- 1International Centre of Insect Physiology and Ecology, Nairobi, Kenya
- 2Department of Agricultural Science and Technology, Kenyatta University, Nairobi, Kenya
- 3Department of Zoology and Entomology, University of Pretoria, Pretoria, South Africa
- 4International Centre for Tropical Agriculture, Kigali, Rwanda
- 5International Centre for Tropical Agriculture, Nairobi, Kenya
- 6Synelixis Solutions S.A., Chalkida, Greece
- 7Department of Agriculture Development, National and Kapodistrian University of Athens, Athens, Greece
Bush bean (Phaseolus vulgaris L.) production is undermined by soil degradation and low biological nitrogen fixation (BNF) capacity. This study evaluated the effect of black soldier fly frass fertilizer (BSFFF) on bush bean growth, yield, nutrient uptake, BNF, and profitability, in comparison with commercial organic fertilizer (Phymyx, Phytomedia International Ltd., Kiambu, Kenya), synthetic fertilizer (NPK), and rhizobia inoculant (Biofix, MEA Fertilizers, Nairobi, Kenya). The organic fertilizers were applied at rates of 0, 15, 30, and 45 kg N ha−1 while the NPK was applied at 40 kg N ha−1, 46 kg P ha−1, and 60 kg K ha−1. The fertilizers were applied singly and in combination with rhizobia inoculant to determine the interactive effects on bush bean production. Results showed that beans grown using BSFFF were the tallest, with the broadest leaves, and the highest chlorophyll content. Plots treated with 45 kg N ha−1 BSFFF produced beans with more flowers (7 – 8%), pods (4 – 9%), and seeds (9 – 11%) compared to Phymyx and NPK treatments. The same treatment also produced beans with 6, 8, and 18% higher 100-seed weight, compared to NPK, Phymyx, and control treatments, respectively. Beans grown in soil amended with 30 kg N ha−1 of BSFFF had 3–14-fold higher effective root nodules, fixed 48%, 31%, and 91% more N compared to Phymyx, NPK, and rhizobia, respectively, and boosted N uptake (19 – 39%) compared to Phymyx and NPK treatments. Application of 45 kg N ha−1 of BSFFF increased bean seed yield by 43%, 72%, and 67% compared to the control, NPK and equivalent rate of Phymyx, respectively. The net income and gross margin achieved using BSFFF treatments were 73 – 239% and 118 – 184% higher than the values obtained under Phymyx treatments. Our findings demonstrate the high efficacy of BSFFF as a novel soil input and sustainable alternative for boosting BNF and improving bush bean productivity.
1 Introduction
Globally, bush beans (Phaseolus vulgaris L.) are highly-valued pulse crops for their critical role in food and nutrition security, income generation, and soil health management (Broughton et al., 2003). Its dietary roles as human food (Araujo et al., 2020) and livestock feed (Ojiewo et al., 2015) make it a salient legume in addressing food insecurity (Katungi et al., 2010). The health risks associated with the consumption of animal proteins have prompted a shift to safer legume-based proteins such as bush beans (Daryanto et al., 2015). Most small-scale farmers incorporate bush beans in cropping systems to boost and maintain soil fertility through biological nitrogen (N) fixation, soil moisture conservation, and erosion control (Sousa et al., 2022).
Bush bean yield remains low partly due to soil nutrient depletion, high fertilizer costs, biotic stress factors (pests and diseases), and climate change effects (low and unreliable rainfall and increased temperatures) (Wanjala et al., 2019; Beebe et al., 2013). The situation has been worsened by the alarming levels of soil degradation coupled with skyrocketing fertilizer prices (FAO, IFAD, UNICEF, WFP and WHO, 2022). For optimal growth and yield, bush beans require 40 kg N ha-1 (Chekanai et al., 2018), 46 kg phosphorus (P) ha-1 (Koskey et al., 2017), 60 kg potassium (K) ha-1 (Tsige et al., 2022), and adequate micronutrients per season. However, the demand cannot be met due to the highly degraded nature of sub-Saharan Africa (SSA) soil. Estimates show that around 29% of arable land in sub–Saharan Africa has undergone intense degradation (Zingore et al., 2015; Nungula et al., 2023) due to poor farming activities. Soils in sub–Saharan Africa lose 10 – 70 kg of N, 2 – 10 kg of P, and 8 – 50 kg of K (Stoorvogel and Smaling, 1998; Nyawade et al., 2019), which is a major setback to agricultural production (Mohammed et al., 2020; Chirwa et al., 2015). To replenish these nutrients, farmers should use organic and mineral fertilizers, as well as bio-fertilizers. However, available organic fertilizers have poor quality (Ndambi et al., 2019; Jensen et al., 2012), while mineral fertilizers are costly, inaccessible, and require a lot of energy to produce them to supply the secondary and micronutrients required for optimal crop production (Fairhurst, 2012). Additionally, the use of mineral fertilizers has raised a lot of concerns due to their negative impact on human health and the environment (Mutuma et al., 2014; Sairaam et al., 2023).
Rhizobia bio-fertilizers naturally supply the N required by beans through the process of biological N fixation (BNF). However, BNF is less effective in most soils due to low populations of effective rhizobia strains, arising from gross reduction in soil biodiversity. The effectiveness of most commercial rhizobia inoculants is affected by competition from the indigenous rhizobacteria (Araujo et al., 2020), soil acidity, moisture stress, high temperatures, and deficiency of the nutrients required for a successful BNF process (Zaheer et al., 2022; Hungria et al., 2013). Eventually, farmers do not benefit from the application of rhizobia-based biofertilizers and continue to experience decreased bush bean yields (Kyomuhendo et al., 2018). Phosphorus is the most critical nutrient required for BNF (Stevens et al., 2019) due to its significant role in supplying the energy required during the process (Schulze et al., 2006). Most tropical soils are acidic and deficient in P (Montanarella et al., 2016; Mugo et al., 2021), therefore not readily available for the plant. Also, acidic soils suppress nodule formation, survival of rhizobium bacteria (Wang et al., 2022; Mirriam et al., 2023), and their infection of the root nodules to cause N fixation (Morón et al., 2005).
Organically managed soils have been reported to boost the BNF process through increased populations, biomass, and activities of free-living N-fixing bacteria (Wessén et al., 2010), majorly due to abundant organic carbon (C) levels that act as energy sources (Fernández-Luqueño et al., 2010). The BNF process can supply around 700 kg of N per hectare in legume cropping systems (Soumare et al., 2020; Herridge et al., 2008). Symbiosis alone can contribute 80% of N fixed on croplands (Mendoza-Suárez et al., 2020; Herridge et al., 2008). This demonstrates the sustainability and economic viability associated with the utilization of N fixation in place of mineral fertilizers for legume crop production (Araujo et al., 2020; Carranca, 2017; Jena et al., 2022). Succeeding crops can also benefit from the fixed nitrogen (Mirriam et al., 2022; Chapagain and Riseman, 2014), due to the high N transfer rate of up to 85% (Paynel et al., 2008; Moyer-Henry et al., 2006). This reduces the requirement of using costly fertilizers which are beyond reach by most smallholder farmers.
Novel and high-quality organic fertilizers such as insect frass fertilizer, which is derived from valorization of organic wastes using saprophytic insect larvae as bioconverters can restore soil health, boost BNF, and increase bush bean yield. Insect frass fertilizer is rich in nutrients, and beneficial microbes, besides being pathogen-free and requiring a short production time (Beesigamukama et al., 2022; Beesigamukama et al., 2021a; Adin Yéton et al., 2019; Lalander et al., 2015). Past studies have demonstrated the potential of insect frass fertilizer to boost beneficial soil microbes, suppress pests and diseases (Tanga and Kababu, 2023; Kemboi et al., 2022), enhance water-holding capacity, soil aeration, and soil aggregation (Beesigamukama et al., 2020b; Pagliai et al., 2004), reduce soil acidity and increase availability of nutrients, especially P, which is key for BNF (Anyega et al., 2021; Beesigamukama et al., 2021b). Additionally, frass fertilizer application suppresses soil-borne fungal pathogens such as Fusarium, Pythium, and Rhizoctonia, in different crops, including bush beans (Kemboi et al., 2022; Quilliam et al., 2020).
Application of BSFFF improves soil microbial activities better than commercial fertilizers (Gebremikael et al., 2015; Kagata and Ohgushi, 2012) and boosts soil ammonium (Beesigamukama et al., 2021b), which is highly preferred by microorganisms as an energy source. Previous studies have also demonstrated that BSFFF enhances plant tolerance to moisture stress (Abiya et al., 2022) and boosts flower formation and pollination (Barragán-Fonseca et al., 2022). The enhanced soil health benefits have resulted in higher nutrient uptake, yield, and economic returns from crops grown using black soldier fly frass fertilizer, compared to mineral fertilizers (Beesigamukama et al., 2022; Agustiyani et al., 2021; Anyega et al., 2021; Tanga et al., 2022).
Although BSFFF has demonstrated high potential in enhancing the growth of cereals and vegetable crops (Anyega et al., 2021; Beesigamukama et al., 2020a; Quilliam et al., 2020; Choi et al., 2009), there is limited evidence about the agronomic potential of this organic fertilizer on leguminous crops such as bush beans. The few studies involving BSFFF and legumes focused on French beans and did not determine BNF and profitability (Anyega et al., 2021; Quilliam et al., 2020). In as much as soil N is required, its overuse suppresses BNF through poor nodulation and inhibition of key enzyme activities especially nitrogenase (Reinprecht et al., 2020; Mingotte et al., 2019). As such, the optimal application rate of BSFFF for high bush bean yield, BNF, and profit margins remains largely unknown. There is limited research attention on the effect of the combined application of BSFFF and commercial rhizobia inoculant on BNF and bean yield, and the optimal BSFFF rate that can supply the starter N dose required during the BNF process. Such information would guide policy makers, researchers, non-government organizations, the private sector, and farmers in the promotion and effective use of BSFFF as a regenerative fertilizer input for improved bush bean production. Therefore, this study was undertaken to determine the effect of BSFFF on bush bean growth, yield, nitrogen uptake, BNF, and profitability, in comparison with commercial organic fertilizer, mineral fertilizer, and rhizobia-based biofertilizer.
2 Materials and methods
2.1 Experimental site
Field studies were conducted for two consecutive seasons (October – December 2022 and March – June 2023) at Kenyatta University Teaching and Demonstration Farm (36°55′34″ N, and 1°10′59″ E, 1720 meters above sea level), Kiambu County, Kenya. The area receives bimodal rainfall; the short rain season runs from October to December, while long rains are received between March and June. The annual average rainfall is 850 mm. Kiambu receives mean monthly temperature and relative humidity of 20 – 27°C and 65 – 84%, respectively. The area is relatively warm and is characterized by Ferralsols (FAO-UNESCO, 1990), with shallow depths, low organic matter content, and medium acidity. Land use history indicated that the site is used for annual crop production where sorghum was the previous crop.
Before experiments, soil sampling was conducted at a depth of 0–30 cm using a zig-zag method and soil auger. The field was subdivided into three sections and ten cores were collected per section; quarter sampling was used to collect a composite sample per section. The samples were air-dried for 5 days, and ground using mortar and pestle, pending analysis of selected physical and chemical properties using standard methods. Soil texture was determined using the Bouyoucos method following procedures described by Okalebo et al. (2002). Available P and total nitrogen were determined using Bray 1 method and using Kjedahl digestion and distillation method, respectively. Soil pH was measured using soil to water suspension (2.5:1), and pH and EC values were read directly using pH (AD1000, Adwa, Bucharest, Romania) and EC meter (AVI, Labtech, Mumbai, India), following procedures of Okalebo et al. (2002). Soil organic carbon (SOC) was determined following the Walkley-Black (wet oxidation) method (Walkley and Black, 1934). As per Jackson and Mahmood (1994), exchangeable cations (K, magnesium [Mg], sodium [Na], and calcium [Ca]) were determined using an Atomic Absorption Spectrophotometer (AAS). Table 1 presents the selected characteristics of the soil used in the study.
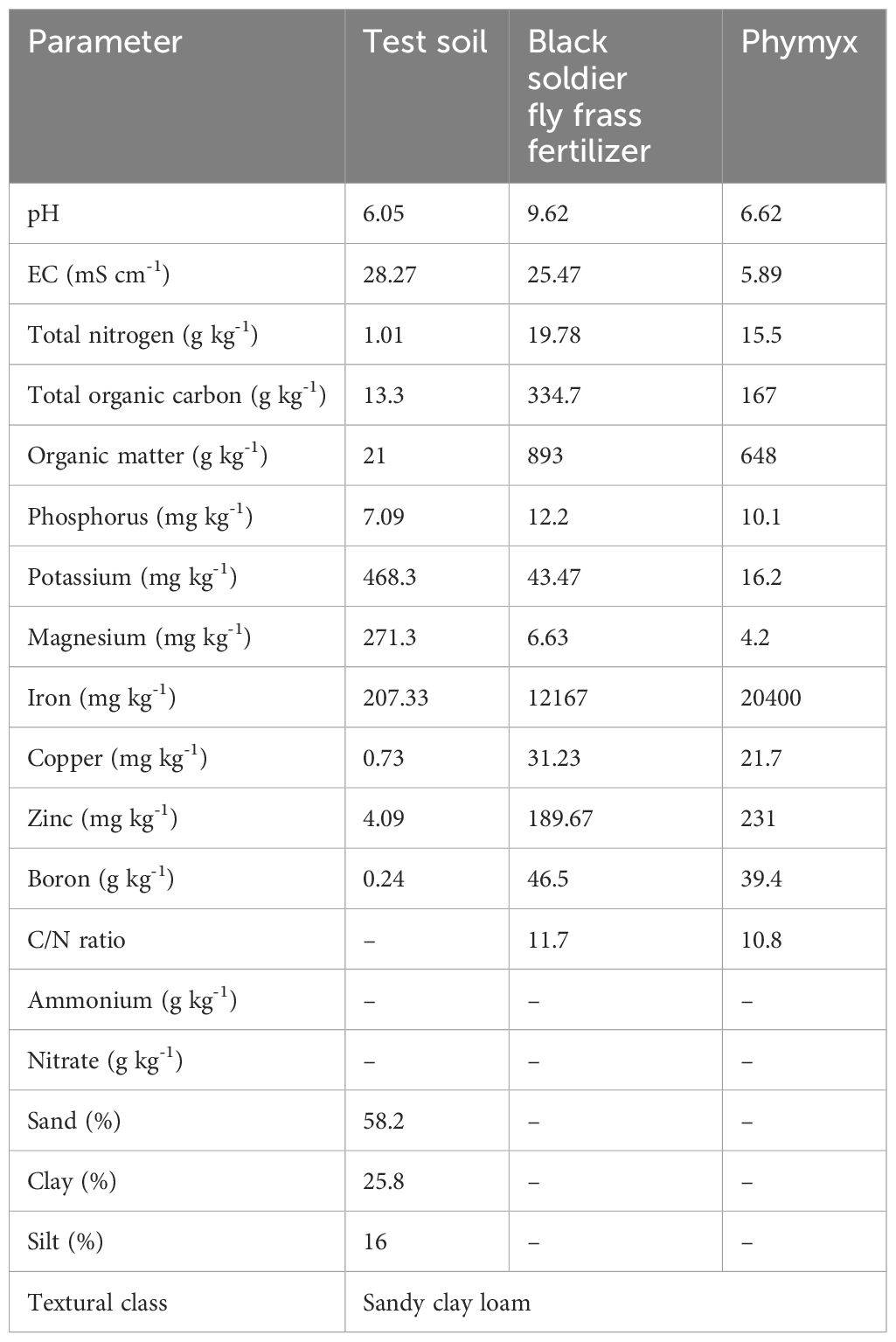
Table 1. Selected chemical and physical characteristics of soil and organic fertilizers used during the field study.
Throughout the study period, the total monthly rainfall and average monthly temperature data were collected from Kenyatta University weather station. The mean monthly temperatures of 25.2– 27.3°C and 24.1 – 26.2°C were recorded during the short and long rain seasons, respectively. The total amount of rainfall received was 243 mm, and 499 mm during the short and long rain seasons, respectively (Figure 1). Rainfall intensity was highest in November and April for the 2022 short rains and 2023 long rains, respectively.
