- 1Center for Viticulture and Enology, College of Food Science and Nutritional Engineering, China Agricultural University, Beijing, China
- 2Key Laboratory of Viticulture and Enology, Ministry of Agriculture and Rural Affairs, Beijing, China
Flavonoids, including proanthocyanidins (PAs), anthocyanins and flavonols are essential secondary metabolites that contribute to the nutritional value and sensory quality of grape berry and red wine. Advances in molecular biology technology have led to substantial progress in understanding the regulation of flavonoid biosynthesis. The influence of terroir on grape berries and wine has garnered increasing attention, yet its comprehensive regulatory network remains underexplored. In terms of application, environmental factors such as water, light, and temperature are more easily regulated in grapevines compared to soil conditions. Therefore, we summarize their effects on flavonoid content and composition, constructing a network that links environmental factors, hormones, and metabolites to provide a deeper understanding of the underlying mechanisms. This review enriches the knowledge of the regulatory network mechanisms governing flavonoid responses to environmental factors in grapes.
1 Introduction
Grapevine (Vitis vinifera) is one of the most widely cultivated and consumed fruits in the world, and its use in wine production is attracting more interest due to its economic and health benefits (Beres et al., 2017; Wei et al., 2023). Proanthocyanidins (PAs, polymers of flavan-3-ols), anthocyanins and flavonols are abundant flavonoids in grapes. These compounds not only protect grapes from UV rays, pests and diseases, but also determine the color, astringency, and stability of red wine (Winkel-Shirley, 2002; Flamini et al., 2013). Flavonoids, characterized by a C6-C3-C6 backbone, are classified into anthocyanins, flavonols, and flavan-3-ols in grape depending on the oxidation and substitution state of the pyran ring in the carbon skeleton (Ferreyra et al., 2012; Gouot et al., 2019a). Anthocyanins are primarily located in the skin of grape, accumulating significantly at the veraison stage and peaking around the harvest stage. Flavonols are also mainly distributed in the skin, with their synthesis starting at the flowering stage, slowing down in the early stages of fruit development, and resuming during fruit ripening. Flavan-3-ols are detected in both the grape skin and seeds, and their synthesis occurs from anthesis until the onset of ripening (Downey et al., 2004; Bogs et al., 2005; He et al., 2010).
The flavonoid pathway in grapevine, derived from the shikimate and phenylpropanoid pathways, has been well-characterized (Ferreira et al., 2018; Gouot et al., 2019a). As shown in Figure 1, phenylalanine, a product of shikimate pathway, is catalyzed by a series of enzymes, including phenylalanine ammonia-lyase (PAL), cinnamate 4-hydroxylase (C4H) and 4-coumarate coenzyme A ligase (4CL), to form 4-coumaroyl-CoA, a precursor in the flavonoid pathway. Subsequently, 4-coumaroyl-CoA and malonyl-CoA are catalyzed by chalcone synthase (CHS) and chalcone isomerase (CHI) to form chalcone, a 15-carbon flavonoid skeleton, and then naringenin, an intermediate product of flavonoid metabolism, which enters various flavonoid metabolic branches (Holton and Cornish, 1995). With the catalysis of flavanone hydroxylases (F3H, F3’H or F3’5’H), naringenin is converted to dihydroflavonols including dihydrokaempferol, dihydromyricetin and dihydroquercetin (Bogs et al., 2006). The next stage involves branching: one branch produces flavonols from dihydrokaempferol by flavonol synthase (FLS), while the other, controlled by dihydroflavonol 4-reductase (DFR), converts dihydromyricetin and dihydroquercetin into leucodelphinidin and leucocyanidin (possessing 2,3-trans conformation), respectively (Martens et al., 2002). Leucodelphinidin and leucocyanidin are then converted to delphinidin and cyanidin via the combined activities of anthocyanidin synthase (ANS) and glutathione-S-transferase (GST) (Eichenberger et al., 2023). They also can be catalyzed by leucoanthocyanidin reductase (LAR) producing 2,3-trans flavan-3-ol (e.g. (+)-catechin) (Yu et al., 2019). Additionally, the delphinidin and cyanidin are converted to 2,3-cis flavan-3-ol (e.g. (-)-epicatechin) with the catalysis of anthocyanidin reductase (ANR) (Xie et al., 2003; Bogs et al., 2005; Jun et al., 2021; Yu et al., 2022). These flavan-3-ol monomers participate in the condensation process of PA as starter units (Dixon et al., 2005; Yu et al., 2023). Flavan-3-ol carbocations, derived either from 2,3-trans-leucoanthocyanidins or from 2,3-cis-leucoanthocyanidins produced by ANR, serve as the direct extension units that form C4-C8 or C4-C6 bonds with the upper unit in non-enzymatic PA polymerization (Wang et al., 2020a; Jun et al., 2018, Jun et al., 2021; Yu et al., 2022, Yu et al., 2023). For another branch, anthocyanidins can further undergo modifications such as glycosylation, acylation, and methylation mediated by UDP-glucose (UFGT), acyltransferases and O-methyltransferase (OMT) respectively, to produce anthocyanins (He et al., 2008). Finally, flavonoids synthesized on the endoplasmic reticulum are typically transported by transporter proteins, including GST and multidrug and toxic extrusion transporter (MATE), to vesicles for storage (Castellarin et al., 2007a).
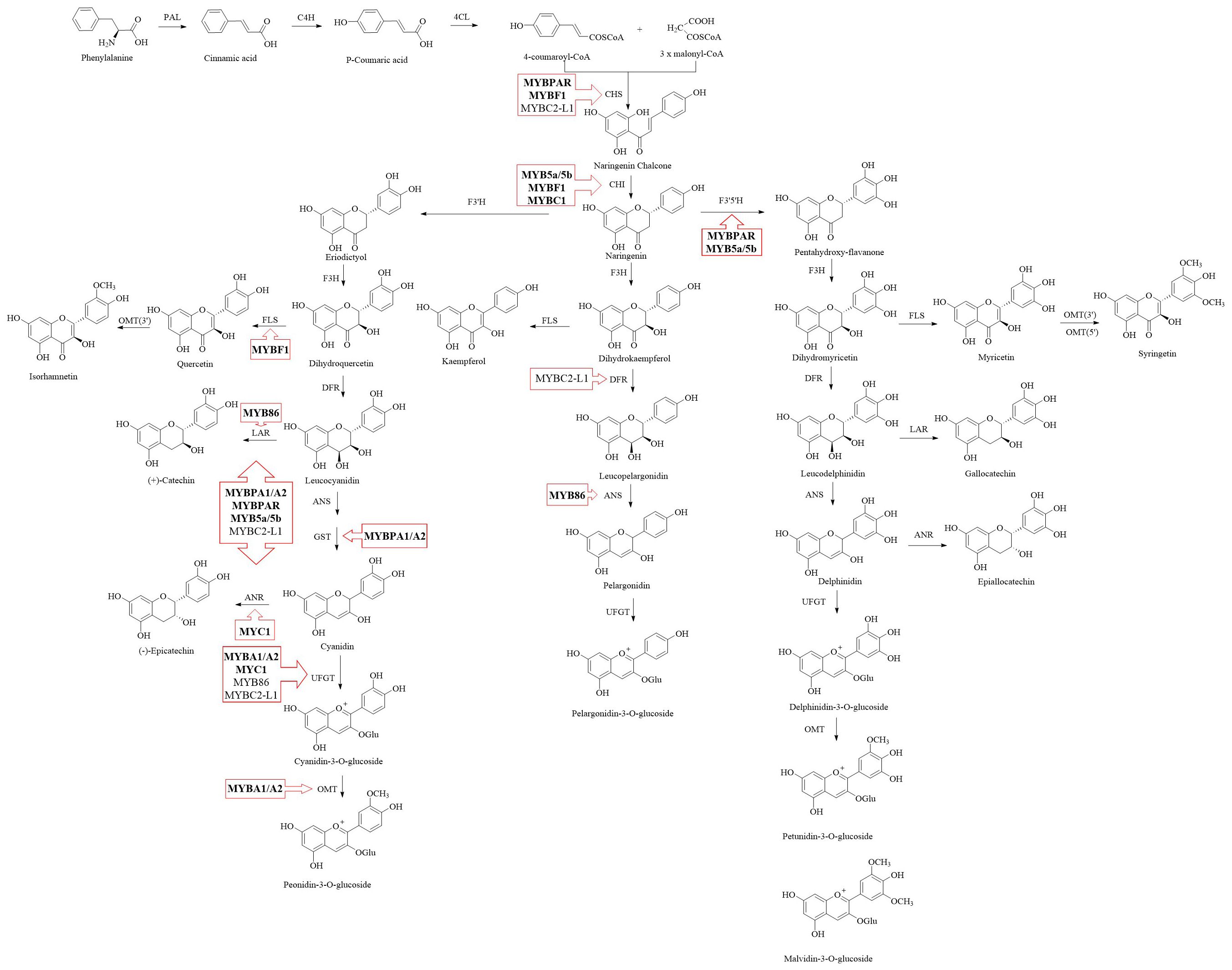
Figure 1. The metabolic pathway of flavonoid in grape. Positive regulators are indicated in bold text, while negative regulators are shown in normal text. PAL, phenylalanine ammonia-lyase; C4H, cinnamate-4-hydroxylase; 4CL, 4-coumarate, CoA-ligase; CHS, chalcone synthase; CHI, chalcone isomerase; F3H, flavanone 3-hydroxylase; F3’H, flavanone 3’-hydroxylase; F3’5’H, flavanone 3’5’-hydroxylase; DFR, dihydroflavonol 4-reductase; ANR, anthocyanidin reductase; ANS, anthocyanidin synthase; FLS, flavonol synthase; UFGT, UDP-glucose, flavonoid 3-O-glucosyltransferase; OMT, O-methyltransferase.
