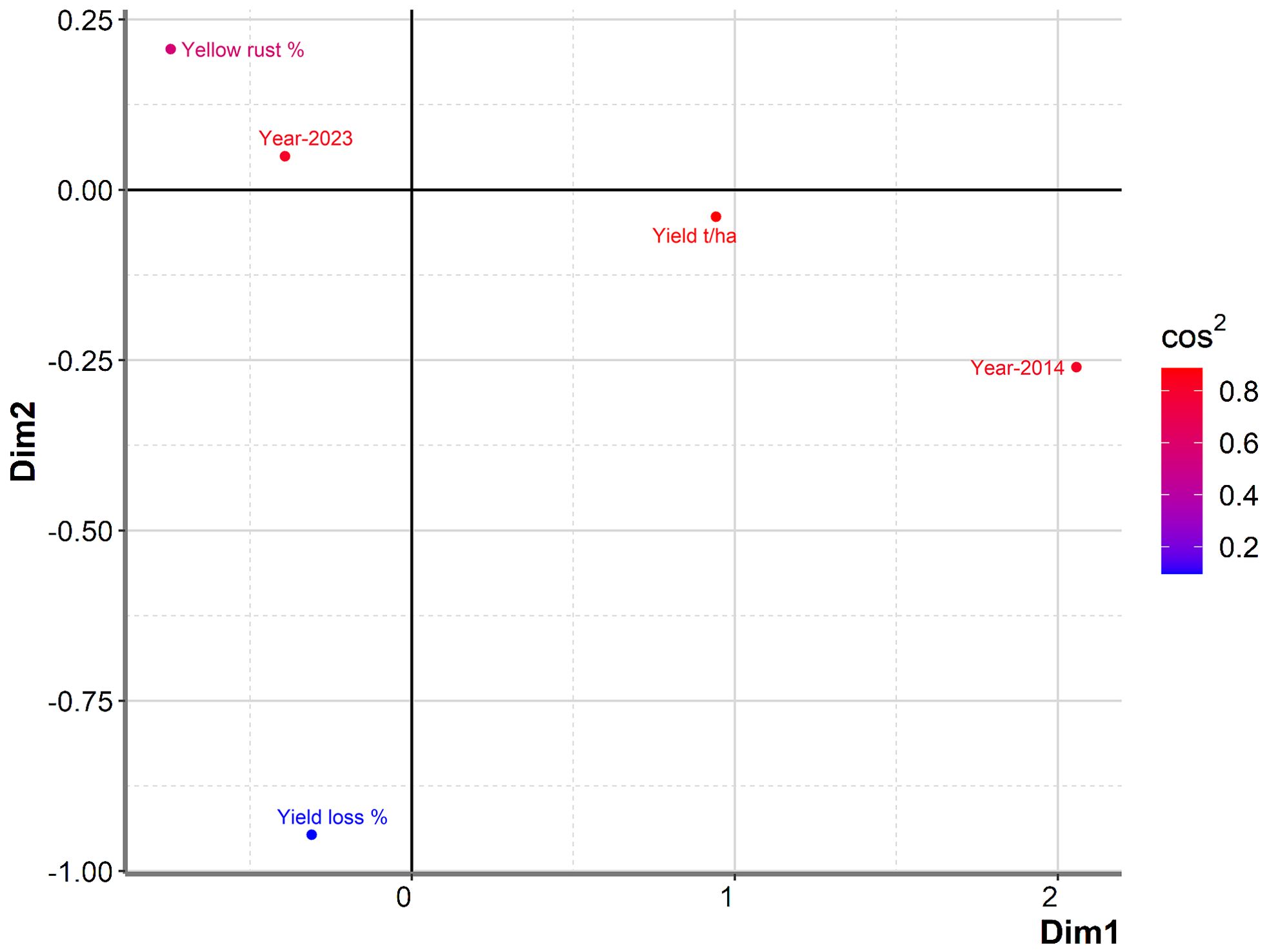
Figure 3. PCAmix analysis showed no notable association between yield and yield losses among commercial wheat varieties assessed in 2014 and 2023. A total of 17 or 89 commercial winter wheat varieties were assessed for yield in 2014 and 2023, respectively, at the Rimski Šančevi locality. Graphical representation of PCAmix analysis between yield, yield loss, disease index of yellow rust and years (2014 and 2023). Dim, dimension; t/ha, tons per hectare.
We also noted variation in yield loss for varieties with similar Pst disease severity within the same season. For instance, the yield loss of 6 varieties infected with yellow rust below 30% in 2014 ranged from 17% to 40%. Moreover, in 2014, 2 varieties (14 and 2) infected with a low level of yellow rust (DI = 10%) showed higher yield losses (26% and 30%) than 2 varieties (77 and 48) infected with a DI of 30%, with yield losses of 13% and 17% (Figure 1B). However, variability in yield losses under the same level of yellow rust infection was even more prominent in 2023 when 77.5% of genotypes were infected with a DI exceeding 50%, and when yield losses of those varieties ranged from 3% to 80% (Figures 2, 4). These results indicate that for every variety, a specific level of yellow rust infection may need to be reached to trigger yield decrement.
