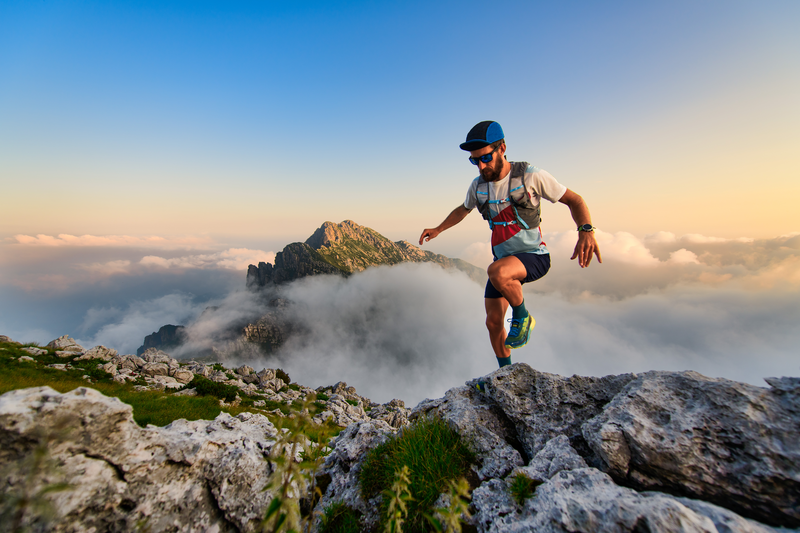
94% of researchers rate our articles as excellent or good
Learn more about the work of our research integrity team to safeguard the quality of each article we publish.
Find out more
ORIGINAL RESEARCH article
Front. Plant Sci. , 27 August 2024
Sec. Plant Nutrition
Volume 15 - 2024 | https://doi.org/10.3389/fpls.2024.1457694
Boron (B), an essential micronutrient for fruit development, also plays a crucial role in maintaining the shelf life of strawberries (Fragaria ananassa Duch.) by affecting cell wall structure and components. We investigated the distribution pattern of B within cells and cell walls in strawberry fruits under different B levels and revealed the relationship between the B distribution in cell walls and fruit firmness after harvesting. Foliar spraying of 0.1% H3BO3 promoted the growth of strawberry seedlings and improved fruit yield and flesh firmness by 45.7% and 25.6%. During the fruit softening and decay process, the content of bound B and cell wall-B decreased while more B was allocated to the protoplast and apoplast. The changes in B distribution in cells were attributed to cell damage during fruit decay, and B extended the freshness period of the fruits by alleviating the decrease of B distribution in cell walls. After leaving the fruits at room temperature for 10 h, the B content in different cell wall components significantly decreased, while foliar spraying of B alleviated the reduction of B content in covalently bound pectin (CBP), cellulose, and hemicellulose. Meanwhile, B spraying on fruit decreased the activity of cell wall degradation enzymes, including polygalacturonase (PG) and pectin lyase (PL), by 20.2% and 38.1%, while enhancing the demethylation of pectin by increasing pectin methylesterase (PME) activity from 21.6 U/g to 25.7 U/g. Thus, foliar spraying of 0.1% H3BO3 enhances the cross-linking of B with cell wall components and maintains cell wall structure, thereby prolonging the shelf life of strawberry fruits.
● Foliar spraying of boron improved flesh firmness and prolonged shelf life of strawberry fruits.
● Boron alleviated the decrease of B in CBP, cellulose and hemicellulose in fruits during fruit softening and decay.
● Boron inhibited cell wall degradation by affecting activities of PG, PL, and PME.
Strawberry (Fragaria ananassa Duch.) is one of the most widely cultivated and important berries in the world, known for its bright colors, unique flavors, and rich nutrient content (Zhang et al., 2022). It is often referred to as the “fruit queen” due to its significant nutritional and economic value. However, after harvest, strawberries have a short shelf life due to physiological factors such as low fruit firmness and high respiratory intensity. These factors make storage, preservation, transportation, and sales challenging, and they can adversely affect the flavor and nutritional value of the fruits (Chen et al., 2022). As a result, numerous scientists have devoted their attention to understanding the internal mechanisms underlying strawberry fruit ripening and softening, seeking ways to extend the postharvest preservation period of strawberries (Quesada et al., 2009; Li et al., 2020; Shi et al., 2023).