It is believed that flavonoid synthesis is mainly controlled by the MYB-bHLH-WD40 (MBW) complex, with the MYB protein playing a central role (Xu et al., 2015). Among the R2R3-MYB transcription factors, several of them have been identified in grapevine for their roles in flavonoid metabolism. VvMYB5a, expressed in the early stages of berry development, and VvMYB5b, expressed throughout berry development, activate the promoters of upstream flavonoid pathway genes (VvCHI, VvF3’5’H) and the PA biosynthesis pathway-related structural genes including VvLAR1 and VvANR (Deluc et al., 2006, Deluc et al., 2008). Overexpression of VvMYB5a and VvMYB5b in tobacco (Nicotiana tabacum) leads to high accumulation of anthocyanins and PAs via upregulating the expression of genes involved in the flavonoid biosynthetic pathway, such as VvCHS, VvCHI, VvF3H, and VvDFR. Moreover, VvCHS and VvCHI can also be regulated by VvMYBF1, but VvMYBF1 mainly regulates VvFLS to produce flavonols, which compete with PAs and anthocyanins synthesis (Czemmel et al., 2009). VvMYBA1 and VvMYBA2 are the key positive regulators of anthocyanin synthesis in grape berries, promoting the expression of VvUFGT, VvGST, VvOMT and Vv3AT genes at the pre-veraison. stage (Kobayashi et al., 2004; Cutanda-Perez et al., 2009; Rinaldo et al., 2015). Matus et al. (2017) reported that VvMYBA1, VvMYBA6.1 and VvMYBA7 regulate the synthesis of anthocyanins in young leaves and tendrils of ‘Pinot Noir’ and buds of ‘Corvina Veronese’ by activating the promoter of VvUFGT, VvOMT and VvF3’5’H genes, with only VvMYBA1 inducing VvF3’5’H. VvMYBPA1 and VvMYBPA2, mainly expressed in seeds and skin in grapevine respectively, result in the accumulation of PAs by significantly upregulating transcript levels of VvANR and VvLAR1 (Bogs et al., 2006; Terrier et al., 2009). Koyama et al. (2014) suggested that VvMYBPAR also regulate PAs content via controlling VvLAR2 expression. Additionally, there are transcription repressors involved in flavonoid synthesis. VvMYBC2-L1/2/3 negatively regulate anthocyanin biosynthesis by inhibiting the expression of VvCHI, VvCHS, VvDFR, and VvUFGT (Cavallini et al., 2015; Zhu et al., 2019). VvMYBC2-L1 also down-regulates VvMYBPA1, VvMYBPA2, VvDFR, VvLDOX, VvANR, VvLAR1 and VvLAR2 expression to suppress the PAs synthesis (Huang et al., 2014). Cheng et al. (2021) found VvMYB86 promotes PAs synthesis through the up-regulation of VvLAR transcription and inhibits anthocyanin biosynthesis via the down-regulation of VvANS and VvUFGT expression. Among bHLH regulators, it has been confirmed that VvMYC1 can interact with VvMYB5a, VvMYB5b, VvMYBA1/A2, and VvMYBPA1, resulting in the induction of the promoter activities of VvUFGT, VvANR, and VvCHI (Hichri et al., 2010). The studies from Matus et al. (2010) and Jiu et al. (2021) reported that WDR1 enhances the anthocyanin accumulation, by forming the complex with VvMYBA2 and VvMYCA1.
The accumulation of flavonoid is affected by both macro- and micro-climates in vineyard (Downey et al., 2006; Gutierrez-Gamboa et al., 2017; Martinez-Gil et al., 2018). In a specific vineyard, environmental factors such as water, light, and temperature can be adjusted through viticulture practices to regulate the qualities of grape berries and wines (Mori et al., 2007a; Azuma et al., 2012; Rienth et al., 2021). A comprehensive understanding of the mechanisms that regulate flavonoid accumulation in response to environmental factors can contribute to the precise control of fruit traits through cultivation. The enzymes and transcription factors responsible for the biosynthesis of anthocyanins and PAs in grapevine have been extensively studied in the context of spatio-temporal accumulation of metabolites, providing the basis for interpreting how gene expression patterns cause grape berry traits under various environmental conditions. However, the lack of suitable working models to understand conflicting results from field experiment reflects the fact that the interaction between genetic background of grapevine and environmental factors has not been fully resolved. In terms of the remodeling of flavonoid biosynthesis network under various abiotic stresses, the essential roles of phytohormones (e.g. abscisic acid (ABA), ethylene, melatonin, gibberellin GA, brassinolide(BR)) in mediating the accumulation of anthocyanins and PAs in grapevine has been revealed by metabolomics and transcriptomic studies in recent years (Mori et al., 2005a; Loreti et al., 2008; Sun et al., 2017).
2 The ABA-centered flavonoid biosynthesis regulation network under water deficit
The current understandings of the effects of water condition on berry qualities are mainly from the practice of regulated deficit irrigation (RDI), a strategy to balance the water usage and the yield (Costa et al., 2007). The modulation of flavonoid biosynthesis in grape berry under water deficit is a complex process, as it may be affected by at least macro climate, grape variety, rootstock, drought timing and even berry size (Roby and Matthews, 2004; Kuhn et al., 2014; Gambetta et al., 2020; Afifi et al., 2021). Moreover, the parameter for representing the water deficit extent varies among studies, such as evapotranspiration (usually ranged from 30% to 80%) and water potentials of leaves or stems (usually ranged from -1.4 to -0.6). It is therefore plausible to observe conflict conclusions drawn from different experiments. By revisiting the existing studies, we here summarized the general molecular mechanism regarding the response of PA and anthocyanin pathways to water deficit, and proposed the omitted aspects that hinder answering the lingering questions from various studies. The commonly used RDI strategies in field studies include the early deficit (from berry setting to veraison), the late deficit (from verasion to harvest) and the seasonal deficit (from berry setting to harvest). It has repeatedly been shown that water deficit has no effect on flavonols, although increases anthocyanin level in red grape skins at harvest, regardless the timing of RDI (Castellarin et al., 2007a; Castellarin et al., 2007b; Deluc et al., 2009; Caceres-Mella et al., 2017; Yang et al., 2020a). This is in line with the increased transcription levels of VvPAL, VvCHS, VvF3H, VvF3’H, VvDFR, VvANS, VvGST and VvUFGT responsible for anthocyanin biosynthesis in the corresponding RDI treatments (Castellarin et al., 2007a; Deluc et al., 2009; Yang et al., 2020a) (Figure 2). All three RDI strategies enhance the methylation of anthocyanins, while the late and seasonal RDI but not the early water deficit significantly increases the levels of delphindin-based and acylated anthocyanins in grape berries (Castellarin et al., 2007a; Olle et al., 2011; Yang et al., 2020a). This suggests that VvOMT is essentially responsive to water deficit across the berry development, while VvF3’5’H and Vv3AT are mainly sensitive to water status post-veraison. However, the patterns of the detected VvF3’5’H transcript levels are not always well-correlated with delphindin derivates accumulations among different RDI treatments (Castellarin et al., 2007a). It is noteworthy that VvF3’5’H gene family in grapevine possess more than ten isoforms, and the levels of 3’5’-OH anthocyanins are dependent on the abundance of VvF3’5’H transcripts pool in grapes (Falginella et al., 2010). Thus, it will be more informative to include the expression data of multiple VvF3’5’H gene isoforms when aiming at clarifying the mechanism about the response of anthocyanin hydroxylation extent to water deficit timing. Under both early and late water deficit treatments, the enhanced anthocyanin accumulation in grape berry associates with ripening acceleration, the process mainly regulated by ABA (Castellarin et al., 2007a). Transcriptome and metabolite data both showed that seasonal water deficit can promote ABA synthesis in red grape berries, especially at veraison (Deluc et al., 2009). And the effect of seasonal RDI on anthocyanin accumulation can be diminished by applying ABA synthesis inhibitor nordihydroguaiaretic acid (NDGA) (Guo et al., 2021). These findings suggest the pivotal role of ABA to enhance anthocyanin biosynthesis in grape berries under water deficit stress. The exogenous ABA application studies showed that most of flavonoid genes activated by ABA in grape berries are overlapped with that triggered by water deficit, including three flavonoid activators VvMYBA1, VvMYBA2 and VvMYBPA1 (Koyama et al., 2010; Caceres-Mella et al., 2017; Sun et al., 2019; Guo et al., 2021). Genetic evidence showed that VvMYBA1/2 target to genes in the entire anthocyanin pathway except VvF3H and VvF3’H, while VvMYBPA1 activates VvF3H, VvF3’H and two PA branch genes VvLAR1 and VvANR (Terrier et al., 2009; Rinaldo et al., 2015). Thus, it is likely that VvMYBA1/2 and VvMYBPA1 complement with each other for channeling the flux to anthocyanins regulated by ABA in grape berries under water deficit stress (Figure 2).
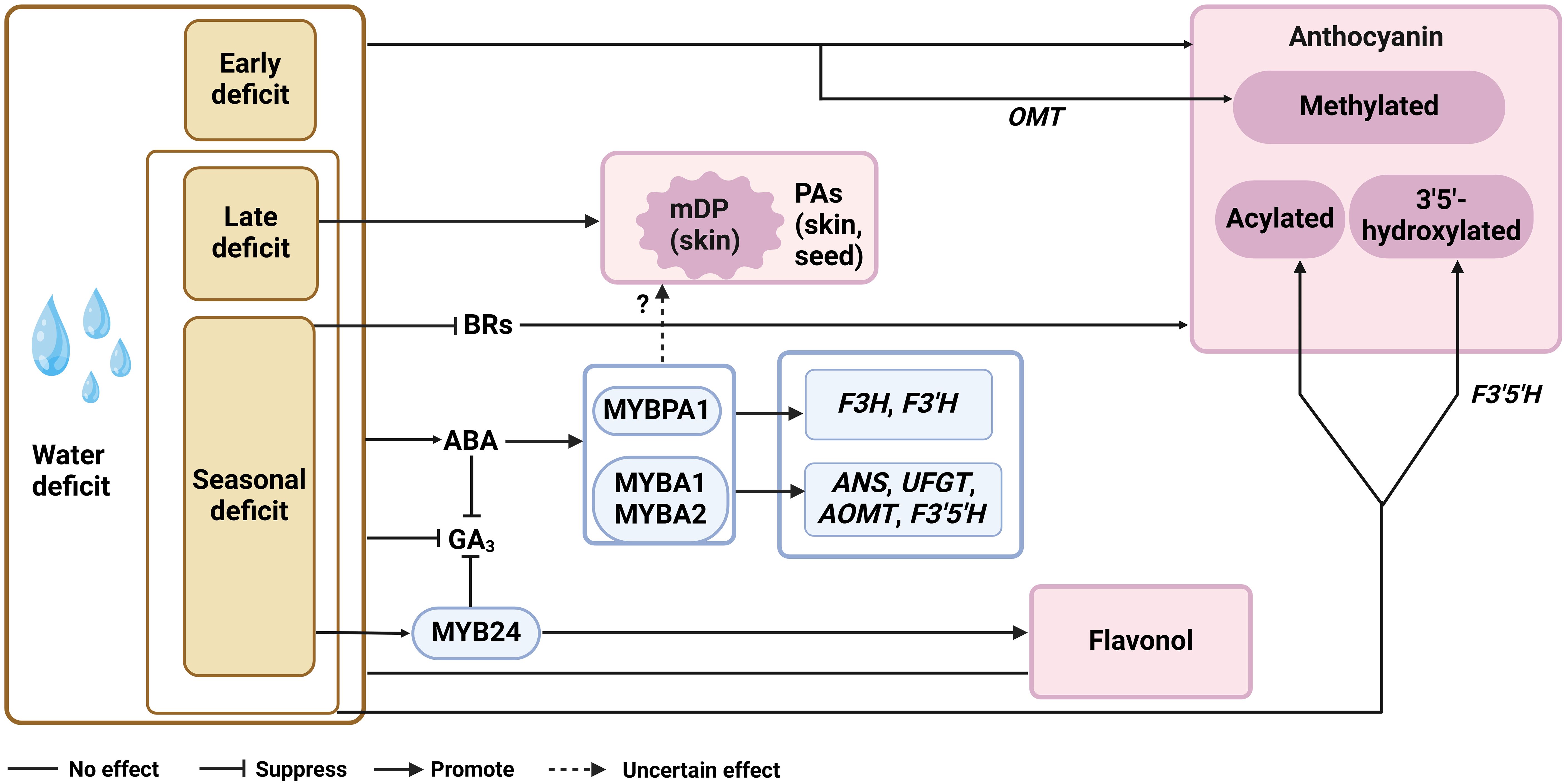
Figure 2. The ABA-centered flavonoid regulation network under water deficit. mDP, mean polymerization degrees; ABA, abscisic acid; GA3, gibberellin, BRs, brassinolide; F3H, flavanone 3-hydroxylase; F3’H, flavanone 3’-hydroxylase; F3’5’H, flavanone 3’5’-hydroxylase; ANS, anthocyanidin synthase; UFGT, UDP-glucose, flavonoid 3-O-glucosyltransferase; OMT, O-methyltransferase.
