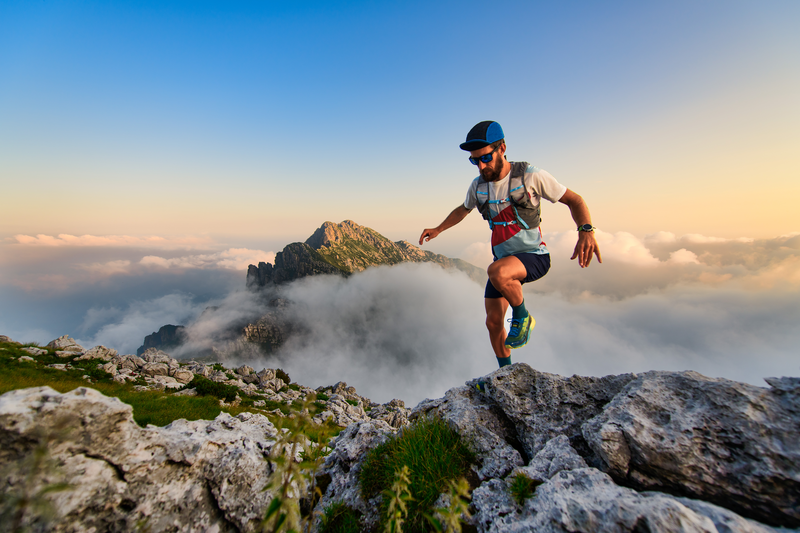
95% of researchers rate our articles as excellent or good
Learn more about the work of our research integrity team to safeguard the quality of each article we publish.
Find out more
ORIGINAL RESEARCH article
Front. Plant Sci. , 02 October 2024
Sec. Plant Nutrition
Volume 15 - 2024 | https://doi.org/10.3389/fpls.2024.1451573
This article is part of the Research Topic Application and Mechanism of Plant Biostimulants, Biochar, Fertilizer Products, and Other Nutrition-related Agrochemicals View all 28 articles
Despite the essential role of nitrogen fertilizers in achieving high crop yields, current application practices often exhibit low efficiency. Optimizing nitrogen (N) fertilization in agriculture is, therefore, critical for enhancing crop productivity while ensuring sustainable food production. This study investigates the effects of nitrification inhibitors (Nis) such as Dimethyl Pyrazole Phosphate (DMPP) and Dimethyl Pyrazole Fulvic Acid (DMPFA), plant growth-promoting bacteria inoculation, and phosphorus (P) application on the soil-plant-microbe system in maize. DMPFA is an organic nitrification inhibitor that combines DMP and fulvic acid for the benefits of both compounds as a chelator. A comprehensive rhizobox experiment was conducted, employing varying levels of P, inoculant types, and Nis, to analyze the influence of these factors on various soil properties, maize fitness, and phenotypic traits, including root architecture and exudate profile. Additionally, the experiment examined the effects of treatments on the bacterial and fungal communities within the rhizosphere and maize roots. Our results showed that the use of Nis improved plant nutrition and biomass. For example, the use of DMPFA as a nitrification inhibitor significantly improved phosphorus use efficiency by up to 29%, increased P content to 37%, and raised P concentration in the shoot by 26%, compared to traditional ammonium treatments. The microbial communities inhabiting maize rhizosphere and roots were also highly influenced by the different treatments. Among them, the N treatment was the major driver in shaping bacterial and fungal communities in both plant compartments. Notably, Nis reduced significantly the abundance of bacterial groups involved in the nitrification process. Moreover, we observed that each experimental treatment employed in this investigation could select, promote, or reduce specific groups of beneficial or detrimental soil microorganisms. Overall, our results highlight the intricate interplay between soil amendments, microbial communities, and plant nutrient dynamics, suggesting that Nis, particularly DMPFA, could be pivotal in bolstering agricultural sustainability by optimizing nutrient utilization.
In the intricate realm of soil fertility and nutrient management, comprehending the dynamics of phosphorus (P) acquisition is essential for optimizing agricultural productivity. Plant growth and development rely on phosphorus, which is vital for biochemical processes like energy transfer and nucleic acid synthesis. The inherent immobility and tendency to form insoluble compounds limit the availability of phosphorus in the soil. Thus, phosphorus fertilization is a common practice in modern agriculture. In most soils, phosphates that are easily accessible to plants typically make up less than 0.1% of the total soil phosphorus (Menezes-Blackburn et al., 2018). From 1961 to 2013, the nutrient utilization efficiency (NUE) for phosphorus fertilizers was estimated to be 16% globally (Dhillon et al., 2017). This low P efficiency is due to the strong tendency of soils to fix phosphorus, necessitating high application rates to maintain consistent yields, which in turn increases the risk of over-fertilization and raises production costs.
In the case of N fertilization, ammonium-based fertilizers have a stronger affinity for soil particles, thereby reducing the risk of N leaching, a common issue with nitrate fertilizers. However, in aerated soils, ammonium is quickly converted by nitrification into nitrate, which is highly mobile in most soils and can be easily leached. To address this issue, a method of choice involves chemically stabilizing ammonium fertilizers or ammonium-rich organic fertilizers using nitrification inhibitors (Nis) (Nkebiwe et al., 2016a). Furthermore, ammonium fertilization can additionally improve the acquisition of sparingly soluble nutrients, such as P, Fe, Zn, and Mn, due to rhizosphere acidification, a process induced by preferential ammonium uptake by the plant and enhanced nitrification (Marschner and Romheld, 1983; Neumann and Römheld, 2003). This effect can be further improved by localized application of P combined with ammonium fertilizers, leading to the formation of rhizosphere hot spots by local proliferation of lateral roots as an effective management strategy for improving nutrient use efficiency (Jing et al., 2010). In soils with lower pH, the same effect may be exploited to improve the acquisition of acid-soluble P fertilizers, such as rock-phosphate (RP) or P fertilizers based on products of waste recycling.
A sustainable approach to enhance plant nutrition and fitness involves the utilization of beneficial microbial inoculants. This methodology is gaining prominence in response to escalating pressure to adopt environmentally friendly agricultural practices. The transition toward more sustainable farming practices holds the potential to augment biodiversity, enhance soil resilience, and promote overall soil health. Plant growth promoting microorganisms (PGPM) have demonstrated the ability to stimulate plant growth through diverse mechanisms, including the modulation of phytohormone levels (Zubair et al., 2019), increased nutrient availability (Behr et al., 2023), and the induction of systemic resistance to protect plants against both below- and aboveground pathogens (Vlot et al., 2021). Concerning various nitrogen fertilization approaches, the stimulatory effects of stabilized ammonium fertilization on beneficial microorganisms employed as plant inoculants (such as selected strains of Pseudomonas, Bacillus, Paenibacillus, and Trichoderma) have been reported in both pot experiments and under field conditions, specifically in maize and tomato cultivation. These effects manifest in terms of enhanced root colonization and the promotion of root growth (Nkebiwe et al., 2016b; Mpanga et al., 2019a, b), leading to a more robust acquisition of nutrients, particularly P (Bradáčová et al., 2019; Mpanga et al., 2019a, b).
Recent research has further recognized the effect of humic acids on improving physio-chemical properties, microbial diversity, and enzyme activities in soils (Sharma et al., 2013; Pukalchik et al., 2019). Similar to beneficial microbes, humic acids and fulvic acids released from lignin-containing organic matter (Aggag et al., 2015), can act as efficient biostimulants, improving plant stress resistance and nutrient acquisition by stimulation of plasmalemma ATPases involved in nutrient uptake and root growth stimulation, solubilization of micro and macro elements, decreased active levels of toxic minerals and increased microbial populations (Yang et al., 2013; Canellas et al., 2015).
In 2021, DMPFA was designed by Malakshahi Kurdestani at the University of Hohenheim (Germany). This compound, which consists of DMP and fulvic acid (FA), functions as a double chelator. Due to their chelating properties, fulvic acids may also contribute to the inhibition of nitrification by the formation of metal complexes, with Cu and Fe otherwise acting as enzymatic co-factors for microbial nitrifiers. The DMPFA patent registration is currently being processed. The type of bonding between FA and DMP are previously reported (Mazzei et al., 2022).
This study investigates the effects of Nis and their interactions with bacteria on P acquisition in conditions where P is sparingly soluble. To evaluate the impact of various agricultural practices, including Nis application, P fertilization, and microbial inoculation, we conducted a greenhouse experiment. In this experiment, maize plants were cultivated in low P soil enriched with RP, Nis, and inoculated with Bacillus atrophaeus, with a control group included for comparison.
