- 1School of Hydraulic Engineering, Zhejiang University of Water Resources and Electric Power, Hangzhou, China
- 2Engineering Research Center of Ecology and Agriculture Use of Wetland, Ministry of Education, Yangtze University, Jingzhou, Hubei, China
Water-saving irrigation and the mixed application of controlled-release nitrogen fertilizer (CRNF) and common urea (CU; with a higher nitrogen release rate) have shown promise in improving rice yield with high resource use efficiency. However, the physiological mechanism underlying this effect remains largely unknown. This study involved a field experiment on rice in Jingzhou City, Central China, in 2020 and 2021. Two irrigation regimes were employed [alternate wetting and drying irrigation (AWD) and conventional flood irrigation (CI)], with three nitrogen (N) compounding modes [00% CU (N1), 60% CRNF + 40% CU (N2), and 100% CRNF (N3)] with an equal N rate of 240 kg ha−1. The results indicated a significant interactive effect of watering regimes and N compounding modes on net photosynthetic rate (Pn), leaf area index (LAI), and SPAD values; activities of superoxide dismutase (SOD), peroxidases (POD), catalase (CAT), glutamine synthetase (GS), glutamine 2-oxoglutarate amidotransferase (GOGAT), and nitrate reductase (NR); and the contents of malondialdehyde (MDA) and soluble protein in rice leaves. Compared with N1, N2 and N3 increased the Pn, LAI, and SPAD values; activities of SOD, POD, CAT, NR, GS, and GOGAT; and soluble protein content but decreased MDA content in the post-growth (heading and maturity) stages by 8.7%–31.2% under the two irrigation regimes. Compared to CI (traditional irrigation), AWD had higher Pn, LAI, and SPAD values; activities of SOD, POD, CAT, NR, GS, and GOGAT; and soluble protein content (increased by 12.1%–38.0%, and lower MDA content (reduced by 13.1%–27.6%) irrespective of N compounding modes. This suggested that AWD combined with N2 and N3 could delay the leaf senescence of rice, thus achieving a larger grain yield. Moreover, AWD significantly decreased water costs (irrigation amount) and labor costs (irrigation frequency), thus increasing total income. N2 decreased fertilizer costs with a higher or comparable total income compared with N3. Therefore, the AWDN2 treatment achieved the highest net income (13,907.1 CNY ha−1 in 2020 and 14,085.7 CNY ha−1 in 2021). AWD interacted with 60% CRNF + 40% (N2) to delay leaf senescence by improving photosynthesis, antioxidant defense system, osmoregulation, and N assimilation, contributing to high grain yield and net income in rice.
1 Introduction
Rice (Oryza sativa L.) is a primary cereal crop serving as the staple diet for approximately 50% of the world’s population. With the increasing world population, the rice yield needs to be boosted by 25% to satisfy growing food demand (Fertitta-Roberts et al., 2019; Su et al., 2023). The production of rice is influenced by soil characteristics (Bueno et al., 2010), cultivation measurement (Ly et al., 2016), and weather conditions (Chiba et al., 2017). Notably, freshwater plays a vital role in determining the physiological processes of rice. Rice cultivation consumes a substantial amount of freshwater resources, with over two-thirds of the irrigation water in China being used for rice cultivation (National Bureau of Statistics, 2022). A previous study has estimated that 30–40 million ha of rice crops will face water scarcity by 2050 (Mboyerwa et al., 2021). Moreover, climatic changes, urbanization, and industrialization have led to a rise in the use of valuable freshwater resources, intensifying the water scarcity for rice cultivation (Ishfaq et al., 2020). Therefore, water-saving irrigation strategies with high water use efficiency (WUE) need to be explored to ensure sustainable rice production.
In addition to irrigation water, the application of nitrogen (N) fertilizer is another important factor affecting rice production, constituting a major resource input for rice crops (Spiertz, 2010; Wang et al., 2018). The application of N fertilizer enhances rice yield (>30%) and is involved in 40% of the rice growth processes (Peng et al., 2021). Applying a high amount of N fertilizer increases grain yield, but low N use efficiency (NUE) is a major concern for intensive rice production (Ding et al., 2018). Low NUE is also accompanied by various environmental issues, including greenhouse gas emissions, water eutrophication, and soil degradation (Ju et al., 2009; Spiertz, 2010; Ding et al., 2018; Qi et al., 2023a). In addition to adverse environmental impacts, the limited yield gain at higher N application rates is a concern, decreasing the economic profits of farmers (Yuan et al., 2022). Moreover, the excessive or non-scientific application of N fertilizer reduces the grain yield of rice (Wang et al., 2016; Xu et al., 2018). N and water work together to determine the physiological processes and final yield of rice. Therefore, efficient management of irrigation water and N fertilizer should be encouraged to synergistically enhance grain yield and water–N use efficiencies of rice cultivation.
Various water-saving irrigation technologies, such as internal drainage (Ramasamy et al., 1997), continuous soil saturation (Borrell et al., 1997), rainfall-adapted irrigation (Yan et al., 2022), intermittent irrigation (Berkhout et al., 2015), non-flooded mulching cultivation (Zhou et al., 2023), and alternate wetting and drying irrigation (AWD) (Belder et al., 2004; Ishfaq et al., 2020; Liang et al., 2023), have been explored to address water shortages in rice cultivation. Of these, AWD is the most popular irrigation strategy because it is simple to operate, easy to follow, and less labor intensive. Moreover, this kind of strategy provides a comparable or even larger grain yield of rice with obviously less volume of irrigation water (Lampayan et al., 2015; Xu et al., 2018; Ishfaq et al., 2020; Zhang et al., 2021; Su et al., 2023). Improved N fertilizer management strategies have also been investigated to improve NUE in rice production. Techniques such as deep placement of N fertilizer, site-based N fertilization, multi-split N management, and precise and smart N application have demonstrated the potential to enhance NUE and grain yield in rice (Liu et al., 2013; Hooper et al., 2014; Ding et al., 2018). In particular, deep placement of urea significantly increased rice yield and NUE and reduced cumulative methane and nitrous oxide emissions compared with broadcast urea (Gaihre et al., 2015; Islam et al., 2022). However, the application of these N management strategies typically relies on knowledge-intensive technologies, specialized equipment, and substantial labor forces, limiting their application (Wang et al., 2018). Alternately, highly efficient N fertilizers, such as controlled-release N fertilizer (CRNF), have shown promise in better meeting N demand and enhancing the growth processes of rice (Song et al., 2014; Zheng et al., 2016; Ding et al., 2018; Li et al., 2022). Additionally, CRNF fertilization is easy to implement and requires low labor costs, making it a hot topic of research both domestically and internationally. The application of CRNF can synergistically improve WUE and NUE compared with common urea (CU) fertilization (Ye et al., 2013; Ding et al., 2018; Sun et al., 2019; Yan et al., 2022; Zhang et al., 2024). However, pure (100%) application of CRNF cannot meet the N demands of rice in the early (tillering) stage (Zhang et al., 2021). Additionally, the material used for CRNF production is expensive, reducing the possibility of adoption by farmers for grain crop production, especially in developing countries (Shaviv, 2001). Therefore, blending the application of CRNF and CU is a newly developed N management strategy for the sustainable development of rice production (Zhang et al., 2021; Yan et al., 2022).
Plants regulate their cellular metabolism and defense mechanisms in the face of drought, waterlogging, salinity, and other abiotic stresses (Bohnert and Jensen, 1996). Superoxide dismutase (SOD), peroxidases (POD), and catalase (CAT) are extremely important protective enzymes involved in the active oxygen metabolism in plants; they scavenge oxygen free radicals in biological systems (Foyer and Noctor, 2000). Malondialdehyde (MDA) is a stable product of membrane lipid peroxidation, and its content can be used to assess the degree of oxidative damage (Ren et al., 2023). Soluble proteins are the main constituent of various cells and organelles, playing a vital role in photosynthesis (Qi et al., 2021). Chlorophyll is the most important and effective pigment required for normal photosynthesis in plants; its content (indicated by the leaf SPAD value) is closely associated with the degree of leaf senescence (Yu et al., 2023). The activities of SOD, POD, and CAT, along with the contents of MDA, soluble proteins, and SPAD values, are key physiological and biochemical indicators reflecting leaf senescence (Foyer and Noctor, 2000; Yu et al., 2023). An imbalance in active oxygen metabolism is one of the main causes of leaf aging. Drought, N deficiency, and their combination can reduce the activities of SOD, POD, and CAT, leading to the accumulation of reactive oxygen species (ROS), an increase in MDA content, chlorophyll degradation, reduced phytase activity, and diminished photosynthetic capacity, thus accelerating leaf senescence (Li et al., 2020; Qi et al., 2021). Implementing scientific irrigation regimes and N fertilization strategies are two effective approaches used to improve rice yield and resource use efficiency by influencing physiological processes (Sun et al., 2012; Wang et al., 2018). Compared with conventional flood irrigation (CI), moderate AWD increases the activities of GS, GOGAT, NR, SOD, POD, and CAT in rice leaves and increases net photosynthetic rate (Pn) and SPAD value, thereby delaying leaf senescence (Fu et al., 2024). Li et al. (2010) also demonstrated that compared with full irrigation, deficit irrigation (alternate partial root-zone irrigation) increased chlorophyll content and SOD and POD activities but reduced MDA content in maize leaves. Moreover, compared with CU fertilization, the application of CRNF increased LAI, Pn, and SPAD values (Wang et al., 2018) and the activities of GS, GOGAT, and NR in rice leaves (Yang et al., 2012). In terms of the water–N coupling effects, AWD attenuated the positive effects of moderate N dosages on the physiological and morphological characteristics of roots (Xu et al., 2018). AWD, when combined with an improved N fertilization mode (reducing N application rates by 20% and delaying N topdressing), enhanced the growth of both roots and shoots in rice plants (Yan et al., 2022). Moderate deficit irrigation interacted with CRNF to maintain the leaf greenness of maize by enhancing the LAI, SPAD value, and Pn (Li et al., 2020). However, the data on the effects of AWD combined with the blended use of CRNF and CU on leaf senescence characteristics and economic benefits in rice are still lacking.
The conventional N application mode usually leads to low NUE under water-saving irrigation conditions (Han et al., 2014). Therefore, clarifying whether a combination of CRNF (compounding CRNF and CU) and AWD can realize the goal of improving rice yield and resource use efficiency is essential (Qi et al., 2023a, b). Also, the physiological mechanism underlying the effect of this combination needs to be illustrated. Therefore, the present study evaluated the LAI, NR, GS, GOGAT, SOD, POD, and CAT activities; Pn and SPAD values; soluble protein and MDA contents; and economic benefits in rice production regarding the combination of CRNF and CU patterns under AWD compared with the CI and clarified the causes of possible differences. It was hypothesized that AWD interacted with the blended use of CRNF and CU to provide proper soil N and water conditions, thus delaying the senescence by improving photosynthesis, antioxidant defense system, osmoregulation, and N assimilation, and thus leading to a greater total income (grain yield) with less costs on resource input (water, N fertilizer, and labor), consequently achieving a high net income.
2 Materials and methods
2.1 Experimental site and materials
Field experiments were conducted in Jingzhou City, Central China (latitude 30° 26′N, longitude 112° 39′E, altitude 30 m) between 2020 and 2021. This region has a subtropical monsoon climate with an average annual precipitation of 1,050 mm. The soil in the tested field was classified as Entisol (Typic Fluvaquent, Entisol, US classification). The initial topsoil (0–40 cm) had a pH of 7.3. The fertilizer characteristics of the soil were as follows: available N, 79.8 mg kg−1; total N, 2.10 g kg−1; Olsen P, 38.8 mg kg−1; total P, 0.48 g kg−1; total K, 0.87 g kg−1; and exchangeable K, 108.8 mg kg−1. The field moisture capacity and soil bulk density were 0.35 cm3 cm−3 and 1.41 g cm−3, respectively. The rainfall during the rice-growing season in the 2 years is shown in Figure 1.
