- 1College of Agronomy, Gansu Agricultural University, Gansu, China
- 2Gansu Key Laboratory of Arid Habitat Crop Science, Gansu Agricultural University, Gansu, China
- 3Science and Technology R&D Department, China Chinese Medicine Co., LTD, Beijing, China
Introduction: Glycyrrhiza uralensis is a perennial medicinal plant. It’s generally cultivated for three years, and should avoid long-term continuous cultivation. However, unreasonable crop rotation and extensive fertilization are common in G. uralensis cultivation, which leads to the imbalance of soil microflora structure, and the obstacle of continuous cropping are becoming increasingly serious. Some microbial fertilizers such as Bacillus amyloliquefaciens, Bacillus subtilis, and complex microbial agent have the advantage of regulating soil microbial community structure and improving the soil environment. Therefore, these three kinds of microbial fertilizers were applied to G. uralensis and their effects on soil microorganisms of G. uralensis were studied.
Methods: Combine microbial fertilizers with conventional fertilization for continuous cropping and rotating G. uralensis. High-throughput sequencing technology was used to determine soil microbial richness, diversity and distribution of community structure in rotating and continuous cropping G. uralensis.
Results and discussion: Continuous cropping reduced G. uralensis soil bacterial diversity by 7.56% and increased fungal richness by 17.01% compared with crop rotation. However, after the application of microbial fertilizers, the fungal richness and diversity of continuous cropping G. uralensis were significantly reduced by 4.76%~20.96%, and the soil bacterial diversity of continuous cropping and rotating G. uralensis was significantly increased by 7.22%~12.03% and 6.75%~11.69% compared with the respective controls, respectively. Continuous cropping and rotating G. uralensis soil dominant bacteria mainly include Proteobacteria, Actinobacteria and Gemmatimonadota, and the dominant fungi include Ascomycota, Basidiomycota and Zygomycota. The activity process of these microbial communities was mainly through carbohydrate metabolism and amino acid synthesis pathway in metabolism. The complex microbial agent significantly increased the relative abundance of soil dominant bacteria communities of continuous cropping and rotating G. uralensis by 3.11~11.54 percentage points, and significantly reduced the relative abundance of soil dominant fungal communities of continuous cropping G. uralensis by 1.57~8.93 percentage points, compared with the control. Of the three microbial fertilizers, the complex microbial agent had the most significant effects on optimizing the soil microbial community structure of continuous cropping and rotating G. uralensis. Conclusion: the application effect of different microbial fertilizers in continuous cropping G. uralensis was better than crop rotation, and the application effect of complex microbial agent was the best, which has more application value and development prospect in the cultivation management of G. uralensis.
1 Introduction
Glycyrrhiza uralensis Fisch is a perennial root plant of Leguminosae Glycyrrhiza L., with root and root stem as medicine, is also widely used in food, health care products, daily chemical industry, animal husbandry and other industries (NPC., 2020). G. uralensis has the characteristics of drought and cold resistance, salt and alkali resistance, and not easy to be covered by sand. It is an excellent plant for windbreak and sand fixation and improved salt-alkali soil in the desert and semi-desert area of northwest China. It has both economic and ecological value (Ji et al., 2024). Nowadays, the reserves of wild G. uralensis resources are decrease sharply, but the demand for G. uralensis is increasing year by year. The wild G. uralensis resources distributed in China have been unable to meet the increasing market demand, and the market contradiction of G. uralensis resources in short supply is becoming increasingly prominent (Yu et al., 2022). This situation promotes the continuous expansion of the cultivation area of G. uralensis in China, and the G. uralensis cultivated in various producing areas has developed into the main supply source of medicinal G. uralensis (Zhi et al., 2022). However, the traditional G. uralensis cultivation technology and management process are relatively extensive, and there are some problems in the planting process, such as not paying attention to reasonable crop rotation and blind application of chemical fertilizers (Ting et al., 2023). These problems lead to the imbalance of soil microbial community structure, the frequent occurrence of soil-borne diseases, and the decline of soil integrated environment, which seriously restricts the green and sustainable development of G. uralensis cultivation (Xin et al., 2022).
In plant-soil-microbe systems, one side, functional strains in microbial fertilizers can modulate signaling pathways, increase gene expression, promote proline synthesis, and inhibit the growth of pathogens (Chaudhary et al., 2022). On the other side, the beneficial strains in microbial fertilizers can form a dominant bacterial community locally in the soil, guide the development of environmental microbiota to a benign direction, so as to facilitate soil organic matter decomposition and nutrient transformation, enhance the absorption of nutrients with plants, and promote plant growth (Zaeem et al., 2019). On the contrary, the pores of soil particles provide contact points for microbial colonization, and the available nutrients such as soil nitrogen, phosphorus and potassium improve the nutrients for soil microorganisms, ensuring the reproduction and growth of microorganisms in microbial fertilizers (Vieira et al., 2020; Deepak et al., 2016).
At present, the rational application of microbial fertilizer has been proved to be an effective measure to balance the structure of crop soil microflora, enhance crop disease resistance, and improve crop yield and quality (Plocek et al., 2024). For instance, foliar sprays of different microbial fertilizers have a positive effect on the growth of Glycine max (L.) Merr. and Zea mays L., including increased enzyme activity, metabolites, nutrient concentration, mitigated effects of salinity, and increased biomass (Vela et al., 2018; Guimaraes et al., 2020). Chinese cabbage with different Trichoderma fungi applied via irrigation resulted in a significant increase in cabbage yield (by 37%) and enhanced enzyme activity in the soil (Ji et al., 2020). In addition, various strains of Bacillus subtilis, Bacillus megaterium, and Bacillus pumilus have resulted in rate of growth increases, stress tolerances, and nutrient metabolites in lettuce and tomatoes. These studies provide the theoretical basis and application ideas for the application of microbial fertilizers in the planting process of G. uralensis.
However, the research and application of microbial fertilizers mainly focus on vegetables, Triticum aestivum L., Zea mays L., and other crops, its influence on the soil microbiological environment of medicinal plants such as G. uralensis is rarely systematically reported (Bao et al., 2024). Therefore, in this study, different types of microbial fertilizers were applied in continuous cropping and rotating G. uralensis based on conventional fertilization. By analyzing the results of high-throughput sequencing, this study compared the microbial richness, diversity, community structure and metabolic pathways of continuous cropping and rotating G. uralensis. Explored the influence of different microbial fertilizers on the soil microorganisms of rotating and continuous cropping G. uralensis, and screened the microbial fertilizers with the best application effect. It is expected to provide effective prevention and control measures to alleviate the obstacles of G. uralensis and provide theoretical basis for the application of microbial fertilizers in the cultivation of G. uralensis.
2 Materials and methods
2.1 Experimental site and basic agrochemical characteristics of soil
The experimental site is located in Changning Town, Minqin County, Gansu Province, China (102° 24′ 55″ E, 38° 53′ 31″ N, elevation of 1 302.0 m). This area belongs to the temperate continental arid climate area, with an annual average temperature of 8.5°C, annual average precipitation of 113.2 mm, average evaporation of 2 412 mm, frost-free period of 162 d. The soil is sandy loam soil, irrigation is convenient, suitable for planting G. uralensis and other rhizome medicinal materials.
In 2019 and 2020, G. uralensis was planted in the continuous experimental fields, and on July 30, 2021, the continuous cropping G. uralensis was harvested. From May to October 2020, Zea mays L. was planted in crop rotation experimental fields, from May to July 2021, Citrullus lanatus was planted here, and on July 30, 2021, Citrullus lanatus was harvested. The soil base fertility (0~20 cm soil layer) in the continuous cropping experimental site was soil organic matter 17.06 g/kg, total nitrogen 12.49 g/kg, total phosphorus 0.29 g/kg, total potassium 5.05 g/kg, ammonium nitrogen 18.97 mg/kg, available phosphorus 16.45 mg/kg, available potassium 142.54 mg/kg. The soil base fertility in the rotation experimental site was soil organic matter 21.12 g/kg, total nitrogen 14.88 g/kg, total phosphorus 0.33 g/kg, total potassium 5.71 g/kg, ammonium nitrogen 22.16 mg/kg, available phosphorus 11.21 mg/kg, available potassium 113.04 mg/kg.
2.2 Experimental materials
G. uralensis seed: provided by China Pharmaceutical Seed Co., LTD., was identified as the G. uralensis Fisch seed by associate professor Qiu Daiyu of Gansu Agricultural University in Gansu, China.
Microbial fertilizers: Bacillus amyloliquefaciens (powder, the effective number of viable bacteria exceeds 1×1010/g), and the main species are Bacillus amyloliquefaciens and its metabolites. Bacillus subtilis (granules, the effective number of viable bacteria exceeds 1×1010/g), and the main species are Bacillus subtilis and its metabolites. Both fertilizers were produced by Jiangxi Weizhixing Biological Technology Co., LTD (Jiangxi, China). Complex microbial agent (liquid, the effective number of viable bacteria exceeds 1×1010/mL), and the main strains are photosynthetic bacteria, lactic acid bacteria, yeast and three major bacterial groups of multiple microorganisms. It was produced by Sinochem Agricultural Ecological Science and Technology Co., LTD (Hubei province, China).
Chemical fertilizers: Diammonium phosphate is a granule (N~P2O5~K2O: 18~46~0, total nutrient exceeds 64.0%), which was produced by Yunnan Xiangfeng Chemical Fertilizer Co., LTD (Yunnan province, China). Compound fertilizer is also a granule (N~P2O5~K2O: 15~15~15), which was produced by Fujian Sinochem Zhisheng Chemical Fertilizer Co., LTD (Fujian province, China).
2.3 Experimental design
In the experiment, split block design was adopted, and the primary area has two kinds of crop rotation and continuous cropping. Four fertilization treatments in the secondary area, namely conventional fertilization (CK), Bacillus amyloliquefaciens (J), Bacillus subtilis (K) and complex microbial agent (F). Three replicates of each treatment, with a total of 24 plots, and the plot area is 5 m ×3 m, and a 1 m-wide protection line was set around the plot.
On August 3, 2021, G. uralensis was sown in continuous cropping and rotation experiment site, and plant spacing 15 cm, line spacing 25 cm, sowing depth 2.5~3.0 cm, 5~7 grains per hole, and after G. uralensis seedling emergence, each hole was reserved with 2 seedlings. Before sowing, the base fertilizer (diammonium phosphate, compound fertilizer) and microbial fertilizers were applied in combination with rotary tillage. Among them, Bacillus amyloliquefaciens and Bacillus subtilis were mixed with base fertilizers and applied at one time. The complex microbial agent is liquid, the stock solution is diluted to 800 times, and applied when irrigation. The amount of fertilization is shown in Table 1. In the second and third year, after the G. uralensis seedlings grow, and on May 20, July 20 and September 20, for the crop rotation and continuous cropping G. uralensis irrigation. Before irrigation, the control group applied topdressing fertilizer, and the treatment groups applied both topdressing fertilizer and microbial fertilizers simultaneously, and the amount of fertilization was the same as that of base fertilizers and microbial fertilizers during sowing.
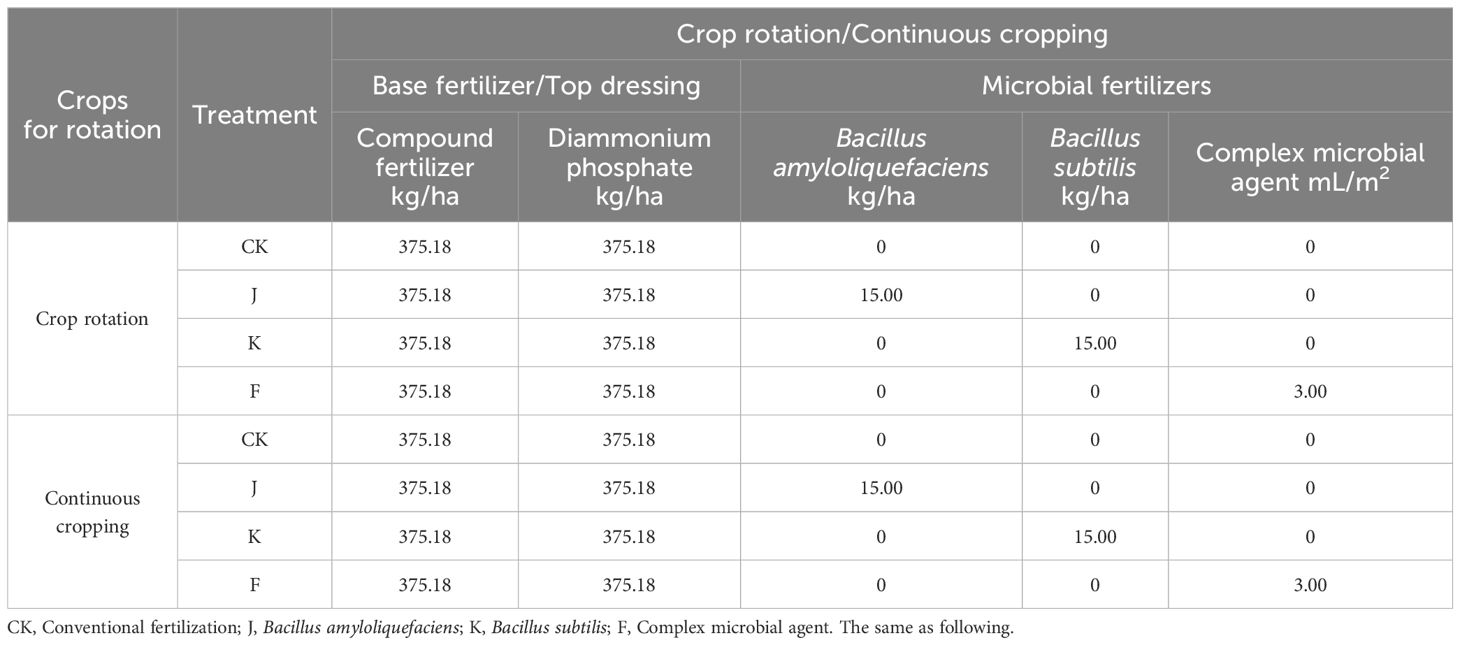
Table 1. Fertilization amount under different treatments in experimental plots of rotating and continuous cropping G. uralensis.