Although VvMYBPA1 is crucial for PA accumulation in the context of grape berry development (Bogs et al., 2007), its enhanced expression seems not effectively promote PA biosynthesis under water deficit stress. Numerous studies have shown that water deficit has little effect on PA accumulation in grape berries at harvest, or its effect on grape PA content does not show a stable trend among different years (Castellarin et al., 2007a; Bucchetti et al., 2011; Olle et al., 2011; Genebra et al., 2014; Herrera et al., 2015; Yu et al., 2016; Caceres-Mella et al., 2017). Compositional analysis suggests that it is the mDP of PA, but not the gallyollation extent of PA, increases in grape skins under long term or late water deficit (Caceres-Mella et al., 2017) (Figure 2). At the transcription level, VvANR expression in grape skins is not responsive to late RDI but can be down-regulated by early RDI, while VvLAR2 transcript level is affected by neither of these two treatments (Castellarin et al., 2007a). With seasonal RDI, VvLAR2 transcript abundance can be transiently elevated before veraison, and the similar response is also observed in the grape berries sprayed with ABA (Lacampagne et al., 2010; Villalobos-González et al., 2016; Caceres-Mella et al., 2017). Moreover, pre-veraison ABA application delayed the expression of VvANR and VvLAR1, which consistent with the lower VvANR and VvLAR enzymatic activities during PA biosynthesis active stage in grape berries (Lacampagne et al., 2010). Although exogenous ABA application to large extent resemble the PA gene expression patterns under water deficit, to how much extent ABA involves in the regulation of PA structural genes expression remains to be answered, and the missing components that disturb the effects of VvMYBPA1 on the expression PA structural genes under water deficit need to be further discovered.
Accompanied with the increased ABA concentration during pre-veraison under seasonal water deficit treatments, BR and GA3 levels are slightly decreased in grape berries in consecutive years (Yang et al., 2020b; Guo et al., 2021) (Figure 2). It has been shown that the spraying of BR before veraison enhanced the accumulation of anthocyanins and PAs in berry skins (Luan et al., 2013; Xi et al., 2013; Vergara et al., 2018), while exogenous application of GA3 does not significantly affect the levels of anthocyanins and PAs (Reynolds et al., 2016; Murcia et al., 2017; Kaplan et al., 2019; Gao et al., 2020; Tyagi et al., 2021), except for that the long-term 80 mg/L GA3 application on ‘Red globe’ promotes anthocyanin biosynthesis (Dong et al., 2023). The trade-off of ABA and BR levels has been proposed as the strategy to balance the stress response and development in plants (Wang et al., 2020b), which might also contribute to tuning the flux between PA and anthocyanin pathways under water deficit. In addition, ABA inhibits GA biosynthesis and induces GA degradation to increase drought tolerance of plants (Shohat et al., 2021; Mukherjee et al., 2023). Recently, VyMYB24 is found to confer the drought tolerance in Chinese wild Vitis species V. yanshanesis and negatively regulate GA3 level under drought stress (Zhu et al., 2022), and its homolog in V. vinifera is responsible for the enhanced flavone and terpenoid accumulation under drought and high light/UV conditions (Savoi et al., 2016; Lu et al., 2021; Zhang et al., 2023). Then further answering whether and how MYB24 participates in the interaction of phytohormone levels and flavonoid gene expression can help with understanding ABA-centered anthocyanins and PAs regulation network under water deficit (Figure 2).
3 The interaction of light and phytohormones in regulating the accumulation of flavonoid
In the viticulture practice, bagging, net-shading, and canopy managements (leaf removal and shoot thinning) are effective approaches to adjust both quantity and quality of sunlight around grape berry clusters. Exposure of grape berries to sunlight generally increases anthocyanin and flavonol levels in skins, mainly by activating the expression of phenylpropanoid genes (VvPAL, Vv4CL), flavonoid genes (VvCHS, VvCHI, VvF3’H, VvF3’5’H, VvUFGT), flavonol gene (VvFLS) and the relevant transcription regulators (VvMYBA1, VvMYB5b, VvMYBF1 and VvHY5) (Matsuyama et al., 2014; Guan et al., 2016; Czemmel et al., 2017; Li et al., 2023) (Figure 3). However, excessive sunlight exposure can result in the sunburn of berry clusters, which induces the degradation of anthocyanins, PAs and flavonols (Sun et al., 2017; Reshef et al., 2018; Torres et al., 2020). Flavonol biosynthesis is particularly sensitive to UV-B light and can be significantly inhibited in berries subjected to light exclusion using light-proof box (Koyama et al., 2012; Loyola et al., 2016). It was found that VvHY5 activated VvMYBF1 transcript level under UV-B, which in turn regulated the expression of VvFLS1, VvGT3 (encoding a glycosyltransferase) and VvRHaT1 (encoding a rhamnosyltransferase), to promote flavonol accumulation. Additionally, VvMYBF1 negatively regulated the expression of VvMYBPA1, indicating a competition between the flavonol and PA branches (Czemmel et al., 2017). Under light exclusion, the proportion of dihydroxylated anthocyanins increased due to the down-regulation of F3’5’H expression (Downey et al., 2004; Azuma et al., 2012; Koyama et al., 2012; Matsuyama et al., 2014; Guan et al., 2016). Guan et al. (2016) also found the proportion of methylated anthocyanins increased. Light exposed and exclusion had little effect on PA content at harvest (Downey et al., 2004; Fujita et al., 2007; Koyama and Goto Yamamoto, 2008; Liu et al., 2015; VanderWeide et al., 2022) (Figure 3). This may be because the treatments are applied at the onset of veraison, while PAs are mainly synthesized in large quantities at the flowering stage. Shading grape clusters during the pre-veraison period, decreased PA levels in berry skins by down-regulating the expression of VvLAR1 and VvANR before ripening (Downey et al., 2004; Jeong et al., 2004; Fujita et al., 2007; Koyama and Goto-Yamamoto, 2008; Liu et al., 2015) (Figure 3). Researchers speculate that extractable PAs decreased more markedly in sunlight-exposed conditions than in shaded berry skins during ripening, suggesting that light promotes the synthesis of insoluble PAs. Downey et al. (2004) and Fujita et al. (2007) also discovered there is no significant effect of light exclusion on the level of PAs in the seeds. While the proportion of the dihydroxylated subunits increased in berry skins, which agreed with the down-regulation of VvF3’5’H levels Koyama and Goto-Yamamoto, 2008). By Shading with light-proof boxes, this group further confirm that light exclusion decreased the mean degree of polymerization (mDP) of PAs in grape skin (Koyama et al., 2012).
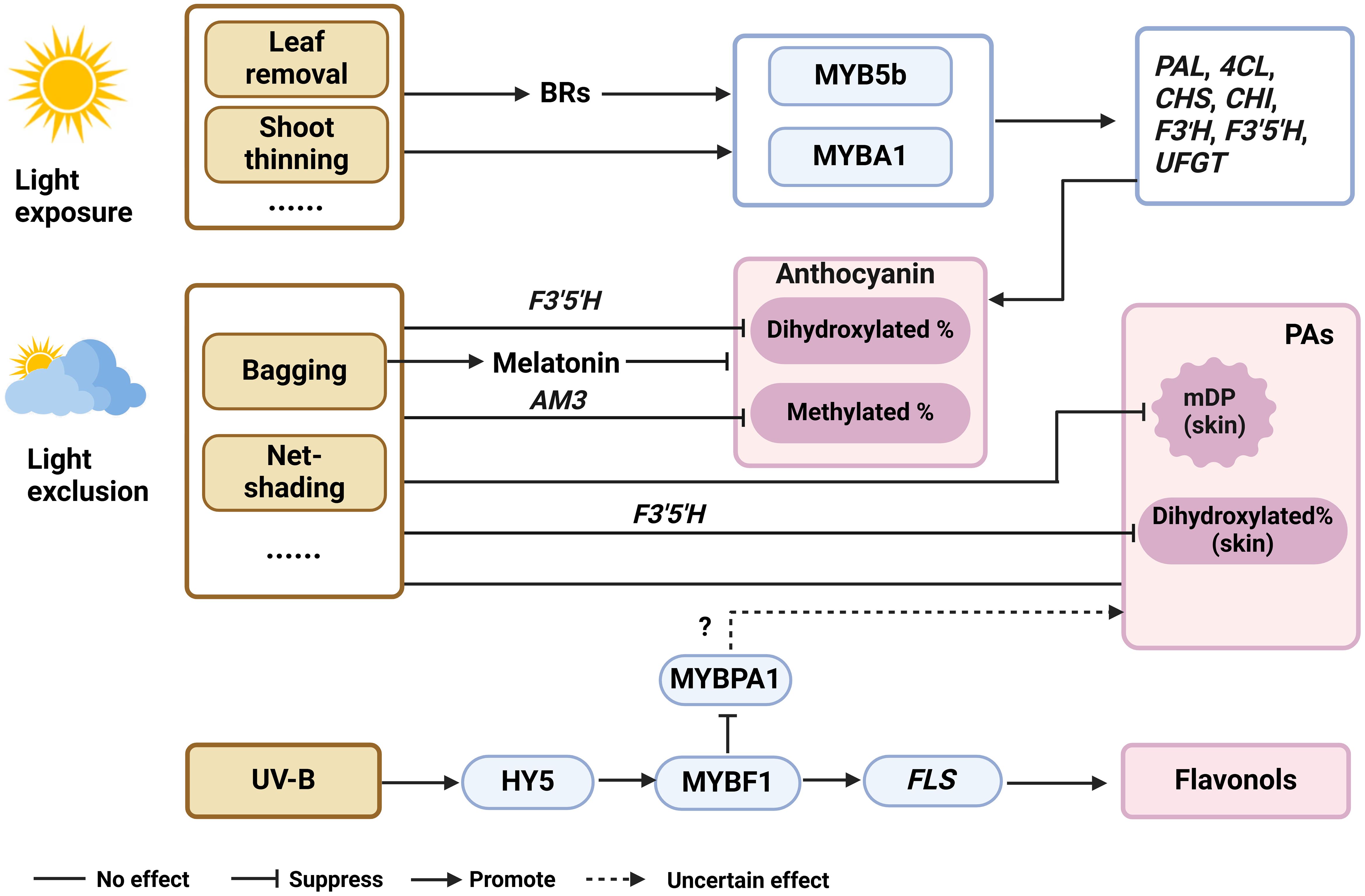
Figure 3. The roles of phytohormones in mediating the biosynthesis of flavonoid under various light conditions. mDP, mean polymerization degrees, BRs, brassinolide, ABA, abscisic acid, UV-B, ultraviolet-B, F3’5’H, flavanone 3’5’-hydroxylase, AM3, MATE3, PAL, phenylalanine ammonia-lyase; 4CL, 4-coumarate, CoA-ligase; CHS, chalcone synthase; CHI, chalcone isomerase; F3’H, flavanone 3’-hydroxylase; UFGT, UDP-glucose, flavonoid 3-O-glucosyltransferase; FLS, flavonol synthase.
