- College of Animal Science and Technology, Northeast Agricultural University, Harbin, China
Arbuscular mycorrhizal fungi (AMF) are universally distributed in soils, including saline soils, and can form mycorrhizal symbiosis with the vast majority of higher plants. This symbiosis can reduce soil salinity and influence plant growth and development by improving nutrient uptake, increasing plant antioxidant enzyme activity, and regulating hormone levels. In this study, rhizosphere soil from eight plants in the Songnen saline–alkaline grassland was used to isolate, characterize, and screen the indigenous advantageous AMF. The promoting effect of AMF on alfalfa (Medicago sativa L.) under salt treatment was also investigated. The findings showed that 40 species of AMF in six genera were identified by high-throughput sequencing. Glomus mosseae (G.m) and Glomus etunicatum (G.e) are the dominant species in saline ecosystems of northern China. Alfalfa inoculated with Glomus mosseae and Glomus etunicatum under different salt concentrations could be infested and form a symbiotic system. The mycorrhizal colonization rate and mycorrhizal dependence of G.m inoculation were significantly higher than those of G.e inoculation. With increasing salt concentration, inoculation increased alfalfa plant height, fresh weight, chlorophyll content, proline (Pro), soluble sugar (SS), soluble protein (SP), peroxidase (POD), superoxide dismutase (SOD), and catalase (CAT) activity while decreasing the malondialdehyde (MDA) content and superoxide anion production rate. The results highlight that inoculation with G.m and G.e effectively alleviated salinity stress, with G.m inoculation having a significant influence on salt resistance in alfalfa. AMF might play a key role in alfalfa growth and survival under harsh salt conditions.
1 Introduction
Soil salinization, resulting from poor irrigation management and drought, is one of the most destructive environmental stresses causing significant declines in arable land area, crop productivity, and quality globally (Shahbaz and Ashraf, 2013). The Songnen Plain, one of the three main grasslands in northeastern China, has approximately 15.24% of its area covered with saline soil. Factors such as natural changes (climate and soil-forming parent material) and human activities (extensive land clearing and imperfect water conservancy facilities) have contributed to the continuous deterioration of environmental conditions in the Songnen grassland (Wang et al., 2009). Serious soil compaction, destruction of the granular structure, high soil pH, and nutrient depletion (Gong et al., 2021) not only limit plant growth and reduce the yields and quality of most crops but also affect the physicochemical properties of soils and the ecological balance (Shrivastava and Kumar, 2015). Methods such as physical improvement, chemical improvement, and hydraulic improvement have been adopted to address these problems (Zhao et al., 2023). However, these methods are time-consuming and labor-intensive, which, to some extent, hinder the restoration of saline–alkaline soils. Rhizosphere biotrophic bacteria such as plant beneficial bacteria (PBB) and arbuscular mycorrhizal fungi (AMF) can assist plants in surviving and growing under adverse conditions and increase their resistance to salinity, extreme temperature, and heavy metal stress, thereby increasing plant yield (Garg and Chandel, 2011). Among them, AMF have become a research focus in recent years due to their ability to increase plant salinity tolerance and promote plant nutrient uptake.
Alfalfa (Medicago sativa L.) is a high-quality leguminous fodder with excellent palatability and a developed root system (Ferreira et al., 2015). It is widely cultivated worldwide and holds significant economic value and ecological importance (Mei et al., 2022a). The growth of alfalfa is severely restricted in agricultural areas with high salinity, particularly during the early stages of seed germination and growth. High salinity inhibits or delays germination and the elongation of branches and roots due to osmotic stress (Li et al., 2010). Research indicates that elevated soil salinity levels decrease the content of trace elements (Fe2+, Mn2+, Cu2+, and Zn2+) in the roots, stems, and leaves of alfalfa, disrupting ion transport ratios and cation transport selectivity ratios (Bhattarai et al., 2021). Salt stress also hinders alfalfa growth by reducing plant growth rate and water absorption while increasing proline (Pro) content and membrane peroxidation (Choi et al., 2018). Additionally, salt stress indirectly impacts alfalfa growth by influencing plant metabolism, such as the symbiotic nitrogen fixation ability of rhizobia (Farooq et al., 2017). As salt concentration rises, the nitrogenase activity of alfalfa nodules decreases. Combined salt stress can destroy the nitrogenase component structure in root nodules, reducing the nitrogen fixation capability and ultimately decreasing the total nitrogen content.
AMF are endophytic fungi widely distributed in soil, capable of forming mycorrhizal symbiosis with most terrestrial plants (Zhang et al., 2024a). It is recognized as a biotechnological tool to increase plant resistance and restore ecosystems (Cao et al., 2020). Research has shown that AMF symbionts improve plant resistance by strengthening the water absorption capacity, promoting nutrient assimilation, sustaining ion balance, and increasing photosynthesis and the plant hormone levels (Han et al., 2024; Wang et al., 2024b). Under stress conditions, AMF’s extensive mycelial network extends the rhizosphere absorption area, increasing the plant’s absorption capacity (Liu et al., 2016; Kakouridis et al., 2022). AMF application reduced the negative effects of salt stress by increasing the absorption of microelements, regulating the absorption of Na and K in wheat (Huang et al., 2023). Plants inoculated with AMF exhibit higher transpiration rates and leaf water potential under salt conditions, significantly improving relative leaf water content and survival ability (Akhzari et al., 2016). Mycorrhizal seedling leaves display significantly higher activities of superoxide dismutase (SOD), catalase (CAT), and ascorbate peroxidase (APX) compared to non-mycorrhizal seedlings, helping Elaeagnus angustifolia seedlings to cope with salinity (Chang et al., 2018). Furthermore, the levels of proline (Pro), soluble protein (SP), and antioxidant enzymes activity are higher in AMF-inoculated plants under high salt stress, indicating that the beneficial effects of mycorrhizal symbiosis are crucial for managing severe salt toxicity (Zong et al., 2023). Additionally, AMF-inoculated plants show stronger net photosynthetic rates, stomatal conductance, and chlorophyll content under salt stress (Chandrasekaran et al., 2019). AMF inoculation promotes the accumulation of alfalfa biomass under salt stress and increases the stomatal constraints of alfalfa leaves, increasing CO2 fixation capacity (Shi-Chu et al., 2019).
Soil salinization markedly inhibits plant growth and severely limits agricultural productivity. Therefore, the restoration of saline-alkali land has become an urgent problem to solve. The Songnen saline-alkali grassland, characterized by specific habitats and rich microbial resources, primarily comprises the dominant groups Ascomycota, Basidiomycota, and Mortierellomycota (Cui et al., 2023), making it a vital strategic resource for microbial strains. The unique environment of this grassland may lead to regional specificity in AMF. Alfalfa, an important forage, was selected as the experimental material due to its high yield, rich nutritional value, and strong stress resistance. However, its growth and development are severely limited in saline-alkali soils (Guo et al., 2024). AMF have been shown to significantly alleviate plant salinity stress (Evelin et al., 2009; Qin et al., 2021), and the role of AMF in enhancing salt stress tolerance in alfalfa has received increasing attention. Previous studies have outlined various mechanisms and strategies by which AMF alleviate salinity stress in alfalfa (Moradi, 2016; Laouane et al., 2019; Shi-Chu et al., 2019). However, the effects of the native AMF species in Songnen grassland to alfalfa salinity stress are still uncertain. Most of the strains used in prior studies were sourced commercially (Li et al., 2020; Zong et al., 2023; Jia et al., 2024). In our experiment, local strains from the Songnen grassland were cultured and propagated, and alfalfa was inoculated to cope with salt stress, aiming to overcome the poor adaptability of exogenous sourced strains to Northeast China’s conditions. Accordingly, AMF was isolated, screened, and identified from the rhizosphere of eight dominant saline-tolerant plants in the Songnen saline-alkali grassland, and the growth-promoting effects of two native dominant AMF inoculations on alfalfa were evaluated for the first time through a pot experiment. The aims were (1) to identify the diversity characteristics and dominant species of AMF in the rhizosphere of plants of Songnen saline-alkali grassland in salinized areas and (2) to analyze the efficiency of dominant AMF species isolated from the rhizosphere of plants in Songnen saline-alkali grassland to alleviate salt stress in alfalfa.
