- 1Maize Research Institute, Sichuan Agricultural University, Sichuan, Chengdu, China
- 2State Key Laboratory of Crop Gene Exploration and Utilization in Southwest China, Sichuan, Chengdu, China
- 3National Key Laboratory of Crop Genetic Improvement, Huazhong Agricultural University, Wuhan, Hubei, China
Fusarium species are the dominant cause of maize ear rot, but they also inflict serious damage to the roots and stalks. Theoretically, the organ where the host interacts with the pathogen most frequently should exhibit the highest degree of symptom-genotype correlation. Because that symptom-genotype correlation is an indicator reflecting the degree of coevolution between pathogen and its hosts. We wonder which organ is the main battlefield for the antagonism between maize and Fusarium. For this purpose, 43 isolates of Fusarium were isolated from infected maize ears. Fusarium verticillioides and F. graminearum are the two dominant pathogens, accounting for 44% and 30%, respectively. Furthermore, 14 elite maize inbreds were exposed to 43 Fusarium isolates and the symptoms of ear rot, stalk rot and root rot were investigated. In general, symptoms caused by F. graminearum were significantly more severe than those caused by other Fusarium species. Surprisingly, the genotype of F. verticillioides showed a strong correlation with stalk and root rot, but not with ear rot. Accordingly, our study may provide the first evidence that the stalk and root of maize, rather than the ear, is the main battlefield for the coevolution between maize and F. verticillioides.
1 Introduction
Maize (Zea mays L.) is a crucial global source of food, feed, and energy. In recent decades, the demand for increased maize yields has been a primary driver behind the ongoing development of commercial varieties. However, as maize yield gains have gradually slowed, the rise of destructive diseases has become the leading factor propelling the renewal of commercial varieties (Ristaino et al., 2021). Therefore, understanding the interaction between pathogens and maize is important for maize production.
Maize ear rot is a destructive disease, mainly caused by Fusarium species, poses a significant threat to maize production and quality (Duan et al., 2016). Fusarium species overwinter in soil, seeds, and plant debris (Xia et al., 2022). They spread to maize grains through the roots, insects, and the air (Xia et al., 2022; Omotayo and Babalola, 2023). They also cause root and stalk rot, affecting overall plant health and yield (María I et al., 2017). In addition, Fusarium species can produce harmful mycotoxins such as deoxynivalenol (DONs), fumonisins (FBs) or Zearalenone (ZENs), which pose a risk to animal and human health if consumed in excessive amounts (María I et al., 2017). In China, significant yield losses and mycotoxin contamination problems have been associated with Fusarium species (Duan et al., 2016; Qin et al., 2020).
The pathogen-host-driven natural selection contributes to shape the genetic and phenotypic diversities and results in coevolution of both organisms (Sironi et al., 2015). Pathogens have a negative effect on host fitness, favoring selection for enhanced defense mechanisms in the affected hosts. Conversely, host defenses are detrimental to the pathogen, leading to selection for novel attack mechanisms. When the interaction persists over time, the ongoing cycles of antagonism can generate highly specific host-pathogen interaction patterns, which are intrinsically determined by specific genotype interaction and are manifested as lineage-specific invasion (Schneider et al., 2015; Delaux and Schornack, 2021). Symptom-genotype correlation, the manifestation of specific genotype interaction, is a simple and feasible indicator reflecting the degree of coevolution between pathogen and its hosts. Theoretically, the organ in which the host interacts with the pathogen most frequently should exhibit the highest degree of symptom-genotype correlation. Given the extensive research conducted on the interaction between Fusarium and maize ears, we subjectively think that ears are the main battlefield for the antagonism between maize and Fusarium species. However, our studies unexpectedly revealed that the genotype of F. verticillioides was more closely related to its pathogenicity to maize stalks and roots than to maize ears. Prior to this study, there was no direct evidence that the localized interactions between Fusarium species and maize were the main factor driving its coevolution. Our study may provide the first evidence that the stalk and root of maize, rather than the ear, is the main battlefield for the coevolution between maize and F. verticillioides.
2 Results
2.1 The isolation frequency of F. verticillioides from maize ear rot is the highest in China
Different isolates of Fusarium spp. were isolated from infected maize ears from 20 cornfields in 7 provinces in China (Supplementary Table S1). Forty-three isolates were isolated and grouped into 4 categories, F. verticillioides (44%), F. graminearum (30%), F. proliferatum (21%), and F. fujikuroi (5%) (Supplementary Table S1), which is consistent with that F. verticillioides was previously reported as the dominant ear rot pathogen (Logrieco et al., 2002; Folcher et al., 2009; Lanubile et al., 2017; Feng et al., 2022). Fumonisin production was high for most F. verticillioides and F. proliferatum isolates but low for F. graminearum isolates when grown on maize medium, and no T2 toxin was detected from any isolates (Figure 1; Supplementary Table S1).
