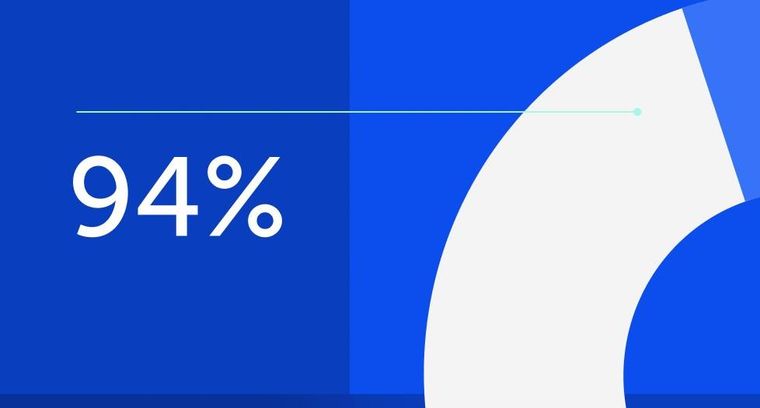
94% of researchers rate our articles as excellent or good
Learn more about the work of our research integrity team to safeguard the quality of each article we publish.
Find out more
ORIGINAL RESEARCH article
Front. Plant Sci., 20 August 2024
Sec. Plant Symbiotic Interactions
Volume 15 - 2024 | https://doi.org/10.3389/fpls.2024.1446437
This article is part of the Research TopicPlant-microbes Interactions and Resistance Against Abiotic StressView all 14 articles
Background: Drought constitutes a major abiotic stress factor adversely affecting plant growth and productivity. Plant-microbe symbiotic associations have evolved regulatory mechanisms to adapt to environmental stress conditions. However, the interactive effects of different fungi on host growth and stress tolerance under drought conditions remain unclear.
Objective: This study explored the effects of varying polyethylene glycol (PEG-6000) concentrations (0%, 15%, 25%, and 35%) on the growth and physiological responses of two ectomycorrhizal fungi (Suillus granulatus (Sg) and Pisolithus tinctorius (Pt)) and two dark septate endophytes (Pleotrichocladium opacum (Po) and Pseudopyrenochaeta sp. (Ps)) isolated from the root system of Pinus tabuliformis. Specifically, the study aimed to evaluate six inoculation treatments, including no inoculation (CK), single inoculations with Sg, Pt, Po, Ps, and a mixed inoculation (Sg: Pt : Po: Ps = 1:1:1:1), on the growth and physiological characteristics of P. tabuliformis seedlings under different water regimes: well-watered at 70% ± 5%, light drought at 50% ± 5%, and severe drought at 30% ± 5% of the maximum field water holding capacity.
Results: All four fungi exhibited the capacity to cope with drought stress by enhancing antioxidant activities and regulating osmotic balance. Upon successful root colonization, they increased plant height, shoot biomass, root biomass, total biomass, and mycorrhizal growth response in P. tabuliformis seedlings. Under drought stress conditions, fungal inoculation improved seedling drought resistance by increasing superoxide dismutase and catalase activities, free proline and soluble protein contents, and promoting nitrogen and phosphorus uptake. Notably, mixed inoculation treatments significantly enhanced antioxidant capacity, osmotic adjustment, and nutrient acquisition abilities, leading to superior growth promotion effects under drought stress compared to single inoculation treatments.
Conclusion: All four fungi tolerated PEG-induced drought stress, with increased antioxidant enzyme activities and osmotic adjustment substances and they promoted the growth and enhanced drought resistance of P. tabuliformis seedlings.
Currently, the trend of global climate change is characterized by rising temperatures and increasing aridity, with precipitation patterns undergoing noticeable changes. The frequency, duration, intensity, and area of droughts have increased significantly. Drought has emerged as a principal factor impacting vegetation growth and recovery (Cook et al., 2014; Huang et al., 2016; Kumar et al., 2024). In arid regions, high summer temperatures adversely affect the survival of tree seedlings and hinder forest regeneration. Simultaneously, soil temperature indirectly influences nutrient synthesis, translocation, and absorption, thereby disrupting the normal physiological processes of young seedlings and eventually impeding vegetation growth and regeneration within the entire forest ecosystem (Chidumayo, 2008). Although drought diminishes soil productivity, constrains water uptake, induces plant osmotic and redox imbalances, and ultimately leads to plant mortality (Alori et al., 2020; Farrell et al., 2017), microbial interventions can effectively alleviate drought stress (Saritha et al., 2021; Li and Liu, 2016; Salehi-Lisar and Bakhshayeshan-Agdam, 2016). Studies have demonstrated that when plants establish mycorrhizal associations in their root systems, their capacity to absorb water and nutrients significantly improves, thereby enhancing their drought resistance (Gleason et al., 2006). Consequently, the inoculation of beneficial fungi represents an effective strategy for maintaining plant health and quality, necessitating the selection of fungal species capable of adapting to drought conditions and the screening of strains and strain combinations exhibiting robust drought tolerance.
Ectomycorrhizal fungi (ECMF) establish ectomycorrhizal associations when their mycelia infect the nutrient roots of host plants. The primary host plants of ECMF belong to the Betulaceae, Pinaceae, Salicaceae, and Fagaceae families (Tedersoo and Brundrett, 2017). Existing studies indicate that Pinaceae are among the earliest ectomycorrhizal plant lineages (Tedersoo et al., 2012). Dark septate endophytes (DSE) are a type of minute endophytes that colonize the epidermal cells, root epidermis, cortex, and even the vascular tissue cells or intercellular spaces of plant roots, forming dark-colored, conspicuous structures with laterally septate hyphae and microsclerotia (Addy et al., 2005; Mandyam et al., 2010; Gaber et al., 2023). These fungi exhibit a high colonization rate in plant roots under drought or extreme environmental conditions (Gucwa-Przepióra et al., 2016), with a broad host range encompassing nearly 600 plant species from 320 genera across 114 families (Jumpponen et al., 1998), garnering increasing research attention in recent years (Santos et al., 2021; Huertas et al., 2024). Both ECMF and DSE promote plant growth and improve stress tolerance, playing crucial roles in ecosystem stability and restoration (Yin et al., 2018; Li et al., 2022a, b). Recent studies by Zhang et al. (2024) revealed that inoculating ECMF strains enhanced the drought resistance of Pinus massoniana seedlings during the early stages of drought stress by influencing water content, photosynthesis, osmolyte accumulation, and antioxidant enzyme activities in the shoots and roots. Similarly, Wang et al. (2021) reported that ECMF inoculation increased water content, photosynthetic rate, and osmolyte accumulation in P. tabuliformis under drought stress. Li et al. (2021) demonstrated that DSE inoculation could increase the biomass of Isatis indigotica and mitigate oxidative damage caused by drought stress. However, the interactive effects of these two fungi on host growth and stress tolerance under drought conditions remain unclear, with limited research exploring the co-existence of both fungi and their impact on host plant drought tolerance.
In recent years, the study of co-inoculation with ectomycorrhizal fungi has emerged as a prominent topic in rhizosphere microecology research (Corrales et al., 2022; Tedersoo et al., 2024). The combined application of ectomycorrhizal fungi and Trichoderma has been shown to effectively enhance the antioxidant capacity of Scots pine seedlings (Yin et al., 2016). Deng et al. (2017) reported that dual inoculation with Suillus luteus and dark septate endophytes improved the resistance of Pinus sylvestris var. mongolica seedlings to damping-off disease, reduced seedling blight incidence, and increased seedling survival rates. Our previous studies have demonstrated that inoculating two ECMF and two DSE strains could improve the growth, root development, nutrient uptake, and soil microbial community composition of P. tabuliformis seedlings (Xu et al., 2022), and effectively regulate the antioxidant defense response and photosynthesis of these seedlings under cadmium stress. The co-inoculation of the two fungi exhibited a more pronounced synergistic effect on plant tolerance to heavy metals (Zhou et al., 2024). Although the direct effects of beneficial microbial inoculants on plant growth have been widely reported (Santos et al., 2017; He et al., 2019), information regarding the contribution of the ECMF and DSE combination to plant growth under drought stress remains limited.