2.4 Soil sample collection
15 July 2021 and 25 May, 28 July, and 28 September 2023, utilizing the five-point sampling method, five G. uralensis plants without pests and diseases were randomly selected in each plot, near the G. uralensis roots, surface litter and debris were removed. Using a sterile shovel (20 cm long, 15 cm wide), soil samples were collected at depth of 0~30 cm. After mixed the five portions of the G. uralensis soil from the same plot thoroughly, the soil was sieved through a 2 mm sieve. Soil samples collected on July 15, 2021, were air-dried, sieved through a 0.15 mm sieve, and stored at room temperature for soil nutrient analysis. Other soil samples were placed in a sterile cryotube, taken back to the laboratory, and stored at -80°C for soil microbial biomass determination.
2.5 Soil nutrient and microbiological measurement
2.5.1 Soil nutrient content determination
Soil properties were determined according to Soil Agricultural Chemistry Analysis (Bao, 2000). The soil organic matter content was analyzed by potassium dichromate titration. The total nitrogen and ammonium nitrogen were assessed by half trace chemical nitrogen method and 2 mol/L KCl extraction-indiophol blue color method. The total phosphorus and available phosphorus were measured using NaOH melting-molybdenum antimony colorimetry and NaHCO3 extraction-molybdenum antimony colorimetry. The total potassium and available potassium were determined by NaOH melting-flame photometry and ammonium acetate extraction-flame photometry.
2.5.2 Analysis of rhizosphere soil microbial richness and diversity
1) Extraction of total DNA from soil samples: extraction was performed according to the MOBIO® Laboratories kit, and total DNA purity and concentration were detected by the Quanti Fluor TM-ST blue fluorescence quantification system and 2% agarose gel electrophoresis.
2) PCR amplification: The universal primers F: 5’-ACTCCTACGGGAGGCAGCA-3’ and R: 5’- GGACTACHVGGGTWTCTAAT-3’ were employed for PCR amplification of the bacterial 16S rRNA gene 16S V3-V4 region, and the universal primers F: 5’- GGAAGTAAAAGTCGTAACAAG G-3’ and R: 5’-GCTGCGTTCTTCATCGATGC-3’ were used for PCR amplification of the fungal ITS gene ITS-V1 region. The amplification procedure was 94°C predenaturation for 5 min for another 30 cycles (94°C was denatured for 30 s, 56°C was annealed for 30 s, and 72°C was extended for 30 s), and extend at 72°C for 7 min.
3) High-throughput sequencing: The purified amplified genes were sequenced on the Illumina PE250 platform. The resulting data were first preprocessed, and then the chimeras and short sequences were removed according to the positive and negative barcode sequence and the primer sequences to obtain the final effective sequence. Cluster analysis of valid sequences using the Qiime platform at the 97% similarity level and taxonomic annotation for each OTU (Operational Taxonomic Units) obtained.
4) Calculate soil microbial richness and diversity indices: Chao1 index was used to reflect community species richness, and Shannon index indicates species diversity.
Where the predicted number of OTUs is SChao1; The number of OTUs actually observed is expressed in Sobs: n1 represents the number of OTUs with only one sequence, and n2 represents the number of OTUs with two sequences (Zhu and Yu, 2022).
Where S is the total number of microbial species in the sample and i represents the ith species; The relative abundance of microbial species i in a sample is represented by Pi, and its formula is as follows: , where N is the total number of individuals of all microbial species in a given sample and ni is the number of individuals of species i (Zhu et al., 2018).
2.6 Statistical analysis
One-way analysis of variance (ANOVA) was conducted using SPSS 27.0 software (SPSS Inc., Chicago, IL, USA) with a significance level of difference set at P<0.05, and multiple comparisons were carried out using the Duncan method. Cluster analysis used the Paiseno Gene Cloud platform (https://www.genescloud.cn/login) with 97% similarity threshold as the standard, and the chart production of the cluster analysis was made using the “Heat Map Dendrogram” tool in the Origin 2021 software (Origin Lab, Northampton, MA, USA). The Venn analysis was executed with the aid of the “Venn Diagram” tool. Additionally, the “vegan” R package (version 3.6.3) was employed to conduct principal coordinate analysis (PCoA) based on the Bray-Curtis distance (The R Foundation for Statistical Computing, Vienna, Austria).
3 Results and analysis
3.1 Analysis of the sequencing results for soil bacteria and fungi
For each treatment, the coverage of bacteria and fungi in rotating and continuous cropping G. uralensis was above 98%. This showed that the sequencing depth of bacteria and fungi can cover the vast majority of bacteria and fungi in the soil, and only a few bacterial and fungal species are undiscovered. The sequencing results could reflect the microbial community structure composition of rotating and continuous cropping G. uralensis in the experiment, and the data cuuld be used for subsequent analysis (Table 2).
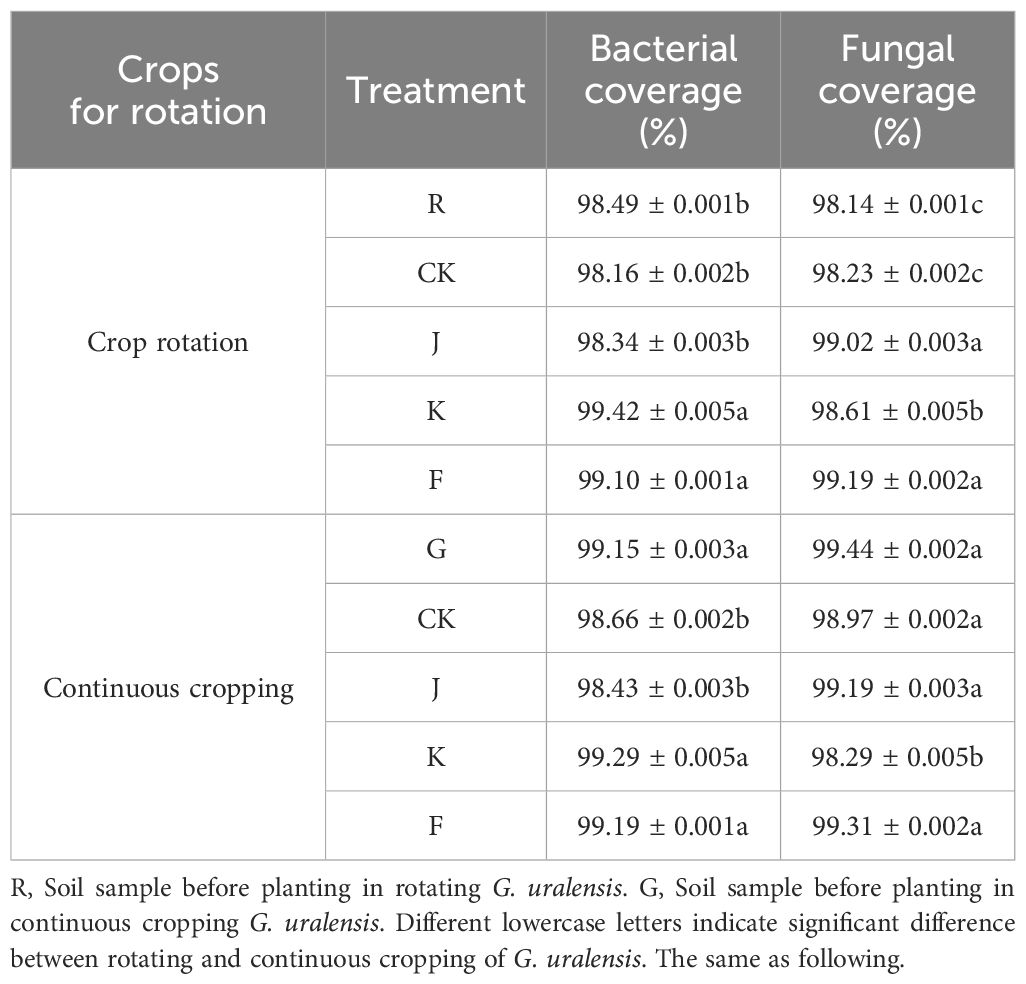
Table 2. Bacterial and fungal sequencing coverage of soil samples of rotating and continuous cropping G. uralensis under different treatments.
Under different treatments, a total of 722,142 valid sequences were obtained from rotating G. uralensis soil bacterial libraries, and 43,329 optimized sequences were obtained after filtering and optimization. OTU clustering of the optimized sequences based on the 97% similarity level yielded an average of 32,401 bacterial OTU. Rotation of G. uralensis soil fungal libraries yielded 750,957 valid sequences, 716,365 optimized sequences, and clustered 3,598 fungal OTU. Among them, the number of bacterial and fungal optimized sequences and OTU number of rotating G. uralensis were significantly different between the J, K, F and CK treatment. Continuous cropping G. uralensis soil bacterial libraries yielded 742,400 valid sequences, 468,168 optimized sequences, and clustered 33,342 fungal OTU, and its fungal libraries yielded 732,143 valid sequences, 704,599 optimized sequences and 3,054 fungal OTU. Among them, J, K and F treatments significantly reduced the number of soil fungi OTU in continuous cropping G. uralensis (Table 3).
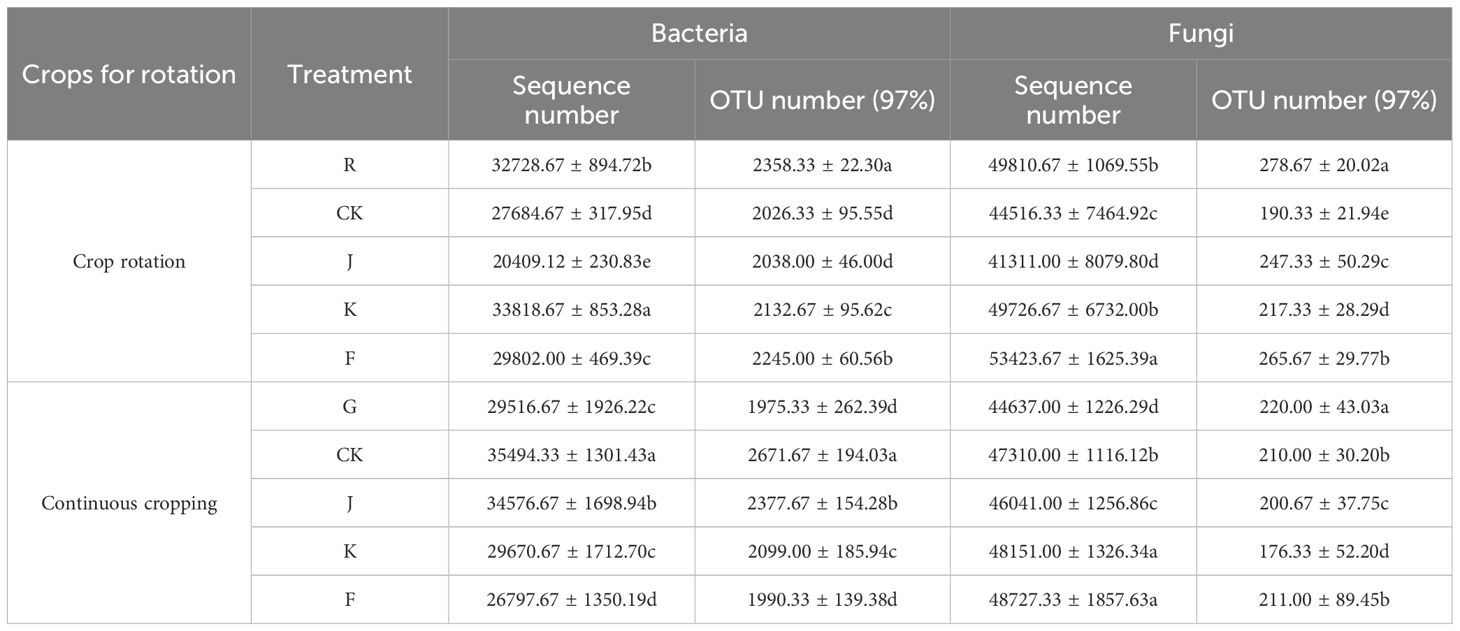
Table 3. Optimized sequences and OTU numbers of soil bacteria and fungi in rotating and continuous cropping G. uralensis under different treatments.
Used the rRNA gene sequence database and the UNITE fungal molecular identification database as the reference databases, the OTU sequences in rotating and continuous cropping G. uralensis soil samples were taxonomic annotated, and the species taxonomic information corresponding to each OTU is shown in Table 4. In rotating G. uralensis soil, the detected bacteria included 17 phyla, 36 classes, 58 orders, 62 families, 65 genera, 1991 species, and the fungi included 6 phyla, 13 classes, 22 orders, 33 families, 43 genera and 405 species. In continuous cropping G. uralensis soil, the detected bacteria included 14 phyla, 29 classes, 56 orders, 65 families, 72 genera, 1700 species; and the fungi included 5 phyla, 12 classes, 21 orders, 30 families, 37 genera, 362 species. Among them, the number of bacteria and fungi of rotating G. uralensis is more than that of continuous cropping G. uralensis.

Table 4. Statistical table of soil bacteria and fungi species in rotating and continuous cropping G. uralensis.