As an essential trace nutrient, boron (B) is crucial not only for the improvement of quality and yield (Aslihan et al., 2011; Singh et al., 2007; Wójcik and Mariusz, 2003), but also plays important roles in the formation and development of reproductive organs in plants (Iwai et al., 2006; Jiang et al., 2007). Numerous studies on apple, pear, tomato, dragon fruit, etc., have shown that application of B was effective in delaying fruit softening and enhancing post-harvest firmness by affecting the activities of cell wall degrading enzymes such as cellulase, pectate lyase (PL), β-galactosidase (β-Gal), polygalacturonase (PG), α-L-arabinofuranosidase (AFase), and pectin methyl esterase (PME) (Kobra et al., 2017; Mohebbi et al., 2020; Gholamnejad et al., 2023). Boron in plants exists in three different forms: water-soluble B, semi-bound B, and bound B (Du et al., 2002). Water soluble B is the main B form in the apoplast, while semi bound B is stored in cells, and bound B is mainly located in pectin polysaccharides in cell walls (Liu et al., 2018). The content and relative distribution of various forms of B in plants are related to plant tissues and external B supply levels. It is widely recognized that B in higher plants primarily binds to cell walls, holding great significance in maintaining the normal structure of cell walls (Matoh et al., 1996; Riaz et al., 2018; Wu et al., 2020). Several studies have demonstrated that B deficiency results in cell wall swelling and an increase in cell wall porosity (Ishii et al., 2001; Kaku et al., 2002). Thus, the distribution of different forms of B in cells and the distribution of B in different cell wall components may induce changes in fruit parenchyma and firmness by affecting fruit cell wall structure and major chemical components (Chen et al., 2011; Lin et al., 2020; Wang et al., 2018; Zhang et al., 2019). Currently, in the process of strawberry cultivation, there is a strong focus on nitrogen, phosphorus, and potassium fertilizers, while medium and trace element fertilizers are often neglected. Therefore, the distribution pattern of B within cells and cell walls in strawberry fruits remains unclear. Additionally, how the distribution pattern of B enhances firmness and prolongs the shelf life of post-harvested strawberry fruits is not yet understood.
This study aims to uncover the effects of foliar B on the different forms of B and the subcellular distribution pattern of B within strawberry fruits. Furthermore, we aimed to explore the crucial role of the B distribution pattern in delaying post-harvest ripening and softening of strawberries. Ultimately, the purpose of the present study was to provide scientific theoretical guidance and innovative ideas for the rational application of B fertilizers in strawberry cultivation.
This experiment was conducted in the solar greenhouse at Qingdao Agricultural University. The growth medium used was a composite matrix consisting of peat, vermiculite, and perlite mixed in a 2:1:1 ratio. After sterilizing the composite matrix with a 40% solution of Carbendazim (diluted 800 times) and covering it with plastic film for 8 days, the mixture was transferred into plastic pots of 30 cm in height, 20 cm in upper diameter, and 15 cm in bottom diameter). Strawberry seedlings exhibiting uniform growth (with a fresh weight of approximately 20 g and a diameter of 0.5 cm to 0.6 cm) were carefully selected and planted in the plastic pots. After transplantation, each pot was provided with 500 mL of nutrient solution lacking B, and throughout the management stage of the strawberry seedlings, 500 mL of B-free nutrient solution was applied every 10 days. The experiment included two distinct B treatments: control (CK) involving foliar spraying of distilled water and the B treatment involving foliar spraying of a 0.1% H3BO3 solution. Each treatment was replicated 10 times with 10 strawberry seedlings. Starting from the flowering period, strawberry leaves were treated with distilled water and 0.1% H3BO3 solutions every 7 days until the strawberries were harvested.
After measuring the soil and plant analyzer development (SPAD) value of 10 leaves from each treatment, the seedlings were separated into roots, leaves, and fruits. Initially, we measured the length of the root system using a graduated ruler and weighed the fresh weight of the roots, leaves, and fruits using an electronic balance. Subsequently, the roots, leaves, and a portion of the fruits were dried in an oven at 80°C until a constant weight was achieved. Lastly, the samples were ground, and additional measurements were taken after determining their dry weight using an electronic balance.
The fresh strawberry fruits of each B treatment were randomly divided into two portions. The firmness of one portion (10 fruits) was immediately determined using the GY-1 fruit firmness tester (with a probe diameter of 3.5 mm), and the firmness of the other portion (10 fruits) was determined after being left at room temperature for 10 h. Based on the outer edge of the fruit equator, two points of each fruit were measured using the GY-1 fruit firmness tester. Finally, the fruit firmness of each treatment was calculated based on the average value of 20 points.
According to the method previously reported by Liu et al. (2011), fruits after harvesting 0 h and 10 h were crushed, and immediately shaken and extracted with distilled water for 24 h at 25°C. The filtrate obtained after filtration was the test solution for water-soluble B (WSB). Then the residue was shaken and extracted with 1 mol/L NaCl for 24 h at 25°C, and the filtrate obtained after filtration was the test solution of semi-bound B (SBB) in the cytoplasm. Finally, the residue was shaken and extracted with 1 mol/L HCl for 24 h at 25°C, and the filtrate was the test solution for bound B (BB) in the cell walls.
Strawberry fruits after harvesting 0 h and 10 h were fractionated into cell walls, organelles, and cell soluble fractions using the method outlined by Weigel and Jäger (1980). After fresh fruits were finely ground in a precooled quartz mortar with a homogenate solution of sucrose (0.25 mmol/L), Tris–HCl (50 mmol/L), and dithiothreitol (1 mmol/L), the mixed solutions were centrifuged at 2,000×g for 10 min at 4°C and filtered using an 80 μm nylon sieve cloth. The precipitates were cell wall materials. The supernatants were then centrifuged at 12,000×g for 45 min at 4°C, and the fragments were organelles, whereas the supernatants were cell soluble fractions.