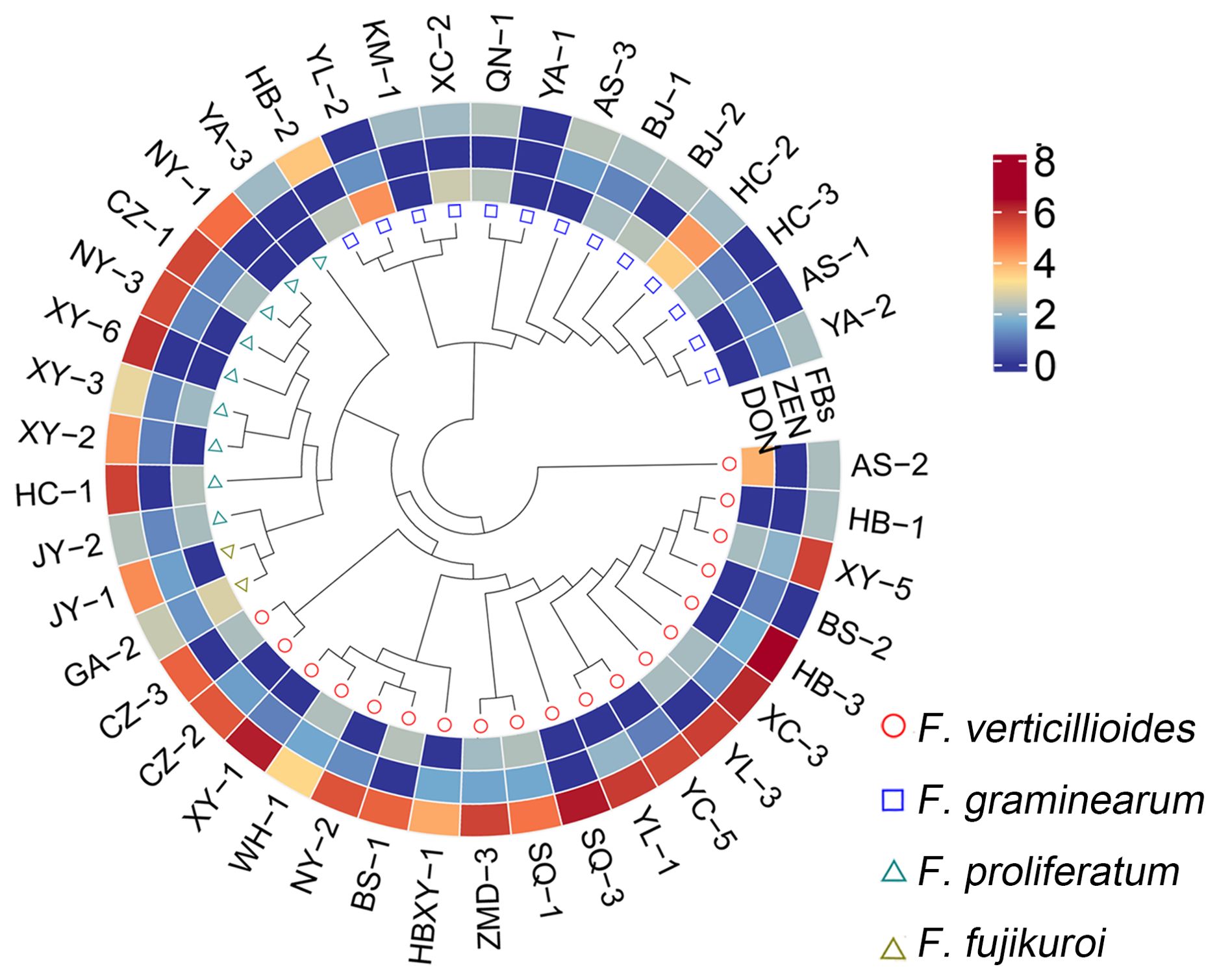
Figure 1. Topological phylogenetic tree of 43 Fusarium isolates and toxigenic capacity. The genetic distance matrix of 43 Fusarium isolates is showed as topological phylogenetic tree. The detail information was shown in Supplementary Table S4. The out layer heatmap represents the toxigenic capacity of different isolates grown in maize medium. The data used in heatmap are modified from Supplementary Table S1 by Log10 conversion. If the contents of FBs, T2 and DON were less than 100 μg/kg and of ZEN less than 10 μg/kg, the result was inaccurate and recorded as zero. FBs, Fumonisin; ZEN, zearalenone; DON, deoxynivalenol.
2.2 The symptoms caused by F. graminearum are significantly more severe than that caused by F. verticillioides and F. proliferatum
The pathogenicity of 43 isolates was tested using 14 elite maize inbred lines. All 43 isolates could infect maize ears, stalks and roots, and pathogen-host interactions showed a wide range of symptom severity (Supplementary Table S2; Figure 2). The pathogenicity of F. graminearum was remarkably higher than that of F. verticillioides and F. proliferatum, and was comparable between F. verticillioides and F. proliferatum (Supplementary Table S2; Figures 2D-F). KN5585 and LH8012 exhibited strong resistance to ear rot caused by F. graminearum (Figure 2A). LX7531, ZNC442 and LX8581 displayed good resistance to ear rot caused by F. verticillioides (Figure 2A). CIMBL145, LH8012, and ZH14 showed effective resistance to most Fusarium-caused stalk rot, whereas Zheng58 was susceptible to most Fusarium-caused stalk rot (Figure 2B).