Several studies have focused on endogenous hormones in grapes under light conditions. Guo et al. (2020) bagged two wine grape varieties, 'Cabernet Sauvignon' and 'Carignane', from fruit set until harvest and found the cluster bagging induced the melatonin synthesis. Meng et al. (2019) found the application of melatonin during the pre-veraison period at the concentration of 100 mg/L decreased the anthocyanin accumulation, while increasing the content of (+)-catechin and (-)-epicatechin in 'Merlot' berry skin. This suggests that light exclusion reduces anthocyanin accumulation by increasing melatonin levels (Figure 3). Previous research in our laboratory found that the transcriptional changes of genes required for the biosynthesis and signal transduction of auxin, ethylene, BR and ABA were in accordance with the flavonoid accumulation in light-exposed berries during development, indicating the importance of phytohormones on berry flavonoid biosynthesis in response to light (Sun et al., 2017). Guan et al. (2016) bagged the grapes from fruit set to maturity, and found that total anthocyanin levels were lower under light-exposed conditions, but ABA concentration was elevated at fruit setting stage, then the difference diminished. UV-B irradiation of grapes from pre-flowering until harvest had no effect on endogenous ABA levels (Berli et al., 2011), suggesting that ABA plays a limited role in mediating the light and UV-B effects on anthocyanin biosynthesis (Figure 3). Liu et al. (2016) analyzed endogenous ethylene biosynthetic and signal-transduction pathways under light and dark condition, and found no significant difference in the expression of genes involved during the grape development, suggesting that ethylene is not responsive to light. When grape clusters are sprayed with exogenous 24-epibrassinolide (a type of BR) under both light and dark conditions, the increase in anthocyanin levels is significantly greater under light condition. Correspondingly, the application of BR up-regulated the expression of flavonoid genes (VvCHI1, VvCHS2, VvCHS3, VvDFR, VvANS, VvMYBA1) under light conditions (Zhou et al., 2018) (Figure 3). Throughout grape development, light increases VvBZR1 transcript, a key transcription factor positively regulating BR, suggesting that light likely increases endogenous BR content. Moreover, it has been repeatedly reported that exogenous supplement of BR enhances anthocyanin accumulation in grape skin (Luan et al., 2013; Xi et al., 2013; Vergara et al., 2018). These findings together indicate that BR and light have synergistic effects on anthocyanin biosynthesis in grapes. Additionally, BR signaling through BRI1-EMS-SUPPRESSOR 1 (BES1) typically inhibits flavonol synthesis to promote plant growth. However, under UV-B stress, this inhibition is lifted, leading to increased flavonol production in Arabidopsis (Liang et al., 2020). This BR-UV-flavonoid interaction network may also help with understanding the flux control between flavonols and anthocyanins/PAs, but it remains to be testified in grapevine in further studies.
4 The limited understanding of phytohormones in mediating the response of the flavonoid pathway to temperature changes
Climate models predict an increase in both average and extreme atmospheric temperatures (Santos et al., 2020; Gashu et al., 2023). The effect of elevated temperatures on flavonoids metabolism has been a focal point in grape research area. Most studies have demonstrated the inhibitory impact of high temperature (equal to or above 30°C) on anthocyanin. Molecular analysis has revealed decreased transcription of phenylpropanoid and flavonoid structural genes, including VvPAL, VvCHS, VvCHI, and VvUFGT, as well as transcription factor genes such as VvMYBA1 and VvMYBA2 under high temperature (Yamane et al., 2006; Mori et al., 2007b; Lecourieux et al., 2017; Pastore et al., 2017; Yan et al., 2020) (Figure 4). In addition to repressing anthocyanin-related genes, high temperatures have been shown to increase peroxidase activity in cells, promoting anthocyanin degradation (Mori et al., 2007b; Yan et al., 2020). High temperatures also alter anthocyanin composition, increasing the proportion of acylated and tri-hydroxylated anthocyanins, which is accompanied by enhanced expression of Vv3AT (Lecourieux et al., 2017; Pastore et al., 2017; Yan et al., 2020). Elevated temperatures reduce endogenous ABA levels, as well as the expression levels of VvMYBA1 and most flavonoid structural genes, suggesting that ABA may play a role in mediating the response of the flavonoid pathway to temperature changes (Yamane et al., 2006; Koshita et al., 2007; Azuma et al., 2012) (Figure 4). Mori et al. (2005a); Mori et al. (2005b) demonstrated that application of 250 ppm ABA through spraying almost diminished the adverse impact of night-time high temperature treatment on the reduction of anthocyanin levels in grape berries. Furthermore, Mori et al. (2005a) reported that high night temperatures (30°C) from veraison to maturity, compared to low night temperatures (15°C), reduced anthocyanin concentration in grape berry skins by inhibiting the expression of VvCHS, VvF3H, VvDFR, and VvUFGT, while flavonol levels remained unchanged. Gaiotti et al. (2018) also found low night temperature (10°C) during veraison favors the accumulation of anthocyanin by upregulating VvCHS, VvF3H, VvUFGT and VvMYBA1. Furthermore, Yan et al. (2020) observed that day temperature exerts a stronger influence on anthocyanin accumulation than night temperature. Their study, which involved setting different day and night temperature regimes, revealed that higher day temperatures led to a more pronounced decrease in anthocyanin concentration compared to variations in night temperatures.
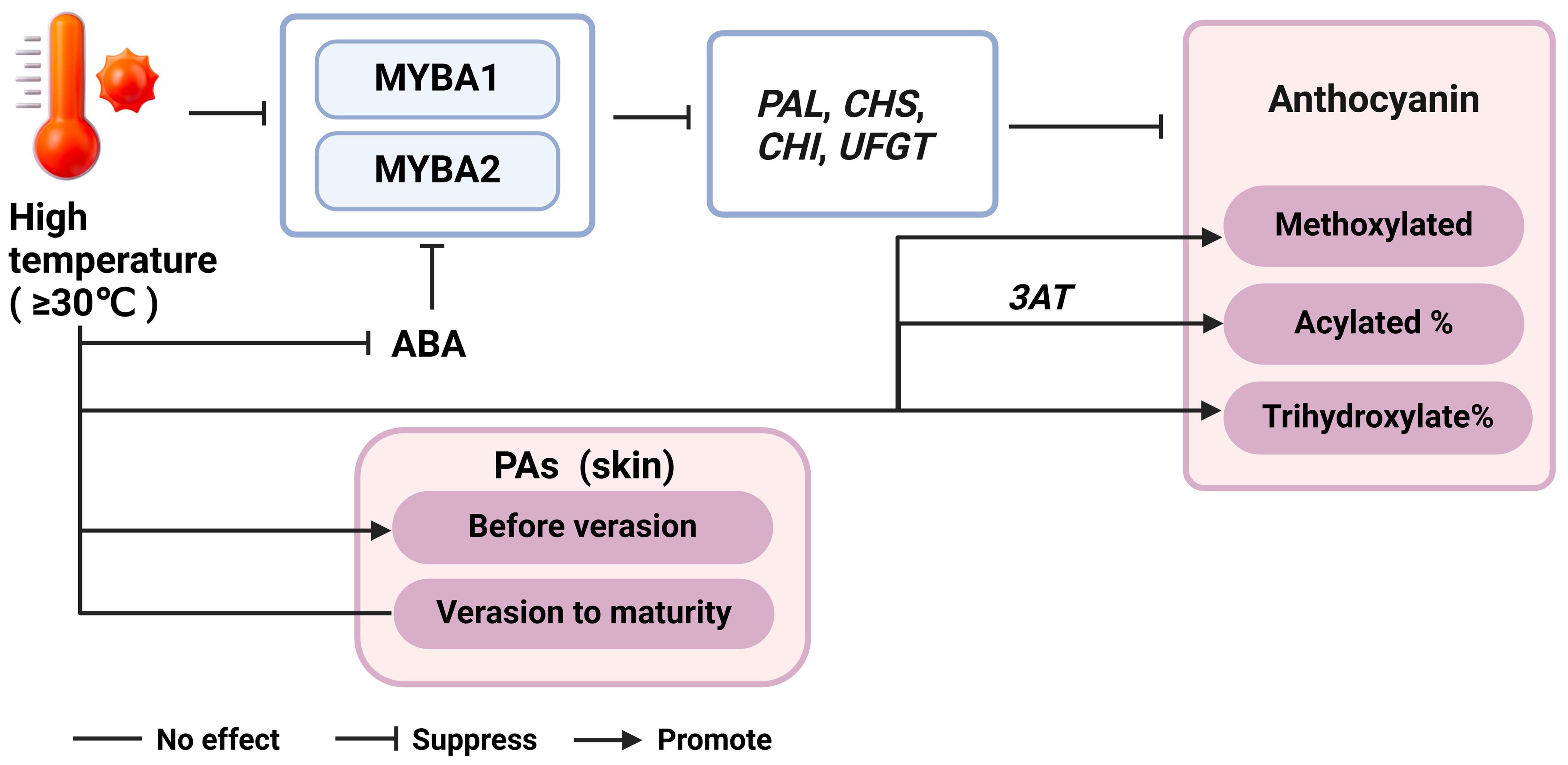
Figure 4. The regulatory network of temperature, phytohormones and flavonoid accumulation. ABA, abscisic acid; PAL, phenylalanine ammonia-lyase; CHS, chalcone synthase; CHI, chalcone isomerase; UFGT, UDP-glucose, flavonoid 3-O-glucosyltransferase.