Following the approach previously described by Hoson et al. (2003), the cell wall materials were further divided into covalently bound pectin (CBP), ion-bound pectin (IBP), hemicellulose, and cellulose. First, the fully dried and ground cell walls were vibrated and extracted in 0.5 mol/L imidazole solution (pH 7.0) for 24 h at 25°C and centrifuged for 10 min (10,000×g). The supernatant was collected, and the residue continued to be vibrated and centrifuged two more times. The supernatant collected three times was defined as CBP. Then precipitates were continuously vibrated in 50 mmol/L Na2CO3 solution [containing 20 mmol/L trans-1, 2-cyclohexenediaminetetraacetic acid (CDTA)] for 24 h at 25°C and centrifuged for 10 min (10,000×g) for three times. The supernatant collected three times after centrifugation was defined as IBP. After adding 4 mol/L KOH solution (containing 0.1% NaBH), the precipitates were continuously extracted at 25°C for 3 h and centrifuged at 10,000×g for 10 min. The obtained supernatant was hemicellulose. Finally, the precipitate was washed with 0.03 mol/L acetic acid and alcohol and dried at 60°C to a constant weight, resulting in cellulose.
The ground samples of organs, subcellular fractions, cell wall components, and solutions of different forms of B were digested in concentrated HNO3 for 2 h at 200°C. Subsequently, the B content was determined using inductively coupled plasma mass spectrometry (ICP-MS) (NexIONTM 350X; PerkinElmer, MA, USA) as described by Wu et al. (2021).
Fresh fruits were collected and ground to measure the activity of polygalacturonase (PG), β-galactosidase (β-GAL), pectin lyase (PL), and PME using the available commercial PG-1-G, β-GALB-1-Y, PL-1-G and PME-2-G kits (Suzhou Comin Biotechnology Co., Ltd.), respectively.
The difference between control and B treatments was analyzed using Student’s t-test through SPSS 25.0, and * indicates a significant difference between the two B treatments at the level of P <0.05. The significance of differences in data between different B levels and different storage times of fruits was measured using analysis of variance (ANOVA) through SPSS 25.0 software, and lowercase letters indicate significant differences between different treatments at the level of P <0.05.
Compared to the control treatment, foliar spraying of 0.1% H3BO3 significantly enhanced the growth and development of strawberry seedlings, resulting in increased root length, SPAD value of leaves, fresh and dry weight of leaves and roots, as well as a higher fruit yield (Table 1). By applying B to the leaves, the fruit yield per plant increased by 45.7%.
In both the control or B treatment, the B content in leaves was the highest. Compared to the control, spraying strawberry leaves with 0.1% H3BO3 significantly increased the B content in roots, leaves, and fruits by 62.4%, 86.2%, and 128.6%, respectively (Figure 1), indicating a higher improvement of B content in strawberry fruits.
Figure 1. The B content in different organs of strawberry under different B levels. Note: n = 10. The data were presented as mean ± SE, and (a, b, c, d) indicate significant difference (p <0.05) between different organs and different B treatments.
The firmness of strawberry fruits was assessed both immediately after harvest and after allowing the fruits to rest at room temperature (22 °C–25 °C) for 10 h. As depicted in Figure 2A, after 10 h, the fruits without B spraying showed obvious decay, while the fruits with B spraying still maintained a fresh and healthy state. Furthermore, foliar spraying of B increased the firmness of both of newly harvested fruits and fruits that had been left for 10 h. The calculation results showed that 10 h after the fruits were harvested, the fruit firmness of the control decreased by 35.8%, while the fruit firmness of B treatment only decreased 19.3% (Figure 2B). The results indicated that foliar spraying of B may extend the storage time of strawberry fruits.
Figure 2. Strawberry fruit phenotype and firmness after harvest 0 h and 10 h under different B levels. (A) Just harvested strawberry fruits; (B) Strawberry fruits stored at room temperature for 10 h; (C) Changes of fruit firmness after storing for 10 h between different B treatment. Note: n = 10. The data were presented as mean ± SE, and (a, b, c) indicate significant differences (P <0.05) between different B treatments and harvest time.
To study the effects of foliar B application on B distribution in fruits, we determined the content of water-soluble B, semi-bounded B, and bounded B. Boron treatment increased the content of B in different forms, especially of bounded B in newly harvested fruits and fruits left for 10 h. However, after leaving the fruits at room temperature for 10 h, the content of bounded B significantly decreased, while the content of water-soluble B and semi bounded B increased (Table 2). By calculating the increase/decrease proportion of different forms of B, we found that foliar spraying of B alleviated the change in B distribution in fruits during storage.
The analysis of B content in various subcellular components (cell walls, organelles, and soluble fractions) of the fruits demonstrated a significant increase in B content in all these components upon the application of B through strawberry foliar spraying (Figure 3A). After leaving the fruits for 10 h, regardless of control or B treatment, the B content in cell walls and organelles decreased, while the B content in cell soluble fractions increased. However, B treatment reduced the change of B content in different cell fractions of fruits during storage (Figure 3A). Notably, the application of B led to a considerable improvement in the B distribution rate within the cell walls and inhibited the distribution of B to the organelles in newly harvested fruits and fruits stored for 10 h (Figure 3B). Furthermore, after storage for 10 h at room temperature, the B allocated in cell walls was released to the cell soluble fraction.
Figure 3. The B content (A) and distribution ratio (B) in subcellular level of fruits after harvest 0 h and 10 h under different B levels. Note: n = 6. The data were presented as mean ± SE, and (a, b, c, d) indicate significant differences (p <0.05) between different harvest times and different B treatments.