We hypothesized that Nis can enhance plant nutrient uptake and increase P availability in low P soils by triggering rhizosphere processes and promoting soil acidification, with a differential effect depending on the specific Nis employed. Additionally, we hypothesized that these processes would significantly alter the microbial community structure in the maize rhizosphere, as changes in soil properties induced by the various experimental treatments would be strongly correlated with shifts in microbial assemblage.
Starting from July 10th, 2022, our rhizobox experiment lasted for six weeks. A low P silt loam soil was used for this experiment. According to the VDLUFA method, the extractable P content with P-CAL was 8.7 mg kg-1, indicating class A (very low, VDLUFA, 2018). The pH of 6.4 in 10-2 M CaCl2 solution reflected near-neutral soil conditions, promoting optimal plant growth. The organic carbon concentration (Corg) was 2.2% and total N concentration (Nt) 0.02%. The substrate was a mixture of 70% soil and 30% quartz (w/w). The substrate was fertilized with P as RP: 150 mg kg-1 dry matter (DM); K as K2SO4: 150 mg kg-1DM. Nitrogen as ammonium and ammonium plus nitrification inhibitors was applied at a rate of 150 mg kg-1 DM.
The experiment was designed as 3-factorial experiment: (1) N fertilization in three levels, (2) P fertilization in 2 levels, and (3) inoculation in 2 levels. The experimental design followed a completely randomized design, with four replicates per treatment. In the experiment, three different N sources were used: (1) Ammonium sulfate (NH4)2SO4 as control, (2) Novatec® solub 21 ((NH4)2SO4 stabilized with DMPP (3,4-dimethylpyrazole phosphate)), and (3) Ammonium sulfate plus DMPFA ((NH4)2SO4 stabilized with 3,4-dimethylpyrazole fulvic acid). P fertilization was used in two levels: (1) rock phosphate (Dolophos 26® rock phosphate (11% P, 1.8% Mg)), and (2) control (without P application). Two levels of inoculation were employed: (1) Bacillus atrophaeus (RhizoVital® C5), and (2) control (without inoculation).
Each rhizobox contained 2 kg of substrate, watered to 65% of its maximum water-holding capacity. Three maize seeds (Zea mays var. Ronaldinio) were sown beneath the substrate surface, and after emergence, the plants were carefully thinned to one per pot.
The rhizoboxes were placed in a greenhouse at the University of Hohenheim, Stuttgart, Germany. The temperature ranged from 13 to 32°C throughout the growing period. Maize plants were harvested 42 days after planting.
DMPFA designed based on 40% m/v 3,4-Dimethyl-1H-pyrazol (CAS-Nummer: 2820-37-3), 20% v/v 2-Propanol as a solvent and 10 g/L fulvic acid. First, 2-Propanol was added to 3,4-Dimethyl-1H-pyrazole and the new solution was left for ten minutes at room temperature. Then fulvic acid was added, and the solution was placed on a magnetic plate and stirred for three hours at 40 Celsius degrees (Supplementary Figure S1). The structure of the used fulvic acid is described in García Díaz et al. (2015).
RhizoVital® C5 (Bacillus atrophaeus ABi05; (Pro: 1x108 CFU kg-1 substrate)) was utilized as a PGPM. Prior to sowing, maize seeds were soaked in the RhizoVital® C5 suspensions for one hour. The microbial interaction continued after the seeds were planted. We carefully inoculated 20 mL of the B. atrophaeus suspension into the seeding hole, promoting a localized and ongoing interaction between the plant roots and the PGPM used in this study. The aim of this approach was to create a mutually beneficial connection between the plant and B.atrophaeus ABi05, potentially improving nutrient absorption and overall plant well-being. In order to sustain this plant-microbial partnership, we introduced a thoughtful routine of two additional weekly applications of the PGPM suspension close to the plant stem.
The plant mineral nutrient status of the dried plant material (root, aboveground) was assessed by using an established wet-chemical extraction method after grinding the plant dry matter (VDLUFA, 2011). P concentration in the extract was measured at 436 nm using vanadate/molybdate as a color agent in the spectrophotometer (Gericke and Kurmis, 1952). K was measured by flame emission photometry and Mn, Zn, Fe, and Mg were determined by atomic absorption spectrometry. Phosphorus and nitrogen use efficiency (PUE and NUE) was calculated by using Equations 1 and 2, respectively.
During the final harvest after six weeks, maize plants were carefully removed from the rhizoboxes, and bulk soil was removed by shaking the plants. Soil still adhering to the roots was then sampled with a brush and defined as rhizosphere soil. The VDLUFA (1991) method was used to determine the soil pH. The shoot and root tissue biomasses were weighed fresh (FW), and their dry weight (DW) was recorded after being dried in an oven at 60°C. To determine root length, the washed roots were placed on transparent Perspex trays with a water film, and pictures were taken with a scanner (Epson, model Expression 1000 XL). The WinRHIZO software was used to calculate the length of the digitalized sample roots.
Just before the harvest of the maize plants from the rhizoboxes, moist sorption filters (5 mm Ø, filter paper: MN815, Macherey-Nagel, Düren, Germany) were placed on the surface of roots growing along the root observation window of the rhizoboxes, as outlined by Haase et al. (2008). Sampling was done for each rhizobox by applying the sorption filters in triplicate in subapical root zones (1–2 cm behind the root tip) and basal root zones (5–6 cm behind the root tip). To establish a negative control, sorption filters were placed on the soil where no visible root growth was present (soil without root contact). After a 4-hour incubation, the sorption filters from each of the rhizoboxes were collected and stored at -20°C (Neumann et al., 2014). Acetonitrile:H2O (1:1) was used to extract root exudates from sorption filters.
A portion of 20 μl was extracted from a 0.6 ml sorption filter and combined with 15 μl of ACCQFLUOR REAG derivatization agent (Waters, Milford, MA, USA) along with 65 μl of borate buffer. This mixture was then incubated at 55°C for 10 minutes. Afterwards, 400 μl of acetonitrile:H2O (1:4) was added, based on modifications to the method described by Cohen and Michaud (1993). The identification of specific amino acids (glutamic acid, asparagine, serine, glutamine, glycine, threonine, histidine, alanine, proline, cysteine, tyrosine, methionine, isoleucine, leucine, and phenylalanine) was carried out using HPLC-MS on a Velos LTQSystem (Thermo Fisher Scientific, Waltham, MA, USA) equipped with an ACCUTAGTM column (Waters, Milford, MA, USA) with 4 μm particle size and dimensions of 150 mm × 3.9 mm. External standards were employed for comparison. A gradient elution method was employed utilizing (A) ammonium formate:methanol:H2O (40:9:60) and (B) acetonitrile (Windisch et al., 2021).
Genomic DNA was extracted from the rhizosphere soil and root material by usingthe DNeasy PowerLyzer PowerSoil Kit (Qiagen). The universal primer pair 799f (Chelius and Triplett, 2001) and 1193r (Bodenhausen et al., 2013), which targets the V5-V7 region of the bacterial 16S rRNA gene, was used to characterize the bacterial community (Weisburg et al., 1991). The primer pair ITS1F/ITS2R was used to amplify the fungal internal transcribed spacer (ITS) rRNA region (White et al., 1990). The PCR protocols used were described in (Francioli et al., 2021). The obtained amplicons were sequenced on an Illumina MiSeq instrument with 2 × 300 base pair kits by LGC Genomics GmbH, Berlin, Germany. Demultiplexing was performed with Illumina bcl2fastq 2.17.1.14 software following the clipping of barcode and sequencing adapters. Only sequences with a quality score of above 20 were retained. DADA2 pipeline was used to determine amplicon sequence variants (ASV) from the raw sequences (Callahan et al., 2016). Alpha diversity metrics were calculated from the normalized sequence library, which was rarefied to 20,000 reads per sample for both microbial groups. Bacterial 16S ASV representative sequences were classified using the naive Bayesian classifier (Wang et al., 2007) for Silva 138. Representative sequences for fungal ITS ASVs were classified using the naive Bayesian classifier (Wang et al., 2007) against the Unite 9.0 reference database (Nilsson and Alexander, 2019). Amplicon sequence data were deposited at the European Nucleotide Archive under the BioProject accession number PRJEB73342 for bacterial and PRJEB73344 for fungal data. Furthermore, a B. atrophaeus ABi05 primer pair, designed from ABi05 genomic DNA sequences, was used for qPCR quantification of B. atrophaeus ABi05 DNA in the DNA samples according to the method described by Buttner et al. (2004). We only observed differences in the number of gene copies of B. atrophaeus ABi05 between the inoculated samples and the control for both rhizosphere and root compartments, while no significant differences were observed across the different treatments (Supplementary Figure S2).