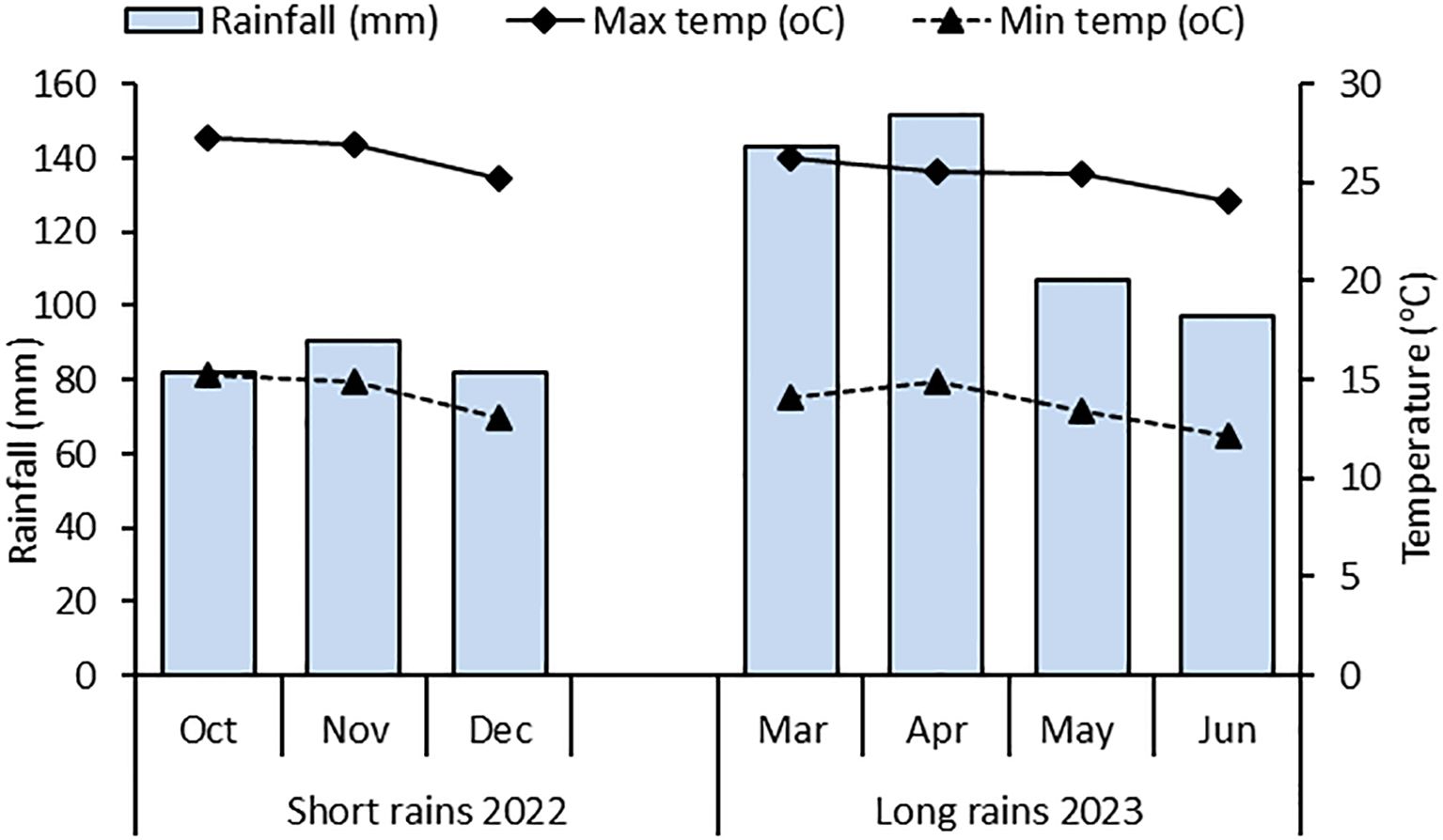
Figure 1. Monthly average temperature (Minimum and maximum) and total monthly rainfall during field experiments.
2.2 Source of experimental materials
The experiment consisted of four fertilizers: Black soldier fly frass fertilizer (BSFFF), commercial organic fertilizer (Phymyx) (Phytomedia International Limited, Kiambu County, Kenya), biofertilizer (Rhizobia inoculant) (Biofix) (MEA Fertilizers, Nairobi, Kenya) and mineral fertilizer (NPK). The BSFFF was produced by composting frass from the black soldier fly (BSF) colony maintained in the animal rearing and quarantine unit of the International Center of Insect Physiology and Ecology (icipe). The BSF frass was obtained by feeding BSF larvae on potato waste following procedures by Beesigamukama et al. (2020) and Shumo et al. (2019). The frass was composted for five weeks using the heap method, resulting in a mature and stable organic fertilizer product (Beesigamukama et al., 2021a). The organic fertilizers were subjected to laboratory analysis using standard procedures and results are presented in Table 1. Bush bean seeds (Rosecoco GLP2 bush bean seeds) were procured from Simlaw Seed Company Limited, Nairobi. This is a high-yielding variety recommended for medium and low-altitude areas of Kenya (KALRO, 2008). The NPK fertilizers (urea, DAP, MOP) were sourced from Kenya Farmers Association (KFA) stores in Nairobi.
2.3 Treatments and experimental setup
There were 15 treatments, grouped into two sets. In the first set of treatments, BSFFF and Phymyx were applied at three rates equivalent to 15, 30, and 45 kg N ha−1 to determine the optimal N application rate for improved bush bean production. The rates were denoted as 15BSFFF, 30BSFFF, and 45BSFFF for 15, 30, and 45 kg N ha−1 BSFFF treatments, respectively, and the respective rates for Phymyx were indicated as 15PHY, 30PHY, and 45PHY. The rates of 15, 30, and 45 kg N ha−1 were supplied by applying 522.6, 1045.3, and 1567.9 kg ha−1 of BSFFF, respectively, and 967.7, 1935.5, and 2903.2 kg ha−1 of Phymyx, respectively. The mineral fertilizer treatment applied at rates of 40 kg N ha−1, 46 kg P ha−1, and 60 kg K ha−1 (Chekanai et al., 2018) was included for comparison purposes. The N, P, and K were supplied using urea (47.8 kg ha−1), diammonium phosphate (100 kg ha−1), and muriate of potash (120.5 kg ha−1), respectively. In the second set of treatments, the mineral and organic fertilizers were applied at the rates described above and combined with rhizobia (Rh) inoculant to determine their interaction effects on bush bean growth, yield, and BNF. These treatments were denoted as Rh+15BSFFF, Rh+30BSFFF, and Rh+45BSFFF for BSFFF, Rh+15PHY, Rh+30PHY, Rh+45PHY for Phymyx, and Rh+NPK for mineral fertilizer. The rhizobia biofertilizer was applied by inoculating bean seeds following the manufacturer’s instructions. The seeds requiring inoculation were first coated with 5% gum Arabic to make them sticky, and later mixed with the inoculum at a rate of 100 g of inoculum per 15 kg of bean seeds (Maingi et al., 2001). The control treatment consisted of unfertilized soil and uninoculated seeds.
The experiment was laid out using a randomized complete block design (RCBD) and replicated three times. Plot sizes of 3 m × 3 m with border width of 0.5 m and inter-block distance of 1 m were used. The bush bean variety Rosecoco GLP2 was used as the test crop. The beans were planted in rows spaced 0.5 m apart with two seeds being sowed per hill at an inter-hill spacing of 0.1 m (Gicharu et al., 2013). The organic and mineral fertilizers were applied using the band method; the organics were applied a day before planting, while the mineral fertilizers were applied at planting. Gap filling was conducted 7 days after planting to maintain a uniform plant population across treatments. Across all the treatments, weeding was conducted using a hand hoe, whereas pests such as aphids, and blight disease were controlled by spraying the crop with recommended pesticides (Prove 1.9 EC) and fungicides (Funguran-OH 50WP) at vegetative stage. The pesticide was diluted at a rate of 15ml per20 litres of water, while the fungicide was dissolved at a rate of 50 grams per 20 litres of water.
2.4 Data collection
2.4.1 Bean growth
Data was collected on leaf length, number of leaves, chlorophyll content, plant height, leaf width, and stem diameter using 10 plants that were randomly selected from the four inner rows of each plot. Data was collected at the following phenological stages: early vegetative growth (14 days after planting) (dap), flower formation (28 dap), pod formation (42 dap), seed filling (56 dap), and senescence (70 dap). Chlorophyll content was measured using a Soil and Plant Analysis Development (SPAD) meter (Konica-Minota 502, Chiyoda, Japan) placed on three of the topmost fully grown leaves. Plant height was measured using a tape measure from the soil surface to the plant tip. The number of leaves was determined by counting the photosynthetically active leaves. Leaf width was measured using a tape measure placed at the broadest part of the leaf whereas leaf length was measured from the petiole base to apex. Leaf areas were the product of leaf width and leaf length. Stem girth was measured using a digital vernier caliper (Silverline Digital Vernier Caliper 300 mm, Toolstream Ltd, Yeovil, UK) placed on the plant stem at 5 cm from the soil surface. The number of flowers was determined at 42 dap by hand counting while pod length number of pods per plant, and that of seeds per pod were determined at the physiological maturity stage (56 dap).
2.4.2 Bean yield
Yield data was collected at the harvesting stage (79 dap) when the pods were completely dry. At harvesting, plants were carefully uprooted to avert seeds from scattering. Shelling was done manually, and fresh weights of the seeds and haulms per plot were measured using a weighing scale. Sub samples of 500 g each for seeds and haulms were air-dried for 72 hours and oven-dried at 60°C for 48 hours to drive off all the moisture. The dry weights obtained were used to determine moisture content and dry matter yield on a hectare basis (t ha−1). Furthermore, 100 randomly selected seeds per treatment were weighed to determine the 100 seed weight (Khanam and Platel, 2016).
2.4.3 Nutrient uptake
Representative samples of the bean seeds and haulms were collected randomly, air-dried for 72 hours and oven-dried at 60°C for 48 hours to a constant weight. The dried samples were ground into powder using an analytical mill and subjected to laboratory analysis to determine the concentrations of N, P, and K using standard laboratory procedures (Okalebo et al., 2002). The nutrient concentrations and yield data obtained in section 2.3 were used to determine nutrient uptake in seeds (Equation 1) and haulms (Equation 2). Total nutrient uptake was computed as the sum of uptake in seeds and haulms.
2.4.4 Determination of biological nitrogen fixation
Biological N fixation was assessed by determining the total number of nodules, number of effective nodules, nodule weight per plant, and quantity of N fixed. The total and effective root nodules were assessed between 50% flowering and early pod formation (42 – 56 dap), this is the stage when active nodule formation occurs (Zhou et al., 2021). Ten randomly selected plants per plot were carefully uprooted to avoid nodule detachment. The total number of root nodules per plant was determined using a tally counter. The nodules were cautiously detached from the roots and placed in plastic sampling bags that were tightly sealed, transported to the laboratory in a cooler box containing dry ice, and stored at -20°C (Korir et al., 2017) to keep them fresh pending nodule assessment. Physical examination, which involved slicing the nodules using a sterile scalpel was done. Any sliced nodule that had pink pigmentation (leghaemoglobin) was considered effective, while those that had other colorations were characterized as ineffective (Jourand et al., 2004).
The amount of N fixed by bush beans was determined using the N-difference method, which computes the difference between the total N uptake of the N-fixing crop (legume) and the non-N-fixing crop (Peoples et al., 1989). Maize (Katumani variety) was used as the reference crop (Cafaro La Menza et al., 2020) since maize (Zea mays L.) and beans have similarities in root architecture (Ordóñez et al., 2018; Nichols et al., 2019). Also, the maturity period of the maize variety used (2.5 – 3 months) is similar to that of the bush bean variety used in this study. Maize was planted in unfertilized plots at a spacing of 75 cm between rows and 30 cm between plants. At maturity, maize yield, and N uptake in grain and stover were determined using standard procedures. The quantity of N fixed by beans was determined using Equation 3.
Where TNCfc and TNCnfc represent the total N uptake of the fixing (beans) and non-fixing (maize) crops, respectively.
2.4.5 Economic returns to bush bean production
The profitability of bush bean production using BSFFF, and commercial fertilizer was assessed by determining net income, benefit to cost ratio, return on investment, and gross margins. Net income was determined as the difference between variable costs and revenue. The variable costs considered during the study included the cost of seeds, fertilizers, rhizobia inoculants, labor, pesticides, and fungicides). The average prices of fertilizers and seeds were sourced from the Kenya Farmers’ Association and Africa Fertilizer Website (Africa Fertilizer, 2022). On the other hand, the price of bush bean seeds kg−1 was obtained from the Humanitarian Data Exchange (2023). The labor costs (land preparation, planting, fertilizer application, weeding, pest, and disease management, and harvesting) were determined using the hourly rate of US$ 1.25 (Kenya Government, 2022). Bean yield was considered as the source of revenue. Return on investment, gross margin, and benefit cost ratio were calculated using Equations 4–7 (Sousa et al., 2022; Chia et al., 2019).
2.5 Statistical analysis
Before analysis, data was checked for normality using the Shapiro-Wilk normality test. A linear mixed effect model from the package lme4, was used to analyze the number of leaves, leaf area, chlorophyll content, stem diameter, and plant height. During analysis, sampling time and fertilizer treatments were considered fixed effects, while replication was a random effect. The number of flowers, pods, pod length, number of seeds per pod, 100-seed weight, seed yield, haulm yield, N, P and K uptake, and quantity of N fixed were analyzed using one-way analysis of variance. The least-square means were computed using the ‘lsmeans’ package followed by mean separation using Tukey’s honestly significant difference (HSD), which was implemented using “cld” function from the “multicompView” package. To examine the relationship between bean growth and yield parameters, principal component analysis (PCA) was performed using “prcomp” function from “ggbiplot” packages. Data were analyzed separately for each season. The statistical analyses were conducted using R software version 4.3.0 (R Core Team, 2022).
3 Results
3.1 Influence of black soldier fly frass fertilizer and other commercial fertilizers on bush bean growth
3.1.1 Number of leaves and leaf area
The number of leaves significantly varied at different growth stages due to the application of different fertilizer treatments (short rain season: χ2 = 2960.74, df = 14, p< 0.001, long rain season: χ2 = 3032.81, df = 14, p< 0.001) (Table 2). On the other hand, the number of leaves varied significantly at different growth stages during the study (short rain season: χ2 = 31385.07, df = 4, p< 0.001, long rain season: χ2 = 128019.82, df = 4, p< 0.001). The interaction effect between treatments and growth stages on the number of leaves was significant (χ2 = 713.85, df = 4, p< 0.001) during the long rain season only. The number of leaves increased to peak values (24 – 37 during short rains and 36 – 47 leaves during long rains) at the late vegetative stage (56 dap) and decreased afterward. Beans grown in soil amended with sole BSFFF at 45 kg N ha−1 had the highest number of leaves during both seasons, which were 3 – 5%, 3 – 18%, and 28 – 52% higher than the values achieved using sole NPK, equivalent rate of Phymyx, and control, respectively. Sole application of BSFFF at a rate of 45 kg N ha−1 significantly (p< 0.001) increased the number of leaves by 7 – 18% relative to the combined application of rhizobia inoculant and BSFFF at rates of 15 and 30 kg N ha−1.
On the other hand, Phymyx applied alone at a rate of 45 kg N ha−1 resulted in a significant (p< 0.001) increase in the number of leaves as compared to when its rate of 15 kg N ha−1 and rhizobia inoculant were applied in combination. At 56 dap, the number of leaves significantly (p< 0.001) increased in plots treated with sole NPK (23%) and BSFFF rate of 45 kg N ha−1 (15%) compared to plots amended with rhizobia inoculant during the short rain season. However, during the long rain season, plots amended with rhizobia inoculant had an 8% higher (p< 0.001) number of leaves, compared to Phymyx applied at a rate of 15 kg N ha−1, while NPK caused a 9% significant increase compared with rhizobia inoculant.
The leaf area of bush beans varied significantly due to different fertilizer treatments (short rain season: χ2 = 6380.17, df = 14, p = 0.003, long rain season: χ2 = 11315.50, df = 14, p< 0.001), and at different growth stages (short rain season: χ2 = 65014.30, df = 4, p = p< 0.001, long rain season: χ2 = 255280.80, df = 4, p = p< 0.001) (Table 3). The interaction effect of fertilizer treatments and growth stages was not significant (short rain season: χ2 = 22.80, df = 56, p = 0.084, long rain season: χ2 = 10.72, df = 56, p =0.063). The leaf area increased throughout the experiments, to peak values of 37.8 – 52.7 cm2 and 90.7 – 124.9 cm2 during the short and long rain seasons, respectively at 70 dap (Table 3). The highest bean leaf area was achieved using sole BSFFF at a rate of 45 kg N ha−1, which was significantly (p< 0.001) higher than the control by 38%.
3.1.2 Leaf chlorophyll content
The leaf chlorophyll content varied significantly due to different fertilizer treatments (short rain season: χ2 = 1571.57, df = 14, p< 0.001, long rain season: χ2 = 1158.69, df = 14, p< 0.001) (Table 4). Additionally, the growth stages significantly influenced the chlorophyll content of bush beans during the short rain season (χ2 = 908.35, df = 4, p< 0.001) and long rain season (χ2 = 1158.69, df = 4, p< 0.001). The interaction effect between fertilizer treatments and growth stages was significant (short rain season: χ2 = 437.36, df = 56, p< 0.001, long rain season: χ2 = 533.45, df = 56, p< 0.001). Chlorophyll content reached peak values at the late vegetative stage (56 dap) during the short and long rain seasons. Thereafter, the chlorophyll content followed a decreasing trend (Table 4).