To ascertain the distribution of B among different cell wall components in strawberry fruits under varying B levels, the fruit cell walls were segregated into covalently bound pectin (CBP), ion-bound pectin (IBP), cellulose, and hemicellulose. Subsequently, the B content within these cell wall components was quantitatively determined using ICP-MS. As depicted in Figure 4, the foliar application of B substantially increased B content in CBP, IBP, cellulose, and hemicellulose. Meanwhile, after 10 h of storage at room temperature, the B content in different cell wall components decreased significantly, regardless of whether the fruits were in the control or B treatment group. Interestingly, compared to the control, foliar spraying of B alleviated the reduction of B content in CBP, cellulose, and hemicellulose in fruits after 10 h.
Figure 4. The B content in different cell wall components of strawberry fruits under different B levels. CBP and IBP represented covalently bound pectin and ion-bound pectin, respectively. n = 6. The data were presented as mean ± SE, and (a, b, c, d) indicate significant differences (p <0.05) between different harvest time and different B treatments.
Several cell wall-degrading enzymes, including PG, PL, PME, and β-Gal, contribute to fruit softening by influencing the composition and structure of the cell wall. The results in Figure 5 indicate that the application of B to strawberries significantly reduced the activity of PG and PL while increasing the activity of PME; however, it had no impact on β-Gal activity. The effects of B on PG and PL were helped mitigate pectin degradation and promote pectin’s demethylation modification. Increased PME can promote pectin demethylation and facilitate the linkage of B with the cell walls and maintenance of a stable cell wall structure, ultimately delaying the softening of strawberry fruits.
Figure 5. The pectinase activity in strawberry fruits under different B levels. (A) polygalacturonase (PG) activity; (B) pectin methylesterase (PME) activity; (C) β-galactosidase (β-Gal) activity; (D) pectin lyase (PL) activity. n = 6. The data were presented as mean ± SE. * indicates significant differences at P <0.05 level.
Boron (B), an essential nutrient for higher plants, contributes to increasing the yield and quality of fruits by promoting fruit setting, growth, and metabolism (Chouliaras et al., 2009; Abdollahi et al., 2011; Xu et al., 2021; Bons and Sharma, 2023). In this study, foliar spraying of 0.1% H3BO3 significantly enhanced plant growth and increased fruit fresh weight (Table 1). These results were mainly attributed to B’s crucial role in plant fertilization and metabolism (Fang et al., 2019; Michailidis et al., 2023). Research on various crops has found that the primary binding site for B is the cell walls (Chormova et al., 2014; Pan et al., 2012). Our study on strawberry fruits showed that the content of bounded B (BB) in cell walls was higher than that of water-soluble B (WSB) and semi-bounded B (SBB) (Table 2). The B content and B allocation in different subcellular fractions (Figure 3) also confirmed that more B was distributed to cell walls. In the meantime, B supplication resulted in a higher allocation proportion of cell wall-B in strawberry fruits whether harvested immediately or after 10 h at room temperature (Figure 3B), indicating that the distribution of B in different subcellular fractions and cell wall components varies under different external B supply levels (Feng et al., 2022). After 10 h, the content of bounded B and B in cell walls of fruits under different B levels decreased, and more B was released to the apoplasts and soluble fractions. However, the changes in B subcellular distribution were smaller in strawberry with foliar B spraying (Table 2 and Figure 3A). Promoted cross-linking of Ca with cell walls can help maintain normal cell wall structure (Liu et al., 2017; Wigati et al., 2023), which may significantly contribute to extending the storage time of fruits. It has been reported that differences of B allocation influence the cell wall structure and composition of plants (Liu et al., 2018; Wu et al., 2017). Thus, the binding of B to cell wall components may be also closely related to fruit softening (Figure 2). The degree of fruit ripening and softening is primarily assessed by fruit firmness. Foliar application of B to strawberries increased fruit firmness at initial harvest and notably delayed the decline in firmness after 10 h (Figure 1), which may contribute to the preservation and storage of strawberry fruits (Jiang et al., 2019).
Boron can bind to different cell wall components (pectin, cellulose, and hemicellulose), with pectin being the primary binding site for B in the cell walls (Yang et al., 2005; Liu et al., 2018). Our results showed that the B content in CBP was the highest in fruits across all treatments (Figure 4). The B allocation in the cell walls of strawberry fruits varied under different external B supply levels and was affected by storage time (Figure 3). However, it is unknown how foliar spraying of B influences the B content in different cell wall components in strawberry fruits and how it affects fruit softening. For strawberries, B spraying during plant growth stages promoted the cross-linking of B with different forms of pectin (IBP, CBP), cellulose, and hemicellulose (Figure 4). However, after 10 h at room temperature, the B content in different cell wall polysaccharides of fruits significantly decreased (Figure 4), suggesting cell wall degradation, disruption of reticular polymers, alterations in cell wall structure (Du et al., 2018; Wu et al., 2017), reduced cell tension, and ultimately, fruit softening (Bakshi and Ananthanarayan, 2020). Interestingly, compared to the control, B spraying slightly reduced the B content in IBP, CBP, cellulose, and hemicellulose of strawberry fruits after 10 h at room temperature (Figure 4). This indicates that foliar application of B to strawberries alleviated cell wall degradation and contributed to the preservation and storage of strawberry fruits (Mohebbi et al., 2020; Gholamnejad et al., 2023).