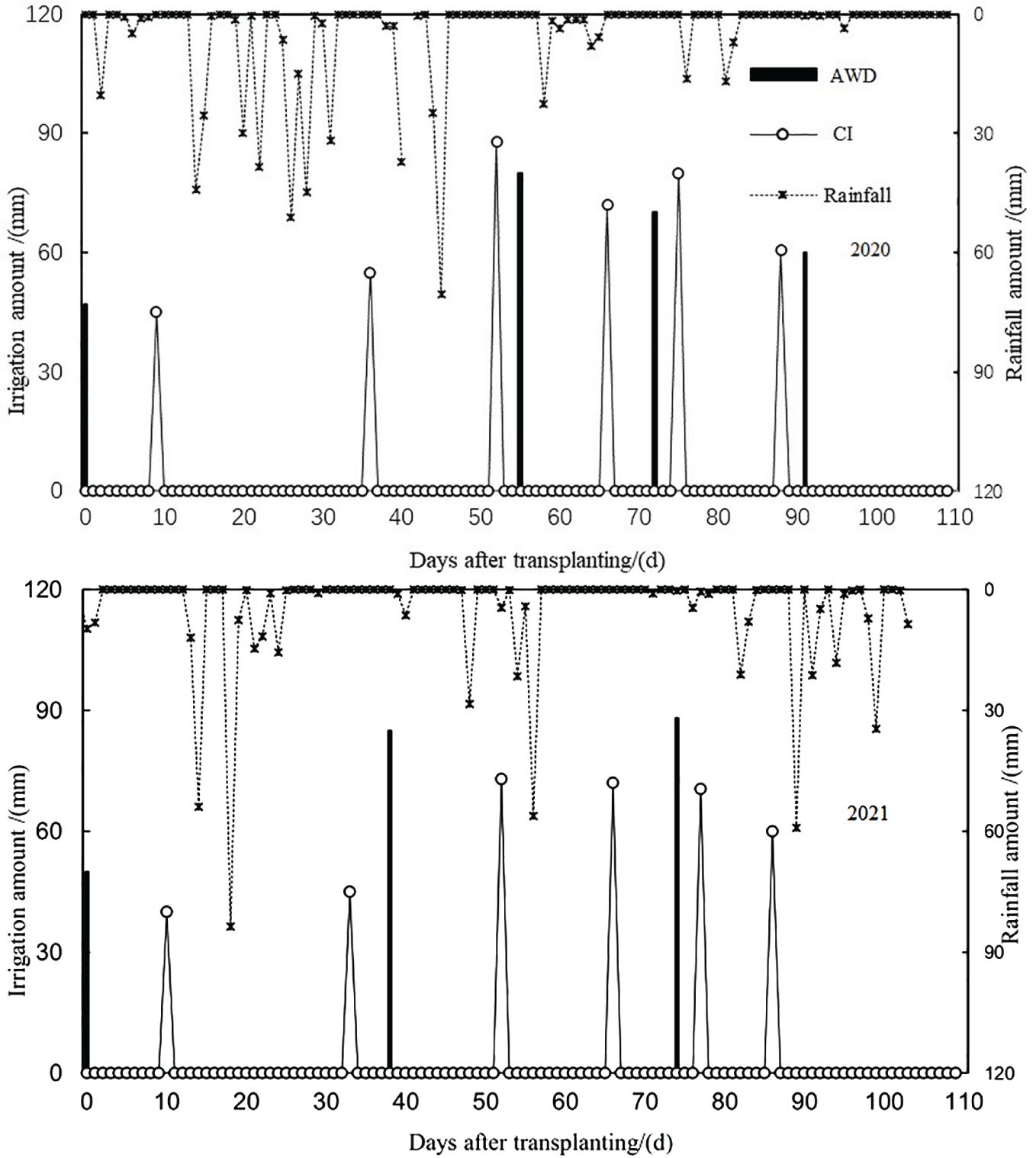
Figure 1. The volume of precipitation and irrigation water during the two rice-growing seasons (2020 and 2021) under conventional flooding irrigation (CI) and alternate wetting and drying irrigation (AWD).
2.2 Experimental design
The variety Liangyou 152 (O. sativa L.), which is now widely cultivated in the local area, was adopted as the tested crop. A 2 × 3 factorial design (two irrigation regimes and three N compounding modes) was conducted in a randomized complete block setup with three replications. The irrigation regimes consisted of CI and AWD. They were started 10 days after transplanting (DAT) and continued until the maturity of rice. A continuous layer (10–60 mm) of water was maintained in the CI plots after transplanting until the final drainage was approximately 10 days before the harvest. In the AWD plots, rice plants were flooded intermittently, and the irrigation (20–40 mm of water) was not conducted until the soil water potential (SWP) reached −10 kPa at 15−20-cm soil depth (Wang et al., 2016). The SWP was monitored using a tensiometer (5-cm sensor). Moreover, the irrigation was initiated for N fertilization and pesticide application. A flow meter (LXSG-50 Flow Meter; Shanghai Water Meter) was used for measuring the amount of irrigation water. The irrigation and drainage were conducted independently.
The N compounding modes consisted of 100% CU (N1), 60% CRNF + 40% CU (N2), and 100% CRNF (N3), with an equal N rate of 240 kg ha−1. Polymer-coated urea containing 43% N (Kingenta Ecological Engineering Co., Ltd., Shandong, China) was used as CRNF (N discharge continued for 90 days). The conduction of N1 exactly followed the N management strategy of local farmers: CU (prilled urea containing 46.4% N) was applied as a basal dose (60%) and as a topdressing in the tillering stage (40%). In N2 and N3, both CRNF and CU were applied once before transplantation. In addition, 529 kg ha−1 calcium superphosphate (P2O5 17%) and 300 kg ha−1 muriate of potash were applied before sowing. Tillering N application was conducted on May 24 and 25. Four-week-old seedlings were transplanted on May 15 and 16 at a density of 24 hills m−2 (3 plants hill−1). The rice plants were harvested on September 16–19 in the 2 years. The individual plots (8 × 5 m2) were edged with a plastic film inserted 30 cm below the soil surface to form a barrier. Diseases, insects, and weeds were well controlled across the two growing seasons.
2.3 Measurements
2.3.1 LAI and SPAD values
The leaf area and SPAD values were collected at the tillering, jointing, heading, filling, and maturity stages, corresponding to 17 and 18, 41 and 42, 75 and 75, 96 and 97, and 125 and 126 DAT in 2020 and 2021, respectively. Leaf area was measured using an area meter (LI-3050C; Li-Cor, Lincoln, NE, USA) on green leaves from eight hills. The LAI was calculated as the ratio of total leaf area to per unit ground area (Li et al., 2020). SPAD values were determined using a portable SPAD-502 chlorophyll meter (Minolta Camera Co., Osaka, Japan).
2.3.2 Physiological measurements
The functional leaves (last fully developed leaves) of rice were measured from three randomly selected plants in the jointing, heading, and maturity stages on the same date as leaf area determination. The Pn was measured between 10:00 AM and 1:00 PM on clear days using a portable photosynthesis system (LI-6400; Li-Cor Inc., NE, USA) when the photosynthetically active radiation above the canopy was 1,500 μmol m−2 s−1. The POD, SOD, and CAT activities were determined using guaiacol colorimetry, nitro blue tetrazolium, and permanganate titration methods, respectively, as previously described (Ren et al., 2018). The MDA content was measured using the thiobarbituric acid method (Du and Bramlage, 1992). The NR, GS, and GOGAT activities of functional leaves were determined using the protocols proposed by Aslam et al. (2001); Lea et al. (1990), and Singh and Srivastava (1986), respectively. The soluble protein content was determined using the method proposed by Mohammadkhani and Heidari (2007).
2.3.3 Grain yield
An 8-m2 area of rice plants in the middle of each plot was harvested for grain yield determination (the grains were air-dried).
2.4 Analysis of economic benefit
The economic benefit of rice was computed using the following formula proposed by Lai et al. (2022):
where TI is the total income (CNY ha−1), RP is the rice price (CNY kg−1), GY is the grain yield (kg ha−1), NI is the net income (CNY ha−1), FC is the fertilizer cost (CNY ha−1), LC is the labor cost (CNY ha−1), WC is the water cost (CNY ha−1), and OC is the other cost (CNY ha−1, including costs of seed, weeding, pest control, and field management).
2.5 Statistical analysis
The analysis of variance was performed using the SAS/STAT statistical analysis package (version 6.12; SAS Institute, Cary, NC, USA). The statistical model used in the study accounted for sources of variation due to replication, year, irrigation regime, N compounding mode, and their interactions (year × irrigation regime, year × N compounding mode, and year × irrigation regime × N compounding mode). The data for each sampling date were analyzed separately, and means were compared using the least significant difference (LSD) test at p < 0.05 (LSD0.05).
3 Results
Watering regime (W), N fertilization strategies, and W × N had significant or extremely significant effects on Pn, LAI, and SPAD value; activities of SOD, POD, CAT, NR, GS, and GOGAT; and contents of MDA and soluble protein in rice leaves. However, no significant difference was observed in these parameters between the two study years (Table 1). Therefore, the aforementioned parameters were presented as mean across the two study years (Tables 2, 3; Figures 2–6).
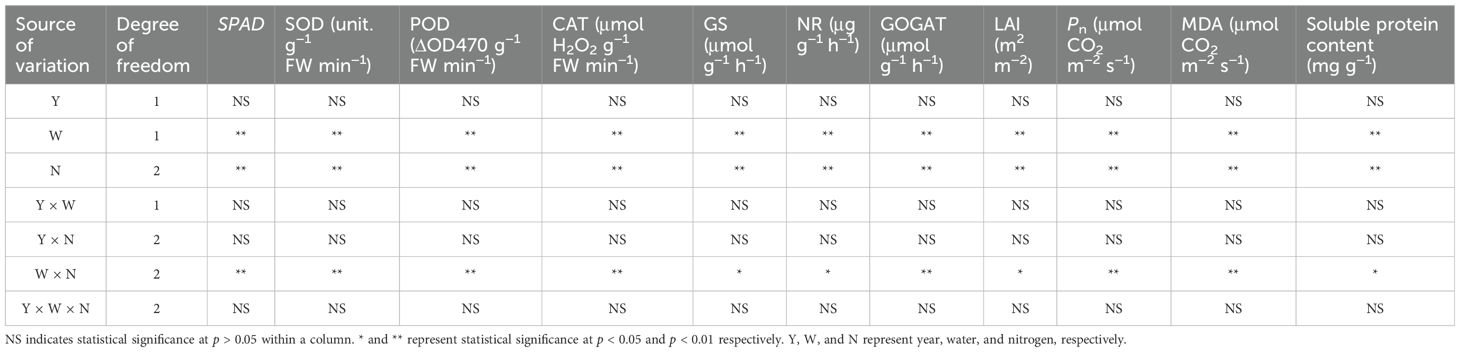
Table 1. Analysis of variance of SPAD value, superoxide dismutase (SOD), peroxidases (POD), catalase (CAT), nitrate reductase (NR), glutamine synthetase (GS), glutamine 2-oxoglutarate amidotransferase (GOGAT), net photosynthetic rate (Pn), leaf area index (LAI), SPAD value, and malondialdehyde (MDA) and soluble protein contents under condition of irrigation regimes and nitrogen management strategies interaction.