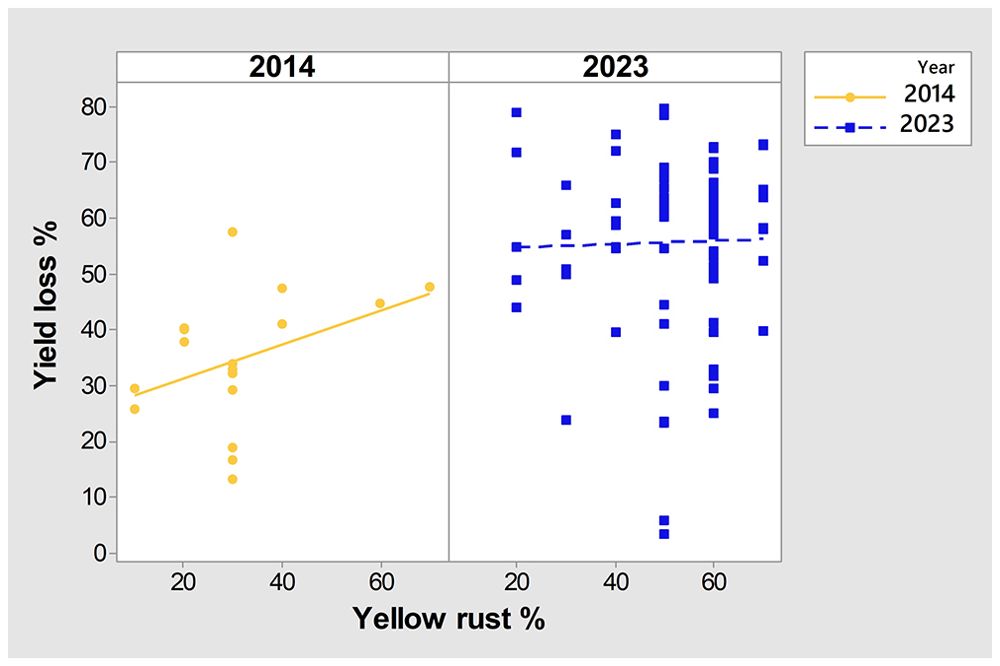
Figure 4. Variation in yield loss for varieties with similar Pst disease severity occurred within the same season. A total of 17 or 89 commercial winter wheat varieties were assessed for yield in the 2014 and 2023 seasons, respectively.
3.3 Fungicide application in 2023 failed to restore yield to levels achieved in the absence of Pst infections
We noted that average yield in fungicide-treated plots of the 8 cultivars tested in both 2014 and 2023 was higher in 2014 (8 t/ha) than in 2023 (4 t/ha). To determine if this could be linked to climatic factors, we assessed monthly temperature and precipitation levels in 2014, 2021, and 2023. We included 2021 in our analysis as Pst infections were generally absent in this year. In general, we found that yield levels in 2014, 2021 and 2023 could not be linked to differences in temperature and precipitation between years (Table 1). For instance, in the absence of Pst infections in 2021, we found that wheat varieties exhibited similar yields to the fungicide-treated plots assessed in 2014 (Figure 5A). Only 2 out of 8 varieties cultivated in both 2014 and 2023 exhibited yields higher than 6 t/ha after delayed fungicide treatment in 2023. Thus, the lower yield in fungicide-treated plots in 2023 could not be attributed to any specific climatic factors analyzed.

Table 1. Temperature (T.) and precipitation (Prec.) data from January to May in 2014, 2021, and 2023 at Rimski šančevi locality.
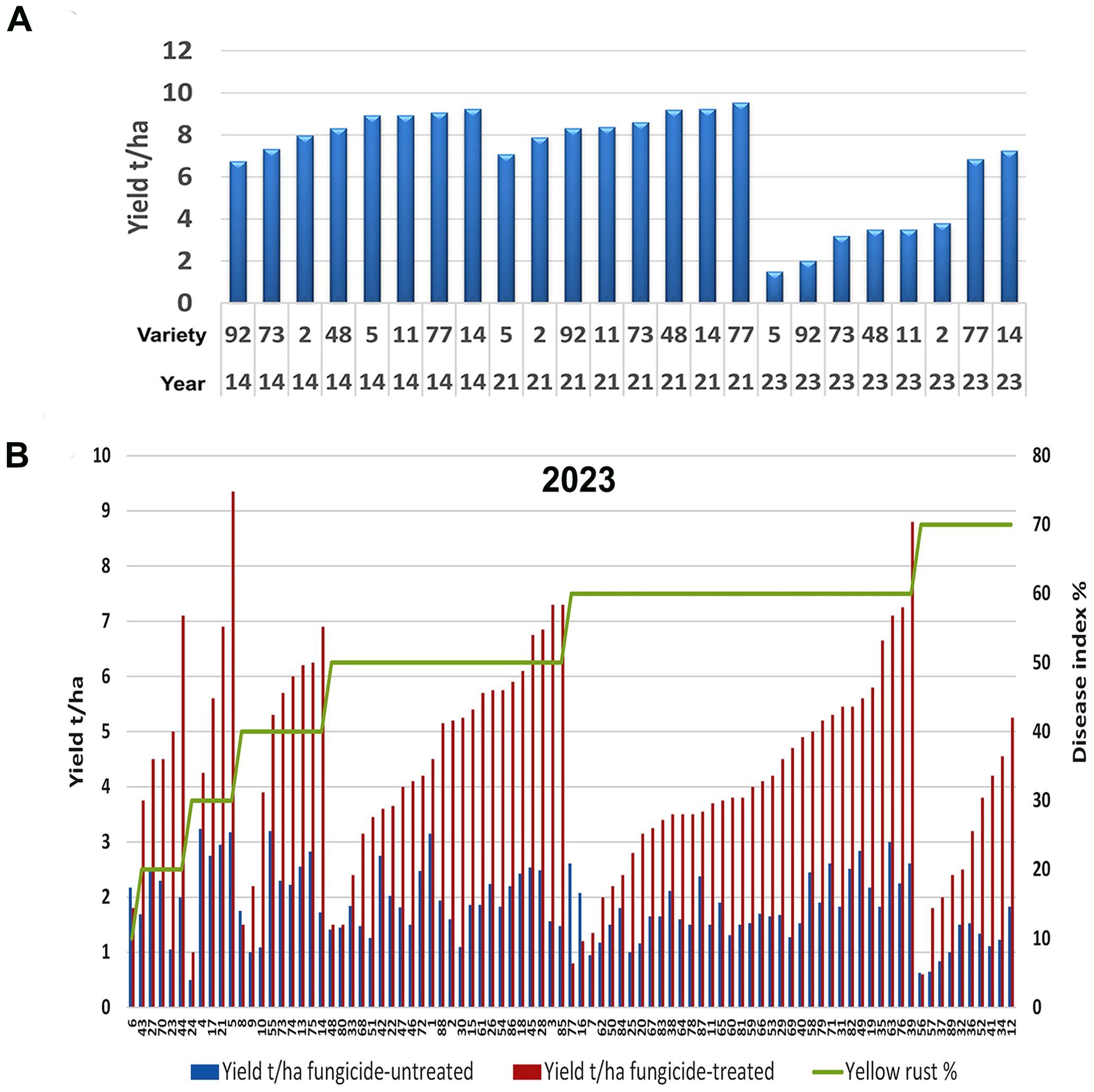
Figure 5. Fungicide application in 2023 did not succeed in restoring yield to levels achieved in the absence of Pst infections, and cultivars had different potentials in overcoming damage under high levels of Pst infection. (A) Contrary to 2023, delayed fungicide application in 2014 restored yield levels to those of untreated plots in 2021 when there was no yellow rust infection at the Rimski Šančevi locality. (B) Yellow rust disease index, yield in fungicide-treated and fungicide un-treated plots were tested in 2023 at Rimski šančevi locality on 89 commercial winter wheat varieties and after delayed fungicide treatment, yields ranged from 1 t/ha to nearly 9 t/ha, even under the same yellow rust infection level exceeding 50%. t/ha, tons per hectare.