Pinus tabuliformis, the predominant tree species in North China, holds immense importance for soil and water conservation, landscape aesthetics, and ecological balance within its distribution range (Gao et al., 2023). P. tabuliformis exhibits sensitivity to climate change-induced warming and drying. North China represents the most suitable growth area and concentrated distribution region for P. tabuliformis. The recruitment and renewal of P. tabuliformis seedlings profoundly influence the structure and species composition of forest communities, bearing great significance for forest resource reserves in this region. The ecological stability of China’s northern regions is facing a more serious threat in the face of increasingly severe drought. As the main afforestation species of plantation forests in the northern region, the plantation forests of P. tabuliformis, under the influence of prolonged and frequent droughts, have suffered from degradation of forest stands, poor natural regeneration, drying up of tree tops and sparse understory vegetation, which have led to a vicious cycle of ecological environment in the region and further accelerated the depletion of forest resources (Chen et al., 2015). Previous studies have revealed the widespread presence of both ECMF and DSE in the roots of P. tabuliformis (Chu et al., 2016, 2019, 2021; Xu et al., 2022).
Therefore, in this study, drought tolerance investigations were conducted on isolated, purified, and identified root symbiotic fungi of P. tabuliformis to screen for strains exhibiting robust drought resistance. Subsequently, the root symbiotic fungi were reinoculated into P. tabuliformis seedlings, and water control experiments were carried out in a greenhouse. This research aims to provide a reference for further investigation of the drought resistance mechanisms of root symbiotic fungi and the screening of drought-resistant fungi. It also offers theoretical and technical support for P. tabuliformis afforestation in arid areas, promoting understory regeneration, preventing soil erosion, and improving forest resource quality. Previous studies on the resistance of mycorrhizal fungi in plants have predominantly focused on single inoculation methods. In contrast, this study introduces a mixed inoculation approach to evaluate the effects of different inoculation methods on plant-microbe symbionts. This experiment primarily examines the physiological and biochemical responses of these symbionts under drought stress conditions. While current research has provided valuable insights, the molecular mechanisms by which fungi enhance drought tolerance in P. tabuliformis remain underexplored. Future research should leverage advanced molecular biology techniques, such as transcriptomics, proteomics, and metabolomics, to elucidate these mechanisms.
The four-plant root symbiotic fungi used in this experiment were two ECMF, Suillus granulatus (Sg) and Pisolithus tinctorius (Pt), and two DSE, Pleotrichocladium opacum (Po) and Pseudopyrenochaeta sp. (Ps), which were isolated from the root of P. tabuliformis (Xu et al., 2022). These fungi were identified by morphological characteristics and phylogenetic analysis of nuclear ribosomal DNA (nrDNA) internal transcribed spacer (ITS) sequences (Supplementary Figures S1, S2). The fungi were cultured on potato dextrose agar media and stored in a 27°C mould incubator.
Uniformly sized and plump fresh seeds of P. tabuliformis were used in the experiments. The seeds were surface-sterilized and placed in Petri dishes in a 25°C light incubator to accelerate germination. Germinated seeds were sown in transparent plastic pots (15 cm height, 8 cm bottom diameter, 12 cm top diameter) filled with sterilized river sand (2 mm sieve, 121°C, 0.1 MPa, 2 h autoclaving). Each pot contained 1,000 g of the sterile sand medium, with four seeds sown per pot. During the seedling cultivation period, sterile water and Hoagland nutrient solution were supplied to ensure normal seedling growth.
The drought stress tolerance of four root symbiotic fungi (Sg, Pt, Po and Ps) isolated from P. tabuliformis was evaluated through solid and liquid culture experiments using polyethylene glycol (PEG-6000) to simulate drought stress. Media containing 0%, 15%, 25%, and 35% PEG-6000 were prepared using potato, glucose, plant gel, and PEG-6000. The experiment comprised 16 treatments with five replicates per treatment. Well-growing fungal colonies on potato dextrose agar were selected, and 6 mm fungal disks were inoculated into 20 mL solid media and 100 mL liquid media, respectively. Solid cultures were incubated at 27°C for 14 days, during which fungal colony morphology was observed and recorded. Liquid cultures were incubated in a thermostatic incubator at 27°C, with continuous shaking at 150 rpm, for 14 days in the dark. After this period, the mycelia were harvested to determine biomass and physiological indices.
A greenhouse pot experiment was conducted with different fungal inoculation treatments and moisture levels as variables. The experiment was performed under natural light conditions at Hebei Agricultural University, with temperatures maintained at 30°C during the day and 21°C at night, a 14-h light/10-h dark photoperiod, and relative humidity of 60% during the day and 70% at night. Six inoculation treatments were employed: no inoculation (CK), single inoculations with Sg, Pt, Po, or Ps, and a mixed inoculation (Sg: Pt : Po: Ps = 1:1:1:1). Liquid inocula was prepared by inoculating three fungal disks of each fungus into 150 mL potato dextrose broth and incubating on a shaking table at 27°C for 14 days. The mycelia were then homogenized and mixed with sterile water (v:v = 3:1) to obtain a mycelial suspension. Seedlings were inoculated by perforated root irrigation with 20 mL of the respective liquid inoculum, while the control group received 20 mL of potato dextrose broth.
One month post-inoculation, root samples were examined to confirm fungal colonization. Drought stress treatments were then imposed, with three soil moisture levels: 70% ± 5% (well-watered, WW), 50% ± 5% (light drought, LD), and 30% ± 5% (severe drought, SD) of the maximum field moisture capacity. Unified watering management was implemented, maintaining soil moisture content within the experimental range by weighing the pots at 17:00 every day. Plastic pots were repositioned every two weeks to minimize positional effects. The experiment consisted of 18 treatments with five replications each. After 40 days of drought stress, seedlings were harvested for relevant index measurements.
The superoxide dismutase (SOD) activity in fungi and plants was determined by the nitroblue tetrazolium photoreduction method (Elavarthi and Martin, 2010), and the catalase (CAT) activity was assessed by the ultraviolet absorption method (Zhang, 1990).
The malondialdehyde (MDA) content was quantified using the thiobarbituric acid method (Zhang, 1990), and proline content was determined following the method of Bates et al. (1973). Soluble protein content was measured by the Coomassie brilliant blue colorimetric method (Campion et al., 2017).
The ectomycorrhizal root colonization rates were calculated by microscopic examination of mycorrhizal and non-mycorrhizal root tips, and the percentages of root tips with evident ectomycorrhizal structures were determined (Brundrett et al., 1996). Dark septate endophyte colonization rates were assessed by trypan blue staining and microscopic quantification of septate hyphae and microsclerotia, with the percentage of colonized root segments calculated (Phillips and Hayman, 1970).
The mycorrhizal growth response (MGR) was calculated according to the method of van der Heijden (2002). If the total dry weight of inoculated plants (M) exceeded the mean total dry weight of non-inoculated plants (NMmean), then MGR (%) = 100 × (1 − NMmean/M). If M < NMmean, then MGR (%) = 100 × (−1 + M/NMmean). A positive MGR value indicated that the inoculation treatment promoted plant growth, while a negative value signified growth inhibition.