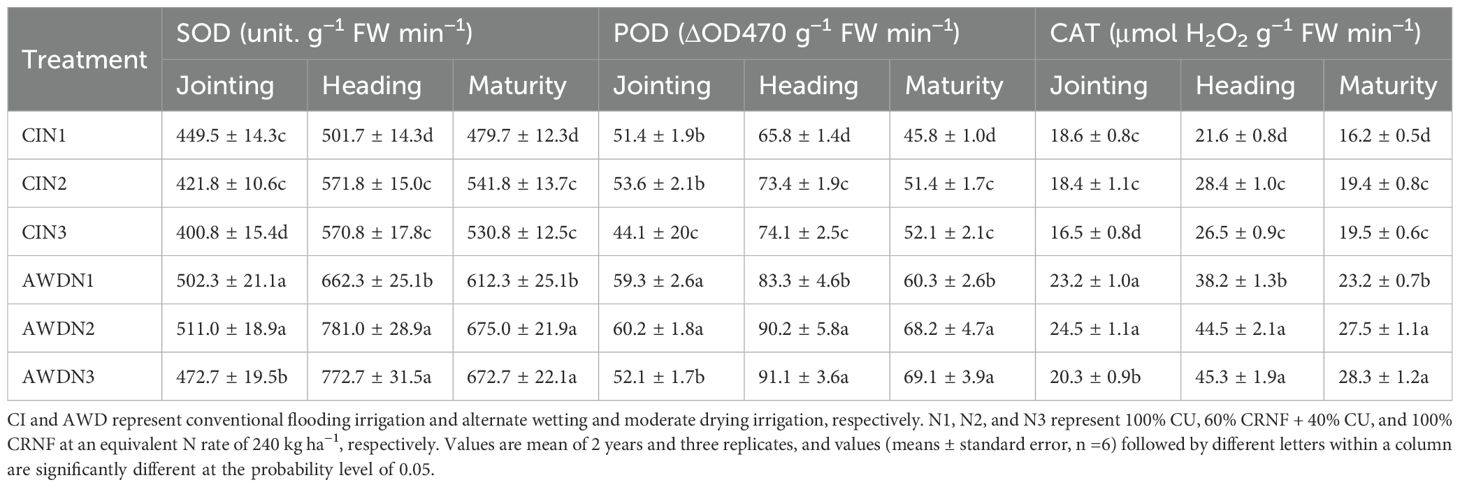
Table 2. Superoxide dismutase (SOD), peroxidases (POD), and catalase (CAT) of rice leaves at the jointing, heading, and maturity stages as affected by different water and nitrogen management strategies.
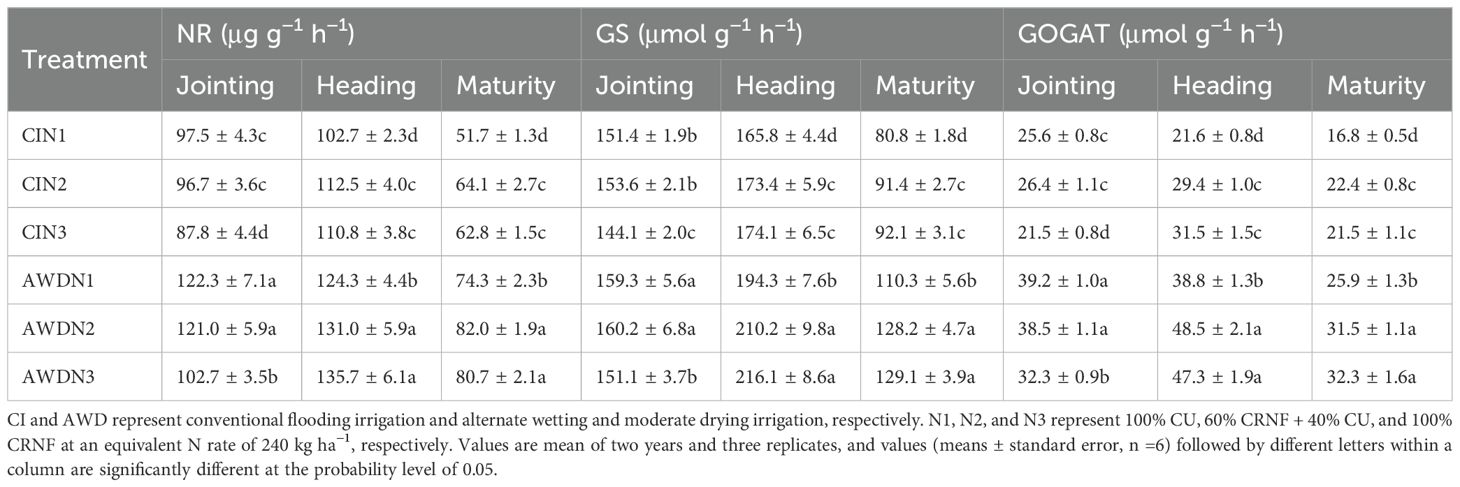
Table 3. Nitrate reductase (NR), glutamine synthetase (GS), and glutamine 2-oxoglutarate amidotransferase (GOGAT) of rice leaves at the jointing, heading, and maturity stages as affected by different water and nitrogen management strategies.
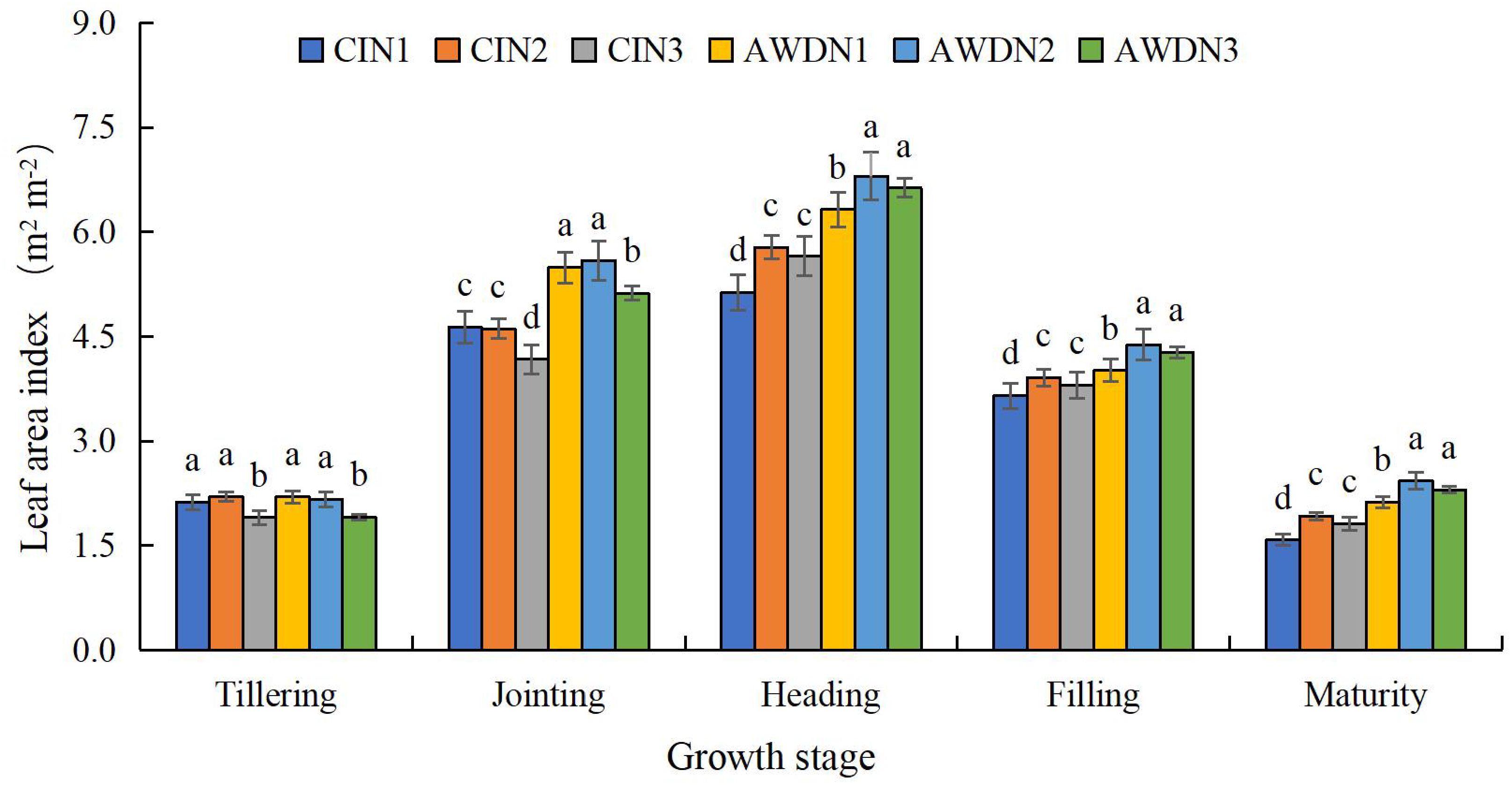
Figure 2. Leaf area index (LAI) of rice as affected by different water and nitrogen management strategies. CI and AWD represent conventional flooding irrigation and alternate wetting and moderate drying irrigation, respectively. N1, N2, and N3 represent 100% CU, 60% CRNF + 40% CU, and 100% CRNF at an equivalent N rate of 240 kg ha−1, respectively. Values (mean ± standard error, n = 6) are mean of 2 years and three replicates. Means by different letters are significantly p < 0.05.
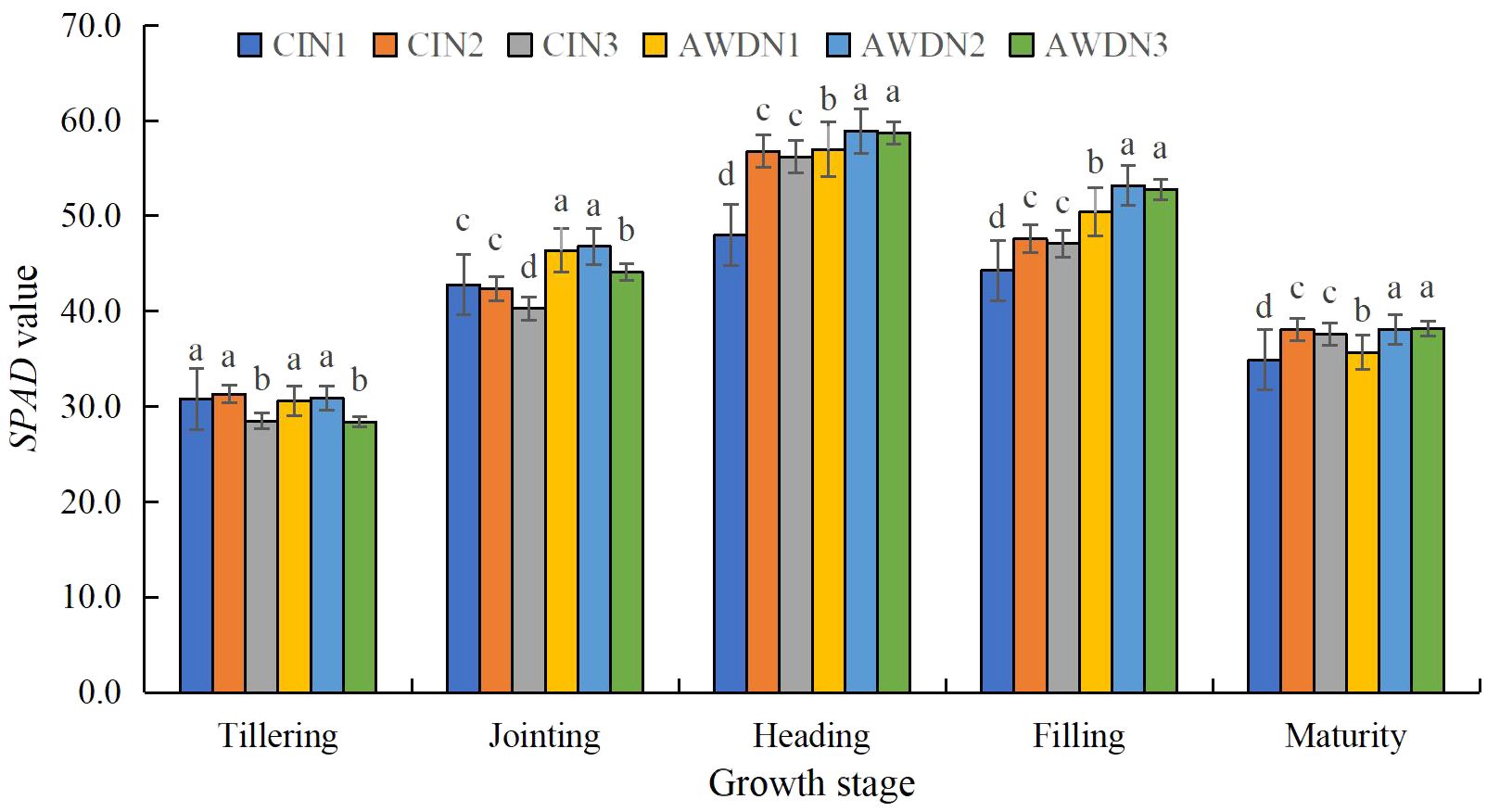
Figure 3. SPAD values of rice leaves as affected by different water and nitrogen management strategies. CI and AWD represent conventional flooding irrigation and alternate wetting and moderate drying irrigation, respectively. N1, N2, and N3 represent 100% CU, 60% CRNF + 40% CU, and 100% CRNF at an equivalent N rate of 240 kg ha−1, respectively. Values (mean ± standard error, n = 6) are mean of 2 years and three replicates. Means by different letters are significantly different at p < 0.05.