The common and unique OTU numbers of soil bacteria and fungi from different treatments are shown in Venn figure (Figures 1, 2). For soil bacteria, the number of common bacterial OTU between soil sample before planting G. uralensis in rotating test site (R), CK, J, K and F treatments was 1226, and the number of unique bacterial OTU was 465, 382, 426, 259 and 472, respectively. Among them, J and K treatment significantly increased the number of unique OTU soil bacteria in rotating G. uralensis. The number of common bacterial OTU between soil sample before planting G. uralensis in continuous cropping test site (G), CK, J, K and F treatments was 1339, and the number of unique bacterial OTU was 267, 278, 271, 376 and 336, respectively. Compared with CK treatment, K and F treatment can increase the unique OTU number of soil bacteria with continuous cropping G. uralensis (Figure 1).
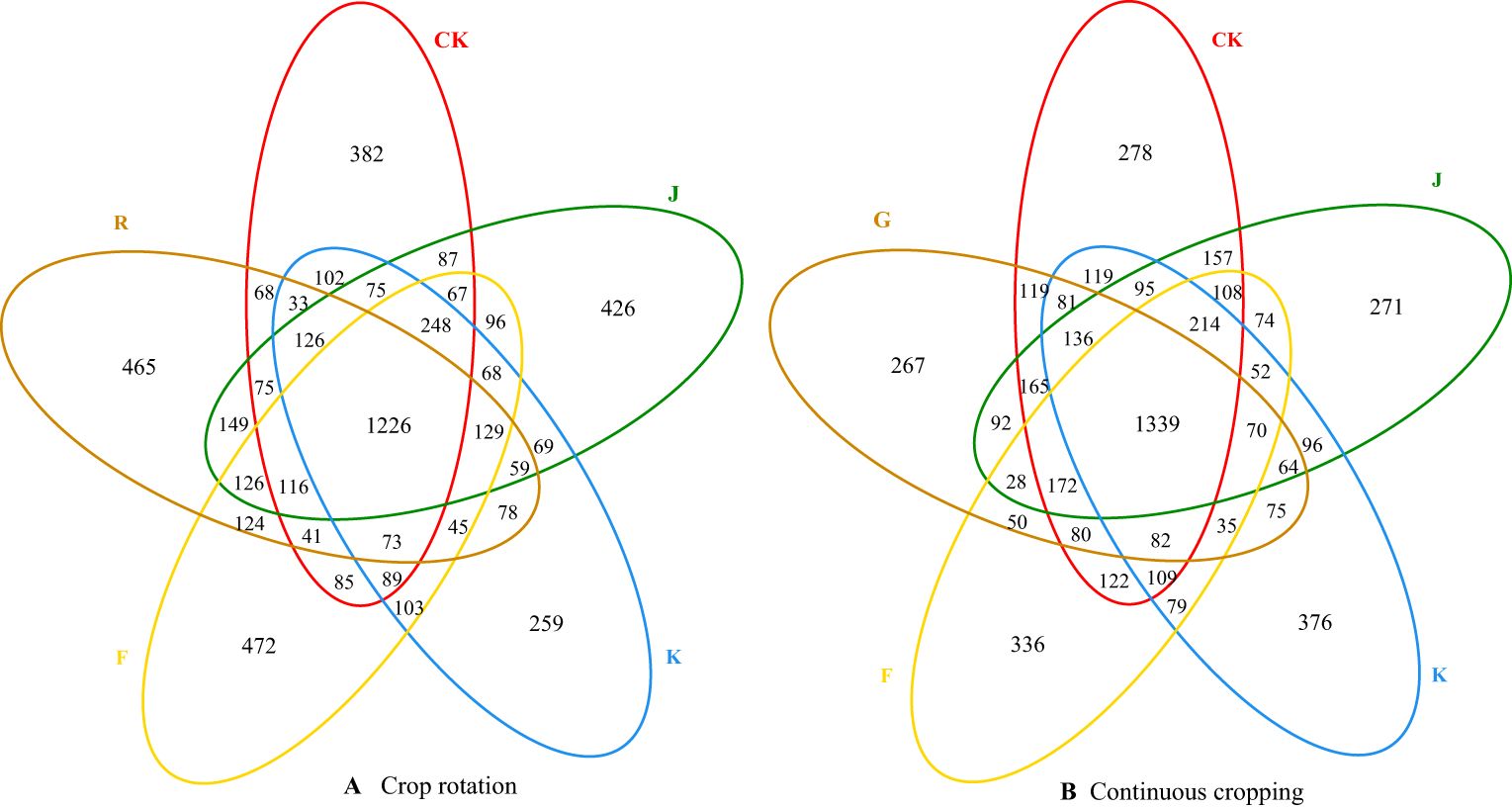
Figure 1. Venn diagram of common and unique OTU numbers of soil bacteria in rotating (A) and continuous cropping (B) G. uralensis with different treatments.
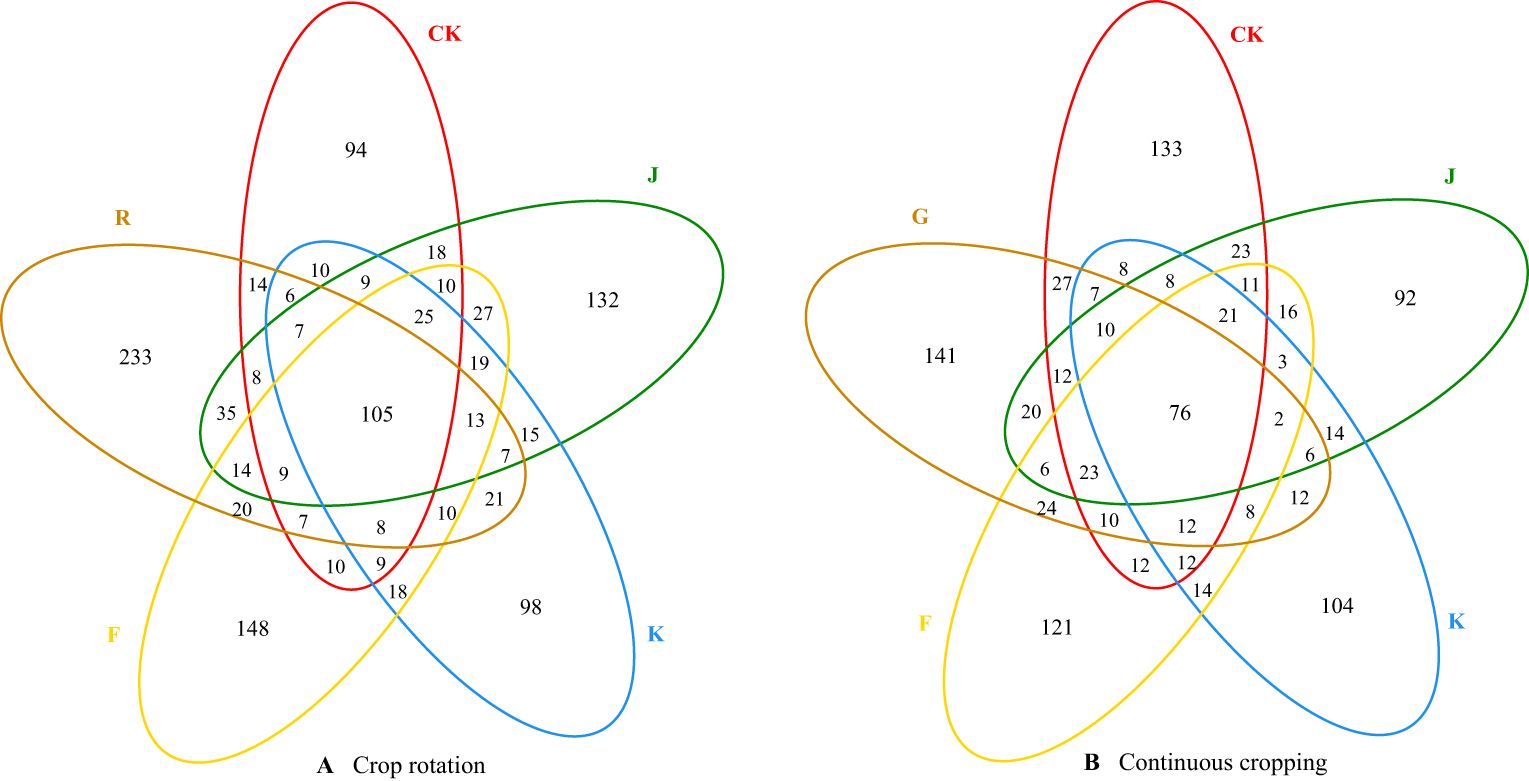
Figure 2. Venn diagram of common and unique OTU numbers of soil fungi in rotating (A) and continuous cropping (B) G. uralensis with different treatments.
The number of common fungal OTU between soil sample before planting G. uralensis in rotating test site (R), CK, J, K and F treatments was 105, and the number of unique fungal OTU was 233, 169, 132, 98 and 148, respectively. The number of common fungal OTU between soil sample before planting G. uralensis in continuous cropping test site (G), CK, J, K and F treatments was 76, and the number of unique fungal OTU was 141, 133, 92, 104 and 121, respectively. Compared with CK, J, K and F treatments significantly reduced the number of unique OTU of rotating and continuous cropping G. uralensis soil fungi (Figure 2).
3.2 Effect of microbial fertilizers on soil bacterial and fungal richness and diversity in G. uralensis
Based on the above OTU numbers, the bacterial and fungal diversity index (Shannon index) and the richness index (Chao1 index) were calculated, and the results are shown in Table 5. For rotating G. uralensis, K and F treatment significantly increased the soil bacterial Chao1 index by 6.75% and 11.69% compared with CK. J treatment significantly increased the soil bacterial Shannon index by 3.19% compared with CK, while K and F treatment had no significant effect on its bacterial Shannon index. For continuous cropping G. uralensis, the microbial fertilizers application did not significantly increase the soil bacterial Chao1 index, but it had a significant promotion effect on the bacterial Shannon index. Among them, J, K and F treatments significantly increased their bacterial Shannon index by 10.31%, 7.22%, and 12.03% compared with CK, respectively.
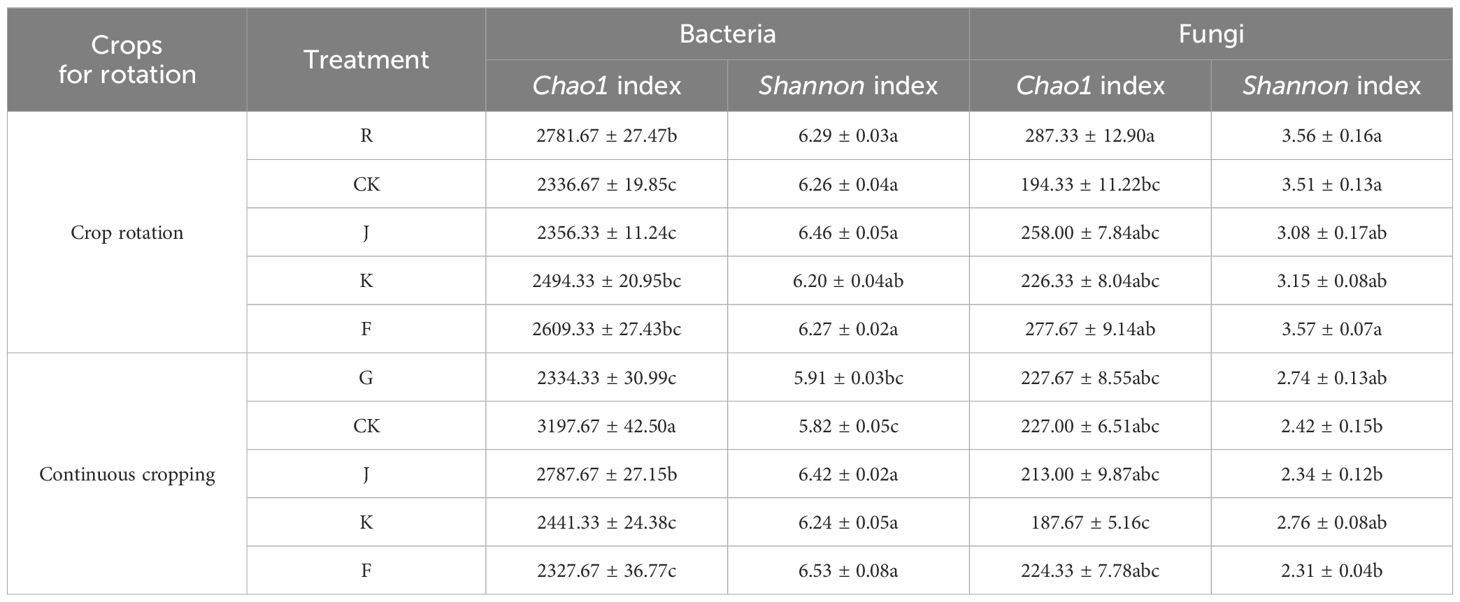
Table 5. Richness and diversity index of soil bacterial and fungal in rotating and continuous cropping G. uralensis under different treatments.
Fertilization can significantly reduce the soil fungal richness and diversity in rotating and continuous cropping G. uralensis. J, K and F treatment significantly reduced the rotating G. uralensis soil fungal Chao1 index by 11.24%, 26.99%, and 3.61% compared with R treatment, respectively. The J and K treatment significantly reduced the Shannon index of rotating G. uralensis soil fungi by 15.58% and 13.02% compared with R treatment, and 13.96% and 11.43% were significantly lower than the CK treatment. The J and K treatments significantly reduced the Chao1 index of continuous cropping G. uralensis soil fungi by 6.57% and 21.39% compared with CK, respectively. The J and F treatments significantly reduced the Shannon index of continuous cropping G. uralensis soil fungi by 3.42% and 4.76% compared with CK, respectively (Table 5).