A variety of pectinases, including polygalacturonase (PG), β-galactosidase (β-Gal), pectin lyase (PL), and pectin methylesterase (PME), collectively impact the cross-linking structure among cell wall components (Saffer, 2018; Bajpai, 2022). The depolymerization caused by pectinases involved in cell wall degradation leads to the breakdown of cell wall components, disruption of reticular polymers, alterations in cell wall structure, reduced cell tension, and ultimately, fruit softening (Bakshi and Ananthanarayan, 2020). Investigations into PG gene expression across various fruits, such as strawberries (Shi et al., 2023), peaches (Ming et al., 2016), tomatoes (Yang et al., 2022), and figs (Wang et al., 2023), have substantiated the link between fruit softening and PG activity. Additionally, PG and PME work together within cell walls to facilitate fruit softening, as highly methylated pectin needs PME demethylation before being hydrolyzed by PG (Shi et al., 2023). However, the dynamics of PME activity during the softening process differ across various fruit types and varieties. For instance, PME activity progressively increases during the ripening and softening of bananas, while it decreases in papayas (Ali et al., 2004). The significant role of PL is to participate in the cleavage of pectin structure during fruit softening and ripening (Marín‐Rodríguez et al., 2002). The PL gene is regarded as a potential candidate for enhancing fruit hardness and impeding softening through molecular approaches (Jiménez-Bermúdez et al., 2002). Although numerous studies have explored inhibiting pectinase activity using exogenous substances to slow down fruit softening and flavor changes, such as procyanidin (Chen et al., 2022), 1-methylcyclopropene (Win et al., 2021), and calcium (Liu et al., 2017; Huang et al., 2023), early-season B spraying on apples did not affect the activity of PG, PME, PL, or β-Gal, but resulted in slight changes in fruit flesh firmness (Mohebbi et al., 2020). A study on tomatoes indicated that B application increased fruit firmness while decreasing pectin methylesterase activity (Gholamnejad et al., 2023). Previous studies have proposed that B promotes pectin synthesis and demethylation while inhibiting pectin degradation in rapeseed roots by suppressing the activity of pectinase enzymes (PG, PL, PME, and β-Gal) (Wu et al., 2020). For strawberries, B spraying during plant growth stages promoted the binding of cell wall-bound B in fruits of newly harvested and those left at room temperature for 10 h. This may be the result of the diminished activity of pectinases involved in pectin degradation (PG, PL) and promoted pectin demethylation (PME) in fruits (Figure 5).
The foliar application of 0.1% H3BO3 to strawberries effectively enhanced plant growth, fruit yield, and quality. Furthermore, B extended the shelf life of strawberry fruits by alleviating cell wall degradation, attributed to facilitated B cross-linking with pectin, cellulose, and hemicellulose, thereby aiding in the maintenance of cell wall structural integrity. These findings offer promising theoretical insights and innovative techniques for retarding the softening and deterioration of strawberry fruits while prolonging their post-harvest preservation period through the strategic use of B application.
The raw data supporting the conclusions of this article will be made available by the authors, without undue reservation.
LZ: Data curation, Funding acquisition, Writing – original draft. CS: Data curation, Funding acquisition, Writing – original draft. HT: Data curation, Methodology, Writing – original draft. JX: Data curation, Methodology, Writing – original draft. XW: Data curation, Writing – review & editing.
The author(s) declare financial support was received for the research, authorship, and/or publication of this article. This study was supported by the Natural Science Foundation of Shandong Province (ZR202102190565), the Start-up Fund for Introduced Talent of Qingdao Agricultural University (1121013).
The authors declare that the research was conducted in the absence of any commercial or financial relationships that could be construed as a potential conflict of interest.
All claims expressed in this article are solely those of the authors and do not necessarily represent those of their affiliated organizations, or those of the publisher, the editors and the reviewers. Any product that may be evaluated in this article, or claim that may be made by its manufacturer, is not guaranteed or endorsed by the publisher.
Abdollahi, M., Eshghi, S., Tafazzoli, E., Moosavi, N. (2011). Effects of paclobutrazol, boric acid and zinc sulfate on vegetative and reproductive growth of strawberry cv. selva. J. Agric. Sci. Technol. 14, 357–363.