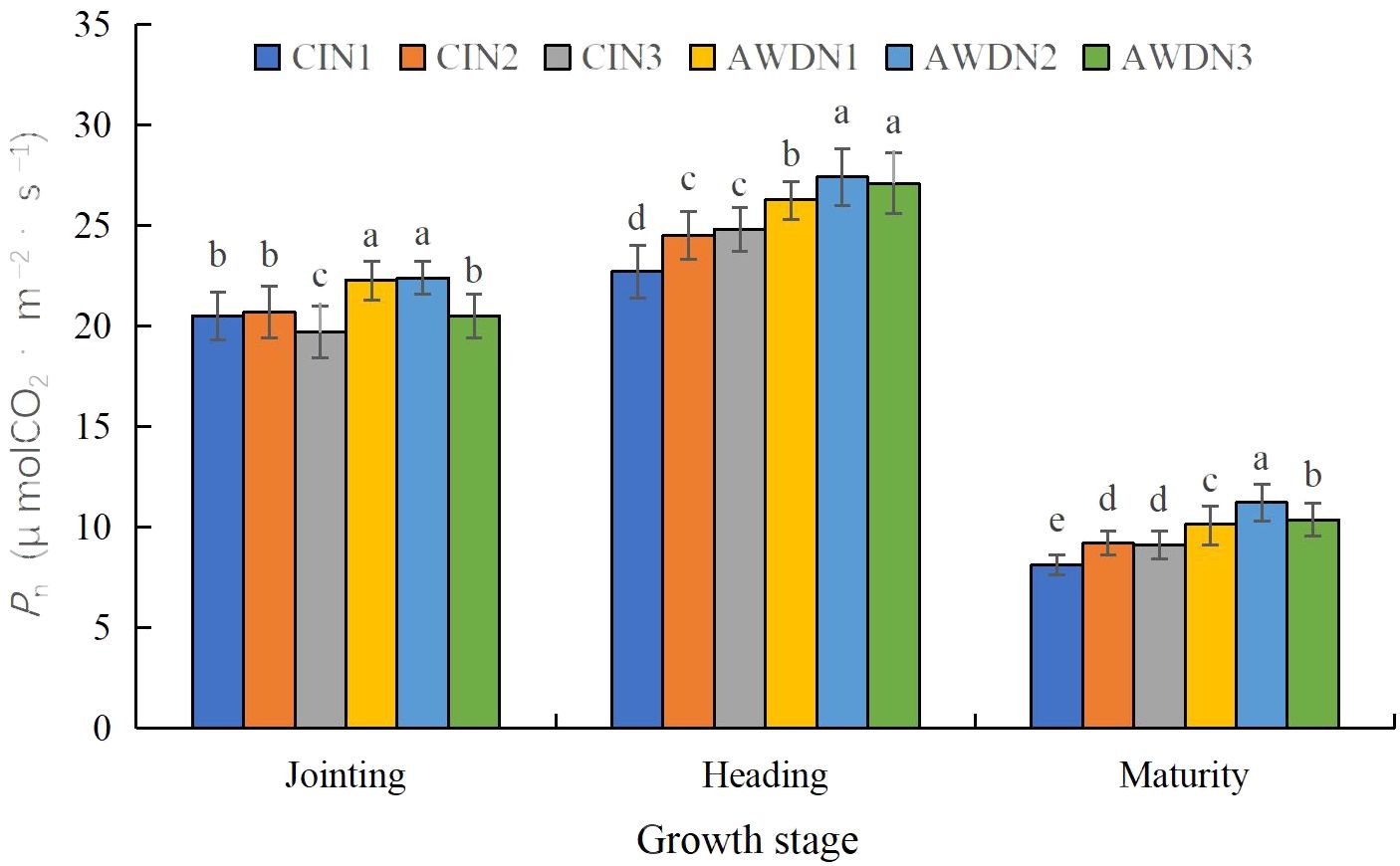
Figure 4. Net photosynthetic rate (Pn) of rice leaves as affected by different water and nitrogen management strategies. CI and AWD represent conventional flooding irrigation and alternate wetting and moderate drying irrigation, respectively. N1, N2, and N3 represent 100% CU, 60% CRNF + 40% CU, and 100% CRNF at an equivalent N rate of 240 kg ha−1, respectively. Values (mean ± standard error, n = 6) are mean of 2 years and three replicates. Means by different letters are significantly different at p < 0.05.
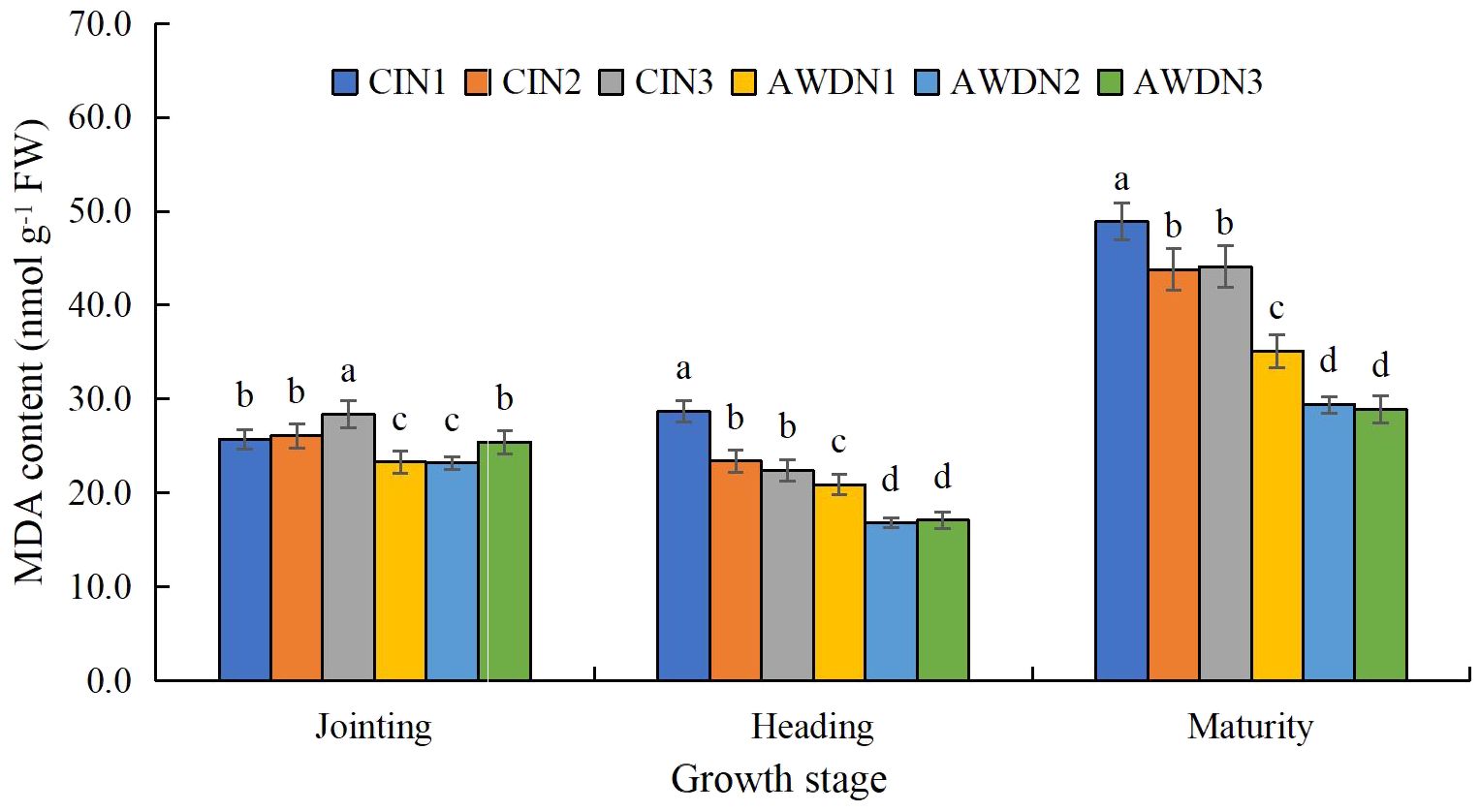
Figure 5. Malondialdehyde (MDA) content of rice leaves affected by different water and nitrogen management strategies. CI and AWD represent conventional flooding irrigation and alternate wetting and moderate drying irrigation, respectively. N1, N2, and N3 represent 100% CU, 60% CRNF + 40% CU, and 100% CRNF at an equivalent N rate of 240 kg ha−1, respectively. Values (mean ± standard error, n = 6) are mean of 2 years and three replicates. Means by different letters are significantly different at p < 0.05.
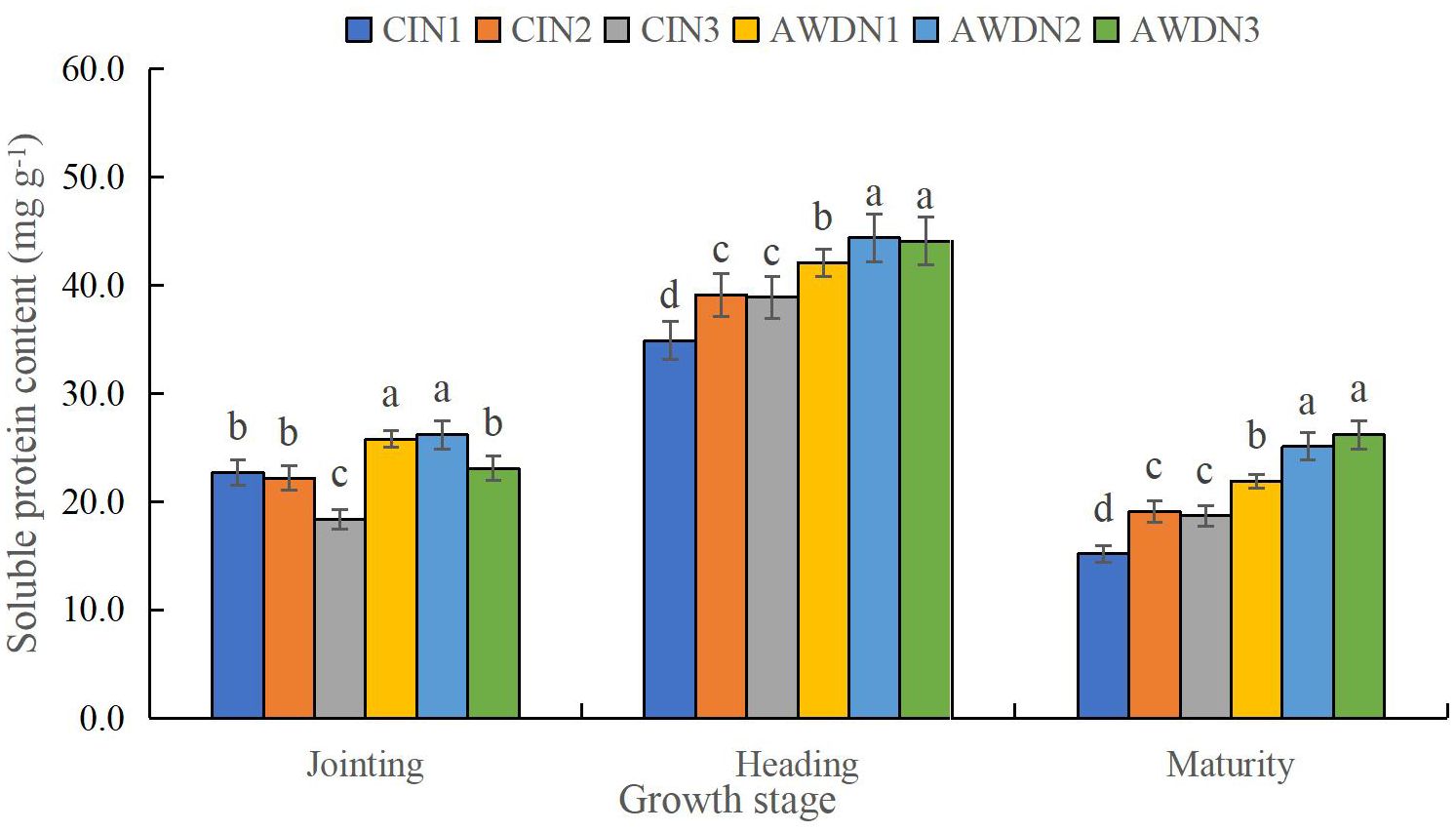
Figure 6. Soluble protein content of rice leaves affected by different water and nitrogen management strategies. CI and AWD represent conventional flooding irrigation and alternate wetting and moderate drying irrigation, respectively. N1, N2, and N3 represent 100% CU, 60% CRNF + 40% CU, and 100% CRNF at an equivalent N rate of 240 kg ha−1, respectively. Values (mean ± standard error, n = 6) are mean of 2 years and three replicates. Means by different letters are significantly different at p < 0.05.