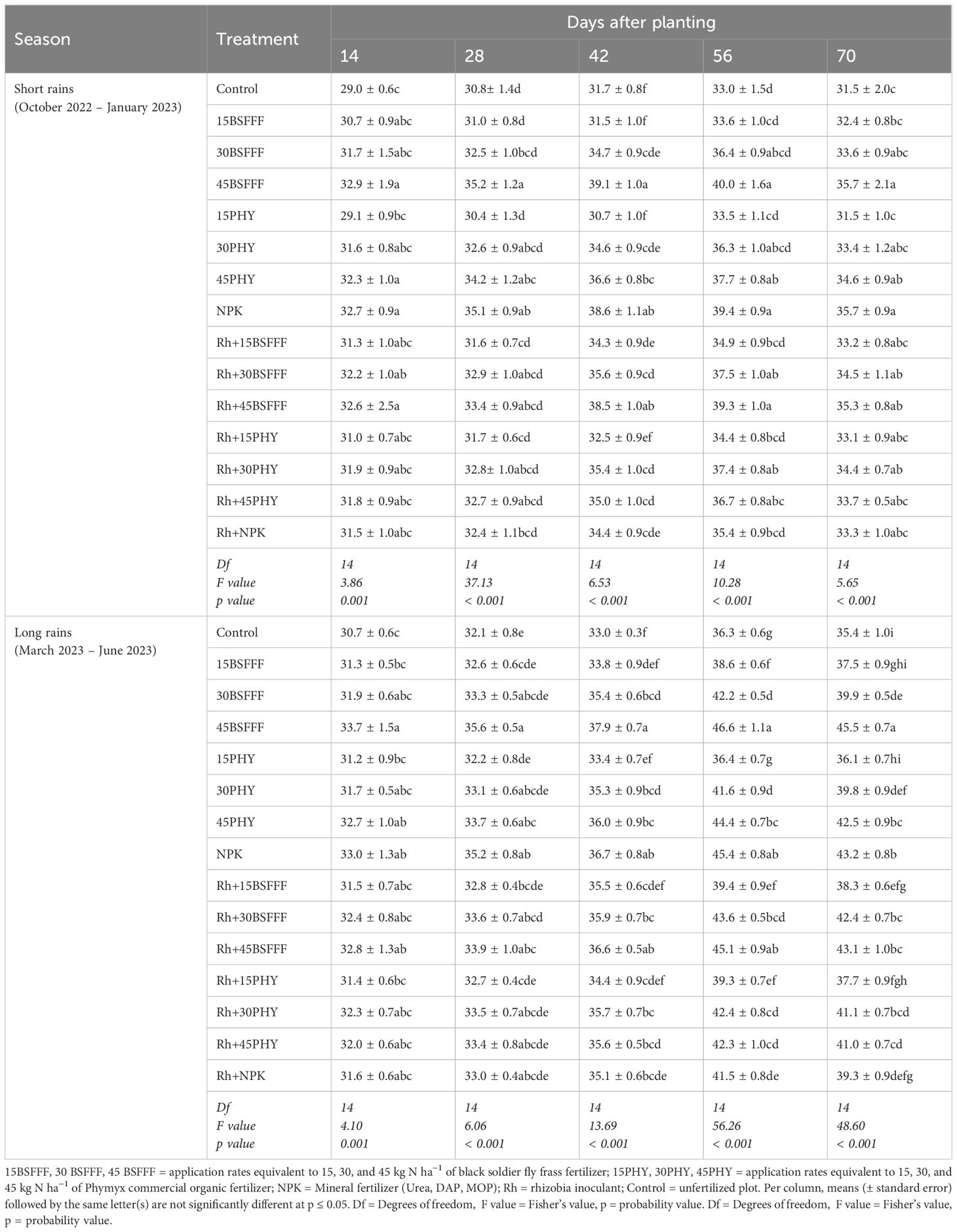
Table 4. Chlorophyll content (SPAD values) of bush beans as influenced by different fertilizer treatments.
Plots treated with sole BSFFF rate of 45 kg N ha−1 produced beans with the highest chlorophyll content, which was significantly (p< 0.001) higher than the values achieved under control and using an equivalent rate of Phymyx by 28 and 5%, respectively. Combined application of rhizobia inoculant and Phymyx at a rate of 15 kg N ha−1 significantly increased chlorophyll by 8% compared to the sole application of Phymyx Also, a combined application of BSFFF rates of 15 and 30 kg N ha−1 and rhizobia increased (p< 0.001) chlorophyll content compared to sole application of BSFFF at the rate of 45 kg N ha−1. Chlorophyll content at 56 dap was significantly (p< 0.001) higher (11%) in plots treated with NPK than those under inoculation during the short rain season. On the other hand, plots amended with rhizobia inoculant recorded (p< 0.001) higher chlorophyll content (8%) than the plots of Phymyx at 15 kg N ha−1, whereas NPK plots performed better (9%) than rhizobia inoculant, during the long rain season.
3.1.3 Plant height
Different fertilizer treatments significantly influenced the heights of bush beans during the short rain season (χ2 = 8152.80, df = 14, p< 0.001) and long rain season (χ2 = 2254.00, df = 14, p< 0.001) (Table 5). The plant height also varied significantly at different growth stages (short rain season: χ2 = 58904.80, df = 4, p< 0.001, long rain season: χ2 = 138910.40, df = 4, p< 0.001). The interaction effect of growth stages and fertilizer treatments was significant during the long rain season only (χ2 = 429.40, df = 56, p< 0.001). There was a sharp rise in plant height between 14 dap and 28 dap during both seasons. Sole application of BSFFF at a rate of 45 kg N ha−1 produced beans with the tallest plants, which were 15% and 6% higher compared to the control and equivalent rate of Phymyx, respectively. For Phymyx, significant (p< 0.001) increases in plant height above that attained in the control were achieved at the rate of 45 kg N ha−1. At 70 dap, bean plants were significantly (p< 0.001) taller in plots under inoculation (13%) compared with those treated with BSFFF at 15 kg N ha−1. Also, an increase in plant height was noted in plots amended with BSFFF at 45 kg N ha−1 (11%) and NPK (19%) compared with those treated with rhizobia inoculant, during the short rain season. During the long rain season (70 dap), a significant (p< 0.001) increase in plant height was only recorded in plots treated with NPK (7%) compared with those amended with rhizobia inoculant.
3.1.4 Stem diameter
Across the seasons, the bean stem diameter varied significantly due to different fertilizer applications (short rain season: χ2 = 420.07, df = 14, p< 0.001, long rain season: χ2 = 269.11, df = 14, p< 0.001) (Table 6). Also, the stem diameter varied significantly (p< 0.001) across the growth stages during the short rain season (χ2 = 343.75, df = 4, p< 0.001) and long rain season (χ2 = 1553.42, df = 4, p< 0.001). The interaction effect between the fertilizer treatments and growth stages was not significant during both seasons (short rain season: χ2 = 13.65, df = 56, p = 0.918, long rain season: χ2 = 25.92, df = 56, p = 0.999).
Stem diameter gradually increased to the highest values at 70 dap (Table 6). Beans that were grown using sole BSFFF at a rate of 45 kg N ha−1 and NPK had 32 and 33% significantly (p< 0.001) higher stem diameters, respectively, compared to the control treatment. Treating soils with NPK resulted in a 15% (p< 0.001) increase in bean stem diameter compared with plots amended with rhizobia inoculant during the short rain season.
3.2 Influence of various fertilizer treatments on nutrient uptake
The different fertilizer treatments significantly influenced the nitrogen (N) uptake of bush beans during the short rain season (χ2 = 68.72, df = 14, p< 0.001) (Figure 2A) and long rain season (χ2 = 36.65, df = 14, p =< 0.001) (Figure 2B). The highest N uptake was observed in bush beans grown using sole BSFFF at a rate of 30 kg N ha−1 during the short rain season (32.4 kg N ha−1) and long rain season (95.4 kg N ha−1), which were 217% (short rain season) and 172% (long rain season) significantly (p< 0.001) higher than the N accumulated by bush beans in the control plots (Figure 2B). Additionally, bush beans grown in plots that had sole BSFFF applied at a rate of 30 kg N ha−1 accumulated a significantly (p< 0.001) higher N compared to sole application of either Phymyx or BSFFF at a rate of 15 kg N ha−1 during the short rain season. During the short rain season, sole BSFFF applied at a rate of 30 kg N ha−1 caused significantly (p< 0.001) higher N uptake compared to combined application of rhizobia inoculant and Phymyx at a rate of 15 kg N ha−1.
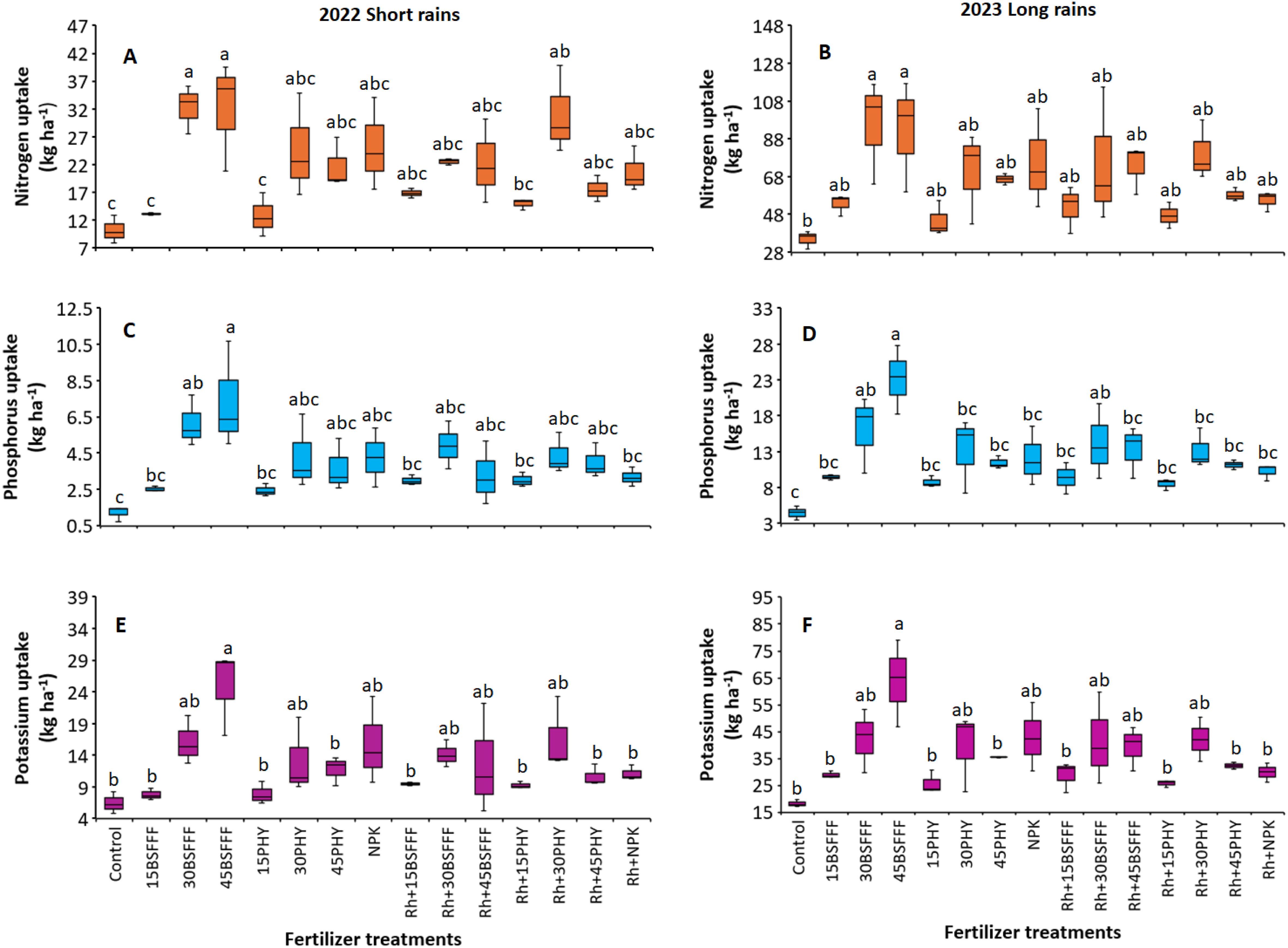
Figure 2. Nitrogen uptake (A, B), Phosphorus uptake (C, D) and potassium uptake (E, F) of bush beans as influenced by different fertilizer treatments during the short rains and long rains seasons.15BSFFF, 30 BSFFF, 45 BFFF = application rates equivalent to 15, 30, and 45 kg N ha−1 of black soldier fly frass fertilizer; 15PHY, 30PHY, 45PHY = application rates equivalent to 15, 30, and 45 kg N ha−1 of Phymyx commercial organic fertilizer; NPK = Mineral fertilizer (Urea, DAP, MOP); Rh = rhizobia inoculant; Control = unfertilized plot. Per panel, means (± standard error) followed by the same letter(s) are not significantly different at p ≤ 0.05.
The P uptake of bush beans was also significantly influenced by the different fertilizer treatments (short rain season: χ2 = 52.89, df = 14, p< 0.001 (Figure 2C), long rain season: χ2 = 72.11, df = 14, p< 0.001) (Figure 2D). The highest P uptake was achieved in beans grown using sole BSFFF supplied at 45 kg N ha-1 during the short rain season and long rain season, and these were significantly (p< 0.001) higher than the value achieved using the control by 3 – 9 folds. Without inoculation, the application rate of 45 kg N ha-1 supplied as BSFFF caused 74% significantly (p< 0.001) higher P uptake compared to when rhizobia inoculant was combined with the treatment during the long rain season. Furthermore, the application of BSFFF singly at the rate of 45 kg N ha-1 boosted P uptake by 91% and 102% (p< 0.001) compared to sole NPK and the equivalent rate of sole Phymyx, respectively, during the long rain season. On the other hand, the application of sole BSFFF at a rate of 45 kg N ha-1 caused significantly (p< 0.001) higher P uptake compared to NPK applied in combination with rhizobia inoculant (131%), and Phymyx and BSFFF applied at a rate of 15 kg N ha-1 with or without rhizobia inoculant during the short rain season. There was a 74% (p< 0.001) increase in P uptake in plots amended with a BSFFF rate of 45 kg N ha-1 compared with plots treated with rhizobia inoculant during the long rain season.
There were significant variations in K uptake of bush beans due to different fertilizer treatments during the study (short rain season: χ2 = 50.17, df = 14, p< 0.001, long rain season: χ2 = 57.28, df = 14, p< 0.001) (Figures 2E, F). The highest K uptake was achieved using sole BSFFF at 45 kg N ha-1, and this was 250 – 287%, 79 – 112%, 114 – 123%, and 79 – 133% higher compared to the values obtained using the control, equivalent rate of Phymyx, combined application of NPK and rhizobia inoculant, and equivalent rate of Phymyx combined with rhizobia inoculant, respectively. Additionally, the sole application of BSFFF at a rate of 45 kg N ha-1 significantly enhanced K uptake relative to Phymyx and BSFFF applied at 15 kg N ha-1.
3.3 Biological nitrogen fixation of bush beans grown using BSF frass fertilizer and other commercial fertilizers
3.3.1 Total and effective root nodules
The different fertilizer treatments caused significant variations in the total number of root nodules during the short rain season (F = 16.87, df = 14, p< 0.001) and long rain season (F = 31.61, df = 14, p< 0.001) (Table 7). Plots treated with sole BSFFF at a rate of 30 kg N ha−1 produced beans with the highest number of root nodules (30 – 36), which were 218% – 276%, 80 – 108%, 44 – 97%, and 36 – 71% higher compared to the control treatment, equivalent rate of Phymyx, sole NPK and combined application of NPK and rhizobia, respectively. The total number of root nodules achieved using sole BSFFF at a rate of 30 kg N ha−1 was 102 – 130% higher (p<0.001) compared to the integrated use of the fertilizer with rhizobia inoculant. On the contrary, combined application of rhizobia inoculant and Phymyx at a rate of 30 kg N ha−1 caused a significant (p< 0.001) increase in the number of root nodules (73 – 77%) compared to sole application of the same Phymyx treatment. The total number of root nodules (p< 0.001) increased due to the application of rhizobia inoculant compared to when Phymyx at 30 kg N ha−1 was applied during the short (77%) and long (73%) rain seasons. On the other hand, the BSFFF rate of 45 kg N ha−1 application led to a 56% significant (p< 0.001) increase in the total number of nodules compared with plots treated with rhizobia inoculant, during the long rain season.