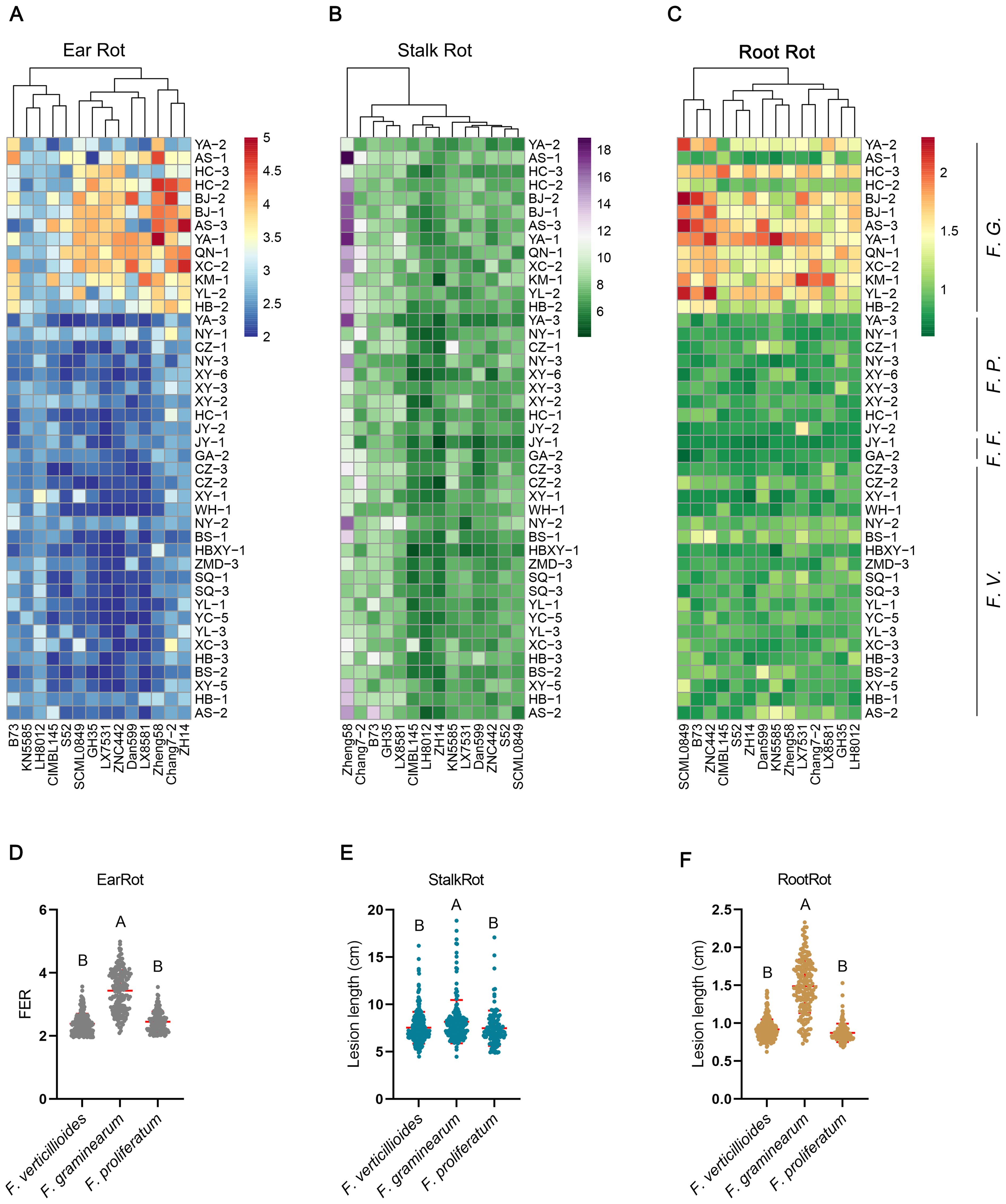
Figure 2. Severity of ear rot, stalk rot, and root rot of different pathogen-host pairwise interactions. (A-C) Severity of symptom for each pathogen-host pairwise was shown as heatmap. (D-F) Scatter plot of symptom severity caused by F. verticillioides (F.V.), F. graminearum (F.G.) and F. proliferatum (F.P.). F.F. is the abbreviation for F. fujikuroi. One point represents one pathogen-host interaction pairwise. The different capital letters above the scatter indicate that the difference between groups is significant at the level of p-value less than 0.01 using ANOVA analysis.