3.1 LAI and SPAD values
Under all treatments, the LAI reached the maximum in the heading stage and then declined until the maturity stage. The LAI in the tillering stage was significantly higher (increased by 12.4%–17.8%) in N1 and N2 plots compared with N3 plots under AWD and CI (Figure 2). The LAI in the jointing stage was significantly higher in AWD-treated plots irrespective of the N treatments. Additionally, the N1 and N2 had a higher LAI in the jointing stage than the N3 under the two irrigation regimes. The LAI in the heading, filling, and maturity stages was 21.4%–45.6% higher in AWD than CI, irrespective of N treatments. Furthermore, the N2 and N3 resulted in a higher LAI in the three aforementioned growth stages than the N1 under the two irrigation regimes. AWDN2 treatment led to the highest LAI in the five measured growth stages (Figure 2). SPAD values in the measured growth stages under different treatments showed similar variations compared with the LAI in the corresponding stages (Figure 3).
3.2 Activities of SOD, POD, and CAT
The activities of SOD, POD, and CAT under all treatments were the highest in the heading stage (Table 2). The N1 and N2 groups had the highest activities of SOD, POD, and CAT (increased by 9.2%–21.3%) in the jointing stage compared with the N3 group under CI and AWD. Additionally, AWD plots had higher activities of SOD, POD, and CAT (increased by 11.9%–35.7%) compared with CI plots, irrespective of the N treatments. Also, AWD had higher activities of SOD, POD, and CAT in the latter growth (heading and maturity) stages. Additionally, N2 and N3 resulted in higher activities of SOD, POD, and CAT (increased by 9.4%–22.7%) than N1 under the two irrigation regimes. The highest activities of SOD, POD, and CAT were found under AWDN3 and AWDN2 treatments, whereas the lowest activities of SOD, POD, and CAT were observed under CIN1 treatment (Table 2).
3.3 Activities of NR, GS, and GOGAT
The activities of NR, GS, and GOGAT showed a rise-and-fall trend in the growing season, peaking in the heading stage before decreasing (Table 3). AWD had higher activities of NR, GS, and GOGAT in the jointing stage (increased by 12.4%–31.7%) compared with CI at each N rate. Additionally, N1 and N2 had higher activities of NR, GS, and GOGAT (increased by 13.5%–22.8%) than N3 under CI and AWD. The CIN3 treatment exhibited the lowest activities of NR, GS, and GOGAT. AWD plots had higher activities of NR, GS, and GOGAT (increased by 17.8%–40.1%) in the heading and maturity stages compared with CI plots. N2 and N3 resulted in higher activities of NR, GS, and GOGAT (increased by 14.5%–36.3%) than N1 under AWD and CI. The AWDN2 and AWDN3 treatment groups had the highest activities of NR, GS, and GOGAT, whereas these activities were the lowest in the CIN1 treatment group (Table 3).
3.4 Pn
The Pn values under all treatments were the highest in the heading stage (Figure 4). The Pn values were significantly (12.8%–28.6%) greater in N1 and N2 plots compared with N3 plots in the jointing stage, irrespective of the irrigation treatment. Additionally, AWD plots had higher Pn (increased by 15.0%–30.1%) than CI plots at each N rate. The Pn was significantly higher (increased by 13.4%–22.5%) in N2 and N3 plots compared with N1 plots in the heading and maturity stages, irrespective of the irrigation regimes; N2 had higher Pn than N3 under AWD. Moreover, AWD increased Pn compared with CI. Hence, AWDN2 treatment provided the highest Pn (Figure 4).
3.5 MDA and soluble protein contents
The MDA content peaked in the maturity stage (Figure 5). It was significantly higher (16.7%–48.2%) in N3 plots compared with N1 and N2 plots in the jointing stage, irrespective of the irrigation treatment. Additionally, CI plots had higher MDA content (increased by 16.8%–32.1%) than AI plots under each N treatment. The MDA content was significantly higher (10.1%–21.9%) in N1 plots compared with N2 and N3 plots in the heading and maturity stages, irrespective of the irrigation regimes. Moreover, CI increased the MDA content compared with AWD. Hence, AWDN2 and AWDN3 treatments led to the lowest MDA content, whereas CIN1 treatment led to the highest MDA content (Figure 5).
The soluble protein content under all treatments was the highest in the heading stage (Figure 6). The soluble protein content was significantly higher (13.7%–28.6%) in N1 and N2 plots compared with N3 plots in the jointing stage under the two irrigation regimes. Additionally, AWD plots had higher soluble protein content (increased by 11.1%–32.0%) than CI plots at each N rate. The soluble protein content was significantly higher in N2 and N3 plots (increased by 9.8%–25.4%) compared with N1 plots in the heading and maturity stages, irrespective of the irrigation treatment. Moreover, AWD increased the soluble protein content by 13.1%–32.8% compared with CI. Hence, AWDN2 and AWDN3 treatments yielded the highest soluble protein content, whereas CIN1 treatments yielded the lowest soluble protein content (Figure 6).
3.6 Economic benefits
The fertilizer cost was the highest in the N3 plot, followed by the N2 plot, and the lowest in N1 in the 2 years (Table 4). AWD significantly lowered labor costs (reduced by 17.3%–24.5%) compared with CI under each N treatment. Additionally, N1 increased the frequency of N fertilization (up to three times), and thus labor cost (increased by 6.7%–8.1%), compared with N2 and N3 under AWD and CI. For the CI plots, the rice field experienced irrigation events seven times in the 2 years, and the total amount of irrigation was 430 mm in 2020 and 414 mm in 2021. For the AWD plots, the total amount of irrigation was 275 mm in 2020 (irrigation four times) and 249 mm in 2021 (irrigation three times). As a result, AWD lowered water costs (reduced by 36.0%–39.8%) compared with CI. AWD significantly increased net income compared with CI under each N treatment. N2 and N3 had a higher net income than N1 under AWD and CI; N2 increased the net income compared with N3 under AWD. Consequently, AWDN2 treatment achieved the highest net income (13,907.1 CNY ha−1 in 2020 and 14,085.7 CNY ha−1 in 2021), whereas CIN1 treatment resulted in the lowest total income (Table 4).
3.7 Grain yield
AWD significantly increased grain yield compared with CI, irrespective of N treatments (Figure 7). N1 resulted in the lowest grain yield under the two irrigation regimes. Also, N2 rather than N3 provided a higher grain yield under AWD. However, the CIN1 treatment resulted in a low yield (Figure 7).
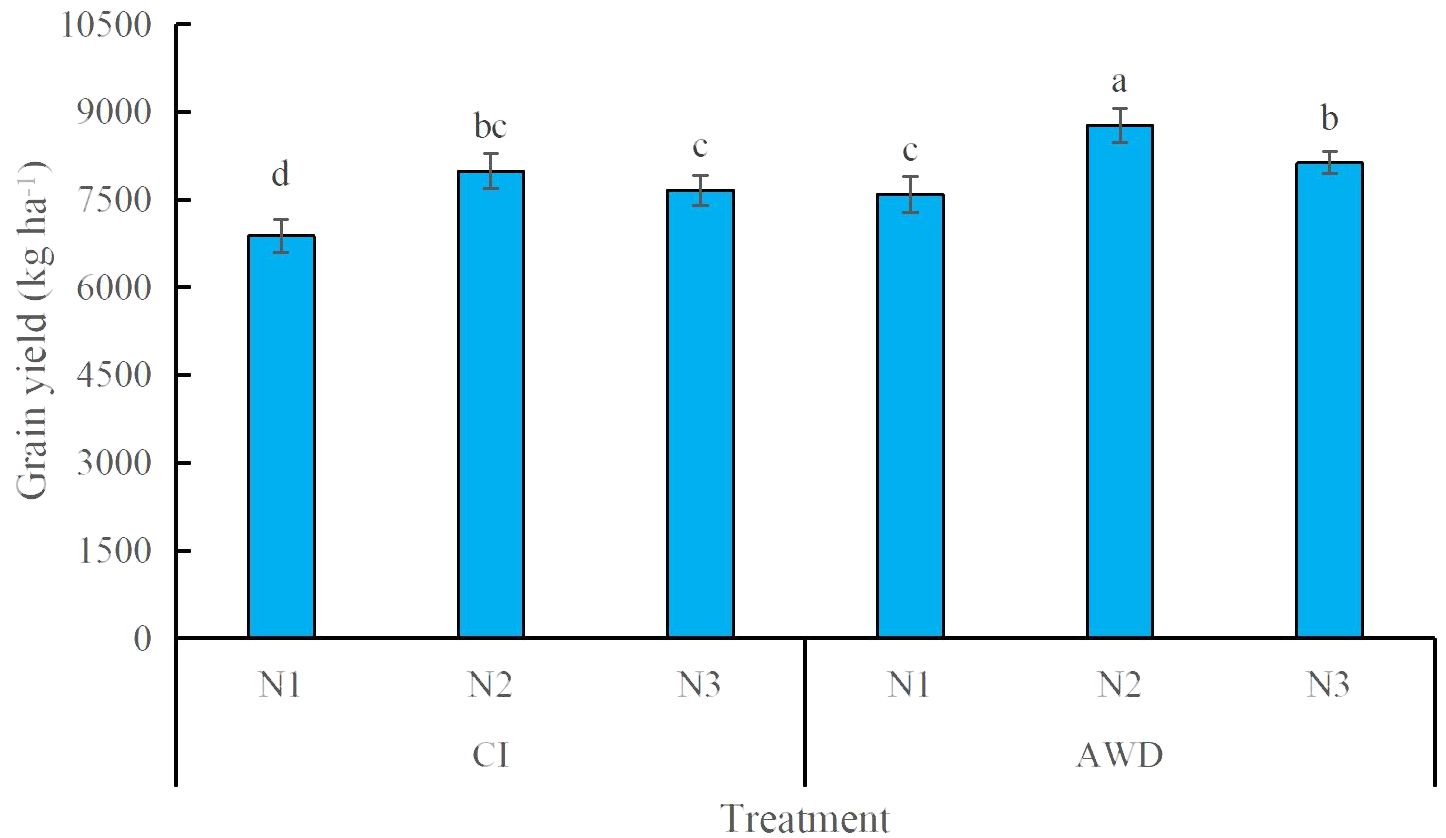
Figure 7. Grain yield of rice leaves affected by different water and nitrogen management strategies. CI and AWD represent conventional flooding irrigation and alternate wetting and moderate drying irrigation, respectively. N1, N2, and N3 represent 100% CU, 60% CRNF + 40% CU, and 100% CRNF at an equivalent N rate of 240 kg ha−1, respectively. Values (mean ± standard error, n = 6) are mean of 2 years and three replicates. Means by different letters are significantly different at p < 0.05.