3.3 Effect of microbial fertilizers on bacterial and fungal community composition in G. uralensis soil
3.3.1 Soil bacterial community composition and their relative abundance changes
Combining Figures 3, 4 shows, rotating G. uralensis soil bacterial communities in 17 phyla. There were 6 classes of dominant bacterial communities with clear classification and relative abundance greater than 5.00%, namely Proteobacteria, Actinobacteria, Gemmatimonadota, Acidobacteriota, Chloroflexi, and Bacteroidota (Figure 3A). In continuous cropping G. uralensis, soil bacterial community in 14 phyla. There are 7 classes of dominant bacterial communities with clear classification and relative abundance greater than 5.00%, namely Actinobacteria, Proteobacteria, Chloroflexi, Bacteroidota, Gemmatimonadota, Firmicutes, and Acidobacteriota. In rotating and continuous cropping of G. uralensis, the relative abundance of dominant bacteria in soil Actinobacteria and Proteobacteria were significantly higher than those of other bacterial communities (Figure 3B).
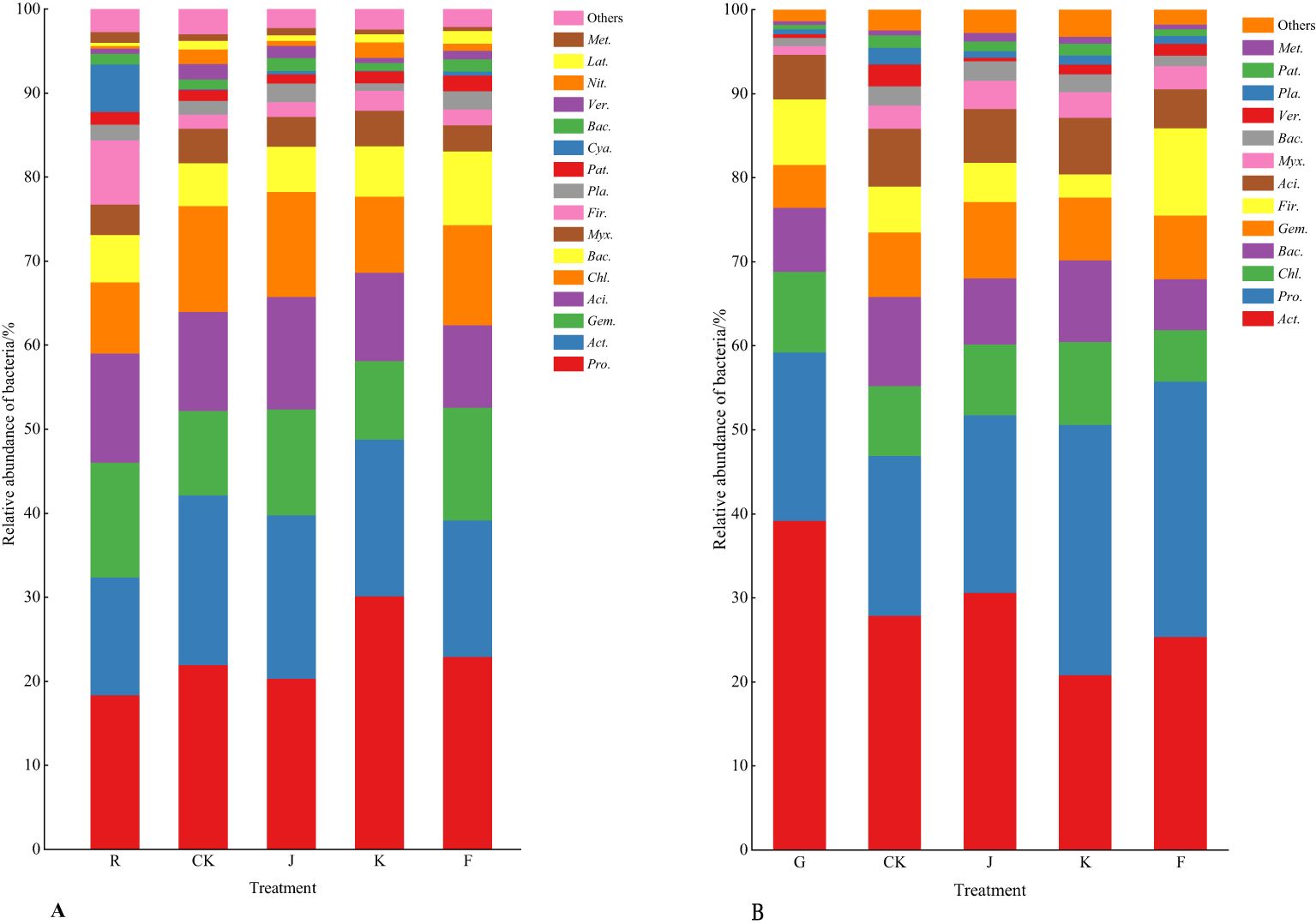
Figure 3. Composition of soil bacterial community in rotating (A) and continuous cropping (B) G. uralensis between different treatments (phylum level) Figure A: Pro., Proteobacteria; Act., Actinobacteria; Gem., Gemmatimonadota; Aci., Acidobacteriota; Chl., Chloroflexi; Bac., Bacteroidota; Myx., Myxococcota; Fir., Firmicutes; Pla., Planctomycetota; Pat., Patescibacteria; Cya., Cyanobacteria; Ver., Verrucomicrobiota; Nit., Nitrospirota; Lat., Latescibacterota. Others, Bacterial unclassified. Figure B: Act., Actinobacteria; Pro., Proteobacteria; Chl., Chloroflexi; Bac., Bacteroidota; Gem., Gemmatimonadota; Fir., Firmicutes; Aci., Acidobacteriota; Myx., Myxococcota; Ver., Verrucomicrobiota; Pla., Planctomycetota; Pat., Patescibacteria. Others, Bacterial unclassified. The same as following.
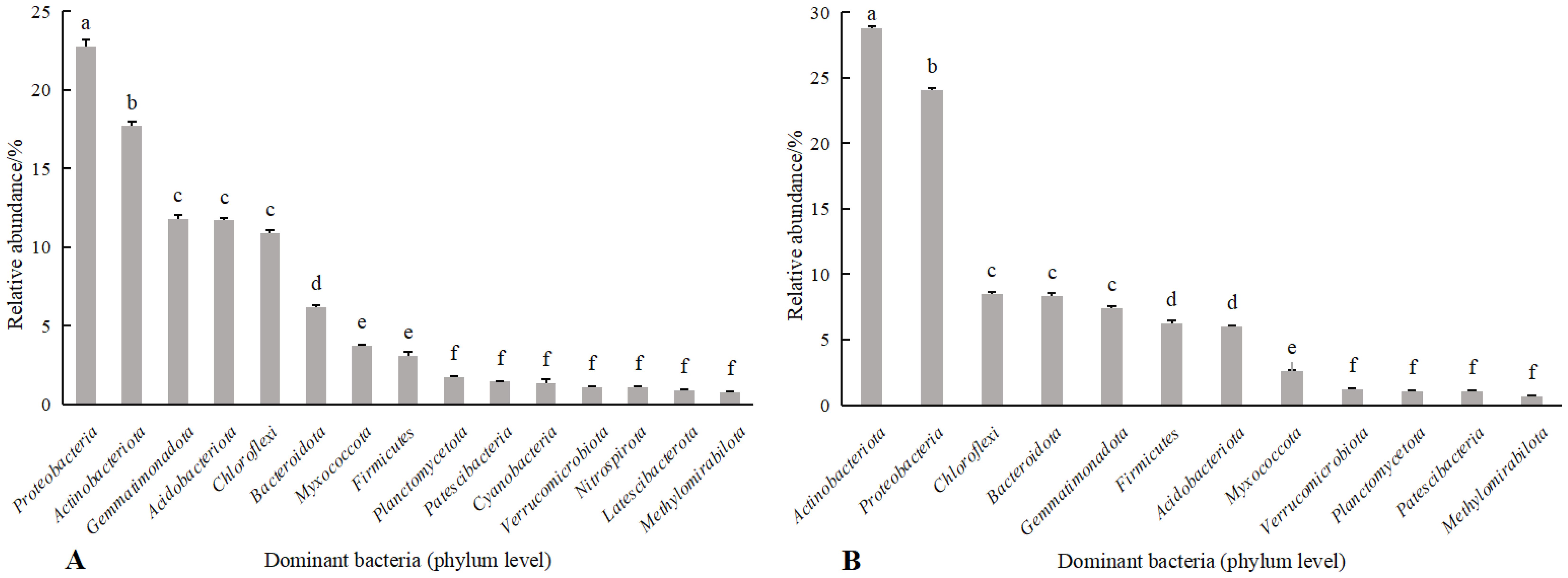
Figure 4. Relative abundance of soil bacterial communities (phylum level) in rotating (A) and continuous cropping (B) of G. uralensis Different lowercase letters indicate significant differences between different dominant bacterial communities (P<0.05). The same as following.
All treatments were clustered to analyze the similarities and differences in bacterial community composition between the different treatments, and the results are shown in Figure 5. The fertilization treatments of rotating G. uralensis RCK, RJ, RF and RK treatments were clustered into one class and R treatment was arranged separately. CJ, CK and CCK treatments were treated with continuous cropping G. uralensis, and the G treatment was arranged separately. It indicated that the bacterial community composition and its abundance of the soil before G. uralensis cultivation were significantly different from that after fertilization. In other words, both conventional fertilization and microbial fertilizers could change the composition and abundance of soil bacterial communities in rotating and continuous cropping G. uralensis.
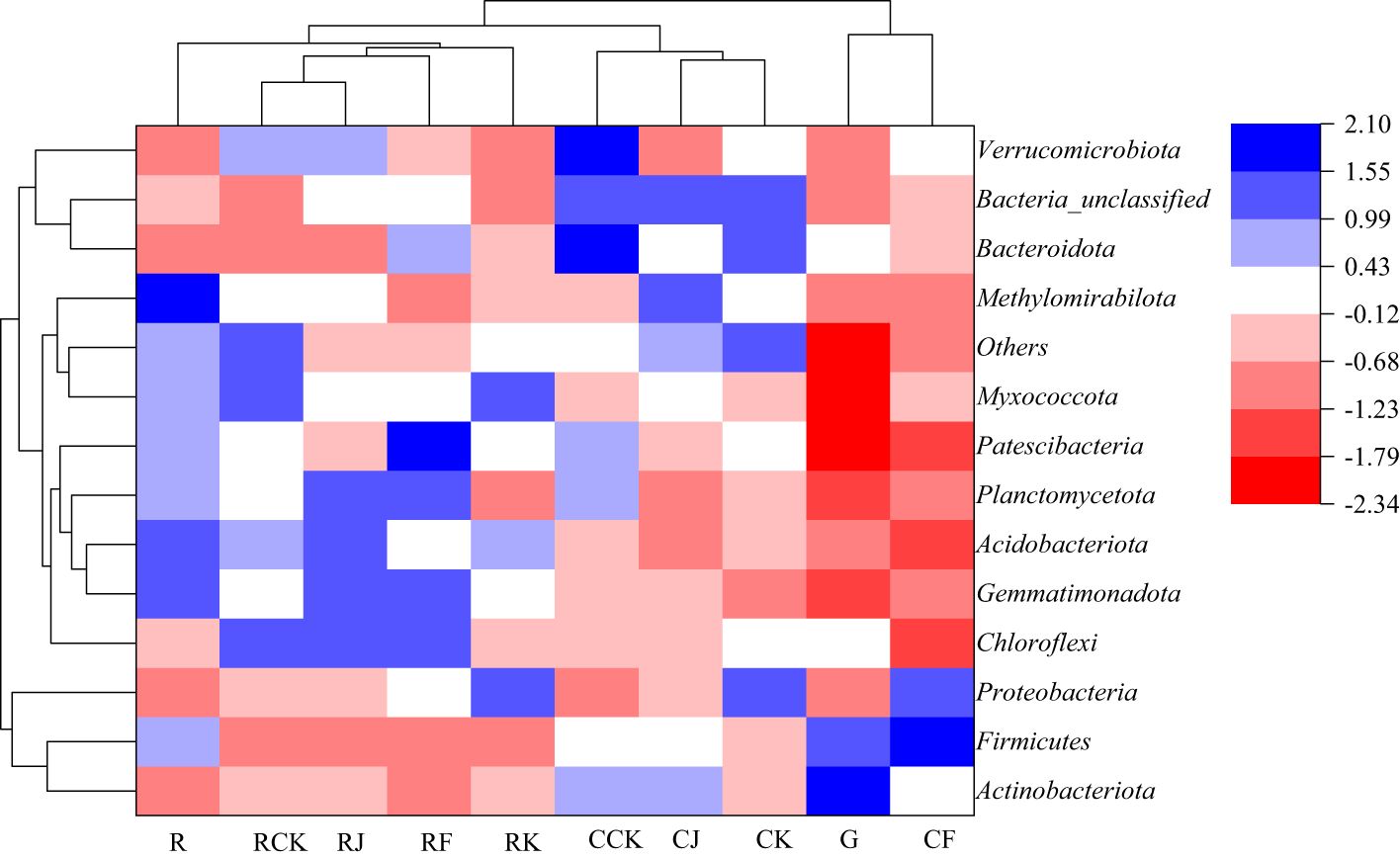
Figure 5. Clustering analysis of soil bacterial communities under different treatments (phylum level) R, Soil sample before planting G. uralensis in rotating cropping. RCK, Conventional fertilization control in rotating G. uralensis. RJ, Bacillus amylolytica in rotating G. uralensis. RK, Bacillus subtilis in rotating G. uralensis. RF, Complex microbial agent in rotating G. uralensis; G, Soil sample before planting G. uralensis in continuous cropping. CCK, Conventional fertilization control in continuous cropping G. uralensis. CJ, Bacillus amylolyticus in continuous cropping G. uralensis. CK, Bacillus subtilis in continuous cropping G. uralensis. CF, Complex microbial agent in continuous cropping G. uralensis. The same as following.