2.3 Stalks and roots are the main battlefield for the coevolution between maize and F. verticillioides
The relationship between fungal genotype, toxigenic capacity, and pathogenicity was further investigated. The correlation between the genotype of F. verticillioides and the symptom of stalk and root rot, but not ear rot, was notable (Figure 3A; Supplementary Tables S3-8). This implies that the coevolution of F. verticillioides and maize is mainly existed in the root and stalk, rather than the ear. The number of isolates of F. graminearum and F. proliferatum observed in this study was relatively low, which may explain why no significant correlation was found between the genotypes of these two pathogens and the different symptoms observed (Supplementary Tables S3-8). To test the effect of racial diversity on the genotype-symptom correlation, different numbers of F. verticillioides were progressively removed. With the decrease of racial diversity, the genotype-symptom correlation coefficient decreased, and the corresponding p value increased (Figure 3B). When the number of isolates was less than 17, the symptom-genotype correlation was no longer significant for both root and stalk rot (Figure 3B). To test the effect of maize diversity on the genotype-symptom correlation, different numbers of maize inbred lines were progressively removed. With the decrease of maize diversity, the genotype-symptom correlation coefficient decreased, and the corresponding p value increased (Figure 3C). These results indicated that in order to ascertain the correlation between genotype and symptoms with sufficient clarity, it is necessary to ensure that the pathogens and hosts under consideration exhibit sufficient diversity. An expansion of the pathogen-host interacting population results in a heightened correlation between genotype and symptoms.
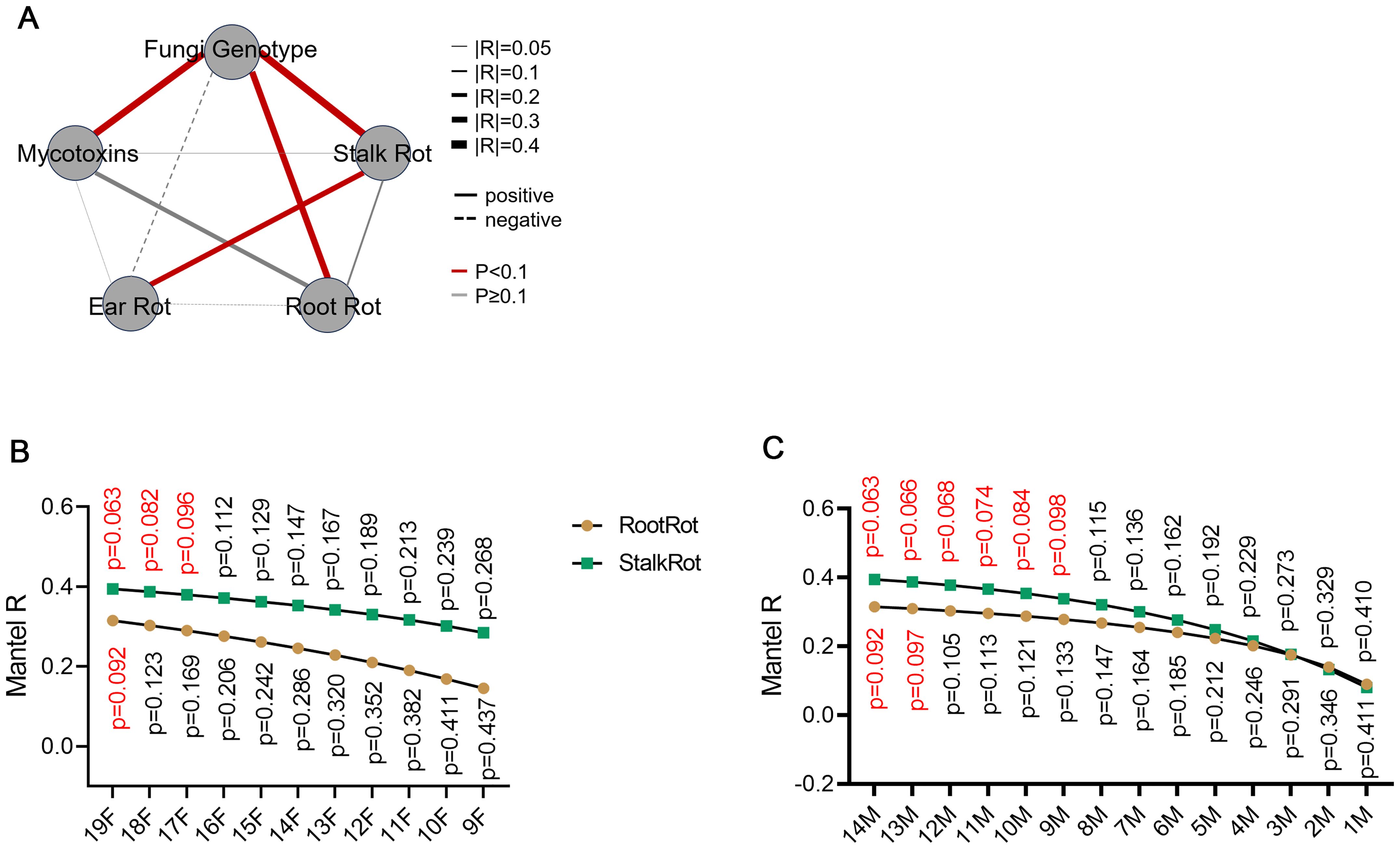
Figure 3. Stalks and roots are the main battlefield for the coevolution between maize and F. verticillioides. (A) Correlation between different traits of F. verticillioides. The correlation is considered statistically significant when the p-value is less than 0.1. The detail information of each trait was shown in Supplementary Tables S4-8. Mantel test was used to calculate the correlation and p-value between different traits. (B) The effect of pathogen diversity on the symptom-pathogenic genotype correlation was tested by retaining different numbers of F. verticillioides isolates. (C) The effect of maize diversity on the symptom-pathogenic genotype correlation was tested by retaining different numbers of inbred maize lines. For (B, C), The distance matrix of different traits was used to calculate correlations using the Mantel test with 999 permutations.
