- 1Department of Biology, College of Science, United Arab Emirates University, Abu Dhabi, United Arab Emirates
- 2Key Laboratory of Land Surface Pattern and Simulation, Institute of Geographic Sciences and Natural Resources Research, Chinese Academy of Sciences (CAS), Beijing, China
- 3Department of Biosciences, Integral University, Lucknow, Uttar Pradesh, India
- 4Department of Botany and Microbiology, College of Science, King Saud University, Riyadh, Saudi Arabia
In the current investigation, the combination of selenium (Se) and epibrassinolide (EBL) exhibited a promising alleviative response against the concurrent stress of heat and drought in wheat plants. The compromised growth and photosynthetic performance of wheat plants under the combined stress of heat and drought were substantially improved with the treatment involving Se and EBL. This improvement was facilitated through the expression of Q9FIE3 and O04939 proteins, along with enhanced antioxidant activities. The heightened levels of antioxidant enzymes and the accumulation of osmoprotectant proline helped mitigate the overaccumulation of reactive oxygen species (ROS), including electrolyte leakage, H2O2 accumulation, and lipid peroxidation, thus conferring tolerance against the combined stress of heat and drought. Studies have demonstrated that Se and EBL can assist wheat plants in recuperating from the adverse effects of heat and drought. As such, they are essential components of sustainable farming methods that aim to increase crop productivity.
1 Introduction
Plants frequently encounter many stressors at the same time, resulting in significant yield losses. As a result, it is critical to investigate the physiological and molecular mechanisms that allow plants to protect themselves against a variety of challenges. According to recent studies, plant responses to combined stresses differ from those to separate stressors and vary depending on the plant species (Zhang and Sonnewald, 2017). Moreover, plants exhibit shared reactions to stimuli and various combinations of stress factors (Zhang and Sonnewald, 2017). To delve into the distinct and widespread mechanisms governing how plants react to individual and concurrent abiotic stressors, we explored the effects of heat stress and drought, both independently and in combination, on physiological and molecular processes.
Drought and heat are two major abiotic stresses constraining wheat productivity worldwide, causing yield losses of up to 86% and 69%, respectively. Both stresses individually and in combination have a variety of negative effects since they share a lot of physiological characteristics (Prasad et al., 2011). It has recently been demonstrated that Se plays an important function in controlling plant development in both normal and severe environments. Because of its positive role in reducing the negative effects of stressors, plants require Se for development and maintenance (Yusuf et al., 2016). Selenite remains bound to the soil and is readily taken up by plants, unlike selenate, which quickly dissolves from the soil, making it unavailable for plant uptake (Fio et al., 1991). Conversely, selenium is a vital trace element necessary for plant survival, protecting detrimental environmental conditions (Feng and Wei, 2012). Selenium has been found to confer resistance to various environmental stresses such as heavy metals, drought, salinity, high temperatures, as well as bacterial and fungal infections (Schiavon et al., 2017; Muhammad et al., 2024). At low concentrations and suitable doses, it serves as an antioxidant; nevertheless, at excessive concentrations, it acts as a prooxidant (Feng and Wei, 2012; Mroczek-Zdyrska and Wójcik, 2012; Cittrarasu et al., 2021; Ikram et al., 2021). Furthermore, the plastid membrane and chloroplast need to maintain their shape and fluidity (Diao et al., 2014). Additionally, Se can promote photosynthesis (Gupta and Gupta, 2017), postpone senescence (Xue et al., 2001), and boost plant production at low concentrations (Germ et al., 2005). Other crops treated with selenium, such as B. juncea (Yusuf et al., 2016), Lolium perenne (Hartikainen et al., 2000), S. tuberosum (Turakainen et al., 2004), and Lens culinaris (Lyons et al., 2009), exhibited similar outcomes (Ekanayake et al., 2015). Brassinosteroids (BRs) represent a novel group of polyhydroxy steroidal hormones identified in plants, serving as intrinsic signals governing plant growth and expansion (Vidya Vardhini, 2017). BRs influence various developmental and physiological processes including seed germination, flowering, hypocotyl elongation, and more (Kim and Wang, 2010; Fariduddin et al., 2011; Mir et al., 2015; Dong et al., 2017; Hussain et al., 2019). Additionally, BRs exhibit the capacity to alleviate abiotic stresses such as chilling stress (Fariduddin et al., 2011), metal stress (Mir et al., 2015), elevated temperature (Hussain et al., 2019), and drought stress (Yusuf et al., 2024). Furthermore, 24-epibrassinolide (EBL), an analogue of BR, provides protective effects against environmental factors like heat stress, drought, low temperature, heavy metals, and salinity (Ali et al., 2007; Farooq et al., 2009; Khan et al., 2015; Khan et al., 2017; Khan et al., 2019; Hussain et al., 2019; Yusuf et al., 2024).
The objective of this study was to uncover the combined effect of heat and drought stress in wheat plants on growth and photosynthetic performance, antioxidant enzymes and differential expression pattern stress-responsive proteins. Additionally, dissects out stress tolerance mechanisms associated with selenium and/or brassinosteroids under combined stress of heat and drought and unlocks their contribution to the developing strategy for improving crop production under multiple stresses at a time.
2 Materials and methods
2.1 Biological material
High-quality and uniform-sized seeds of Triticum aestivum (wheat) cv. PBW-373 underwent surface sterilization using a half-strength standard treatment solution (composed of 15% commercial bleach and 0.01% Triton X-100) for a duration of 15 minutes. Subsequently, they were thoroughly rinsed with deionized water multiple times to remove any remaining traces of the sterilizing agents that may have adhered to the seed surface.
2.2 Hormone and selenium preparation
24-Epibrassinolide (EBL) was procured from Sigma–Aldrich USA. A stock solution with a concentration of 10-4 M was prepared and subsequently diluted to achieve the desired experimental concentrations of 10-8 M, as per the methodology outlined by Khan et al. (2015). Additionally, Tween-20 was employed as a surfactant by mixing with EBL before the application. Sodium selenate (Na2SeO4) served as the selenium source, with a required concentration of 10 μM prepared by diluting a stock solution (1.0 mM) of Se, a concentration chosen based on findings from our previous study (Naz et al., 2015).
2.3 Experimental design with treatment pattern
The surface sterilized seeds were sown in forty plastic pots, all equal size (10 inches in diameter) and volume filled with commercial potting mix and farmyard manure. These pots were arranged in a greenhouse under environmentally controlled conditions. These pots were then divided into eight sets, each containing five pots, serving as replicates for each treatment. In set I: Plants received no stress, EBL, or Se treatment, but foliage was sprayed with deionized water (served as control) at 13, 14, and 15 days of growth. Set II: Plants were exposed to 10 μM of Se through the soil at 10, 11, and 12 days of growth, and foliage was sprayed with 10-8 M of EBL at 13, 14, and 15 days of growth. Set III: Plants experienced a high day/night temperature of 40/35°C with a 12-hour photoperiod and 60–75% relative humidity at 20, 21, and 22 days of growth in a plant growth chamber. Set IV: Drought stress was induced by exposing plants to 15% PEG through the soil at 24, 25, and 26 days of growth. Set V – a combination of sets III and IV. Set VI – a combination of sets II and III. Set VII – a combination of sets II and IV. Set VIII – a combination of sets II, III, and IV. At the 45-day growth stage, plants from all sets were harvested to evaluate various growth parameters, leaf gas exchange traits, biochemical parameters, and protein expression. Each assay was repeated five times, with 15 plants utilized per treatment (three plants per pot).
2.4 Plant growth analysis
The plants were carefully removed from the pots, along with the potting mix, and subsequently rinsed with gentle running tap water to remove soil from the roots. The lengths of both the roots and shoots were then measured using a meter scale. Afterward, the plants were dried using tissue towels and placed in an oven set at 70°C for 72 hours. Following the drying process, the plant samples were allowed to cool to room temperature before their dry mass was recorded.
Leaf area was determined using a leaf area meter (AM 350, ADC Bioscientific, UK).
2.5 Leaf relative water content
Relative water content of leaf was determined by the method described by Fariduddin et al. (2014) by using the formula:
2.6 Total chlorophyll content
Total chlorophyll content was assessed following the procedure described by Arnon (1949).
2.7 Determination of photosynthetic traits
To evaluate different photosynthetic characteristics including the net photosynthetic rate (PN) and stomatal conductance (gs), fully expanded leaves were chosen between 11:00 and 12:00 h. An infrared gas analyzer (IRGA) portable photosynthetic system (LI-COR 6400, LI-COR, Lincoln, NE, USA) was utilized for measurements. The environmental conditions such as air temperature, relative humidity, CO2 concentration, and photosynthetic photon flux density (PPFD) were maintained at 25°C, 85%, 600 µmol mol-1, and 800 µmol mol-2 s-1, respectively.
2.8 Determination of rubisco and carbonic anhydrase activity
Rubisco activity was assessed spectrophotometrically at 340 nm, observing the oxidation of NADH at 30°C, following the methodology outlined in our prior research (Yusuf et al., 2021). The assessment of carbonic anhydrase activity was conducted following the procedures detailed in Naz et al. (2015).
2.9 Assessment of stress biomarker
Lipid peroxidation, electrolyte leakage, and H2O2 content in all plant samples were quantified following the methodology outlined by Yusuf et al. (2021).
2.10 Assessment of protein profile and content, antioxidant enzymes, and proline accumulation
Assessment of protein content, and profile determination of antioxidant enzymes, and proline accumulation were performed according to the method mentioned in our previous study (Khan et al., 2019; Yusuf et al., 2021).
2.11 Statistical analysis
The data collected from the experiment underwent statistical analysis through analysis of variance (ANOVA) using IBM SPSS Statistics for Windows, Version 19.0 (IBM Corp., Armonk, NY). Results were expressed as treatment mean ± SE, and treatment means were compared utilizing the least significant difference (LSD) test at a significance level of p ≤ 0.05.
3 Experimental results
3.1 Growth and biomass yield
Combined stress of heat and drought as well as EBL and Se showed significant effect on wheat plant height and dry biomass. However, exposure to heat stress at temperatures of 45/35°C resulted in a reduction of shoot length by 20% and root length by 25.6% compared to their respective control conditions. Moreover, when both EBL and Se were administered concurrently, there was a notable increase in shoot and root length, regardless of the stress treatments. Furthermore, the combined application of EBL and Se led to a significant enhancement in shoot length (34.4%) and root length (46.1%) compared to control plants. This combination effectively alleviated the impact of combined heat and drought stress, resulting in greater increases in plant length and dry mass compared to plants subjected to heat stress (Figure 1).