2 Materials and methods
2.1 Study site
This study site is located in Songnen grassland (Zhaodong City, Heilongjiang Province, China; 139–140 masl; 46°2′45″–46°3′12″ N, 125°53′51″–125°54′1″ E; Supplementary Figure S1). The region experiences hot and rainy summers and cold and dry winters, with a mean annual precipitation of 569.1 mm. The soil pH is 8.2. The predominant plant community consists of Leymus chinensis (Trin.) and Puccinellia tenuiflora (Griseb.), along with other companion species. Species diversity has decreased due to salinity stress and grazing.
2.2 Sample collection and sequencing
All soil samples used in the experiment were collected in September 2019 in Zhaodong, China, from the rhizosphere soil of eight salinity-tolerant plant species (Table 1). In the saline plot, three plants of each species were randomly selected, and impurities such as litter and stones were removed from the soil surface. Soil from the plant rhizosphere at a depth of 5–20 cm was dug up. The roots in the soil were shaken to separate loose soil, which was then mixed into one sample, sealed in a plastic bag, and returned to the laboratory on ice. All the rhizosphere soil samples were divided into two parts. One part was naturally air-dried and sieved (2 mm) for soil analysis, and the rest was stored at -80°C for sequencing.
DNA from rhizosphere soil samples of eight plants was extracted using the Power Soil DNA Isolation Kit (Mo Bio Laboratories, Inc., USA) according to the manufacturer’s instructions. Three repetitions were set, and quality and purity were checked using 1% agarose gel electrophoresis. Two pairs of primers (AML1F-AML2R and AMV4-5NF_AMDGR) were selected to amplify partial 16s rRNA gene fragments of AMF by nested polymerase chain reaction (PCR). Amplicon libraries were prepared and identified using the Illumina MiSeq sequencing method at Meijorbio Go. (Shanghai, China). Clean sequences were clustered into operational taxonomic units (OTUs) based on 97% similarity, and the most abundant sequences were selected as representative sequences. Annotation through the Silva and Unite databases was used to categorize soil microbial species. AMF alpha diversity indices including Chao, Sobs, Shannon, and Simpson index were calculated using QIIME (http://qiime.org/scripts/assign_taxonomy.html). Significant differences in alpha diversity were assessed with a non-parametric test in QIIME.
2.3 Experimental design and treatments
AMF spores were isolated using wet sieving and sucrose centrifugation (McKenney and Lindsey, 1987). The screened substances were stored in a petri dish at 4°C, and each soil sample was processed three times. Individual spores were placed under a light microscope with a pipette to observe their color, shape, and other superficial characteristics. Spore species were determined based on the Arbuscular Mycorrhizal Fungal Resources and Germplasm Resources of China, the International Arbuscular Mycorrhizal Fungi Conservation Center (INVAM), and species descriptions and pictures from published articles. Two dominant AMF species spores, Glomus mosseae and Glomus etunicatum, selected from the Songnen grassland rhizosphere soil were propagated. The propagation method involved sterilizing seedling trays with 75% alcohol, filling them to the top with sterilized quartz sand culture substrate (sterilized for 120 min at 121°C and 103 kPa), and digging a 6- to 7-cm-deep hole with a sterilized glass rod. Under a stereoscopic microscope, fresh, bright, full spores were collected and placed on the roots of Trifolium repens L. seedlings. The seedlings were then immediately transferred into pre-dug holes and compacted with glass rods. The setup was left in the dark for 24 h before being transferred to a greenhouse for 3 months (16-h light/8-h dark photoperiod, 23–26°C). Root colonization was checked after 15–20 days. After 3 months of cultivation, the roots and rhizosphere soil were collected.
Five salt concentration gradients (0, 50, 100, 150, and 200 mmol/L NaCl) were set up (Tani et al., 2018; Shi-Chu et al., 2019), with three replicates for each concentration. Three treatments were applied for each salt concentration: control (non-inoculation), inoculation with Glomus mosseae (G.m), and inoculation with Glomus etunicatum (G.e). The pot experiment was conducted in a greenhouse using the alfalfa variety “Dongnong No. 1” from Northeast Agricultural University. All the seeds were washed three times in distilled water, surface-disinfected with 75% alcohol, and germinated on wet paper in an incubator (16-h light/8-h dark photoperiod, 23–26°C). Every 10 seedlings were transplanted into a pot (12 cm in diameter; 15 cm in depth) and placed in the greenhouse for cultivation under a 16-h light/8-h dark photoperiod at approximately 23–26°C. The potting substrate consisted of sterilized soil (120 min, 121°C, 103 kPa) and vermiculite (3:1, V:V) to ensure drainage. Additionally, each pot was inoculated with 25 g of AMF mycorrhiza. An equal amount of sterilized soil was added to the non-inoculated treatment. Regular watering and seedling management were performed, and after 30 days of cultivation, the plants were watered with 100 mL of the corresponding NaCl concentration every 2 days. At 10 days later, the above-ground parts were sampled.
2.4 Experimental measurement methods
The mycorrhizal colonization rate of alfalfa roots after 40 days of growth was examined using the alkali dissociation-Trypan blue staining method (Jakobsen et al., 1992). Specifically, alfalfa roots were washed with distilled water, cut into segments of approximately 1.0 cm, then treated with 10% KOH, and boiled in a water bath at 90°C. After cooling, the roots were rinsed with distilled water, stained with Trypan blue dye in 90°C water bath, and then decolorized with lactate glycerin solution. The roots were randomly selected and placed on slides, and the mycorrhizal colonization rate was observed under a microscope. Five intact alfalfa plants with good growth were selected as a group to calculate mycorrhizal dependency using the formula (Hu et al., 2020).
The effects of AMF inoculation on alfalfa under salt treatment were determined by measuring various parameters. Fresh alfalfa height was measured from the root neck to the top of the plants, and fresh weight was recorded for the above-ground parts (Hu et al., 2020). Chlorophyll content was determined by acetone extraction, and the optical density (OD) of chlorophyll a (Chla) and chlorophyll b (Chlb) was measured at 663 and 645 nm, respectively. Total chlorophyll, Chla, and Chlb concentrations were calculated using the formula (Helaoui et al., 2020). The concentration of O2·- was measured according to a previously described method (Liu et al., 2020). Malondialdehyde (MDA) content was estimated using the thiobarbituric acid (TBA) reaction (Ortega-Villasante et al., 2005), and pro content was determined using the methodology described by Bates et al. (1973). Physiological traits, including peroxidase (POD), SOD, and CAT activities, as well as soluble sugar (SS) and SP content, were measured using reagent kits (Suzhou Keming, Suzhou, China). Specific test procedures followed the manufacturer’s instructions for the reagent kits. Three technical replicates were performed for all index determinations.
2.5 Statistical analysis
Diversity and taxonomic analyses of microorganisms were performed using the I-Sanger cloud platform of Shanghai Meiji Biomedical Technology Co. Excel 2019 was used to summarize and organize the data, and all data were tested for normality and homogeneity of variance. Two-way ANOVA was used to analyze the interactive effects between G.m, G.e, and salt concentration. One-way ANOVA was performed to analyze the effects of the same salt concentration on indicators in the control group, G.m group, and G.e group, and Duncan multiple comparison (P < 0.05) was used to test their differences. IBM SPSS Statistics 27 was used for statistical analysis, and Origin 2021 was used for graphing.
3 Results
3.1 AMF identification
The effective sequences obtained by high-throughput sequencing were clustered into 156 OTUs. AMF species annotation was carried out by comparison with the MaarjAM database (https://www.maarjam.botany.ut.ee), and 40 species of six genera were identified (34 in Glomus, two in Archaeospora, one in Diversispora, one in Gigaspora, and two in Paraglomus), but no species of Acaulospora was detected (Supplementary Table S1).
At the genus level, Glomus was the dominant genus in the rhizosphere soils of plants, accounting for 89.33%, while unclassified accounted for 10.16% and was the second most abundant genus (Figure 1A). At the species level, Glomus Wirsel OTU6 VTX00202 had the highest relative abundance, accounting for 29.27%, making it the dominant species. This was followed by unclassified_g_Glomus_f_ Glomeraceae, for which no specific bacterial species was detected, and Glomus sp VTX00304 had the least relative abundance, accounting for only 0.05% (Figure 1B).