The dried above-ground and below-ground portions of P. tabuliformis seedlings were ground into a fine powder and digested using the H2SO4-H2O2 method until the digestion solution became colorless and transparent (Bao, 2000). The digested solution was filtered and used to determine nitrogen and phosphorus contents in the aboveground and belowground parts by the Kjeldahl method and molybdenum antimony colorimetric method, respectively.
Statistical analysis were performed with SPSS software (Version 24; SPSS Inc., Chicago, Ill., USA). For the in vitro experiment, a two-way analysis of variance (ANOVA) was conducted to analyze the effects of PEG-6000 treatment and fungal species on the growth and physiological responses of the four fungi. For the pot experiment, two-way ANOVA examined the effects of drought treatment and fungal inoculation on the growth and physiological responses of P. tabuliformis seedlings. Mean values were compared using Duncan’s multiple range test at a significance level of p < 0.05. Correlation analyses were conducted to assess the influence of different fungal inoculants on plant parameters. Principal component analysis (PCA) was employed to analyze characteristics including fungal colonization rates, plant biomass, enzyme activities, and nutrient element contents. All graphs were generated using GraphPad Prism software (version 10.1.0).
The two-way ANOVA results (Supplementary Table S1) revealed significant effects of PEG stress, fungal species, and their interaction on the biomass of the four root symbiotic fungi. The biomass of the Sg and Ps strains initially increased and then decreased with increasing PEG-6000 concentration, reaching a maximum under the 15% PEG-6000 treatment in Ps strain, and 15% and 25% PEG-6000 treatments in Sg strain (Figure 1A). In contrast, the biomass of the Pt strain first decreased and then increased, with a 27.0% increase under the 35% PEG-6000 treatment compared to the 0% treatment. The biomass of the Po strain showed a gradual increase with rising PEG-6000 concentrations and reached the highest under 35% PEG-6000 treatment. Overall, the biomass accumulation of the Sg strain was higher than that of the Pt strain, and the Ps strain had greater biomass accumulation than the Po strain across all PEG-6000 concentrations.
Figure 1. Effects of different concentrations of PEG-6000 (0%, 15%, 25%, and 35%) on the biomass (A), superoxide dismutase activity (B), catalase activity (C), proline (D) and soluble protein (E) of Suillus granulatus (Sg), Pisolithus tinctorius (Pt), Pleotrichocladium opacum (Po), and Pseudopyrenochaeta sp. (Ps). Data (means ± SD, n = 3) are significantly different (p < 0.05) if followed by different letters above the bars.
With increasing PEG-6000 concentrations, both SOD and CAT activities in the Sg and Po strains increased, reaching their maximum under the 25% PEG-6000 treatment (Figures 1B, C). Compared to the control treatment, the SOD activity in the Pt strain significantly increased under the 15% and 25% PEG-6000 treatments, reaching its peak at 25% PEG-6000. The CAT activity in the Pt strain showed no significant difference from the control at 15% PEG-6000 but significantly increased under the 25% and 35% PEG-6000 treatments, with the highest activity observed at 35% PEG-6000. In contrast, the SOD and CAT activities in the Ps strain remained significantly higher under all PEG-6000 treatments compared to the 0% PEG-6000 treatment. Overall, the two dark septate endophytes (Po and Ps) exhibited greater improvements in antioxidant enzyme activities in response to PEG-6000 stress than the two ectomycorrhizal fungi (Sg and Pt).
All four fungi influenced the accumulation of proline and soluble proteins in response to drought stress induced by PEG-6000 (Figures 1D, E). For the Sg, Pt, and Po strains, proline content peaked at the 15% PEG-6000 treatment, significantly higher than the control, and subsequently decreased with increasing PEG-6000 concentrations. In contrast, PEG-6000 stress consistently and significantly increased proline levels in the Ps strain, with no significant difference between the 15% and 25% treatments. However, at 35% PEG-6000, the Ps strain exhibited significantly higher proline content compared to the other treatments (Figure 1D).
PEG-6000 stress consistently and significantly increased soluble protein levels in the Sg, Po, and Ps strains, while it resulted in a significant reduction in the Pt strain. The Sg strain reached its maximum soluble protein content at 15% PEG-6000, followed by a decreasing trend with increasing PEG-6000 concentrations. Conversely, soluble protein content in the Po and Ps strains increased significantly with rising PEG-6000 levels, peaking at 35% PEG-6000. In contrast, the Pt strain exhibited the lowest soluble protein content at 35% PEG-6000 (Figure 1E).
In the non-inoculated treatments, no fungal structure was observed in the roots of P. tabuliformis (Figure 2A). The fungal colonization rates showed that, except for the Po inoculation under SD treatment, all other inoculation treatments under various drought conditions had colonization rates exceeding 50% (Figure 2A). The colonization rates of Sg increased with drought severity, whereas the rates for other treatments first increased and then decreased. The colonization rates for Pt, Po, and mix treatments peaked under LD conditions. The mix treatment had higher colonization rates than other treatments under WW and LD conditions.
Figure 2. Effects of different inoculation treatments on the growth performance of Pinus tabuliformis seedlings under drought stress. Data (means ± SD, n = 3) are significantly different (p < 0.05) if followed by different letters above the bars. (A), fungal colonization rate; (B), mycorrhizal growth response; (C), plant height; (D), shoot biomass and (E), total biomass. Sg, Suillus granulatus; Pt, Pisolithus tinctorius; Po, Pleotrichocladium opacum; Ps, Pseudopyrenochaeta sp.; Mix, mixed inoculation of four root symbiotic fungi. WW, well-watered; LD, light drought; SD, severe drought.
There was a significant interaction between fungal inoculation and drought stress on the mycorrhizal growth response of the seedlings (Supplementary Table S2). With increasing drought stress, the mycorrhizal growth response values for the inoculation treatments initially increased and then decreased (Figure 2B). All inoculation treatments positively affected the growth of P. tabuliformis seedlings under drought conditions. The MGR values of the mixed inoculation treatment were higher than those of individual inoculations, indicating the greatest growth promotion effects under drought stress.
The plant height of non-inoculated, Sg-, Po-, and Ps-inoculated seedlings decreased with increasing drought stress (Figure 2C). The plant height of Pt- and mix-inoculated seedlings first increased and then decreased, peaking under LD conditions. Shoot and total biomass of non-inoculated seedlings decreased with increasing drought stress, while inoculated seedlings showed an initial increase followed by a decrease, reaching a maximum under LD conditions (Figures 2D, E). Under all drought conditions, mixed inoculation treatments resulted in significantly higher shoot and total biomass compared to single inoculations.
With increasing drought stress, the SOD activity of non-inoculated seedlings gradually increased, while that of inoculated seedlings first increased and then decreased (Figure 3A). The SOD activity of all inoculated seedlings peaked under LD conditions. CAT activity in seedlings from all treatments increased first and then decreased with increasing drought stress severity (Figure 3B). Under LD and SD treatments, inoculated seedlings had higher SOD and CAT activities than non-inoculated ones, indicating that inoculation treatments enhanced antioxidant enzyme activities to cope with drought stress.
Figure 3. Effects of different inoculation treatments on the physiological characteristics of Pinus tabuliformis seedlings under drought stress. Data (means ± SD, n = 3) are significantly different (p < 0.05) if followed by different letters above the bars. (A), superoxide dismutase activity; (B), catalase activity; (C), malondialdehyde content; (D), proline and (E), soluble protein. Sg, Suillus granulatus; Pt, Pisolithus tinctorius; Po, Pleotrichocladium opacum; Ps, Pseudopyrenochaeta sp.; Mix, mixed inoculation of four root symbiotic fungi. WW, well-watered; LD, light drought; SD, severe drought.