Ali, Z. M., Chin, L. H., Lazan, H. (2004). A comparative study on wall degrading enzymes, pectin modifications and softening during ripening of selected tropical fruits. Plant Sci. 167, 317–327. doi: 10.1016/j.plantsci.2004.03.030
Aslihan, E., Turan, M., Gunes, A., Sambo, A. E. P. (2011). Boron application improves on yield and chemical composition of strawberry. Acta Agriculturae Scandinavica Section B-Soil Plant Sci. 61, 245–252. doi: 10.1080/09064711003776867
Bajpai, P. (2022). Regulation of cell-wall degrading enzymes production in fungi. Lignocellulosic Biomass in Biotechnology. Elsevier Inc. doi: 10.1016/C2019-0-04439-1
Bakshi, G., Ananthanarayan, L. (2020). Pectin degrading enzymes and their inhibitors in brined amla (Phyllanthus emblica) and lemon (Citrus limon L.) fruits during storage. Food Measure 14, 95–105. doi: 10.1007/s11694-019-00271-9
Bons, H. K., Sharma, A. (2023). Impact of foliar sprays of potassium, calcium, and boron on fruit setting behavior, yield, and quality attributes in fruit crops: a review. J. Plant Nutr. 46, 3232–3246. doi: 10.1080/01904167.2023.2192242
Chen, F., Liu, H., Yang, H., Lai, S., Cheng, X., Xin, Y. (2011). Quality attributes and cell wall properties of strawberries (fragaria annanassa duch.) under calcium chloride treatment. Food Chem. 126, 450–459. doi: 10.1016/j.foodchem.2010.11.009
Chen, J., Li, Y. X., Li, F. F., Hong, K. Q., Yuan, D. B. (2022). Effects of procyanidin treatment on the ripening and softening of banana fruit during storage. Scientia Hortic. 292, 110644. doi: 10.1016/j.scienta.2021.110644
Chormova, D., Messenger, D. J., Fry, S. C. (2014). Rhamnogalacturonan- II cross-linking of plant pectins via boron bridges occurs during polysaccharide synthesis and/or secretion. Plant Signaling Behav. 9, e28169. doi: 10.4161/psb.28169
Chouliaras, V., Tasioula, M., Chatzissavvidis, C., Therios, I., Tsabolatidou, E. (2009). The effects of a seaweed extract in addition to nitrogen and boron fertilization on productivity, fruit maturation, leaf nutritional status and oil quality of the olive (Olea europaea L.) cultivar Koroneiki. J. Sci. Food Agriculture 89, 984–988. doi: 10.1002/jsfa.3543
Du, C. W., Wang, Y. H., Xu, F. S. (2002). Study on boron forms and their relationship in rape cultivars with different boron efficiency. J. Plant Nutr. Fertilizers 8, 105–109. doi: 10.1006/jfls.2001.0409
Du, C. Q., WU, X. W., Yan, L., Liu, Y. L., Jiang, C. C. (2018). Effect of boron deficiency and low ph on cell wall components and boron distribution in cell of trifoliate root. Acta Hortic. Sinica 45, 1272–1282. doi: 10.16420/j.issn.0513-353x.2018-0050
Fang, K. F., Du, B. S., Zhang, Q., Xing, Y., Cao, Q. Q., Qin, L. (2019). Boron deficiency alters cytosolic Ca2+ concentration and affects the cell wall components of pollen tubes in Malus domestica. Plant Biol. 21, 343–351. doi: 10.1111/plb.12941
Feng, Y. M., Luo, G. T., Qu, M., Xuan, Z. Y., Li, X. W., Mai, J. W., et al. (2022). Effects of boron on aluminum adsorption and desorption of cell wall components of pea root tips. J. Plant Nutr. Fertilizers 28, 1893–1900. doi: 10.11674/zwyf.2022063
Gholamnejad, S., Haghighi, M., Etemadi, N., Pessarakli, M. (2023). Effects of boron on nutrient partitioning, Ca movement, and fruit quality of tomatoes. J. Plant Nutr. 46, 697–713. doi: 10.1080/01904167.2022.2071731
Hoson, T., Soga, K., Wakabayashi, K. (2003). Growth and cell wall changes in rice roots during spaceflight. Plant Soil 255, 19–26. doi: 10.1023/A:1026105431505
Huang, W. N., Shi, Y. N., Yan, H., Wang, H., Wu, D., Grierson, D., et al. (2023). The calcium-mediated homogalacturonan pectin complexation in cell walls contributes the firmness increase in loquat fruit during postharvest storage. J. Advanced Res. 49, 47–62. doi: 10.1016/j.jare.2022.09.009
Ishii, T., Matsunaga, T., Hayashi, N. (2001). Formation of rhamnogalacturonan II-borate dimer in pectin determines cell wall thickness of pumpkin tissue. Plant Physiol. 126, 1698–1705. doi: 10.1104/pp.126.4.1698
Iwai, H., Hokura, A., Oishi, M., Chida, H., Ishii, T., Sakai, S., et al. (2006). The gene responsible for borate cross-linking of pectin rhamnogalacturonan-II is required for plant reproductive tissue development and fertilization. Proc. Natl. Acad. Sci. 103, 16592–16597. doi: 10.1073/pnas.0605141103
Jiang, G. H., Xie, M., Fang, L., Wang, H. R., Wu, Y. J., Sun, C. B. (2007). Effects of boron, calcium, pesticide and germicide on pollen germinationand tube growth of strawberry. J. Fruit Sci. 24, 234–236. doi: 10.3969/j.issn.1009-9980.2007.02.023
Jiang, Y. L., Yu, L., Hu, Y. W., Zhu, Z. C., Zhuang, C. J., Zhao, Y. Y., et al. (2019). Electrostatic spraying of chitosan coating with different deacetylation degree for strawberry preservation. Int. J. Biol. Macromolecules 139, 1232–1238. doi: 10.1016/j.ijbiomac.2019.08.113