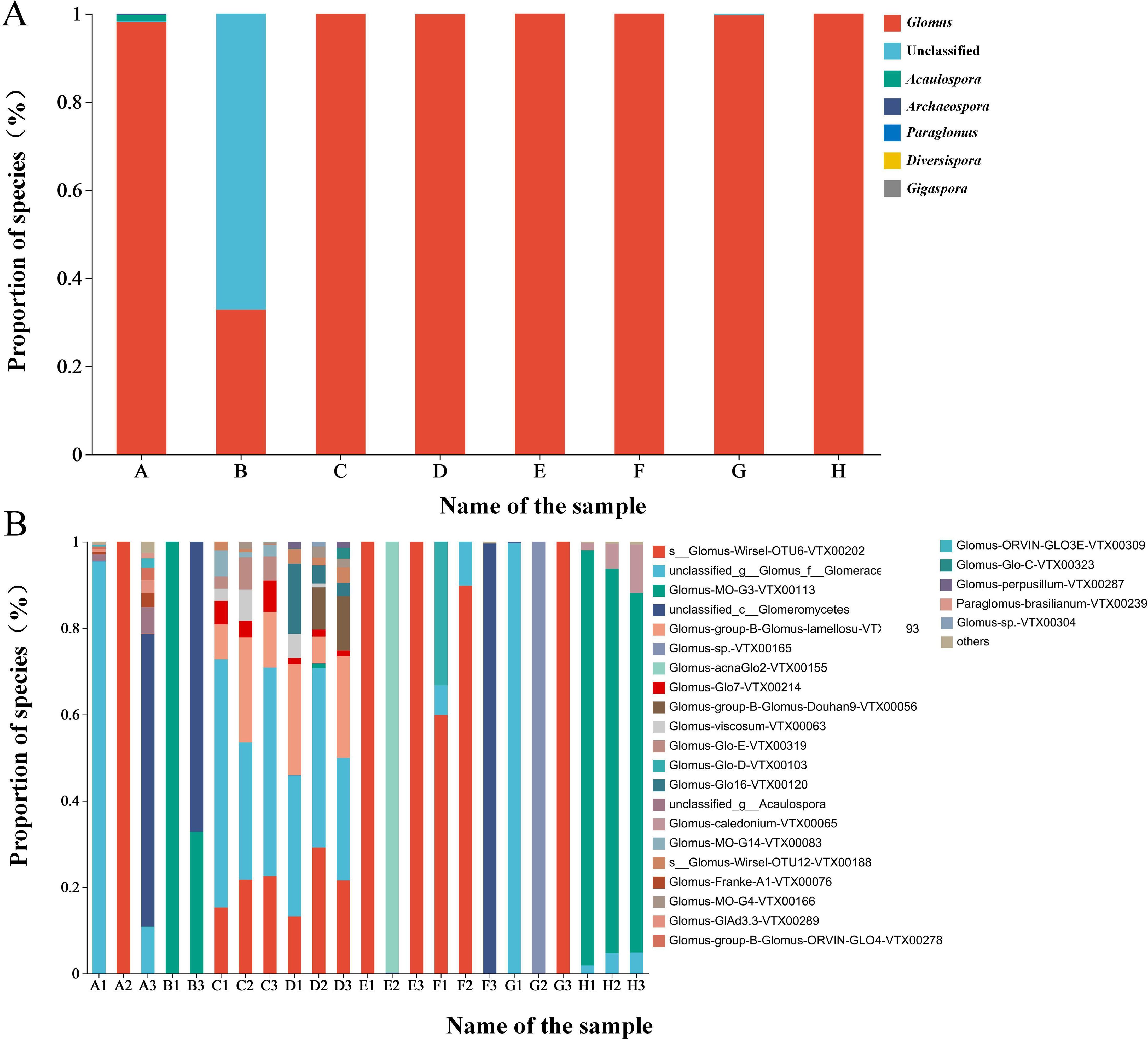
Figure 1. (A) Species composition of rhizosphere AMF of different plants at the genus level (B) Species composition of rhizosphere AMF of different plants at the species level.
3.2 AMF diversity
The distribution of rhizosphere AMF varies among different plants. Among the eight soil samples, the most abundant AMF species were isolated from Leymus chinensis, Tragopogon mongolicum, and Puccinellia tenuiflora, with 12, 13, and 15 AMF species isolated, respectively. At least seven species of AMF were isolated from Clematis hexapetala. Glomus mosseae and Glomus etunicatum were the two most widely distributed AMF, being isolated from all soil samples (Supplementary Table S2).
The AMF community species richness and diversity indices varied significantly in the rhizosphere soils of different plants. The Sobs index indicated that species richness was highest in the Arundinella anomala samples (55.0 ± 22.0a), followed by Taraxacum mongolicum and Puccinellia tenuiflora, and lowest in the Clematis hexapetala samples (3.0 ± 2.0b). The Chao index showed that species richness was highest in the Puccinellia tenuiflora samples (45.33 ± 7.50a), followed by Taraxacum mongolicum and Arundinella anomala, and lowest in Clematis hexapetala (3.0 ± 2.0b). The Shannon and Simpson indices indicated similar results, showing a relatively high diversity in Taraxacum mongolicum and Puccinellia tenuiflora samples, while the lowest diversity was observed in the Artemisia mongolica sample (Table 2).
3.3 Mycorrhizal colonization of alfalfa
The native dominant AMF (G.m and G.e) inoculated with alfalfa under salt stress can infect and form a symbiotic system. Mycorrhizal colonization and dependence were higher in plants inoculated with G.m compared to those inoculated with G.e. Mycorrhizal colonization rates ranged from 33.3% to 76.6% for G.m and from 26.7% to 76.6% for G.e, while non-inoculated plants showed no colonization (Figure 2C). The colonization rate tended to decrease with increasing salt concentration. At 150 and 200 mM, inoculation with G.m resulted in significantly higher colonization rates than inoculation with G.e (p < 0.05, Figure 2A). Mycorrhizal dependence increased and then decreased with rising salt concentration, reaching a maximum at 100 mM (Figure 2B).