Under drought stress, the MDA content of seedlings increased with drought severity, while inoculation treatments reduced MDA accumulation (Figure 3C). There was no significant difference in MDA content between inoculated and control seedlings under WW conditions. Under LD conditions, inoculation treatments significantly reduced MDA content by 22.8%, 35.4%, 12.0%, 32.0%, and 35.9%, respectively. Under SD conditions, the reductions were 30.9%, 40.5%, 27.0%, 33.3%, and 41.2%, respectively. mixed inoculation treatments significantly reduced MDA content under LD and SD conditions.
With increasing drought severity, the proline content of non-inoculated, Sg-, Po-, and mix-inoculated seedlings gradually increased, while the proline content of Pt- and Ps-inoculated seedlings first increased and then decreased (Figure 3D). Under SD conditions, the proline content of all inoculation treatments significantly increased compared to the control, except for the Ps inoculation. The mix-inoculated treatment had the highest proline content.
Under WW, LD, and SD conditions, all fungal inoculation treatments significantly increased the soluble protein content in the seedlings (Figure 3E). Specifically, under WW conditions, the soluble protein content in the mix and Pt treatments was significantly higher than in the Sg, Po, and Ps treatments. Under LD and SD conditions, the soluble protein content of the mixed-inoculated seedings was significantly higher than that of the other four single inoculation treatments.
With increasing drought severity, the N content in the shoots and roots of non-inoculated and Sg-inoculated seedlings gradually decreased, while N content in the shoots and roots of Ps- and mix-inoculated seedlings first increased and then decreased (Figures 4A, B). The P content in the shoots of non-inoculated seedlings gradually decreased, while the P content in the roots first increased and then decreased (Figures 4C, D). The P content in the shoots and roots of Sg-, Ps-, and mix-inoculated seedlings gradually decreased, whereas in Po-inoculated seedlings, it first increased and then decreased with increasing drought severity (Figures 4C, D).
Figure 4. Effects of different inoculation treatments on nitrogen and phosphorus content in the shoots and roots of Pinus tabuliformis seedlings under drought stress Data (means ± SD, n = 3) are significantly different (p < 0.05) if followed by different letters above the bars. (A), shoot nitrogen content; (B), root nitrogen content; (C), shoot nitrogen content and (D), root phosphorus content. Sg, Suillus granulatus; Pt, Pisolithus tinctorius; Po, Pleotrichocladium opacum; Ps, Pseudopyrenochaeta sp.; Mix, mixed inoculation of four root symbiotic fungi. WW, well-watered; LD, light drought; SD, severe drought.
Correlation analysis of different inoculation treatments on the growth and physiological indexes of P. tabuliformis seedlings under drought stress revealed significant positive correlations between fungal colonization rates and various growth and nutrient parameters (Figure 5). The colonization rates of Pt-inoculated seedlings were significantly positively correlated with plant height, total biomass, root N content, shoot P content, and root P content (p < 0.05) (Figure 5B). Po-inoculated seedlings showed significant positive correlations with root biomass, SOD, CAT, and shoot P content (p < 0.05) (Figure 5C). Mix-inoculated seedlings exhibited significant positive correlations with total biomass and shoot N content (p < 0.05) (Figure 5E). Higher fungal colonization rates of Pt-, Po-, and mix-inoculated seedlings promoted seedling growth and nutrient absorption.
Figure 5. Correlations among indicators in the pot experiment. (A) Sg, (B) Pt, (C) Po, (D) Ps, (E) Mix; *: (p < 0.05); **: p < 0.01; ***: p < 0.001; FCR, Fungal colonization rate; MGR, Mycorrhizal growth response; PL, Plant height; SB, Shoot biomass; RB, Root biomass; TB, Total biomass; SOD, Superoxide dismutase activity; CAT, Catalase activity; MDA, Malondialdehyde content; Pro, Proline; SP, Soluble protein; SNC, Shoot N content; RNC, Root N content; SPC, Shoot P content; RPC, Root P content.
The root biomass of Po-inoculated seedlings was significantly positively correlated with SOD, CAT, and shoot P content, while total biomass was significantly positively correlated with SOD and CAT (p < 0.05) (Figure 5C). Ps-inoculated seedlings’ root biomass was significantly positively correlated with soluble protein and shoot N content (p < 0.05) (Figure 5D). The plant height of mix-inoculated seedlings was significantly positively correlated with SOD, CAT, shoot N content, and root N content (p < 0.05) (Figure 5E). These results indicate that the strong antioxidant defense and nutrient uptake abilities of Po- and mix-inoculated seedlings significantly promote growth and improve drought resistance.
PCA was used to evaluate the similarity among different inoculation treatments and the relationships between plant height, biomass, enzyme activity, antioxidant capacity, and nutrient composition (Figure 6). The first principal component (PC1) and the second principal component (PC2) explained 46.4% and 19.9% of the variance, respectively. There were significant differences between control and inoculated treatments under drought stress (Figure 6A). The mix inoculation treatment was significantly separated from other treatments. Antioxidant enzyme activity, osmotic adjustment substances, and nutrient content were the main factors affecting plant growth and were positively correlated with biomass (Figure 6B). The results indicated that inoculation treatments promoted plant growth and improved antioxidant capacity, enhancing the tolerance of P. tabuliformis seedlings to drought stress.
Figure 6. Principal component analysis (PCA) of indicators in the pot experiment. (A) Sg indicates Suillus granulatus; Pt indicates Pisolithus tinctorius; Po indicates Pleotrichocladium opacum; Ps indicates Pseudopyrenochaeta sp.; Mix indicates mixed inoculation of four root symbiotic fungi.; (B) PL, Plant height; SB, Shoot biomass; RB, Root biomass; TB, Total biomass; SOD, Superoxide dismutase activity; CAT, Catalase activity; MDA, Malondialdehyde content; Pro, Proline; SP, Soluble protein; SNC, Shoot N content; RNC, Root N content; SPC, Shoot P content; RPC, Root P content.
The biomass of fungi is a crucial indicator of their resistance under stressful environments. All four fungi exhibited normal growth under PEG stress, indicating their tolerance to PEG-induced drought stress. Abiotic stresses, such as drought and salt, have been shown to induce an increase in plant peroxisome content (Fahy et al., 2017; Castillo et al., 2008; Sanad et al., 2019). Li et al. (2022b) demonstrated that strains with greater drought resistance exhibit significant increases in antioxidant enzyme activities (SOD, POD, and CAT) under drought stress, indicating that increased antioxidant enzyme activity is a response to drought in sensitive strains. In this study, the biomass of the Sg, Po, and Ps strains, except for the Pt strain, was greater under other PEG-6000 treatments compared to the 0% PEG-6000 treatment. This may be related to the higher contents of antioxidant enzymes and osmotic adjustment substances in these strains under PEG-6000 treatments. SOD and CAT are critical protective enzymes in the antioxidant system, responsible for scavenging reactive oxygen species (ROS) and H2O2, working together to maintain the balance between ROS production and scavenging (Raja et al., 2017). This study found that the activities of antioxidant enzymes and the contents of osmotic adjustment substances increased in all four fungi under PEG stress, with SOD and CAT activities showing consistent changes. This indicates that all four fungi could alleviate oxidative damage by synthesizing antioxidant enzymes to scavenge ROS produced by PEG-6000 stress. Simultaneously, they improved their accumulation of proline and soluble proteins, promoting cellular osmoregulation to protect mycelial cell membrane structures, thereby enhancing stress tolerance. This study also revealed that DSE strains had greater biomass than ECMF strains, suggesting that DSE strains were less affected by PEG-6000 stress, possibly due to their highly melanized hyphae. Melanin in fungi serves to protect them from harmful environmental conditions (Fernandez and Koide, 2013).