We also noted diversity in genotype potentials in overcoming damage occurring under high levels of Pst infection resulted in diversity in yield achievements in fungicide-treated plots. For instance, in yield analysis of 89 cultivars in fungicide-treated plots in 2023, yields in untreated plots did not exceed 3 t/ha, but yields after delayed fungicide treatment ranged from 1 t/ha to almost 9 t/ha under the same level of yellow rust infection that exceeded 50% (Figure 5B). These results indicate that commercial varieties did not respond in the same manner to fungicide application in 2014 and 2023.
3.4 Potential recent change in the Pst population in Serbia
Due to changes in yellow rust disease severity noted in Serbia between 2014 and 2023, we decided to analyze the Pst population composition. We collected 26 Pst-infected wheat samples from the 2022-23 wheat season and subjected these samples to transcriptome sequencing. To determine the relationship between Pst isolates from Serbia in 2022/23, those prevalent in Serbia in 2014 and the broader dominant global Pst race groups, we gathered publicly available genomic and transcriptomic data from an additional 169 Pst isolates collected across 27 countries and conducted population genetic analysis (Supplementary Table S1). Phylogenetic analysis of these 195 Pst isolates indicated that Pst isolates identified in Serbia in 2022/23 formed a distinct clade that branched from the PstS10 race group (Figure 6). Whereas Pst isolates collected in Serbia in 2014 clustered within a clade that contained Pst isolates predominantly assigned to the PstS7 race group. This analysis supports a potential recent diversification within the Pst population detected in Serbia that coincides with the increase in yellow rust disease severity in the region.
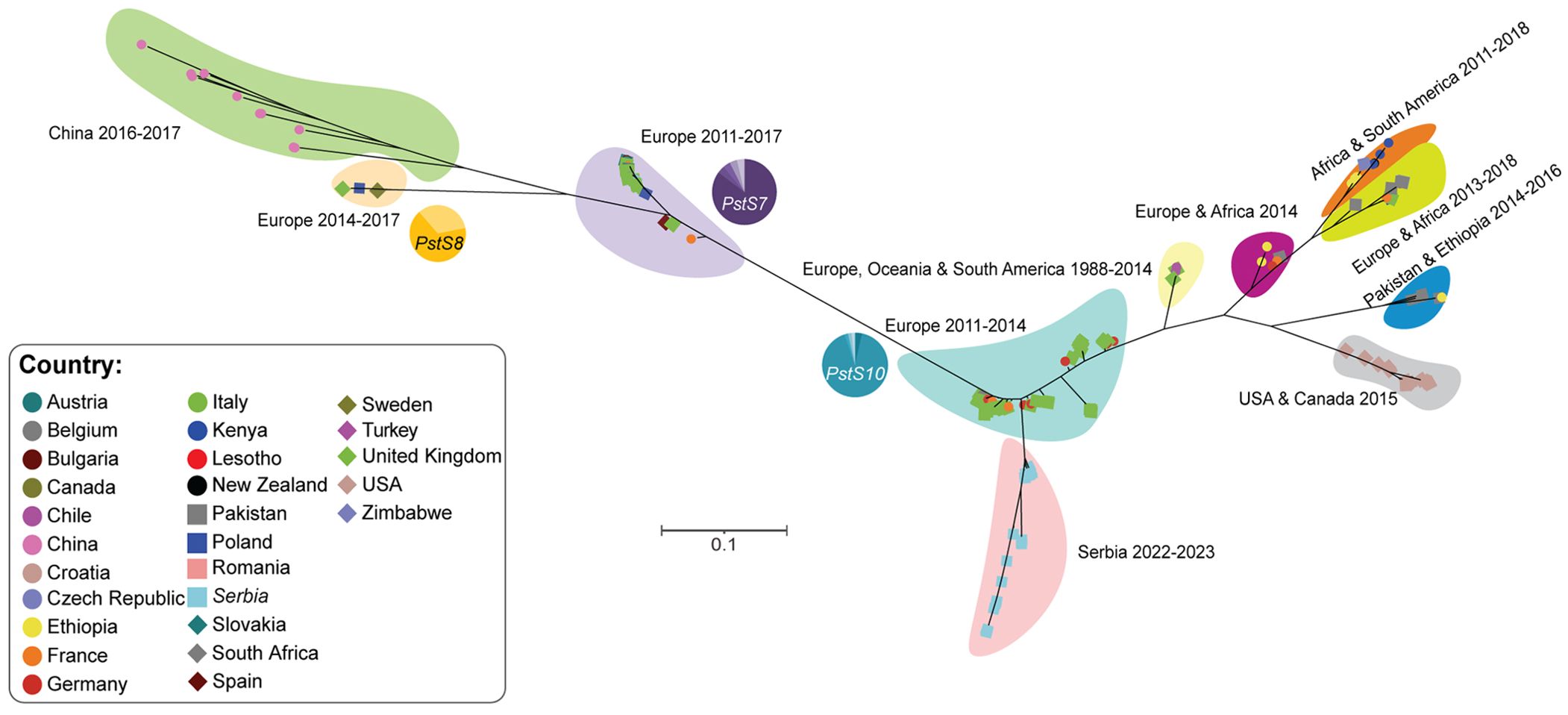
Figure 6. Phylogenetic analysis revealed that Pst isolates collected in 2022/23 in Serbia form a distinct clade. A total of 195 genomic and transcriptomic datasets representing Pst isolates from 27 countries were used for phylogenetic analysis. The third codon position of 4,682 gene models was extracted for each Pst isolate and phylogenetic analysis performed using a maximum likelihood approach. The proportion of Pst isolates belonging to three known race groups (PstS7, PstS8 and PstS10) are indicated with pie-charts next to the corresponding shaded area of the phylogeny.