Univariate Analysis of Variance (ANOVA) followed by Tukey’s honestly significant difference (HSD) post hoc test was used to test for differences in soil and plant properties among the different treatments. All the variables were tested for normality using Shapiro-Wilk and Jarque-Bera tests and the equality of group variances was examined using Levene’s test. A log10 transformation was applied to all variables that did not meet the parametric assumptions. Correlations among the soil and plant traits were determined using Spearman’s rank correlation. Differences in bacterial and fungal ASV richness (ASV detected in each sample) between the different treatments were estimated using ANOVA followed by a Tukey’s HSD post hoc test. For β-Diversity analysis, we first calculated Bray-Curtis dissimilarities using the Hellinger transformation (square root transformation of relative abundances; Legendre and Gallagher (2001)). Permutational multivariate analysis of variances (PERMANOVA) based on the Bray-Curtis dissimilarity index was performed to analyze the effect of the plant-soil compartment, the nitrogen and phosphorus fertilization, and the B. atrophaeus inoculation on the bacterial and fungal community structure, using 999 permutations for each test. The soil and plant properties were fitted to the nonmetric multidimensional scaling ordination using the “envfit” function in the “vegan” package of R, and goodness-of-fit statistics (R2) were calculated with p-values based on 999 permutations. The linear discriminant analysis effect size (LEfSe) (Segata et al., 2011) was applied to identify biomarker taxa explaining differences between the bacterial and fungal microbiota due to the above mentioned experimental factors in both plant-soil compartments. All the data were analyzed with R version 3.6 (R Core Team, 2014).
Shoot and root biomass were significantly affected by all three experimental factors, with nitrogen fertilization having the most pronounced effect (Tables 1, 2). Notably, the use of DMPFA increased the total maize biomass by 13% compared to the control treatment (Table 1; Figure 1). RP fertilization and B. atrophaeus inoculation also increased plant biomass, as shown in Table 2. DMPFA and RP had a significant effect on root length<0.2 mm diameter, and they significantly increased this trait compared to the control, while no effect was found with B. atrophaeus inoculation. An increase in the root length of the roots with a diameter of 0.2 to 0.6 mm was observed with the employment of Nis, RP, and B. atrophaeus. A similar result was observed for the root with a diameter larger than 0.6 mm. DMPFA and B. atrophaeus significantly increased the total root length, but RP had no effect (Supplementary Figure S3).
Table 1. Morphological and physiological response in maize (cv Ronaldinio) at 42 DAS (day after sowing) due to employing nitrification inhibitors compared with ammonium.
Table 2. Morphological and physiological response in maize (cv Ronaldinio) due to RP fertilization and Bacillus atrophaeus inoculation compared with control.
Figure 1. Root and shoot dry weight(g) in the treatments with ammonium, ammonium+ DMPFA and ammonium+ DMPP and phosphorus in two levels (Control and RP) and inoculation in two levels (Control and Bacillus atrophaeus) in maize (cv Ronaldinio) at 42 DAS. (Mean of four replications with standard error, Tukey test, p ≤ 0.05).
There were significant quantitative differences in the exuded compounds across the treatment groups. Notably, DMPFA and DMPP treatments resulted in a 195% and 135% increase in the exudation of sucrose, respectively, compared to the control plants (Table 1). RP also increases the exudate sucrose, while B. atrophaeus inoculation had no effect (Table 2; Supplementary Figure S4). Similarly, glucose concentration in the root exudates followed the same trend as sucrose. On the contrary, glutamine was shown to increase its concentration in the exudation profile along with Nis and B. atrophaeus application, while RP decreased its amount (Supplementary Figure S5). We lacked complete data on other root exudates due to their quantities being below detectable levels.
The soil pH was also affected by the experimental treatments. Using Nis and RP caused a significant decrease in soil pH, while B. atrophaeus inoculation did not have any additional effect (Figure 2). Acid phosphatase activity was increased under Nis and RP treatment, while B. atrophaeus inoculation decreased it. Contrary, alkaline phosphatase was solely increased by Nis.
Figure 2. Rhizosphere pH 42 DAS for ammonium, ammonium+ DMPFA and ammonium+ DMPP with phosphorus in two levels (Con and RP) and inoculation in two levels (Con and Bacillus atrophaeus) in maize. (Tukey test, p ≤ 0.05).
P concentration showed a 26% increase in DMPFA compared with the control, but no effect on P concentration was observed for DMPP (Table 1). While the use of B. atrophaeus did not show significant differences, RP application also increased P concentration. Accordingly, P content mirrored the results related to P concentration. Control and Nis treatment exhibited no significant difference in K concentration. Although inoculation did not show a significant difference, RP had a significant impact on reducing K concentration. The concentration of Ca in Nis was significantly larger than control, while RP treatment reduced it. Conversely, the concentration of some cations decreased when Nis were employed. For instance, both Nis treatments led to a decrease in Mn concentration, with DMPFA and DMPP showing reductions of 19% and 30%, respectively, compared to the control. Notably, DMPP application led to a decrease in Mg and Zn concentrations by 8% and 14%, respectively. PUE was significantly greater than control in the DMPFA treatment, with a 27% increase (Figure 3A). RP and B. atrophaeus treatments did not affect NUE and PUE (Table 2). NUE was increased under Nis, with DMFA and DMPP showing an increase compared to control of 18% and 12%, respectively (Figure 3B).
Figure 3. (A) Phosphorus use efficiency (PUE%) and (B) Nitrogen Use efficiency (NUE%) for ammonium, ammonium+ DMPFA and ammonium+ DMPP with inoculation in two levels (Con and Bacillus atrophaeus) in maize (cv Ronaldinio) at 42 DAS. (Tukey test, p ≤ 0.05).
By employing ammonium and Nis, separate clusters emerge, and traits are denoted by distinct lines that show in principal component analysis (PCA) of the plant nutritional and rhizosphere traits based on the type of nitrification inhibitors (Figure 4). Figure 5 depicts how morphological, physical, nutritional status, and biochemical plant indicators are linearly related. Pearson’s correlation coefficients heatmap demonstrated a strong positive correlation between P content, Ca content, acid and alkaline phosphatase activity, glucose, and sucrose with biomass. The heatmap further indicates a strong negative correlation between rhizosphere pH and all indicators, except for the Zn content.
Figure 4. Principal component analysis (PCA) of the plant nutritional and rhizosphere traits based on the type of nitrification inhibitors.
Figure 5. Heat map of Pearson’s correlation coefficients between plant traits (∗P< 0.05, ∗∗P< 0.01, ∗∗∗P< 0.001). B, biomass(g); FRL, fine root length (0<L<0.2mm); TRL, total root length (mm); Ca, Ca concentration mg/g dm; Fe, Fe concentration mg/g dm; K, K concentration mg/g dm; Mg, Mg concentration mg/g dm; Mn, Mn concentration mg/g dm; P, P concentration mg/g dm; Zn, Zn concentration mg/g dm; ACP, Acid phosphatase activity mg pNP/g Soil FM/hr; ALP, Alkaline phosphatase activity mg pNP/g Soil FM/hr; Glu, Glutamic acid µg/cm root; Ser, Serin µg/cm root; Asn, Asparagine µg/cm root; Gly, Glycine µg/cm root; Gln, Glutamine µg/cm root; Ala, Alanine µg/cm root; Gl, Glucose µg/cm root; Suc, Sucrose µg/cm root; R. pH, Rhizosphere pH.
A median of 40,009 bacterial 16S rRNA gene reads and 22671 ITS fungal high-quality reads were recovered from the 72 rhizosphere samples (12 treatments × 2 compartments (root and rhizosphere) x 3 replicates), clustering in 3,025 bacterial and, 1,056 fungal ASVs. Overall, bacterial sequences were attributed to 30 bacterial phyla, 79 classes, 179 orders, 273 families, and 488 genera. Proteobacteria were the most prevalent phylum in all treatments comprising 36.9% of the total bacterial reads, followed by Actinobacteria (25.6% of reads) and Verrucomicrobiota (12.1%) (Supplementary Figure S6). Candidatus_Udaeobacter (7.5% of reads), Streptomyces (5.6% of reads), and Massilia (5.4% of reads) were the most abundant bacterial genera across all samples. Bacterial richness ranged from 320 to 694 ASVs, with rhizosphere samples showing larger values than root samples; no significant differences were observed between treatments (Supplementary Figure S7).
Fungal sequences were assigned to 10 phyla, 29 classes, 63 orders, 121 families, 233 genera. Ascomycota (85.6% of reads) was the dominant fungal phylum, followed by Basidiomycota (7.5% of reads) (Supplementary Figure S6). Fusarium (17.4%), Penicillium (5.5%), and Neocosmospora (5.3%) were the most representative fungal genera in our dataset. As expected, and similar to bacteria, fungal richness was higher in the rhizosphere than in the root (ranging from 23 to 248 ASVs), and no significant differences were observed between the investigated treatments (Supplementary Figure S7).