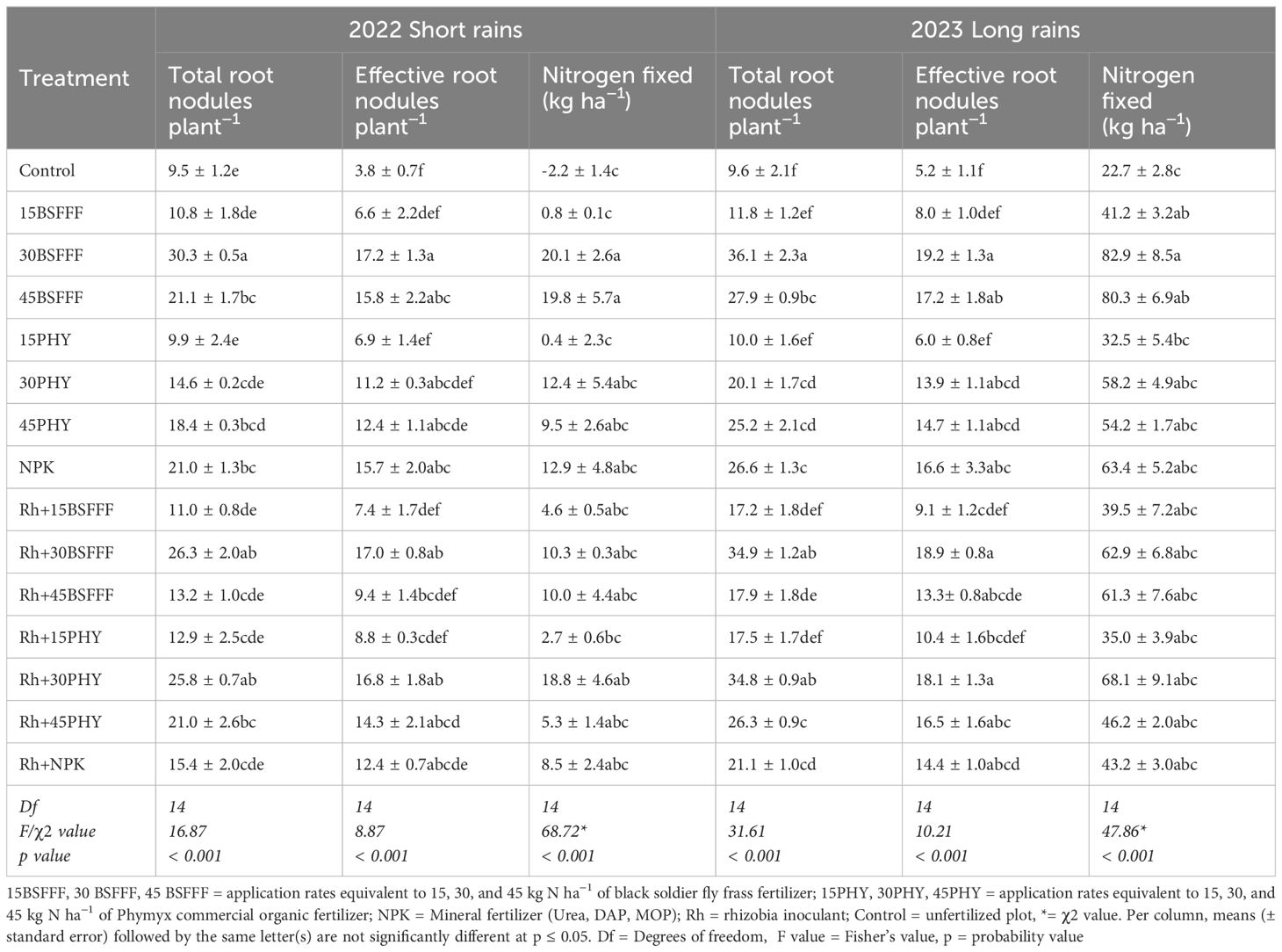
Table 7. Total and effective root nodules, and amount of nitrogen fixed by bush beans grown in soil amended with different fertilizer treatments.
The number of effective root nodules also varied significantly due to different fertilizer treatments (short rain season: F=8.87, df = 14, p< 0.001, long rain season: F=10.21, df = 14, p< 0.001) (Table 7). Plots amended with sole BSFFF at a rate of 30 kg N ha-1 produced beans with the highest number of effective root nodules (short rain season: 17 nodules, long rain season: 19 nodules), which were 13 – 14-fold, 84, 95 – 161% and 85 – 218% (p< 0.001) higher compared to those grown using the control treatment, BSFFF at a rate of 30 kg N ha-1 in combination with rhizobia, Phymyx at a rate of 15 kg N ha-1 and BSFFF applied at a rate of 15 kg N ha-1 either singly or in combination with rhizobia, respectively.
3.3.2 Amount of nitrogen fixed
Across the two seasons, the different fertilizer treatments significantly influenced the amount of N fixed by bush beans (short rain season: χ2 = 68.72, df = 14, p< 0.001, long rain season: χ2 = 38.65, df = 14, p< 0.001) (Table 7). The highest quantity of N fixed by bush beans was achieved in beans grown using sole BSFFF at a rate of 30 kg N ha-1, which was 265% (p< 0.001) higher than N fixed in the control plots across the two seasons. For plots treated with sole BSFFF, significant differences in the quantity of N fixed were attained at rates above 15 kg ha−1. For Phymyx, significant (p< 0.001) differences were only achieved when the rate of 30 kg ha−1 was combined with rhizobia inoculant during the short rain season. Across treatments, the quantity of N fixed was higher during the long rain season compared to the values achieved during short rain season.
3.4 Influence of black soldier fly frass fertilizer and commercial fertilizers on bush bean reproduction and yield
3.4.1 Number of flowers and pods per plant
The different fertilizer treatments caused significant differences in the number of flowers during the study (short rain season: F = 4.22, df = 14, p< 0.001, long rain season: F = 7.30, df = 14, p< 0.001) (Figures 3A, B). Beans grown in soil amended with either BSFFF or Phymyx applied at sole rates of 30 and 45 kg N ha−1 significantly (p< 0.001) recorded higher number of flowers by 27 – 50% (BSFFF) and 20 – 39% (Phymyx) than the crops grown in unamended soil. The highest number of flowers was recorded in plots treated with sole BSFFF at a rate of 45 kg N ha−1, which was significantly (p< 0.001) higher than equivalent rates of 15 kg N ha−1 of sole Phymyx (28%) and BSFFF (32%), and combined application of rhizobia inoculant and 15 kg N ha−1 of either BSFFF (20%) or Phymyx (21%).
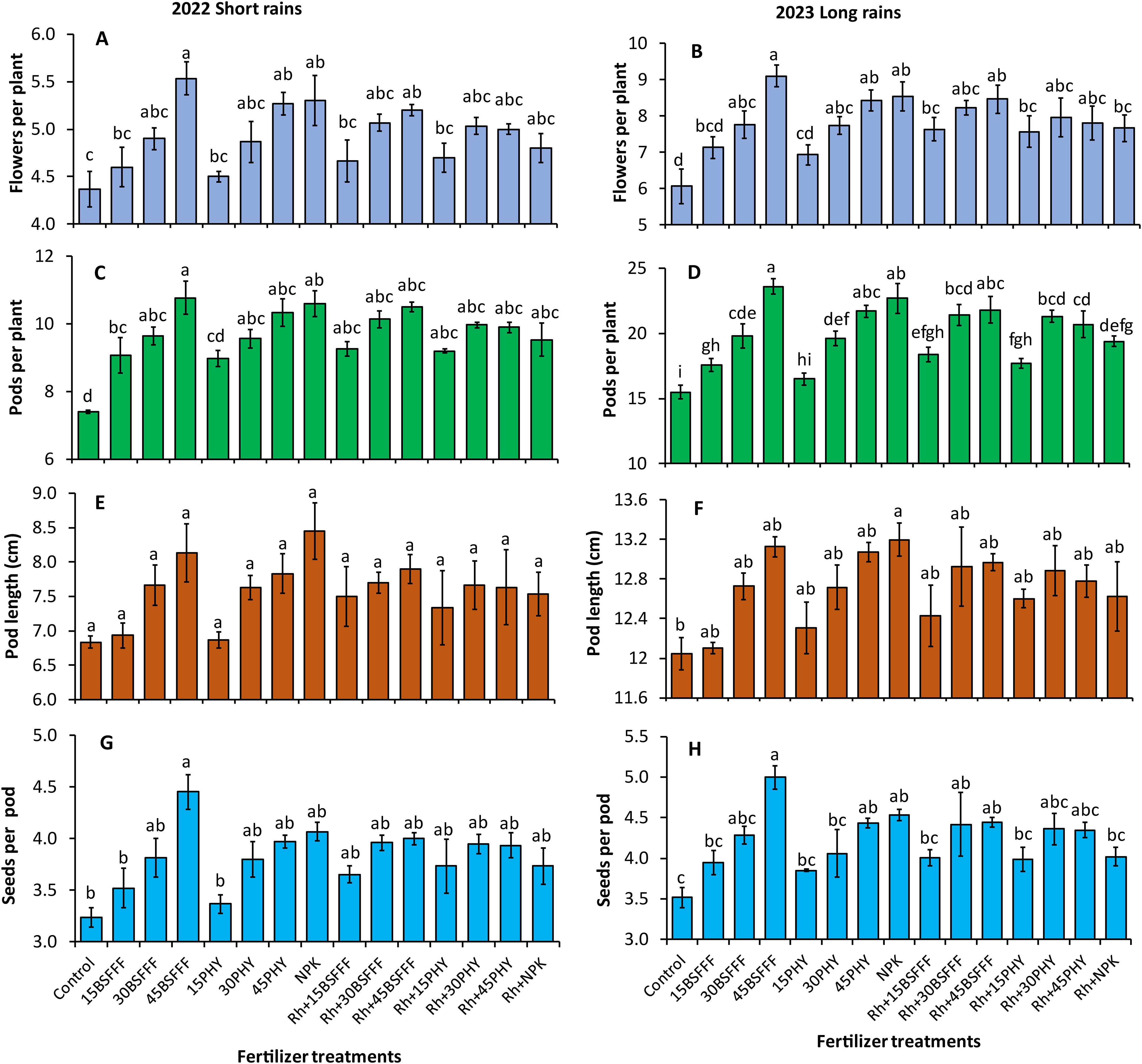
Figure 3. Number of flowers (A, B), number of pods (C, D), pod length (E, F), and number of seeds per pod (G, H) of bush beans as influenced by different fertilizer treatments during the short rains and long rains seasons. 15BSFFF, 30 BSFFF, 45 BSFFF = application rates equivalent to 15, 30, and 45 kg N ha−1 of black soldier fly frass fertilizer; 15PHY, 30PHY, 45PHY = application rates equivalent to 15, 30, and 45 kg N ha−1 of Phymyx commercial organic fertilizer; NPK = Mineral fertilizer (Urea, DAP, MOP); Rh = rhizobia inoculant; Control = unfertilized plot. Per panel, means (± standard error) followed by the same letter(s) are not significantly different at p ≤ 0.05.
The number of bush bean pods was significantly influenced by different fertilizer treatments during the study (short rain season: F = 7.50, df = 14, p< 0.001, long rain season: F = 36.02, df = 14, p< 0.001) (Figures 3C, D). Bush beans grown in soil amended with sole BSFFF treatments achieved a 14–52% (p< 0.001) higher number of pods compared with beans grown in the control plots (Figures 3C, D). For Phymyx, only amendment at sole application rates of 30 and 45 kg N ha−1 produced beans with a significantly (p< 0.001) higher number of pods compared to the control plots during the short rain season. The highest number of pods was produced by beans grown in plots treated with sole BSFFF at a rate of 45 kg N ha−1, and this was higher (p< 0.001) than the values achieved using a combination of rhizobia inoculant with all Phymyx treatments (11 – 33%), NPK (22%), BSFFF rate of 15 N ha−1 (28%) and BSFFF rate of 30 kg N ha−1 (10%) (p< 0.001).
3.4.2 Pod length and number of seeds per pod
The different fertilizer treatments significantly affected bean pod lengths during the long rain season only (short rain season: F=1.81, df = 14, P= 0.085, long rain season: F=2.71, df = 14, p = 0.0107) (Figures 3E, F). A significant (p< 0.001) increase in the pod sizes (10%) was realized in plots treated with sole NPK compared with the control.
The number of seeds per pod also varied significantly during the study (short rain season: F=2.84, df = 14, p = 0.008, long rain season: F=4.53, df = 14, p< 0.001) (Figures 3G, H). Beans grown in plots treated with sole BSFFF applied at a rate of 45 kg N ha−1 produced significantly (p< 0.001) higher number of seeds per pod compared to those grown in unamended soil (38 – 42%), plots amended with a combination of NPK and rhizobia inoculant (24%) during the long rain season, sole Phymyx or BSFFF applied at 15 kg N ha−1 during the short rain season, and Phymyx or BSFFF at a rate of 15 kg N ha−1 applied in combination with rhizobia inoculant during the long rain season.
3.4.3 One hundred seeds weight
There was a significant influence of the fertilizer treatments on the 100-seed weight of bush beans during the short rain season (F=80.10, df = 14, p< 0.001) (Figure 4A) and long rain season (F=14.13, df = 14, p< 0.001) (Figure 4B). The heaviest seeds were recorded in plots amended with sole BSFFF at a rate of 45 kg N ha−1, which were 68 and 18% significantly (p< 0.001) higher than the control during the short and long rain seasons, respectively. Also, Phymyx applied at 45 kg N ha−1 significantly (p< 0.001) increased 100-seed weight over the control plots.
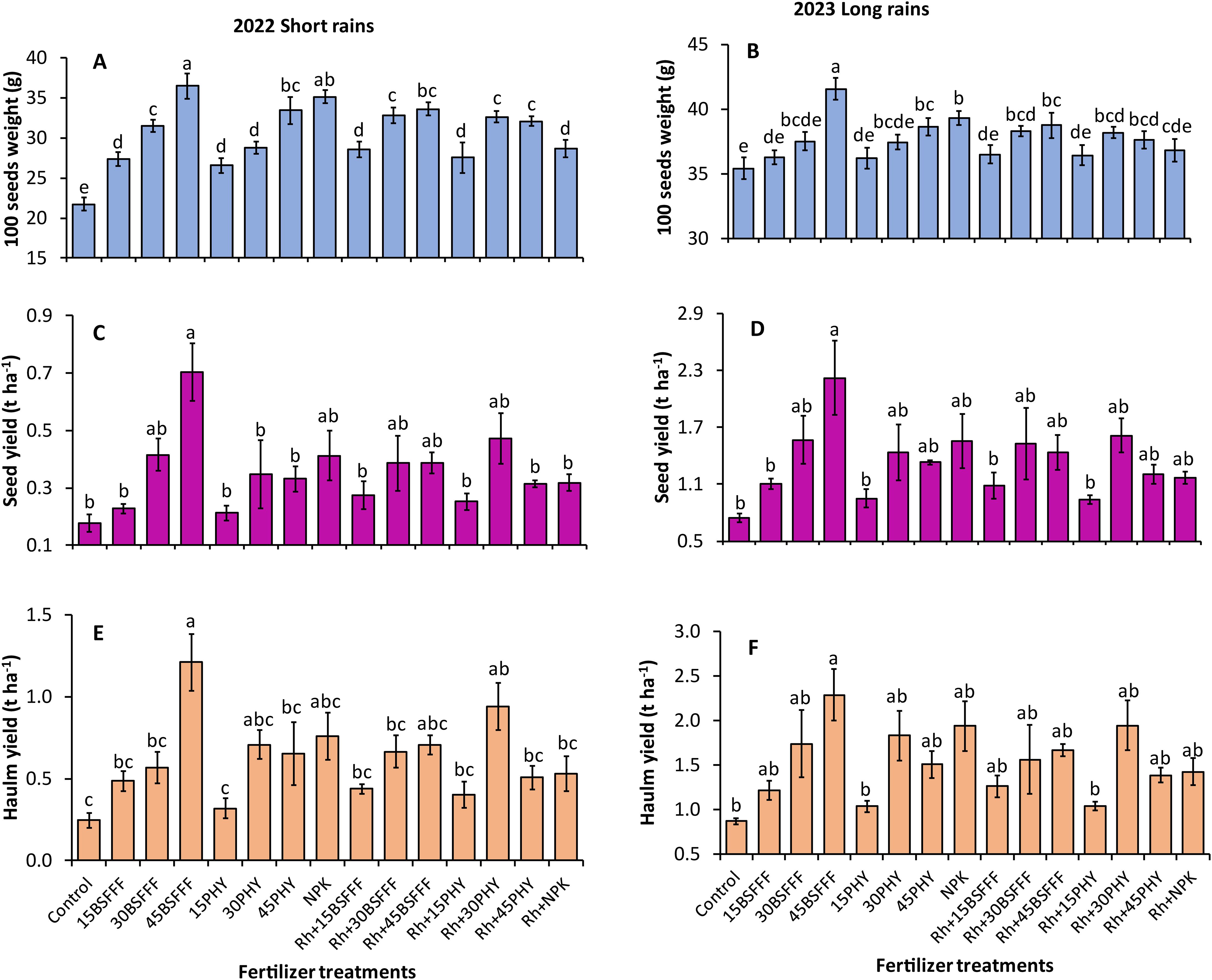
Figure 4. 100 seed weight (A, B), seed yield (C, D), and haulm yield (E, F) of bush beans as influenced by different fertilizer treatments during the short rains and long rains seasons. 15BSFFF, 30 BSFFF, 45 BSFFF = application rates equivalent to 15, 30, and 45 kg N ha−1 of black soldier fly frass fertilizer; 15PHY, 30PHY, 45PHY = application rates equivalent to 15, 30, and 45 kg N ha−1 of Phymyx commercial organic fertilizer; NPK = Mineral fertilizer (Urea, DAP, MOP); Rh = rhizobia inoculant; Control = unfertilized plot. Per panel, mean (± standard error) followed by the same letter(s) are not significantly different at p ≤ 0.05.
The 100 seed weight achieved using sole BSFFF applied at 45 kg N ha−1 was significantly (p< 0.001) higher than the value achieved using an equivalent rate of Phymyx during the short rain season (9%), BSFFF at a rate of 45 kg N ha−1 combined with rhizobia inoculant (7 – 9%) and sole NPK (6%). It was noted that the combination of rhizobia inoculant with Phymyx at a rate of 30 kg N ha−1 significantly enhanced the 100 seed weight compared to the equivalent sole rate of Phymyx during the short rain season. There was a 9% (short rain season) and 7% (short rain season) (p< 0.001) increase in 100 seed weight in plots treated with a BSFFF rate of 45 kg N ha−1 compared with plots amended with rhizobia inoculant. Also, the application of NPK resulted in 22 and 7% significant (p< 0.001) increase in the 100 seed weight, compared with plots of rhizobia inoculant during the short and long rain seasons, respectively. Additionally, plots amended with rhizobia inoculant (p< 0.001) outperformed those treated with a Phymyx rate of 30 kg N ha−1 by 13% during the short rain season.