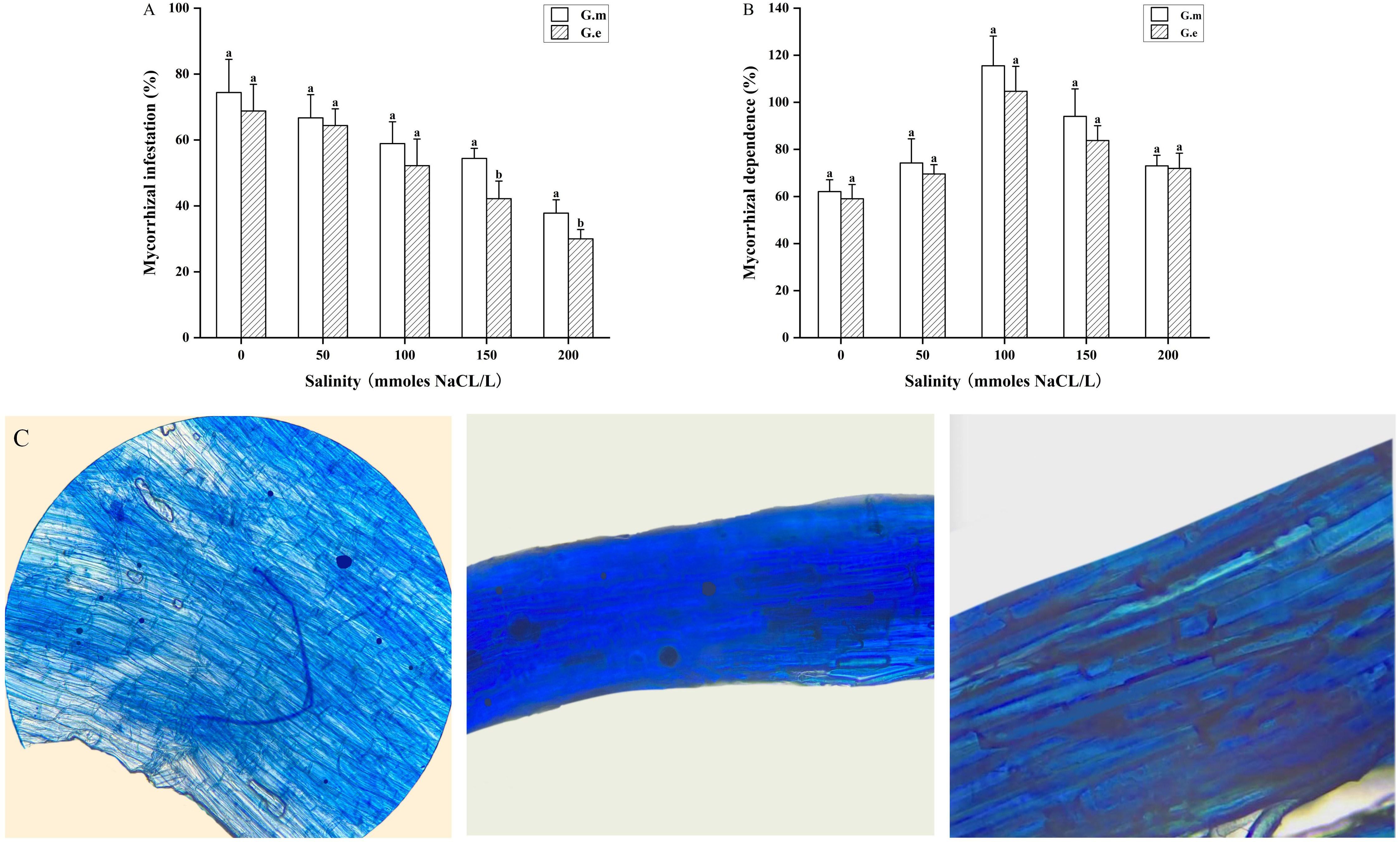
Figure 2. (A) Infestation rate of alfalfa under different treatments. (B) Dependence of alfalfa under different treatments. (C) Microstructure of AMF mycorrhiza of alfalfa. Bar groups with different lowercase letters indicate significant differences (P < 0.05) between treatments under the same salt concentration.
3.4 Interactive effect of AMF and salt concentration on indicators
Salt concentration had a highly significant impact on all indicators (P < 0.01, Table 3). Inoculation with G.m significantly affected all indicators except Chl b (P < 0.01). Inoculation with G.e did not affect Chl b, but it significantly impacted MDA (P < 0.05, Table 3). The interaction between inoculation with G.m, inoculation with G.e, and salt concentration had a highly significant impact on Chl b, Chl, Pro content, and O2·- production rate (P < 0.01, Table 3) but no significant impact on plant height, fresh weight, SS, SP, and POD.
3.5 Effects on alfalfa growth indicators
The plant height and fresh weight of alfalfa decreased with increasing salt concentration. AMF-inoculated plants at corresponding salinity levels were significantly taller and had higher fresh weight than control plants (P < 0.05). The plant height and fresh weight of AMF-inoculated plants were significantly different from control plants at 150 and 200 mM (P < 0.05, Figures 3A, B). Plant height increased by 56.67% and 33.33% and by 86.36% and 63.63%, while fresh weight increased by 14.16% and 9.7% and by 41.61% and 27.95%, respectively, with increasing salt concentration. There was no significant difference between G.m and G.e.
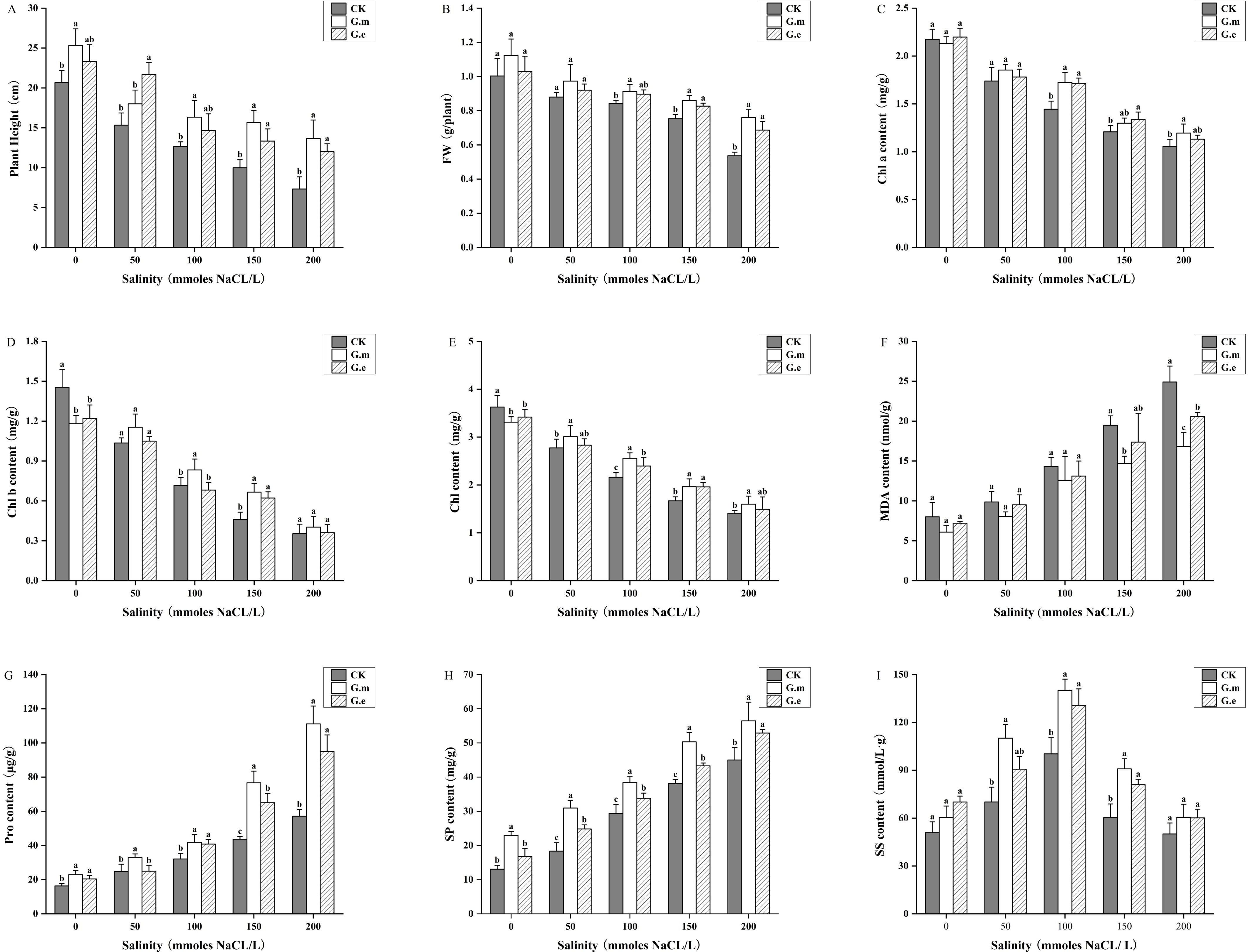
Figure 3. (A) Alfalfa plant height under different treatments. (B) Fresh weight of alfalfa under different treatments. (C) Chl a content of alfalfa under different treatments. (D) Chl b content of alfalfa under different treatments. (E) Chlorophyll content of alfalfa under different treatments. (F) MDA content in alfalfa under different treatments. (G) Pro content of alfalfa under different treatments. (H) SP content of alfalfa under different treatments. (I) SS content of alfalfa under different treatments. Bar groups with different lowercase letters indicate significant differences (P < 0.05) between treatments under the same salt concentration. Data are means ± standard error.