4 Discussion
In this study, we demonstrated that yield achievements of susceptible wheat genotypes are not uniform in years favorable for Pst infection. It appears that for every wheat variety, there exists a specific level of yellow rust infection that triggers yield decrement. As little was known about the potential of fungicides to reduce yield decrement if applied later in the growing season when yellow rust disease severity index (DI) exceeds 20%, we analyzed fungicide effects on yield responses of susceptible genotypes. These results indicate that this relationship is complex and highly associated with the aggressiveness of the dominant Pst race(s) and the capabilities of the host plant to overcome damage caused by high levels of Pst infection. This indicates that more research is needed to elucidate the factors affecting plant responses to high levels of Pst infection and the potential of fungicide treatments to reduce yield losses under these circumstances.
Beyond the presence of the pathogen, climatic conditions are an important factor influencing pathogen outbreaks, alongside the susceptibility of host plants. It is known that elevated temperatures in January and February favor Pst infection in Serbia’s agro-ecological conditions. In Serbia, from 2014 to 2023, temperatures in January varied by almost 10°C, and in February by 7°C. Total precipitation also ranged from 1 to 65.5 mm in March and 11.1 to 74.5 mm in April. In 2014, yellow rust predominated over leaf rust for the first time in Serbia, causing enormous damage in wheat production areas (Jevtić et al., 2017, 2020), with the ‘Warrior’ race of Pst causing the epidemic in Serbia in 2014 (Jevtić et al., 2017, 2020). Whereas, in 2023 we detected a diversification in the Pst isolates identified, with all samples analyzed clustering in a clade branching from the PstS10 race group. This indicates a potential shift in the dominant Pst race present in Serbia in the past decade.
Virulence phenotypes of yellow rust are categorized into genetic lineages and designated with “Pst” followed by a sequential number (Ali et al., 2014; Hovmøller et al., 2016; Thach et al., 2016). Yellow rust races in the Northwestern European population, identified before 2011, belonged to a single clonal lineage known as PstS0 (Ali et al., 2014). From 2011 onwards, yellow rust races from the PstS7 (Warrior), PstS8 (Kranich), and PstS10 lineages became widespread in Europe, accounting for over 80% of the examined isolates (Ali et al., 2014; Hubbard et al., 2015; Hovmøller et al., 2016). After its appearance in Europe, PstS7 also emerged in North Africa (Ali et al., 2014; Hovmøller et al., 2016; Shahin, 2020). PstS10, previously referred to as Warrior (-), has been the dominant lineage in most parts of Europe since 2014 (Ali et al., 2014). Virulence pathotypes that predominates in other parts of the world are: PstS, closely related to PstS2, predominates in North America while PstS2 in East Africa (Hovmøller et al., 2008; Ali et al., 2014; Walter et al., 2016); PstS3 is widespread across West Asia, southern Europe, and North Africa (Ali et al., 2014); PstS4 comprises races primarily found on triticale in Northern Europe (Hovmøller et al., 2008; Hovmøller et al., 2016); Two races with distinct microsatellite profiles fall under PstS5 (Ali et al., 2014; Hovmøller et al., 2016; Thach et al., 2016) and PstS6 is another lineage common in East Africa (Ali et al., 2014; Hovmøller et al., 2016; Thach et al., 2016).
The occurrence of a more aggressive Pst race in Serbia in 2023 was also associated with lower yields in untreated plots compared to 2014. Additionally, it has been reported that varietal resistance to yellow rust prevents yield losses, and that yield responses of single susceptible varieties could differ in years conducive to yellow rust (Zhou et al., 2022). The study by Jevtić et al. (2017) yielded similar results, where the diversity in yield loss responses of susceptible cultivars to leaf rust and powdery mildew ranged from 20% to 50% over a ten-year period, despite the same level of leaf rust and powdery mildew infection at 20%. However, our study found that there might be a specific level of yellow rust infection that triggers yield decrement individually for each variety, and that susceptible varieties, although infected with high levels of yellow rust, can respond differently to the damage caused. This is consistent with similar analysis conducted for Fusarium head blight (FHB), where each wheat genotype was shown to tolerate a specific range of FHB disease pressure without showing a significant difference in expression of yield potential. Furthermore, it has been shown that defense mechanisms towards FHB are genotype-specific, indicating that resistant genotypes exhibit unique transcriptome profiles during Fusarium-host interactions (Pan et al., 2018).