The effect of temperature on PAs and flavonols accumulation is still not well understood. High night temperatures (above 8°C compared to environmental temperatures) during PA biosynthesis stages promoted flavan-3-ols synthesis in grape skins, while these differences were no longer significant after veraison until maturity, and no effect on flavonols regardless of the period of treatment (Cohen et al., 2012a; Cohen et al., 2012b). Pastore et al. (2017) found that PAs were not affected by heat stress (average temperature 26°C), while flavonols were decreased in Sangiovese grape skins. Similarly, studies by Gouot et al. (2019b) and Wu et al. (2019) observed no significant effect on flavan-3-ol monomer and PA levels in grape skins and seeds. In the study by Wu et al. (2019), a moderate increase in grape temperature was achieved by creating a local greenhouse effect, which led to an increase in PA levels before veraison but a decrease thereafter (Figure 4). This indicates that the timing and duration of heat exposure (at least 1.5°C higher than the ambient temperature) are crucial in determining the impact on PA accumulation. Overall, these findings highlight the nuanced and complex nature of temperature effects on grape phenolic compounds, emphasizing the need for further research to fully understand these interactions and their implications for viticulture under changing climatic conditions (Cohen et al., 2012a; Cohen et al., 2012b; Pastore et al., 2017; Gouot et al., 2019b; Wu et al., 2019).
5 Conclusion and prospect
In general, flavonoid metabolism constitute a complex process in grape. Environmental factors including water, light and temperature, significantly affect their biosynthesis. It is worth noting that there are interactions between these factors in field practice. For instance, bagging not only affects the light intensity, but also impacts on the temperature of grape. Therefore, the investigation of the interaction of various environmental factors on flavonoid metabolism is crucial for future research. Moreover, PAs is mainly synthesized at the flowering stage, so treatment of environmental factors at flowering stage may be more significant for the study of PAs regulation mechanism. Phytohormones signal network is complex and interactive during grape development. They play an important role as a medium in the process of studying environmental regulation of flavonoid. But there are limited studies that have conducted in-depth research, especially for temperature and light.
Additionally, although gene editing technology has been applied to optimize crop traits in other species such as rice and tomato, its implementation in grapes remains challenging due to the inhibition of polyphenols and other related antioxidant pathways (Osakabe et al., 2018). Consequently, scientists are focusing on more traditional hybridization methods to achieve the desired flavonoid characteristics in grapes (Yu et al., 2019). In the short term, adjusting the microclimate (water, light, and temperature) and hormone levels in grapes may be the most valuable strategy in the field. Furthermore, these cultivation treatments can help identify genes related to environmental factors and plant hormones, which can serve as breeding markers for regulating flavonoid traits.
Author contributions
TS: Writing – original draft, Writing – review & editing, Conceptualization, Investigation. YS: Writing – review & editing. YL: Supervision, Writing – review & editing. CD: Funding acquisition, Supervision, Writing – review & editing. KY: Conceptualization, Funding acquisition, Investigation, Supervision, Writing – review & editing.
Funding
The author(s) declare financial support was received for the research, authorship, and/or publication of this article. This research was supported by the National Natural Science Foundation of China (32202452 and U20A2042); Science and Technology Major Project of Xinjiang Uygur Autonomous Region (2022A02002).
Conflict of interest
The authors declare that the research was conducted in the absence of any commercial or financial relationships that could be construed as a potential conflict of interest.
Publisher’s note
All claims expressed in this article are solely those of the authors and do not necessarily represent those of their affiliated organizations, or those of the publisher, the editors and the reviewers. Any product that may be evaluated in this article, or claim that may be made by its manufacturer, is not guaranteed or endorsed by the publisher.
References
Afifi, M., Obenland, D., El-kereamy, A. (2021). The complexity of modulating anthocyanin biosynthesis pathway by deficit irrigation in table grapes. Front. Plant Sci. 12, 713277. doi: 10.3389/fpls.2021.713277
Azuma, A., Yakushiji, H., Koshita, Y., Kobayashi, S. (2012). Flavonoid biosynthesis-related genes in grape skin are differentially regulated by temperature and light conditions. Planta 236, 1067–1080. doi: 10.1007/s00425-012-1650-x
Beres, C., Costa, G. N. S., Cabezudo, I., da Silva-James, N. K., Teles, A. S. C., Cruz, A. P. G., et al. (2017). Towards integral utilization of grape pomace from winemaking process: A review. Waste Manage. 68, 581–594. doi: 10.1016/j.wasman.2017.07.017
Berli, F. J., Fanzone, M., Piccoli, P., Bottini, R. (2011). Solar UV-B and ABA are involved in phenol metabolism of Vitis vinifera L. increasing biosynthesis of berry skin polyphenols. J. Agric. Food Chem. 59, 4874–4884. doi: 10.1021/jf200040z
Bogs, J., Downey, M. O., Harvey, J. S., Ashton, A. R., Tanner, G. J., Robinson, S. P. (2005). Proanthocyanidin synthesis and expression of genes encoding leucoanthocyanidin reductase and anthocyanidin reductase in developing grape berries and grapevine leaves. Plant Physiol. 139, 652–663. doi: 10.1104/pp.105.064238
Bogs, J., Ebadi, A., McDavid, D., Robinson, S. P. (2006). Identification of the flavonoid hydroxylases from grapevine and their regulation during fruit development. Plant Physiol. 140, 279–291. doi: 10.1104/pp.105.073262
Bogs, J., Jaffé, F. W., Takos, A. M., Walker, A. R., Robinson, S. P. (2007). The grapevine transcription factor VvMYBPA1 regulates proanthocyanidin synthesis during fruit development. Plant Physiol. 143, 1347–1361. doi: 10.1104/pp.106.093203
Bucchetti, B., Matthews, M. A., Falginella, L., Peterlunger, E., Castellarin, S. D. (2011). Effect of water deficit on Merlot grape tannins and anthocyanins across four seasons. Scientia Hortic. 128, 297–305. doi: 10.1016/j.scienta.2011.02.003
Caceres-Mella, A., Inmaculada Talaverano, M., Villalobos-Gonzalez, L., Ribalta-Pizarro, C., Pastenes, C. (2017). Controlled water deficit during ripening affects proanthocyanidin synthesis, concentration and composition in Cabernet Sauvignon grape skins. Plant Physiol. Biochem. 117, 34–41. doi: 10.1016/j.plaphy.2017.05.015
Castellarin, S. D., Matthews, M. A., Di Gaspero, G., Gambetta, G. A. (2007a). Water deficits accelerate ripening and induce changes in gene expression regulating flavonoid biosynthesis in grape berries. Planta 227, 101–112. doi: 10.1007/s00425-007-0598-8
Castellarin, S. D., Pfeiffer, A., Sivilotti, P., Degan, M., Peterlunger, E., Di Gaspero, G. (2007b). Transcriptional regulation of anthocyanin biosynthesis in ripening fruits of grapevine under seasonal water deficit. Plant Cell Environ. 30, 1381–1399. doi: 10.1111/j.1365-3040.2007.01716.x
Cavallini, E., Tomas Matus, J., Finezzo, L., Zenoni, S., Loyola, R., Guzzo, F., et al. (2015). The phenylpropanoid pathway is controlled at different branches by a set of R2R3-MYB C2 repressors in grapevine. Plant Physiol. 167, 1448–U1552. doi: 10.1104/pp.114.256172
Cheng, J., Yu, K. J., Shi, Y., Wang, J., Duan, C. Q. (2021). Transcription factor VviMYB86 oppositely regulates proanthocyanidin and anthocyanin biosynthesis in grape berries. Front. Plant Sci. 11, 613677. doi: 10.3389/fpls.2020.613677
Cohen, S. D., Tarara, J. M., Gambetta, G. A., Matthews, M. A., Kennedy, J. A. (2012a). Impact of diurnal temperature variation on grape berry development, proanthocyanidin accumulation, and the expression of flavonoid pathway genes. J. Exp. Bot. 63, 2655–2665. doi: 10.1093/jxb/err449
Cohen, S. D., Tarara, J. M., Kennedy, J. A. (2012b). Diurnal temperature range compression hastens berry development and modifies flavonoid partitioning in grapes. Am. J. Enol. Vitic. 63, 112–120. doi: 10.5344/ajev.2011.11015
Costa, J. M., Ortuno, M. F., Chaves, M. M. (2007). Deficit irrigation as a strategy to save water: Physiology and potential application to horticulture. J. Integr. Plant Biol. 49, 1421–1434. doi: 10.1111/j.1672-9072.2007.00556.x
Cutanda-Perez, M. C., Ageorges, A., Gomez, C., Vialet, S., Terrier, N., Romieu, C., et al. (2009). Ectopic expression of VlmybA1 in grapevine activates a narrow set of genes involved in anthocyanin synthesis and transport. Plant Mol. Biol. 69, 633–648. doi: 10.1007/s11103-008-9446-x
Czemmel, S., Hoell, J., Loyola, R., Arce-Johnson, P., Antonio Alcalde, J., Tomas Matus, J., et al. (2017). Transcriptome-wide identification of novel UV-B- and light modulated flavonol pathway genes controlled by VviMYBF1. Front. Plant Sci. 8, 1084. doi: 10.3389/fpls.2017.01084
Czemmel, S., Stracke, R., Weisshaar, B., Cordon, N., Harris, N. N., Walker, A. R., et al. (2009). The grapevine R2R3-MYB transcription factor VvMYBF1 regulates flavonol synthesis in developing grape berries. Plant Physiol. 151, 1513–1530. doi: 10.1104/pp.109.142059