3.6 Effects on physiological indicators of alfalfa
Chl a, Chl b, and total chlorophyll content decreased with increasing salt concentration. Chl a content in alfalfa inoculated with G.m and G.e was significantly higher compared to non-inoculated plants at 100 mM (P < 0.05, Figure 3C). The trends for Chl b and total chlorophyll content were similar, with AMF inoculation showing higher levels than non-inoculated plants without salt stress. The Chl b and total chlorophyll contents in G.m- and G.e-inoculated plants were dramatically higher than in control plants at 100 and 150 mM (P < 0.05, Figures 3D, E). The chlorophyll content in G.m-inoculated plants was significantly higher than in non-inoculated plants at 200 mM. MDA content increased with increasing salt concentrations. AMF inoculation resulted in lower MDA content compared to control plants at the same salt concentration, reaching a maximum at 200 mM, which was significantly different from control plants (P < 0.05, Figure 3F).
3.7 Effects on osmoregulatory substances of alfalfa
The trends for Pro and SP content were similar, increasing with rising salt concentrations. AMF-inoculated plants at the same salt concentrations had significantly higher levels than control plants (P < 0.05). The Pro content in alfalfa inoculated with both G.m and G.e reached the highest levels at 200 mM, with G.m showing the most significant increase from 43.13 to 111.12 μg/g (P < 0.05, Figure 3G). The SP content in plants inoculated with G.m and G.e was significantly higher at 50, 100, and 150 mM (P < 0.05), but the difference disappeared at 200 mM (P < 0.05, Figure 3H). SS content in alfalfa increased and then decreased with rising salt concentrations, peaking at 100 mM, where the difference between AMF-inoculated and control plants was significant (P < 0.05). No significant difference was observed at 200 mM (Figure 3I).
3.8 Effects on antioxidant enzyme activities of alfalfa
The O2·- production rate in alfalfa under salinity stress increased with NaCl concentration. There was no difference between AMF-inoculated and non-inoculated plants without salt stress. However, AMF-inoculated plants showed significantly lower O2·- production rates than non-inoculated plants with increasing salt concentration (P < 0.05, Figure 4A). Salt stress and AMF inoculation significantly impacted antioxidant enzyme activity. SOD, POD, and CAT activities showed similar trends, increasing with rising salt concentrations. The activity levels in AMF-inoculated plants were significantly higher compared to control plants (P < 0.05), with the maximum observed at 200 mM. SOD activity in plants inoculated with G.m and G.e increased by 131.69% and 113.10%, respectively, compared to the control at 50 mM (P < 0.05, Figure 4B). POD and CAT activities in plants inoculated with G.m were significantly higher than in those inoculated with G.e at high concentrations (P < 0.05, Figures 4C, D).
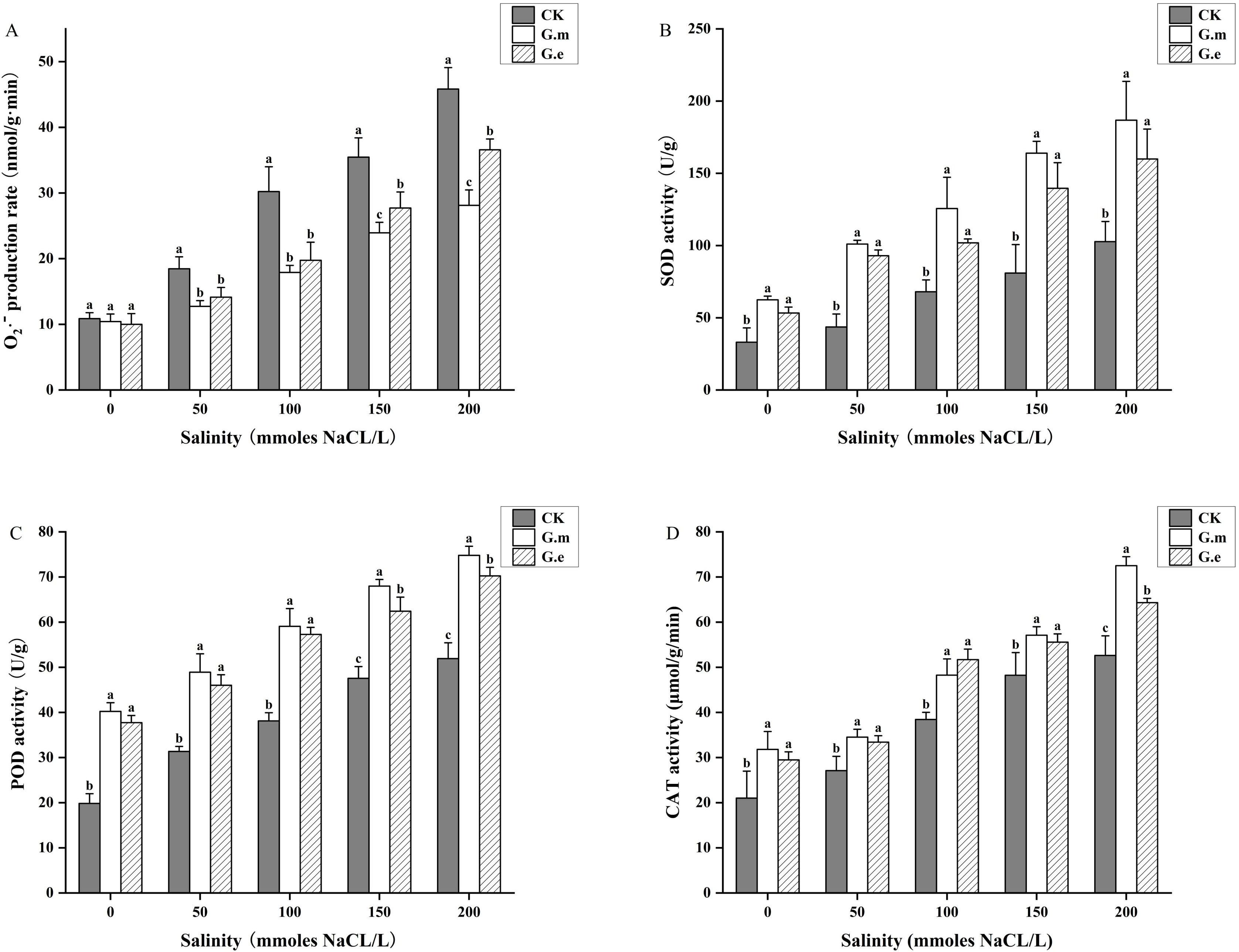
Figure 4. (A) O2·- production rate of alfalfa under different treatments. (B) SOD activity of alfalfa under different treatments. (C) POD activity of alfalfa under different treatments. (D) CAT activity of alfalfa under different treatments. Bar groups with different lowercase letters indicate significant differences (P < 0.05) between treatments under the same salt concentration. Data are means ± standard error.
3.9 Correlation coefficients between physiological indicators of alfalfa
Pearson correlation analysis of 12 physiological indicators of alfalfa inoculated with AMF under salt stress revealed several significant relationships. The Pro content was significantly positively correlated with plant height and MDA content. The SS content was highly obviously positively correlated with the Chl b content and significantly positively correlated with the Chl content. The SP content showed a significant positive correlation with SOD and POD activities. CAT activity was negatively correlated with Chl content, while SS content had a positive correlation with POD activity (Table 4).