Although wheat pathogens evolve in response to agro-ecological conditions, the combined effect of biotic and abiotic stressors on disease occurrence has frequently been overlooked in research (White et al., 2011; Juroszek and von Tiedemann, 2013). Heeb et al. (2019) advocated for a climate-smart pest management (CSPM) approach, yet they acknowledged the formidable challenge of creating a comprehensive model to predict short-term climate change-induced pest outbreaks at the local level. Understanding that the regulatory network of plant responses to abiotic and biotic stressors and their effects on yield consist of many components that may function antagonistically or synergistically (Glazebrook, 2005; Yasuda et al., 2008; Kissoudis et al., 2014). Our study highlights that when assessing yield achievements and cultivar responses to different levels of yellow rust pressure, more attention should be given to the combined effects of abiotic and biotic stressors. Furthermore, although the majority of studies have addressed fungicide efficacy in lowering host plant infection under diverse levels of pathogen pressure and have also investigated the most efficient timing for fungicide application, little is known about host plant responses to fungicide treatments when disease development is aborted later in the growing season and how it affects final yield achievements.
We found that yield loss between different genotypes in untreated plots ranged from 3% to 80% under yellow rust infection of 50%. This indicates that fungicide efficacy in reducing yield losses, if applied later in the growing season on susceptible genotypes, is complex. Generally, it can be expected that yield losses would be greater if susceptible varieties are infected in the early growth stages (Ash and Brown, 1990; Gaunt and Cole, 1991; Murray et al., 1995), due to the complexity of effects of Pst infection on all yield parameters (Ash and Brown, 1990). In our study, the first visible symptoms were recorded at the 30-32 BBCH (Stem elongation) in 2014, and at 20-23 BBCH (Tillering) in 2023. The higher aggressiveness of Pst races and much earlier infection in 2023 could have led to the lower yield achievements in untreated plots in 2023 compared to 2014. However, our study also indicated a significant difference in varietal responses to delayed fungicide application when high levels of Pst infections were already established. Although fungicide treatment resulted in the abortion of yellow rust development in later growth stages, varieties in our study showed different potential to recover from the damage caused by Pst infection and reimburse yield distortion. Consequently, our study indicates that more research should be done to elucidate mechanisms affecting host plant recovery from high levels of Pst infection, which would directly influence studies dealing with fungicide efficacy in preventing yield losses, especially when a high level of yellow rust infection is reached.
Establishing correlations between genotype and phenotype is essential for breeding wheat that is better adapted to the changing climate. This challenge is compounded by the fact that plant growth in natural habitats is influenced by a combination of abiotic and biotic stressors. Most existing research has concentrated on plant responses to individual stressors, leading to a limited understanding of adaptation to multiple stress factors. Given that 60% of expression changes under combinatorial stress conditions could not be predicted based on responses to individual stressors (Kissoudis et al., 2014), it can be inferred that the differences between susceptible and resistant plant reactions may be more closely linked to variations in the timing and magnitude of responses to combined stressors, rather than solely to the expression of individual genes (van Loon et al., 2006). There are documented instances of disease resistance being compromised by elevated temperatures and humidity, evidence suggests that temperature variations—rather than fixed thresholds—affect resistance mechanisms against yellow rust in wheat (Bryant et al., 2014). Additionally, drought and salinity have been shown to impact pathogen resistance responses, and the interaction between plants and pathogens can further influence plant responses to abiotic stress (Kissoudis et al., 2014). As a result, analyzing the association between morphological and physiological resistance traits conferred by adult plant resistance (APR) genes against obligate pathogens, alongside abiotic stressors and the expression of abiotic stress-related genes under field conditions, could yield a more detailed genotype profile. This, in turn, would aid in making informed decisions for breeding programs aimed at improving resistance to both biotic and abiotic stressors.
Given that phenotyping in plant breeding will always be an important practice, as determination of genetic variability may not always explain the complexity of phenotype markers (Velu and Singh, 2013), this study filled relevant knowledge gaps to facilitate the improvement of screening methods for winter wheat varieties’ responses to yellow rust infection. Our results indicated that the capabilities of commercial winter wheat varieties to overcome damage caused by high levels of Pst infection (>50%) are different, which affects fungicide efficacy in preventing yield losses. Our findings also suggest that the level of pathogen pressure that triggers susceptibility reactions of varieties is cultivar-specific and needs to be investigated in more detail in order to ensure more reliable yellow rust risk prediction in the future.
Data availability statement
Newly generated RNA-seq data was deposited in the European Nucleotide Archive (ENA) database under the accession number: PRJEB77002. Accession numbers for additional genomic and RNA-seq data used in the phylogenetic analysis is provided in Supplementary Table S1.
Author contributions
VŽ: Conceptualization, Formal analysis, Investigation, Methodology, Software, Visualization, Writing – original draft. LS: Formal analysis, Investigation, Writing – review & editing. DS: Data curation, Funding acquisition, Investigation, Methodology, Resources, Software, Supervision, Validation, Visualization, Writing – review & editing. RJ: Conceptualization, Data curation, Funding acquisition, Investigation, Methodology, Project administration, Resources, Supervision, Validation, Writing – review & editing.
Funding
The author(s) declare that no financial support was received for the research, authorship, and/or publication of this article.
Acknowledgments
This research was funded by Ministry of Science, Technological Development and Innovation of the Republic of Serbia, institutional funding - 200032 (Institute of Field and Vegetable Crops, Novi Sad) (RS-MESTD-inst-2020-200032). We thank Césarée Morier-Gxoyiya (JIC) for assistance with processing RNA-seq data. LS and DS are supported by the Biotechnology Biological Sciences Research Council (BBSRC) Institute Strategic Programs BB/X010996/1 and BB/X011003/1 and the John Innes Foundation.
Conflict of interest
The authors declare that the research was conducted in the absence of any commercial or financial relationships that could be construed as a potential conflict of interest.