Compartmentalization emerged as the primary factor driving maize microbiota assembly, capturing 49.1% of the bacterial and 32.4% of fungal community variation (Supplementary Table S1). PERMANOVA analysis revealed that the rhizosphere and roots were characterized by distinct microbiota, and principal coordinate analysis (PCoA) clearly reflected this finding (Figure 6). Within each investigated compartment, we observed substantial and significant effects of different N and P fertilization, as well as B. atrophaeus inoculation, on the maize microbiota structure (Tables 3, 4). The type of nitrogen fertilization represented the most critical factor for bacterial structure assembly in both compartments, accounting for 12.3% and 16.2% of the variation in the rhizosphere and root, respectively. The effect of differential N fertilization was also evident in the ordination plots, especially for the bacterial community associated with maize roots (Figure 6). B. atrophaeus inoculation significantly shaped the bacterial microbiome, capturing 6.6% of community variance in the root and 3.4% in the rhizosphere. Phosphorous fertilization marginally influenced the root bacterial community structure (3.2% of variation), while it had no effect on the rhizosphere bacteria assembly. We detected a significant interaction between N fertilization and B. atrophaeus inoculation, explaining approximately an additional 10% of the bacterial community variation in both compartments. Only in the root compartment we observe a significant interaction between N and P inoculation (6.6% of variation) and between B. atrophaeus inoculation and P fertilization (3.2%). A significant interaction among all three experimental variables on the bacterial community assembly was found in both compartments, accounting for 6.7% and 7.7% in the rhizosphere and root, respectively. These significant interactions suggest a differential response of the maize bacterial microbiota to the various experimental combinations established in the experiment.
Figure 6. Principal coordinates analysis (PCoA) of the Bray-Curtis dissimilarity metrics of the (A) bacterial and (B) fungal communities detected in this study.
Table 3. The effect of N fertilization, Bacillus inoculation and P fertilization on the bacterial community structure associated with the maize rhizosphere and root samples.
Table 4. The effect of N fertilization, Bacillus inoculation and P fertilization on the fungal community structure associated with the maize rhizosphere and root samples.
Similar to bacteria, the fungal community was primarily affected by N fertilization, capturing around 10.5% of community variation in both compartments. B. atrophaeus inoculation and P fertilization marginally affected fungal community structure, accounting for 4.4% variation in the rhizosphere and 3.9% variation in the root. An interaction between N fertilization and B. atrophaeus treatment was also found to be significant for fungal community assembly, explaining 10.6% and 8.1% of the variance in the rhizosphere and root, respectively. Fungal assembly was significantly influenced by the interaction between N and P fertilization, capturing 12.2% of variation in the roots and 6.7% in the rhizosphere. A significant interaction among all three experimental variables on fungal community structure was found 8.1% of community variance in the rhizosphere and 7.2% variation in the root.
Next, we assessed the contribution of measured edaphic properties to maize microbiota assembly. Soil pH, P, Fe, and Mn concentrations were significantly correlated with bacterial rhizosphere community assembly, while the bacterial root microbiome was significantly related to shifts in soil pH along with P, K, Mn, and Mg concentrations (Supplementary Table S2). Fungal community assembly in the rhizosphere was correlated with Zn concentration, whereas the maize root fungal population assembly dynamics were associated with Mg and Zn soil concentrations (Supplementary Table S3).
Since all three experimental variables (N, P, and B. atrophaeus inoculation) significantly influenced maize microbial assembly, we focused our analysis on samples that received RP with all N and B. atrophaeus combinations. The evident differences observed in bacterial beta-diversity between the fertilization treatments were mainly reflected in substantial shifts in the composition of the bacterial communities. Importantly, biomarker analysis indicated that these compositional shifts in the bacterial microbiota occurring in both compartments (rhizosphere and root) due to the application of different fertilizers were highly phylogenetically clustered (Figure 7). Indeed, an enrichment of the Actinobacteria classes Actinomycetia and Thermoleophilia, and the Proteobacteria class Alphaproteobacteria, was observed in soil samples receiving DMPFA without B. atrophaeus inoculation. Conversely, samples related to DMPPA receiving the B. atrophaeus treatment showed a significant increase in bacterial taxa affiliated with the class Gemmatimonadetes (phylum Gemmatimonadota), while the treatment DMPP without B. atrophaeus showed an enrichment in bacterial members associated with the Acidobacteria class Blastocatellia. Interestingly, soil samples associated with the ammonium treatment exhibited a significant increase in the sole detected bacterial genera involved in the nitrification process, such as Nitrosospira and Sphingomonas, with their abundance significantly lower in the other treatments, especially in the DMPP treatment (Supplementary Figure S8). Regarding nitrification, we also identified taxa associated with the archaeal family Nitrosotaleaceae, but their proportion was marginal and did not differ across the treatments.
Figure 7. LEfSe analysis at multiple taxonomic levels comparing maize bacterial community structure between the treatment investigated in (A) rhizosphere and (B) root compartments. Cladogram illustrating the taxonomic groups, explaining the most variation among root communities. Each ring represents a taxonomic level, with phylum (p_), class (c_), order (o_) and family (f_) emanating from the center to the periphery. Each circle is a taxonomic unit found in the dataset, with circles or nodes shown in colors (other than yellow) indicating where a taxon was significantly more abundant.
The bacteria community inhabiting maize roots also displayed strong phylogenetic shifts in the most dominant taxa due to the different treatments applied. For instance, root samples treated with ammonium and inoculated with B. atrophaeus showed an enrichment of bacteria associated with the Acidobacteriota phylum, while samples related to the treatment DMPFA + B. atrophaeus showed a significantly higher proportion of the phyla Proteobacteria and Fibrobacteriota compared to the other treatments. Contrarily, bacterial members affiliated with the Latescibacteriota phylum and the class Actinobacteria (Actinobacteriota phylum) were significantly more abundant in the DMPP without B. atrophaeus inoculation, while such N treatment together with B. atrophaeus showed a significantly higher proportion of the class Gammaproteobacteria (phylum Proteobacteria).
The fungal communities also exhibited a phylogenetic response to the different treatments, albeit not as pronounced as the bacterial population, reflecting the beta-diversity results (Figure 8). In the rhizosphere, for example, DMPFA + B. atrophaeus treatment was characterized by a significantly higher abundance of the classes Dothideomycetes (phylum Ascomycota) and Tremellomycetes (Basidiomycota). Taxa associated with the order Helotiales (Ascomycota) were enriched under ammonium treatment, especially with B. atrophaeus inoculation. Notably, fungi affiliated to the genus Alternaria, putative maize pathogens, were found significantly more abundant in the DMPP treatment, particularly without B. atrophaeus inoculation. Important shifts in the major fungal clades were also observed in the root compartment. Intriguingly, several Ascomycota classes were differentially enriched in the different treatments that did not involve B. atrophaeus inoculation. This was the case of Dothideomycetes in the ammonium treatment, Eurotiomycetes in DMPP and Sordariomycetes in DMPFA. Notably, taxa related to Fusarium, an important fungal genus encompassing important maize pathogenic fungi, were enriched under DMPFA. Penicillium and Trichoderma, two putative beneficial fungal genera, were found more abundant in the maize root under ammonium and DMPFA treatment, respectively.
Figure 8. LEfSe analysis at multiple taxonomic levels comparing maize fungal community structure between the treatment investigated in (A) rhizosphere and (B) root compartments. Cladogram illustrating the taxonomic groups, explaining the most variation among root communities. Each ring represents a taxonomic level, with phylum (p_), class (c_), order (o_) and family (f_) emanating from the center to the periphery. Each circle is a taxonomic unit found in the dataset, with circles or nodes shown in colors (other than yellow) indicating where a taxon was significantly more abundant.
The experiment showed how various Nis affect plant nutrient uptake, growth indicators, rhizosphere indicators, and soil microbial activity. While previous research (Mpanga et al., 2019a, b) has discussed the effects of microbial inoculation and Nis application, using DMPFA as a new nitrification inhibitor posed a challenge in this experiment.
Effects of Nis, B. atrophaeus, and RP on maize growth and nutrient uptake. The importance of roots less than 2 mm in diameter lies in their ability to take up water and nutrients and their rapid turnover rates (Block et al., 2006). Based on our results, the length of fine roots developed 29% more with DMPFA than with ammonium. Although the root length difference is not substantial for those roots with a bigger diameter, DMPFA shows a 24% increase in total root length compared to ammonium. The Nis likely resulted in a greater amount of root and shoot biomass due to the well-structured fine roots. In these scenarios, a larger root system that acidifies the soil might contribute to the solubilization of different phosphorus sources (such as Calcium-P, RP) and micronutrients. This would not only help plant species with limited capacity to mobilize nutrients through their roots, but also stimulate the development of mechanisms to solubilize sparingly soluble soil nutrients.