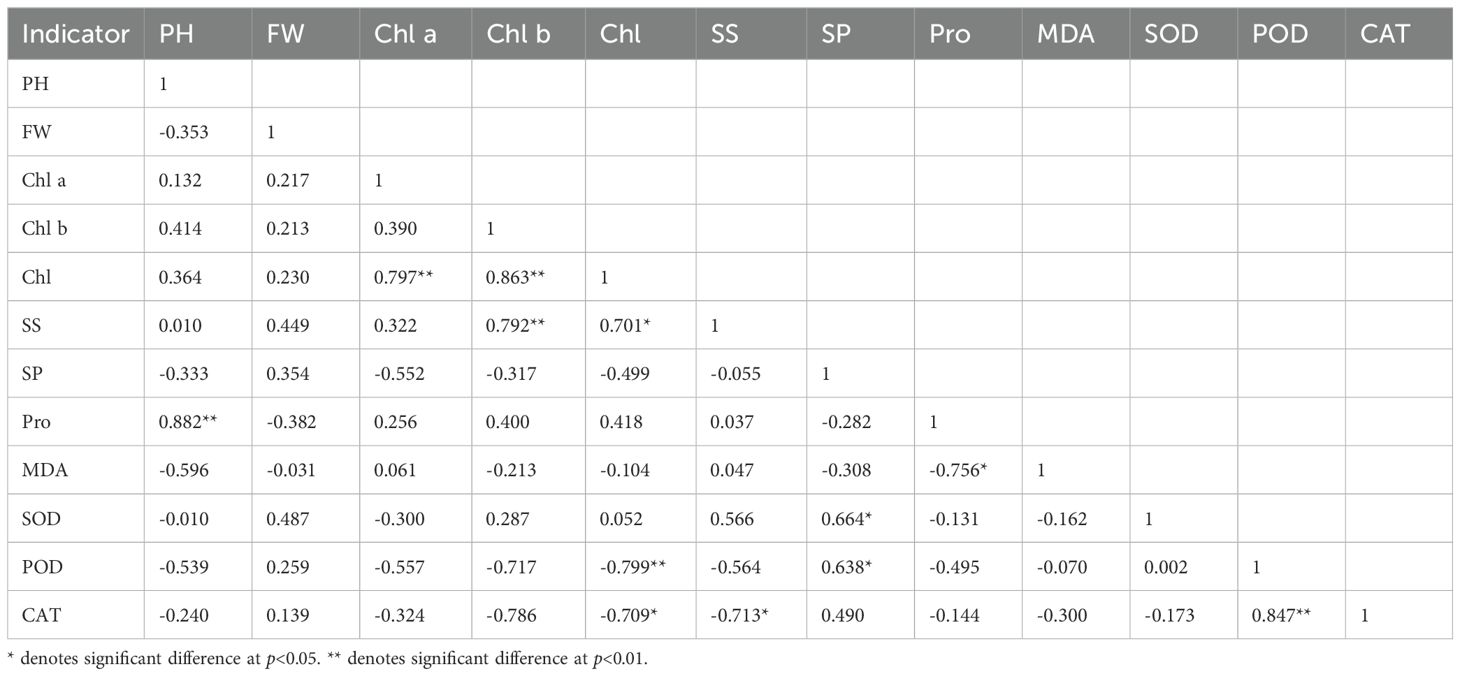
Table 4. Correlation coefficient of the physiological indicators of AMF-inoculated alfalfa under salt stress.
4 Discussion
4.1 AMF diversity of salinity-tolerant plants of Songnen grassland
AMF, as a type of “biofertilizer,” not only promotes the absorption of mineral elements and water in plants but also improves resistance to diseases and adverse conditions, which is crucial for agroforestry production (Marro et al., 2022). We analyzed the distribution and diversity of rhizosphere AMF in the Songnen saline-alkali grassland ecosystem in China. Different host plants have specific functions, physiological metabolisms, and root exudates, leading to varied AMF community diversity among different vegetation types (Kokkoris et al., 2020). AMF community also vary with soil conditions, nutritional status, and microbial group dynamics (Frew, 2019; Blažková et al., 2021), as different AMF have distinct nutrient acquisition capabilities (Zhang et al., 2021). A previous study showed that the soil nutrient levels on the eastern slope of the Helan Mountains first increased and then decreased with increasing altitude, and the diversity of soil AMF communities followed the same trend (Shao et al., 2024). Geographical distance and climatic conditions can also have indirect effects (Li et al., 2023b). In our study, 40 AMF species from six genera were identified from the rhizosphere soil using high-throughput sequencing, with Glomus detected as the dominant genus. Our results align with the dominant genera of rhizosphere AMF in Inula japonica and Iris lactea in the Songnen saline-alkali grassland. Among them, G. mosseae and G. etunicatum were isolated from all soil samples and were the most widely distributed AMF, indicating optimal affinity with the experimental plants. Furthermore, Glomus has been identified as a dominant genus in many studies on Songnen saline-alkali grasslands and in various ecosystems (Shao et al., 2024; Guo et al., 2022; Zheng et al., 2022). This phenomenon could be related to the spore formation pattern of Glomus and the suitable concentration of organic matter and N and P elements in the soil, improving its competitiveness (Avio et al., 2006). Different rhizosphere AMF species were observed in the rhizospheres of different plants, showing that different host plants affect the AMF distribution (Sýkorová et al., 2007).
The diversity and structure of soil microbial communities are crucial for soil function and the ecological environment (Lin et al., 2022). Research has shown that the diversity indices of rhizosphere AMF in different plant soils, such as Sobs, Chao, Shannon, and Simpson, vary significantly. Consistent with previous studies, plant communities greatly affect the AMF community diversity (Brigido et al., 2017). AMF and host plants exhibit a certain preference when forming symbiotic systems, and their affinity and mutual selectivity determine the survival and development of AMF to a significant extent (Croll et al., 2008; Wang et al., 2024a). This might be associated with the different nutritional requirements and types of root cells in various plants. Studies have found that differences in longitude, latitude, or altitude create varying water, light, temperature, and soil gradients, affecting the AMF diversity (Zhao et al., 2020). Furthermore, abiotic factors such as insect herbivores, climate change, N and P addition, and human interference influence AMF diversity (Mei et al., 2022b; Li et al., 2023b). A variety of rhizosphere AMF symbionts are distributed in the Songnen saline land, allowing for the selection and utilization of the optimal AMF. This provides a crucial theoretical basis for restoring saline-alkali land.
4.2 Effect of dominant AMF on alfalfa colonization rate
AMF can form mutualistic symbioses with plant roots, acting as a symbiotic rhizosphere barrier to decrease the harmful impact of salinity stress (Malik et al., 2022). The higher the mycorrhizal colonization rate, the stronger the symbiotic ability between plants and AMF. In this study, AMF inoculation on alfalfa resulted in a decrease in mycorrhizal colonization rate with increasing NaCl concentration. This indicates that factors affecting AMF colonization status are not only related to the affinity between plants and AMF but also to environmental factors, especially soil conditions that affect plant root growth (Rúa et al., 2016; Liu et al., 2023b). Research suggests that salt stress impacts mycorrhizal colonization by inhibiting spore germination and mycelial growth (Zhang et al., 2024b). There is a degree of mutual selectivity between AMF and host plants, and the AMF selected by plants may vary in different environments. The study showed that inoculation with G. mosseae resulted in a higher colonization rate than G. etunicatum under different NaCl stress levels. Plant AMF mycorrhizal dependence is an indicator of the symbiotic relationship between plants and AMF. In this experiment, mycorrhizal dependence showed an increasing and then decreasing trend with rising salt concentration, similar to the findings in maize (Ahmed et al., 2023). This may be because alfalfa can tolerate low salt concentrations, and AMF gradually plays a role as the salt concentration increases to 100 mmol/L. However, at 150 mmol/L, the growth of alfalfa is disrupted, inhibiting the elongation of AMF mycelium and reducing alfalfa’s mycorrhizal dependence. Additionally, reduced mycorrhizal dependence may also be due to the inhibition of AMF development and structure by salinity stress (Hashem et al., 2019).
4.3 Effects of dominant AMF on alfalfa
Plant growth and development are severely restricted by salinity stress (Guo et al., 2024). Salt stress is closely related to water status, increasing ion content and reducing the water absorption capacity of plants, leading to a rapid decrease in biomass (Li et al., 2019). Our study showed that the above-ground height and fresh weight of alfalfa decreased with increasing salt concentration. However, inoculation with AMF effectively improved plant height and fresh weight under certain salt stress conditions. This demonstrates that AMF inoculation can effectively promote the growth and development of alfalfa. AMF colonization can protect the host plant from the harmful impacts of salt stress (Santander et al., 2019). Our results agree with the findings that AMF colonization in wheat increased shoot length and fresh weight and that AMF inoculation increased the shoot biomass of tomato under salt treatment (Huang et al., 2023; Liu et al., 2023a). Inoculation with AMF under salt stress may expand the root absorption area, making it easier for plants to absorb water and nutrients, thus promoting growth (Wang et al., 2022).