The toxigenic capacity is correlated well with fungal genotype in F. verticillioides and F. proliferatum, but not in F. graminearum (Figure 3A; Supplementary Table S3). The toxigenic capacity is not correlated with the symptom of ear rot, stalk rot, or root rot, indicating that the toxigenic capacity may not be a dominant determinate of pathogenicity.
3 Discussion
F. verticillioides and F. graminearum are the most widely reported causes of maize ear rot (Logrieco et al., 2002; Folcher et al., 2009; Gai et al., 2017; Lanubile et al., 2017; Feng et al., 2022). They also cause stalk, root and sheath rots (Duan et al., 2016; Lanubile et al., 2017; Wang et al., 2021). In this study, 44% of isolates are F. verticillioides, 30% are F. graminearum (30%), and 21% are F. proliferatum (21%). It is consistent with previous reports that F. verticillioides and F. graminearum are the dominant maize ear rot pathogens.
The distributions and pathogenicity of F. verticillioides and F. graminearum vary greatly in different environmental and climatic conditions (Ezrari et al., 2021; Liu et al., 2022). Although both F. verticillioides and F. graminearum can cause ear, stalk, and root rot in maize, there seems to be no studies that systematically compare the pathogenicity of the two pathogens at the population level. It was reported that the optimum temperature for growth of F. verticillioides is about 30°C, while cool growing conditions (20°C) promote the growth of F. graminearum (Samapundo et al., 2005; Hay et al., 2021; Ding et al., 2024). In this study, the field temperatures after inoculation were usually between 25 and 35°C, which may be more suitable for the growth of F. verticillioides. However, the results clearly showed that the severity of disease caused by F. graminearum to 14 elite maize inbred lines was significantly greater than that of F. verticillioides. This indicates that the pathogenicity of F. graminearum to maize is generally stronger than that of F. verticillioides.
F. verticillioides can survive in soil, seeds, and plant debris for extended periods. It is primarily a soil- and seed-borne fungus that moves from the root upward to the maize stalks and ears, causing stalk and ear rot (Omotayo and Babalola, 2023). It can also survive the winter as viable spores and is spread by airborne or insect vectors and rainfall (Omotayo and Babalola, 2023). The widespread existence of F. verticillioides in the soil made it necessary for maize roots to cope with the invasion of F. verticillioides at all times. Given the important economic attributes of maize ear, ear rot has received more attention than root and stalk rot. Thus, we subjectively think that ears may be the main battlefield for the antagonism between maize and Fusarium species before this study. To the best of our knowledge, there is currently no evidence can indicate the main organ in which the coevolution of maize and F. verticillioides has occurred. The organ where the pathogen frequently interacts with the host is expected to show the highest degree of symptom-genotype correlation (Schneider et al., 2015; Sironi et al., 2015; Delaux and Schornack, 2021). This correlation serves as a simple and feasible indicator of coevolution, as it is intrinsically shaped by the genotype interactions between pathogen and its hosts (Schneider et al., 2015; Sironi et al., 2015; Delaux and Schornack, 2021). Our findings indicate that the genotype of F. verticillioides is strongly associated with stalk and root rot, but not with ear rot. Consequently, this study may provide the first evidence that the stalk and root of maize, rather than the ear, is the main battlefield for the antagonism and coevolution between maize and F. verticillioides. The characteristics of the soil- and seed-borne form of F. verticillioides may explain why the antagonism and coevolution occurred mainly in the maize stalk and root.
Theoretically, a stronger long-term antagonism may result in a higher symptom-genotype correlation, while an increasing size of the pathogen-host interacting population may enhance this correlation (Schneider et al., 2015; Sironi et al., 2015; Delaux and Schornack, 2021). However, the specific size of the pathogen-host interacting population in which this correlation can be remarkably observed remains unclear. In previous studies, the correlation between genotype and symptoms was usually low or not statistically significant at all (Gai et al., 2017; Li et al., 2019; Han et al., 2020; Dong et al., 2022; Feng et al., 2022; Liu et al., 2024)(Supplementary Table S9). This may be due to the fact that the pathogen-host interacting populations were constructed with only one pathogen or host. In line with this, a decrease in the diversity of F. verticillioides or maize will decrease the genotype-symptom correlation coefficient and increase the corresponding p-value. In order to ascertain the correlation between genotype and symptoms with sufficient clarity, it is necessary to ensure that the pathogens and hosts under consideration exhibit sufficient diversity. Here, we suggested that the size of the pathogen-host interacting population is larger than a 20 × 15 matrix will be better. Our results showed that symptom-genotype correlation is a simple and feasible indicator of the degree of coevolution between pathogens and hosts, when the size of the pathogen-host interacting population is appropriate.