Jiménez-Bermúdez, S., Redondo-Nevado, J., Muñoz-Blanco, J., Caballero, J. L., López-Aranda, J. M., Valpuesta, V. (2002). Manipulation of strawberry fruit softening by antisense expression of a pectate lyase gene. Plant Physiol. 128, 751–759. doi: 10.1104/pp.010671
Kaku, T., Tabuchi, A., Wakabayashi, K., Kamisaka, S., Hoson, T. (2002). Action of xyloglucan hydrolase within the native cell wall architecture and its effect on cell wall extensibility in azuki bean epicotyls. Plant Cell Physiol. 43, 21–26. doi: 10.1093/pcp/pcf004
Kobra, K., Nima, A., Mohammad, S. (2017). Improvement of postharvest quality of asian pear fruits by foliar application of boron and calcium. Horticulturae 3, 15. doi: 10.3390/horticulturae3010015
Li, B. J., Grierson, D., Shi, Y. N., Chen, K. S. (2020). Roles of abscisic acid in regulating ripening and quality of strawberry, a model non-climacteric fruit. Horticulture Res. 9, uhac089. doi: 10.1093/hr/uhac089
Lin, Y., Lin, H., Wang, H., Lin, M., Lin, Y. (2020). Effects of hydrogen peroxide treatment on pulp breakdown, softening, and cell wall polysaccharide metabolism in fresh longan fruit. Carbohydr. Polymers 242, 116427. doi: 10.1016/j.carbpol.2020.116427
Liu, H., Chen, F., Lai, S., Tao, J., Yang, H., Jiao, Z. (2017). Effects of calcium treatment and low temperature storage on cell wall polysaccharide nanostructures and quality of postharvest apricot (prunus Armeniaca). Food Chem. 225, 87–97. doi: 10.1016/j.foodchem.2017.01.008
Liu, G. D., Hu, P., Zhang, J. H., Zhou, G. F., Zeng, Y., Zhong, B. L., et al. (2018). Effect of boron deficiency on boron distribution in different plant parts and boron concentration in leaf cell wall components in navel orange plants. J. Plant Nutr. Fertilizers 24, 179–186. doi: 10.11674/zwyf.17107
Liu, G. D., Jiang, C. C., Wang, Y. H., Peng, S. A., Zeng, Q. L. (2011). Effect of boron on leaf boron forms of Newhall navel orange grafted on two rootstocks. Scientia Agricultura Sinica 44, 982–989. doi: 10.3864/j.issn.0578-1752.2011.05.015
Marín-Rodríguez, M. C., Orchard, J., Seymour, G. B. (2002). Pectate lyases, cell wall degradation and fruit softening. J. Exp. Bot. 53, 2115–2119. doi: 10.1093/jxb/erf089
Matoh, T., Kawaguchi, S., Kobayashi, M. (1996). Ubiquity of a borate rhamnogalacturonan II complex in the cell walls of higher plants. Plant Cell Physiol. 37, 636–640. doi: 10.1093/oxfordjournals.pcp.a028992
Michailidis, M., Bazakos, C., Kollaros, M., Adamakis, I. D. S., Ganopoulos, I., Molassiotis, A., et al. (2023). Boron stimulates fruit formation and reprograms developmental metabolism in sweet cherry. Physiologia Plantarum 175, e13946. doi: 10.1111/ppl.13946
Ming, Q., Yike, Z., Xiangyan, Y., Mingyu, H., Jinjin, L., Fang, L. (2016). Identification and expression analysis of polygalacturonase family members during peach fruit softening. Int. J. Mol. Sci. 17, 1933. doi: 10.3390/ijms17111933
Mohebbi, S., Babalar, M., Zamani, Z., Askari, M. A. (2020). Influence of early season boron spraying and postharvest calcium dip treatment on cell-wall degrading enzymes and fruit firmness in “starking delicious” apple during storage. Scientia Horticulturae 259, 108822. doi: 10.1016/j.scienta.2019.108822
Pan, Y., Wang, Z. H., Yang, L., Wang, Z. F., Shi, L., Naran, R., et al. (2012). Differences in cell wall components and allocation of boron to cell walls confer variations in sensitivities of Brassica napus cultivars to boron deficiency. Plant Soil 354, 383–394. doi: 10.1007/s11104-011-1074-6
Quesada, M. A., Blanco-Portales, R., Posé, S., García-Gago, J. A., Jiménez-Bermúdez, S., Muñoz-Serrano, A., et al. (2009). Antisense down-regulation of the FaPG1 gene reveals an unexpected central role for polygalacturonase in strawberry fruit softening. Plant Physiol. 150, 1022–1032. doi: 10.1104/pp.109.138297
Riaz, M., Yan, L., Wu, X. W., Hussain, S., Aziz, O., Jiang, C. C. (2018). Boron deprivation induced inhibition of root elongation is provoked by oxidative damage, root injuries and changes in cell wall structure. Environ. Exp. Botany 156, 74–85. doi: 10.1016/j.envexpbot.2018.08.032
Saffer, A. M. (2018). Expanding roles for pectins in plant development. J. Integr. Plant Biol. 60, 910–923. doi: 10.1111/jipb.12662
Shi, Y. N., Li, B. J., Grierson, D., Chen, K. S. (2023). Insights into cell wall changes during fruit softening from transgenic and naturally occurring mutants. Plant Physiol. 192, 1671–1683. doi: 10.1093/plphys/kiad128