Deluc, L., Barrieu, F., Marchive, C., Lauvergeat, V., Decendit, A., Richard, T., et al. (2006). Characterization of a grapevine R2R3-MYB transcription factor that regulates the phenylpropanoid pathway. Plant Physiol. 140, 499–511. doi: 10.1104/pp.105.067231
Deluc, L., Bogs, J., Walker, A. R., Ferrier, T., Decendit, A., Merillon, J. M., et al. (2008). The transcription factor VvMYB5b contributes to the regulation of anthocyanin and proanthocyanidin biosynthesis in developing grape berries. Plant Physiol. 147, 2041–2053. doi: 10.1104/pp.108.118919
Deluc, L. G., Quilici, D. R., Decendit, A., Grimplet, J., Wheatley, M. D., Schlauch, K. A., et al. (2009). Water deficit alters differentially metabolic pathways affecting important flavor and quality traits in grape berries of Cabernet Sauvignon and Chardonnay. BMC Genomics 10, 212. doi: 10.1186/1471-2164-10-212
Dixon, R. A., Xie, D. Y., Sharma, S. B. (2005). Proanthocyanidins - a final frontier in flavonoid research? New Phytol. 165, 9–28. doi: 10.1111/j.1469-8137.2004.01217.x
Dong, Y. J., Wu, Y. X., Zhang, Z. X., Wang, S. C., Cheng, J., Gao, Y. L., et al. (2023). Transcriptomic analysis reveals GA3 is involved in regulating flavonoid metabolism in grape development for facility cultivation. Mol. Genet. Genomics 298, 845–855. doi: 10.1007/s00438-023-02019-z
Downey, M. O., Dokoozlian, N. K., Krstic, M. P. (2006). Cultural practice and environmental impacts on the flavonoid composition of grapes and wine: a review of recent research. Am. J. Enol. Vitic. 57, 257–268. doi: 10.5344/ajev.2006.57.3.257
Downey, M. O., Harvey, J. S., Robinson, S. P. (2004). The effect of bunch shading on berry development and flavonoid accumulation in Shiraz grapes. Aust. J. Grape Wine Res. 10, 55–73. doi: 10.1111/j.1755-0238.2004.tb00008.x
Eichenberger, M., Schwander, T., Hüppi, S., Kreuzer, J., Mittl, P. R. E., Peccati, F., et al. (2023). The catalytic role of glutathione transferases in heterologous anthocyanin biosynthesis. Nat. Catalysis 6, 924–938. doi: 10.1038/s41929-023-01018-y
Falginella, L., Castellarin, S. D., Testolin, R., Gambetta, G. A., Morgante, M., Di Gaspero, G. (2010). Expansion and subfunctionalisation of flavonoid 3′, 5′-hydroxylases in the grapevine lineage. BMC Genomics 11, 562. doi: 10.1186/1471-2164-11-562
Ferreira, V., Pinto-Carnide, O., Arroyo-Garcia, R., Castro, I. (2018). Berry color variation in grapevine as a source of diversity. Plant Physiol. Biochem. 132, 696–707. doi: 10.1016/j.plaphy.2018.08.021
Ferreyra, M. L. F., Rius, S. P., Casati, P. (2012). Flavonoids: biosynthesis, biological functions, and biotechnological applications. Front. Plant Sci. 3, 222. doi: 10.3389/fpls.2012.00222
Flamini, R., Mattivi, F., De Rosso, M., Arapitsas, P., Bavaresco, L. (2013). Advanced knowledge of three important classes of grape phenolics: anthocyanins, stilbenes and flavonols. Int. J. Mol. Sci. 14, 19651–19669. doi: 10.3390/ijms141019651
Fujita, A., Soma, N., Goto-Yamamoto, N., Mizuno, A., Kiso, K., Hashizume, K. (2007). Effect of shading on proanthocyanidin biosynthesis in the grape berry. J. Jpn. Soc Hortic. Sci. 76, 112–119. doi: 10.2503/jjshs.76.112
Gaiotti, F., Pastore, C., Filippetti, I., Lovat, L., Belfiore, N., Tomasi, D. (2018). Low night temperature at veraison enhances the accumulation of anthocyanins in Corvina grapes (Vitis Vinifera L.). Sci. Rep. 8, 8719. doi: 10.1038/s41598-018-26921-4
Gambetta, G. A., Herrera, J. C., Dayer, S., Feng, Q. S., Hochberg, U., Castellarin, S. D. (2020). The physiology of drought stress in grapevine: towards an integrative definition of drought tolerance. J. Exp. Bot. 71, 4658–4676. doi: 10.1093/jxb/eraa245
Gao, X. T., Wu, M. H., Sun, D., Li, H. Q., Chen, W. K., Yang, H. Y., et al. (2020). Effects of gibberellic acid (GA3) application before anthesis on rachis elongation and berry quality and aroma and flavour compounds in Vitis vinifera L. ‘Cabernet Franc’ and ‘Cabernet Sauvignon’ grapes. J. Sci. Food Agric. 100, 3729–3740. doi: 10.1002/jsfa.10412
Gashu, K., Verma, P. K., Acuña, T., Agam, N., Bustan, A., Fait, A. (2023). Temperature differences between sites lead to altered phenylpropanoid metabolism in a varietal dependent manner. Front. Plant Sci. 14. doi: 10.3389/fpls.2023.1239852
Genebra, T., Santos, R. R., Francisco, R., Pinto-Marijuan, M., Brossa, R., Serra, A. T., et al. (2014). Proanthocyanidin accumulation and biosynthesis are modulated by the irrigation regime in tempranillo seeds. Int. J. Mol. Sci. 15, 11862–11877. doi: 10.3390/ijms150711862
Gouot, J. C., Smith, J. P., Holzapfel, B. P., Walker, A. R., Barril, C. (2019a). Grape berry flavonoids: a review of their biochemical responses to high and extreme high temperatures. J. Exp. Bot. 70(2), 397–423. doi: 10.1093/jxb/ery392
Gouot, J. C., Smith, J. P., Holzapfel, B. P., Barril, C. (2019b). Impact of short temperature exposure of Vitis vinifera L. cv. Shiraz grapevine bunches on berry development, primary metabolism and tannin accumulation. Environ. Exp. Bot. 168, 103886. doi: 10.1016/j.envexpbot.2019.103866
Guan, L., Dai, Z., Wu, B.-H., Wu, J., Merlin, I., Hilbert, G., et al. (2016). Anthocyanin biosynthesis is differentially regulated by light in the skin and flesh of white-fleshed and teinturier grape berries. Planta 243, 23–41. doi: 10.1007/s00425-015-2391-4
Guo, S.-H., Xu, T.-F., Shi, T.-C., Jin, X.-Q., Feng, M.-X., Zhao, X.-H., et al. (2020). Cluster bagging promotes melatonin biosynthesis in the berry skins of Vitis vinifera cv. Cabernet Sauvignon and Carignan during development and ripening. Food Chem. 305, 125502. doi: 10.1016/j.foodchem.2019.125502
Guo, S.-H., Yang, B.-H., Wang, X.-W., Li, J.-N., Li, S., Yang, X., et al. (2021). ABA signaling plays a key role in regulated deficit irrigation-driven anthocyanins accumulation in ‘Cabernet Sauvignon ‘ grape berries. Environ. Exp. Bot. 181, 104290. doi: 10.1016/j.envexpbot.2020.104290
Gutierrez-Gamboa, G., Garde-Cerdan, T., Portu, J., Moreno-Simunovic, Y., Martinez-Gil, A. M. (2017). Foliar nitrogen application in Cabernet Sauvignon vines: Effects on wine flavonoid and amino acid content. Food Res. Int. 96, 46–53. doi: 10.1016/j.foodres.2017.03.025
He, F., Mu, L., Yan, G. L., Liang, N. N., Pan, Q. H., Wang, J., et al. (2010). Biosynthesis of anthocyanins and their regulation in colored grapes. Molecules 15, 9057–9091. doi: 10.3390/molecules15129057
He, F., Pan, Q.-H., Shi, Y., Duan, C.-Q. (2008). Biosynthesis and genetic regulation of proanthocyanidins in plants. Molecules 13, 2674–2703. doi: 10.3390/molecules13102674
Herrera, J. C., Bucchetti, B., Sabbatini, P., Comuzzo, P., Zulini, L., Vecchione, A., et al. (2015). Effect of water deficit and severe shoot trimming on the composition of Vitis vinifera L. Merlot grapes and wines. Aust. J. Grape Wine Res. 21, 254–265. doi: 10.1111/ajgw.12143
Hichri, I., Heppel, S. C., Pillet, J., Leon, C., Czemmel, S., Delrot, S., et al. (2010). The basic helix-loop-helix transcription factor MYC1 is involved in the regulation of the flavonoid biosynthesis pathway in grapevine. Mol. Plant 3, 509–523. doi: 10.1093/mp/ssp118
Holton, T. A., Cornish, E. C. (1995). Genetics and biochemistry of anthocyanin biosynthesis. Plant Cell 7, 1071–1083. doi: 10.1105/tpc.7.7.1071
Huang, Y.-F., Vialet, S., Guiraud, J.-L., Torregrosa, L., Bertrand, Y., Cheynier, V., et al. (2014). A negative MYB regulator of proanthocyanidin accumulation, identified through expression quantitative locus mapping in the grape berry. New Phytol. 201, 795–809. doi: 10.1111/nph.12557
Jeong, S. T., Goto-Yamamoto, N., Kobayashi, S., Esaka, A. (2004). Effects of plant hormones and shading on the accumulation of anthocyanins and the expression of anthocyanin biosynthetic genes in grape berry skins. Plant Sci. 167, 247–252. doi: 10.1016/j.plantsci.2004.03.021
Jiu, S., Guan, L., Leng, X., Zhang, K., Haider, M. S., Yu, X., et al. (2021). The role of VvMYBA2r and VvMYBA2w alleles of the MYBA2 locus in the regulation of anthocyanin biosynthesis for molecular breeding of grape (Vitis spp.) skin coloration. Plant Biotechnol. J. 19, 1216–1239. doi: 10.1111/pbi.13543
Jun, J. H., Lu, N., Docampo-Palacios, M., Wang, X., Dixon, R. A. (2021). Dual activity of anthocyanidin reductase supports the dominant plant proanthocyanidin extension unit pathway. Sci. Adv. 7, eabg4682. doi: 10.1126/sciadv.abg4682
Jun, J. H., Xiao, X. R., Rao, X. L., Dixon, R. A. (2018). Proanthocyanidin subunit composition determined by functionally diverged dioxygenases. Nat. Plant 4, 1034–1043. doi: 10.1038/s41477-018-0292-9
Kaplan, M., Najda, A., Klimek, K., Borowy, A. (2019). Effect of gibberellic acid (GA3) inflorescence application on content of bioactive compounds and antioxidant potential of grape (Vitis L.) ‘Einset seedless’ Berries. S. Afr. J. Enol. Vitic. 40, 1–10. doi: 10.21548/40-1-3004
Kobayashi, S., Goto-Yamamoto, N., Hirochika, H. (2004). Retrotransposon-induced mutations in grape skin color. Science 304, 982–982. doi: 10.1126/science.1095011
Koshita, Y., Asakura, T., Fukuda, H., Tsuchida, Y. (2007). Nighttime temperature treatment of fruit clusters of ‘Aki Queen’ grapes during maturation and its effect on the skin color and abscisic acid content. Vitis 46, 208–209.