Microorganisms are natural partners in plant defense mechanisms under adverse conditions (Meena et al., 2017). In this study, typical mycorrhizal structures and DSE hyphae and microsclerotia were observed in root samples inoculated with ECMF and DSE strains, indicating effective colonization even under drought conditions. Additionally, the mycorrhizal growth response values of inoculation treatments were all positive under drought stress, with mixed inoculation treatments showing greater effects than individual treatments. Among the four individual inoculation treatments, the Ps-inoculated seedlings under SD conditions exhibited higher fungal colonization rates, mycorrhizal growth responses, and biomass compared to those inoculated with the other three fungi. This increased performance is likely due to the reduction in MDA content and the increase in soluble protein content in the Ps-inoculated seedlings, which enhances cell membrane stability and improves dehydration resistance, allowing the plants to better adapt to stressful environments. These findings align with previous pure culture experiments where the Ps strain demonstrated higher biomass and stronger drought tolerance under the highest PEG concentration stress. Thus, different fungi exhibit varying levels of drought tolerance and influence the drought resistance of plants differently. Studies have shown that inoculation with Amanita vaginata significantly promotes the growth of P. tabuliformis (Zhang et al., 2011), and the biomass of Hedysarum scoparium inoculated with DSE under drought stress was significantly greater than that of non-inoculated treatments (Li et al., 2019). However, few studies have investigated the co-inoculation of ECMF and DSE strains under drought stress. This experiment demonstrated that inoculating ECMF and DSE strains under drought stress promoted increases in plant height, shoot biomass, root biomass, and total biomass of P. tabuliformis seedlings. These growth indices were significantly higher in mixed inoculation treatments compared to non-inoculated and individual inoculation treatments. Increased plant biomass indicates more organic matter to support plants, enhancing their survival in arid environments. Our study revealed that inoculating root symbiotic fungi under drought stress effectively mitigated adverse environmental effects on P. tabuliformis seedlings, promoting growth and improving drought resistance. Furthermore, mixed inoculation treatments had synergistic rather than competitive effects on the growth of P. tabuliformis seedlings under drought conditions.
Drought stress leads to the overproduction of ROS in plants, causing degradation of lipids, proteins, and nucleic acids, damaging plant cells, and reducing growth and development (Gill and Tuteja, 2010; Kapoor et al., 2019). Plants produce enzymes such as SOD and CAT to scavenge ROS, protecting cells from oxidative damage and preserving membrane integrity (Wu et al., 2006; Raja et al., 2017). In this study, antioxidant enzyme activities in P. tabuliformis seedlings inoculated with root symbiotic fungi increased under drought stress, indicating that these fungi protect host plants from oxidative damage by enhancing antioxidant enzyme activities. Under LD conditions, mixed inoculation treatments significantly increased SOD and CAT activities in seedlings compared to individual treatments, indicating a greater ability to eliminate ROS. MDA content, an indicator of oxidative damage, reflects the degree of stress injury to plants. Excessive MDA accumulation often results from oxidative damage to membrane lipids, with lower MDA levels indicating higher membrane stability (Quiroga et al., 2020). In this study, MDA content in P. tabuliformis seedlings decreased after inoculation with root symbiotic fungi, indicating improved cell membrane stability. Under LD and SD conditions, mixed inoculation treatments resulted in significantly lower MDA content compared to control and individual inoculation treatments, consistent with findings in P. sylvestris var. mongolica seedlings (Zhao et al., 2020). However, under WW conditions, there was no significant difference in MDA content between mixed inoculation and control treatments, indicating no significant synergistic effect of co-inoculation under well-watered conditions.
Osmotic adjustment is a crucial mechanism for plants to regulate water potential under drought stress. Proline and soluble protein are key osmotic adjustment substances that allow plants to adapt to stressful environments by increasing dehydration tolerance through accumulation (Pal et al., 2018; Sharma and Verslues, 2010; Azmat and Moin, 2019). In this study, proline content in inoculated seedlings under LD and SD conditions was significantly higher than in control treatments, with mixed inoculation treatments showing the highest proline content under SD conditions. Rezaei-Chiyaneh et al. (2021) also found that co-inoculated plants under severe water stress had the highest proline content. This study indicates that co-inoculation of ECMF and DSE strains promotes proline biosynthesis, enhancing osmotic regulation under severe water stress. Higher contents of soluble protein in inoculated plants suggest greater osmotic ability, helping seedlings maintain turgor pressure under drought conditions (Liu et al., 2021). Therefore, root symbiotic fungi improve osmotic balance by increasing the content of osmotic adjustment substances, enhancing plant tolerance and alleviating the negative effects of drought.
Mycorrhizal symbionts significantly affect the uptake of nitrogen and phosphorus by plants (Veresoglou et al., 2012). Research has shown that fungal inoculation improves nutrient recovery efficiency, accumulation, and growth in host plants (Surono and Narisawa, 2017; Vergara et al., 2017, 2018; Lu et al., 2016). In this experiment, N contents in the shoots and roots of inoculated plants under LD and SD conditions were significantly higher than in control treatments, with mixed inoculation treatments showing the highest N contents under LD conditions. Mycorrhizal fungi increase nitrogen uptake under drought stress by enhancing root hydraulic conductivity (Graham and Syversen, 1984). Mycorrhizal fungi also promote nitrogen uptake by decomposing organic matter (Hodge et al., 2001; Goussous and Mohammad, 2009), helping maintain water status under water scarcity. Mixed inoculation treatments significantly increased P content in shoots and roots under WW conditions and were more effective than individual treatments under LD and SD conditions. Fungi can explore more soil volume than non-mycorrhizal plants, enhancing P uptake (Evelin et al., 2009). Adding mycorrhizal fungi to soil increases microbial biomass and CO2 release, forming weak acids that dissolve phosphorus-bearing minerals, and increasing phosphorus availability (Subramanian et al., 2006). Thus, plants inoculated with mycorrhizal fungi exhibit higher productivity even under adverse conditions. In summary, root symbiotic fungi improve the uptake of nutrients such as N and P, promoting growth and enhancing plant resistance. The synergistic effects of ECMF and DSE on nutrient absorption are greater than individual inoculations.
Drought stress affects a range of physiological changes in plants, and root symbiotic fungi can help plants withstand the adverse effects of drought. This study demonstrated that root symbiotic fungi (Sg, Po, Ps, and Pt) enhance the growth and drought resistance of P. tabuliformis seedlings. All fungi tolerated PEG-induced drought stress, with increased antioxidant enzyme activities and osmotic adjustment substances. DSE strains showed greater resilience due to their melanized hyphae. Acids produced by root symbiotic fungi under drought stress may help plants to activate elements in the soil that plants fail to take up. Therefore, the determination of the composition and content of metabolites of root symbiotic fungi under drought stress could be carried out in future studies. Inoculation, especially mixed inoculation, significantly improved plant height, biomass, and drought resistance. The fungi increased antioxidant enzymes and osmotic substances, mitigating oxidative damage and enhancing osmotic regulation. Additionally, they improved nutrient uptake, particularly nitrogen and phosphorus. These findings suggest that root symbiotic fungi, particularly in combination, are effective in enhancing drought resistance and growth of P. tabuliformis, providing valuable insights for afforestation and forest management in arid regions.
The original contributions presented in the study are included in the article/Supplementary Material. Further inquiries can be directed to the corresponding author.
LX: Writing – review & editing, Writing – original draft, Methodology, Data curation. JH: Writing – review & editing, Writing – original draft, Visualization. YM: Writing – review & editing. YYZ: Writing – review & editing, Validation, Data curation. BL: Writing – review & editing, Data curation. JZ: Writing – review & editing. YZ: Writing – review & editing, Writing – original draft, Supervision, Funding acquisition, Data curation.