3.4.4 Seed and haulm yield
There were significant differences in the seed yield of bush beans grown using different fertilizer treatments in the study (short rain season: χ2 = 58.45, df = 14, p< 0.001, long rain season: χ2 = 43.58, df = 14, p< 0.001) (Figures 4C, D). Application of sole BSFFF at a rate of 45 kg N ha-1 produced the highest bush bean yield during the short rain season (0.7 t ha-1) and long rain season (2.2 t ha-1), which were significantly (p< 0.001) higher than the values achieved using unfertilized soil by 199 – 250%. During the short rain season, the sole application of BSFFF at a rate of 45 kg N ha−1 produced higher (p< 0.001) bean seed yield compared to the equivalent rate of Phymyx (113%), a combination of BSFFF applied at 45 kg N ha-1 and rhizobia inoculant and NPK and rhizobia inoculant (123%) during the short rain season. Also, the bush bean seed yield achieved using sole BSFFF at a rate of 45 kg N ha-1 was significantly (p< 0.001) higher than the yields achieved using Phymyx (157 – 231%) and BSFFF (101 – 136%) applied at rates of 15 kg N ha-1 alone or in combination with rhizobia inoculant. The application of the BSFFF rate of 45 kg N ha-1 caused a 102% significant increase in seed yield compared with plots treated with rhizobia inoculant during the short rain season.
The different fertilizer treatments also caused significant differences in the haulm yield of bush beans (short rain season: χ2 = 77.48, df = 14, p< 0.001 (Figure 4E), long rain season: χ2 = 46.58, df = 14, p< 0.001) (Figure 4F). The haulm yield achieved using sole BSFFF applied at a rate of 45 kg N ha−1 was significantly higher than the yields obtained in the control plot (163 – 389%), equivalent rate of Phymyx in sole or combination with rhizobia (113 – 139%), rhizobia inoculant (82%) and NPK combined with rhizobia inoculant (129%) during the short rain season. Likewise, the haulm yield achieved using sole BSFFF at a rate of 45 kg N ha−1 was 120 – 121% higher than the values obtained using Phymyx at a rate of 15 kg N ha−1 either applied singly or in combination with rhizobia inoculant during the long rain season. For Phymyx, a significant (p< 0.001) increase in haulm yield relative to the control was only attained when the fertilizer was applied at 30 kg N ha−1 in combination with rhizobia inoculant during the short rain season. There was an 82% (p< 0.001) increase in haulm yield in plots treated with 45 kg N ha−1 of BSFFF compared with plots amended with rhizobia inoculant during the short rain season.
3.5 Multivariate analysis of relationships between bean growth and yield parameters as influenced by different fertilizer treatments
The principal component analysis (PCA) revealed that the different fertilizer treatments significantly influenced bush bean growth and yield parameters (Figure 5). During the short rain season (Figure 5A), the first two components of the explained for 94.6% of the total variance. The first principal component (PC1) accounted for 89.7% while PC2 accounted for 4.9% of the total variance. The number of bean seeds per pod, number of pods, leaf area, and stem diameter positively correlated with both seed and haulm yields. During the long rain season (Figure 5B), the first two components represented 98.5% of the total variance. In this case, PC1 explained 89.4%, whereas PC2 explained 9.1%. Bean leaf area, plant height, and chlorophyll content were positively correlated with seed yield. On the other hand, most growth parameters, such as the stem diameter, number of leaves, pod length and number of pods positively correlated with haulm yield. The number of leaves, number of flowers, pod length, and number of pods, number, 100 seed weight, chlorophyll and plant height were positively correlated during both seasons.
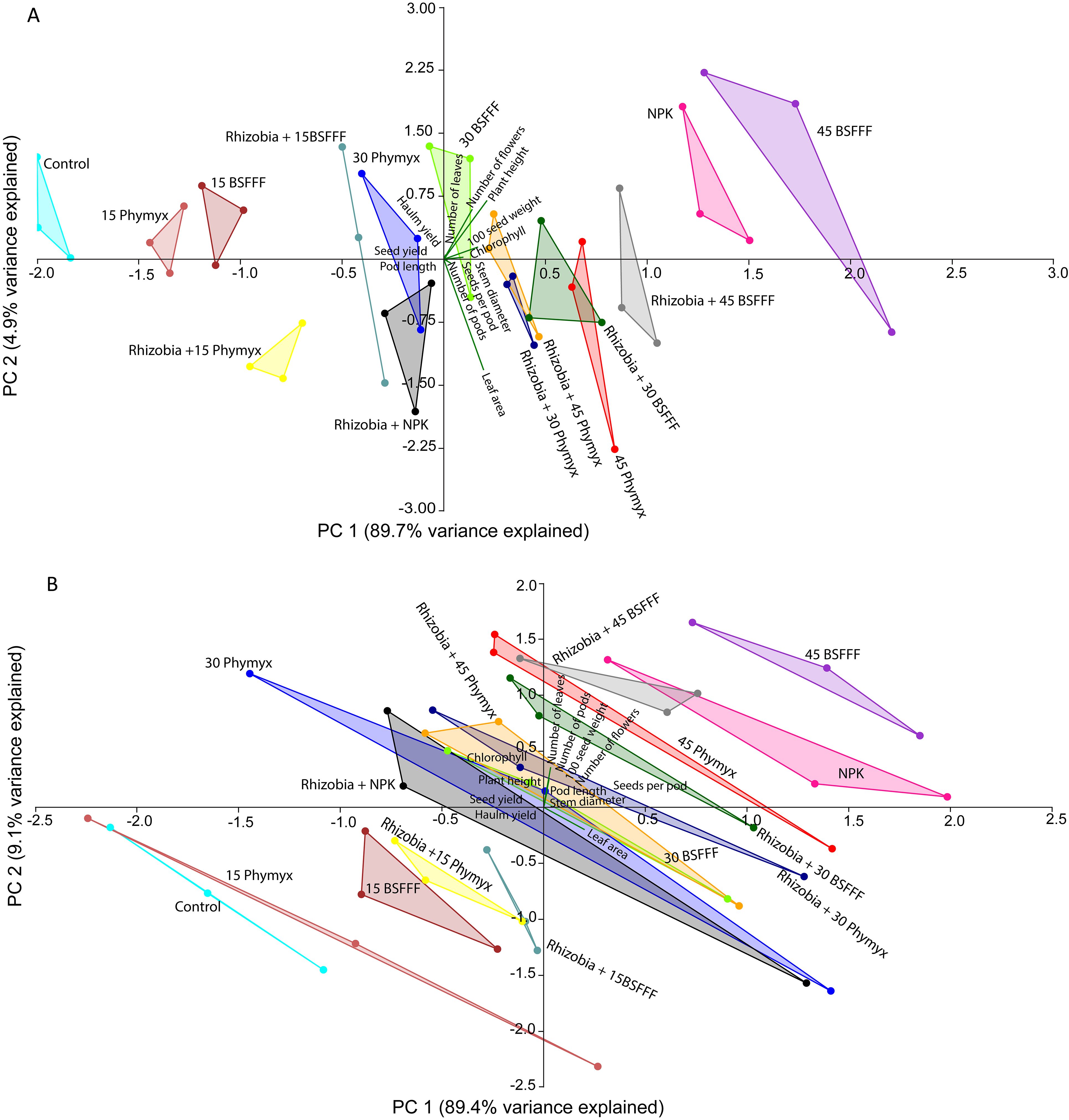
Figure 5. Biplots showing the relationship between bush bean growth and yield parameters for the first two principal components (PC1 and PC2) as influenced by different fertilizer treatments during short rain (A) and long rain (B) cropping seasons. 15BSFFF, 30 BSFFF, 45 BSFFF = application rates equivalent to 15, 30, and 45 kg N ha−1 of black soldier fly frass fertilizer; 15PHY, 30PHY, 45PHY = application rates equivalent to 15, 30, and 45 kg N ha−1 of Phymyx commercial organic fertilizer; NPK = Mineral fertilizer (Urea, DAP, MOP); Rh = rhizobia inoculant; Control = unfertilized plot.
3.6 Profitability of bush bean production using various fertilizer treatments
3.6.1 Net income and gross margin
The long rain season was more rewarding economically than the short rain season (Table 8). Plots amended with sole NPK generated the highest net income during the long rain season, which was more than its combined application with rhizobia inoculant. Without inoculation, bush beans grown using BSFFF at rates of 15, 30, and 45 kg N ha−1 generated 73 – 239% higher net income compared with equivalent rates of Phymyx. With inoculation, plots treated with BSFFF generated 141– 817% more net income only at application rates of 15 and 45 kg N ha−1 compared with equivalent rates of Phymyx, however at a rate of 30 kg N ha−1 Phymyx recorded 6% more values than the equivalent rate of BSFFF. Under inoculation, BSFFF at a rate of 45 kg N ha−1 yielded more net income (124%) than its combined application with rhizobia inoculant. Phymyx applied at a rate of 30 kg N ha−1 with rhizobia inoculant recorded a relatively higher net income (13%) than without inoculation. Additionally, BSFFF at a rate of 45 kg N ha−1 applied alone resulted in 32% more net income than when NPK was applied with rhizobia inoculant.
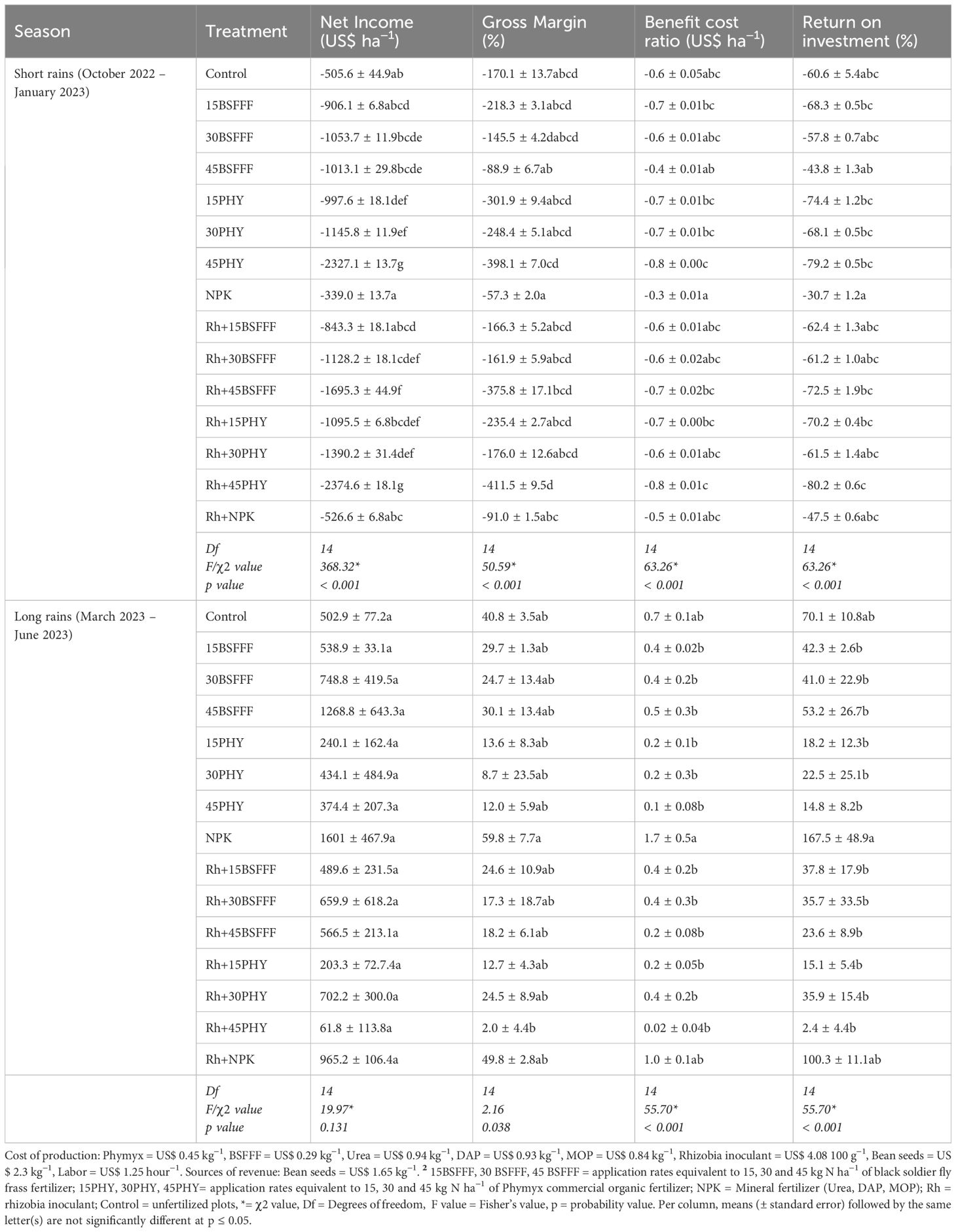
Table 8. Economic indices (net income, gross margin, benefit cost ratio and return on investment) of bush beans production as influenced by different fertilizer treatments.
The highest gross margin was recorded in plots treated with sole NPK, which was 20% more than values generated from its combined application with rhizobia inoculant (Table 8). The gross margin achieved using sole BSFFF at a rate of 45 kg N ha−1 was 65% and 150% higher compared to when the same fertilizer was combined with rhizobia inoculant and the value achieved using similar rate of Phymyx, respectively. Combined application of Phymyx at 30 kg N ha−1 with rhizobia inoculant yielded 181% higher gross margin compared to its sole application.
3.6.2 Benefit-cost ratio and return on investment
Bush beans grown using sole NPK yielded the highest benefit-cost ratio (1.7) which was 67% higher than the inoculated ones (Table 8). Plots amended with sole BSFFF at a rate of 45 kg N ha−1 generated 253% more benefit-cost ratio compared with the ones that had received an equivalent rate of Phymyx. In addition, when BSFFF at a rate of 45 kg N ha−1 was applied singly, the benefit-cost ratio increased by 121% more than its combined application with rhizobia inoculant.
Plots amended with sole NPK generated the highest return on investment (ROI), which was 67% more than its combined application with rhizobia inoculant. Plots treated with sole NPK yielded the highest ROI that was significantly (p< 0.001) higher than the values achieved using BSFFF (3 – 7-fold) and Phymyx (4.7 – 70-fold) treatments. The sole BSFFF treatments yielded 82 – 260% higher ROI compared to equivalent rates of Phymyx. There was a decline in ROI as the application rates of BSFFF and Phymyx increased, but the decreases were not significant (Table 8).
4 Discussion
4.1 Influence of black soldier fly frass fertilizer and commercial fertilizers on bush bean growth and yield
The higher growth rate and yield of bush beans grown in fertilized soil compared to the unfertilized soil indicate the significant role of fertilizers in boosting crop production in degraded soils which dominate farmlands across Africa and the tropics (Wortmann et al., 2019; Nungula et al., 2023). As demonstrated, BSFFF improved the growth of bush beans better than commercial fertilizers, which is in tandem with previous studies that have shown the benefits of insect frass fertilizers in boosting the growth of different crops (Anyega et al., 2021; Beesigamukama et al., 2020a). This could be attributed to a faster release of nutrients such as nitrogen and phosphorus for plant uptake, which are critical for physiological processes such as chlorophyll formation (Mulambula et al., 2019; Xiang et al., 2012). Past studies have reported taller plants and higher chlorophyll content in crops grown using BSFFF, compared to commercial fertilizers (Klammsteiner et al., 2020; Quilliam et al., 2020). The comparable performance of frass fertilizer and mineral fertilizer in terms of number of leaves and stem diameter has been previously reported (Klammsteiner et al., 2020) and illustrates that frass fertilizer can be a sustainable alternative to mineral fertilizers. Also, the growth hormones present in BSFFF and the ability to suppress plant pests (Anedo et al., 2024; Kisaakye et al., 2024; Tanga and Kababu, 2023) and diseases (Kemboi et al., 2022; Lagat et al., 2021) could have mostly contributed to improved growth of bush beans observed during the study.
On the other hand, the combined application of the organic fertilizers and rhizobia inoculant did not significantly affect the growth of beans compared to the sole application of BSFFF at a rate of 45 kg N ha−1. This could mostly be due to underlying effects such as incompatibility of the bio-fertilizer with the organic fertilizers. The rhizobia inoculant could have also faced competition from the indigenous rhizobacteria, which counteracted the effect on the growth of bush beans (Zaheer et al., 2022). Bio-fertilizer performance is also affected by dynamics in soil conditions such as temperature, salinity, pH, and moisture (Zaheer et al., 2022; Mwadalu et al., 2022; Mohammadi et al., 2012). It is further reported that bush beans can be inoculated by more than one species of rhizobia (Sousa et al., 2022; Cordeiro et al., 2017; Gicharu et al., 2013), therefore, limiting growth through negative interaction when elite strains of rhizobia were introduced. This, therefore, illustrates the inefficiency of elite strains of rhizobacteria on the growth of bush beans in addition to being host-specific, thus limiting their effectiveness in bush bean production.