Publisher’s note
All claims expressed in this article are solely those of the authors and do not necessarily represent those of their affiliated organizations, or those of the publisher, the editors and the reviewers. Any product that may be evaluated in this article, or claim that may be made by its manufacturer, is not guaranteed or endorsed by the publisher.
Supplementary material
The Supplementary Material for this article can be found online at: https://www.frontiersin.org/articles/10.3389/fpls.2024.1464454/full#supplementary-material
Supplementary Table S1 | Details of the Pst isolates used for conducting the phylogenetic analysis.
References
Ahmad, S., Afzal, M., Noorka, I. R., Iqbal, Z., Akhtar, N., Iftkhar, Y., et al. (2010). Prediction of yield losses in wheat (Triticum aestivum l.) caused by yellow rust in relation to epidemiological factors in faisalabad. Pak J. Bot. 42, 401–407.
Ali, S., Gladieux, P., Leconte, M., Gautier, A., Justesen, A. F., Hovmøller, M. S., et al. (2014). Origin, migration routes and worldwide population genetic structure of the wheat yellow rust pathogen Puccinia striiformis f.sp. tritici. PloS Pathog. 10, e1003903. doi: 10.1371/journal.ppat.1003903
Ash, G., Brown, J. (1990). Yield losses in wheat caused by stripe rust (Puccinia striiformis West.) in northern New South Wales. Aust. J. Exp. Agric. 30, 103–108. doi: 10.1071/ea9900103
Boshoff, W. H. P., Visser, B., Lewis, C. M., Adams, T. M., Saunders, D. G. O., Terefe, T., et al. (2020). First report of Puccinia striiformis f. sp. tritici, causing stripe rust of wheat, in Zimbabwe. Plant Dis. 104, 290–290. doi: 10.1094/pdis-07-19-1395-pdn
Brown, J. K. M., Hovmøller, M. S. (2002). Aerial dispersal of pathogens on the global and continental scales and its impact on plant disease. Science 297, 537–541. doi: 10.1126/science.1072678
Bryant, R. R. M., McGrann, G. R. D., Mitchell, A. R., Schoonbeek, H., Boyd, L. A., Uauy, C., et al. (2014). A change in temperature modulates defence to yellow (stripe) rust in wheat line UC1041 independently of resistance gene Yr36. BMC Plant Biol. 14, 10. doi: 10.1186/1471-2229-14-10
Bueno-Sancho, V., Persoons, A., Hubbard, A., Cabrera-Quio, L. E., Lewis, C. M., Corredor-Moreno, P., et al. (2017). Pathogenomic analysis of wheat yellow rust lineages detects seasonal variation and host specificity. Genome Biol. Evol. 9, 3282–3296. doi: 10.1093/gbe/evx241
Cao, X., Yao, D., Duan, X., Liu, W., Fan, J., Ding, K., et al. (2014). Effects of powdery mildew on 1000-kernel weight, crude protein content and yield of winter wheat in three consecutive growing seasons. J. Integr. Agric. 13, 1530–1537. doi: 10.1016/S2095-3119(14)60806-6
Carmona, M. A., Sautua, F. J., Pérez-Hernández, O., Grosso, C., Vettorello, L., Milanesio, B., et al. (2019). Rapid emergency response to yellow rust epidemics caused by newly introduced lineages of puccinia striiformis f. sp. tritici in argentina. Trop. Plant Pathol. 44, 385–391. doi: 10.1007/s40858-019-00295-y
Chen, W., Wellings, C., Chen, X., Kang, Z., Liu, T. (2014). Wheat stripe (yellow) rust caused by Puccinia striiformis f. sp. tritici. Mol. Plant Pathol. 15, 433–446.
Chen, X. (2020). Pathogens which threaten food security: Puccinia striiformis, the wheat stripe rust pathogen. Food Secur. 12, 239–251. doi: 10.1007/s12571-020-01016-z
Chen, X. M. (2005). Epidemiology and control of stripe rust [Puccinia striiformis f. sp. tritici] on wheat. Can. J. Plant Pathol. 27, 314–337. doi: 10.1080/07060660509507230
CIMMYT (1979). Instructions for the management and reporting of results for wheat program international yield and screening nurseries. Centro internacional de mejoramiento de maiz y trigo international maize and wheat improvement center (Londres, Mexico: CIMMYT). Available online at: https://repository.cimmyt.org/entities/publication/026bfc21-9d7c-42a6-9522-15fdcaf2ad30.
Corazza, L., Islongo, M. T. (1987). “Wheat and barley powdery mildew in italy,” in Integrated control of cereal mildews: Monitoring the pathogen. Eds. Wolfe, M. S., Limpert, E. (Springer).