4 Materials and methods
4.1 Isolation of Fusarium spp.
Infected maize ears were collected from 20 locations in 7 provinces in southwest China. Asymptomatic kernels adjacent to the infected aera were surface sterilized with 75% ethanol for 5 min and incubated on potato dextrose agar (PDA) medium at 25°C. Pure culture of pathogen was established by single spore isolation. Three housekeeping genes, the translation elongation factor EF1α, the largest (RPB1) and second largest (RPB2) subunits of RNA polymerase, were amplified by polymerase chain reaction (PCR) for resequencing. Sequences were aligned in the Fusarium database (https://fusarium.mycobank.org/) for species identification. Primers are listed in Supplementary Table S10.
4.2 Analysis of genetic distance
The sequences of three housekeeping genes (EF1α, RPB1, and RPB2) were used for phylogenetic analysis and computing of pairwise genetic distance. Analyses were conducted using the Maximum Composite Likelihood model in MEGA7 (Kumar et al., 2016). Pairwise genetic distance was further used for correlation analysis with other phenotypes.
4.3 Determination of the content of four mycotoxins
As previous reported, Fusarium species were cultured in solid maize sand medium at 28 °C in the dark for 5 days. The contents of fumonisins (FBs), deoxynivalenol (DON), T-2, and zearalenone (ZEN) in the medium were determined with FD-600 (Femdetection, China) using immunofluorescence-based rapid quantitative test strips (Feng et al., 2022).
4.4 Artificial inoculation and symptom investigation of ear, stalk and root rots
To investigate the severity of ear and stalk rots, 14 elite inbred maize lines were planted in 2022 in Xishuangbanna (21° 53′ N, 100° 59′ E) and in 2023 in Chongzhou, China (30° 33′ N, 103° 39′ E). Seedlings were planted with 3.5 m single rows and 0.8 m row widths. Approximately 60 days after planting, a hole about 1 cm deep was made in the middle of the second stalk node using a 1 mm diameter electric drill. The hole was inoculated with 200 μL of spore suspension (5 × 106 spores/mL) and the lesion length was measured when the seed was mature. Fourteen days after silking for each inbred maize line, 200 μL of spore suspension (5 × 106 spores/mL) was inoculated into the ears using the side-needle syringe method. The severity of ear rot was assessed as in our previous report, based on the agricultural industry standard of the People’s Republic of China (NY/T1248.8-2016) (Feng et al., 2022). For each pathogen-host interaction, 20 ears or stalks from each replicate and two replicates at each location were investigated.
To assess the severity of root rot, healthy seeds were soaked in water overnight and incubated on wet seed germination paper at 25°C for about 2 days in the dark. Seeds with uniform growth were selected and transferred to fresh wet seed germination paper in a 13 × 13 cm Petri dish for 3 days of continuous growth. A wound was created by needle at the root position at about 4 cm from the seed, and then a hyphae-covered clump of PDA (0.3 cm diameter) was touched to induce root rot. The seeds were covered with wet germination paper to keep them moist and to ensure that the roots grew straight. About 4 days after inoculation, the lesion length was measured to reflect the severity of root rot. The best linear unbiased prediction (BLUP) values for each trait from two years were used to calculate symptoms distance matrix. Representative photos illustrating the inoculation site and symptoms of ear rot, stem rot, and root rot are presented in Supplementary Figure S1.
4.5 Correlation analysis between different traits
The vegdist function of the vegan package (https://CRAN.R-project.org/package=vegan) (Dixon, 2003) was used to construct the distance matrix of different isolates for the toxin production, the symptoms of ear, stalk and root rot. The mantel function was then used to calculate the correlation and p-value between different traits. To test the effect of pathogen and host diversity on the correlation, different numbers of pathogens or hosts were removed and the corresponding correlation and p value was calculated. For example, if three of the fourteen inbred maize lines were removed, 364 combinations and 364 correlation and p-values would be generated. Therefore, the correlation and p value would be calculated from the mean of the 364 values when the host diversity was eleven.
Data availability statement
The datasets presented in this study can be found in online repositories. The names of the repository/repositories and accession number(s) can be found in the article/Supplementary Material.
Author contributions
XF: Conceptualization, Data curation, Funding acquisition, Visualization, Writing – original draft, Writing – review & editing. HX: Data curation, Formal analysis, Investigation, Methodology, Resources, Validation, Writing – original draft. XX: Data curation, Formal analysis, Investigation, Validation, Writing – original draft. ML: Data curation, Formal analysis, Investigation, Writing – original draft. ZZ: Formal analysis, Investigation, Validation, Writing – original draft. QW: Investigation, Methodology, Validation, Writing – original draft. XZ: Data curation, Formal analysis, Validation, Writing – original draft. XG: Formal analysis, Methodology, Visualization, Writing – original draft. YL: Project administration, Resources, Supervision, Writing – review & editing, Writing – original draft.