Ammonium fertilization further enhances the absorption of less soluble nutrients, such as P, Fe, Zn, and Mn, by inducing rhizosphere acidification. The study conducted by Ögüt et al. (2011) revealed that certain B. atrophaeus strains can boost proton release from roots due to ammonium, and also increase rhizosphere acid phosphatase activities in response to a lower rhizosphere pH. Rhizosphere acidification occurred in the treatment with a nitrification inhibitor in this experiment, despite the soil having a slightly acidic pH (6.4) prior to the experiment. Both Nis enhanced Ca, Fe, and P concentrations in the plant biomass, but there was no significant difference between K concentration between ammonium with and without Nis. Using RP decreased Ca and Fe concentration compared to the control, as was previously reported in another research (Martinengo et al., 2024; Mathan and Amberger, 1974). In a previous study, Dreyer et al. (2020) suggested that the use of Nis could cause decreased Mn availability in the bulk soil. In our experiment, we observed a 30% and 19% decrease in Mn concentration in DMPP and DPMFA compared to ammonium. Additionally, DMPP led to an 8% decrease in plant Mg concentration and a 14% decrease in Zn concentration compared to ammonium. In contrast, DMPFA did not exhibit any reduction in these cations. The negative correlation between ammonium and cation uptake is highlighted in some studies (Santos Silva et al., 2020; Bonomelli et al., 2021).
DMPFA application exhibited a significant difference in plant P concentration compared to ammonium alone, while no difference was observed between DMPP and ammonium. But P content in the plant was significantly larger for DMPFA and DMPP by 37% and 8% in comparison with ammonium, respectively. RP enhanced the plant P concentration in the treatment compared to the control. Previous research shows the increasing in P availability for maize with using nitrification inhibitors (Mpanga et al., 2019a). DMPFA induced a noteworthy 27% rise in PUE (Figure 3). However, there was no significant divergence between DMPP and ammonium alone. The NUE (%) in the DMPFA and DMPP treatments were significantly greater than that in the treatment with ammonium by 18% and 12% respectively, as expected. Utilizing DMP-based Nis has the potential to be a powerful strategy for reducing N losses (Guzman-Bustamante et al., 2022).
Phosphatases play a vital role in P acquisition for both plant roots and soil microbes. Phosphatase activity was significantly larger when Nis were used compared to ammonium alone. Compared with ammonium, an increasing 193% and 117% for acid phosphatase activity and 38% and 46% for alkaline phosphatase activity were observed when using DMPFA and DMPP, respectively. Increasing alkaline phosphatase activity and promote P release Our microbial analysis confirmed that the use of Nis resulted in greater activity in the root and microbial community, explaining the observed discrepancies. Application of Nis enhanced the secretion of amino acids from the roots. The increase in glutamine and asparagine in the DMPFA was remarkable.
Soil microbial communities are profoundly influenced by various agricultural practices, including fertilization (Francioli et al., 2016; Guo et al., 2020; Lewin et al., 2024), microbial inoculation (Mawarda et al., 2020; Berg et al., 2021; Behr et al., 2023) and the application of crop protection products (Cesco et al., 2021; Li et al., 2023; Sim et al., 2023). The presence of fulvic acid also controls the type of microbial community in the soil (Piccolo et al., 2019). Our study elucidates the significant impact of three experimental variables—nitrogen inhibitors (Nis), B. atrophaeus inoculation, and RP addition—on the community composition of maize rhizosphere and root microbiota. Notably, Nis emerges as the primary determinant shaping bacterial and fungal communities across both soil-plant compartments. The documented influence of Nis application on soil microbiota assembly (Hou et al., 2023; Liu et al., 2024) underscores its pivotal role in mediating microbial dynamics. Given the notable alterations induced by Nis application in soil properties, including reduced pH and modified concentrations of soil nutrients and root-exuded metabolites in the rhizosphere, corresponding shifts in microbial composition were expected. Indeed, observed changes in microbiota assembly across treatments were primarily associated with variations in soil pH and alterations in the concentrations of P, K, Fe, Mg, and Mn due to Nis application. Soil pH is recognized as a key factor influencing the structure of rhizosphere and root microbiota across diverse soils and ecosystems (Bardelli et al., 2017; Tripathi et al., 2018; Shi et al., 2022). Similarly, soil nutrient concentrations and availability are recognized as significant drivers shaping rhizosphere and root microbiota, with their alterations holding substantial consequences for microbial assembly processes (Francioli et al., 2020; Custódio et al., 2022; Mang et al., 2023). Among microbial groups significantly responding to Nis application, a marked decrease in the relative abundance of ammonia-oxidizing bacteria (AOB) affiliated with the genus Nitrosospira was observed. Nitrosospira represents the primary nitrifying bacterial group detected in our study, capable of oxidizing ammonia, a crucial initial step in the nitrification process. Both Nis treatments employed in our experiment reduced Nitrosospira abundance compared to the control treatment (sole ammonium fertilization), with DMPP demonstrating greater efficacy in depleting bacterial taxa associated with this genus. These findings align with prior research indicating DMPP application significant reduction of AOB abundance (Lu et al., 2019; Bachtsevani et al., 2021) and provide novel insights into the DMPFA effect in diminishing the AOB soil community. The negative influence of Nis on the AOB community may occur through direct inhibition of specific nitrifying bacteria responsible for ammonia oxidation, altering microbial composition in the soil, or through indirect mechanisms involving modifications in nutrient availability, shifts in soil pH, and alterations in competitive microbial interactions (Hayatsu et al., 2008). In this line, it has been reported that the growth rate of AOB decreases with decreasing pH because NH3, the actual substrate of AOB, occurs only at very low concentrations when soil pH is less than 6, even at high ammonium concentrations (Suzuki et al., 1974). This observation further supports the negative indirect effect of Nis on the AOB community through decreased soil pH observed in our study.
The inoculation with B. atrophaeus and the addition of RP exhibited a significant, though modest, direct impact on the microbiota structure associated with the maize root and rhizosphere. Notably, B. atrophaeus successfully established and persisted in the maize rhizosphere (Supplementary Figure S2), consistent with other studies highlighting its strong capability to colonize the rhizosphere of maize and other crops affiliated with the Poaceae family (Andrić et al., 2020; Mahdi et al., 2021; Behr et al., 2023; Zuo et al., 2023). The establishment of B. atrophaeus in the rhizosphere of our maize plants significantly altered the indigenous soil microbial community composition and structure, aligning with recent findings (Behr et al., 2023; Hou et al., 2024), and this effect was more pronounced in the treatments where B. atrophaeus was coupled with Nis and RP use. Importantly, a significant interaction among all three experimental variables was observed, accounting for considerable variance in microbiota composition. This suggests a potential differential response of bacterial and fungal communities to the various treatment combinations investigated in this study. For instance, B. atrophaeus inoculation combined with DMPP and RP supplementation increased the abundance of Gemmatimonadetes in maize rhizosphere, an important bacterial class contributing to soil organic carbon cycling due to its metabolic strategies (Liu et al., 2020). Actinobacteria, notable for their role in promoting agricultural soil quality as remarkable organic matter decomposers (Strap, 2011), exhibited significantly higher abundance in soils treated with DMPFA and RP compared to other soils surveyed. The utilization of DMPFA, RP, and B. atrophaeus enriched the proportion of Proteobacteria in maize roots, the largest bacterial phylum known for its metabolic versatility and genetic diversity. Members of this phylum have generally been described as copiotrophic bacteria (Fierer et al., 2007; Lienhard et al., 2014; Francioli et al., 2016), which are fast-growing organisms that prefer nutrient-rich environments. Since the application of DMFPA and RP showed a significant increase in almost all the measured macro- and micronutrients, especially with the DMFPA application, it is likely that this treatment promoted the growth of Proteobacteria populations. Moreover, certain treatment combinations selectively promoted or diminished specific groups of soil microorganisms, which could be categorized as either beneficial or detrimental. For instance, taxa associated with putative beneficial fungal genera such as Penicillium and Trichoderma exhibited enrichment under the influence of ammonium and DMPFA treatments, respectively, when supplemented with RP. On the contrary, taxa related to Fusarium, an important fungal genus encompassing important maize pathogenic fungi, were enriched in the DMPFA and RP treatment. Our findings demonstrate that specific management practices can selectively enrich, promote, or suppress specific populations of soil microorganisms. This highlights the importance of furthering our understanding of how various management strategies impact soil and plant-associated microbial communities and the associated soil microbial processes. Such knowledge is crucial for developing sustainable agricultural production systems that optimize crop yields.