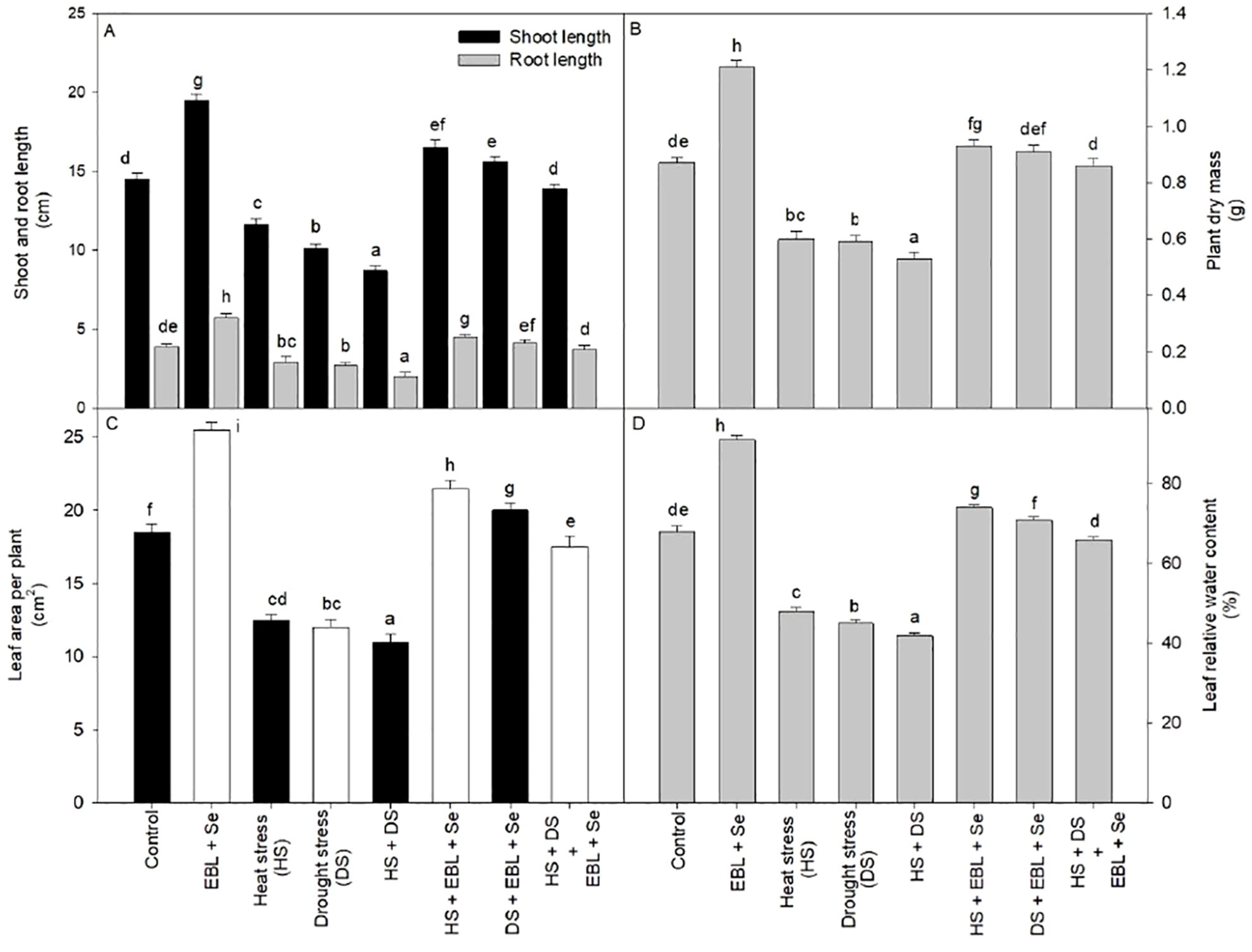
Figure 1. Effect of 24-epibrassinolide (EBL) in combination with selenium (Se) induced changes in (A) shoot and root length, (B) dry mass of plant, (C) leaf area per plant, and (D) leaf relative water content under combined stress of heat and drought at 45 days after sowing. All the data are the mean of five replicates (n=5) and vertical bars shows standard errors (± SE). Different letters indicate a significant difference between control and treatment by LSD test p ≤ 0.05.
Similar pattern of result observed in terms of leaf area of plants.
3.2 Leaf relative water content
In the presence of heat, drought, or both stresses, plants demonstrated diminished relative water content in comparison to the control group. The most pronounced decline in leaf water content occurred in plants exposed to both heat and drought simultaneously, with a reduction of 38.2% compared to untreated control plants (Figure 1D). Conversely, the simultaneous application of EBL and Se successfully countered the loss of leaf relative water content, elevating it by 33.8% compared to the control plants.
3.3 Total chlorophyll content
When heat and dryness were combined, the plants’ chlorophyll content dropped by 38.4% when compared to the control group. On the other hand, compared to control plants, the combined application of EBL and Se dramatically raised the chlorophyll content by 37.6% (Figure 2A). Furthermore, the co-administration of EBL and Se significantly mitigated the reduction in chlorophyll levels caused by the combined effects of drought and heat stress.
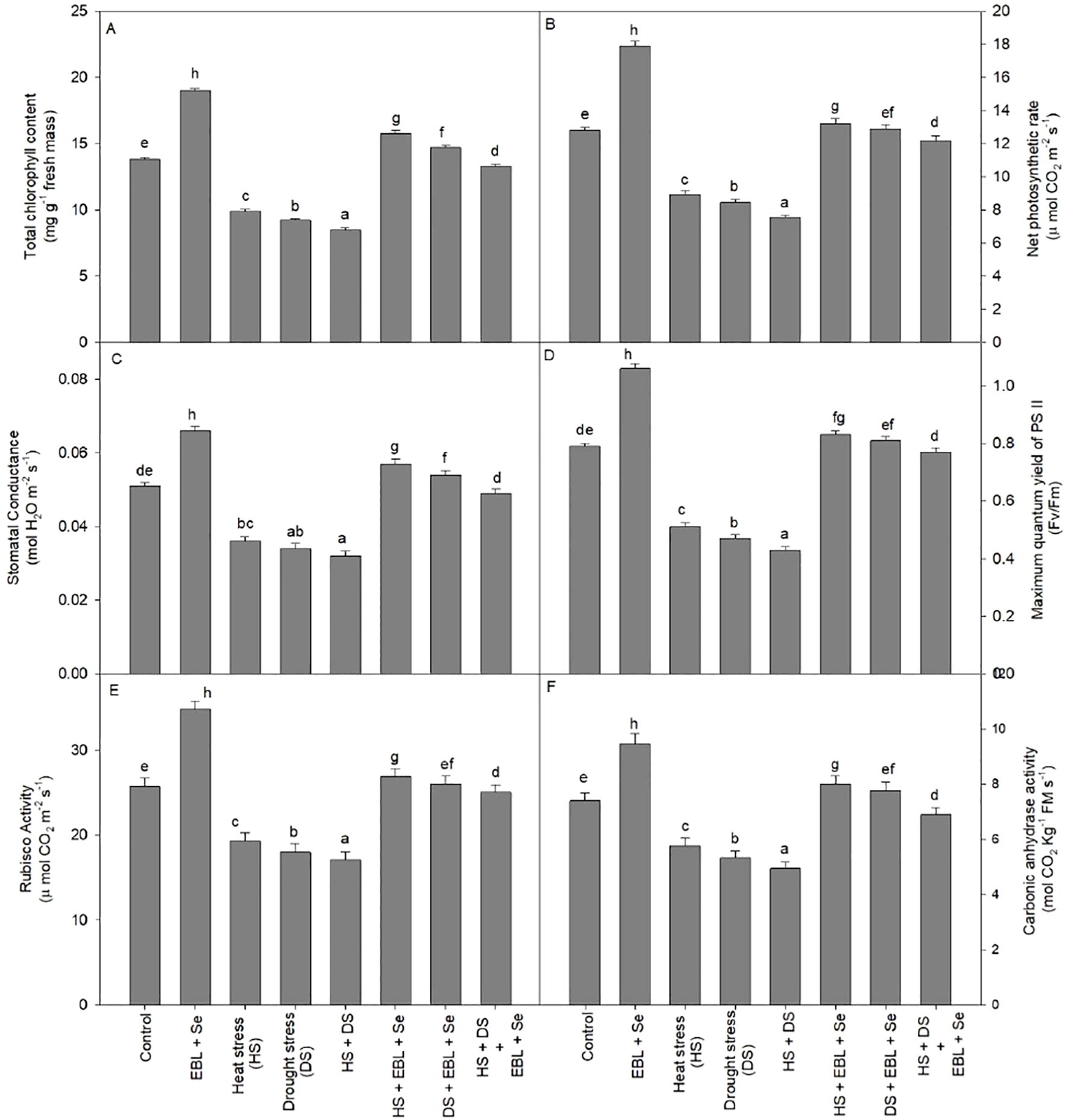
Figure 2. Effect of 24-epibrassinolide (EBL) in combination with selenium (Se) induced changes in (A) total chlorophyll content, (B) net photo synthetic rate, (C) stomatal conductance, (D) maximum quantum yield of PSII, (E) Rubisco activity, and (F) carbonic anhydrase activity under combined stress of heat and drought at 45 days after sowing. All the data are the mean of five replicates (n=5) and vertical bars shows standard errors (± SE). Different letters indicate a significant difference between control and treatment by LSD test p≤0.05.
3.4 Performance of photosynthetic traits
Exposure to heat, drought, or their combination resulted in notable decreases in net photosynthetic rate and stomatal conductance compared to their respective control plants (Figures 2B, C). The most significant reductions in net photosynthetic rate (41.4%) and stomatal conductance (37.2%) were observed in plants experiencing both heat and drought stress simultaneously. However, the concurrent application of EBL and selenium effectively addressed the challenges posed by heat and drought, restoring both net photosynthetic rate and stomatal conductance. Additionally, under non-stress conditions, the combined use of EBL and Se augmented photosynthetic characteristics beyond those observed in control plants.
The maximum quantum yield of PSII (Fv/Fm) similarly responded, protecting PSII machinery through the supplementation of EBL + Se under stressful conditions in wheat plants.