The effects of different fertilization treatments on the relative abundance of major dominant bacteria in rotating and continuous cropping G. uralensis soils are shown in Table 6. Compared to the relative abundance of soil dominant bacteria before planting G. uralensis in rotation (R) and continuous cropping (G), the relative abundance of Pro., Act., and Chl. in rotating G. uralensis were significantly increased by 2.21~11.97, 2.03~6.33, and 0.97~5.30 percentage points under different fertilization treatments, and the relative abundance of Pro., Gem., and Aci. in continuous cropping G. uralensis increased significantly by 1.51~10.72,1.94~4.05 and 1.16~2.84 percentage points. Compared to the CK treatment with rotating G. uralensis, the J treatment significantly increased the relative abundance of Gem. and Aci. dominant bacteria by 3.22 and 2.88 percentage points, respectively. The K treatment significantly increased the relative abundance of Pro. dominant bacteria by 8.09 percentage points, and the F treatment significantly increased the relative abundance of Gem. and Bac. dominant bacteria by 3.11 and 3.85 percentage points, respectively. Compared to the CK treatment with continuous cropping G. uralensis, the J treatment significantly increased the relative abundance of Pro. and Act. dominant bacteria by 2.33 and 2.97 percentage points, respectively. The K treatment significantly increased the relative abundance of Pro. and Chl. dominant bacteria by 10.91 and 2.30 percentage points, respectively, and the F treatment significantly increased the relative abundance of Pro. dominant bacteria in soil by 11.54 percentage points.
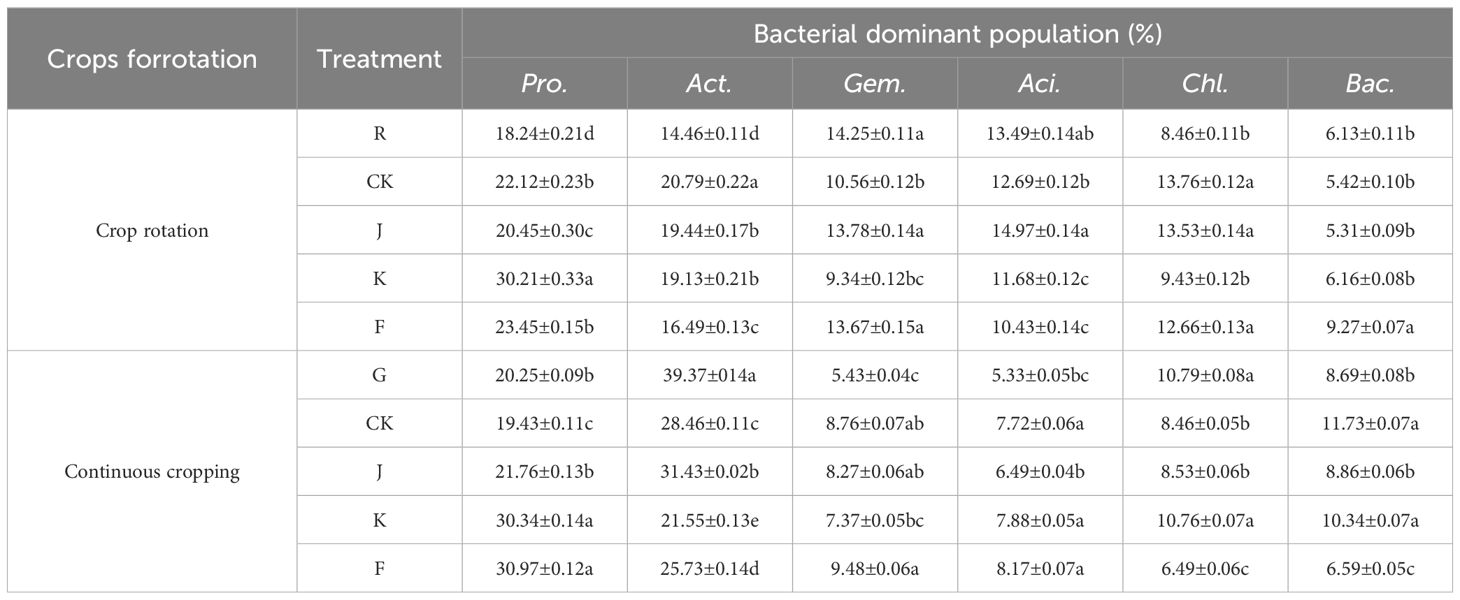
Table 6. Effects of treatments on the relative abundance of soil dominant bacterial communities in rotating and continuous cropping G. uralensis.
3.3.2 Soil fungal community composition and their relative abundance changes
According to Figures 6, 7, the soil dominant fungal communities of rotating G. uralensis were Ascomycota, Basidiomycota, Zygomycota, and Glomeromycota, with relative abundance of 74.74%, 12.74%, 4.58%, and 0.32%. The dominant fungal community of continuous cropping G. uralensis was Ascomycota, Basidiomycota and Zygomycota, and the relative abundance was 70.12%, 25.85%, and 1.81% respectively. The relative abundance of soil Basidiomycota in continuous cropping G. uralensis was 13.11 percentage points higher than rotating G. uralensis.
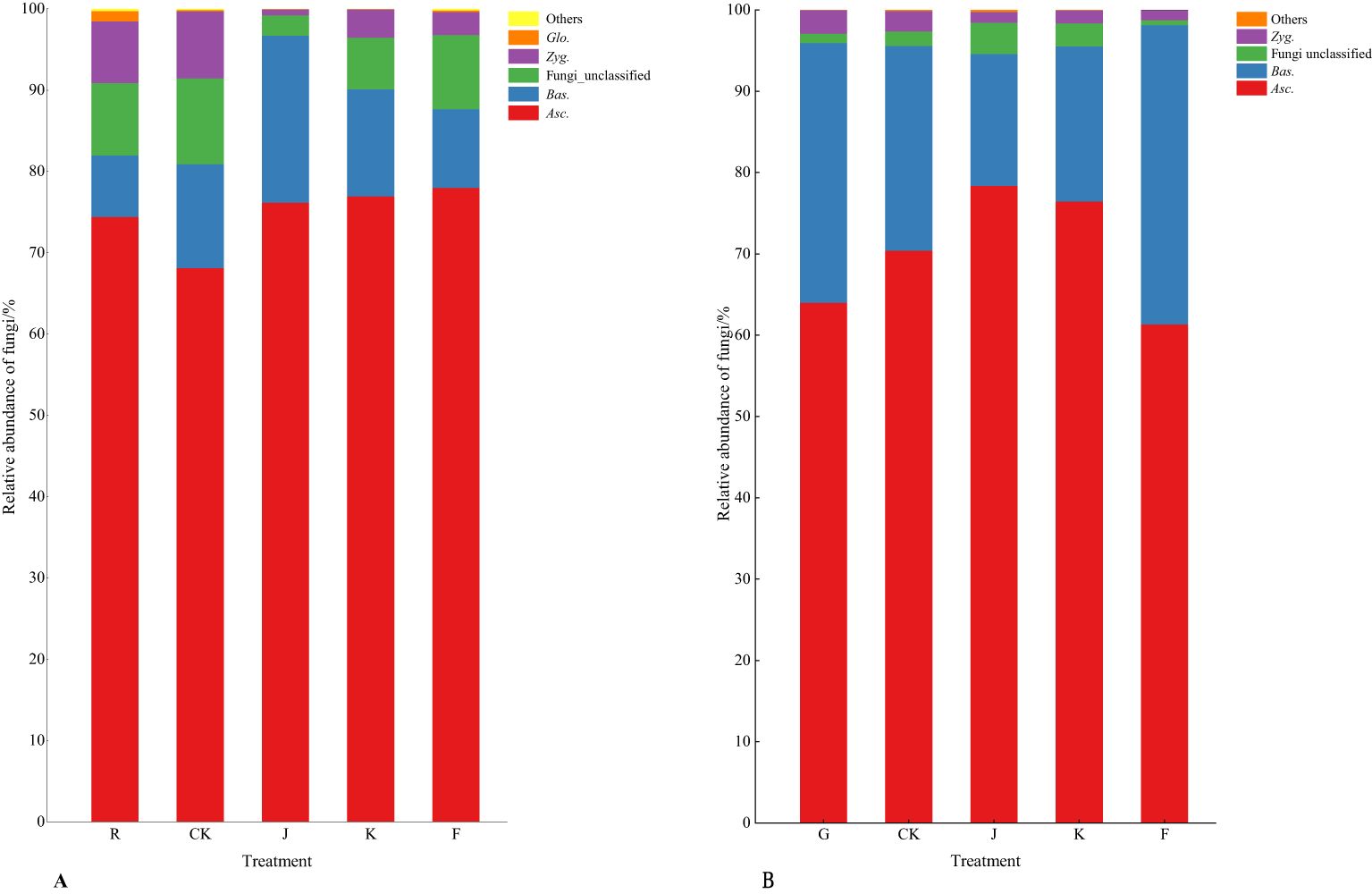
Figure 6. Composition of soil fungal community in rotating (A) and continuous cropping G. uralensis (B) between different treatments (phylum level) Figure A: Asc., Ascomycota; Bas., Basidiomycota; Zyg., Zygomycota; Glo., Glomeromycota. Others, Bacterial unclassified. Figure B: Asc., Ascomycota; Bas., Basidiomycota; Zyg., Zygomycota. Others, Bacterial unclassified.
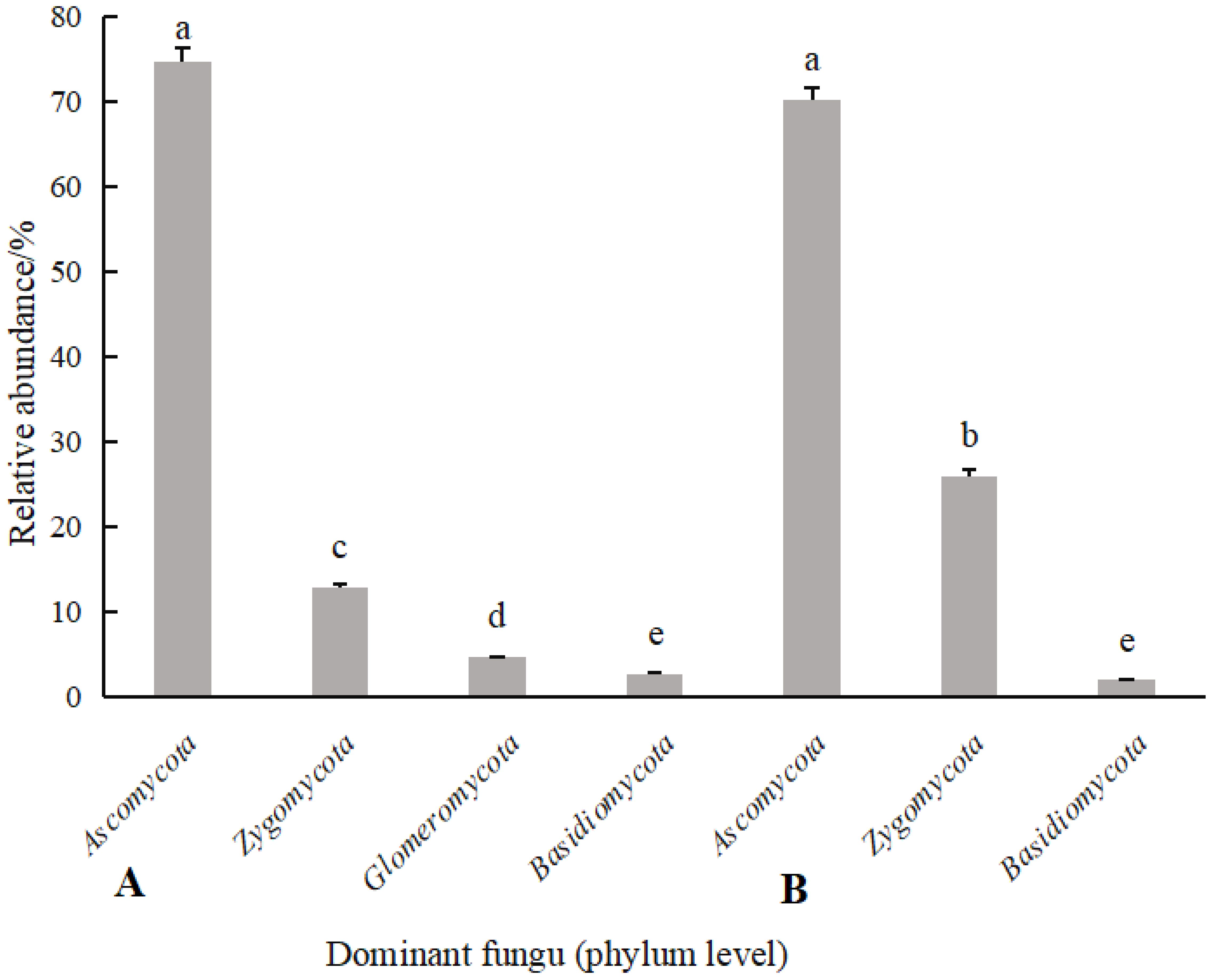
Figure 7. Relative abundance of soil fungal communities in rotating (A) and continuous cropping (B) of G. uralensis (phylum level).