Funding
The author(s) declare that financial support was received for the research, authorship, and/or publication of this article. We acknowledge funding from the Scientific and Technological Innovation 2023-Major Project of Biological Breeding of the Ministry of Agriculture (2023ZD04029), the National Key R&D Program of China (2021YFD1200700), the Natural Science Foundation of Sichuan Province (2023NSFSC0221), and the key Research Program of the Department of Science and Technology of Sichuan province (2021YFN0034).
Conflict of interest
The authors declare that the research was conducted in the absence of any commercial or financial relationships that could be construed as a potential conflict of interest.
Publisher’s note
All claims expressed in this article are solely those of the authors and do not necessarily represent those of their affiliated organizations, or those of the publisher, the editors and the reviewers. Any product that may be evaluated in this article, or claim that may be made by its manufacturer, is not guaranteed or endorsed by the publisher.
Supplementary material
The Supplementary Material for this article can be found online at: https://www.frontiersin.org/articles/10.3389/fpls.2024.1461896/full#supplementary-material
References
Delaux, P.-M., Schornack, S. (2021). Plant evolution driven by interactions with symbiotic and pathogenic microbes. Science 371. doi: 10.1126/science.aba6605
Ding, Y., Ma, N., Haseeb, H. A., Dai, Z., Zhang, J., Guo, W. (2024). Genome-wide transcriptome analysis of toxigenic Fusarium verticillioides in response to variation of temperature and water activity on maize kernels. Int. J. Food Microbiol. 410, 110494. doi: 10.1016/j.ijfoodmicro.2023.110494
Dixon, P. (2003). VEGAN, a package of R functions for community ecology. J. Veget. Sci. 14, 927–930. doi: 10.1111/j.1654-1103.2003.tb02228.x
Dong, S., Jiang, K., Huai, B., Ye, L., You, J., Ma, Y., et al. (2022). Genetic variability and pathogenicity of Fusarium verticillioides isolates from the summer-sown maize regions in China. Plant Pathol. 72, 582–592. doi: 10.1111/ppa.13673
Duan, C., Qin, Z., Yang, Z., Li, W., Sun, S., Zhu, Z., et al. (2016). Identification of pathogenic Fusarium spp. Causing maize ear rot and potential mycotoxin production in China. Toxins (Basel) 8. doi: 10.3390/toxins8060186
Ezrari, S., Radouane, N., Tahiri, A., Amiri, S., Lazraq, A., Lahlali, R. (2021). Environmental Effects of Temperature and Water Potential on Mycelial Growth of Neocosmospora solani and Fusarium spp. Causing Dry Root Rot of Citrus. Curr. Microbiol. 78, 3092–3103. doi: 10.1007/s00284-021-02570-1
Feng, X., Xiong, H., Zheng, D., Xin, X., Zhang, X., Wang, Q., et al. (2022). Identification of Fusarium verticillioides resistance alleles in three maize populations with teosinte gene introgression. Front. Plant Sci. 13, 942397. doi: 10.3389/fpls.2022.942397
Folcher, L., Jarry, M., Weissenberger, A., Ge´Rault, F., Eychenne, N., Delos, M., et al. (2009). Comparative activity of agrochemical treatments on mycotoxin levels with regard to corn borers and Fusarium mycoflora in maize (Zea mays L.) fields. Crop Prot. 28, 302–308. doi: 10.1016/j.cropro.2008.11.007
Gai, X. T., Xuan, Y. H., Gao, Z. G. (2017). Diversity and pathogenicity of Fusarium graminearum species complex from maize stalk and ear rot strains in northeast China. Plant Pathol. 66, 1267–1275. doi: 10.1111/ppa.2017.66.issue-8
Han, G., Li, C., Xiang, F., Zhao, Q., Zhao, Y., Cai, R., et al. (2020). Genome-wide association study leads to novel genetic insights into resistance to Aspergillus flavus in maize kernels. BMC Plant Biol. 20. doi: 10.1186/s12870-020-02404-5
Hay, W. T., Mccormick, S. P., Vaughan, M. M. (2021). Effects of atmospheric CO2 and temperature on wheat and corn susceptibility to Fusarium graminearum and deoxynivalenol contamination. Plants 10, 2582. doi: 10.3390/plants10122582
Kumar, S., Stecher, G., Tamura, K. (2016). MEGA7: molecular evolutionary genetics analysis version 7.0 for bigger datasets. Mol. Biol. Evol. 33, 1870–1874. doi: 10.1093/molbev/msw054
Lanubile, A., Maschietto, V., Borrelli, V. M., Stagnati, L., Logrieco, A. F., Marocco, A. (2017). Molecular basis of resistance to Fusarium ear rot in maize. Front. Plant Sci. 8. doi: 10.3389/fpls.2017.01774