Our findings revealed positive correlations between bean growth parameters and seed yield. The highest number of flowers, pods, and seeds per pod achieved by beans grown in soils amended with sole BSFFF treatment at a rate of 45 kg N ha−1 could be attributed to the ability of insect frass fertilizer to promote flower formation and pollination (Barragán-Fonseca et al., 2022). Additionally, the higher number of pods could be attributed to the higher chlorophyll content due to optimal release of N and P, and a resultant transfer of photosynthates for pod and seed formation (Goswami et al., 2019). The higher K and P concentrations, as well as micronutrients in BSFFF compared with commercial organic fertilizer, further explains the better flower and seed reproduction in beans grown using BSFFF compared to the commercial organic fertilizer (Phymyx) (Kakon et al., 2016; Dhanjal et al., 2003).
Bush beans grown during the long rain season accumulated more nutrients compared to their counterparts in the short rain season, due to favorable rainfall that enhanced faster release and uptake of nutrients by bush bean plants. This aligns with previous studies that demonstrated higher nutrient uptake of chickpeas under a sufficient irrigation regime than the water-stressed soils (Chtouki et al., 2022). This study revealed the benefits of using sole BSFFF for sufficient nutrient uptake, clearly evidenced by the higher macro nutrient uptake compared with those grown using commercial fertilizers. It is well documented that the novel insect frass fertilizer improves soil health in terms of pH, and nutrient availability, and even curbs the challenge of moisture stress (Beesigamukama et al., 2020b; Adin Yéton et al., 2019; Kagata and Ohgushi, 2012). Previous studies further found that the fertilizer is rich in beneficial microorganisms, higher carbon levels, and mineralization rate to enhance faster release and uptake of nutrients compared to commercial fertilizers (Agustiyani et al., 2021; Beesigamukama et al., 2020a, b; Gold et al., 2020). The higher nutrient uptake in beans grown using sole BSFFF compared with commercial fertilizers, could be due to higher BNF success and better root formation linked to P availability within the rhizosphere (Beesigamukama et al., 2021b; Xiang et al., 2012). This could have contributed to higher bush bean yield from BSFFF-amended plots compared to those fertilized using commercial fertilizers (Devi et al., 2012), and aligns with previous studies that reported increased yield of crops such as maize, tomatoes, and French beans using BSF frass fertilizer (Anyega et al., 2021; Beesigamukama et al., 2020a). Therefore, the highest bean yield associated with sole BSFFF at a rate of 45 kg N ha−1 implies that frass fertilizer can be relied on as a substitute for commercial fertilizers for improved bush bean yield.
Our study endorses the optimal application of sole BSFFF at a rate of 45 kg N ha−1 to be adopted in bush bean cropping systems to attain a yield potential of 1.5 – 2 tonnes ha-1 (Mukankusi et al., 2019; Kalyebara and Buruchara, 2008). Even though the combined application of rhizobia inoculant and BSFFF did not increase bush bean yield better than a sole dose of BSFFF, it was noted that the integrated application of 30 kg N ha−1 BSFFF with rhizobia inoculant provides the starter dose required for BNF process and boosts the crop yield. Therefore, stakeholders along the bush bean value chain can adopt either sole BSFFF at a rate of 45 kg N ha−1 or use a lower rate of 30 kg N ha−1 combined with rhizobia inoculant to reduce reliance on expensive and unsustainable commercial fertilizers. This will go a long way in boosting bean productivity and improving food and nutrition security, especially among smallholder farmers. It is crucial to note that for best results, BSFFF should be accompanied by other agronomic practices such as timely planting, plant spacing, weed control, pest, and disease control as well as timely harvesting to eliminate cases of yield loss.
4.2 Biological nitrogen fixation and economic returns to bush bean production using BSF frass fertilizer and commercial fertilizers
The higher number of effective root nodules and quantity of N fixed achieved by bush beans grown in soil amended using sole BSFFF compared to commercial rhizobia inoculant and organic fertilizers highlight the superiority of BSFFF in boosting BNF and improving nitrogen cycling in legume cropping systems. The better nodulation and N fixation associated with BSFFF treatment could be largely attributed to the positive role of BSFFF in creating conducive soil conditions for the BNF process. The high pH values of BSFFF could have reduced soil acidity which unlocked P making it available for uptake and availed calcium for the formation of nod factors necessary for the attachment of rhizobia to root surfaces, as previously reported by Beesigamukama et al. (2021b). Studies have found that nodules are greater sinks for P compared to shoots and roots of legumes (Mulambula et al., 2019; Shahid et al., 2009) due to the critical role of phosphorus in the formation of energy required for effective nodulation and BNF among pulse crops (Agustiyani et al., 2021). The BSFFF also supplies micronutrients such as molybdenum (Mo) and iron (Fe) (Beesigamukama et al., 2023) which are required for nodulation and BNF success (Nasar et al., 2021; Hänsch and Mendel 2009). Further, the BSFFF is rich in ammonium (NH4+) (Beesigamukama et al., 2021b), which is highly preferred by microorganisms as an energy source during the BNF process. Conversely, the lower nitrate (NO3-1) concentration in plots of sole BSFFF could have enhanced BNF more than commercial fertilizers since high NO3-1 is toxic to BNF, as it subdues the expression of nitrogenase activities (Reinprecht et al., 2020; Mingotte et al., 2019; Faghire et al., 2011).
Past studies have shown the greater populations of beneficial microbiota such as nitrifying bacteria contained in the BSFFF (Gold et al., 2020; Surendra et al., 2020; Choi and Hassanzadeh, 2019), which could have played a key role in ensuring effective nodulation and BNF, compared to commercial fertilizers. Beyond nutrients supply, the insect frass fertilizer also improves other soil properties which are key for BNF success (Beesigamukama et al., 2023; Poveda et al., 2019). Improved water-holding capacity, tolerance to moisture stress, proper soil aeration, and soil aggregation have been reported in soils amended with BSFFF and could have enhanced the activities of native and active rhizobia for improved nodulation and BNF process (Beesigamukama et al., 2020b; Pagliai et al., 2004). Studies by Abiya et al. (2022) have demonstrated the benefits of BSFFF in boosting tolerance to moisture stress, while Beesigamukama et al. (2020b) reported higher moisture storage in soils amended with BSFFF. Additionally, the BSFFF is known to suppress soil-borne pathogens that cause diseases in different crops, including beans (Kemboi et al., 2022; Lagat et al., 2021; Quilliam et al., 2020). Our sister studies have demonstrated the high potential of BSFFF in suppressing soil-dwelling pests such as nematodes root maggots (Anedo et al., 2024; Kisaakye et al., 2024; Tanga and Kababu, 2023), and an array of above ground pests (Abiya et al., 2022), thus protecting and ensuring the health of bean crops for high BNF success. Nonetheless, future studies will be necessary to validate the soil health benefits of BSFFF, survival of rhizobia in soil, carbon sequestration, and greenhouse gas mitigation in bean cropping systems.
The lower number of effective nodules and lower N fixation associated with rhizobia inoculant could be largely attributed to low populations of effective strains, incompatibility, competition from the native nitrifying bacteria, and unfavorable soil conditions in terms of micronutrient deficiency and moisture stress (Divito and Sadras, 2014; Hungria et al., 2013; Ndakidemi et al., 2006). On the other hand, the lower BNF associated with commercial organic fertilizer treatments could be due to lower populations of rhizobia and limited nutrient supply (Fernández-Calviño et al., 2010; Widmer et al., 2006). The findings reveal the ineffectiveness of rhizobia inoculant for BNF in degraded soils and bean cropping systems ravaged by climate change impacts. The higher BNF success and bean yield achieved using BSFFF imply that to ensure high BNF success in highly weathered soils, it is better to create favorable soil conditions and stimulate activities of native rhizobia populations, rather than supplying elite rhizobia strains in degraded soils with adverse environmental conditions.
Our findings show that BSFFF applied at the rate of 30 kg N ha−1 could boost the activities of indigenous nitrogen-fixing bacteria and fix appreciable amounts of nitrogen for individual use by legumes, while sparing some for other crops in rotation. The highest quantity of nitrogen fixed (82.9 kg N ha−1) attained through the use of sole BSFFF at a rate of 30 kg N ha-1, was higher than the recommended N rate of 40 kg N ha−1 for bush bean production (Chekanai et al., 2018), indicating that BSFFF can be relied on to boost the supply of the nitrogen required for the entire growth cycle of beans.
The higher economic returns achieved using NPK fertilizer compared to organic fertilizers (Phymyx and BSFFF) and biofertilizers have been previously reported and could be attributed to higher labor costs associated with organic fertilizer production and application (Beesigamukama et al., 2021c; Mucheru-Muna et al., 2014). Because the BSFFF is an emerging input, its price is slightly high due to high demand; it is anticipated that scaling production of BSFFF will reduce the price and increase the profits of crop production. On the other hand, previous economic assessments (Beesigamukama et al., 2021c) have revealed that a circular economy model involving the direct use of locally produced BSF frass fertilizer for crop production boosts profit margins compared to NPK, which is critical especially for smallholder farmers that are often time-rich but financially constrained. Furthermore, it is anticipated the residual benefits of BSFFF on soil health will reduce the need for seasonal application, leading to higher profit margins. On the other hand, the higher net income and profit margins associated with BSFFF compared to Phymyx could be attributed to its superior nutrient quality, and lower cost compared with Phymyx. Similar findings were reported by Tanga et al. (2022) where the BSFFF yielded higher economic returns to maize production compared to commercial organic fertilizers. This illustrates the potential of substituting the costly yet less effective commercial organic fertilizers (Gram et al., 2020).
Our findings have outlined the critical role played by BSFFF as a sustainable organic input with reduced ecological footprint compared to commercial fertilizers. Compared to mineral fertilizers, insect frass fertilizer offers multiple benefits and plays a critical role in rejuvenating the highly weathered and degraded soils of SSA and other tropical regions. This novel and climate-smart fertilizer technology is holistic in nature as a circular economy strategy for recycling organic waste residues into high-quality fertilizer for crop production and insect-based livestock feed while boosting household income, conserving the environment, and creating jobs and opportunities for women, youth, and other marginalized communities. Ultimately, the insect-driven fertilizer technology contributes to food and nutrition security, poverty reduction, responsible consumption, biodiversity conservation, climate change mitigation, and the realization of several sustainable development goals in general.
5 Conclusion
Our study provides, for the first time, evidence of the efficacy of black soldier fly frass fertilizer in enhancing bush bean growth, yield, and biological N fixation. The highest bush bean growth rate, nutrient uptake, and yield were achieved from soil amended with BSF frass fertilizer applied at a rate equivalent to 45 kg N ha-1 while the highest net income was achieved at a rate equivalent to 15 kg N ha-1. We have demonstrated that BSF frass fertilizer application at rates equivalent to 30 kg N ha-1 can boost the biological N fixation process and enable bush beans to fix enough nitrogen required for optimal growth and yield, thus eliminating the necessity for inorganic fertilizer or biofertilizer application in bean cropping systems. Our findings show that boosting biological nitrogen fixation in bush beans requires strategies that restore soil health to stimulate indigenous rhizobia populations, rather than supplying elite strains of rhizobia in soils with multiple degradation challenges. Therefore, the adoption of BSF frass fertilizer in bush bean cropping systems will counteract the high costs of mineral fertilizers, contribute to sustainable soil health management, and accelerate the transition to circular, regenerative, and climate-smart agri-food systems. Nevertheless, further studies are warranted to determine nodule occupancy of bush beans grown in soils amended using BSF frass fertilizer, and the mid-long-term effects of this fertilizer on soil health, carbon sequestration, and greenhouse gas emissions.
Data availability statement
The original contributions presented in the study are included in the article/supplementary material. Further inquiries can be directed to the corresponding authors.
Author contributions
AC: Conceptualization, Data curation, Formal analysis, Investigation, Methodology, Software, Validation, Visualization, Writing – original draft, Writing – review & editing. DB: Conceptualization, Data curation, Formal analysis, Investigation, Methodology, Project administration, Resources, Software, Supervision, Validation, Visualization, Writing – original draft, Writing – review & editing. HG: Conceptualization, Data curation, Formal analysis, Investigation, Methodology, Resources, Software, Supervision, Validation, Visualization, Writing – original draft, Writing – review & editing. SC: Conceptualization, Validation, Visualization, Writing – original draft, Writing – review & editing. SS: Conceptualization, Funding acquisition, Project administration, Writing – original draft, Writing – review & editing. SE: Conceptualization, Funding acquisition, Project administration, Writing – original draft, Writing – review & editing. BA: Conceptualization, Funding acquisition, Writing – original draft, Writing – review & editing. JR: Conceptualization, Funding acquisition, Writing – original draft, Writing – review & editing. TZ: Conceptualization, Funding acquisition, Writing – original draft, Writing – review & editing, Project administration. GA: Writing – original draft, Writing – review & editing, Project administration. AZ: Writing – original draft, Writing – review & editing, Project administration. VZ: Conceptualization, Writing – original draft, Writing – review & editing, Project administration. AT: Funding acquisition, Project administration, Validation, Visualization, Writing – original draft, Writing – review & editing. CT: Conceptualization, Data curation, Formal analysis, Funding acquisition, Investigation, Methodology, Project administration, Resources, Software, Supervision, Validation, Visualization, Writing – original draft, Writing – review & editing.
Funding
The author(s) declare financial support was received for the research, authorship, and/or publication of this article. The authors gratefully acknowledge the financial support for this research by the following organizations and agencies: Australian Centre for International Agricultural Research (ACIAR) (ProteinAfrica –Grant No: LS/2020/154), Global Affairs Canada (BRAINS project: P011585), Novo Nordisk Foundation (RefIPro: NNF22SA0078466), the Rockefeller Foundation (WAVE-IN—Grant No: 2021 FOD 030); Bill and Melinda Gates Foundation (INV-032416); IKEA Foundation (G-2204-02144), European Commission (HORIZON 101060762 NESTLER and HORIZON 101136739 INNOECOFOOD), the Curt Bergfors Foundation Food Planet Prize Award; Norwegian Agency for Development Cooperation, the Section for Research, Innovation, and Higher Education grant number RAF–3058 KEN–18/0005 (CAP–Africa); the Swedish International Development Cooperation Agency (Sida); the Swiss Agency for Development and Cooperation (SDC); the Australian Centre for International Agricultural Research (ACIAR); the Norwegian Agency for Development Cooperation (Norad); the German Federal Ministry for Economic Cooperation and Development (BMZ); the Federal Democratic Republic of Ethiopia; and the Government of the Republic of Kenya. The funders had no role in study design, data collection and analysis, decision to publish, or preparation of the manuscript. The views expressed herein do not necessarily reflect the official opinion of the donors.
Acknowledgments
The authors are greatly indebted to Faith N. Wamurango, Shem Ondiaka, Isaiah E. Rachami, Joshua M. Wambua, Judy Gitonga, Paul T. Kibogo, and Raphael K. Kioko from icipe’s Insects for Food, Feed and Other Uses Program (INSEFF), and Kennedy K. Kilel, Mathew Theuri, Karen Kaaria and Lucy W. Muchiri from Kenyatta University’s soil and plant analysis laboratory for the technical support provided during the entire study.
Conflict of interest
Authors TZ, GA, AZ, and VZ were employed by Synelixis Solutions S.A.
The remaining authors declare that the research was conducted in the absence of any commercial or financial relationships that could be construed as a potential conflict of interest.
Publisher’s note
All claims expressed in this article are solely those of the authors and do not necessarily represent those of their affiliated organizations, or those of the publisher, the editors and the reviewers. Any product that may be evaluated in this article, or claim that may be made by its manufacturer, is not guaranteed or endorsed by the publisher.
References
Abiya, A. A., Kupesa, D. M., Beesigamukama, D., Kassie, M., Mureithi, D., Thairu, D., et al. (2022). Agronomic performance of kale (Brassica oleracea) and swissc chard (Beta vulgaris) grown on soil amended with black soldier fly frass fertilizer under wonder multistorey gardening system. Agronomy 12, 2211. doi: 10.3390/agronomy12092211
Adin Yéton, B. G., Aliou, S., Noël, O., Guillaume, L. A., Attanda, M. I., Victor, A. C., et al. (2019). Decomposition and nutrient release pattern of agro-processing by-products biodegraded by fly larvae in Acrisols. Arch. Agron. Soil Sci. 65, 1610–1621. doi: 10.1080/03650340.2019.1572118
Africa Fertilizer. (2022). National fertilizer prices. Available online at: https://africafertilizer.org/national/#tab-id-3 (Accessed 20th APril 2023).