Despite being used at low application rates, Nis may be affected in the rhizosphere by the synergistic effects of fulvic acid as a chelator and bio stimulant, along with DMP as a chelator and nitrification inhibitor. Further experiments are needed to explore this.
DMPFA has the potential to increase PUE, P content, and P concentration in maize, whereas DMPP only increases P content. Nis, especially DMPFA, may influence the soil microorganisms responsible for releasing Mg, Zn, Fe. As Nis alters microbial activity, it can affect the availability of cation negatively. While Nis enhanced plant biomass and growth indicators, there were some decreases in cation concentrations that should be considered in stress and diseased conditions. The interaction between Nis and inoculation, as well as P in its sparingly soluble form, affects the biotic and abiotic indices in maize. Further experimentation with DMPFA across different soil conditions and plant species is necessary to fully understand its potential.
The datasets presented in this study can be found in online repositories. The names of the repository/repositories and accession number(s) can be found in the article/Supplementary Material.
AM: Conceptualization, Data curation, Formal analysis, Investigation, Methodology, Project administration, Resources, Software, Validation, Visualization, Writing – original draft, Writing – review & editing. DF: Data curation, Formal analysis, Investigation, Methodology, Software, Supervision, Validation, Visualization, Writing – original draft, Writing – review & editing, Resources. RR: Conceptualization, Data curation, Formal analysis, Investigation, Methodology, Project administration, Supervision, Validation, Visualization, Writing – review & editing, Resources, Software. AP: Writing – review & editing. NM: Formal analysis, Writing – review & editing. XC: Data curation, Formal analysis, Methodology, Supervision, Validation, Visualization, Writing – review & editing. TM: Conceptualization, Data curation, Formal analysis, Funding acquisition, Investigation, Methodology, Project administration, Resources, Software, Supervision, Validation, Visualization, Writing – original draft, Writing – review & editing.
The author(s) declare financial support was received for the research, authorship, and/or publication of this article. The project was funded by the Deutsche Forschungsgemeinschaft (DFG, German Research Foundation)-328017493/GRK 2366 (Sino-German International Research Training Group AMAIZE-P).
The authors declare that the research was conducted in the absence of any commercial or financial relationships that could be construed as a potential conflict of interest.
All claims expressed in this article are solely those of the authors and do not necessarily represent those of their affiliated organizations, or those of the publisher, the editors and the reviewers. Any product that may be evaluated in this article, or claim that may be made by its manufacturer, is not guaranteed or endorsed by the publisher.
The Supplementary Material for this article can be found online at: https://www.frontiersin.org/articles/10.3389/fpls.2024.1451573/full#supplementary-material
Aggag, A. M., Alzoheiry, A. M., Abdallah, A. E. (2015). Effect of kaolin and fulvic acid anti-transpirants on tomato plants grown under different water regimes. Alexandria Sci. Exchange J. 36, 169–179. doi: 10.21608/asejaiqjsae.2015.2875
Andrić, S., Meyer, T., Ongena, M. (2020). Bacillus responses to plant-associated fungal and bacterial communities. Front. Microbiol. 11. doi: 10.3389/fmicb.2020.01350
Bachtsevani, E., Papazlatani, C. V., Rousidou, C., Lampronikou, E., Menkissoglu-Spiroudi, U., Nicol, G. W., et al. (2021). Effects of the nitrification inhibitor 3,4-dimethylpyrazole phosphate (DMPP) on the activity and diversity of the soil microbial community under contrasting soil pH. Biol. Fertil. Soils 57, 1117–1135. doi: 10.1007/s00374-021-01602-z
Bardelli, T., Gómez-Brandón, M., Ascher-Jenull, J., Fornasier, F., Arfaioli, P., Francioli, D., et al. (2017). Effects of slope exposure on soil physico-chemical and microbiological properties along an altitudinal climosequence in the Italian Alps. Sci. total Environ. 575, 1041–1055. doi: 10.1016/j.scitotenv.2016.09.176
Behr, J. H., Kampouris, I. D., Babin, D., Sommermann, L., Francioli, D., Kuhl-Nagel, T., et al. (2023). Beneficial microbial consortium improves winter rye performance by modulating bacterial communities in the rhizosphere and enhancing plant nutrient acquisition. Front. Plant Sci. 14. doi: 10.3389/fpls.2023.1232288
Berg, G., Kusstatscher, P., Abdelfattah, A., Cernava, T., Smalla, K. (2021). Microbiome modulation—Toward a better understanding of plant microbiome response to microbial inoculants. Front. Microbiol. 12. doi: 10.3389/fmicb.2021.650610
Block, R., Van Rees, K., Knight, J. (2006). A review of fine root dynamics in Populus plantations. Agroforestry Syst. 67, 73–84. doi: 10.1007/s10457-005-2002-7
Bodenhausen, N., Horton, M. W., Bergelson, J. (2013). Bacterial communities associated with the leaves and the roots of arabidopsis thaliana. PloS One 8, e56329. doi: 10.1371/journal.pone.0056329
Bonomelli, C., de Freitas, S. T., Aguilera, C., Palma, C., Garay, R., Dides, M., et al. (2021). Ammonium excess leads to Ca restrictions, morphological changes, and nutritional imbalances in tomato plants, which can be monitored by the N/Ca ratio. Agronomy 11, 1437. doi: 10.3390/agronomy11071437
Bradáčová, K., Florea, A. S., Bar-Tal, A., Minz, D., Yermiyahu, U., Shawahna, R., et al. (2019). Microbial consortia versus single-strain inoculants: an advantage in PGPM-assisted tomato production. Agronomy 9, 105. doi: 10.3390/agronomy9020105
Buttner, M. P., Cruz, P., Stetzenbach, L. D., Klima-Comba, A. K., Stevens, V. L., Cronin, T. D. (2004). Determination of the efficacy of two building decontamination strategies by surface sampling with culture and quantitative PCR analysis. Appl. Environ. Microbiol. 70, 4740–4747. doi: 10.1128/AEM.70.8.4740-4747.2004
Callahan, B. J., Sankaran, K., Fukuyama, J. A., McMurdie, P. J., Holmes, S. (2016). Bioconductor Workflow for Microbiome Data Analysis: from raw reads to community analyses [version 2; peer review: 3 approved]. F1000Research 5, 1492. doi: 10.12688/f1000research.8986.2
Canellas, L. P., Olivares, F., Aguiar, N., Jones, D., Nebbioso, A., Mazzei, P., et al. (2015). Humic and fulvic acids as biostimulants in horticulture. Scientia Hortic. 182, 78–92. doi: 10.1016/j.scienta.2015.09.013
Cesco, S., Lucini, L., Miras-Moreno, B., Borruso, L., Mimmo, T., Pii, Y., et al. (2021). The hidden effects of agrochemicals on plant metabolism and root-associated microorganisms. Plant Sci. 311, 111012. doi: 10.1016/j.plantsci.2021.111012
Chelius, M. K., Triplett, E. W. (2001). The diversity of archaea and bacteria in association with the roots of zea mays L. Microbial. Ecol. 41, 252–263. doi: 10.1007/s002480000087
Cohen, S. A., Michaud, D. P. (1993). Synthesis of a fluorescent derivatizing reagent, 6-Aminoquinolyl-N-Hydroxysuccinimidyl Carbamate, and its application for the analysis of hydrolysate amino acids via high-performance liquid chromatography. Analytical Biochemistry 211, 279–287. doi: 10.1006/abio.1993.1270
Custódio, V., Gonin, M., Stabl, G., Bakhoum, N., Oliveira, M. M., Gutjahr, C., et al. (2022). Sculpting the soil microbiota. Plant J. 109, 508–522. doi: 10.1111/tpj.15568
Dhillon, J., Torres, G., Driver, E., Figueiredo, B., Raun, W. R. (2017). World phosphorus use efficiency in cereal crops. Agron. J. 109, 1670–1677. doi: 10.2134/agronj2016.08.0483
Dreyer, M., Wichmann, M., Rischen, M., Görlach, B. M., Ehmke, A., Pitann, B., et al. (2020). Ammonium-driven nitrification plays a key role in increasing Mn availability in calcareous soils. J. Plant Nutr. Soil Sci. 183, 389–396. doi: 10.1890/05-1839
Fierer, N., Bradford, M. A., Jackson, R. B. (2007). Toward an ecological classification of soil bacteria. Ecology 88, 1354–1364. doi: 10.1890/05-1839
Francioli, D., Cid, G., Kanukollu, S., Ulrich, A., Hajirezaei, M.-R., Kolb, S. (2021). Flooding causes dramatic compositional shifts and depletion of putative beneficial bacteria on the spring wheat microbiota. Front. Microbiol. 12. doi: 10.3389/fmicb.2021.773116
Francioli, D., Schulz, E., Lentendu, G., Wubet, T., Buscot, F., Reitz, T. (2016). Mineral vs. Organic amendments: microbial community structure, activity and abundance of agriculturally relevant microbes are driven by long-term fertilization strategies. Front. Microbiol. 7. doi: 10.3389/fmicb.2016.01446