Li, N., Lin, B., Wang, H., Li, X., Yang, F., Ding, X., et al. (2019). Natural variation in ZmFBL41 confers banded leaf and sheath blight resistance in maize. Nat. Genet. 51, 1540–1548. doi: 10.1038/s41588-019-0503-y
Liu, N., Chen, Y., Liu, J., Su, Q., Zhao, B., Sun, M., et al. (2022). Transcriptional differences between major Fusarium pathogens of maize, Fusarium verticillioides and Fusarium graminearum with different optimum growth temperatures. Front. Microbiol. 13. doi: 10.3389/fmicb.2022.1030523
Liu, C., He, S., Chen, J., Wang, M., Li, Z., Wei, L., et al. (2024). A dual-subcellular localized β-glucosidase confers pathogen and insect resistance without a yield penalty in maize. Plant Biotechnol. J. 22, 1017–1032. doi: 10.1111/pbi.14242
Logrieco, A., Mule, G., Moretti, A., Bottalico, A. (2002). Toxigenic Fusarium species and mycotoxins associated with maize ear rot in Europe. Eur. J. Plant Pathol. 108, 597–609. doi: 10.1023/A:1020679029993
María I, D., Eliana, C., Sebastián A, S. (2017). Fusarium–plant interaction: state of the art – a review. Plant Prot. Sci. 53, 61–70. doi: 10.17221/182/2015-pps
Omotayo, O. P., Babalola, O. O. (2023). Fusarium verticillioides of maize plant: Potentials of propitious phytomicrobiome as biocontrol agents. Front. Fungal Biol. 4. doi: 10.3389/ffunb.2023.1095765
Qin, P. W., Xu, J., Jiang, Y., Hu, L., van der Lee, T., Waalwijk, C., et al. (2020). Survey for toxigenic Fusarium species on maize kernels in China. World Mycotoxin J. 13, 213–224. doi: 10.3920/WMJ2019.2516
Ristaino, J. B., Anderson, P. K., Bebber, D. P., Brauman, K. A., Cunniffe, N. J., Fedoroff, N. V., et al. (2021). The persistent threat of emerging plant disease pandemics to global food security. Proc. Natl. Acad. Sci. U.S.A. 118, e2022239118. doi: 10.1073/pnas.2022239118
Samapundo, S., Devliehgere, F., De Meulenaer, B., Debevere, J. (2005). Effect of Water Activity and Temperature on Growth and the Relationship between Fumonisin Production and the Radial Growth of Fusarium verticillioides and Fusarium proliferatum on Corn. J. Food Prot. 68, 1054–1059. doi: 10.4315/0362-028X-68.5.1054
Schneider, D. S., Masri, L., Branca, A., Sheppard, A. E., Papkou, A., Laehnemann, D., et al. (2015). Host–pathogen coevolution: the selective advantage of Bacillus thuringiensis virulence and its cry toxin genes. PloS Biol. 13, e1002169. doi: 10.1371/journal.pbio.1002169
Sironi, M., Cagliani, R., Forni, D., Clerici, M. (2015). Evolutionary insights into host–pathogen interactions from mammalian sequence data. Nat. Rev. Genet. 16, 224–236. doi: 10.1038/nrg3905
Wang, W., Wang, B., Sun, X., Qi, X., Zhao, C., Chang, X., et al. (2021). Symptoms and pathogens diversity of Corn Fusarium sheath rot in Sichuan Province, China. Sci. Rep. 11. doi: 10.1038/s41598-021-82463-2
Keywords: pathogen-host interaction, coevolution, maize, fusarium, ear rot
Citation: Xiong H, Xing X, Liu M, Zhang Z, Wang Q, Zhang X, Gou X, Lu Y and Feng X (2024) Stalks and roots are the main battlefield for the coevolution between maize and Fusarium verticillioides. Front. Plant Sci. 15:1461896. doi: 10.3389/fpls.2024.1461896
Received: 09 July 2024; Accepted: 25 September 2024;
Published: 16 October 2024.
Edited by:
Brigitte Mauch-Mani, Université de Neuchâtel, SwitzerlandReviewed by:
Hosahatti Rajashekara, Directorate of Cashew Research (ICAR), IndiaThabiso Eric Motaung, University of Pretoria, South Africa
Copyright © 2024 Xiong, Xing, Liu, Zhang, Wang, Zhang, Gou, Lu and Feng. This is an open-access article distributed under the terms of the Creative Commons Attribution License (CC BY). The use, distribution or reproduction in other forums is permitted, provided the original author(s) and the copyright owner(s) are credited and that the original publication in this journal is cited, in accordance with accepted academic practice. No use, distribution or reproduction is permitted which does not comply with these terms.
*Correspondence: Xuanjun Feng, xuanjunfeng@sicau.edu.cn
†These authors have contributed equally to this work
‡ORCID: Xuanjun Feng, orcid.org/0000-0002-3800-4029