3.5 Rubisco and carbonic anhydrase activity
When compared to control plants, plants under heat and/or drought stress showed noticeably lower Rubisco activity (Figure 2E). On the other hand, Rubisco activity was greatly increased when EBL and Se were administered in combination to plants that were stressed by heat and drought. The combination showed the largest increase, with Rubisco activity rising by 35.4% in comparison to control plants. In addition, the combination treatment of Se and EBL mitigated the negative effects of heat and drought, allowing Rubisco to improve its performance.
The CA activity significantly increased by the combination of EBL and Se treatments, as shown in Figure 2F, and was greater by 27.9% above the control plants. However, compared to the control plants, CA activity reduced integrity by 33.1% when heat and drought stressors were present. Furthermore, in heat and drought-stressed plants, the combination of Se and EBL enhanced the activity of CA, restoring its integrity.
3.6 Electrolyte leakage
Plants displayed to heat and drought stress produced more EL when compared to their respective controls, (39.2%). In non-stressed plants, however, the application of EBL and Se lowered the EL by 15.07%, respectively, when compared to the controls (Figure 3A). Furthermore, applying EBL and Se to plants that had been showing to heat and drought stress helped to partially reverse ion leakage.
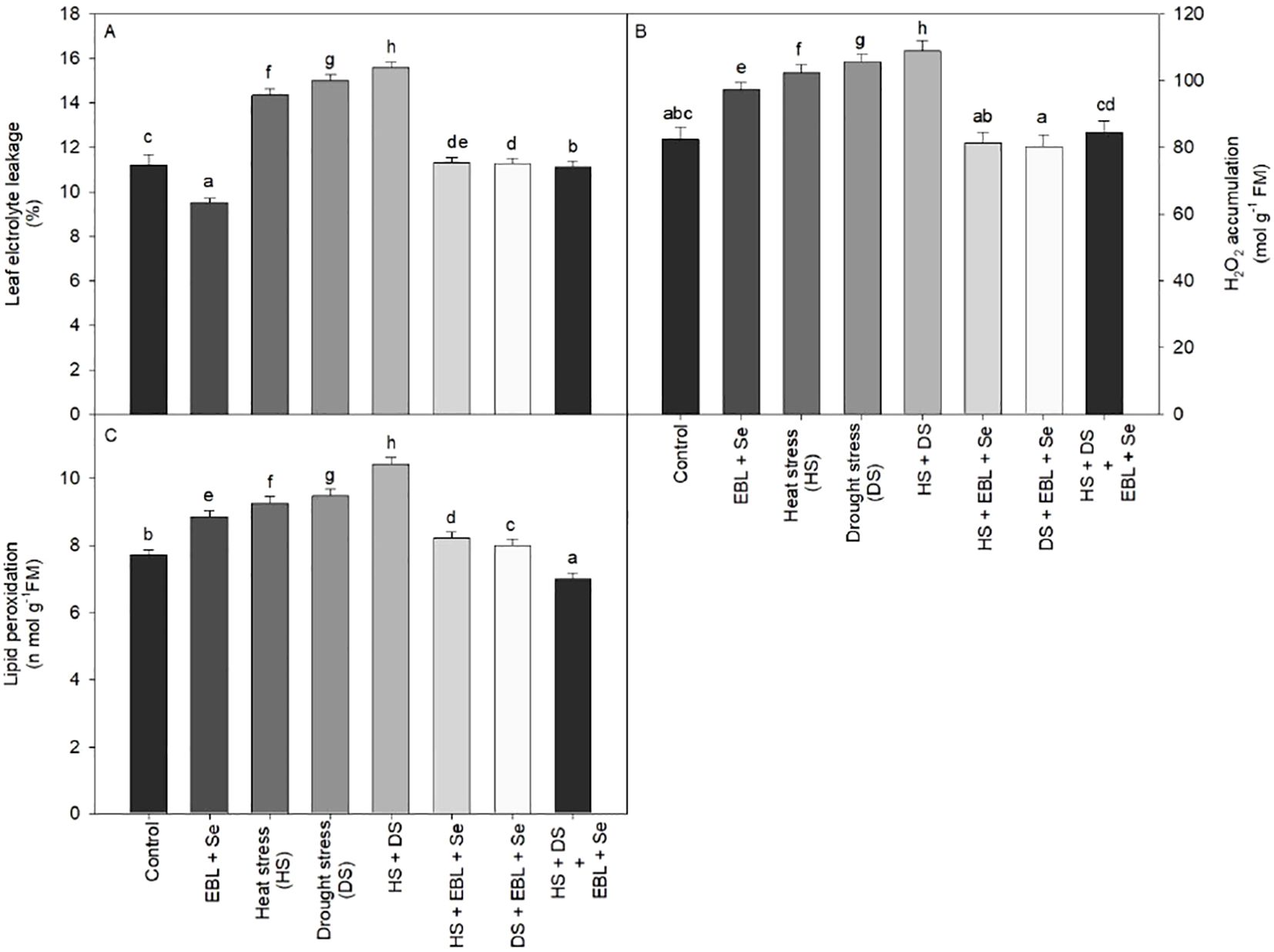
Figure 3. Effect of 24-epibrassinolide (EBL) in combination with selenium (Se) induced changes in (A) leaf electrolyte leakage, (B) H2O2 accumulation, and (C) lipid peroxidation under combined stress of heat and drought at 45 days after sowing. All the data are the mean of five replicates (n=5) and vertical bars shows standard errors (± SE). Different letters indicate a significant difference between control and treatment by LSD test p≤0.05.
3.7 Lipid peroxidation and H2O2 accumulation
As plants were exposed to heat and drought stress single or in combination, both lipid peroxidation and H2O2 buildup in leaves in contrast to their corresponding control group (Figures 3B, C). Heat and drought stressed plants increased LPO and H2O2 by 35.01% and. 31.99%, in compared to the control, respectively. However, LPO and H2O2 accumulation decreased in heat and drought-stressed plants when BR and Se were applied in combination as a follow-up treatment.
3.8 Protein content
When EBL + Se was given to the plants, the amount of protein in the leaves increased significantly—by 37.9% when compared to the control plants (Figure 4C). On the other hand, plants under stress from heat and dryness had significantly less protein than the corresponding control plants. Furthermore, the combination of Se and EBL effectively reduced the negative effects of heat and drought while increasing protein levels above those of the control plants.
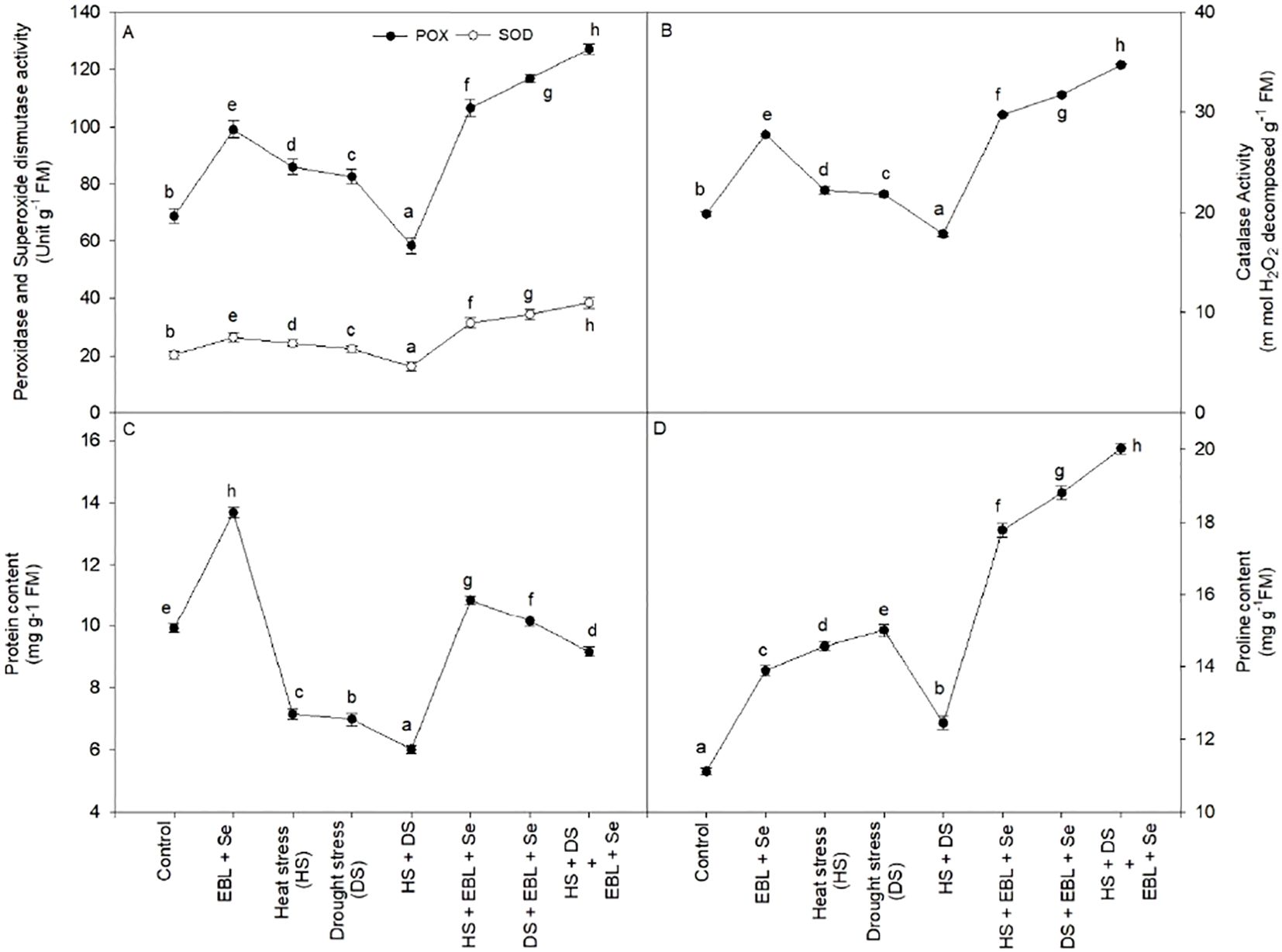
Figure 4. Effect of 24-epibrassinolide (EBL) in combination with selenium (Se) induced changes in (A) peroxidase and superoxide dismutase activity, (B) catalase activity, (C) protein content, and (D) proline content under combined stress of heat and drought at 45 days after sowing. All the data are the mean of five replicates (n=5) and vertical bars shows standard errors (± SE). Different letters indicate a significant difference between control and treatment by LSD test p ≤ 0.05.