Agustiyani, D., Agandi, R., Arinafril Nugroho, A. A., Antonius, S. (2021). The effect of application of compost and frass from Black Soldier Fly Larvae (Hermetia illucens L.) on growth of Pakchoi (Brassica rapa L.). IOP Conf. Ser. Earth Environ. Sci. 762, 1-10. doi: 10.1088/1755-1315/762/1/012036
Anedo, E. O., Beesigamukama, D., Mochoge, B., Korir, N. K., Haukeland, S., Cheseto, X., et al. (2024). Evolving dynamics of insect frass fertilizer for sustainable nematode management and potato production. Front. Plant Sci. 15. doi: 10.3389/fpls.2024.1343038
Anyega, A. O., Korir, N. K., Beesigamukama, D., Changeh, G. J., Nkoba, K., Subramanian, S., et al. (2021). Black soldier fly-composted organic fertilizer enhances growth, yield, and nutrient quality of three key vegetable crops in Sub-Saharan Africa. Front. Plant Sci. 12. doi: 10.3389/fpls.2021.680312
Araujo, J., Urbano, B., González-Andrés, F. (2020). Comparative environmental life cycle and agronomic performance assessments of nitrogen fixing rhizobia and mineral nitrogen fertiliser applications for pulses in the Caribbean region. J. Clean. Prod. 267, 122065. doi: 10.1016/j.jclepro.2020.122065
Barragán-Fonseca, K. Y., Nurfikari, A., Zande, E. M., Wantulla, M., Loon, J. J., Boer, W., et al. (2022). Insect frass and exuviae to promote plant growth and health. Trends Plant Sci. 27, 646–654. doi: 10.1016/j.tplants.2022.01.007
Beebe, S. E., Rao, I. M., Blair, M. W., Acosta-Gallegos, J. A. (2013). Phenotyping common beans for adaptation to drought. Front. Physiol. 4. doi: 10.3389/FPHYS.2013.00035/BIBTEX
Beesigamukama, D., Mochoge, B., Korir, N. K., Fiaboe, K. K., Nakimbugwe, D., Khamis, F. M., et al. (2021a). Low-cost technology for recycling agro-industrial waste into nutrient-rich organic fertilizer using black soldier fly. Waste Manage. 119, 183–194. doi: 10.1016/j.wasman.2020.09.043
Beesigamukama, D., Mochoge, B., Korir, N. K., Fiaboe, K. K. M., Nakimbugwe, D., Khamis, F. M., et al. (2020a). Exploring black soldier fly frass as novel fertilizer for improved growth, yield, and nitrogen use efficiency of maize under field conditions. Front. Plant Sci. 11. doi: 10.3389/fpls.2020.574592
Beesigamukama, D., Mochoge, B., Korir, N., Ghemoh, C. J., Subramanian, S., Tanga, C. M. (2021b). In situ nitrogen mineralization and nutrient release by soil amended with black soldier fly frass fertilizer. Sci. Rep. 11, 14799. doi: 10.1038/s41598-021-94269-3
Beesigamukama, D., Mochoge, B., Korir, N., Menale, K., Muriithi, B., Kidoido, M., et al. (2021c). Economic and ecological values of frass fertiliser from black soldier fly agro-industrial waste processing. J. Insects Food Feed 8, 245–254. doi: 10.3920/JIFF2021.0013
Beesigamukama, D., Mochoge, B., Korir, N. K., Musyoka, M. W., Fiaboe, K. K., Nakimbugwe, D., et al. (2020b). Nitrogen fertilizer equivalence of black soldier fly frass fertilizer and synchrony of nitrogen mineralization for maize production. Agronomy 10, 1395. doi: 10.3390/agronomy10091395
Beesigamukama, D., Subramanian, S., Tanga, C. M. (2022). Nutrient quality and maturity status of frass fertilizer from nine edible insects. Sci. Rep. 12, 1–14. doi: 10.1038/s41598-022-11336-z
Beesigamukama, D., Tanga, C. M., Sevgan, S., Ekesi, S., Kelemu, S. (2023). Waste to value: Global perspective on the impact of entomocomposting on environmental health, greenhouse gas mitigation and soil bioremediation. Sci. Total Environ. 902, 166067. doi: 10.1016/j.scitotenv.2023.166067
Broughton, W. J., Hernández, G., Blair, M., Beebe, S., Gepts, P., Vanderleyden, J. (2003). Beans (Phaseolus spp.)–model food legumes. Plant Soil 252, 55–128. doi: 10.1023/A:1024146710611
Cafaro La Menza, N., Monzon, J. P., Lindquist, J. L., Arkebauer, T. J., Knops, J. M., Unkovich, M., et al. (2020). Insufficient nitrogen supply from symbiotic fixation reduces seasonal crop growth and nitrogen mobilization to seed in highly productive soybean crops. Plant Cell Environ. 43, 1958–1972. doi: 10.1111/pce.13804
Carranca, C. (2017). “Legumes: properties and symbiosis,” in Symbiosis: Chapter: Legumes: Properties and symbiosis. Evolution, Biology and Ecological Effects. Eds. Camisão, A. H., Pedroso, C. C. (Nova Science Publishers, New York).
Chapagain, T., Riseman, A. (2014). Barley–pea intercropping: Effects on land productivity, carbon and nitrogen transformations. Field Crops Res. 166, 18–25. doi: 10.1016/j.fcr.2014.06.014
Chekanai, V., Chikowo, R., Vanlauwe, B. (2018). Response of common bean (Phaseolus vulgaris L.) to nitrogen, phosphorus and rhizobia inoculation across variable soils in Zimbabwe. Agriculture Ecosyst. Environ. 266, 167–173. doi: 10.1016/j.agee.2018.08.010
Chia, S. Y., Tanga, C. M., Osuga, I. M., Alaru, A. O., Mwangi, D. M., Githinji, M., et al. (2019). Effect of dietary replacement of fishmeal by insect meal on growth performance, blood profiles and economics of growing pigs in Kenya. Animals 9, 705. doi: 10.3390/ani9100705
Chirwa, P. W., Larwanou, M., Syampungani, S., Babalola, F. D. (2015). Management and restoration practices in degraded landscapes of Eastern Africa and requirements for up-scaling. Int. Forestry Rev. 17, 20–30. doi: 10.1505/146554815816007009
Choi, Y. C., Choi, J. Y., Kim, J. G., Kim, M. S., Kim, W. T., Park, K. H., et al. (2009). Potential Usage of Food Waste as a Natural Fertilizer after Digestion by Hermetia illucens (Diptera: Stratiomyidae). Int. J. Ind. Entomol. 19, 171–174. doi: 10.1016/j.jbiotec.2019.10.015
Choi, S., Hassanzadeh, N. (2019). BSFL frass: a novel biofertilizer for improving plant health while minimizing environmental impact. Candian Sci. Fair J. 2, 41–46. doi: 10.18192/csfj.v2i220194146
Chtouki, M., Laaziz, F., Naciri, R., Garré, S., Nguyen, F., Oukarroum, A. (2022). Interactive effect of soil moisture content and phosphorus fertilizer form on chickpea growth, photosynthesis, and nutrient uptake. Sci. Rep. 12, 1–13. doi: 10.1038/s41598-022-10703-0
Cordeiro, A. B., Ribeiro, R. A., Helene, L. C. F., Hungria, M. (2017). Rhizobium esperanzae sp. nov., a N2-fixing root symbiont of Phaseolus vulgaris from Mexican soils. Int. J. Syst. Evol. Microbiol. 67, 3937–3945. doi: 10.1099/ijsem.0.002225
Daryanto, S., Wang, L., Jacinthe, P. A. (2015). Global synthesis of drought effects on food legume production. PloS One 10, 1–16. doi: 10.1371/journal.pone.0127401
Devi, K. N., Singh, K. N. L., Sunanda, T., Nanita, H., Singh, B., Singh, K. K., et al. (2012). Response of soybean [Glycine max L. Merrill] to sources and levels of phosphorus. J. Agric. Sci. 4, 44–53. doi: 10.5539/jas.v4n6p44
Dhanjal, R., Prakash, O., Ahlawat, I. P. S. (2003). Physiological variations in french bean (Phaseolus vularis) cultivars as affected by plant density and nitrogen. Indian J. Plant Physiol. 8, 34–37.
Divito, G. A., Sadras, V. O. (2014). How do phosphorus, potassium and sulphur affect plant growth and biological nitrogen fixation in crop and pasture legumes? A meta-analysis. Field Crops Res. 156, 161–171. doi: 10.1016/j.fcr.2013.11.004
Faghire, M., Bargaz, A., Farissi, M., Palma, F., Mandri, B., Lluch, C., et al. (2011). Effect of salinity on nodulation, nitrogen fixation and growth of common bean (Phaseolus vulgaris) inoculated with rhizobial strains isolated from the Haouz region of Morocco. Symbiosis 55, 69–75. doi: 10.1007/s13199-011-0144-0
Fairhurst, T. (ed.) (2012). Africa soil health consortium: Handbook for Integrated Soil Fertility Management. Africa Soil Health Consortium, Nairobi.
FAO, IFAD, UNICEF, WFP and WHO. (2022). The State of Food Security and Nutrition in the World 2022. Repurposing food and agricultural policies to make healthy diets more affordable (Rome: FAO). doi: 10.4060/cc0639en
FAO-UNESCO (1990). A world dataset of derived soil properties by FAO-UNESCO soil unit for global modelling. Soil Use Manage. 13, 9–16. doi: 10.1111/j.1475-2743.1997.tb00550.x
Fernández-Calviño, D., Martín, A., Arias-Estévez, M., Bååth, E., Díaz-Raviña, M. (2010). Microbial community structure of vineyard soils with different pH and copper content. Appl. Soil Ecol. 46, 276–282. doi: 10.1016/j.apsoil.2010.08.001
Fernández-Luqueño, F., Reyes-Varela, V., Martínez-Suárez, C., Salomón-Hernández, G., Yáñez-Meneses, J., Ceballos-Ramírez, J. M., et al. (2010). Effect of different nitrogen sources on plant characteristics and yield of common bean (Phaseolus vulgaris L.). Bioresource Technol. 101, 396–403. doi: 10.1016/j.biortech.2009.07.058
Gebremikael, M. T., Steel, H., Bert, W., Maenhout, P., Sleutel, S., De Neve, S. (2015). Quantifying the contribution of entire free-living nematode communities to carbon mineralization under contrasting C and N availability. PloS One 10, e0136244. doi: 10.1371/journal.pone.0136244
Gicharu, G., Gitonga, N., Boga, H., Cheruiyot, R., Maingi, J. (2013). Effect of inoculating selected climbing bean cultivars with different rhizobia strains on nitrogen fixation. Online Int. J. Microbiol. Res. 1, 25–31. Available at: http://www.onlineresearchjournals.org/OIJMR.
Gold, M., von Allmen, F., Zurbrügg, C., Zhang, J., Mathys, A. (2020). Identification of bacteria in two food waste black soldier fly larvae rearing residues. Front. Microbiol. 11. doi: 10.3389/fmicb.2020.582867
Goswami, L., Sarkar, S., Mukherjee, S., Das, S., Barman, S., Raul, P., et al. (2014). Vermicomposting of Tea Factory Coal Ash: Metal accumulation and metallothionein response in Eisenia fetida (Savigny) and Lampito mauritii (Kinberg). Bioresour. Technol. 166, 96-102. doi: 10.1016/j.biortech.2014.05.032
Gram, G., Roobroeck, D., Pypers, P., Six, J., Merckx, R., Vanlauwe, B. (2020). Combining organic and mineral fertilizers as a climate-smart integrated soil fertility management practice in sub-Saharan Africa: A meta-analysis. PloS One 15, 1–30. doi: 10.1371/journal.pone.0239552
Hänsch, R., Mendel, R. R. (2009). Physiological functions of mineral micronutrients (Cu, Zn, Mn, Fe, Ni, Mo, B, Cl). Curr. Opin. Plant Biol. 12, 259-266. doi: 10.1016/j.pbi.2009.05.006
Herridge, D. F., Peoples, M. B., Boddey, R. M. (2008). Global inputs of biological nitrogen fixation in agricultural systems. Plant Soil. 311, 1–18. doi: 10.1007/s11104-008-9668-3
Humanitarian Data Exchange. (2023). Kenya bean prices 2022. Available online at: https://data.humdata.org/dataset/wfp-food-prices-for-Kenya. (Accessed on 24th April 2023).
Hungria, M., Nogueira, M. A., Araujo, R. S. (2013). Co-inoculation of soybeans and common beans with rhizobia and azospirilla: strategies to improve sustainability. Biol. Fertility Soils 49, 791–801. doi: 10.1007/s00374-012-0771-5
Jackson, K. W., Mahmood, T. M. (1994). Atomic absorption, atomic emission, spectrometry. Anal. Chem. 64, 50–66. doi: 10.1021/ac00036a003
Jena, J., Maitra, S., Hossain, A., Pramanick, B., Gitari, H. I., Praharaj, S., et al. (2022). “Role of legumes in cropping system for soil ecosystem improvement,” in Ecosystem services: types, management and benefits. Eds. Jatav, H. S., Rajput, V. D. (Nova Science Publishers, Inc, 415 Oser Avenue, Suite N Hauppauge, NY, 11788 USA). doi: 10.52305/PFZA6988.
Jensen, E. S., Peoples, M. B., Boddey, R. M., Gresshoff, P. M., Hauggaard-Nielsen, H., JR Alves, B., et al. (2012). Legumes for mitigation of climate change and the provision of feedstock for biofuels and biorefineries. A review. Agron. Sustain. Dev. 32, 329–364. doi: 10.1007/s13593-011-0056-7
Jourand, P., Giraud, E., Béna, G., Sy, A., Willems, A., Gillis, M., et al. (2004). Methylobacterium nodulans sp. nov., for a group of aerobic, facultatively methylotrophic, legume root-nodule-forming and nitrogen-fixing bacteria. Int. J. Syst. Evol. Microbiol. 54, 2269–2273. doi: 10.1099/ijs.0.02902-0
Kagata, H., Ohgushi, T. (2012). Positive and negative impacts of insect frass quality on soil nitrogen availability and plant growth. Population Ecol. 54, 75–82. doi: 10.1007/s10144-011-0281-6
Kakon, S. S., Bhuiya, M. S. U., Hossain, S. M. A., Naher, Q., Bhuiyan, M. D. (2016). Effect of nitrogen and phosphorus on growth and seed yield of French bean. Bangladesh J. Agric. Res. 41, 759–772. doi: 10.3329/bjar.v41i4.30706
KALRO. (2008). Better bean varieties. Kenyan agricultural and livestock research organisation. Available online at: https://www.kalro.org/fileadmin/publications/brochuresI/Beanvariety.pdf. (Accessed on 28th July 2022).
Kalyebara, R., Buruchara, R. A. (2008). The impact of new bean technologies on rural livelihoods in seven African countries. Centro Internacional de Agricultura Tropical (CIAT), Kampala, UG. 2 p. (Highlights: CIAT in Africa no. 44).
Katungi, E., Farrow, A., Mutuoki, T., Gebeyehu, S., Karanja, D., Alamayehu, F., et al. (2010). Improving common bean productivity: An Analysis of socioeconomic factors in Ethiopia and Eastern Kenya. CIAT, Cali, Colombia.
Kemboi, V. J., Kipkoech, C., Njire, M., Were, S., Lagat, M. K., Ndwiga, F., et al. (2022). Biocontrol potential of chitin and chitosan extracted from black soldier fly pupal exuviae against bacterial wilt of tomato. Microorganisms 10, 165. doi: 10.3390/microorganisms10010165
Kenya Government. (2022). The regulation of wages. General amendment order 2022 (Nairobi, Kenya: Kenya Government).