4 Discussion
The effect of AWD, CRNF, and their interaction on rice yield and resource use efficiency has been evaluated previously (Ye et al., 2013; Li et al., 2018; Zhang et al., 2021; Yan et al., 2022; Fu et al., 2024). Moreover, it has been shown that AWD combined with the mixed application of CRNF and CU can improve grain yield, WUE, and NUE of rice (Zhang et al., 2021). Nevertheless, the physiological mechanism underlying this effect remains largely unknown. Also, the combined impact of AWD and the blended application of CRNF and CU on economic benefits in rice is unclear. This study found that the irrigation regimes and the N fertilizer compounding modes worked together to affect Pn, LAI, and SPAD value; activities of SOD, POD, CAT, NR, GS, and GOGAT; and contents of MDA and soluble protein in rice leaves. Moreover, they influenced the total and net income in rice. Obviously, AWD interacted with N2 or N3 to provide a positive synergistic interaction for delaying leaf senescence compared with N1 under the same watering regime. Moreover, the results indicated that AWD combined with N2 could delay leaf senescence by improving photosynthesis, antioxidant defense system, osmoregulation, and N assimilation, contributing to high grain yield and net income in rice.
4.1 Effects of AWD and N management strategies on the leaf senescence characteristics of rice
Leaf senescence is characterized by a reduction in the LAI and SPAD values. Chlorophyll is a light-harvesting molecule, and its content (SPAD value) indicates the potential of the plants to yield photosynthate by absorbing light energy (Ren et al., 2023). The LAI, which signifies the photosynthetic capacity of leaves, directly influences dry matter accumulation and yield formation (Gitelson et al., 2014). In the present study, AWD resulted in a higher LAI (Figure 2) and SPAD value in the heading, filling, and maturity stages (Figure 3), suggesting that alternate wetting and drying irrigation can sustain normal functioning of leaves in rice in the post-growth stages. Three possible scenarios may account for this phenomenon. First, AWD enhanced gas exchange between soil and atmosphere due to wetting and drying cycles, creating an oxygen-rich area around the rhizosphere. This expedited the mineralization of soil organic matter and restrained the immobilization of soil N (Cao et al., 2022). Second, AWD is known to improve the morphological and physiological characteristics of roots in rice (Xu et al., 2018; Zhang et al., 2021), enhancing the vitality of roots to absorb soil water and nutrients (Wang et al., 2016). This leads to high relative water content in leaves (Yang et al., 2022) and active N metabolism (Yan et al., 2022), corresponding to the enhanced Pn (Figure 4) and activities of NR, GS, and GOGAT (Table 4). Third, AWD decreased MDA content (Figure 5) and increased the activities of SOD, POD, and CAT (Table 4), suggesting better ROS scavenging in AWD-treated plants. Also, N2 and N3 compared with N1 resulted in a greater LAI (Figure 2) and SPAD value in the post-growth stages (Figure 3). This could be related to the discharge characteristics of CRNF as “peak value cutting and valley value filling”. In other words, a continuous and sustained N release occurred throughout the rice-growing season involving CRNF plots (Peng et al., 2014). The enhanced soil N availability promotes the development of a strong and vigorous root system (Ye et al., 2013; Qi et al., 2023b), enhancing water and N utilization (Zhang et al., 2021). CU is rapidly hydrolyzed by urease when applied to the soil (Guo et al., 2021). The excessive decomposition of CU leads to its incomplete utilization by crops. Moreover, CU fertilization (basal application + tillering topdressing) for N1 in this study usually overlapped with the plum rain season (well known for continuous overcast and rains in the middle and lower Yangtze River) (Qi et al., 2020). The heavy rainfall induced enormous N losses even at a moderate N rate (Li et al., 2018; Qi et al., 2023a). This could lead to soil N deficiency in the latter growth stages for N1, lowering LAI and SPAD values. Hence, the mixed application of CRNF and CU (as in N2) increased soil N content in various growth stages (Zhang et al., 2021; Qi et al., 2023a). This was supported by the higher Pn, LAI, and SPAD values and activities of NR, GS, and GOGAT observed in the jointing stage in the N2 plots. In addition, CRNF blended with CU obviously improved soil NO3−–N and NH4+–N contents in the plow layer (0–40 cm) across the rice-growing season (Zheng et al., 2016). This was in line with the results of other studies on the maize leaf senescence characteristics under the mixed application of CRNF and CU (Guo et al., 2021; Yu et al., 2023). AWDN2 and AWDN3 treatments provided the highest LAI and SPAD values in the post-growth stages.
Plant senescence is closely related to the ability to scavenge the ROS (Choudhury et al., 2017). The activities of SOD, POD, and CAT can reflect a plant’s ability to scavenge ROS. CAT and POD metabolize H2O2, whereas SOD catalyzes the disproportionation of O2− to H2O2 (Ahmed et al., 2002). MDA can combine with proteins of the cell membrane structure, inactivating the related proteins; its content can be used to reflect the degree of lipid peroxidation (Biemelt et al., 2000). Compared with CI, AWD had higher SOD, POD, and CAT activities, whereas the MDA content was smaller, suggesting enhanced ROS scavenging ability in alternate wetting and drying irrigation. This was consistent with the previously published findings (Fu et al., 2023, Fu et al., 2024). Notably, the root systems in AWD grow better in oxygen-enriched rhizosphere (Xu et al., 2018), leading to various positive physiological effects based on ABA signaling (Liu et al., 2005). Moreover, appropriate N fertilization is useful for SOD, POD, and CAT activities (Zhang and Li, 2007). Compared with N1, N2 and N3 provided higher SOD, POD, and CAT activities and lower MDA content, suggesting better scavenging in CRNF-treated plants. This was also consistent with the findings of Yu et al. (2023) on maize. CRNF leads to better soil N availability (Zheng et al., 2016), improving root growth and vitality to enhance water and nutrient utilization (Yan et al., 2022). Additionally, optimal N management strategies upregulate antioxidant enzyme activity by enhancing the expression of related genes (Ozcubukcu et al., 2014). Therefore, AWD combined with N2 and N3 resulted in the highest SOD, POD, and CAT activities and lowest MDA contents in rice leaves.
Soluble proteins are involved in various metabolic activities, and hence, a decrease in their levels reflects the degree of leaf senescence. The levels of Pn can be used to reflect the photosynthetic capacity (Li et al., 2020). GS, GOGAT, and NR are the key metabolism-related enzymes involved in the N assimilation of rice plants (Sun et al., 2015; Wang et al., 2016). AWD increased the activities of GS, GOGAT, and NR (Table 4) and soluble protein content (Figure 6) compared with CI, resulting in a higher Pn (Figure 4) in various growth stages. This suggested that alternate wetting and drying irrigation improved the soil aeration conditions and enhanced soil N availability to the root system, producing more assimilates and enhancing the assimilation of inorganic N and photosynthetic capability, thus establishing a solid foundation for higher grain yield (Figure 7). Enhanced Pn was directly associated with higher soluble protein content, LAI, and SPAD values under AWD (Wang et al., 2016). Moreover, a higher LAI was always accompanied by a higher relative water content of leaves (Li et al., 2010). The rise in water status inhibited ABA production in leaves, increasing stomatal conductance (Bahrun et al., 2002). Stomata opening can increase the Pn levels (Kang and Zhang, 2004). Alternately, the root oxidation capacity, absorption area, and cytokinin content were significantly positively correlated with N metabolism-related enzyme activity in rice leaves (Fu et al., 2024). AWD increased rhizosphere oxygen content, resulting in high root oxidation capacity and root cytokinin content (Qi et al., 2023b), thereby upregulating photosynthetic acclimation (Gu et al., 2017). This was supported by higher N accumulation in various growth stages in AWD-treated plants (Supplementary Table S1). The enhanced N uptake in the post-growth stage was accompanied by lasting greenness in plant leaves, providing more metabolites to the roots, thus contributing to vigorous root growth (Qi et al., 2023b) and high water–N absorptive capacity (Xu et al., 2018). In turn, the shoot growth and grain yield were enhanced due to AWD (Wang et al., 2016). Compared with N1, N2 and N3 significantly increased the activities of GS, GOGAT, and NR (Table 4), soluble protein content (Figure 6), and Pn (Figure 4) in the heading and maturity stages, suggesting that CRNF treatments can enhance the assimilation of inorganic N and photosynthetic capability in rice. This established a solid foundation for enhancing grain filling (Sun et al., 2012), leading to high grain yield (Figure 7). The enhanced contents of nitrate N and ammonium N in soil under CRNF treatments accounted for the improvement in plant N nutrition (Guo et al., 2021). Alternatively, a close relationship existed between soil N availability and the activities of key N assimilatory enzymes of rice leaves in the post-growth stage (Yang et al., 2012). In addition, the CRNF promoted the transfer of N from the organs to the grains, increasing grain N content (Wang et al., 2016). Therefore, AWD combined with N2 and N3 improved N metabolism and photosynthetic capacity, achieving a higher N accumulation and grain yield.
4.2 Effects of AWD and N management strategies on the economic benefits of rice
The input cost and yield benefits are two important factors determining the economic efficiency of rice. Grain yield is significantly and positively correlated with total income. The application of N fertilizer and irrigation regime are conjunct to influence crop yield and thus the economic performance (Yuan et al., 2022). In this study, N1 resulted in the lowest grain yield and, thus, low total income. Although N2 and N3 led to higher total income, the higher total income from N3 was partially offset by higher fertilizer costs. The highest net income in the 2 years was obtained under AWDN2 treatment (13,907.1 CNY ha−1 in 2020 and 14,085.7 CNY ha−1 in 2021). The reduced water cost (irrigation amount) and labor cost (irrigation frequencies) accounted for the enhanced net income. Furthermore, AWDN2 resulted in smaller N loss via runoff and leaching (Qi et al., 2023a). Thus, alternate wetting and drying irrigation combined with the mixed application of CRNF and CU is recommended for delaying leaf senescence while realizing considerable ecological and economic benefits. However, the impacts of different ratios of CRNF and CU on leaf senescence remain unclear. Also, the findings of this study should be validated in other regions with different weather conditions. The mechanisms underlying delayed leaf senescence under water and N coupling should be clarified from the perspective of rhizosphere microorganisms in the future.
5 Conclusions
AWD interaction with CRNF or mixed application of CRNF and CU delayed leaf senescence by improving photosynthesis, antioxidant defense system, osmoregulation, and N assimilation, thus achieving higher grain yield. Moreover, AWD reduced water costs by decreasing both the amount and frequency of irrigation. Also, the mixed application of CRNF and CU lowered fertilization costs compared with using 100% CRNF alone. Thus, AWD combined with the mixed application of CRNF and CU not only delayed leaf senescence but also improved the net income of rice. Thus, the present study provided the theoretical basis to sustain the beneficial effects of the blended use of CRNF and CU on rice growth and resource utilization under AWD.
Data availability statement
The original contributions presented in the study are included in the article/Supplementary Material. Further inquiries can be directed to the corresponding author.
Author contributions
DQ: Funding acquisition, Writing – original draft. SC: Data curation, Investigation, Writing – original draft. WY: Project administration, Writing – review & editing. YD: Writing – original draft.
Funding
The author(s) declare financial support was received for the research, authorship, and/or publication of this article. This work has been supported by the State Key Laboratory of Water Resources and Hydropower Engineering Science, Wuhan University, China (2020NSG05).
Acknowledgments
The authors would like to thank all the reviewers who participated in the review, as well as MJEditor (www.mjeditor.com) for providing English editing services during the preparation of this manuscript. The authors are also thankful to Ms. Chen Pan and Mr. Yu Yang for providing assistance in field investigation and nitrogen content measurements.
Conflict of interest
The authors declare that the research was conducted in the absence of any commercial or financial relationships that could be construed as a potential conflict of interest.
Publisher’s note
All claims expressed in this article are solely those of the authors and do not necessarily represent those of their affiliated organizations, or those of the publisher, the editors and the reviewers. Any product that may be evaluated in this article, or claim that may be made by its manufacturer, is not guaranteed or endorsed by the publisher.