The results of the similarity and differential clustering analysis of soil fungal community composition across all treatments are shown in Figure 8. Under different fertilization treatments, the relative abundance of Ascomycota and Zygomycota of rotating and continuous cropping G. uralensis was similar in each other, while Basidiomycota was quite different from the above two fungi, such as Ascomycota and Zygomycota. In addition, RJ, RK, and RF treatments clustered into a large class, R and RCK treatment alone clustered into one class. This indicated that the composition and abundance of soil fungal community of rotating G. uralensis were significantly different from that of conventional fertilization compared with microbial fertilizers application. The G treatment with other treatments separately into a class, such as CJ, CK, and CCK treatments, indicated that both conventional fertilization and microbial fertilizers could significantly affect the fungal community composition and abundance of continuous cropping G. uralensis. The RJ and RK treatments cluster into a class, simultaneously, the CK and CJ treatments also cluster into a class, suggested some similarity in the fungal community composition and abundance of rotating and continuous cropping G. uralensis soil treated by Bacillus amyloliquefaciens and Bacillus subtilis.
Compared to the relative abundance of soil dominant fungi before planting G. uralensis in rotation (R) and continuous cropping (G), microbial fertilizers were not significant in reducing the relative abundance of soil dominant fungal communities in rotating G. uralensis. However, microbial fertilizers could significantly reduce the relative abundance of the dominant fungal communities Asc., Bas. and Zyg. of continuous cropping G. uralensis (Table 7). For the soil dominant fungi of rotating G. uralensis, compared with CK treatment, the F treatment significantly reduced the relative abundance of soil Bas. and Zyg. by 3.00 and 4.35 percentage points, while J and K treatment did not significantly reduce the effect. For soil dominant fungi of continuous cropping G. uralensis, compared with CK treatment, F treatment significantly reduced the relative abundance of Asc. by 8.93 percentage points, and the J and K treatment significantly reduced the relative abundance of Bas. by 9.04 and 5.68 percentage points, respectively. The J, K and F treatments significantly reduced the relative abundance of soil Zyg. by 2.24, 0.98 and 1.57 percentage points compared with CK treatment, respectively (Table 7).
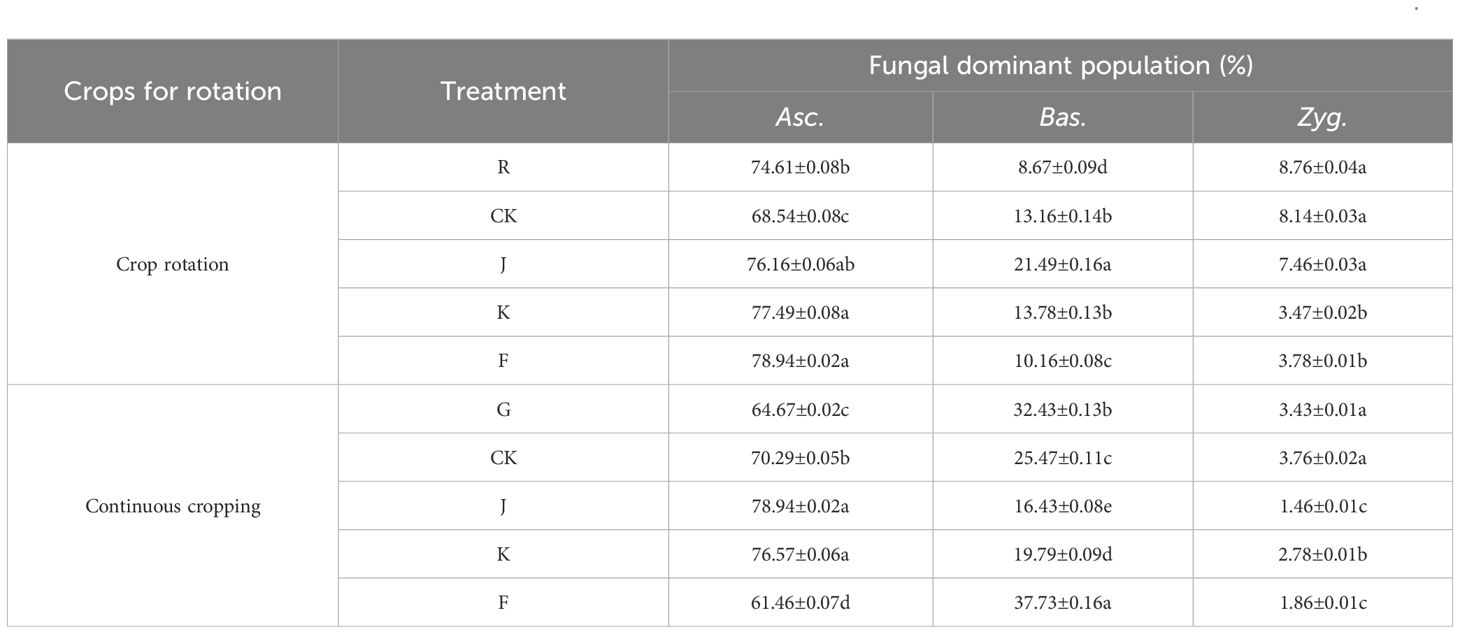
Table 7. Effects of treatments on the relative abundance of dominant fungal communities in soil of rotating and continuous cropping G. uralensis.
In conclusion, under the effects of microbial fertilizers, the composition of dominant bacterial and fungal communities in rotating and continuous cropping G. uralensis was basically the same, but the relative abundance of the dominant microflora varied significantly. Different microbial fertilizers could significantly increase the relative abundance of dominant bacterial communities of soil Proteobacteria, Actinobacteria and Chloroflexi in rotating and continuous cropping G. uralensis. In particular, microbial fertilizers could significantly reduce the relative abundance of dominant fungi in Ascomycota, Basidiomycota and Zygomycota of continuous cropping G. uralensis. Among them, the effect of the complex microbial agent was the most significant.
3.4 Effect of microbial fertilizers on soil bacterial and fungal community structure in G. uralensis
3.4.1 Analysis of the differences in soil bacterial community structure
Further performed PCoA (Principal Coordinates Analysis) for all soil samples, thus reflected the differences in the distribution of soil bacterial and fungal community structure between the different treatments, and tested by permutational multivariate analysis of variance whether the difference in soil bacterial and fungal community structure was significant under different fertilization treatments. The closer the distance between the samples, the higher the structural similarity of species composition. Figure 9A shows that the interpretation rate of PC1 was 36.35% and PC2 was 15.08%, and the permutational multivariate analysis R2 = 0.649, P = 0.001, indicated that the structure of soil bacterial communities in rotating and continuous cropping G. uralensis were significantly different under different fertilization treatments. For the rotating of G. uralensis, the close distance between CK, K, and J treatments indicated that the structure of soil bacterial community composition was similar between conventional fertilization treatments and Bacillus amyloliquefaciens and Bacillus subtilis. The F treatment is distant from J and K treatments indicated a significant difference in the spatial structure distribution of soil bacterial community composition between the F treatment and the J and K treatments. Among them, the bacterial community composition of complex microbial agent treatment was abundant and diverse in spatial distribution compared with other treatments.
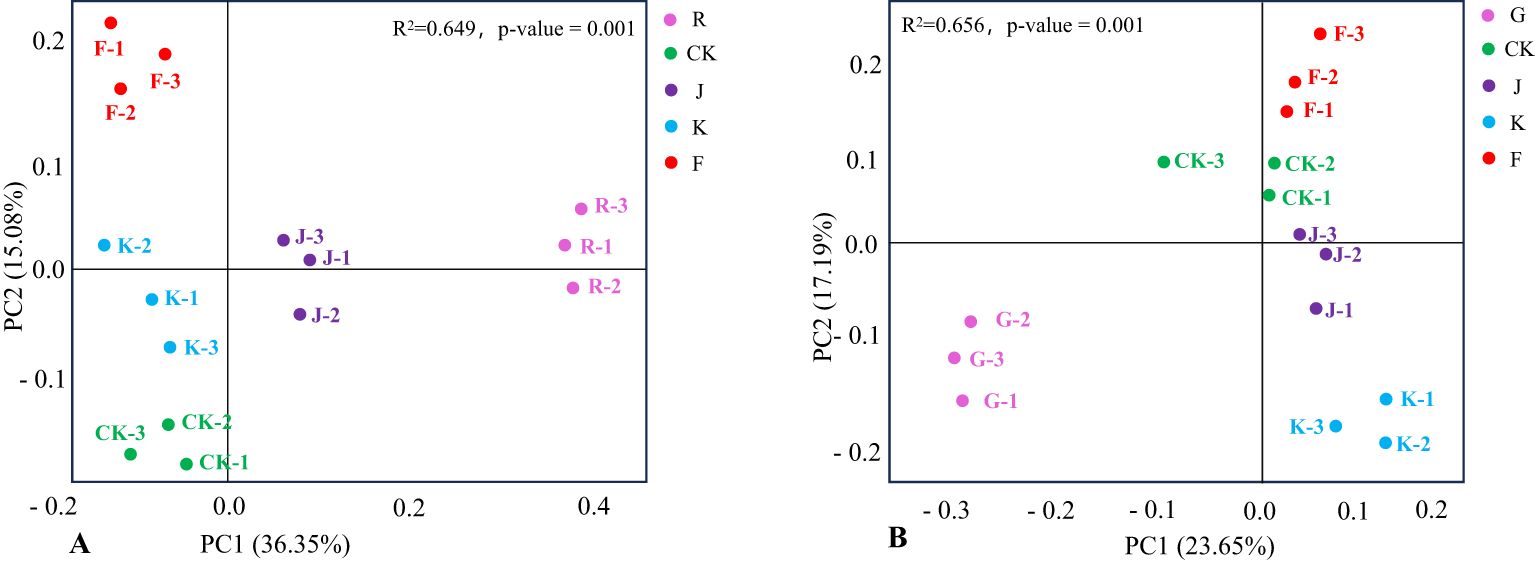
Figure 9. PCoA of soil bacterial communities in rotating (A) and continuous cropping (B) G. uralensis under different treatments.
Under different treatments of continuous cropping G. uralensis, the G treatment is distant from CK, J, F and K treatments, and the CK, J, and F treatments were located closer to each other. It showed that both conventional fertilization and microbial fertilizers coould significantly affect the bacterial community composition structure of continuous cropping G. uralensis soil. In this, the structure of soil bacterial community composition of Bacillus amyloliquefaciens and complex microbial agent treatment was basically similar to that of conventional fertilization treatment. Furthermore, the K treatment is distant from J and F treatment, suggested that the composition of soil bacterial community under the treatment of Bacillus subtilis was significantly different from that under the treatment of Bacillus amyloliquefaciens and complex microbial agent (Figure 9B).
3.4.2 Analysis of the differences in soil fungal community structure
The R and G treatments of rotating and continuous cropping G. uralensis were far from other fertilization treatments, indicated that fertilization significantly affected the fungal community structure in rotating (R2 = 0.631, P = 0.001) and continuous cropping (R2 = 0.692, P = 0.001) G. uralensis. Moreover, the distance between K and F treatment of rotating and continuous cropping G. uralensis was close, and the distance between K and J treatment was relatively distant. It suggested that the soil fungal community structure of Bacillus subtilis treatment and complex microbial agent treatment in rotating and continuous cropping G. uralensis were similar, but there are differences between them and the treatment of Bacillus amyloliquefaciens (Figure 10).
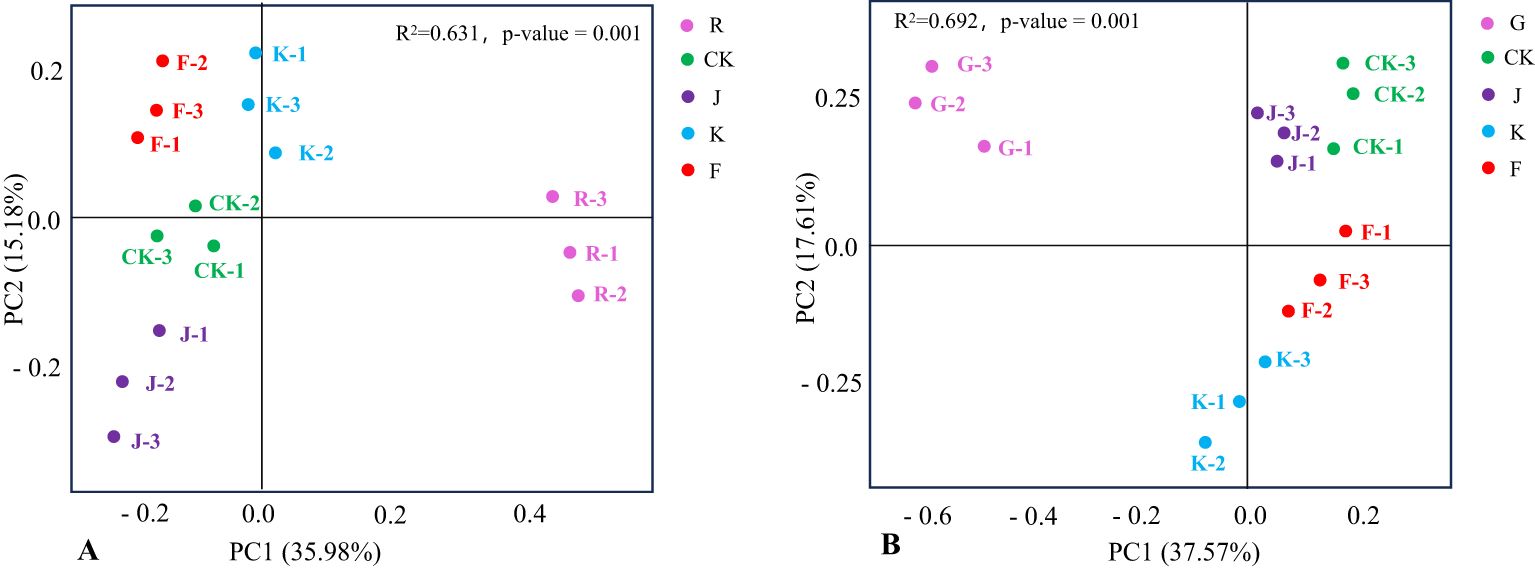
Figure 10. PCoA of soil fungal communities in rotating (A) and continuous cropping (B) G. uralensis under different treatments.