Khanam, A., Platel, K. (2016). Influence of domestic processing on the bioaccessibility of selenium from selected food grains and composite meals. J. Food Sci. Technol. 53, 1634–1639. doi: 10.1007/s13197-015-2075-x
Kisaakye, J., Beesigamukama, D., Haukeland, S., Subramanian, S., Thiongo, P. K., Kelemu, S., et al. (2024). Chitin-enriched insect frass fertilizer as a biorational alternative for root-knot nematode (Meloidogyne incognita) management. Front. Plant Sci. 15. doi: 10.3389/fpls.2024.1361739
Klammsteiner, T., Turan, V., Fernandez-Delgado Juarez, M., Oberegger, S., Insam, H. (2020). Suitability of black soldier fly frass as soil amendment and implication for organic waste hygienization. Agronomy 10, 1578. doi: 10.3390/agronomy10101578
Korir, H., Mungai, N. W., Thuita, M., Hamba, Y., Masso, C. (2017). Co-inoculation effect of rhizobia and plant growth promoting rhizobacteria on common bean growth in a low phosphorus soil. Front. Plant Sci. 8. doi: 10.3389/fpls.2017.00141
Koskey, G., Mburu, S. W., Njeru, E. M., Kimiti, J. M., Ombori, O., Maingi, J. M. (2017). Potential of native rhizobia in enhancing nitrogen fixation and yields of climbing beans (Phaseolus vulgaris L.) in contrasting environments of Eastern Kenya. Front. Plant Sci. 8. doi: 10.3389/fpls.2017.00443
Kyomuhendo, P., Tenywa, M. M., Semalulu, O., Lenssen, A. W., Yost, R. S., Mazur, R. E., et al. (2018). Limiting nutrients for bean production on contrasting soil types of Lake Victoria Crescent of Uganda. Afr. Crop Sci. J. 26, 543–554. doi: 10.4314/acsj.v26i4.8
Lagat, M. K., Were, S., Ndwigah, F., Kemboi, V. J., Kipkoech, C., Tanga, C. M. (2021). Antimicrobial activity of chemically and biologically treated chitosan prepared from black soldier fly (Hermetia illucens) pupal shell waste. Microorganisms 26, 2417. doi: 10.3390/microorganisms9122417
Lalander, C. H., Fidjeland, J., Diener, S., Eriksson, S., Vinnerås, B. (2015). High waste-to-biomass conversion and efficient Salmonella spp. reduction using black soldier fly for waste recycling. Agron. Sustain. Dev. 35, 261–271. doi: 10.1007/s13593-014-0235-4
Maingi, J. M., Shisanya, C. A., Gitonga, N. M., Hornetz, B. (2001). Nitrogen fixation by common bean (Phaseolus vulgaris L.) in pure and mixed stands in semi-arid south-east Kenya. Eur. J. Agron. 14, 1–12. doi: 10.1016/S1161-0301(00)00080-0
Mendoza-Suárez, M. A., Geddes, B. A., Sánchez-Cañizares, C., Ramírez-González, R. H., Kirchhelle, C., Jorrin, B., et al. (2020). Optimizing Rhizobium-legume symbioses by simultaneous measurement of rhizobial competitiveness and N2 fixation in nodules. Proc. Natl. Acad. Sci. U.S.A. 117, 9822–9831. doi: 10.1073/pnas.1921225117
Mingotte, F. L. C., Lemos, L. B., Jardim, C. A., Fornasieri, D. (2019). Crop systems and topdressing nitrogen on grain yield and technological attributes of common bean under no-tillage. Pesquisa Agropecuária Tropica 49, e54003. doi: 10.1590/1983-40632019v4954003
Mirriam, A., Mugwe, J., Nasar, J., Kisaka, O., Ranjan, S., Gitari, H. (2023). Role of phosphorus and inoculation with Bradyrhizobium in enhancing soybean production. Adv. Agric. 2023, 3231623. doi: 10.1155/2023/3231623
Mirriam, A., Mugwe, J., Raza, M. A., Seleiman, M. F., Maitra, S., Gitari, H. H. (2022). Aggrandizing soybean yield, phosphorus use efficiency and economic returns under phosphatic fertilizer application and inoculation with Bradyrhizobium. J. Soil Sci. Plant Nutr. 22, 1–13. doi: 10.1007/s42729-022-00985-8
Mohammadi, K., Sohrabi, Y., Heidari, G., Khalesro, S., Majidi, M. (2012). Effective factors on biological nitrogen fixation. Afr. J. Agric. Res. 7, 1782–1788. doi: 10.5897/ajarx11.034
Mohammed, S. B., Mohammad, I. F., Pangirayi, T. B., Vernon, G., Dzidzienyo, D. K., Umar, M. L., et al. (2020). Farmers’ knowledge, perception, and use of phosphorus fertilization for cowpea production in Northern Guinea Savannah of Nigeria. Heliyon 6, e05207. doi: 10.1016/j.heliyon.2020.e05207
Montanarella, L., Pennock, D.J., McKenzie, N., Badraoui, M., Chude, V., Baptista, I., et al. (2016). World’s soils are under threat. Soil 2, 79–82. doi: 10.5194/soil-2-79-2016
Morón, B., Soria-Díaz, M. E., Ault, J., Verroios, G., Noreen, S., Rodríguez-Navarro, D. N., et al. (2005). Low pH changes the profile of nodulation factors produced by Rhizobium tropici CIAT899. Chem. Biol. 12, 1029–1040. doi: 10.1016/j.chembiol.2005.06.014
Moyer-Henry, J. W., Burton, D. W., Israel, T. W. (2006). Nitrogen transfer between plants: A 15 N natural abundance study with crop and weed species. Plant and Soil 282, 7–20. doi: 10.1007/s11104-005-3081-y
Mucheru-Muna, M., Mugendi, D., Pypers, P., Mugwe, J., Kung’u, J., Vanlauwe, B., et al. (2014). Enhancing maize productivity and profitability using organic inputs and mineral fertilizer in central Kenya small-hold farms. Exp. Agriculture. 50, 250–269. doi: 10.1017/S0014479713000525
Mugo, N. J., Karanja, N. N., Gachene, C. K., Dittert, K., Gitari, H. I., Schulte-Geldermann, E. (2021). Response of potato crop to selected nutrients in Central and Eastern highlands of Kenya. Cogent Food Agriculture. 7, 1898762. doi: 10.1080/23311932.2021.1898762
Mukankusi, C., Raatz, B., Nkalubo, S., Berhanu, F., Binagwa, P., Kilango, M., et al. (2019). Genomics, genetics and breeding of common bean in Africa: A review of tropical legume project. Plant Breed. 138, 401–414. doi: 10.1111/pbr.12573
Mulambula, S., Gathungu, G., Ndukhu, H., Ogolla, F. (2019). Effects of integrated application of rhizobium and phosphatic fertilizer on growth, nodulation and yields of soybean in Meru South Kenya. J. Environ. Sustainability Advanced Res. 5, 11–19. doi: 10.22161/ijeab.45.28
Mutuma, S. P., Okello, J. J., Karanja, N. K., Woomer, P. L. (2014). Smallholder farmers’ use and profitability of legume inoculants in western Kenya. Afr. Crop Sci. J. 22, 205–213.
Mwadalu, R., Mochoge, B., Mwangi, M., Maitra, S., Gitari, H. (2022). Response of Gadam sorghum (Sorghum bicolor) to farmyard manure and inorganic fertilizer application. Int. J. Agriculture Environment Biotechnol. 15, 51–60. doi: 10.30954/0974-1712.01.2022.6
Nasar, J., Khan, W., Khan, M. Z., Gitari, H. I., Gbolayori, J. F., Moussa, A. A., et al. (2021). Photosynthetic activities and photosynthetic nitrogen use efficiency of maize crop under different planting patterns and nitrogen fertilization. J. Soil Sci. Plant Nutr. 21, 2274–2284. doi: 10.1007/s42729-021-00520-1
Ndakidemi, P. A., Dakora, F. D., Nkonya, E. M., Ringo, D., Mansoor, H. (2006). Yield and economic benefits of common bean (Phaseolus vulgaris) and soybean (Glycine max) inoculation in northern Tanzania. Aust. J. Exp. Agric. 46, 571–577. doi: 10.1071/EA03157
Ndambi, O. A., Pelster, D. E., Owino, J. O., De Buisonje, F., Vellinga, T. (2019). Manure management practices and policies in sub-Saharan Africa: implications on manure quality as a fertilizer. Front. Sustain. Food Syst. 3. doi: 10.3389/fsufs.2019.00029
Nichols, V. A., Ordóñez, R. A., Wright, E. E., Castellano, M. J., Liebman, M., Hatfield, J. L., et al. (2019). Maize root distributions strongly associated with water tables in Iowa, USA. Plant Soil 444, 225–238. doi: 10.1007/s11104-019-04269-6
Nungula, E. Z., Mugwe, J., Nasar, J., Massawe, H. J., Karuma, A. N., Maitra, S., et al. (2023). Land degradation unmasked as the key constraint in sunflower (Helianthus annus) production: Role of GIS in Revitalizing this vital sector. Cogent Food Agriculture. 9, 2267863. doi: 10.1080/23311932.2023.2267863
Nyawade, S. O., Gachene, C. K. K., Karanja, N. N., Gitari, H. I., Schulte-Geldermann, E., Parker, M. (2019). Controlling soil erosion in smallholder potato farming systems using legume intercrops. Geoderma Regional 17, e00225. doi: 10.1016/j.geodrs.2019.e00225
Ojiewo, C., Keatinge, D. J. D. H., Hughes, J., Tenkouano, A., Nair, R., Varshney, R., et al. (2015). The role of vegetables and legumes in assuring food, nutrition, and income security for vulnerable groups in Sub-Saharan Africa. World Med. Health Policy 7, 187–210. doi: 10.1002/wmh3.148
Okalebo, J. R., Gathua, K. W., Woomer, P. L. (2002). Laboratory methods of soil and plant analysis. A working manual. 2nd ed. (Nairobi, Kenya: TSBF-CIAT and SACRED Africa).
Ordóñez, R. A., Castellano, M. J., Hatfield, J. L., Helmers, M. J., Licht, M. A., Liebman, M., et al. (2018). Maize and soybean root front velocity and maximum depth in Iowa, USA. Field Crops Res. 215, 122–131. doi: 10.1016/j.fcr.2017.09.003
Pagliai, M., Vignozzi, N., Pellegrini, S. (2004). Soil structure and the effect of management practices. Soil Tillage Res. 79, 131–143. doi: 10.1016/j.still.2004.07.002
Paynel, F., Lesuffleur, F., Bigot, J., Diquélou, S., Cliquet, J. B. (2008). A study of 15N transfer between legumes and grasses. Agron. Sustain. Dev. 28, 281–290. doi: 10.1051/agro:2007061
Peoples, M. B., Faizah, A. W., Rerkasem, B., Herridge, D. F. (eds). (1989). Methods for evaluating nitrogen fixation by nodulated legumes in the field Vol. 11 (St, Bruce ACT, Australia: Australian Centre for International Agricultural Research). Available at: https://www.aciar.gov.au/node/7846.
Poveda, J., Jiménez-Gómez, A., Saati-Santamaría, Z., Usategui-Martín, R., Rivas, R., García-Fraile, P. (2019). Mealworm frass as a potential biofertilizer and abiotic stress tolerance-inductor in plants. Appl. Soil Ecol. 142, 110–122. doi: 10.1016/j.apsoil.2019.04.016
Quilliam, R. S., Nuku-Adeku, C., Maquart, P., Little, D., Newton, R., Murray, F. (2020). Integrating insect frass biofertilisers into sustainable peri-urban agro-food systems. J. Insects Food Feed 6, 315–322. doi: 10.3920/JIFF2019.0049
R Core Team. (2022). R: A language and environment for statistical computing. Vienna, Austria: R Foundation for Statistical Computing. Available at: https://www.R-project.org/.
Reinprecht, Y., Schram, L., Marsolais, F., Smith, T. H., Hill, B., Pauls, K. P. (2020). Effects of nitrogen application on nitrogen fixation in common bean production. Front. Plant Sci. 11. doi: 10.3389/fpls.2020.01172
Sairaam, M., Maitra, S., Praharaj, S., Nath, S., Shankar, T., Sahoo, U., et al. (2023). “An Insight into the Consequences of Emerging Contaminants in Soil and Water and Plant Responses,” in Emerging contaminants and plants. Ed. Aftab, T. (Springer, Cham). doi: 10.1007/978-3-031-22269-6_1.
Schulze, J., Temple, G., Temple, S. J., Beschow, H., Vance, C. P. (2006). Nitrogen fixation by white lupin under phosphorus deficiency. Ann. Bot. 98, 731–740. doi: 10.1093/aob/mcl154
Shahid, M. Q., Saleem, M. F., Khan, H. Z., Anjum, S. A. (2009). Performance of soybean (Glycine max L.) under different phosphorus levels and inoculation. Pakistan J. Agric. Sci. 46, 237.
Shumo, M., Osuga, I. M., Khamis, F. M., Tanga, C. M., Fiaboe, K. K. M., Subramanian, S., et al. (2019). The nutritive value of black soldier fly larvae reared on common organic waste streams in Kenya. Sci. Rep. 9, 1–14. doi: 10.1038/s41598-019-46603-z
Soumare, A., Diedhiou, A. G., Thuita, M., Hafidi, M., Ouhdouch, Y., Gopalakrishnan, S., et al. (2020). Exploiting biological nitrogen fixation: A route towards a sustainable agriculture. Plants 9, 1–22. doi: 10.3390/plants9081011
Sousa, S. W., Soratto, R. P., Peixoto, D. S., Campos, T. S., da Silva, M. B., Souza, A. G. V., et al. (2022). Effects of Rhizobium inoculum compared with mineral nitrogen fertilizer on nodulation and seed yield of common bean. A meta-analysis. Agron. Sustain. Dev. 42, 52. doi: 10.1007/s13593-022-00784-6
Stevens, G. G., Pérez-Fernández, M. A., Morcillo, R. J. L., Kleinert, A., Hills, P., Brand, D. J., et al. (2019). Roots and nodules response differently to P starvation in the mediterranean-type legume virgilia divaricata. Front. Plant Sci. 10. doi: 10.3389/fpls.2019.00073
Stoorvogel, J. J., Smaling, E. M. A. (1998). Research on soil fertility decline in tropical environments: Integration of spatial scales. Nutr. Cycl. Agroecosyst. 50, 151–158. doi: 10.1007/978-94-017-3021-1_15
Surendra, K. C., Tomberlin, J. K., van Huis, A., Cammack, J. A., Heckmann, L. H. L., Khanal, S. K. (2020). Rethinking organic wastes bioconversion: Evaluating the potential of the black soldier fly (Hermetia illucens (L.)) (Diptera: Stratiomyidae) (BSF). Waste Manage. 117, 58–80. doi: 10.1016/j.wasman.2020.07.050
Tanga, C. M., Beesigamukama, D., Kassie, M., Egonyu, P. J., Ghemoh, C. J., Nkoba, K., et al. (2022). Performance of black soldier fly frass fertiliser on maize (Zea mays L.) growth, yield, nutritional quality, and economic returns. J. Insects Food Feed 8, 185–196. doi: 10.3920/JIFF2021.0012
Tanga, C. M., Kababu, M. O. (2023). New insights into the emerging edible insect industry in Africa. Anim. Front. 13, 26–40. doi: 10.1093/af/vfad039
Tsige, B. A., Dechassa, N., Tana, T., Laekemariam, F., Alemayehu, Y. (2022). Effect of mineral nitrogen, phosphorus, and potassium fertilizers on the productivity of faba bean (Vicia faba L.) in acidic soils of wolaita zone, Southern Ethiopia. Int. J. Agron. 2022, 1–18. doi: 10.1155/2022/2232961
Walkley, A., Black, I. A. (1934). An examination of the degtjareff method for determining soil organic matter, and a proposed modification of the chromic acid titration method. Soil Sci. 37, 29–38. doi: 10.1097/00010694-193401000-00003
Wang, T., Cao, X., Chen, M., Lou, Y., Wang, H., Yang, Q., et al. (2022). Effects of soil acidification on bacterial and fungal communities in the Jiaodong Peninsula, Northern China. Agronomy 12, 927. doi: 10.3390/agronomy12040927
Wanjala, S. P., Karanja, D., Wambua, S., Otiep, G., Odhiambo, C., Birachi, E. (2019). Market arrangements used by small scale bean farmers in Kenya: What needs to change for sustainable trade volumes? Afr. Crop Sci. J. 27, 119–131. doi: 10.4314/acsj.v27i2.1
Wessén, E., Hallin, S., Philippot, L. (2010). Differential responses of bacterial and archaeal groups at high taxonomical ranks to soil management. Soil Biol. Biochem. 42, 1759–1765. doi: 10.1016/j.soilbio.2010.06.013
Widmer, F., Rasche, F., Hartmann, M., Fliessbach, A. (2006). Community structures and substrate utilization of bacteria in soils from organic and conventional farming systems of the DOK long-term field experiment. Appl. Soil Ecol. 33, 294–307. doi: 10.1016/j.apsoil.2005.09.007
Wortmann, C. S., Kaizzi, K. C., Maman, N., Cyamweshi, A., Dicko, M., Garba, M. (2019). Diagnosis of crop secondary and micro-nutrient deficiencies in sub-Saharan Africa. Nutr. Cycl. Agroecosyst. 113, 127–140. doi: 10.1007/s10705-018-09968-7
Xiang, D., Yong, T., Yang, W., Yan, W., Gong, W., Cui, L., et al. (2012). Effect of phosphorus and potassium nutrition on growth and yield of soybean in relay strip intercropping system. Sci. Res. Essays 7, 342–351. doi: 10.5897/SRE11.1086
Zaheer, M. S., Ali, H. H., Erinle, K. O., Wani, S. H., Okon, O. G., Nadeem, M. A., et al. (2022). Inoculation of Azospirillum brasilense and exogenous application of trans-zeatin riboside alleviates arsenic induced physiological damages in wheat (Triticum aestivum). Environ. Sci. pollut. Res. 29, 33909–33919. doi: 10.1007/s11356-021-18106-w
Zhou, S., Zhang, C., Huang, Y., Chen, H., Yuan, S., Zhou, X. (2021). Characteristics and research progress of legume nodule senescence. Plants 10, 1103. doi: 10.3390/plants10061103
Zingore, Z., Mutegi, J., Agesa, B., Tamene, L., Kihara, J. (2015). Soil degradation in sub-saharan Africa and crop production options for soil rehabilitation. Better Crops Plant Food 99, 24–26. Available at: https://hdl.handle.net/10568/68702. (Accessed on 17th July 2023).
Keywords: BSF frass fertilizer, symbiotic nitrogen fixation, bush bean yield, circular economy, soil health, climate-smart fertilizer
Citation: Chepkorir A, Beesigamukama D, Gitari HI, Chia SY, Subramanian S, Ekesi S, Abucheli BE, Rubyogo JC, Zahariadis T, Athanasiou G, Zachariadi A, Zachariadis V, Tenkouano A and Tanga CM (2024) Insect frass fertilizer as a regenerative input for improved biological nitrogen fixation and sustainable bush bean production. Front. Plant Sci. 15:1460599. doi: 10.3389/fpls.2024.1460599
Received: 06 July 2024; Accepted: 19 August 2024;
Published: 05 September 2024.
Edited by:
Roberto Ruggeri, University of Tuscia, ItalyReviewed by:
Andrew D. Cartmill, Massey University, New ZealandPhatu William Mashela, University of Limpopo, South Africa
Copyright © 2024 Chepkorir, Beesigamukama, Gitari, Chia, Subramanian, Ekesi, Abucheli, Rubyogo, Zahariadis, Athanasiou, Zachariadi, Zachariadis, Tenkouano and Tanga. This is an open-access article distributed under the terms of the Creative Commons Attribution License (CC BY). The use, distribution or reproduction in other forums is permitted, provided the original author(s) and the copyright owner(s) are credited and that the original publication in this journal is cited, in accordance with accepted academic practice. No use, distribution or reproduction is permitted which does not comply with these terms.
*Correspondence: Chrysantus M. Tanga, ctanga@icipe.org; Dennis Beesigamukama, dbeesigamukama@icipe.org