Singh, R., Sharma, R. R., Tyagi, S. K. (2007). Pre-harvest foliar application of calcium and boron influences physiological disorders, fruit yield and quality of strawberry (Fragaria× ananassa Duch.). Scientia Hortic. 112, 215–220. doi: 10.1016/j.scienta.2006.12.019
Wang, Y., Fan, Z., Zhai, Y., Huang, H., Vainstein, A., Ma, H. (2023). Polygalacturonase gene family analysis identifiesfcpg12as a key player in fig (Ficus Carical.) fruit softening. BMC Plant Biol. 23, 320. doi: 10.1186/s12870-023-04315-7
Wang, D., Yeats, T. H., Uluisik, S., Rose, J. K. C., Seymour, G. B. (2018). Fruit softening:Revisiting the role of pectin. Trends Plant Sci. 23, 302–310. doi: 10.1016/j.tplants.2018.01.006
Weigel, J. J., Jager, H. J. (1980). Subcellular distribution and chemical form of cadmium in bean plant. Plant Physiol. 65, 480–482. doi: 10.1104/pp.65.3.480
Wigati, L. P., Wardana, A. A., Tanaka, F. (2023). Strawberry preservation using combination of yam bean starch, agarwood Aetoxylon bouya essential oil, and calcium propionate edible coating during cold storage evaluated by TOPSIS-Shannon entropy. Prog. Organic Coatings 175, 107347. doi: 10.1016/j.porgcoat.2022.107347
Win, N. M., Yoo, J., Naing, A. H., Kwon, J. G., Kang, I. K. (2021). 1-methylcyclopropene (1-MCP) treatment delays modification of cell wall pectin and fruit softening in “hwangok” and “picnic” apples during cold storage. Postharvest Biol. Technol. 180, 180. doi: 10.1016/j.postharvbio.2021.111599
Wójcik, P., Mariusz, L. (2003). Effect of calcium and boron sprays on yield and quality of “Elsanta” strawbethem. J. Plant Nutr. 26, 671–682.
Wu, X. W., Riaz, M., Du, C. Q., Yan, L., Jiang, C. C. (2017). Boron deficiency in trifoliate orange induces changes in pectin composition and architecture of components in root cell walls. Front. Plant Sci. 8, 1882. doi: 10.3389/fpls.2017.01882
Wu, X. W., Song, H. X., Guan, C. Y., Zhang, Z. H. (2020). Boron alleviates cadmium toxicity in brassica napus by promoting the chelation of cadmium onto the root cell wall components. Sci. Total Environment 728, 138833. doi: 10.1016/j.scitotenv.2020.138833
Wu, X. W, Tian, H, Li, L, Guan, C. Y, Zhang, Z. H (2021). Cd-accumulating oilseed rape has stronger cd tolerance due to stronger cd fixation in pectin and hemicellulose and higher cd chelation. Environ pollut 285, 117218. doi: 10.1016/j.envpol.2021.117218
Xu, W., Wang, P., Yuan, L., Chen, X., Hu, X. (2021). Effects of application methods of boron on tomato growth, fruit quality and flavor. Horticulturae 7, 223. doi: 10.3390/horticulturae7080223
Yang, Y., Lu, L., Sun, D. (2022). Fungus polygalacturonase-generated oligogalacturonide restrains fruit softening in ripening tomato. J. Agric. Food Chem. 3, 70. doi: 10.1021/acs.jafc.1c04972
Yang, Y. H., Yu, M., Du, C. W., Wu., L. S., Wang, Y. H. (2005). Boron distribution in cell wall components in rape cultivars (Brassica napus) with different boron use efficiency. Acta Agronomica Sinica 31, 608–611. doi: 10.3321/j.issn:0496-3490.2005.05.014
Zhang, T., Tang, H., Vavylonis, D., Cosgrove, D. J. (2019). Disentangling loosening from softening: insights into primary cell wall structure. Plant J. 100, 1101–1117. doi: 10.1111/tpj.14519
Keywords: boron, strawberry, fruit softening, cell wall, subcellular distribution
Citation: Zhang L, Sun C, Tian H, Xu J and Wu X (2024) Foliar spraying of boron prolongs preservation period of strawberry fruits by altering boron form and boron distribution in cell. Front. Plant Sci. 15:1457694. doi: 10.3389/fpls.2024.1457694
Received: 01 July 2024; Accepted: 02 August 2024;
Published: 27 August 2024.
Edited by:
Haijun Gong, Northwest A&F University, ChinaReviewed by:
Dayan Sanhueza, Andres Bello University, ChileCopyright © 2024 Zhang, Sun, Tian, Xu and Wu. This is an open-access article distributed under the terms of the Creative Commons Attribution License (CC BY). The use, distribution or reproduction in other forums is permitted, provided the original author(s) and the copyright owner(s) are credited and that the original publication in this journal is cited, in accordance with accepted academic practice. No use, distribution or reproduction is permitted which does not comply with these terms.
*Correspondence: Xiuwen Wu, d3V4aXV3ZW4wNjA1QDE2My5jb20=
†These authors have contributed equally to this work
Disclaimer: All claims expressed in this article are solely those of the authors and do not necessarily represent those of their affiliated organizations, or those of the publisher, the editors and the reviewers. Any product that may be evaluated in this article or claim that may be made by its manufacturer is not guaranteed or endorsed by the publisher.
Research integrity at Frontiers
Learn more about the work of our research integrity team to safeguard the quality of each article we publish.