3.9 Activities of catalase, peroxidase, and superoxide dismutase
The activity of the enzymes CAT, POX, and SOD increased dramatically in response to heat and/or drought stress as compared to the comparable control plants. However, after being subjected to a combination of heat and drought stress, wheat plants treated with EBL and Se showed the greatest increases in CAT, POX, and SOD activities (Figures 4A, B). When CAT, POX, and SOD readings were compared to the equivalent control plants, the increases were 75.0%, 84.9%, and 89.9%, respectively.
3.10 Proline content
Under the stresses of heat and drought, the leaves’ proline content rose (Figure 4D). In heat and drought stress conditions, proline levels increased relative to the control. Moreover, the proline content was enhanced much more after the EBL and Se treatments. The plants that were treated to heat and drought stress and then given EBL and Se treatment accumulated the maximum proline content (80.1%) as compared to their respective control plants.
3.11 Impact of treatments on protein profile
A varying pattern of protein expression was recorded during the comparison of protein profiles. Each treatment boosted different protein species in the plant cell. HS enhanced the expression of P82280 protein up to 80%. Whereas the protein P42056 contents were enhanced 80.7% due to HS. Protein species P42056 and Q9S795 were enhanced more than 100% (i.e., 114.5, and 107.4%, respectively) after exposing the plants with DS. Only one protein (O04939) exhibited the expression level more than 100% after having Se treatment. Selenium could elevate the expression of two protein species more than 100%, i.e., Q9FIE3 (115.7%) and O04939 (102.2%). However, an elevation of 95.4% was recorded in the protein P42849 after Se treatment. The PCA analysis screened the protein species with strongest correlation coefficients with all the external factors, i.e., HS, DS, EBL, and Se. HS had the strongest correlation coefficient of 2.3 with P82280 protein. However, P42056 exhibited the highest correlation coefficient with DS (Figure 5).
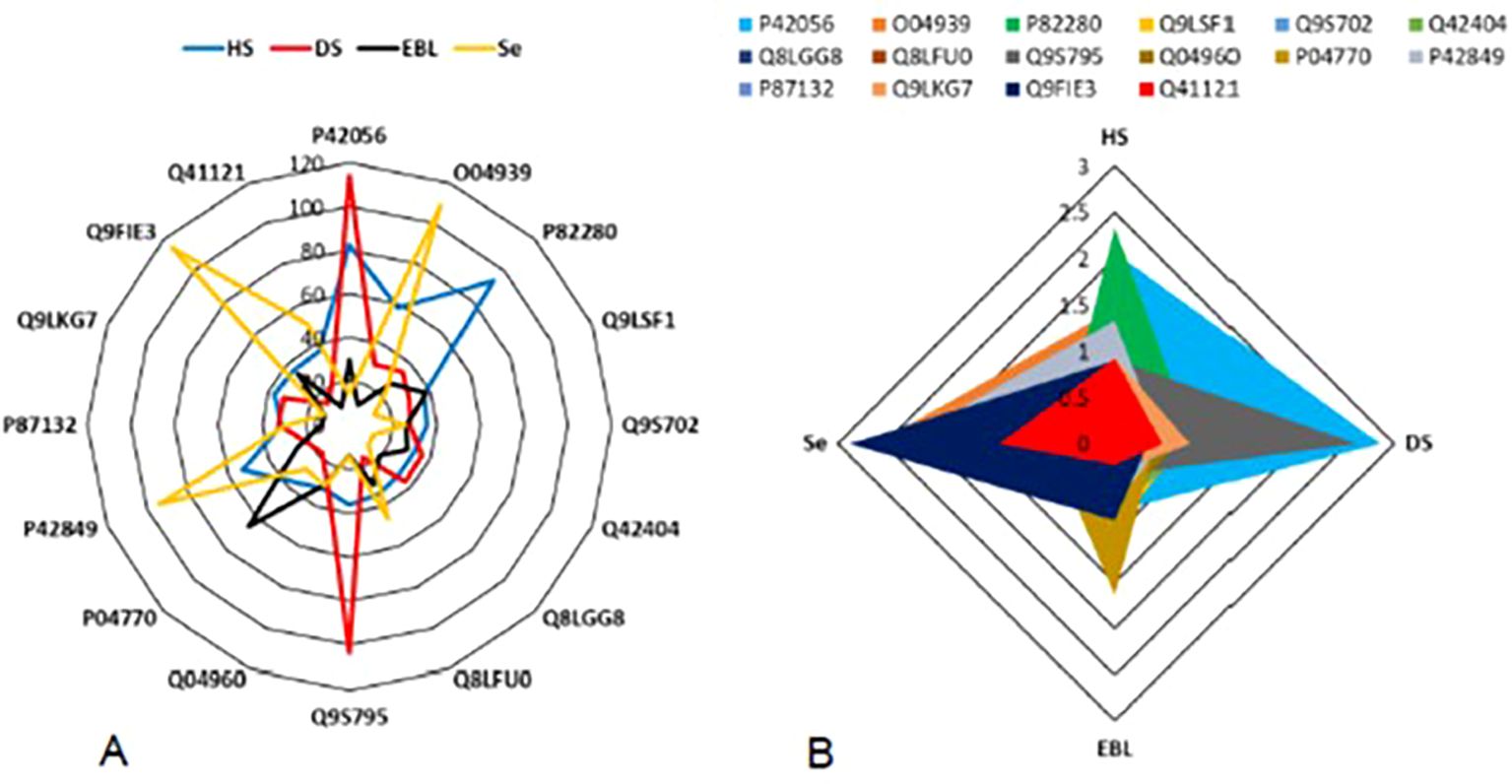
Figure 5. Impact of plant stressors, i.e., heat stress (HS) and drought stress (DS), and the stress alleviators i.e., epibrassinolide (EBL), and selenium (Se) on protein profile of the plant (A). The correlation-based screening of differentially expressed proteins representing the affinity of the protein species with external factors (B).
3.12 Structural analysis of protein
The position of phi (φ) and psi (ψ) angles illustrated the detailed activity and stability of the protein species. Overall, protein species P42056 had a significantly higher number of C-N-C angles than protein species P82280. It showed the increased number of active sites on the outer surface of the globular protein. However, the structure of the P82280 protein was more stable than the P42056. Its stability was evident from its small structure, energy level, and the presence of a higher number of proline residues in the structure. Glycine residues with C-N-C bonding were also more in number in the P42056 as compared to protein species P82280. Protein P82280 exhibited no C-Ca-C proline residue (Figure 6). In addition to this, protein species Q9FIE3 had a linear structure with one lobed end. Its long linear structure made it an unstable structure with 0.5 QMean energy score. There was more density of phi (φ) and psi (ψ) angles in protein Q9FIE3. Furthermore, the ratio of N-C-N angles was significantly higher than C-Ca-C angles. All the glycine residues were detected in the upper two quadrates (1st and 4th) of the Ramachandran plot. Similarly, proline and preproline residues were present in the 3rd and 4th quadrates. Protein species O04939 had a globular and stable structure. It’s QMean score was 0.78. The preproline contents were detected in the 3rd quadrate only (Figure 7).
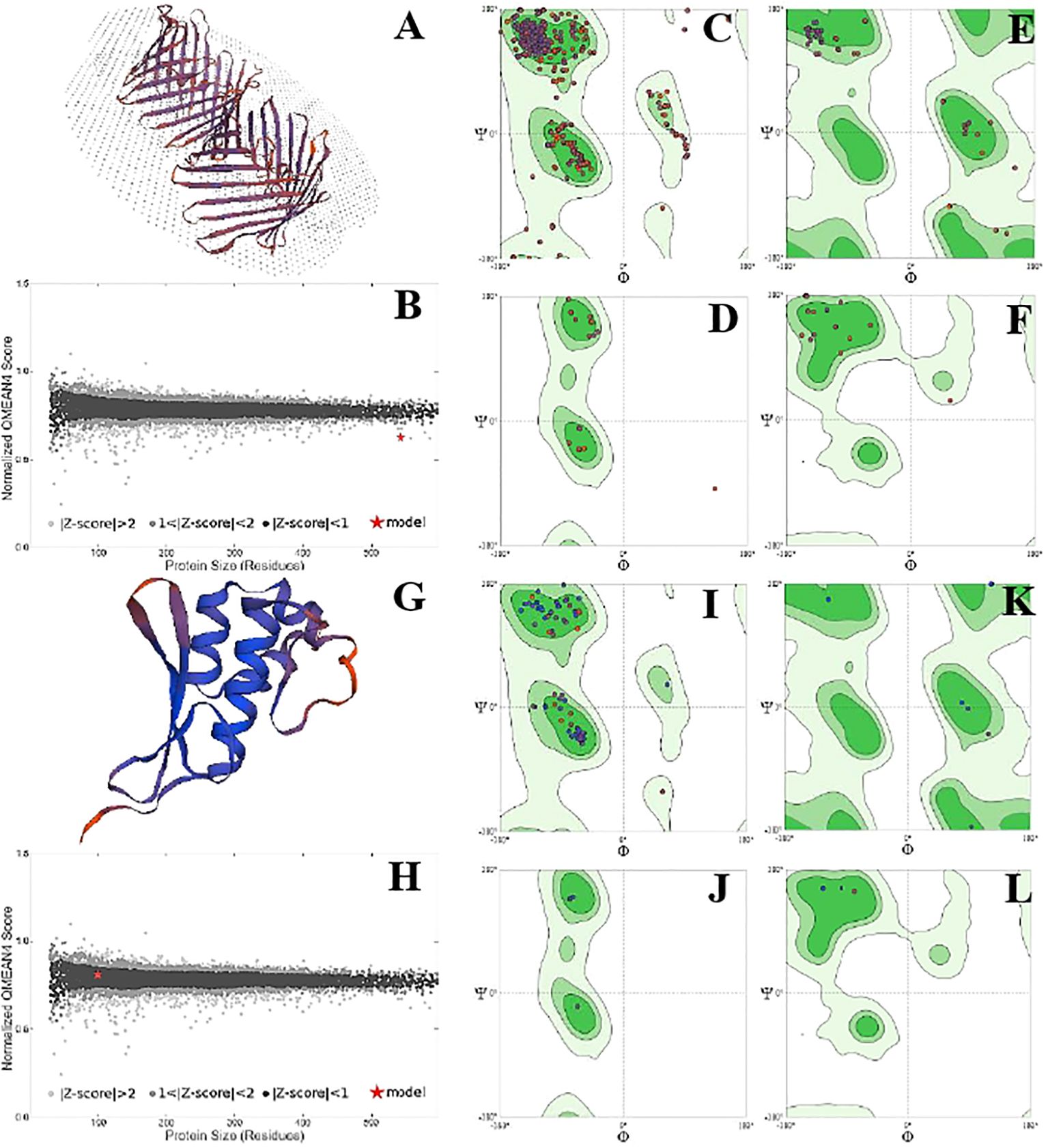
Figure 6. Structural analysis of protein P42056. Structural illustration of protein in ribbon model (A), energy map (B), the position of phi (φ) and psi (ψ) angles in the 3D structure (C), The position of preproline (D), the position of glycine (E), and the position of proline (F). Structural analysis of protein P82280. Structural illustration of protein in ribbon model (G), energy map (H), the position of phi (φ)and psi (ψ) angles in the 3D structure (I), The position of preproline (J), the position of glycine (K), and the position of proline (L).