Changes in chlorophyll content can reflect the impact of salinity stress on plant photosynthesis. In this study, increasing salt concentration had a significant negative impact on chlorophyll content in alfalfa leaves, consistent with previous studies (Hashem et al., 2018; Huang et al., 2023). The likely reason is that high salt concentrations affect the role of Mg in the plant, slowing down the chlorophyll synthesis rate. Chl a, Chl b, and total chlorophyll content in alfalfa inoculated with AMF at higher NaCl concentrations (100 and 150 mM) showed significant differences from control plants. Similar results have been observed in studies on tomato (Kong et al., 2020), indica rice (Tisarum et al., 2020), and Citrus aurantium (Hadian-Deljou et al., 2020). Notably, the differences reduced at 200 mM, with inoculation with G. mosseae being more effective than G. etunicatum. The findings indicated that the presence of AMF promoted chlorophyll synthesis within a certain range. This promotion may be due to AMF’s contribution to maintaining the intact ultrastructure of chloroplast thylakoids and mitochondria (Liu et al., 2023c) and upregulating the expression and activity of enzymes related to chlorophyll synthesis (Li et al., 2023a). Additionally, the increase in chlorophyll content in mycorrhizal plants may be due to the release of cytokinin-like substances by the fungus, promoting chloroplast development (Navarro et al., 2014).
Plants subjected to salt stress mobilize osmoregulatory mechanisms, produce osmoregulatory substances, and balance cell membrane permeability to resist injury (Xu et al., 2023). Our study found that SS, SP, and Pro content increased with rising salt concentrations and were significantly higher in AMF-inoculated plants compared to non-inoculated ones. This suggests that plants resist salinity stress by accumulating SS, SP, and Pro and that AMF can effectively alleviate the harmful effects of NaCl stress on the osmotic metabolic system. Similar results were observed in studies on poplar seedlings and Xanthoceras sorbifolium Bunge (Zong et al., 2023; Han et al., 2024). This indicates that AMF inoculation may increase the secretion of osmoregulatory substances under salinity stress, reduce osmotic potential, maintain normal cell metabolism, and improve plant salt resistance (Zong et al., 2023). Notably, there was no significant difference in SS content between AMF-inoculated and uninoculated plants at 200 mM, suggesting that excessively high salt concentrations may impair plant metabolic activity, reducing SS synthesis and accelerating decomposition.
As salt concentration increases, the plant’s antioxidant system fails to clear all reactive oxygen species (ROS), leading to membrane lipid peroxidation and increased production of MDA (Latef and Chaoxing, 2011; Ali et al., 2019). In this study, the MDA content in alfalfa increased with higher salt concentrations and was significantly reduced by AMF inoculation. This is consistent with the research of Wang et al., which showed that AMF inoculation in maize under salt stress reduced the MDA content (Wang et al., 2020). Increased MDA may be associated with the increase in antioxidant enzyme activities caused by oxidative damage (Wang et al., 2023). Salinity and other stressors can induce the formation of ROS, with O2·- being a particularly toxic type that is highly reactive and harmful, causing damage to proteins and lipids (Chen et al., 2020). AMF inoculation significantly reduced the O2·- production rate under salt stress compared to non-inoculated plants, indicating that AMF inoculation could reduce the degree of membrane lipid peroxidation in alfalfa.
Salt stress can cause lipid peroxidation, carbohydrate oxidation, and enzyme activity damage in cell membranes, leading to the production of excessive ROS that damage cells (Yan et al., 2021). Antioxidant enzymes such as SOD, POD, and CAT are commonly found in plants and help reduce damage to plant membrane systems under adverse conditions. AMF contribute to the direct or indirect clearance of ROS and increase antioxidant enzyme activity in plants under salinity stress (Mehta and Vyas, 2023), which is consistent with our results. We observed that SOD, POD, and CAT activities in alfalfa increased with rising salt concentrations and were significantly higher in AMF-inoculated plants compared to non-inoculated ones. Research has shown that AMF inoculation significantly increases antioxidant oxidase activities in Gossypium hirsutum and Cucumis sativus L. under salinity stress (Hashem et al., 2018; Zhang et al., 2024b). It can be inferred that AMF colonization benefits the synthesis of antioxidant enzymes and improves the defense system of antioxidant enzymes (Evelin et al., 2019). Moreover, the higher activity of antioxidant enzymes in AMF mycorrhizal plants could be related to the lower accumulation of lipid peroxidation (Latef and Chaoxing, 2011), resulting in reduced oxidative damage.
5 Conclusion
The diversity of AMF in the rhizospheres of eight common plants in the Songnen saline-alkali grassland and the impact of dominant native AMF species on the salt tolerance of alfalfa are explored for the first time. A total of 40 species of AMF from six genera were identified from rhizosphere soil samples of different plants in Songnen grassland. G.m and G.e were identified as dominant AMF species. The utility of these two dominant AMF species from the locations in alleviating salinity stress in pot-cultured alfalfa was tested in greenhouse conditions. The significant findings were that AMF improve the salt-alkali tolerance of alfalfa under salt stress by increasing the plant height, fresh weight, chlorophyll, SS, SP, Pro content, and SOD, POD, and CAT activities while reducing the MDA content. Future research should focus on multiple bacterial strains to improve alfalfa resistance, especially on cultivating and propagating multiple native bacterial strains, to provide a theoretical basis for the improvement of saline-alkali land.
Data availability statement
The raw data supporting the conclusions of this article will be made available by the authors, without undue reservation.
Author contributions
WX: Conceptualization, Data curation, Investigation, Methodology, Writing – original draft. QL: Data curation, Formal analysis, Writing – original draft. BW: Investigation, Software, Writing – review & editing. NZ: Methodology, Writing – review & editing. RQ: Investigation, Writing – original draft. YY: Resources, Writing – review & editing. MY: Formal analysis, Writing – original draft. FW: Visualization, Writing – original draft. LM: Conceptualization, Methodology, Supervision, Writing – review & editing. GC: Conceptualization, Funding acquisition, Project administration, Writing – review & editing.
Funding
The author(s) declare financial support was received for the research, authorship, and/or publication of this article. This work was supported by research and the Natural Science Foundation of Heilongjiang Province of China (LH2022C034); the National Natural Science Foundation of China (32201469) and Breeding Technology Innovation and New Provenance Creation of Cold-resistant and High-yield Alfalfa (2022ZD040120401).
Conflict of interest
The authors declare that the research was conducted in the absence of any commercial or financial relationships that could be construed as a potential conflict of interest.
Publisher’s note
All claims expressed in this article are solely those of the authors and do not necessarily represent those of their affiliated organizations, or those of the publisher, the editors and the reviewers. Any product that may be evaluated in this article, or claim that may be made by its manufacturer, is not guaranteed or endorsed by the publisher.