de Vallavieille-Pope, C., Ali, S., Leconte, M., Enjalbert, J., Delos, M., Rouzet, J. (2012). Virulence dynamics and regional structuring of Puccinia striiformis f. sp. tritici in France between 1984 and 2009. Plant Dis. 96, 131–140. doi: 10.1094/PDIS-02-11-0078
Dobin, A., Davis, C. A., Schlesinger, F., Drenkow, J., Zaleski, C., Jha, S., et al. (2013). STAR: ultrafast universal RNA-seq aligner. Bioinformatics 29, 15–21. doi: 10.1093/bioinformatics/bts635
El Jarroudi, M., Kouadio, L., Bock, C. H., El Jarroudi, M., Junk, J., Pasquali, M., et al. (2017). A threshold-based weather model for predicting stripe rust infection in winter wheat. Plant Dis. 101, 693–703. doi: 10.1094/PDIS-12-16-1766-RE
Gaunt, R., Cole, M. (1991). An analysis of yield reduction caused by stripe rust in Rongotea wheat. Aust. J. Agric. Res. 42, 45–52. doi: 10.1071/ar9910045
Glazebrook, J. (2005). Contrasting mechanisms of defense against biotrophic and necrotrophic pathogens. Annu. Rev. Phytopathol. 43, 205–227. doi: 10.1146/annurev.phyto.43.040204.135923
Heeb, L., Jenner, E., Cock, M. J. W. (2019). Climate-smart pest management: building resilience of farms and landscapes to changing pest threats. J. Pest Sci. 92, 951–969. doi: 10.1007/s10340-019-01083-y
Hovmøller, M. S., Justesen, A. F., Brown, J. K. M. (2002). Clonality and long-distance migration of Puccinia striiformis f.sp. tritici in north-west Europe. Plant Pathol. 51, 24–32. doi: 10.1046/j.1365-3059.2002.00652.x
Hovmøller, M. S., Sørensen, C. K., Walter, S., Justesen, A. F. (2011). Diversity of Puccinia striiformis on cereals and grasses. Annu. Rev. Phytopathol. 49, 197–217. doi: 10.1146/annurev-phyto-072910-095230
Hovmøller, M. S., Walter, S., Bayles, R. A., Hubbard, A., Flath, K., Sommerfeldt, N., et al. (2016). Replacement of the European wheat yellow rust population by new races from the centre of diversity in the near-Himalayan region. Plant Pathol. 65, 402–411. doi: 10.1111/ppa.12433
Hovmøller, M. S., Yahyaoui, A. H., Milus, E. A., Justesen, A. F. (2008). Rapid global spread of two aggressive strains of a wheat rust fungus. Mol. Ecol. 17, 3818–3826. doi: 10.1111/j.1365-294X.2008.03886.x
Hubbard, A., Lewis, C. M., Yoshida, K., Ramirez-Gonzalez, R. H., de Vallavieille-Pope, C., Thomas, J., et al. (2015). Field pathogenomics reveals the emergence of a diverse wheat yellow rust population. Genome Biol. 16, 23. doi: 10.1186/s13059-015-0590-8
Jevtić, R., Jerković, Z., Denčić, S., Stojanović, S. (1997). Pojava žute rđe (Puccinia striiformis) na pšenici u 1997.
Jevtić, R., Lalić, B., Mihailović, D. T., Eitzinger, J., Alexandrov, V., Ventrella, D., et al. (2009). “Adaptation to diseases, pests and weeds caused by climatic changes and evaluation of associated risks in European regions – results from the ADAGIO Project,” in Impact of climate change and adaptation in agriculture. Eds. Eitzinger, J., Kubu, G. (University of Natural Ressources and Applied Life Sciences (BOKU, Vienna)), 121–123. Extended Abstracts of the International Symosium.
Jevtić, R., Župunski, V. (2023). The challenge of managing yellow rust (Puccinia striiformis f.sp. tritici) in winter wheat: how combined climate and pathogen stressors impact variability in genotype reactions. Front. Plant Sci. 14. doi: 10.3389/fpls.2023.1270087
Jevtić, R., Župunski, V., Lalošević, M., Jocković, B., Orbović, B., Ilin, S. (2020). Diversity in susceptibility reactions of winter wheat genotypes to obligate pathogens under fluctuating climatic conditions. Sci. Rep. 10, 19608. doi: 10.1038/s41598-020-76693-z
Jevtić, R., Župunski, V., Lalošević, M., Župunski, L. (2017). Predicting potential winter wheat yield losses caused by multiple disease systems and climatic conditions. Crop Prot. 99, 17–25. doi: 10.1016/j.cropro.2017.05.005
Jin, Y., Szabo, L. J., Carson, M. (2010). Century-old mystery of Puccinia striiformis life history solved with the identification of berberis as an alternate host. Phytopathology® 100, 432–435. doi: 10.1094/PHYTO-100-5-0432
Juroszek, P., von Tiedemann, A. (2013). Climate change and potential future risks through wheat diseases: a review. Eur. J. Plant Pathol. 136, 21–33. doi: 10.1007/s10658-012-0144-9
Kissoudis, C., van de Wiel, C., Visser, R. G. F., van der Linden, G. (2014). Enhancing crop resilience to combined abiotic and biotic stress through the dissection of physiological and molecular crosstalk. Front. Plant Sci. 5. doi: 10.3389/fpls.2014.00207
Li, H., Durbin, R. (2009). Fast and accurate short read alignment with Burrows–Wheeler transform. Bioinformatics 25, 1754–1760. doi: 10.1093/bioinformatics/btp324
Li, H., Handsaker, B., Wysoker, A., Fennell, T., Ruan, J., Homer, N., et al. (2009). The sequence alignment/map format and SAMtools. Bioinformatics 25, 2078–2079. doi: 10.1093/bioinformatics/btp352
Linde, C. C., Zhan, J., McDonald, B. A. (2002). Population structure of Mycosphaerella graminicola: from lesions to continents. Phytopathology® 92, 946–955. doi: 10.1094/PHYTO.2002.92.9.946
Lyon, B., Broders, K. (2017). Impact of climate change and race evolution on the epidemiology and ecology of stripe rust in central and eastern USA and Canada. Can. J. Plant Pathol. 39, 385–392. doi: 10.1080/07060661.2017.1368713
Ma, D., Zhou, X., Jing, J., Wang, W., Wang, L. (2013). Inheritance analysis of adult plant high-temperature stripe rust resistance in wheat cultivar xiaoyan 6. Acta Phytophylacica Sin. 40, 33–37.
Mihailovic, D. T., Lalic, B., Jevtic, R., Keserovic, Z., Petrovic, Ž., Jasnic, S. (2009). “Climate change impacts and adaptation options in Serbia – Results from the ADAGIO Project,” in Impact of climate change and adaptation in agriculture. Eds. Eitzinger, J., Kubu, G. (University of Natural Ressources and Applied Life Sciences (BOKU, Vienna), 78–80. Extended Abstracts of the International Symosium.