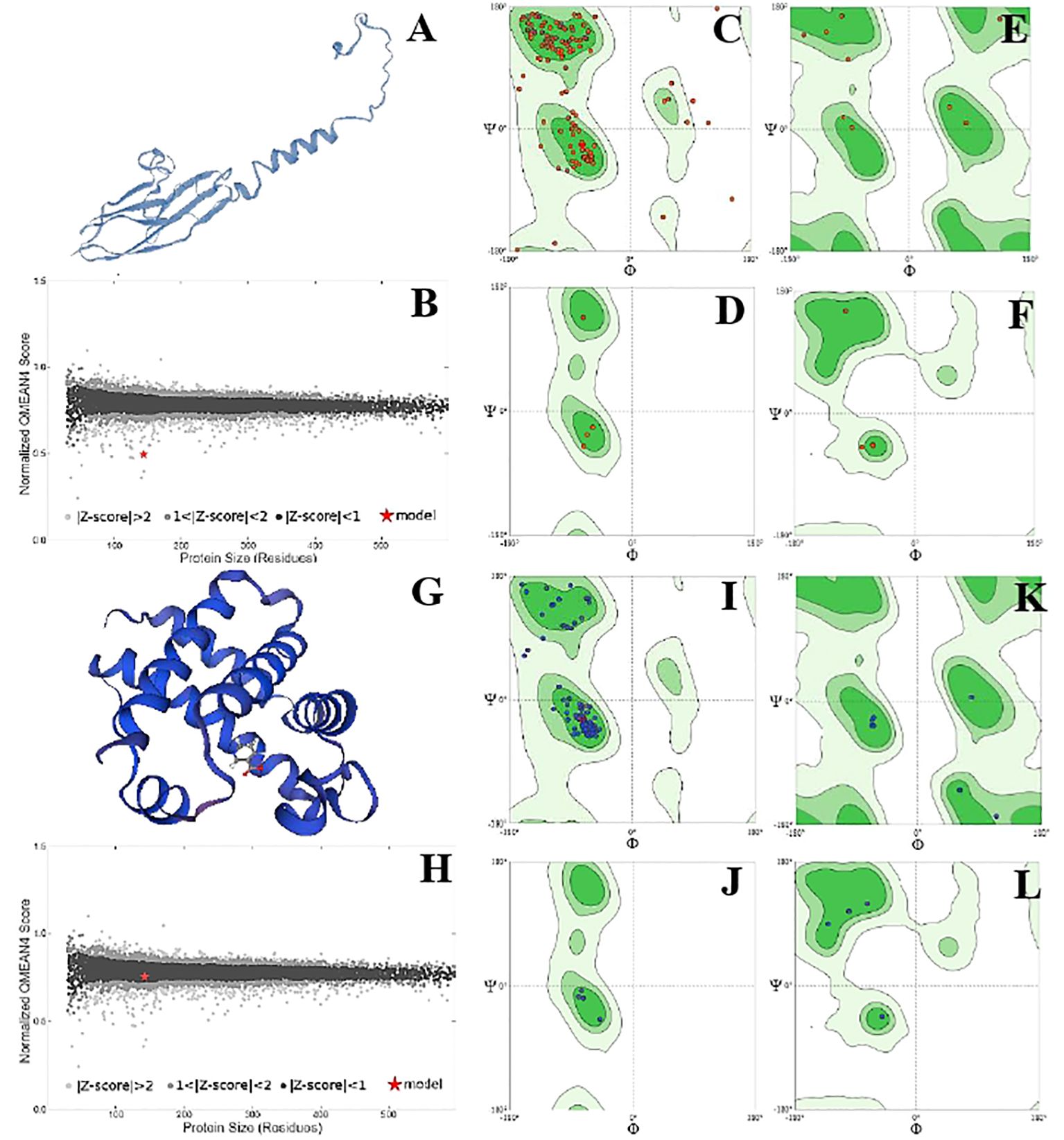
Figure 7. Structural analysis of protein Q9FIE3. Structural illustration of protein in ribbon model (A), energy map (B), the position of phi (φ)and psi (ψ) angles in the 3D structure (C), The position of preproline (D), the position of glycine (E), and the position of proline (F). Structural analysis of protein 004939. Structural illustration of protein in ribbon model (G), energy map (H), the position of phi (φ)and psi (ψ) angles in the 3D structure (I), The position of preproline (J), the position of glycine (K), and the position of proline (L).
4 Discussion
In various abiotic conditions, lipid peroxidation, H2O2 accumulation, and electrolyte leakage serve as biochemical markers indicative of damage caused by free radicals (Verma and Dubey, 2003). Additionally, heightened production of reactive oxygen species (ROS) is a common characteristic of these metabolic processes (Foyer and Noctor, 2003; Gill and Tuteja, 2010). Our findings support this notion, showing an increase in lipid peroxidation, H2O2 accumulation, and electrolyte leakage due to heat and drought stress (Figure 3). Consequently, oxidative stress is believed to underlie the damage incurred by plants due to heat and drought. According to earlier research, oxidative bursts and the production of ROS are brought on by heat and drought stress. Overproduction of ROS during abiotic stress conditions upsets the balance between ROS generation and removal (del Río et al., 2006; Navrot et al., 2007). ROS in plant tissues are regulated by osmoprotectants like proline, non-enzymatic low molecular weight antioxidants like glutathione, carotenoids, and tocopherols, and antioxidant enzymes like superoxide dismutase, catalase, peroxidase, and glutathione reductase (Schützendübel and Polle, 2002; Szabados and Savouré, 2010). Despite both Se and ROS serving as important signaling molecules in plants, their effects on plant damage, growth, and stress resistance differ significantly (Apel and Hirt, 2004). Conferring to our current research, applying Se and EBL to plants boosted proline accumulation (Figure 4D) and antioxidant enzyme activity (Figure 4), regardless of whether heat and drought stress were present. Additionally, plants under stress from heat and drought displayed the highest values; these plants were then given a combination of EBL and Se (Figure 4).
To combat the excessive oxidative stress induced by ROS, plants have evolved a number of complicated cellular and systemic homeostatic systems. These processes include both non-enzymatic defense like free amino acids, particularly proline, and enzymatic defenses such as SOD, CAT, and POX. These levels were even higher after Se and EBL treatment. Plants treated with EBL and Se under drought stress conditions showed the highest activity of CAT, POX, and SOD (Figure 4). Furthermore, a great deal of research has indicated that selenium (Se) might be a quasi-essential element that plants need to provide defense against a range of abiotic stressors, including salt (Hawrylak-Nowak, 2009) and heavy metals (Mroczek-Zdyrska and Wójcik, 2012). The enhanced expression of the det2 gene is responsible for the EBL-induced boost of antioxidant enzymes. This gene improves the antioxidant system, hence increasing Arabidopsis tolerance to oxidative stress (Cao et al., 2005). Our findings support previous research that found that exogenously administered BRs altered antioxidant enzyme activity in response to diverse abiotic stressors (Khan et al., 2019). Proline is thought to work as a signaling molecule, regulating mitochondrial processes and triggering particular gene expression, which may be necessary for plants to recover from abiotic challenges (Szabados and Savouré, 2010). In line with these findings, the current study discovered that extreme heat and drought in the presence of both EBL and Se increased proline accumulation significantly (Figure 4D). Furthermore, proline metabolism influences a variety of developmental and stress responses, and its accumulation contributes in providing tolerance to a wide range of environmental stressors (Szabados and Savouré, 2010). Under stress, BRs can increase proline buildup by expressing genes involved in proline biosynthesis (Ozdemir et al., 2004; Zeng et al., 2010). Additionally, it was demonstrated that applying Se to Brassica napus plants increased both the enzymatic and non-enzymatic antioxidant systems, hence strengthening the plants’ resistance to oxidative damage caused by metals (Hasanuzzaman et al., 2012).
Our current investigation found that growth metrics, such as leaf area, fresh and dry mass of shoot and root, and root and shoot length, were considerably reduced under heat and drought stress conditions (Figures 1A–D). The relationship between heat and drought stress is well-established, since lower transpiration and stomatal conductance during droughts can make heat stress worse by raising leaf temperatures (Lipiec et al., 2013). In the field, rapid declines in plant growth and productivity can result from simultaneous heat waves and droughts, particularly in tropical and subtropical locations (Niinemets, 2016; Zandalinas et al., 2017). Increased temperatures often result in changes to the structure of leaves, which are characterized by increased surface areas and thinner leaves (Luomala et al., 2005; Poorter et al., 2009). Although Wahid et al. (2007) found comparable effects on cell density across high temperature and drought stress, there is still a dearth of knowledge about how high temperature affects leaf architecture (Wahid et al., 2007). In line with earlier research, our findings showed that supplementing wheat with low dosages of Se increased its growth, leaf area, and chlorophyll content (Naz et al., 2015; Yusuf et al., 2016). Comparable results have been noted for ryegrass, lettuce, wheat, and B. juncea, among other plant species (Hartikainen et al., 2000; Ramos et al., 2010; Naz et al., 2015; Boldrin et al., 2016; Yusuf et al., 2016).