The author(s) declare financial support was received for the research, authorship, and/or publication of this article. This work was supported by the National Natural Science Foundation of China (32001112), Science Foundation of Hebei Province (C2023204238).
The present work was supported by Hebei Agricultural University, China.
The authors declare that the research was conducted in the absence of any commercial or financial relationships that could be construed as a potential conflict of interest.
All claims expressed in this article are solely those of the authors and do not necessarily represent those of their affiliated organizations, or those of the publisher, the editors and the reviewers. Any product that may be evaluated in this article, or claim that may be made by its manufacturer, is not guaranteed or endorsed by the publisher.
The Supplementary Material for this article can be found online at: https://www.frontiersin.org/articles/10.3389/fpls.2024.1446437/full#supplementary-material
Addy, H. D., Piercey, M. M., Currah, R. S. (2005). Microfungal endophytes in root. Can. J. Bot. 83, 1–13. doi: 10.1139/b04-171
Alori, E. T., Emmanuel, O. C., Glick, B. R., Babalola, O. O. (2020). Plant-archaea relationships: a potential means to improve crop production in arid and semi-arid regions. World J. Microb. Biot. 36, 133. doi: 10.1007/s11274-020-02910-6
Azmat, R., Moin, S. (2019). The remediation of drought stress under VAM inoculation through proline chemical transformation action. J. Photochem. Photobiol. 193, 155–161. doi: 10.1016/j.jphotobiol.2019.03.002
Bates, L. S., Waldren, R. P., Teare, I. D. (1973). Rapid determination of proline for water stress studies. Plant Soil 39, 205–207. doi: 10.1007/BF00018060
Brundrett, M., Bougher, N., Dell, B., Grove, T., Malajczuk, N. (1996). Working with Mycorrhizas in Forestry and Agriculture. (Canberra: Australian Centre for International Agricultural Research). doi: 10.13140/2.1.4880.5444
Campion, E. M., Loughran, S. T., Walls, D. (2017). Protein quantitation andanalysis of purity. Methods Mol. Biol. 1485, 225–255. doi: 10.1007/978-1-4939-6412-3_12
Castillo, M. C., Sandalio, L. M., Del Rio, L. A., Leon, J. (2008). Peroxisome proliferation, wound-activated responses and expression of peroxisome-associated genes are cross-regulated but uncoupled in Arabidopsis thaliana. Plant Cell Environ. 31, 492–505. doi: 10.1111/j.1365-3040.2008.01780.x
Chen, Z. J., Zhang, X. L., He, X. Y., Davi, N. K., Li, L. L., Bai, X. P. (2015). Response of radial growth to warming and CO2 enrichment in southern Northeast China:a case of. Clim. Change 130, 559–571. doi: 10.1007/s10584-015-1356-8
Chidumayo, E. N. (2008). Implications of climate warming on seedling emergence and mortality of African savanna woody plants. Plant Ecol. 198, 61–71. doi: 10.1007/s11258-007-9385-7
Chu, H., Wang, C., Li, Z., Wang, H., Xiao, Y., Chen, J., et al. (2019). The dark septate endophytes and ectomycorrhizal fungi effect on Pinus tabulaeformis Carr. seedling growth and their potential effects to pine wilt disease resistance. Forests 10, 140. doi: 10.3390/f10020140
Chu, H., Wang, C., Wang, H., Chen, H., Tang, M. (2016). Pine wilt disease alters soil properties and root-associated fungal communities in Pinus tabulaeformis forest. Plant Soil 404, 237–249. doi: 10.1007/s11104-016-2845-x
Chu, H., Wang, H., Zhang, Y., Li, Z., Wang, C., Dai, D., et al. (2021). Inoculation with ectomycorrhizal fungi and dark septate endophytes contributes to the resistance of Pinus spp. to pine wilt disease. Front. Microbiol. 12, 687304. doi: 10.3389/FMICB.2021.687304
Cook, B. I., Smerdon, J. E., Seager, R., Coats, S. (2014). Global warming and 21st century drying. Clim. Dyn. 43, 2607–2627. doi: 10.1007/s00382-014-2075-y
Corrales, A., Koch, R. A., Vasco-Palacios, A. M., Smith, M. E., Ge, Z. W., Henkel, T. W. (2022). Diversity and distribution of tropical ectomycorrhizal fungi. Mycologia 114, 11–15. doi: 10.1080/00275514.2022.2115284
Deng, X., Song, X. S., Yin, D. C., Song, R. Q. (2017). Effect of inoculating Phialocephala fortinii D575 and Suillus luteus N94 on the growth of Pinus sylvestris var. mongolica and its resistant to damping-off. For. Pest Dis. 36, 21–25. doi: 10.3969/j.issn.1671-0886.2017.01.006 (in Chinese)
Elavarthi, S., Martin, B. (2010). Spectrophotometric assays for antioxidant enzymes in plants. Methods Mol. Biol. 639, 273–280. doi: 10.1007/978-1-60761-702-0_16
Evelin, H., Kapoor, R., Giri, B. (2009). Arbuscular mycorrihizal fungi in alleviation of salt stress: a review. Ann. Bot. 104, 1263–1280. doi: 10.1093/aob/mcp251
Fahy, D., Sanad, M. N., Duscha, K., Lyons, M., Liu, F., Bozhkov, P., et al. (2017). Impact of salt stress, cell death, and autophagy on peroxisomes: quantitative and morphological analyses using small fluorescent probe N-BODIPY. Sci. Rep. 7, 1–18. doi: 10.1038/srep39069
Farrell, C., Szota, C., Arndt, S. K. (2017). Does the turgor loss point characterize drought response in dryland plants? Plant Cell Environ. 40, 1500–1511. doi: 10.1111/pce.12948
Fernandez, C. W., Koide, R. T. (2013). The function of melanin in the ectomycorrhizal fungus Cenococcum geophilum under water stress. Fungal Ecol. 6, 479–486. doi: 10.1016/j.funeco.2013.08.004
Gaber, D. A., Berthelot, C., Blaudez, D., Kovács, G. M., Franken, P. (2023). Impact of dark septate endophytes on salt stress alleviation of tomato plants. Front. Microbiol. 14, 1124879. doi: 10.3389/fmicb.2023.1124879
Gao, J., Wang, J. F., Li, Y. H. (2023). Effects of soil nutrients on plant nutrient traits in natural Pinus tabuliformis forests. Plants 12, 735–735. doi: 10.3390/PLANTS12040735
Gill, S. S., Tuteja, N. (2010). Reactive oxygen species and antioxidant machinery in abiotic stress tolerance in crop plants. Plant Physiol. Bioch. 48, 909–930. doi: 10.1016/j.plaphy.2010.08.016
Gleason, F. H., Midgley, D. J., Letcher, P. M., McGee, P. A. (2006). Can soil Chytridiomycota survive and grow in different osmotic potentials. Mycol. Res. 110, 869–875. doi: 10.1016/j.mycres.2006.04.002
Goussous, S. J., Mohammad, M. J. (2009). Comparative effect of two arbuscular mycorrhizae and N and P fertilizers on growth and nutrient uptake of onions. Int. J. Agric. Biol. 11, 463–467. doi: 10.3763/ijas.2009.0459
Graham, J. H., Syversen, J. P. (1984). Influence of vesicular-arbuscular mycorrhiza on the hydraulic conductivity of roots of two Citrus root stocks. New Phytol. 97, 277–284. doi: 10.1111/j.1469-8137.1984.tb04132.x