Supplementary material
The Supplementary Material for this article can be found online at: https://www.frontiersin.org/articles/10.3389/fpls.2024.1444819/full#supplementary-material
Supplementary Table 1 | Nitrogen (N) uptake by rice (kg ha-1) as affected by different water and nitrogen management strategies. CI and AWD represent conventional flooding irrigation and alternate wetting and moderate drying irrigation, respectively. N1, N2, and N3 represent 100% CU, 60%CRNF + 40% CU, and 100% CRNF at an equivalent N rate of 240 kg ha−1, respectively. Values are mean of 2 years and three replicates. Means within a column followed by different letters are significantly different at P< 0.05.
Abbreviations
AWD, alternate wetting and drying irrigation; CI, conventional flood irrigation; CRNF, controlled-release nitrogen fertilizer; CU, common urea; Pn, net photosynthetic rate; LAI, leaf area index; SOD, superoxide dismutase; POD, peroxidases; CAT, catalase; GS, glutamine synthetase; GOGAT, glutamine 2-oxoglutarate amidotransferase; NR, nitrate reductase; MDA, malondialdehyde; NUE, nitrogen use efficiency; WUE, water use efficiency; SWP, soil water potential.
References
Ahmed, S., Nawata, E., Hosokawa, M., Domae, Y., Sakuratani, T. (2002). Alterations in photosynthesis and some antioxidant enzymatic activities of mungbean subjected to waterlogging. Plant Sci. 163, 117–123. doi: 10.1016/S0168-9452(02)00080-8
Aslam, M., Travis, R. L., Rains, D. W. (2001). Enhancement of nitrate reductase activity and metabolic nitrate concentration by methionine sulfoximine in barley roots. Plant Sci. 161, 133–142. doi: 10.1016/S0168-9452(01)00396-X
Bahrun, A., Jensen, C. R., Asch, F., Mogensen, V. O. (2002). Drought-induced changes in xylem pH, ionic composition, and ABA concentration act as early signals in field-grown maize (Zea mays L.). J. Exp. Bot. 53, 251–263. doi: 10.1093/jexbot/53.367.251
Belder, P., Bouman, B. A. M., Cabangon, R. J., Lu, G., Quilang, E. J. P., Li, Y., et al. (2004). Effect of water-saving irrigation on rice yield and water use in typical lowland conditions in Asia. Agric. Water Manage. 65, 193–210. doi: 10.1016/j.agwat.2003.09.002
Berkhout, E., Glover, D., Kuyvenhoven, A. (2015). On-farm impact of the system of Rice intensification (SRI): Evidence and knowledge gaps. Agric. Sys. 132, 157–166. doi: 10.1016/j.agsy.2014.10.001
Biemelt, S., Keetman, U., Mock, H. P., Grimm, B. (2000). Expression and activity of isoenzymes of superoxide dismutase in wheat roots in response to hypoxia and anoxia. Plant Cell Environ. 23, 135–144. doi: 10.1046/j.1365-3040.2000.00542.x
Bohnert, H. J., Jensen, R. G. (1996). Strategies for engineering water-stress tolerance in plants. Trends Biotechnol. 14, 89–97. doi: 10.1016/0167-7799(96)80929-2
Borrell, A., Garside, A., Fukai, S. (1997). Improving efficiency of water use for irrigated rice in a semi-arid tropical environment. Field Crop Res. 52, 231–248. doi: 10.1016/S0378-4290(97)00033-6
Bueno, C. S., Bucourt, M., Kobayashi, N., Inubushi, K., Lafarge, T. (2010). Water productivity of contrasting rice genotypes grown under water-saving conditions in the tropics and investigation of morphological traits for adaptation. Agri.Water Manage. 98, 241–250. doi: 10.1016/j.agwat.2010.08.015
Cao, X. C., Zhang, J. H., Yu, Y. J., Ma, Q. X., Kong, Y. L., Pan, W. K., et al. (2022). Alternate wetting–drying enhances soil nitrogen availability by altering organic nitrogen partitioning in rice-microbe system. Geoderma 424, 115993. doi: 10.1016/j.geoderma.2022.115993
Chiba, M., Terao, T., Watanabe, H., Matsumura, O., Takahashi, Y. (2017). Improvement in Rice grain quality by deep-flood irrigation and its underlying mechanisms. Japan Agric.Res. Quart 51, 107–116. doi: 10.6090/jarq.51.107
Choudhury, F. K., Rivero, R. M., Blumwald, E., Mittler, R. (2017). Reactive oxygen species, abiotic stress and stress combination. Plant J. 90, 856–867. doi: 10.1111/tpj.2017.90.issue-5
Ding, W., Xu, X., He, P., Ullah, S., Zhang, J., Cui, Z., et al. (2018). Improving yield and nitrogen use efficiency through alternative fertilization options for rice in China: a meta-analysis. Field Crop Res. 227, 11–18. doi: 10.1016/j.fcr.2018.08.001
Du, Z. Y., Bramlage, W. J. (1992). Modified thiobarbituric acid assay for measuring lipid oxidation in sugar-rich plant tissue extracts. J. Agric. Food Chem. 40, 1566–1570. doi: 10.1021/jf00021a018
Fertitta-Roberts, C., Oikawa, P. Y., Jenerette, G. D. (2019). Evaluating the GHG mitigation potential of alternate wetting and drying in rice through life cycle assessment. Sci. Total Environ. 653, 1343–1353. doi: 10.1016/j.scitotenv.2018.10.327
Foyer, C. H., Noctor, G. (2000). Oxygen processing in photosynthesis: regulation and signalling. New Phytol. 146, 359–388. doi: 10.1046/j.1469-8137.2000.00667.x
Fu, J., Ma, M. J., Zhang, Q. F., Duan, J. Q., Wang, Y. T., Wang, F. H., et al. (2024). Effects of alternate wetting and drying irrigation and different nitrogen application levels on photosynthetic characteristics and nitrogen absorption and utilization of japonica rice. Acta Agron.Sin. 50, 1787–1804. doi: 10.3724/SP.J.1006.2024.32056
Fu, J., Wang, Y., Yang, W. B., Wang, Y. T., Li, B. Y., Wang, F. H., et al. (2023). Effects of alternate wetting and drying irrigation and nitrogen coupling on grain filling physiology and root physiology in rice. Acta Agron. Sin. 49, 808–820. doi: 10.3724/SP.J.1006.2023.22032
Gaihre, Y. K., Singh, U., Islam, S. M., Huda, A., Islam, M. R., Satter, M. A., et al. (2015). Impacts of urea deep placement on nitrous oxide and nitric oxide emissions from rice fields in Bangladesh. Geoderma. 259-260, 370–379. doi: 10.1016/j.geoderma.2015.06.001
Gitelson, A., Peng, Y., Arkebauer, T., Schepers, J. (2014). Relationships between gross primary production,green LAI, and canopy chlorophyll content in maize: implications for remote sensing of primary production. Remote Sens Environ. 144, 65–72. doi: 10.1016/j.rse.2014.01.004
Gu, J. F., Chen, Y., Zhang, H., Li, Z. K., Zhou, Q., Yu, C., et al. (2017). Canopy light and nitrogen distributions are related to grain yield and nitrogen use efficiency in rice. Field Crop Res. 206, 74–85. doi: 10.1016/j.fcr.2017.02.021
Guo, J. J., Fan, J. L., Zhang, F. C., Yan, S. C., Zheng, J., Wu, Y., et al. (2021). Blending urea and slow release nitrogen fertilizer increases dryland maize yield and nitrogen use efficiency while mitigating ammonia volatilization. Sci. Total Environ. 790, 148058. doi: 10.1016/j.scitotenv.2021.148058
Han, K., Yang, Y., Zhou, C. J., Shangguan, Y. X., Zhang, L., Li, N., et al. (2014). Management of furrow irrigation and nitrogen application on summer maize. Agron. J. 106, 1402–1410. doi: 10.2134/agronj13.0367
Hooper, P., Zhou, Y., Coventry, D. R., McDonald, G. K. (2014). Use of nitrogen fertilizer in a targeted way to improve grain yield, quality, and nitrogen use efciency. Agron. J. 107, 903–915. doi: 10.2134/agronj14.0363
Ishfaq, M., Farooq, M., Zulfiqar, U., Hussain, S., Akbar, N., Nawaz, A., et al. (2020). Alternate wetting and drying: a water-saving and ecofriendly rice production system. Agric. Water Manage. 241, 106363. doi: 10.1016/j.agwat.2020.106363
Islam, S. M., Gaihre, Y. K., Islam, M. R., Ahmed, M. N., Akter, M., Singh, U., et al. (2022). Mitigating greenhouse gas emissions from irrigated rice cultivation through improved fertilizer and water management. J. Environ. Manage. 307, 114520. doi: 10.1016/j.jenvman.2022.114520
Ju, X. T., Xing, G. X., Chen, X. P., Zhang, S., Zhang, L., Liu, X., et al. (2009). Reducing environmental risk by improving N management in intensive Chinese agricultural systems. PANS 106, 3041–3046. doi: 10.1073/pnas.0813417106
Kang, S. Z., Zhang, J. H. (2004). Controlled alternate partial root zone irrigation: its:physiological consequences and impact on water use efficiency. J. Exp. Bot. 55, 2437–2446. doi: 10.1093/jxb/erh249
Lai, Z., Fan, J., Yang, R., Xu, X., Liu, L., Li, S., et al. (2022). Interactive effects of plant density and nitrogen rate on grain yield, economic benefit, water productivity and nitrogen use efficiency of drip-fertigated maize in northwest China. Agric. Water Manage. 263, 107453. doi: 10.1016/j.agwat.2021.107453
Lampayan, R. M., Rejesus, R. M., Singleton, G. R., Bouman, B. A. (2015). Adoption and economics of alternate wetting and drying water management for irrigated lowland rice. Field Crop Res. 170, 95–108. doi: 10.1016/j.fcr.2014.10.013
Lea, P. J., Blackwell, R. D., Chen, F. L. (1990). “Enzymes of primary metabolism,” in Methods in Plant Biochemistry. Ed. Harborne, J. B. (Academic Press, New York, NY), 257–276.
Li, G. H., Fu, P. X., Cheng, G. G., Lu, W. P., Lu, D. L. (2022). Delaying application time of slow-release fertilizer increases soil rhizosphere nitrogen content, root activity, and grain yield of spring maize. Crop J. 10, 1798–1806. doi: 10.1016/j.cj.2022.04.014
Li, P., Lu, J., Wang, Y., Wang, S., Hussain, S., Ren, T., et al. (2018). Nitrogen losses,use efficiency, and productivity of early rice under controlled-release urea. Agric. Ecosys. Environ. 251, 78–87. doi: 10.1016/j.agee.2017.09.020
Li, F. S., Wei, C. H., Zhang, F. C., Zhang, J. H., Nong, M. L., Kang, S. Z. (2010). Wateruse efficiency and physiological responses of maize under partial root-zone irrigation. Agric. Water Manage. 97, 1156–1164. doi: 10.1016/j.agwat.2010.01.024
Li, G. H., Zhao, B., Dong, S. T., Zhang, J. W., Liu, P., Lu, W. P. (2020). Controlled-release urea combining with optimal irrigation improved grain yield, nitrogen uptake, and growth of maize. Agric. Water Manage. 227, 105834. doi: 10.1016/j.agwat.2019.105834
Liang, K. M., Zhong, X. H., Fu, Y. Q., Hu, X. Y., Li, M. J., Pan, J. F., et al. (2023). Mitigation of environmental N pollution and greenhouse gas emission from double rice cropping system with a new alternate wetting and drying irrigation regime coupled with optimized N fertilization in South China. Agric. Water Manage. 282, 108282. doi: 10.1016/j.agwat.2023.108282
Liu, L. J., Chen, T. T., Wang, Z. Q., Zhang, H., Yang, J. C., Zhang, J. H. (2013). Combination of site-specifc nitrogen management and alternate wetting and drying irrigation increases grain yield and nitrogen and water use efciency in super rice. Field Crop Res. 154, 226–235. doi: 10.1016/j.fcr.2013.08.016
Liu, F. L., Jensen, C. R., Shahnazari, A., Andersen, M. N., Jacobsen, S. E. (2005). ABA regulated stomatal control and photosynthetic water use efficiency of potato (Solanum tuberosum L.) during progressive soil drying. Plant Sci. 168, 831–836. doi: 10.1016/j.plantsci.2004.10.016
Ly, P., Jensen, L. S., Bruun, T. B., De Neergaard, A. (2016). Factors explaining variability in rice yields in a rain-fed lowland rice ecosystem in southern Cambodia. Netherlands J. Agric. Sci. 78, 129–137. doi: 10.1016/j.njas.2016.05.003
Mboyerwa, P. A., Mtakwa, P. W., Kibret, K., Aschalew, A., Uphoff, N. T. (2021). Evaluation of growth, yield, and water productivity of Paddy Rice with water-saving irrigation and optimization of nitrogen fertilization. Agronomy 11, 1629–1629. doi: 10.3390/agronomy11081629
Mohammadkhani, N., Heidari, R. (2007). Effects of drought stress on protective enzyme activities and lipid peroxidation in two maize cultivars. Pak. J. Biol. Sci. 10, 3835–3840. doi: 10.3923/pjbs.2007.3835.3840
National Bureau of Statistics (2022). China statistical yearbook. Available online at: http://www.stats.gov.cn/tjsj/ndsj/ (Accessed March 8, 2022).