Furthermore, as suggested by Xie et al. (2011), the transcriptional impacts of brassinosteroids (BR) and their influence on biomass could prove instrumental in enhancing agricultural yields and resilience. BR signaling governs both cell growth and proliferation, crucial processes for biomass production. By directly modulating the expression of CELLULOSE SYNTHASE (CESA) genes in A. thaliana, BR promotes plant development through cell elongation, thereby augmenting cellulose content and biomass accumulation (Xie et al., 2011). Heat stress impedes cell elongation and induces cell cycle arrest by downregulating genes such as CESA and certain cyclins (Guerriero et al., 2014; Zhao et al., 2014). Together with CESA, BR also increases the production of enzymes that aid in the expansion and loosening of cell walls, such as expansins, xyloglucan endotransglucosylase, and pectin-lyase (Uozu et al., 2000; Guo et al., 2019). Considering that BR can rescue cell growth from heat stress (Hatfield and Prueger, 2015). BR can boost crop biomass and overall production in both favorable and stressful conditions by targeting CESA gene and its homologs. In addition, the fresh and dry weight of wheat plants were raised by the foliar application of EBL (Bajguz and Tretyn, 2003).
The use of EBL in combination with Se considerably improved SPAD chlorophyll levels, photosynthetic machinery, and photosystem II (Fv/Fm) in the current study (Figure 2). In our current investigation, both heat and drought stress exerted a significant adverse impact on photosynthesis-related parameters, leading to a reduction in chlorophyll levels and photosystem II productivity (Figure 2). The onset of senescence and changes in the activity of interconnected enzymes are believed to induce changes in cytoplasmic composition and modulate sink activity, thereby impeding the transport of photosynthates and ultimately decreasing the rate of photosynthesis (Cakmak, 2005). Earlier research has demonstrated that heat, salt, and water stress reduce the effectiveness of transpiration rates and photosynthesis, either separately or in combination (Arbona et al., 2013; Zandalinas et al., 2016). The main cause of this decrease is stress-induced stomatal closure, although non-stomatal restrictions including decreased leaf growth, senescence, and compromised photosynthetic apparatus performance can also have an impact (Saibo et al., 2009; Rahnama et al., 2010). In the latter case, decreased internal CO2 availability combined with suppression of essential photosynthetic enzymes and ATP synthases is frequently implicated. It has been demonstrated that heat and dryness inhibit electron transport, cause protein degradation, and liberate calcium and magnesium ions from protein-binding partners (Wahid et al., 2007; Rexroth et al., 2011; Zlatev and Lidon, 2012; Zandalinas et al., 2016).
As a result, the current investigation revealed that plants treated with Se had dramatically improved PN, and gs, in both stressed and non-stressed environments. The potential rationale for using Se was to enhance photosynthetic efficacy by influencing the activation of defensive mechanisms in plants at various levels. Furthermore, Se treatment has been shown to significantly increase photosynthesis by upregulating chlorophyll synthesis (Zhang et al., 2014; Naz et al., 2015; Yusuf et al., 2016) and photosynthetic characteristics (PN, and gs) (Zhang et al., 2014; Naz et al., 2015; Yusuf et al., 2016). Se treatment enhanced the leaf area, total chlorophyll content, photosynthesis-related characteristics, and photosystem II in B. juncea during stress conditions (Yusuf et al., 2016). Additionally, EBL-treated plants had improved photosynthetic parameters (PN, and gs) to improve CO2 adjustment and increased the efficiency of the light-picking complex by raising chlorophyll content. The present findings align with the research undertaken by other investigators, demonstrating that the exogenous application of BRs enhanced photosynthesis and associated characteristics, as well as the quantum yield of PSII under stressful conditions (Khan et al., 2015; Yusuf et al., 2017).
Several research explained the severe effects of environmental stressors on agricultural crops (Khan et al., 2019; Shah et al., 2020; Ahmad et al., 2021; Tariq et al., 2021). Besides considering the deleterious roles of drought and heat, it is very important to study the physiological mechanisms of the stressors. It is important to study the proteins behind the plant stressor impacts (Khan et al., 2018; Shah et al., 2020). Therefore, the current study is a precious advancement because it provides a detailed view of the proteins involved in heat and drought stress. Furthermore, the study also gives a comprehensive view of the stress alleviators. The proteins involved in stress alleviation can be used to eliminate the stress effects from the plants (Shah et al., 2020; Tariq et al., 2021). For that purpose, their expression can be enhanced in the plants. Drought and heat are the two trenchant factors severely affecting our crops and their production (Ahmad et al., 2021). There are multiple reports of complete crop failure due to severe drought hits or abrupt heat shocks. It is very important to study the molecular mechanisms of these environmental factors to cope with the changing climate. Further studies can help to develop resistant cultivars against drought and heat. Proteins are the functional unit of the cellular processes (Bashir et al., 2016). Previously several proteins have been reported to deal with external stimuli (Shafique and Ahmad, 2013; Ahmad et al., 2014; Akram et al., 2014; Ahmad et al., 2019). Similarly, a single protein may be involved in dealing the multiple stimuli. The current study first time reports two stress-related proteins and two stress alleviation-related proteins. Furthermore, the study provides a full report on their structural and functional stability, allowing researchers to pick stable proteins for stress-related research. The proteins can then be introduced into new cultivars to help them adapt to changing climate conditions. To do this, genetic engineering and other molecular approaches can add protein-encoding genes into new cultivars or plants, increasing their resilience to drought and heat stress.
5 Conclusion
The combined application of Se and BRs demonstrated encouraging alleviative effects in wheat plants subjected to concurrent heat and drought stress, evidenced by the upregulation of specific proteins (Q9FIE3 and O04939) and enhanced synthesis of primary antioxidant enzymes (catalase, peroxidase, and superoxide dismutase) to counteract ROS. These responses were effectively reflected in the growth performance and photosynthetic efficiency of wheat plants under the combined stress of heat and drought. This study revealed new and important insights into the potentialities of Se and BRs mediated amelioration of combined stress of heat and drought through modulating protein expression, and enhanced production of antioxidants, and osmolytes along with improved photosynthetic performance. Moreover, for the first time in this study, two stress-related proteins and two stress-relieving proteins are reported in response to EBL, Se, heat, and drought stress. Furthermore, the study provides a full report on their structure and functional stability, enabling researchers to choose stable proteins for stress-related studies. The gene responsible for the expression of these proteins could be incorporated into the new cultivars to cope with changing climate. For that purpose, genetic engineering and other molecular techniques can introduce protein-encoding genes into new cultivars or plants to enhance their resistance to drought and heat stress.
Data availability statement
The raw data supporting the conclusions of this article will be made available by the authors, without undue reservation.
Author contributions
TK: Conceptualization, Data curation, Methodology, Validation, Visualization, Writing – original draft. AAh: Investigation, Software, Writing – review & editing. TS: Data curation, Methodology, Validation, Writing – original draft. MY: Investigation, Supervision, Writing – review & editing. MF: Conceptualization, Data curation, Investigation, Writing – review & editing. AAl: Conceptualization, Formal analysis, Validation, Writing – review & editing.
Funding
The author(s) declare financial support was received for the research, authorship, and/or publication of this article from the research office, United Arab Emirates University, UAE. The funders had no role in study design, data collection and analysis, decision to publish, or preparation of the manuscript.
Conflict of interest
The authors declare that the research was conducted in the absence of any commercial or financial relationships that could be construed as a potential conflict of interest.
The author(s) declared that they were an editorial board member of Frontiers, at the time of submission. This had no impact on the peer review process and the final decision.
Publisher’s note
All claims expressed in this article are solely those of the authors and do not necessarily represent those of their affiliated organizations, or those of the publisher, the editors and the reviewers. Any product that may be evaluated in this article, or claim that may be made by its manufacturer, is not guaranteed or endorsed by the publisher.
References
Ahmad, A., Akram, W., Shahzadi, I., Wang, R., Hu, D., Bashir, Z., et al. (2019). Benzenedicarboxylic acid upregulates O48814 and Q9FJQ8 for improved nutritional contents of tomato and low risk of fungal attack. J. Sci. Food Agric. 99, 6139–6154. doi: 10.1002/jsfa.9836
Ahmad, A., Shafique, S., Shafique, S., Akram, W. (2014). Penicillium oxalicum directed systemic resistance in tomato against alternaria alternata. Acta Physiologiae Plantarum 36, 1231–1240. doi: 10.1007/s11738-014-1500-5
Ahmad, A., Shahzadi, I., Mubeen, S., Yasin, N. A., Akram, W., Khan, W. U., et al. (2021). Karrikinolide alleviates BDE-28, heat and cd stressors in brassica alboglabra by correlating and modulating biochemical attributes, antioxidative machinery and osmoregulators. Ecotoxicol. Environ. Saf. 213, 112047. doi: 10.1016/j.ecoenv.2021.112047
Akram, W., Anjum, T., Ahmad, A. (2014). Basal Susceptibility of Tomato Varieties against Different Isolates of F. Oxysporum f. Sp. Lysopersici. Int. J. Agric. Biol. 16, 171–176.
Ali, B., Hayat, S., Ahmad, A. (2007). 28-homobrassinolide ameliorates the saline stress in chickpea (Cicer arietinum L.). Environ. Exp. Bot. 59, 217–223. doi: 10.1016/j.envexpbot.2005.12.002
Apel, K., Hirt, H. (2004). REACTIVE OXYGEN SPECIES: metabolism, oxidative stress, and signal transduction. Annu. Rev. Plant Biol. 55, 373–399. doi: 10.1146/annurev.arplant.55.031903.141701
Arbona, V., Manzi, M., de Ollas, C., Gómez-Cadenas, A. (2013). Metabolomics as a tool to investigate abiotic stress tolerance in plants. Int. J. Mol. Sci. 14, 4885–4911. doi: 10.3390/ijms14034885
Arnon, D. I. (1949). Copper enzymes in isolated chloroplasts. Polyphenoloxidase in beta vulgaris. Plant Physiol. 24, 1–15. doi: 10.1104/pp.24.1.1
Bajguz, A., Tretyn, A. (2003). The chemical characteristic and distribution of brassinosteroids in plants. Phytochemistry 62, 1027–1046. doi: 10.1016/s0031-9422(02)00656-8
Bashir, Z., Shafique, S., Ahmad, A., Shafique, S., Yasin, N. A., Ashraf, Y., et al. (2016). Tomato plant proteins actively responding to fungal applications and their role in cell physiology. Front. Physiol. 7.