Francioli, D., van Ruijven, J., Bakker, L., Mommer, L. (2020). Drivers of total and pathogenic soil-borne fungal communities in grassland plant species. Fungal Ecol. 48, 100987. doi: 10.1016/j.funeco.2020.100987
García Díaz, C., Nebbioso, A., Piccolo, A., Barrera, J., Martínez-Palou, R. (2015). Remediation of hydrocarbon-contaminated soil by washing with novel chemically modified humic substances. J. Environ. Qual. 44. doi: 10.2134/jeq2014.09.0399
Gericke, S., Kurmis, B. (1952). The colorimetric determination of phosphoric acid with ammonium vanadate-molybdate and its application in plant analysis. Z. Plant Nutr. Soil 59, 235–247. doi: 10.1002/j.1522-2624.1952.tb00066.x
Guo, Z., Wan, S., Hua, K., Yin, Y., Chu, H., Wang, D., et al. (2020). Fertilization regime has a greater effect on soil microbial community structure than crop rotation and growth stage in an agroecosystem. Appl. Soil Ecol. 149, 103510. doi: 10.1016/j.apsoil.2020.103510
Guzman-Bustamante, I., Schulz, R., Müller, T., Müller, T. (2022). Split N application and DMP based Nis mitigate N2O losses in a soil cropped with winter wheat. Nutrient Cycl. Agroecosys. 123, 119–135. doi: 10.1007/s10705-022-10211-7
Haase, S., Philippot, L., Neumann, G., Marhan, S., Kandeler, E. (2008). Local response of bacterial densities and enzyme activities to elevated atmospheric CO2 and different N supply in the rhizosphere of Phaseolus vulgaris L. Soil Biol. Biochem. 40, 1225–1234. doi: 10.1016/j.soilbio.2007.12.025
Hayatsu, M., Tago, K., Saito, M. (2008). Various players in the nitrogen cycle: Diversity and functions of the microorganisms involved in nitrification and denitrification. Soil Sci. Plant Nutr. 54, 33–45. doi: 10.1111/j.1747-0765.2007.00195.x
Hou, P., Zhao, S., He, S., Zhou, Y., Petropoulos, E., Willett, I., et al. (2023). Effect of nitrification inhibitor addition on rice N utilization and soil bacterial community. J. Soil Sci. Plant Nutr. 23, 1019–1029. doi: 10.1007/s42729-022-01100-7
Hou, Y., Wei, C., Zeng, W., Hou, M., Wang, Z., Xu, G., et al. (2024). Application of rhizobacteria to improve microbial community structure and maize (Zea mays L.) growth in saline soil. Environ. Sci. Pollut. Res. 31, 2481–2494. doi: 10.1007/s11356-023-31361-3
Jing, J., Ruia, Y., Zhanga, F., Rengel, Z., Shen, J. (2010). Localized application of phosphorus and ammonium improves growth of maize seedlings by stimulating root proliferation and rhizosphere acidification. Field Crops Res. 119, 355–364. doi: 10.1016/j.fcr.2010.08.005
Legendre, P., Gallagher, E. D. (2001). Ecologically meaningful transformations for ordination of species data. Oecologia 129, 271–280. doi: 10.1007/s004420100716
Lewin, S., Schaller, J., Kolb, S., Francioli, D. (2024). Amorphous silica fertilization ameliorated soil properties and promoted putative soil beneficial microbial taxa in a wheat field under drought. Appl. Soil Ecol. 196, 105286. doi: 10.1016/j.apsoil.2024.105286
Li, J., Luo, J., Lindsey, S., Wang, S., Deng, F., Wang, W. (2023). Changes in soil microbial communities in response to repeated application of nitrification inhibitors. Appl. Soil Ecol. 182, 104726. doi: 10.1016/j.apsoil.2022.104726
Lienhard, P., Terrat, S., Prévost-Bouré, N. C., Nowak, V., Régnier, T., Sayphoummie, S., et al. (2014). Pyrosequencing evidences the impact of cropping on soil bacterial and fungal diversity in Laos tropical grassland. Agron. Sustain. Dev. 34, 525–533. doi: 10.1007/s13593-013-0162-9
Liu, R., Wang, Z., Wang, L., Li, Z., Fang, J., Wei, X., et al. (2020). Bulk and active sediment prokaryotic communities in the Mariana and Mussau Trenches. Front. Microbiol. 11. doi: 10.3389/fmicb.2020.01521
Liu, Y., Wang, W., Nessa, A., Yang, S., Zhang, W., Zhang, M. (2024). Metagenomic insight into antagonistic effects of a nitrification inhibitor and phosphate-solubilizing bacteria on soil protease activity. Land Degrad. Dev. 35, 455–466. doi: 10.1002/ldr.4927
Lu, Y., Zhang, X., Jiang, J., Kronzucker, H. J., Shen, W., Shi, W. (2019). Effects of the biological nitrification inhibitor 1,9-decanediol on nitrification and ammonia oxidizers in three agricultural soils. Soil Biol. Biochem. 129, 48–59. doi: 10.1016/j.soilbio.2018.11.008
Mahdi, I., Fahsi, N., Hafidi, M., Benjelloun, S., Allaoui, A., Biskri, L. (2021). Rhizospheric phosphate solubilizing Bacillus atrophaeus GQJK17 S8 increases quinoa seedling, withstands heavy metals, and mitigates salt stress. Sustainability 13, 3307. doi: 10.3390/su13063307
Mang, M., Maywald, N. J., Li, X., Ludewig, U., Francioli, D. (2023). Nitrogen fertilizer type and genotype as drivers of P acquisition and rhizosphere microbiota assembly in juvenile maize plants. Plants 12, 544. doi: 10.3390/plants12030544
Marschner, H., Romheld, V. (1983). In Vivo Measurement of Root-Induced pH Changes at the Soil-Root Interface: Effect of Plant Species and Nitrogen Source. Z. für Pflanzenphysiol. 111, 249–251. doi: 10.1016/S0044-328X(83)80083-X
Martinengo, S., Santoro, V., Schiavon, M., Celi, L., Martin, M., Said-Pullicino, D. (2024). The influence of phosphorus availability on rice root traits driving iron plaque formation and dissolution, and implications for phosphorus uptake. Plant Soil 494, 603–616. doi: 10.1007/s11104-023-06306-x
Mathan, K. K., Amberger, A. (1977). Influence of iron on the uptake of phosphorus by maize. Plant Soil 46, 413–422. doi: 10.1007/BF00010097
Mawarda, P. C., Le Roux, X., van Elsas, J. D., Salles, J. F. (2020). Deliberate introduction of invisible invaders: A critical appraisal of the impact of microbial inoculants on soil microbial communities. Soil Biol. Biochem. 148, 107874. doi: 10.1016/j.soilbio.2020.107874
Mazzei, P., Cangemi, S., Malakshahi Kurdestani, A., Mueller, T., Piccolo, A. (2022). Quantitative evaluation of noncovalent interactions between 3,4-dimethyl-1H-pyrazole and dissolved humic substances by NMR spectroscopy. Environ. Sci. Technol. 56, 11771–11779. doi: 10.1021/acs.est.2c00900
Menezes-Blackburn, D., Giles, C., Darch, T., George, T. S., Blackwell, M., Stutter, M., et al. (2018). Opportunities for mobilizing recalcitrant phosphorus from agricultural soils: a review. Plant Soil 427, 5–16. doi: 10.1007/s11104-017-3362-2
Mpanga, I. K., Gomez-Genao, N. J., Moradtalab, N., Wanke, D., Chrobaczek, V., Ahmed, A., et al. (2019a). The role of N form supply for PGPM-host plant interactions in maize. Plant Nutr. Soil Sci. 182, 908–920. doi: 10.1002/jpln.201900133
Mpanga, I. K., Nkebiwe, P. M., Kuhlmann, M., Cozzolino, V., Piccolo, A., Geistlinger, J., et al. (2019b). The form of N supply determines plant growth promotion by P-solubilizing microorganisms in maize. Microorganisms 7, 38. doi: 10.3390/microorganisms7020038
Neumann, G., Römheld, V. (2003). Root-induced changes in the availability of nutrients in the rhizosphere. ChemInform. 34. doi: 10.1002/chin.200304265
Neumann, G., Bott, S., Ohler, M., Mock, H.-P., Lippmann, R., Grosch, R., et al. (2014). Root exudation and root development of lettuce (Lactuca sativa L. cv. Tizian) as affected by different soils. Front. Microbiol. 5. doi: 10.3389/fmicb.2014.00002
Nilsson, E. M., Alexander, R. W. (2019). Bacterial wobble modifications of NNA-decoding tRNAs. IUBMB Life 71, 1158–1166. doi: 10.1002/iub.2120
Nkebiwe, P. M., Weinmann, M., Bar-Tal, A. (2016a). Fertilizer placement to improve crop nutrient acquisition and yield: a review and meta-analysis. Field Crops Res. 196, 389–401. doi: 10.1016/j.fcr.2016.07.018
Nkebiwe, P. M., Weinmann, M., Müller, T. (2016b). Improving fertilizer-depot exploitation and maize growth by inoculation with plant growth-promoting bacteria: from lab to field. Chem. Biol. Technol. Agric. 3, 15. doi: 10.1186/s40538-016-0065-5
Ögüt, M., Er, F., Neumann, G. (2011). Increased proton extrusion of wheat roots by inoculation with phosphorus-solubilizing microorganisms. Plant Soil 339, 285–297. doi: 10.1007/s11104-010-0578-9
Piccolo, A., Spaccini, R., Savy, D., Drosos, M., Cozzolino, V. (2019). “The Soil Humeome: Chemical Structure, Functions and Technological Perspectives,” in Sustainable Agrochemistry. Ed. Vaz, S., Jr. (Cham, Switzerland: Springer), 183–222. doi: 10.1007/978-3-030-17891-8_7
Pukalchik, M., Kydralieva, K., Yakimenko, O. (2019). Outlining the potential role of humic products in modifying biological properties of the soil- A review. Front. Environ. Sci. 80. doi: 10.3389/fenvs.2019.00080
R Development Core Team (2014). R: A Language and Environment for Statistical Computing, (Vienna, Austria: R Foundation for Statistical Computing).