Ozcubukcu, S., Ergun, N., Ilhan, E. (2014). Waterlogging and nitric oxide induce gene expression and increase antioxidant enzyme activity in wheat (Triticum aestivum L.). Acta Biol. Hung 65, 47–60. doi: 10.1556/ABiol.65.2014.1.5
Peng, J., Feng, Y., Wang, X., Li, J., Xu, G., Phonenasay, S., et al. (2021). Effects of nitrogen application rate on the photosynthetic pigment, leaf fluorescence characteristics, and yield of indica hybrid rice and their interrelations. Sci. Rep. 11, 7485. doi: 10.1038/s41598-021-86858-z
Peng, Y., Sun, Y. J., Jiang, M. J., Xu, H., Qin, J., Yang, Z. Y., et al. (2014). Effects of water management and slow/controlled release nitrogen fertilizer on biomass and nitrogen accumulation, translocation, and distribution in rice (in Chinese). Acta Agron. Sin. 40, 859–870. doi: 10.3724/SP.J.1006.2014.00859
Qi, D. L., Li, X., Pan, C., Li, J. F., Xu, Y., Zhu, J. Q. (2021). Effect of nitrogen supply methods on the gas exchange, antioxidant enzymatic activities, and osmoregulation of maize (Zea mays L.) under alternate partial root-zone irrigation. J. Soil Sci. Plant Nutri. 21, 2083–2095. doi: 10.1007/s42729-021-00504-1
Qi, D. L., Wu, Q. X., Zhu, J. Q. (2020). Nitrogen and phosphorus losses from paddy fields and the yield of rice with different water and nitrogen management practices. Sci. Rep. 10, 9734. doi: 10.1038/s41598-020-66757-5
Qi, D. L., Zhu, J. Q., Wang, X. G. (2023a). Nitrogen loss via runoff and leaching from paddy fields with the proportion of controlled−release urea and conventional urea rates under alternate wetting and drying irrigation. Environ. Sci. Poll. Res. 30, 61741–61752. doi: 10.1007/s11356-023-26480-w
Qi, D. L., Zhu, J. Q., Wang, X. G. (2023b). Root growth in rice (Liangyou 152) under alternate wetting and drying irrigation and mixed application of polymer-coated and common urea. J. Soil Sci. Plant Nutri. 23, 6838–6850. doi: 10.1007/s42729-023-01546-3
Ramasamy, S., ten Berge, H. F. M., Purushothaman, S. (1997). Yield formation in rice in response to drainage and nitrogen application. Field Crop Res. 51, 65–82. doi: 10.1016/S0378-4290(96)01039-8
Ren, B. Z., Yu, W. Z., Liu, P., Zhao, B., Zhang, J. W. (2023). Responses of photosynthetic characteristics and leaf senescence in summer maize to simultaneous stresses of waterlogging and shading. Crop J. 11, 269–277. doi: 10.1016/j.cj.2022.06.003
Ren, B. Z., Zhang, J. W., Dong, S. T., Liu, P., Zhao, B. (2018). Responses of carbon metabolism and antioxidant system of summer maize to waterlogging at different stages. J. Agron. Crop Sci. 00, 1–10. doi: 10.1111/jac.2018.204.issue-5
Shaviv, A. (2001). Advances in controlled-release fertilizers. Adv. Agron. 71, 1–49. doi: 10.1016/S0065-2113(01)71011-5
Singh, R. D., Srivastava, H. S. (1986). Increase in glutamate synthase (NADH) activity in maize seedlings in response to nitrate and ammonium nitrogen. Phys. Plant 66, 413–416. doi: 10.1111/j.1399-3054.1986.tb05944.x
Song, C., Guan, Y., Wang, D., Zewudie, D., Li, F. M. (2014). Palygorskitecoated fertilizers with a timely release of nutrients increase potato productivity in a rain-fed cropland. Field Crop Res. 166, 10–17. doi: 10.1016/j.fcr.2014.06.015
Spiertz, J. H. J. (2010). Nitrogen, sustainable agriculture and food security: A review. Agron. Sustain Dev. 30, 43–55. doi: 10.1007/978-90-481-2666-839
Su, W. Q., Cang, B. F., Ullah, R., Song, Z., Cui, J. J., Li, Y. Z., et al. (2023). Interaction of the coupled effects of irrigation mode and nitrogen fertilizer timing on rice yield in different regions. Irrig. Drain. 72, 999–1012. doi: 10.1002/ird.v72.4
Sun, H., Li, J., Song, W., Tao, J., Huang, S., Chen, S., et al. (2015). Nitric oxide generated by nitrate reductase increases nitrogen uptake capacity by inducing lateral root formation and inorganic nitrogen uptake under partial nitrate nutrition in rice. J. Exp. Bot. 66, 2449–2459. doi: 10.1093/jxb/erv030
Sun, Y., Ma, J., Sun, Y., Xu, H., Yang, Z., Liu, S., et al. (2012). The effects of different water and nitrogen managements on yield and nitrogen use efficiency in hybrid rice of China. Field Crop Res. 127, 85–98. doi: 10.1016/j.fcr.2011.11.015
Sun, Y., Mi, W., Su, L., Shan, Y., Wu, L. (2019). Controlled-release fertilizer enhances rice grain yield and N recovery efficiency in continuous non-flooding plastic film mulching cultivation system. Field Crop Res. 231, 122–129. doi: 10.1016/j.fcr.2018.11.013
Wang, L., Xue, C., Pan, X., Chen, F., Liu, Y. (2018). Application of controlled-release urea enhances grain yield and nitrogen use efficiency in irrigated rice in the Yangtze River Basin, China. Front. Plant Sci. 9, 999. doi: 10.3389/fpls.2018.00999
Wang, Z. Q., Zhang, W. Y., Beebout, S. S., Zhang, H., Liu, L. J., Yang, J. C., et al. (2016). Grain yield, water and nitrogen use efciencies of rice as influenced by irrigation regimes and their interaction with nitrogen rates. Field Crop Res. 193, 54–69. doi: 10.1016/j.fcr.2016.03.006
Xu, G. W., Lu, D. K., Wang, H. Z., Li, Y. J. (2018). Morphological and physiological traits of rice roots and their relationships to yield and nitrogen utilization as influenced by irrigation regime and nitrogen rate. Agric. Water Manage. 203, 385–394. doi: 10.1016/j.agwat.2018.02.033
Yan, J., Wu, Q. X., Qi, D. L., Zhu, J. Q. (2022). Rice yield, water productivity, and nitrogen use efficiency responses to nitrogen management strategies under supplementary irrigation for rain-fed rice cultivation. Agric. Water Manage. 263, 107486. doi: 10.1016/j.agwat.2022.107486
Yang, Z. Y., Li, N., Ma, P., Li, Y., Zhang, R. P., Song, Q., et al. (2022). Improving nitrogen and water use efficiencies of hybrid rice through methodical nitrogen–water distribution management. Field Crop Res. 246, 107698. doi: 10.1016/j.fcr.2019.10769
Yang, Y. C., Zhang, M., Li, Y. C., Fan, X. H., Geng, Y. Q. (2012). Controlled release urea improved nitrogen use efficiency, activities of leaf enzymes, and rice yield. Soil Sci. Soc Am. J. 76, 2307–2317. doi: 10.2136/sssaj2012.0173
Ye, Y., Liang, X., Chen, Y., Liu, J., Gu, J., Guo, R., et al. (2013). Alternate wetting and drying irrigation and controlled-release nitrogen fertilizer in late-season rice. Effects on dry matter accumulation, yield, water and nitrogen use. Field Crop Res. 144, 212–244. doi: 10.1016/j.fcr.2012.12.003
Yu, H. D., Chu, Z. Y., Wang, S. Y., Guo, Y. Q., Ren, B. Z., Zhang, J. W. (2023). Effects of different controlled nitrogen ratios on leaf senescence and grain filling characteristics of summer maize. Sci. Agric.Sin.a 56, 3511–3529. doi: 10.3864/j.issn.0578-1752.2023.18.003
Yuan, Y., Lin, F., Maucieri, C., Zhang, Y. J. (2022). Efficient irrigation methods and optimal nitrogen dose to enhance wheat yield, inputs efficiency and economic benefits in the North China Plain. Agron. 12, 273. doi: 10.3390/agronomy12020273
Zhang, L. X., Li, S. X. (2007). Effects of nitrogen, potassium and glycinebetaine on the lipid peroxidation and protective enzyme activities in water-stressed summer maize. Acta Agron. Sin. 33, 482–490.
Zhang, Y. J., Ren, W. C., Zhu, K. Y., FU, J. Y., Wang, W. L., Wang, Z. Q., et al. (2024). Substituting readily available nitrogen fertilizer with controlled-release nitrogen fertilizer improves crop yield and nitrogen uptake while mitigating environmental risks: A global meta-analysis. Field Crop Res. 306, 109221. doi: 10.1016/j.fcr.2023.109221
Zhang, W. Y., Xu, Y. J., Wang, Z. Q., Liu, L. J., Zhang, H., Gu, J. F., et al. (2021). Alternate wetting and drying irrigation combined with the proportion of polymer-coated urea and conventional urea rates increases grain yield, water and nitrogen use efficiencies in rice. Field Crop Res. 268, 108165. doi: 10.1016/j.fcr.2021.108165
Zheng, W. K., Zhang, M., Liu, Z. G., Zhou, H. Y., Lu, H., Zhang, W. T., et al. (2016). Combining controlled-release urea and normal urea to improve the nitrogen use efficiency and yield under wheat-maize double cropping system. Field Crop Res. 197, 52–62. doi: 10.1016/j.fcr.2016.08.004
Zhou, J. J., Tang, S., Pan, W. K., Xu, M., Liu, X., Ni, L., et al. (2023). Long-term application of controlled-release fertilizer enhances rice production and soil quality under non-flooded plastic film mulching cultivation conditions. Agric. Ecos. Environ. 358, 108720. doi: 10.1016/j.agee.2023.108720
Keywords: economic benefits, high-efficiency N fertilizer, leaf senescence, rice, water-saving irrigation
Citation: Qi D, Chen S, Yue W and Duan Y (2024) Leaf senescence characteristics and economic benefits of rice under alternate wetting and drying irrigation and blended use of polymer-coated and common urea. Front. Plant Sci. 15:1444819. doi: 10.3389/fpls.2024.1444819
Received: 06 June 2024; Accepted: 27 November 2024;
Published: 24 December 2024.
Edited by:
Mohammad Mofizur Rahman Jahangir, Bangladesh Agricultural University, BangladeshReviewed by:
Jiangwen Nie, Yangtze University, ChinaFucang Zhang, Northwest A&F University, China
S.M. Mofijul Islam, Bangladesh Rice Research Institute, Bangladesh
Copyright © 2024 Qi, Chen, Yue and Duan. This is an open-access article distributed under the terms of the Creative Commons Attribution License (CC BY). The use, distribution or reproduction in other forums is permitted, provided the original author(s) and the copyright owner(s) are credited and that the original publication in this journal is cited, in accordance with accepted academic practice. No use, distribution or reproduction is permitted which does not comply with these terms.
*Correspondence: Dongliang Qi, cWRsMTk4Nzk5QDEyNi5jb20=