Boldrin, P. F., de Figueiredo, M. A., Yang, Y., Luo, H., Giri, S., Hart, J. J., et al. (2016). Selenium promotes sulfur accumulation and plant growth in wheat (Triticum aestivum). Physiol. Plant 158, 80–91. doi: 10.1111/ppl.12465
Cakmak, I. (2005). The role of potassium in alleviating detrimental effects of abiotic stresses in plants. J. Plant Nutr. Soil Sci. 168, 521–530. doi: 10.1002/jpln.200420485
Cao, S., Xu, Q., Cao, Y., Qian, K., An, K., Zhu, Y., et al. (2005). Loss-of-function mutations in DET2 gene lead to an enhanced resistance to oxidative stress in arabidopsis. Physiologia Plantarum 123, 57–66. doi: 10.1111/j.1399-3054.2004.00432.x
Cittrarasu, V., Kaliannan, D., Dharman, K., Maluventhen, V., Easwaran, M., Liu, W. C., et al. (2021). Green synthesis of selenium nanoparticles mediated from ceropegia bulbosa roxb extract and its cytotoxicity, antimicrobial, mosquitocidal and photocatalytic activities. Sci. Rep. 11, 1032. doi: 10.1038/s41598-020-80327-9
del Río, L. A., Sandalio, L. M., Corpas, F. J., Palma, J. M., Barroso, J. B. (2006). Reactive oxygen species and reactive nitrogen species in peroxisomes. Production, scavenging, and role in cell signaling. Plant Physiol. 141, 330–335. doi: 10.1104/pp.106.078204
Diao, M., Ma, L., Wang, J., Cui, J., Fu, A., Liu, H. (2014). Selenium promotes the growth and photosynthesis of tomato seedlings under salt stress by enhancing chloroplast antioxidant defense system. J. Plant Growth Regul. 33, 671–682. doi: 10.1007/s00344-014-9416-2
Dong, Y. J., Wang, W. W., Hu, G. Q., Chen, W. F., Zhuge, Y. P., Wang, Z. L., et al. (2017). Role of exogenous 24-epibrassinolide in enhancing the salt tolerance of wheat seedlings. J. Soil Sci. Plant Nutr. 17, 554–569. doi: 10.4067/S0718-95162017000300001
Ekanayake, L. J., Thavarajah, D., Vial, E., Schatz, B., McGee, R., Thavarajah, P. (2015). Selenium fertilization on lentil (Lens culinaris medikus) grain yield, seed selenium concentration, and antioxidant activity. Field Crops Res. 177, 9–14. doi: 10.1016/j.fcr.2015.03.002
Fariduddin, Q., Khan, T. A., Yusuf, M. (2014). Hydrogen peroxide mediated tolerance to copper stress in the presence of 28-homobrassinolide in vigna radiata. Acta Physiologiae Plantarum 36, 2767–2778. doi: 10.1007/s11738-014-1647-0
Fariduddin, Q., Yusuf, M., Chalkoo, S., Hayat, S., Ahmad, A. (2011). 28-homobrassinolide improves growth and photosynthesis in cucumis sativus L. through an enhanced antioxidant system in the presence of chilling stress. Photosynthetica 49, 55–64. doi: 10.1007/s11099-011-0022-2
Farooq, M., Aziz, T., Wahid, A., Lee, D.-J., Siddique, K. H. M. (2009). Chilling tolerance in maize: agronomic and physiological approaches. Crop Pasture Sci. 60, 501. doi: 10.1071/cp08427
Feng, R. W., Wei, C. Y. (2012). Antioxidative mechanisms on selenium accumulation in pteris vittata L., a potential selenium phytoremediation plant. Plant Soil Environ. 58, 105–110.
Fio, J. L., Fujii, R., Deverel, S. J. (1991). Selenium mobility and distribution in irrigated and nonirrigated alluvial soils. Soil Sci. Soc. America J. 55, 1313–1320. doi: 10.2136/sssaj1991.03615995005500050020x
Foyer, C. H., Noctor, G. (2003). Redox sensing and signalling associated with reactive oxygen in chloroplasts, peroxisomes and mitochondria. Physiologia Plantarum 119, 355–364. doi: 10.1034/j.1399-3054.2003.00223.x
Germ, M., Kreft, I., Osvald, J. (2005). Influence of UV-B exclusion and selenium treatment on photochemical efficiency of photosystem II, yield and respiratory potential in pumpkins (Cucurbita pepo L.). Plant Physiol. Biochem. 43, 445–448. doi: 10.1016/j.plaphy.2005.03.004
Gill, S. S., Tuteja, N. (2010). Reactive oxygen species and antioxidant machinery in abiotic stress tolerance in crop plants. Plant Physiol. Biochem. 48, 909–930. doi: 10.1016/j.plaphy.2010.08.016
Guerriero, G., Legay, S., Hausman, J.-F. (2014). Alfalfa cellulose synthase gene expression under abiotic stress: A hitchhiker’s guide to RT-qPCR normalization. PloS One 9, e103808. doi: 10.1371/journal.pone.0103808
Guo, Y.F., Shan, W., Liang, S.M., Wu, C.J., Wei, W., Chen, J., et al. (2019). MaBZR1/2 act as transcriptional repressors of ethylene biosynthetic genes in banana fruit. Physiologia plantarum 165, 555–568. doi: 10.1111/PPL.12750
Gupta, M., Gupta, S. (2017). An overview of selenium uptake, metabolism, and toxicity in plants. Front. Plant Sci. 7.
Hartikainen, H., Xue, T., Piironen, V. (2000). Selenium as an anti-oxidant and pro-oxidant in ryegrass. Plant Soil 225, 193–200. doi: 10.1023/A:1026512921026
Hasanuzzaman, M., Hossain, M. A., da Silva, J. A. T., Fujita, M. (2012). “Plant Response and Tolerance to Abiotic Oxidative Stress: Antioxidant Defense Is a Key Factor,” in Crop Stress and its Management: Perspectives and Strategies. Eds. Venkateswarlu, B., Shanker, A. K., Shanker, C., Maheswari, M. (Springer Netherlands: Dordrecht), 261–315, ISBNISBN 978-94-007-2220-0.
Hatfield, J. L., Prueger, J. H. (2015). Temperature extremes: effect on plant growth and development. Weather Climate Extremes 10, 4–10. doi: 10.1016/j.wace.2015.08.001
Hawrylak-Nowak, B. (2009). Beneficial effects of exogenous selenium in cucumber seedlings subjected to salt stress. Biol. Trace Elem. Res. 132, 259–269. doi: 10.1007/s12011-009-8402-1
Hussain, M., Khan, T. A., Yusuf, M., Fariduddin, Q. (2019). Silicon-mediated role of 24-epibrassinolide in wheat under high-temperature stress. Environ. Sci. pollut. Res. 26, 17163–17172. doi: 10.1007/s11356-019-04938-0
Ikram, M., Javed, B., Raja, N. I., Mashwani, Z.-R. (2021). Biomedical potential of plant-based selenium nanoparticles: A comprehensive review on therapeutic and mechanistic aspects. Int. J. Nanomed. 16, 249–268. doi: 10.2147/IJN.S295053
Khan, T. A., Fariduddin, Q., Yusuf, M. (2015). Lycopersicon Esculentum under Low Temperature Stress: An Approach toward Enhanced Antioxidants and Yield. Environ. Sci. pollut. Res. 22, 14178–14188. doi: 10.1007/s11356-015-4658-5
Khan, T. A., Fariduddin, Q., Yusuf, M. (2017). Low-temperature stress: is phytohormones application a remedy? Environ. Sci. pollut. Res. 24, 21574–21590. doi: 10.1007/s11356-017-9948-7
Khan, W. U., Yasin, N. A., Ahmad, S. R., Ali, A., Ahmad, A., Akram, W., et al. (2018). Role of burkholderia cepacia CS8 in cd-stress alleviation and phytoremediation by catharanthus roseus. Int. J. Phytoremediation 20, 581–592. doi: 10.1080/15226514.2017.1405378
Khan, T. A., Yusuf, M., Ahmad, A., Bashir, Z., Saeed, T., Fariduddin, Q., et al. (2019). Proteomic and physiological assessment of stress sensitive and tolerant variety of tomato treated with brassinosteroids and hydrogen peroxide under low-temperature stress. Food Chem. 289, 500–511. doi: 10.1016/j.foodchem.2019.03.029
Kim, T.-W., Wang, Z.-Y. (2010). Brassinosteroid signal transduction from receptor kinases to transcription factors. Annu. Rev. Plant Biol. 61, 681–704. doi: 10.1146/annurev.arplant.043008.092057
Lipiec, J., Doussan, C., Nosalewicz, A., Kondracka, K. (2013). Effect of drought and heat stresses on plant growth and yield: A review. Int. Agrophysics 27, 463–477.
Luomala, E.-M., Laitinen, K., Sutinen, S., Kellomäki, S., Vapaavuori, E. (2005). Stomatal density, anatomy and nutrient concentrations of scots pine needles are affected by elevated CO2 and temperature. Plant Cell Environ. 28, 733–749. doi: 10.1111/j.1365-3040.2005.01319.x
Lyons, G. H., Genc, Y., Soole, K., Stangoulis, J. C. R., Liu, F., Graham, R. D. (2009). Selenium increases seed production in brassica. Plant Soil 318, 73–80. doi: 10.1007/s11104-008-9818-7
Mir, B., Khan, T., Fariduddin, Q. (2015). 24-epibrassinolide and spermidine modulate photosynthesis and antioxidant systems in vigna radiata under salt and zinc stress. Int. J. Advanced Res. 3, 592–608.
Mroczek-Zdyrska, M., Wójcik, M. (2012). The influence of selenium on root growth and oxidative stress induced by lead in vicia faba L. Minor plants. Biol. Trace element Res. 147, 320–328. doi: 10.1007/s12011-011-9292-6
Muhammad, M., Waheed, A., Wahab, A., Majeed, M., Nazim, M., Liu, Y. H., et al. (2024). Soil salinity and drought tolerance: an evaluation of plant growth, productivity, microbial diversity, and amelioration strategies. Plant Stress 11, 100319.