3.5 Effect of microbial fertilizers on soil microbial KEGG metabolic pathways in G. uralensis
Changes in soil bacterial and fungal richness, diversity, community composition, and structural distribution can cause changes in soil microbial metabolic functions. Therefore, in this study, the sequence of bacterial community genes in rotating and continuous cropping G. uralensis were further aligned with KEGG (Kyoto Encyclopedia of Genes and Genomes) data, and later, functional annotation was performed. The results are shown in Figure 11. Under the action of different microbial fertilizers, the main functions of rotating and continuous cropping G. uralensis soil bacterial functional genes in the primary metabolic pathway of the KEGG database included metabolism, genetic information processing and eavironmental information processing. Among them, the metabolism accounted for the highest proportion, followed by genetic information processing, this showed that the function of soil microorganisms in rotating and continuous cropping G. uralensis is mainly by metabolism. In the secondary metabolic pathway of the KEGG database, the main functions of rotating and continuous cropping G. uralensis soil microorganisms are carbohydrate metabolism, amino acid metabolism and energy metabolism. There was no significant difference in the proportion of main functions of soil microorganisms between rotating and continuous cropping G. uralensis. Suggested that under the action of the microbial fertilizers, the change of continuous cropping and rotating system had little effect on the main functions of G. uralensis soil microorganisms (Figure 11).
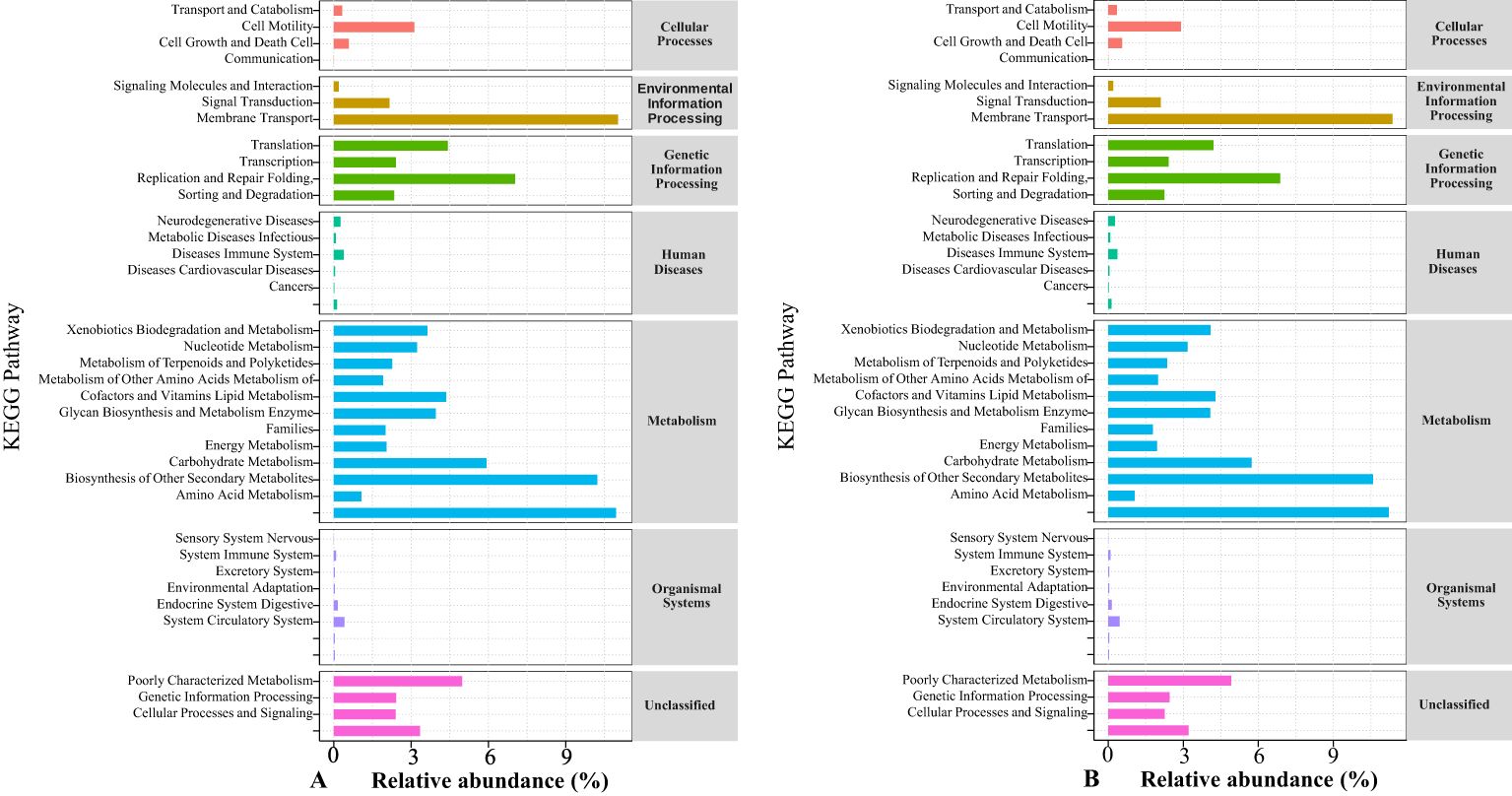
Figure 11. Annotated pathway statistical map of soil bacterial community gene sequences of rotating (A) and continuous cropping (B) G. uralensis in KEGG database.
4 Discussion
In this study, under different fertilization treatments, the species and quantity of soil bacterial and fungal communities in rotating G. uralensis were higher than those in continuous cropping G. uralensis (Table 5). This result was analyzed according to the research results of “the soil microbial richness and diversity of rotaing Zea mays L. were significantly higher than that of continuous stubble (Ma et al., 2024; Gillespie et al., 2021)”. On the one hand, because microbial fertilizers could significantly enrich the species and number of microorganisms in the G. uralensis surface soil (Wen et al., 2024). On the other hand, the root system of rotating G. uralensis is deeper than that of continuous cropping G. uralensis, and the deep G. uralensis root system is more conducive to activating the microbial life activities in deep soil. This “top-down” mechanism of action, the common activation of soil microbial life activities, so that the rotating G. uralensis soil microbial species and quantity increase faster (Anton et al., 2023; Giuseppe et al., 2021; Roohi et al., 2020).
The results of this study showed that Bacillus amylodiolitica, Bacillus subtilis and complex microbial agent significantly increased the soil bacterial diversity of continuous cropping G. uralensis by 7.22% to 12.03%, and also significantly reduced the soil fungal richness and diversity of continuous cropping G. uralensis by 4.76% to 20.96%. However, the effect of microbial fertilizers on soil bacteria and fungi of rotating G. uralensis was not particularly significant, compared with the effect of microbial fertilizers on continuous cropping G. uralensis (Tables 5–7). Analysis of its reasons, may be that the original microorganisms in the soil of rotating G. uralensis had many kinds, high abundance and strong adaptability, preferentially occupied the nutrient or reproductive sites, and inhibited the growth and reproduction of functional microorganisms in the microbial fertilizers (Lijing et al., 2024; Rose et al., 2024). For the soil of continuous cropping G. uralensis, the species and number of original microorganisms are small and the ability to compete for breeding sites is weak, while the functional microorganisms in microbial fertilizers are abundant and have strong enrichment ability, which can preferentially propagat in the soil of continuous cropping G. uralensis (He et al., 2024; Li et al., 2024). In addition, after the application of microbial fertilizers for continuous cropping G. uralensis, the soil nutrient content increases, and the soil environment is more conducive to the growth of beneficial microflora in microbial fertilizers (Holatko et al., 2024). Therefore, in the results of this experiment, the effect of microbial fertilizers affected the soil microbial community structure of continuous cropping G. uralensis was significantly stronger than that of rotating G. uralensis. In addition to that, the microorganisms in microbial fertilizers grow and play its role, itself requires a certain adaptation time, and the overall regulation of soil microbes, it also takes a long time (Han et al., 2024; Xin et al., 2023). This may also be the main reason for the small effect of microbial fertilizers on the soil microbes of rotating G. uralensis in a short time.
In this reserach, the effect of the complex microbial agent on the species and quantity of G. uralensis soil was most remarkable, which increased the relative abundance of Gemmatimonadota and Proteobacteria of rotating and continuous cropping G. uralensis soil by 0.03 and 0.11 percentage points, respectively, and significantly reduced the relative abundance of dominant fungi in Basidiomycota and Zygomycota was 0.09 and 0.02 percentage points, respectively (Tables 6, 7). This result was consistent with the results of the study that “the compound bacterial fertilizer had the best effect on regulating the soil microflora structure of Lilium brownii var. and Pseudostellaria heterophylla in continuous cultivation (Yan et al., 2022; Lin et al., 2012)”. The main reason is that the complex microbial agent itself contains a complete variety of functional microorganisms with a stable structure, and the synergistic ability of different strains is stronger than that of single strain type microbial fertilizers (Vitorino et al., 2024; Lixinyue et al., 2024).
Basidiomycota is a rotten soil fungal pathogen, which occupies a relatively high proportion in soil fungi, and it is easy to enrich and develop into dominant fungal communities with the increase of plant continuous cropping years (Manici et al., 2020; Traxler et al., 2023). Consequently, leading to the aggravation of soil “fungalization” and the increased incidence of plant root rot (Sang et al., 2024). In the present study, the relative abundance of Basidiomycota in continuous cropping G. uralensis soil was significantly higher by 0.12 percentage points than that of rotating G. uralensis (Figures 4, 7), which is consistent with the above phenomenon.
Soil microorganisms decompose and transform soil nutrients through multiple metabolic pathways (Liu et al., 2024; Lihualiang et al., 2024). Metabolism, the main pathway by which Astragalus memeranaceus soil microorganisms exert their effects. Moreover, this metabolic pathway accounted for more than 50.09% of the different fertilization treatments of crop rotation and continuous cropping of Astragalus memeranaceus (Qin et al., 2024). The results of this study also indicated that the microbial activity of rotating and continuous cropping G. uralensis is dominated by metabolism under the action of different microbial fertilizers (Figure 11). Uniformly indicated that the metabolic capacity of soil microorganisms is enhanced, which is conducive to improving the decomposition efficiency of soil organic matter and the absorption efficiency of soil nutrients by plants, and ensuring the supply of essential nutrients for plant growth.
5 Conclusion
Continuous cropping would lead to the decrease of the richness and diversity of G. uralensis soil microorganisms, and the serious “fungalization” of soil. After the application of microbial fertilizers such as Bacillus amyloliquefaciens, Bacillus subtilis, and complex microbial agent, the richness and diversity of continuous cropping G. uralensis soil bacteria were significantly increased (by 7.22% to 12.03%), and the fungal richness and diversity were significantly decreased (by 3.42% to 4.76%), and the soil microbial community structure was optimized. These microbial fertilizers were better in optimizing soil microbial community structure of continuous cropping G. uralensis than in rotating G. uralensis. This provided theoretical support for the efficient fertilization of G. uralensis cultivation in the desert and semi-desert areas of western China, in particular, the complex microbial agent was very promising in G. uralensis cultivation. However, there are still some shortcomings in this study: “linking soil microorganisms, soil physical and chemical properties and G. uralensis yield and quality, and analyzing their interrelationship and mechanism of action”. In future studies, should combine equation models, metabolomics and transcriptomics, focusing on the mechanism of action between soil microbiotic environment and G. uralensis yield and quality, and explore the influence of different strains in microbial fertilizers on the yield and quality of G. uralensis.
Data availability statement
The original contributions presented in the study are publicly available. This data can be found here: https:/www.ncbi.nlm.nih.gov/bioproject/PRJNA1200788.
Author contributions
XW: Conceptualization, Data curation, Formal analysis, Investigation, Methodology, Software, Supervision, Writing – original draft, Writing – review & editing. DQ: Funding acquisition, Project administration, Resources, Supervision, Validation, Visualization, Writing – original draft, Writing – review & editing. KJ: Conceptualization, Project administration, Software, Supervision, Validation, Visualization, Writing – review & editing. GG: Conceptualization, Data curation, Investigation, Methodology, Software, Writing – original draft. FB: Conceptualization, Funding acquisition, Project administration, Software, Supervision, Writing – review & editing.
Funding
The author(s) declare that financial support was received for the research, authorship, and/or publication of this article. The author(s) declare financial support was received for the research, authorship, and/or publication of this article. This research was funded by the National Natural Science Foundation of China, grant number 31960395.
Conflict of interest
Author FB was employed by the company China Chinese Medicine Co., LTD, Beijing, China
The remaining authors declare that the research was conducted in the absence of any commercial or financial relationships that could be constructed as a potential conflict of interest.
Publisher’s note
All claims expressed in this article are solely those of the authors and do not necessarily represent those of their affiliated organizations, or those of the publisher, the editors and the reviewers. Any product that may be evaluated in this article, or claim that may be made by its manufacturer, is not guaranteed or endorsed by the publisher.