Gucwa-Przepióra, E., Chmura, D., Sokołowska, K. (2016). AM and DSE colonization of invasive plants in urban habitat: a study of Upper Silesia (southern Poland). J. Plant Res. 129, 603–614. doi: 10.1007/s10265-016-0802-7
He, C., Wang, W. Q., Hou, J. L. (2019). Plant growth and soil microbial impacts of enhancing licorice with inoculating dark septate endophytes under drought stress. Front. Microbiol. 10. doi: 10.3389/fmicb.2019.02277
Hodge, A., Campbell, C. D., Fitter, A. H. (2001). An arbuscular mycorrhizal fungus accelerates decomposition and acquires nitrogen directly from organic material. Nature 413, 297–299. doi: 10.1038/35095041
Huang, J. P., Ji, M. X., Xie, Y. K., Wang, S. S., He, Y. L., Ran, J. J. (2016). Global semi-arid climate change over last 60 years. Clim. Dyn. 46, 1131–1150. doi: 10.1007/s00382-015-2636-8
Huertas, V., Jiménez, A., Diánez, F., Chelhaoui, R., Santos, M. (2024). Importance of dark septate endophytes in agriculture in the face of climate change. J. Fungi 10, 329. doi: 10.3390/JOF10050329
Jumpponen, A., Mattson, K. G., Trappe, J. M. (1998). Mycorrhizal functioning of Phialocephala fortinii with Pinus contorta on glacier forefront soil: interactions with soil nitrogen and organic matter. Mycorrhiza 7, 261–265. doi: 10.1007/s005720050190
Kapoor, D., Singh, S., Kumar, V., Romero, R., Prasad, R., Singh, J. (2019). Antioxidant enzymes regulation in plants in reference to reactive oxygen species (ROS) and reactive nitrogen species (RNS). Plant Gene 19, 100182. doi: 10.1016/j.plgene.2019.100182
Kumar, D., Singh, R., Upadhyay, S. K., Verma, K. K., Tripathi, R. M., Liu, H., et al. (2024). Review on interactions between nanomaterials and phytohormones: Novel perspectives and opportunities for mitigating environmental challenges. Plant Sci. 340, 111964. doi: 10.1016/j.plantsci.2023.111964
Li, X., He, X. L., Zhou, Y., Hou, Y. T., Zuo, Y. L. (2019). Effects of dark septate endophytes on the performance of Hedysarum scoparium under water deficit stress. Front. Plant Sci. 10. doi: 10.3389/fpls.2019.00903
Li, M., Hou, L., Liu, J., Yang, J., Zuo, Y., Zhao, L., et al. (2021). Growth-promoting effects of dark septate endophytes on the non-mycorrhizal plant Isatis indigotica under different water conditions. Symbiosis 85, 291–303. doi: 10.1007/S13199-021-00813-0
Li, X., Liu, F. (2016). “Drought stress memory and drought stress tolerance in plants: biochemical and molecular basis,” in Drought Stress Tolerance in Plants, vol. 1 . Eds. Hossain, I. M. A., Wani, S. H., Bhattacharjee, S., Burritt, D. J., Tran, L. S. P. (Springer, Berlin), 17–44. doi: 10.1007/978-3-319-28899-4_2
Li, M., Ren, Y., He, C., Yao, J., Wei, M., He, X. (2022a). Complementary effects of dark septate endophytes and trichoderma strains on growth and active ingredient accumulation of Astragalus mongholicus under drought stress. J. Fungi 8, 920–920. doi: 10.3390/JOF8090920
Li, M. T., Yuan, C., Zhang, X. H., Pang, W. B., Zhang, P. P., Xie, R. Z., et al. (2022b). The transcriptional responses of ectomycorrhizal fungus, Cenococcum geophilum, to drought stress. J. Fungi 9, 15–15. doi: 10.3390/JOF9010015
Liu, N., Zhao, Z. Y., Jiang, X. L., Xing, X. K. (2021). Review and prospect of researches on the mechanisms of mycorrhizal fungi in improving plant drought resistance. Mycosystema 214, 851–872. doi: 10.13346/j.mycosystema.200370
Lu, N., Yu, M., Cui, M., Luo, Z. J., Feng, Y., Cao, S., et al. (2016). Effects of different ectomycorrhizal fungal inoculates on the growth of Pinus tabulaeformis seedlings under greenhouse conditions. Forests 7, 316. doi: 10.3390/f7120316
Mandyam, K., Loughin, T., Jumpponen, A. (2010). Isolation and morphological and metabolic characterization of common endophytes in annually burned tallgrass prairie. Mycologia 102, 813–821. doi: 10.3852/09-212
Meena, K. K., Sorty, A. M., Bitla, U. M., Choudhary, K., Gupta, P., Pareek, A., et al. (2017). Abiotic stress responses and microbe-mediated mitigation in plants: the omics strategies. Front. Plant Sci. 8. doi: 10.3389/fpls.2017.00172
Pal, M., Tajti, J., Szalai, G., Peeva, V., Vegh, B., Janda, T. (2018). Interaction of polyamines, abscisic acid and proline under osmotic stress in the leaves of wheat plants. Sci. Rep. 8, 12839. doi: 10.1038/s41598-018-31297-6
Phillips, J. M., Hayman, O. S. (1970). Improved procedures for clearing roots and staining parasitic and vesicular-arbuscular mycorrhizal fungi for rapid assessment of infection. Trans. Br. Mycol. Soc 55, 158–160. doi: 10.1016/S0007-1536(70)80110-3
Quiroga, G., Erice, G., Aroca, R., Zamarreño, Á.M., García-Mina, J. M., Ruiz-Lozano, J. M. (2020). Radial water transport in arbuscular mycorrhizal maize plants under drought stress conditions is affected by indole-acetic acid (IAA) application. J. Plant Physiol. 246-247, 153115. doi: 10.1016/j.jplph.2020.153115
Raja, V., Majeed, U., Kang, H., Andrabi, K., John, R. (2017). Abiotic stress: Interplay between ROS, hormones and MAPKs. Environ. Exp. Bot. 137, 142–157. doi: 10.1016/j.envexpbot.2017.02.010
Rezaei-Chiyaneh, E., Mahdavikia, H., Subramanian, S., Alipour, H., Siddique, K. H. M., Smith, D. L. (2021). Co-inoculation of phosphate-olubilizing bacteria and mycorrhizal fungi: Effect on seed yield, physiological variables, and fixed oil and essential oil productivity of ajowan (Carum copticum L.) under water deficit. J. Soil Sci. Plant Nutr. 21, 3159–3179. doi: 10.1007/s42729-021-00596-9
Salehi-Lisar, S. Y., Bakhshayeshan-Agdam, H. (2016). “Drought stress in plants: causes, consequences, and tolerance,” in Drought Stress Tolerance in Plants, vol. 1 . Eds. Hossain, M., Wani, S., Bhattacharjee, S., Burritt, D., Tran, L. S. (Springer, Cham), 1–16. doi: 10.1007/978-3-319-28899-4_1
Sanad, M. N., Smertenko, A., Garland-Campbell, K. A. (2019). Differential dynamic changes of reduced trait model for analyzing the plastic response to drought phases: a case study in spring wheat. Front. Plant Sci. 10. doi: 10.3389/fpls.2019.00504
Santos, M., Cesanelli, I., Diánez, F., Sánchez-Montesinos, B., Moreno-Gavira, A. (2021). Advances in the role of dark septate endophytes in the plant resistance to abiotic and biotic stresses. J. Fungi 7, 939. doi: 10.3390/JOF7110939
Santos, S. G. D., Silva, P. R. A. D., Garcia, A. C., Zilli, J. E., Berbara, R. L. L. (2017). Dark septate endophyte decreases stress on rice plants. Braz. J. Microbiol. 48, 333–341. doi: 10.1016/j.bjm.2016.09.018