Koyama, K., Goto-Yamamoto, N. (2008). Bunch shading during different developmental stages affects the phenolic biosynthesis in berry skins of ‘Cabernet sauvignon’ Grapes. J. Am. Soc Hortic. Sci. 133, 743–753. doi: 10.21273/jashs.133.6.743
Koyama, K., Ikeda, H., Poudel, P. R., Goto-Yamamoto, N. (2012). Light quality affects flavonoid biosynthesis in young berries of Cabernet Sauvignon grape. Phytochemistry 78, 54–64. doi: 10.1016/j.phytochem.2012.02.026
Koyama, K., Numata, M., Nakajima, I., Goto-Yamamoto, N., Matsumura, H., Tanaka, N. (2014). Functional characterization of a new grapevine MYB transcription factor and regulation of proanthocyanidin biosynthesis in grapes. J. Exp. Bot. 65, 4433–4449. doi: 10.1093/jxb/eru213
Koyama, K., Sadamatsu, K., Goto-Yamamoto, N. (2010). Abscisic acid stimulated ripening and gene expression in berry skins of the Cabernet Sauvignon grape. Funct. Integr. Genomics 10, 367–381. doi: 10.1007/s10142-009-0145-8
Kuhn, N., Guan, L., Dai, Z. W., Wu, B. H., Lauvergeat, V., Gomès, E., et al. (2014). Berry ripening: recently heard through the grapevine. J. Exp. Bot. 65, 4543–4559. doi: 10.1093/jxb/ert395
Lacampagne, S., Gagné, S., Gény, L. (2010). Involvement of abscisic acid in controlling the proanthocyanidin biosynthesis pathway in grape skin: new elements regarding the regulation of tannin composition and leucoanthocyanidin reductase (LAR) and anthocyanidin reductase (ANR) activities and expression. J. Plant Growth Regul. 29, 81–90. doi: 10.1007/s00344-009-9115-6
Lecourieux, F., Kappel, C., Pieri, P., Charon, J., Pillet, J., Hilbert, G., et al. (2017). Dissecting the biochemical and transcriptomic effects of a locally applied heat treatment on developing cabernet sauvignon grape berries. Front. Plant Sci. 8, 53. doi: 10.3389/fpls.2017.00053
Li, H., Bai, Y., Yang, Y., Zheng, H., Xu, X., Li, H., et al. (2023). Transcriptomic analyses reveal light-regulated anthocyanin accumulation in ‘ZhongShan-HongYu’ grape berries. Scientia Hortic. 309, 111669. doi: 10.1016/j.scienta.2022.111669
Liang, T., Shi, C., Peng, Y., Tan, H., Xin, P., Yang, Y., et al. (2020). Brassinosteroid-activated BRI1-EMS-SUPPRESSOR 1 inhibits flavonoid biosynthesis and coordinates growth and UV-B stress responses in plants. Plant Cell 32, 3224–3239. doi: 10.1105/tpc.20.00048
Liu, L., Gregan, S., Winefield, C., Jordan, B. (2015). From UVR8 to flavonol synthase: UV-B-induced gene expression in Sauvignon blanc grape berry. Plant Cell Environ. 38, 905–919. doi: 10.1111/pce.12349
Liu, M.-y., Song, C. Z., Chi, M., Wang, T. M., Zuo, L. L., Li, X. L., et al. (2016). The effects of light and ethylene and their interaction on the regulation of proanthocyanidin and anthocyanin synthesis in the skins of Vitis vinifera berries. Plant Growth Regul. 79, 377–390. doi: 10.1007/s10725-015-0141-z
Loreti, E., Povero, G., Novi, G., Solfanelli, C., Alpi, A., Perata, P. (2008). Gibberellins, jasmonate and abscisic acid modulate the sucrose-induced expression of anthocyanin biosynthetic genes in Arabidopsis. New Phytol. 179, 1004–1016. doi: 10.1111/j.1469-8137.2008.02511.x
Loyola, R., Herrera, D., Mas, A., Wong, D. C. J., Hoell, J., Cavallini, E., et al. (2016). The photomorphogenic factors UV-B RECEPTOR 1, ELONGATED HYPOCOTYL 5, and HY5 HOMOLOGUE are part of the UV-B signalling pathway in grapevine and mediate flavonol accumulation in response to the environment. J. Exp. Bot. 67, 5429–5445. doi: 10.1093/jxb/erw307
Lu, S., Wang, J., Zhuge, Y., Zhang, M., Liu, C., Jia, H., et al. (2021). Integrative analyses of metabolomes and transcriptomes provide insights into flavonoid variation in grape berries. J. Agric. Food Chem. 69, 12354–12367. doi: 10.1021/acs.jafc.1c02703
Luan, L. Y., Zhang, Z. W., Xi, Z. M., Huo, S. S., Ma, L. N. (2013). Brassinosteroids regulate anthocyanin biosynthesis in the ripening of grape berries. S. Afr. J. Enol. Vitic. 34, 196–203. doi: 10.21548/34-2-1094
Martens, S., Teeri, T., Forkmann, G. (2002). Heterologous expression of dihydroflavonol 4-reductases from various plants. FEBS Lett. 531, 453–458. doi: 10.1016/s0014-5793(02)03583-4
Martinez-Gil, A. M., Gutierrez-Gamboa, G., Garde-Cerdan, T., Perez-Alvarez, E. P., Moreno-Simunovic, Y. (2018). Characterization of phenolic composition in Carignan noir grapes (Vitis vinifera L.) from six wine-growing sites in Maule Valley, Chile. J. Sci. Food Agric. 98, 274–282. doi: 10.1002/jsfa.8468
Matsuyama, S., Tanzawa, F., Kobayashi, H., Suzuki, S., Takata, R., Saito, H. (2014). Leaf Removal Accelerated Accumulation of Delphinidin-based Anthocyanins in ‘Muscat Bailey A’ Vitis x labruscana (Bailey) and Vitis vinifera (Muscat Hamburg) Grape Skin. J. Jpn. Soc Hortic. Sci. 83, 17–22. doi: 10.2503/jjshs1.CH-062
Matus, J. T., Cavallini, E., Loyola, R., Höll, J., Finezzo, L., Dal Santo, S., et al. (2017). A group of grapevine MYBA transcription factors located in chromosome 14 control anthocyanin synthesis in vegetative organs with different specificities compared with the berry color locus. Plant J. 91, 220–236. doi: 10.1111/tpj.13558
Matus, J. T., Poupin, M. J., Canon, P., Bordeu, E., Alcalde, J. A., Arce-Johnson, P. (2010). Isolation of WDR and bHLH genes related to flavonoid synthesis in grapevine (Vitis vinifera L.). Plant Mol. Biol. 72, 607–620. doi: 10.1007/s11103-010-9597-4
Meng, J.-F., Yu, Y., Shi, T.-C., Fu, Y.-S., Zhao, T., Zhang, Z.-W. (2019). Melatonin treatment of pre-veraison grape berries modifies phenolic components and antioxidant activity of grapes and wine. Food Sci. Technol. 39, 35–42. doi: 10.1590/1678-457x.24517
Mori, K., Goto-Yamamoto, N., Kitayama, M., Hashizume, K. (2007a). Loss of anthocyanins in red-wine grape under high temperature. J. Exp. Bot. 58, 1935–1945. doi: 10.1093/jxb/erm055
Mori, K., Goto-Yamamoto, N., Kitayama, M., Hashizume, K. (2007b). Effect of high temperature on anthocyanin composition and transcription of flavonoid hydroxylase genes in ‘Pinot noir’ grapes (Vitis vinifera). J. Horticult. Sci. Biotechnol. 82, 199–206. doi: 10.1080/14620316.2007.11512220
Mori, K., Saito, H., Goto-Yamamoto, N., Kitayama, M., Kobayashi, S., Sugaya, S., et al. (2005a). Effects of abscisic acid treatment and night temperatures on anthocyanin composition in Pinot noir grapes. Vitis 44, 161–165. doi: 10.1016/j.pnpbp.2004.11.023
Mori, K., Sugaya, S., Gemma, H. (2005b). Decreased anthocyanin biosynthesis in grape berries grown under elevated night temperature condition. Scientia Hortic. 105, 319–330. doi: 10.1016/j.scienta.2005.01.032
Mukherjee, A., Dwivedi, S., Bhagavatula, L., Datta, S. (2023). Integration of light and ABA signaling pathways to combat drought stress in plants. Plant Cell Rep. 42, 829–841. doi: 10.1007/s00299-023-02999-7
Murcia, G., Fontana, A., Pontin, M., Baraldi, R., Bertazza, G., Piccoli, P. N. (2017). ABA and GA3 regulate the synthesis of primary and secondary metabolites related to alleviation from biotic and abiotic stresses in grapevine. Phytochemistry 135, 34–52. doi: 10.1016/j.phytochem.2016.12.007
Olle, D., Guiraud, J. L., Souquet, J. M., Terrier, N., Ageorges, A., Cheynier, V., et al. (2011). Effect of pre- and post-veraison water deficit on proanthocyanidin and anthocyanin accumulation during Shiraz berry development. Aust. J. Grape Wine Res. 17, 90–100. doi: 10.1111/j.1755-0238.2010.00121.x
Osakabe, Y., Liang, Z. C., Ren, C., Nishitani, C., Osakabe, K., Wada, M., et al. (2018). CRISPR-Cas9-mediated genome editing in apple and grapevine. Nat. Protoc. 13, 2844–2863. doi: 10.1038/s41596-018-0067-9
Pastore, C., Dal Santo, S., Zenoni, S., Movahed, N., Allegro, G., Valentini, G., et al. (2017). Whole plant temperature manipulation affects flavonoid metabolism and the transcriptome of grapevine berries. Front. Plant Sci. 8, 929. doi: 10.3389/fpls.2017.00929
Reshef, N., Agam, N., Fait, A. (2018). Grape berry acclimation to excessive solar irradiance leads to repartitioning between major flavonoid groups. J. Agric. Food Chem. 66, 3624–3636. doi: 10.1021/acs.jafc.7b04881
Reynolds, A., Robbins, N., Lee, H. S., Kotsaki, E. (2016). Impacts and interactions of abscisic acid and gibberellic acid on sovereign coronation and skookum seedless table grapes. Am. J. Enol. Vitic. 67, 327–338. doi: 10.5344/ajev.2016.15108
Rienth, M., Vigneron, N., Darriet, P., Sweetman, C., Burbidge, C., Bonghi, C., et al. (2021). Grape berry secondary metabolites and their modulation by abiotic factors in a climate change scenario-A review. Front. Plant Sci. 12, 643258. doi: 10.3389/fpls.2021.643258
Rinaldo, A. R., Cavallini, E., Jia, Y., Moss, S. M. A., McDavid, D. A. J., Hooper, L. C., et al. (2015). A grapevine anthocyanin acyltransferase, transcriptionally regulated by VvMYBA, can produce most acylated anthocyanins present in grape skins. Plant Physiol. 169, 1897–1916. doi: 10.1104/pp.15.01255