References
Anton, G., Živa, P., Klemen, E., Rok, M., Marjetka, S. (2023). Combined effects of long-term tillage and fertilization regimes on soil organic carbon, microbial biomass, and abundance of the total microbial communities and N-functional guilds. Appl. Soil Ecol. 188, 0929–09333. doi: 10.1016/J.APSOIL.2023.104876
Bao, Y. M., Ma, B., Neil, B., Mclaughlin, N. B., Niu, Y., Wang, D. Q., et al. (2024). The impact of salinization on soil bacterial diversity, yield and quality of. Glycyrrhiza uralensis Fisch. Front. Microbiol. 15, 8462–8470. doi: 10.3389/FMICB.2024.1448301
Chaudhary, P., Singh, S., Chaudhary, A., Sharma, A., Kumar, G. (2022). Overview of biofertilizers in crop production and stress management for sustainable agriculture. Front. Plant Sci. 13, 718–722. doi: 10.3389/fpls.2022
Deepak, B., Wahid, M. A., Kumar, R. S., Narendra, T. (2016). Biofertilizers function as key player in sustainable agriculture by improving soil fertility, plant tolerance and crop productivity. Microbial Cell factories 13, 66–71. doi: 10.1186/1475-2859-13-66
Gillespie, L. M., Hattenschwiler, S., Milcu, A., Wambsganss, J., Shihan, A., Fromin, N. (2021). Three species mixing affects soil microbial functioning indirectly via root and litter traits and soil parameters in European forests. Funct. Ecol. 35, 2197–2201. doi: 10.1111/1365-2435.13877
Giuseppe, B., Armando, V. L., Gaetano, A., Badalucco, L., Frenda, A. S., Giambalvo, D., et al. (2021). Long-term effects of contrasting tillage systems on soil C and N pools and on main microbial groups differ by crop sequence. Soil Tillage Res. 21, 211–218. doi: 10.1016/J.STILL.2021.104995
Guimaraes, I. T., Oliveira, F., Leal, C., Souza, M. W., Alves, T. (2020). Foliar application of biofertilizer in semi-hydroponic lettuce fertigated with saline nutrient solution. Comunicata Scientiae 11, 3115–3120. doi: 10.14295/cs.v11i0.3115
Han, S., Ji, X., Huang, L., Liu, G. J., Ye, J. Y., Wang, A. Y. (2024). Effects of aftercrop tomato and maize on the soil microenvironment and microbial diversity in a long-term cotton continuous cropping field. Front. Microbiol. 15, 5249–5266. doi: 10.3389/FMICB.2024.1410219
He, Y. M., Shen, C., Zhang, H. J., Wang, Y. D. (2024). Effects of continuous cropping on the physiochemical properties, pesticide residues, and microbial community in the root zone soil of. Lycium barbarum. Environ. Sci. 45, 5578–5590. doi: 10.13227/j.hjkx.202311078
Holatko, J., Brtnicky, M., Baltazar, T., Vladimir, S., Jan, F., Antonin, K., et al. (2024). Long-term effects of wheat continuous cropping vs wheat in crop rotation on carbon content and mineralisation, aggregate stability, biological activity, and crop yield. Eur. J. Agron. 158, 127218–127224. doi: 10.1016/J.EJA.2024.127218
Ji, X., Liu, N., Huang, S., Zhang, C. (2024). A comprehensive review of Glycyrrhiza uralensis: the preparation, chemical composition, bioactivities and its applications. Am. J. Chin. Med. 52, 45–50. doi: 10.1142/S0192415X24500289
Ji, S., Liu, Z., Liu, B., Wang, Y., Wang, J. (2020). The effect of Trichoderma biofertilizer on the quality of flowering Chinese cabbage and the soil environment. Scientia Hortic. 262, 109069. doi: 10.1016/J.SCIENTA.2019
Li, C. W., Chen, G. Z., Zhang, X. X., Zou, N., Zhang, H. X., Li, H. K., et al. (2024). Soil metabolic disturbance drives replant disease (intraspecific negative plant-soil feedback): Insights from an experiment examining soil impacts up to 20 years after a ginseng crop. Geoderma 451, 117059–117065. doi: 10.1016/J.GEODERMA.2024.117059
Lijing., Lei, Y. F., Wen, Y. Y., Zhu, J. Y., Di, X. Y., Zeng, Y., et al. (2024). Short-term effects of cenchrus fungigraminus/potato or broad bean interplanting on rhizosphere soil fertility, microbial diversity, and greenhouse gas sequestration in southeast China. Microorganisms 12, 1653–1665. doi: 10.3390/MICROORGANISMS12081665
Lixinyue., Liu, Q., Gao, Y. G., Zang, P., Zheng, T. (2024). Effects of a co-bacterial agent on the growth, disease control, and quality of ginseng based on rhizosphere microbial diversity. BMC Plant Biol. 24, 677–684. doi: 10.1186/S12870-024-05347-3
Lihualiang., Yang, Y., Lei, J. X., Gou, W. K., James, M. C., Qi, P. (2024). Effects of continuous cropping of Codonopsis pilosula on rhizosphere soil microbial community structure and metabolomics. Agronomy 14, 2014–2020. doi: 10.3390/AGRONOMY14092014
Lin, M. C., Wang, H. B., Lin, H. F. (2012). Effects of Pseudostellariae heterophylla continuous cropping on rhizosphere soil microorganisms. CHN J. Ecol. 31, 106–111. doi: 10.13292/j.1000-4890.2012.0010
Liu, T., Tong, D., Chen, S., Ning, C., Zhang, X. Y., Ekaterina, F., et al. (2024). Fertilization shapes microbial life strategies, carbon and nitrogen metabolic functions in Camellia oleifera soil. J. Environ. Manage. 370, 122896–122902. doi: 10.1016/J.JENVMAN.2024.122896
Ma, J. M., Liu, D., Zhao, P. Y., Dou, M., Yang, X. H., Liu, S. L., et al. (2024). Intercropping of tobacco and maize at seedling stage promotes crop growth through manipulating rhizosphere microenvironment. Front. Plant Sci. 15. doi: 10.3389/FPLS.2024.1470229
Manici, M. L., Caputo, F., Sabata, D. D., Fornasier, F. (2020). The enzyme patterns of Ascomycota and Basidiomycota fungi reveal their different functions in soil. Appl. Soil Ecol. 2024, 196, 105323–105329. doi: 10.1016/J.APSOIL.2024.105323
National Pharmacopeia Committee (2020). Pharmacopoeia of the people’s republic of China (Part I) (Beijing: China Medical Science and Technology Press).
Plocek, G., Rueda, K. D., Simpson, C. (2024). Impacts of Bacillus amyloliquefaciens and Trichoderma spp. on Pac Choi (Brassica rapa var. chinensis) grown in different hydroponic systems. Front. Plant Sci. 15, 3289–3295. doi: 10.3389/fpls.2024.1438038
Qin, Z., Yun, W., Liang, Y., Ye, A., Xie, X. F., Zhang, M. L., et al. (2024). Impacts of continuous cropping on the rhizospheric and endospheric microbial communities and root exudates of. Astragalus mongholicus. BMC Plant Biol. 24, 331–340. doi: 10.1186/S12870-024-05024-5
Roohi, M., Arif, S. M., Yasmeen, T., Muhammad, R., Muhammad, R., Sher, M. S., et al. (2020). Effects of cropping system and fertilization regime on soil phosphorous are mediated by rhizosphere-microbial processes in a semi-arid agroecosystem. J. Environ. Manage. 271, 111033–111041. doi: 10.1016/j.jenvman.2020.111033
Rose, J. T., Parvin, S., McInnes, J., Zwieten, L., Gibson, A., Kearney, L., et al. (2024). Summer cover crop and temporary legume-cereal intercrop effects on soil microbial indicators, soil water and cash crop yields in a semi-arid environment. Field Crops Res. 312, 109384–109390. doi: 10.1016/J.FCR.2024.109384
Sang, Y., Ren, K., Chen, Y., Wang, B., Meng, Y. F., Zhou, W. B., et al. (2024). Integration of soil microbiology and metabolomics to elucidate the mechanism of the accelerated infestation of tobacco by the root-knot nematode. Front. Microbiol. 15. doi: 10.3389/FMICB.2024.1455880
Ting, T. H., Xia, N. L., Dan, L., Ji, C. G., Chen, C. X., He, C. (2023). Genotype and environment factors driven G. uralensis growth and rhizospheric soil fungal community changes. Front. Microbiol. 14, 6315–6421. doi: 10.3389/FMICB.2023.1308412
Traxler, L., Wollenberg, A., Steinhauser, G., Chyzhevskyi, I., Sergiy Dubchak, S., Großmann, S. (2023). Survival of the basidiomycete Schizophyllum commune in soil under hostile environmental conditions in the Chernobyl Exclusion Zone. J. Hazard. Mater. 54, 78–84. doi: 10.1016/j.jhazmat.2020.124002
Vela, C., Lopez, D., Tecpoyotl, Z. G., Verret, V., Gardarin, A., Makowski, D., et al. (2018). The organo-mineral fertilization on the yield of faba bean in soil and hydroponics in protected agriculture. Rev. Mexicana Cienc. Agrıcolas 9, 1603–1614. doi: 10.29312/remexca.v9i8.1717
Vieira, S., Sikorski, J., Gebala, A., Boeddinghaus, S., Marhan, S. T., McMillan, M., et al. (2020). Bacterial colonization of minerals in grassland soils is selective and highly dynamic. Environ. Microbiol. 22, 917–933. doi: 10.1111/1462-2920.14751
Vitorino, L. C., Silva, E. J., Oliveira, M. S., Silva, I. O., Bessa, L. A., Santos, L. S., et al. (2024). Effect of a Bacillus velezensis and Lysinibacillus fusiformis based biofertilizer on phosphorus acquisition and grain yield of soybean. Front. Plant Sci. 15, 5419–5430. doi: 10.3389/fpls.2024
Wen, M. M., Liu, Y., Yang, C. D., Dou, Y., Zhu, S. Q., Tan, G. Y., et al. (2024). Effects of manure and nitrogen fertilization on soil microbial carbon fixation genes and associated communities in the Loess Plateau of China. Sci. Total Environ. 954, 176581–176585. doi: 10.1016/j.scitotenv.2024.176581
Xin, R. L., Da, Y., Yu, Z., Qin, Y. L., Tian, L., Yang, S. D., et al. (2023). Changes in root metabolites and soil microbial community structures in rhizospheres of sugarcanes under different propagation methods. Microb. Biotechnol. 17, 578–581. doi: 10.1111/1751-7915.14372
Xin, G., Na, Y., Yan, Z., Liu, W. H., Li, T. F. (2022). Long-term watermelon continuous cropping leads to drastic shifts in soil bacterial and fungal community composition across gravel mulch fields. BMC Microbiol. 22, 189–189. doi: 10.1186/S12866-022-02601-2
Yan, L. Y., Li, P. Z., Yuan, P. L., Lei, H., Yang, H. Y., Shi, G. Y. (2022). Silicon fertilizer and microbial agents changed the bacterial community in the consecutive replant soil of lilies. Agronomy 12, 1525–1530. doi: 10.3390/AGRONOMY12071530
Yu, X. H., Xin, R. P., Xue, M. Z., Rui, L. H., Zong, S. L. (2022). Resource sustainability and challenges: Status and competitiveness of international trade in G. uralensis extracts under the belt and road initiative. Global Ecol. Conserv. 34, 451–456. doi: 10.1016/J.GECCO.2022.E02014
Zaeem, M., Nadeem, M., Pham, T. H., Ashiq, W., Ali, W., Gilani, S. S., et al. (2019). The potential of corn-soybean intercropping to improve the soil health status and biomass production in cool climate boreal ecosystems. Sci. Rep. 9, 13148–13152. doi: 10.1038/s41598-019-49558-3
Zhi, B. Y., Shu, H. D., Jia, W. Z., Li, D. Y., Liu, Y., Li, H., et al. (2022). Analysis of international competitiveness of the China’s G. uralensis industry from the perspective of global trade. J. Ethnopharmacol. 298, 115606–115611. doi: 10.1016/J.JEP.2022.115613
Zhu, S. Y., Wang, Y. Z., Xu, X. M., Liu, T. M., Wu, D. Q., Zheng, X., et al. (2018). Potential use of high-throughput sequencing of soil microbial communities for estimating the adverse effects of continuous cropping on ramie (Boehmeria nivea L. Gaud). PloS One 13, 497–502. doi: 10.1371/journal.pone.0197095
Keywords: microbial fertilizers, Glycyrrhiza uralensis, crop rotation, continuous cropping, soil microorganism
Citation: Qiu D, Wang X, Jiang K, Gong G and Bao F (2025) Effect of microbial fertilizers on soil microbial community structure in rotating and continuous cropping Glycyrrhiza uralensis. Front. Plant Sci. 15:1452090. doi: 10.3389/fpls.2024.1452090
Received: 20 June 2024; Accepted: 27 November 2024;
Published: 07 January 2025.
Edited by:
Xue Qiang Zhao, Chinese Academy of Sciences (CAS), ChinaReviewed by:
Arshad Jalal, King Abdullah University of Science and Technology, Saudi ArabiaRenuka Ratnayake, National Institute of Fundamental Studies (NIFS), Sri Lanka
Copyright © 2025 Qiu, Wang, Jiang, Gong and Bao. This is an open-access article distributed under the terms of the Creative Commons Attribution License (CC BY). The use, distribution or reproduction in other forums is permitted, provided the original author(s) and the copyright owner(s) are credited and that the original publication in this journal is cited, in accordance with accepted academic practice. No use, distribution or reproduction is permitted which does not comply with these terms.
*Correspondence: Daiyu Qiu, Z3NxZHlAMTYzLmNvbQ==