Santos Silva, B., de Mello Prado, R., Calero Hurtado, A., Aparecida de Andrade, R., Pereira da Silva, G. (2020). Ammonia toxicity affects cations uptake and growth in papaya plants inclusive with silicon addition. Acta Biológica Colombiana 25, 345–353. doi: 10.15446/abc.v25n3.79490
Segata, N., Izard, J., Waldron, L., Gevers, D., Miropolsky, L., Garrett, W. S., et al. (2011). Metagenomic biomarker discovery and explanation. Genome Biol. 12, R60. doi: 10.1186/gb-2011-12-6-r60
Sharma, S. B., Sayyed, R. Z., Trivedi, M. H., Gobi, T. A. (2013). Phosphate solubilizing microbes: sustainable approach for managing phosphorus deficiency in agricultural soils. SpringerPlus 2, 587. doi: 10.1186/2193-1801-2-587
Shi, Y., Xu, M., Zhao, Y., Cheng, L., Chu, H. (2022). Soil pH determines the spatial distribution, assembly processes, and co-existence networks of microeukaryotic community in wheat fields of the North China plain. Front. Microbiol. 13. doi: 10.3389/fmicb.2022.911116
Sim, J. X. F., Drigo, B., Doolette, C. L., Vasileiadis, S., Donner, E., Karpouzas, D. G., et al. (2023). Repeated applications of fipronil, propyzamide, and flutriafol affect soil microbial functions and community composition: A laboratory-to-field assessment. Chemosphere 331, 138850. doi: 10.1016/j.chemosphere.2023.138850
Strap, J. L. (2011). “Actinobacteria–Plant Interactions: A Boon to Agriculture,” in Bacteria in Agrobiology: Plant Growth Responses. Ed. Maheshwari, D. (Berlin, Heidelberg: Springer), 203–218. doi: 10.1007/978-3-642-20332-9_13
Suzuki, I., Dular, U., Kwok, S. (1974). Ammonia or ammonium ion as substrate for oxidation by Nitrosomonas Europaea cells and extracts. J. Bacteriol. 120, 556–558. doi: 10.1128/jb.120.1.556-558.1974
Tripathi, B. M., Stegen, J. C., Kim, M., Dong, K., Adams, J. M., Lee, Y. K. (2018). Soil pH mediates the balance between stochastic and deterministic assembly of bacteria. ISME J. 12, 1072–1083. doi: 10.1038/s41396-018-0082-4
VDLUFA (2018). Phosphordüngung nach Bodenuntersuchung und Pflanzenbedarf (Speyer: Verband Deutscher Landwirtschaftlicher Untersuchungs- und Forschungsanstalten).
VDLUFA Verlag (2011). “VDLUFA Methode 2.1.3: Mikrowellenbeheitzer Druckaufschluss,” in Handbuch Der Landwirtschaftlichen Versuchs-Und Untersuchungsmethodik (VDLUFA Methodenbuch); Band VII Umweltanalytik, vol. 4. (Darmstadt, Germany: Aufl. VDLUFA Verlag).
Vlot, A. C., Sales, J. H., Lenk, M., Bauer, K., Brambilla, A., Sommer, A., et al. (2021). Systemic propagation of immunity in plants. New Phytol. 229, 1234–1250. doi: 10.1111/nph.16953
Wang, Q., Garrity, G. M., Tiedje, J. M., Cole, J. R. (2007). Naive Bayesian classifier for rapid assignment of rRNA sequences into the new bacterial taxonomy. Appl. Environ. Microbiol. 73, 5261–5267. doi: 10.1128/AEM.00062-07
Weisburg, W. G., Barns, S. M., Pelletier, D. A., Lane, D. J. (1991). 16S ribosomal DNA amplification for phylogenetic study. J. bacteriol. 173, 697–703. doi: 10.1128/jb.173.2.697-703.1991
White, T. J., Bruns, T., Lee, S., Taylor, J. (1990). “Amplification and direct sequencing of fungal ribosomal RNA genes for phylogenetics,” in PCR Protocols. Eds. Innis, M. A., Gelfand, D. H., Sninsky, J. J., White, T. J. (San Diego, CA: Academic Press), 315–322. doi: 10.1016/B978-0-12-372180-8.50042-1
Windisch, S., Sommermann, L., Babin, D., Chowdhury, S. P., Grosch, R., Moradtalab, N., et al. (2021). Impact of long-term organic and mineral fertilization on rhizosphere metabolites, root–microbial interactions and plant health of lettuce. Front. Microbiol. 11. doi: 10.3389/fmicb.2020.597745
Yang, S., Zhang, Z., Cong, L., Wang, X., Shi, S. (2013). Effect of fulvic acid on the phosphorus availability in acid soil. J. Soil Sci. Plant Nutr. 13, 526–533. doi: 10.4067/S0718-95162013005000041
Zubair, M., Hanif, A., Farzand, A., Sheikh, T. M. M., Khan, A. R., Suleman, M., et al. (2019). Genetic screening and expression analysis of psychrophilic bacillus spp. Reveal their potential to alleviate cold stress and modulate phytohormones in wheat. Microorganisms 7, 337. doi: 10.3390/microorganisms7090337
Keywords: nitrification inhibitors (NIs), phosphorus acquisition, DMPFA (Dimethyl pyrazole fulvic acid), nutrient uptake, soil microbial activity
Citation: Malakshahi Kurdestani A, Francioli D, Ruser R, Piccolo A, Maywald NJ, Chen X and Müller T (2024) Optimizing nitrogen fertilization in maize: the impact of nitrification inhibitors, phosphorus application, and microbial interactions on enhancing nutrient efficiency and crop performance. Front. Plant Sci. 15:1451573. doi: 10.3389/fpls.2024.1451573
Received: 19 June 2024; Accepted: 04 September 2024;
Published: 02 October 2024.
Edited by:
Roberto Ruggeri, University of Tuscia, ItalyReviewed by:
Simon Xu, Chinese Academy of Agricultural Sciences, ChinaCopyright © 2024 Malakshahi Kurdestani, Francioli, Ruser, Piccolo, Maywald, Chen and Müller. This is an open-access article distributed under the terms of the Creative Commons Attribution License (CC BY). The use, distribution or reproduction in other forums is permitted, provided the original author(s) and the copyright owner(s) are credited and that the original publication in this journal is cited, in accordance with accepted academic practice. No use, distribution or reproduction is permitted which does not comply with these terms.
*Correspondence: Ali Malakshahi Kurdestani, YW1rXzE5NzkyMDAwQHlhaG9vLmNvbQ==; Davide Francioli, ZGF2aWRlLmZyYW5jaW9saUBocy1nbS5kZQ==
Disclaimer: All claims expressed in this article are solely those of the authors and do not necessarily represent those of their affiliated organizations, or those of the publisher, the editors and the reviewers. Any product that may be evaluated in this article or claim that may be made by its manufacturer is not guaranteed or endorsed by the publisher.
Research integrity at Frontiers
Learn more about the work of our research integrity team to safeguard the quality of each article we publish.