Roby, G., Matthews, M. A. (2004). Relative proportions of seed, skin and flesh, in ripe berries from Cabernet Sauvignon grapevines grown in a vineyard either well irrigated or under water deficit. Aust. J. Grape Wine Res. 10, 74–82. doi: 10.1111/j.1755-0238.2004.tb00009.x
Santos, J. A., Fraga, H., Malheiro, A. C., Moutinho-Pereira, J., Dinis, L. T., Correia, C., et al. (2020). A review of the potential climate change impacts and adaptation options for european viticulture. Appl. Sciences-Basel 10, 3092. doi: 10.3390/app10093092
Savoi, S., Wong, D. C. J., Arapitsas, P., Miculan, M., Bucchetti, B., Peterlunger, E., et al. (2016). Transcriptome and metabolite profiling reveals that prolonged drought modulates the phenylpropanoid and terpenoid pathway in white grapes (Vitis vinifera L.). BMC Plant Biol. 16, 67. doi: 10.1186/s12870-016-0760-1
Shohat, H., Eliaz, N. I., Weiss, D. (2021). Gibberellin in tomato: metabolism, signaling and role in drought responses. Mol. horticulture 1, 15–15. doi: 10.1186/s43897-021-00019-4
Sun, R.-Z., Cheng, G., Li, Q., He, Y.-N., Wang, Y., Lan, Y.-B., et al. (2017). Light-induced variation in phenolic compounds in cabernet sauvignon grapes (Vitis vinifera L.) involves extensive transcriptome reprogramming of biosynthetic enzymes, transcription factors, and phytohormonal regulators. Front. Plant Sci. 8, 547. doi: 10.3389/fpls.2017.00547
Sun, Y., Liu, Q., Xi, B., Dai, H. (2019). Study on the regulation of anthocyanin biosynthesis by exogenous abscisic acid in grapevine. Scientia Hortic. 250, 294–301. doi: 10.1016/j.scienta.2019.02.054
Terrier, N., Torregrosa, L., Ageorges, A., Vialet, S., Verries, C., Cheynier, V., et al. (2009). Ectopic expression of VvMybPA2 promotes proanthocyanidin biosynthesis in grapevine and suggests additional targets in the pathway. Plant Physiol. 149, 1028–1041. doi: 10.1104/pp.108.131862
Torres, N., Martinez-Luscher, J., Porte, E., Kurtural, S. K. (2020). Optimal ranges and thresholds of grape berry solar radiation for flavonoid biosynthesis in warm climates. Front. Plant Sci. 11. doi: 10.3389/fpls.2020.00931
Tyagi, K., Maoz, I., Kochanek, B., Sela, N., Lerno, L., Ebeler, S. E., et al. (2021). Cytokinin but not gibberellin application had major impact on the phenylpropanoid pathway in grape. Horticulture Res. 8, 51. doi: 10.1038/s41438-021-00488-0
VanderWeide, J., Falchi, R., Calderan, A., Peterlunger, E., Vrhovsek, U., Sivilotti, P., et al. (2022). Juxtaposition of the Source-to-Sink Ratio and Fruit Exposure to Solar Radiation on cv. Merlot (Vitis vinifera L.) Berry Phenolics in a Cool versus Warm Growing Region. J. Agric. Food Chem. 70, 10429–10442. doi: 10.1021/acs.jafc.2c01528
Vergara, A. E., Díaz, K., Carvajal, R., Espinoza, L., Alcalde, J. A., Pérez-Donoso, A. G. (2018). Exogenous applications of brassinosteroids improve color of red table grape (Vitis vinifera L. Cv. “Redglobe”) berries. Front. Plant Sci. 9, 363. doi: 10.3389/fpls.2018.00363
Villalobos-González, L., Peña-Neira, A., Ibáñez, F., Pastenes, C. (2016). Long-term effects of abscisic acid (ABA) on the grape berry phenylpropanoid pathway: Gene expression and metabolite content. Plant Physiol. Biochem. 105, 213–223. doi: 10.1016/j.plaphy.2016.04.012
Wang, P. Q., Liu, Y. J., Zhang, L. J., Wang, W. Z., Hou, H., Zhao, Y., et al. (2020a). Functional demonstration of plant flavonoid carbocations proposed to be involved in the biosynthesis of proanthocyanidins. Plant J. 101, 18–36. doi: 10.1111/tpj.14515
Wang, Q., Yu, F., Xie, Q. (2020b). Balancing growth and adaptation to stress: Crosstalk between brassinosteroid and abscisic acid signaling. Plant Cell Environ. 43, 2325–2335. doi: 10.1111/pce.13846
Wei, X. F., Wang, W. Y., Min, Z., Li, Z. Y., Ouyang, Y. N., Ruan, X. R., et al. (2023). Transcriptomics combined with metabolisms reveals the effect of light-exclusive films on the quality and polyphenols of ‘Cabernet Sauvignon’ grapes. Food Res. Int. 170, 112754. doi: 10.1016/j.foodres.2023.112754
Winkel-Shirley, B. (2002). Biosynthesis of flavonoids and effects of stress. Curr. Opin. Plant Biol. 5, 218–223. doi: 10.1016/s1369-5266(02)00256-x
Wu, J., Drappier, J., Hilbert, G., Guillaumie, S., Dai, Z. W., Geny, L., et al. (2019). The effects of a moderate grape temperature increase on berry secondary metabolites. Oeno One 53, 321–333. doi: 10.20870/oeno-one.2019.53.2.2434
Xi, Z. M., Zhang, Z. W., Huo, S. S., Luan, L. Y., Gao, X., Ma, L. N., et al. (2013). Regulating the secondary metabolism in grape berry using exogenous 24-epibrassinolide for enhanced phenolics content and antioxidant capacity. Food Chem. 141, 3056–3065. doi: 10.1016/j.foodchem.2013.05.137
Xie, D. Y., Sharma, S. B., Paiva, N. L., Ferreira, D., Dixon, R. A. (2003). Role of anthocyanidin reductase, encoded by BANYULS in plant flavonoid biosynthesis. Science 299, 396–399. doi: 10.1126/science.1078540
Xu, W. J., Dubos, C., Lepiniec, L. (2015). Transcriptional control of flavonoid biosynthesis by MYB-bHLH-WDR complexes. Trends Plant Sci. 20, 176–185. doi: 10.1016/j.tplants.2014.12.001
Yamane, T., Jeong, S. T., Goto-Yamamoto, N., Koshita, Y., Kobayashi, S. (2006). Effects of temperature on anthocyanin biosynthesis in grape berry skins. Am. J. Enol. Vitic. 57, 54–59. doi: 10.5344/ajev.2006.57.1.54
Yan, Y. F., Song, C. Z., Falginella, L., Castellarin, S. D. (2020). Day temperature has a stronger effect than night temperature on anthocyanin and flavonol accumulation in ‘Merlot’ (Vitis vinifera L.) grapes during ripening. Front. Plant Sci. 11. doi: 10.3389/fpls.2020.01095
Yang, B., He, S., Liu, Y., Liu, B. C., Ju, Y. L., Kang, D. Z., et al. (2020a). Transcriptomics integrated with metabolomics reveals the effect of regulated deficit irrigation on anthocyanin biosynthesis in Cabernet Sauvignon grape berries. Food Chem. 314, 126170. doi: 10.1016/j.foodchem.2020.126170
Yang, B., Yao, H., Zhang, J., Li, Y., Ju, Y., Zhao, X., et al. (2020b). Effect of regulated deficit irrigation on the content of soluble sugars, organic acids and endogenous hormones in Cabernet Sauvignon in the Ningxia region of China. Food Chem. 312, 126020. doi: 10.1016/j.foodchem.2019.126020
Yu, K., Dixon, R. A., Duan, C. (2022). A role for ascorbate conjugates of (+)-catechin in proanthocyanidin polymerization. Nat. Commun. 13, 3425. doi: 10.1038/s41467-022-31153-2
Yu, K., Jun, J. H., Duan, C., Dixon, R. A. (2019). VvLAR1 and VvLAR2 are bifunctional enzymes for proanthocyanidin biosynthesis in grapevine. Plant Physiol. 180, 1362–1374. doi: 10.1104/pp.19.00447
Yu, K., Song, Y., Lin, J., Dixon, R. A. (2023). The complexities of proanthocyanidin biosynthesis and its regulation in plants. Plant Commun. 4, 100498. doi: 10.1016/j.xplc.2022.100498
Yu, R., Cook, M. G., Yacco, R. S., Watrelot, A. A., Gambetta, G., Kennedy, J. A., et al. (2016). Effects of Leaf Removal and Applied Water on Flavonoid Accumulation in Grapevine (Vitis vinifera L. cv. Merlot) Berry in a Hot Climate. J. Agric. Food Chem. 64, 8118–8127. doi: 10.1021/acs.jafc.6b03748
Zhang, C., Dai, Z., Ferrier, T., Orduna, L., Santiago, A., Peris, A., et al. (2023). MYB24 orchestrates terpene and flavonol metabolism as light responses to anthocyanin depletion in variegated grape berries. Plant Cell. 35, 4238–4265. doi: 10.1093/plcell/koad228
Zhou, Y., Yuan, C., Ruan, S., Zhang, Z., Meng, J., Xi, Z. (2018). Exogenous 24-epibrassinolide interacts with light to regulate anthocyanin and proanthocyanidin biosynthesis in cabernet sauvignon (Vitis vinifera L.). Molecules 23, 93. doi: 10.3390/molecules23010093
Zhu, Z., Li, G., Liu, L., Zhang, Q., Han, Z., Chen, X., et al. (2019). A R2R3-MYB transcription factor, VvMYBC2L2, functions as a transcriptional repressor of anthocyanin biosynthesis in grapevine (Vitis vinifera L.). Molecules 24, 92. doi: 10.3390/molecules24010092
Keywords: proanthocyanidin, anthocyanin, flavonoid, water, light, temperature, phytohormones, grape
Citation: Shi T, Su Y, Lan Y, Duan C and Yu K (2024) The molecular basis of flavonoid biosynthesis response to water, light, and temperature in grape berries. Front. Plant Sci. 15:1441893. doi: 10.3389/fpls.2024.1441893
Received: 31 May 2024; Accepted: 08 August 2024;
Published: 27 August 2024.
Edited by:
Deyu Xie, North Carolina State University, United StatesReviewed by:
Nan Lu, University of North Texas, United StatesChaofeng Li, Southwest University, China
Xinlong Dai, Guizhou University, China
Copyright © 2024 Shi, Su, Lan, Duan and Yu. This is an open-access article distributed under the terms of the Creative Commons Attribution License (CC BY). The use, distribution or reproduction in other forums is permitted, provided the original author(s) and the copyright owner(s) are credited and that the original publication in this journal is cited, in accordance with accepted academic practice. No use, distribution or reproduction is permitted which does not comply with these terms.
*Correspondence: Keji Yu, yukeji@cau.edu.cn