Murray, G. M., Ellison, P. J., Watson, A. (1995). Effects of stripe rust on the wheat plant. Australas. Plant Pathol. 24, 261–270. doi: 10.1071/APP9950261
Pan, Y., Liu, Z., Rocheleau, H., Fauteux, F., Wang, Y., McCartney, C., et al. (2018). Transcriptome dynamics associated with resistance and susceptibility against fusarium head blight in four wheat genotypes. BMC Genomics 19, 642. doi: 10.1186/s12864-018-5012-3
Peterson, R. F., Campbell, A. B., Hannah, A. E. (1948). A diagrammatic scale for estimating rust intensity on leaves and stems of cereals. Can. J. Res. 26c, 496–500. doi: 10.1139/cjr48c-033
Price, M. N., Dehal, P. S., Arkin, A. P. (2010). FastTree 2 – Approximately maximum-likelihood trees for large alignments. PloS One 5, e9490. doi: 10.1371/journal.pone.0009490
Pritsch, C., Muehlbauer, G. J., Bushnell, W. R., Somers, D. A., Vance, C. P. (2000). Fungal development and induction of defense response genes during early infection of wheat spikes by fusarium graminearum. Mol. Plant-Microbe Interactions® 13, 159–169. doi: 10.1094/MPMI.2000.13.2.159
Schwessinger, B., Sperschneider, J., Cuddy, W. S., Garnica, D. P., Miller, M. E., Taylor, J. M., et al. (2018). A near-complete haplotype-phased genome of the dikaryotic wheat stripe rust fungus Puccinia striiformis f. sp. tritici reveals high interhaplotype diversity. mBio 9. doi: 10.1128/mbio.02275-17
Shahin, A. A. (2020). Occurrence of new races and virulence changes of the wheat stripe rust pathogen (Puccinia striiformis f. sp. tritici) in Egypt. Arch. Phytopathol. Plant Prot. 53, 552–569. doi: 10.1080/03235408.2020.1767330
Sørensen, C. K., Hovmøller, M. S., Leconte, M., Dedryver, F., de Vallavieille-Pope, C. (2014). New races of Puccinia striiformis found in Europe Reveal race specificity of long-term effective adult plant resistance in wheat. Phytopathology® 104, 1042–1051. doi: 10.1094/PHYTO-12-13-0337-R
Thach, T., Ali, S., de Vallavieille-Pope, C., Justesen, A. F., Hovmøller, M. S. (2016). Worldwide population structure of the wheat rust fungus Puccinia striiformis in the past. Fungal Genet. Biol. 87, 1–8. doi: 10.1016/j.fgb.2015.12.014
van Loon, L. C., Rep, M., Pieterse, C. M. J. (2006). Significance of inducible defense-related proteins in infected plants. Annu. Rev. Phytopathol. 44, 135–162. doi: 10.1146/annurev.phyto.44.070505.143425
Velu, G., Singh, R. P. (2013). “Phenotyping in wheat breeding,” in Phenotyping for plant breeding. Eds. Panguluri, S., Kumar, A. (Springer, New York, NY), 41–71. doi: 10.1007/978-1-4614-8320-5_2
Walter, S., Ali, S., Kemen, E., Nazari, K., Bahri, B. A., Enjalbert, J., et al. (2016). Molecular markers for tracking the origin and worldwide distribution of invasive strains of puccinia striiformis. Ecol. Evol. 6, 2790–2804. doi: 10.1002/ece3.2069
Wegulo, S. N., Breathnach, J. A., Baenziger, P. S. (2009). Effect of growth stage on the relationship between tan spot and spot blotch severity and yield in winter wheat. Crop Prot. 28, 696–702. doi: 10.1016/j.cropro.2009.04.003
White, J. W., Hoogenboom, G., Kimball, B. A., Wall, G. W. (2011). Methodologies for simulating impacts of climate change on crop production. Field Crops Res. 124, 357–368. doi: 10.1016/j.fcr.2011.07.001
Xi, K., Kumar, K., Holtz, M. D., Turkington, T. K., Chapman, B. (2015). Understanding the development and management of stripe rust in central alberta. Can. J. Plant Pathol. 37, 21–39. doi: 10.1080/07060661.2014.981215
Yasuda, M., Ishikawa, A., Jikumaru, Y., Seki, M., Umezawa, T., Asami, T., et al. (2008). Antagonistic interaction between systemic acquired resistance and the abscisic acid–mediated abiotic stress response in arabidopsis. Plant Cell 20, 1678–1692. doi: 10.1105/tpc.107.054296
Zadoks, J. C. (1961). Yellow rust on wheat studies in epidemiology and physiologic specialization. Tijdschr. Plantenziekten 67, 69–256. doi: 10.1007/BF01984044
Keywords: wheat, wheat yellow rust, wheat yield, yield loss, control
Citation: Župunski V, Savva L, Saunders DGO and Jevtić R (2024) A recent shift in the Puccinia striiformis f. sp. tritici population in Serbia coincides with changes in yield losses of commercial winter wheat varieties. Front. Plant Sci. 15:1464454. doi: 10.3389/fpls.2024.1464454
Received: 14 July 2024; Accepted: 09 October 2024;
Published: 28 October 2024.
Edited by:
Ahmad Fakhoury, Southern Illinois University Carbondale, United StatesReviewed by:
Atef A. Shahin, Agricultural Research Center, EgyptGangming Zhan, Northwest A and F University, China
Copyright © 2024 Župunski, Savva, Saunders and Jevtić. This is an open-access article distributed under the terms of the Creative Commons Attribution License (CC BY). The use, distribution or reproduction in other forums is permitted, provided the original author(s) and the copyright owner(s) are credited and that the original publication in this journal is cited, in accordance with accepted academic practice. No use, distribution or reproduction is permitted which does not comply with these terms.
*Correspondence: Radivoje Jevtić, radivoje.jevtic@ifvcns.ns.ac.rs