Saritha, M., Kumar, P., Panwar, N. R., Burman, U. (2021). Intelligent plant-microbe interactions. Arch. Agron. Soil Sci. 68, 1002–1018. doi: 10.1080/03650340.2020.1870677
Sharma, S., Verslues, P. E. (2010). Mechanisms independent of abscisic acid (ABA) or proline feedback have a predominant role in transcriptional regulation of proline metabolism during low water potential and stress recovery. Plant Cell Environ. 33, 1838–1851. doi: 10.1111/j.1365-3040.2010.02188.x
Subramanian, K., Santhanakrishnan, P., Balasubramanian, P. (2006). Responses of field grown tomato plants to arbuscular mycorrhizal fungal colonization under varying intensities of drought stress. Sci. Hortic. 107, 245–253. doi: 10.1016/j.scienta.2005.07.006
Surono, S., Narisawa, K. (2017). The dark septate endophytic fungus Phialocephala fortinii is a potential decomposer of soil organic compounds and a promoter of Asparagus officinalis growth. Fungal Ecol. 28, 1–10. doi: 10.1016/j.funeco.2017.04.001
Tedersoo, L., Bahram, M., Toots, M., Diédhiou, A. G., Henkel, T. W., Kjøller, R., et al. (2012). Towards global patterns in the diversity and community structure of ectomycorrhizal fungi. Mol. Ecol. 21, 4160–4170. doi: 10.1111/J.1365-294X.2012.05602.X
Tedersoo, L., Brundrett, M. (2017). Evolution of ectomycorrhizal symbiosis in plants. Ecol. Stud. 230, 407–467. doi: 10.1007/978-3-319-56363-3_19
Tedersoo, L., Drenkhan, R., Abarenkov, K., Anslan, S., Bahram, M., Bitenieks, K., et al. (2024). The influence of tree genus, phylogeny, and richness on the specificity, rarity, and diversity of ectomycorrhizal fungi. Environ. Microbiol. 16, e13253–e13253. doi: 10.1111/1758-2229.13253
van der Heijden, M. G. A. (2002). “Arbuscular mycorrhizal fungi as a determinant of plant diversity: in search of underlying mechanisms and general principles,” in Mycorrhizal Ecology. Eds. Heijden, M. G. A., Sanders, I. R. (Springer Berlin Heidelberg, New York, NY), 244–265.
Veresoglou, S. D., Chen, B. D., Rillig, M. C. (2012). Arbuscular mycorrhiza and soil nitrogen cycling. Soil Biol. Biochem. 46, 53–62. doi: 10.1016/j.soilbio.2011.11.018
Vergara, C., Araujo, K. E. C., Urquiaga, S., Santa-Catarina, C., Schultz, N., da Silva, A. E., et al. (2018). Dark septate endophytic fungi increase green manure-15N recovery efficiency, N contents, and micronutrients in rice grains. Front. Plant Sci. 9. doi: 10.3389/fpls.2018.00613
Vergara, C., Araujo, K. E. C., Urquiaga, S., Schultz, N., de Carvalho Balieiro, F., Medeiros, P. S., et al. (2017). Dark septate endophytic fungi help tomato to acquire nutrients from ground plant material. Front. Microbiol. 8. doi: 10.3389/fmicb.2017.02437
Wang, J., Zhang, H., Gao, J., Zhang, Y., Liu, Y., Tang, M. (2021). Effects of ectomycorrhizal fungi (Suillus variegatus) on the growth, hydraulic function, and non-structural carbohydrates of Pinus tabulaeformis under drought stress. BMC Plant Biol. 21, 171. doi: 10.1186/S12870-021-02945-3
Wu, Q. S., Xia, R. X., Zou, Y. N. (2006). Reactive oxygen metabolism in mycorrhizal and non-mycorrhizal citrus (Poncirus trifoliata) seedlings subjected to water stress. J. Plant Physiol. 163, 1101–1110. doi: 10.1016/j.jplph.2005.09.001
Xu, L., Niu, X., Li, X., Zheng, Y., Feng, H., Fu, Q., et al. (2022). Effects of nitrogen addition and root fungal inoculation on the seedling growth and rhizosphere soil microbial community of Pinus tabulaeformis. Front. Microbiol. 13. doi: 10.3389/FMICB.2022.1013023
Yin, D., Deng, X., Song, R. (2016). Synergistic effects between Suilllus luteus and Trichoderma virens on growth of Korean spruce seedlings and drought resistance of Scotch pine seedlings. J. For. Res. 27, 193–201. doi: 10.1007/s11676-015-0131-z
Yin, D., Song, R., Qi, J., Deng, X. (2018). Ectomycorrhizal fungus enhances drought tolerance of Pinus sylvestris var. mongolica seedlings and improves soil condition. J. For. Res. 29, 1775–1788. doi: 10.1007/s11676-017-0583-4
Zhang, R. Q., Tang, M., Chen, H., Tian, Z. Q. (2011). Effects of ectomycorrhizal fungi on damping-off and induction of pathogenesis-relatedproteins in Pinus tabulaeformis seedlings inoculated with Amanita vaginata. For. Pathol. 41, 262–269. doi: 10.1111/j.1439-0329.2010.00669.x
Zhang, X., Zhang, J., He, J., Li, M., Matsushita, N., Geng, Q., et al. (2024). Physiological and transcriptome responses of Pinus massoniana seedlings inoculated by various ecotypes of the ectomycorrhizal fungus Cenococcum geophilum during the early stage of drought stress. J. Fungi 10, 71. doi: 10.3390/JOF10010071
Zhao, M., Hao, W. Y., Ning, X. Z., Hao, L. F., Yan, H. X., Mu, Y. N., et al. (2020). Screening of excellent ectomycorrhizal fungi-tree for drought resistant with Pinus sylvestris var. mongolica. Bull. Botanical Res. 40, 133–140. doi: 10.7525/j.issn.1673-5102.2020.01.018
Keywords: antioxidant activities, ectomycorrhizal fungi, mixed inoculation, osmotic adjustment, PEG-6000, root symbiotic fungi
Citation: Xu L, He J, Meng Y, Zheng Y, Lu B, Zhang J and Zhou Y (2024) Enhancing drought resistance in Pinus tabuliformis seedlings through root symbiotic fungi inoculation. Front. Plant Sci. 15:1446437. doi: 10.3389/fpls.2024.1446437
Received: 09 June 2024; Accepted: 01 August 2024;
Published: 20 August 2024.
Edited by:
Marzena Sujkowska-Rybkowska, Warsaw University of Life Sciences, PolandReviewed by:
Chandra Shekhar Seth, University of Delhi, IndiaCopyright © 2024 Xu, He, Meng, Zheng, Lu, Zhang and Zhou. This is an open-access article distributed under the terms of the Creative Commons Attribution License (CC BY). The use, distribution or reproduction in other forums is permitted, provided the original author(s) and the copyright owner(s) are credited and that the original publication in this journal is cited, in accordance with accepted academic practice. No use, distribution or reproduction is permitted which does not comply with these terms.
*Correspondence: Yong Zhou, emhvdXlvbmcyNzVAc2luYS5jb20=
†These authors have contributed equally to this work
Disclaimer: All claims expressed in this article are solely those of the authors and do not necessarily represent those of their affiliated organizations, or those of the publisher, the editors and the reviewers. Any product that may be evaluated in this article or claim that may be made by its manufacturer is not guaranteed or endorsed by the publisher.
Research integrity at Frontiers
Learn more about the work of our research integrity team to safeguard the quality of each article we publish.