Navrot, N., Rouhier, N., Gelhaye, E., Jacquot, J.-P. (2007). Reactive oxygen species generation and antioxidant systems in plant mitochondria. Physiologia Plantarum 129, 185–195. doi: 10.1111/j.1399-3054.2006.00777.x
Naz, F. S., Yusuf, M., Khan, T. A., Fariduddin, Q., Ahmad, A. (2015). Low level of selenium increases the efficacy of 24-epibrassinolide through altered physiological and biochemical traits of brassica juncea plants. Food Chem. 185, 441–448. doi: 10.1016/j.foodchem.2015.04.016
Niinemets, Ü. (2016). Uncovering the hidden facets of drought stress: secondary metabolites make the difference. Tree Physiol. 36, 129–132. doi: 10.1093/treephys/tpv128
Ozdemir, F., Bor, M., Demiral, T., Turkan, I. (2004). Effects of 24-epibrassinolide on seed germination, seedling growth, lipid peroxidation, proline content and antioxidative system of rice (Oryza sativa L.) under salinity stress. Plant Growth Regul. 42, 203–211. doi: 10.1023/B:GROW.0000026509.25995.13
Poorter, H., Niinemets, Ü., Poorter, L., Wright, I. J., Villar, R. (2009). Causes and consequences of variation in leaf mass per area (LMA): A meta-analysis. New Phytol. 182, 565–588. doi: 10.1111/j.1469-8137.2009.02830.x
Prasad, P. V. V., Pisipati, S. R., Momcilovic, I., Ristic, Z. (2011). Independent and combined effects of high temperature and drought stress during grain filling on plant yield and chloroplast protein synthesis elongation factor (EF-Tu) expression in spring wheat. J. Agron. Crop Sci. 197, 430–441.
Rahnama, A., Poustini, K., Tavakkol Afshari, R., Tavakoli, A. (2010). Growth and stomatal responses of bread wheat genotypes in tolerance to salt stress. Int. J. Biol. Life Sci. 6, 216–221.
Ramos, S. J., Faquin, V., Guilherme, L. R. G., Castro, E. M., Ávila, F. W., Carvalho, G. S., et al. (2010). Selenium biofortification and antioxidant activity in lettuce plants fed with selenate and selenite. Plant Soil Environ. 56, 584–588. doi: 10.17221/113/2010-PSE
Rexroth, S., Mullineaux, C. W., Ellinger, D., Sendtko, E., Rögner, M., Koenig, F. (2011). The plasma membrane of the cyanobacterium gloeobacter violaceus contains segregated bioenergetic domains. Plant Cell 23, 2379–2390. doi: 10.1105/tpc.111.085779
Saibo, N. J. M., Lourenço, T., Oliveira, M. M. (2009). Transcription factors and regulation of photosynthetic and related metabolism under environmental stresses. Ann. Bot. 103, 609–623. doi: 10.1093/aob/mcn227
Schiavon, M., Lima, L. W., Jiang, Y., Hawkesford, M. J. (2017). Effects of Selenium on Plant Metabolism and Implications for Crops and Consumers Vol. 11. Eds. Pilon-Smits, E. A. H., Winkel, L. H. E., Lin, Z.-Q. (Springer, Cham: Springer International Publishing AG), 256–275, ISBNISBN 978-3-319-56249-0.
Schützendübel, A., Polle, A. (2002). Plant responses to abiotic stresses: heavy metal-induced oxidative stress and protection by mycorrhization. J. Exp. Bot. 53, 1351–1365.
Shafique, S., Ahmad, A. (2013). Ecofriendly response of citrus peels to alternaria leaf spots of tomato: exclusive role of peel phenolics. Int. J. Agric. Biol. 15, 1236–1242.
Shah, A. A., Khan, W. U., Yasin, N. A., Akram, W., Ahmad, A., Abbas, M., et al. (2020). Butanolide alleviated cadmium stress by improving plant growth, photosynthetic parameters and antioxidant defense system of brassica oleracea. Chemosphere 261, 127728. doi: 10.1016/j.chemosphere.2020.127728
Szabados, L., Savouré, A. (2010). Proline: A multifunctional amino acid. Trends Plant Sci. 15, 89–97. doi: 10.1016/j.tplants.2009.11.009
Tariq, M., Shah, A. A., Yasin, N. A., Ahmad, A., Rizwan, M. (2021). Enhanced performance of bacillus megaterium OSR-3 in combination with putrescine ammeliorated hydrocarbon stress in nicotiana tabacum. Int. J. Phytoremediation 23, 119–129. doi: 10.1080/15226514.2020.1801572
Turakainen, M., Hartikainen, H., Seppänen, M. M. (2004). Effects of selenium treatments on potato (Solanum tuberosum L.) growth and concentrations of soluble sugars and starch. J. Agric. Food Chem. 52, 5378–5382. doi: 10.1021/jf040077x
Uozu, S., Tanaka-Ueguchi, M., Kitano, H., Hattori, K., Matsuoka, M. (2000). Characterization of XET-related genes of rice. Plant Physiol. 122, 853. doi: 10.1104/pp.122.3.853
Verma, S., Dubey, R. S. (2003). Lead toxicity induces lipid peroxidation and alters the activities of antioxidant enzymes in growing rice plants. Plant Sci. 164, 645–655. doi: 10.1016/S0168-9452(03)00022-0
Vidya Vardhini, B. (2017). Modifications of morphological and anatomical characteristics of plants by application of brassinosteroids under various abiotic stress conditions - A review. Plant Gene 11, 70–89. doi: 10.1016/j.plgene.2017.06.005
Wahid, A., Gelani, S., Ashraf, M., Foolad, M. (2007). Heat tolerance in plants: an overview. Environ. Exp. Bot. 61, 199–223. doi: 10.1016/j.envexpbot.2007.05.011
Xie, L., Yang, C., Wang, X. (2011). Brassinosteroids can regulate cellulose biosynthesis by controlling the expression of CESA genes in arabidopsis. J. Exp. Bot. 62, 4495–4506. doi: 10.1093/jxb/err164
Xue, T., Hartikainen, H., Piironen, V. (2001). Antioxidative and growth-promoting effect of selenium on senescing lettuce. Plant Soil 237, 55–61. doi: 10.1023/A:1013369804867
Yusuf, M., Almehrzi, A. S. S., Alnajjar, A. J. N., Alam, P., Elsayed, N., Khalil, R., et al. (2021). Glucose modulates copper induced changes in photosynthesis, ion uptake, antioxidants and proline in cucumis sativus plants. Carbohydr. Res. 501, 108271.
Yusuf, M., Fariduddin, Q., Khan, T. A., Hayat, S. (2017). Epibrassinolide reverses the stress generated by combination of excess aluminum and salt in two wheat cultivars through altered proline metabolism and antioxidants. South Afr. J. Bot. 112, 391–398. doi: 10.1016/j.sajb.2017.06.034
Yusuf, M., Khan, T. A., Fariduddin, Q. (2016). Interaction of epibrassinolide and selenium ameliorates the excess copper in brassica juncea through altered proline metabolism and antioxidants. Ecotoxicol. Environ. Saf. 129, 25–34. doi: 10.1016/j.ecoenv.2016.03.001
Yusuf, M., Saeed, T., Almenhali, H. A., Azzam, F., Hamzah, A. I. A. H.., Khan, T. A. (2024). Melatonin improved efficiency of 24-epibrassinolide to counter the collective stress of drought and salt through osmoprotectant and antioxidant system in pea plants. Sci. Horti. 323, 112453.
Zandalinas, S. I., Rivero, R. M., Martínez, V., Gómez-Cadenas, A., Arbona, V. (2016). Tolerance of citrus plants to the combination of high temperatures and drought is associated to the increase in transpiration modulated by a reduction in abscisic acid levels. BMC Plant Biol. 16, 105. doi: 10.1186/s12870-016-0791-7
Zandalinas, S. I., Sales, C., Beltrán, J., Gómez-Cadenas, A., Arbona, V. (2017). Activation of secondary metabolism in citrus plants is associated to sensitivity to combined drought and high temperatures. Front. Plant Sci. 7.
Zeng, H., Tang, Q., Hua, X. (2010). Arabidopsis brassinosteroid mutants det2-1 and bin2-1 display altered salt tolerance. J. Plant Growth Regul. 29, 44–52. doi: 10.1007/S00344-009-9111-X
Zhang, H., Sonnewald, U. (2017). Differences and commonalities of plant responses to single and combined stresses. Plant J. 90, 839–855.
Zhang, M., Tang, S., Huang, X., Zhang, F., Pang, Y., Huang, Q., et al. (2014). Selenium uptake, dynamic changes in selenium content and its influence on photosynthesis and chlorophyll fluorescence in rice (Oryza sativa L.). Environ. Exp. Bot. 107, 39–45. doi: 10.1016/j.envexpbot.2014.05.005
Zhao, L., Wang, P., Hou, H., Zhang, H., Wang, Y., Yan, S., et al. (2014). Transcriptional regulation of cell cycle genes in response to abiotic stresses correlates with dynamic changes in histone modifications in maize. PloS One 9, e106070. doi: 10.1371/journal.pone.0106070
Keywords: abiotic stress, steroidal hormone, photosynthesis, osmoprotectant, wheat
Citation: Khan TA, Ahmad A, Saeed T, Yusuf M, Faisal M and Alatar AA (2024) Investigating the influence of selenium and epibrassinolide on antioxidant activity, proline accumulation, and protein expression profiles in wheat plants experiencing heat and drought stress. Front. Plant Sci. 15:1441483. doi: 10.3389/fpls.2024.1441483
Received: 31 May 2024; Accepted: 28 August 2024;
Published: 22 October 2024.
Edited by:
Anket Sharma, Texas Tech University, United StatesReviewed by:
Krishna Mohan Dr. Pathi, Julius Kühn-Institut, GermanyReena Dubey, Ghent University, Belgium
Copyright © 2024 Khan, Ahmad, Saeed, Yusuf, Faisal and Alatar. This is an open-access article distributed under the terms of the Creative Commons Attribution License (CC BY). The use, distribution or reproduction in other forums is permitted, provided the original author(s) and the copyright owner(s) are credited and that the original publication in this journal is cited, in accordance with accepted academic practice. No use, distribution or reproduction is permitted which does not comply with these terms.
*Correspondence: Mohammad Yusuf, bXl1c3VmLmFsaWdAdWFldS5hYy5hZQ==
†These authors have contributed equally to this work
‡ORCID: Mohammad Yusuf, orcid.org/0000-0002-2716-4916