Supplementary material
The Supplementary Material for this article can be found online at: https://www.frontiersin.org/articles/10.3389/fpls.2024.1438771/full#supplementary-material
References
Ahmed, M., Abdel-Fattah, G. G., Holford, P., Korany, S. M., Alsherif, E. A., AbdElgawad, H., et al. (2023). Funneliformis constrictum modulates polyamine metabolism to enhance tolerance of Zea mays L. @ to salinity. Microbiological Res. 266, 127254. doi: 10.1016/j.micres.2022.127254
Akhzari, D., Mahdavi, S., Pessarakli, M., Ebrahimi, M. (2016). Effects of arbuscular mycorrhizal fungi on seedling growth and physiological traits of Melilotus officinalis L. grown under salinity stress conditions. Commun. Soil Sci. Plant Anal. 47, 822–831. doi: 10.1080/00103624.2016.1146897
Ali, A. Y. A., Ibrahim, M. E. H., Zhou, G., Nimir, N. E. A., Jiao, X., Zhu, G., et al. (2019). Ameliorative effects of jasmonic acid and humic acid on antioxidant enzymes and salt tolerance of forage sorghum under salinity conditions. Agron. J. 111, 3099–3108. doi: 10.2134/agronj2019.05.0347
Avio, L., Pellegrino, E., Bonari, E., Giovannetti, M. (2006). Functional diversity of arbuscular mycorrhizal fungal isolates in relation to extraradical mycelial networks. New Phytol. 172, 347–357. doi: 10.1111/j.1469-8137.2006.01839.x
Bates, L. S., Waldren, R., Teare, I. (1973). Rapid determination of free proline for water-stress studies. Plant Soil 39, 205–207. doi: 10.1007/BF00018060
Bhattarai, S., Liu, N., Karunakaran, C., Tanino, K. K., Fu, Y.-B., Coulman, B., et al. (2021). Tissue specific changes in elements and organic compounds of alfalfa (Medicago sativa L.) cultivars differing in salt tolerance under salt stress. J. Plant Physiol. 264, 153485. doi: 10.1016/j.jplph.2021.153485
Blažková, A., Jansa, J., Püschel, D., Vosátka, M., Janoušková, M. (2021). Is mycorrhiza functioning influenced by the quantitative composition of the mycorrhizal fungal community? Soil Biol. Biochem. 157, 108249. doi: 10.1016/j.soilbio.2021.108249
Brigido, C., Van Tuinen, D., Brito, I., Alho, L., Goss, M. J., Carvalho, M. (2017). Management of the biological diversity of AM fungi by combination of host plant succession and integrity of extraradical mycelium. Soil Biol. Biochem. 112, 237–247. doi: 10.1016/j.soilbio.2017.05.018
Cao, Y., Wu, X., Zhukova, A., Tang, Z., Weng, Y., Li, Z., et al. (2020). Arbuscular mycorrhizal fungi (AMF) species and abundance exhibit different effects on saline-alkaline tolerance in Leymus chinensis. J. Plant Interact. 15, 266–279. doi: 10.1080/17429145.2020.1802524
Chandrasekaran, M., Chanratana, M., Kim, K., Seshadri, S., Sa, T. (2019). Impact of arbuscular mycorrhizal fungi on photosynthesis, water status, and gas exchange of plants under salt stress–a meta-analysis. Front. Plant Sci. 10, 433629. doi: 10.3389/fpls.2019.00457
Chang, W., Sui, X., Fan, X.-X., Jia, T.-T., Song, F.-Q. (2018). Arbuscular mycorrhizal symbiosis modulates antioxidant response and ion distribution in salt-stressed Elaeagnus angustifolia seedlings. Front. Microbiol. 9, 338240. doi: 10.3389/fmicb.2018.00652
Chen, J., Zhang, H., Zhang, X., Tang, M. (2020). Arbuscular mycorrhizal symbiosis mitigates oxidative injury in black locust under salt stress through modulating antioxidant defence of the plant. Environ. Exp. Bot. 175, 104034. doi: 10.1016/j.envexpbot.2020.104034
Choi, J., Summers, W., Paszkowski, U. (2018). Mechanisms underlying establishment of arbuscular mycorrhizal symbioses. Annu. Rev. Phytopathol. 56, 135–160. doi: 10.1146/annurev-phyto-080516-035521
Croll, D., Wille, L., Gamper, H. A., Mathimaran, N., Lammers, P. J., Corradi, N., et al. (2008). Genetic diversity and host plant preferences revealed by simple sequence repeat and mitochondrial markers in a population of the arbuscular mycorrhizal fungus Glomus intraradices. New Phytol. 178, 672–687. doi: 10.1111/j.1469-8137.2008.02381.x
Cui, H., Li, Y., Wang, W., Chen, L., Han, Z., Ma, S., et al. (2023). Effects of male and female strains of salix linearistipularis on physicochemical properties and microbial community structure in saline–alkali soil. Microorganisms 11, 2455. doi: 10.3390/microorganisms11102455
Evelin, H., Devi, T. S., Gupta, S., Kapoor, R. (2019). Mitigation of salinity stress in plants by arbuscular mycorrhizal symbiosis: current understanding and new challenges. Front. Plant Sci. 10, 450967. doi: 10.3389/fpls.2019.00470
Evelin, H., Kapoor, R., Giri, B. (2009). Arbuscular mycorrhizal fungi in alleviation of salt stress: a review. Ann. Bot. 104, 1263–1280. doi: 10.1093/aob/mcp251
Farooq, M., Gogoi, N., Hussain, M., Barthakur, S., Paul, S., Bharadwaj, N., et al. (2017). Effects, tolerance mechanisms and management of salt stress in grain legumes. Plant Physiol. Biochem. 118, 199–217. doi: 10.1016/j.plaphy.2017.06.020
Ferreira, J. F., Cornacchione, M. V., Liu, X., Suarez, D. L. (2015). Nutrient composition, forage parameters, and antioxidant capacity of alfalfa (Medicago sativa, L.) in response to saline irrigation water. Agriculture 5, 577–597. doi: 10.3390/agriculture5030577
Frew, A. (2019). Arbuscular mycorrhizal fungal diversity increases growth and phosphorus uptake in C3 and C4 crop plants. Soil Biol. Biochem. 135, 248–250. doi: 10.1016/j.soilbio.2019.05.015
Garg, N., Chandel, S. (2011). Arbuscular mycorrhizal networks: process and functions. Sustain. Agric. 2, 907–930. doi: 10.1051/agro/2009054
Gong, H., Li, Y., Li, S. (2021). Effects of the interaction between biochar and nutrients on soil organic carbon sequestration in soda saline-alkali grassland: A review. Global Ecol. Conserv. 26, e01449. doi: 10.1016/j.gecco.2020.e01449
Guo, R., Zhou, Z., Cai, R., Liu, L., Wang, R., Sun, Y., et al. (2024). Metabolomic and physiological analysis of alfalfa (Medicago sativa L.) in response to saline and alkaline stress. Plant Physiol. Biochem. 207, 108338. doi: 10.1016/j.plaphy.2024.108338
Guo, Y., Zhang, H., Bao, Y., Tan, H., Liu, X., Rahman, Z. U. (2022). Distribution characteristics of soil AM fungi community in soft sandstone area. J. Environ. Manage. 316, 115193. doi: 10.1016/j.jenvman.2022.115193
Hadian-Deljou, M., Esna-Ashari, M., Mirzaie-Asl, A. (2020). Alleviation of salt stress and expression of stress-responsive gene through the symbiosis of arbuscular mycorrhizal fungi with sour orange seedlings. Scientia Hortic. 268, 109373. doi: 10.1016/j.scienta.2020.109373
Han, S., Cheng, Y., Wu, G., He, X., Zhao, G. (2024). Enhancing salt tolerance in poplar seedlings through arbuscular mycorrhizal fungi symbiosis. Plants 13, 233. doi: 10.3390/plants13020233
Hashem, A., Abd_Allah, E. F., Alqarawi, A. A., Wirth, S., Egamberdieva, D. (2019). Comparing symbiotic performance and physiological responses of two soybean cultivars to arbuscular mycorrhizal fungi under salt stress. Saudi J. Biol. Sci. 26, 38–48. doi: 10.1016/j.sjbs.2016.11.015
Hashem, A., Alqarawi, A. A., Radhakrishnan, R., Al-Arjani, A.-B. F., Aldehaish, H. A., Egamberdieva, D., et al. (2018). Arbuscular mycorrhizal fungi regulate the oxidative system, hormones and ionic equilibrium to trigger salt stress tolerance in Cucumis sativus L. Saudi J. Biol. Sci. 25, 1102–1114. doi: 10.1016/j.sjbs.2018.03.009
Helaoui, S., Boughattas, I., Hattab, S., Mkhinini, M., Alphonse, V., Livet, A., et al. (2020). Physiological, biochemical and transcriptomic responses of Medicago sativa to nickel exposure. Chemosphere 249, 126121. doi: 10.1016/j.chemosphere.2020.126121
Hu, S., Chen, Z., Vosatka, M., Vymazal, J. (2020). Arbuscular mycorrhizal fungi colonization and physiological functions toward wetland plants under different water regimes. Sci. Total Environ. 716, 137040. doi: 10.1016/j.scitotenv.2020.137040
Huang, S., Gill, S., Ramzan, M., Ahmad, M. Z., Danish, S., Huang, P., et al. (2023). Uncovering the impact of AM fungi on wheat nutrient uptake, ion homeostasis, oxidative stress, and antioxidant defense under salinity stress. Sci. Rep. 13, 8249. doi: 10.1038/s41598-023-35148-x
Jakobsen, I., Abbott, L., Robson, A. (1992). External hyphae of vesicular—arbuscular mycorrhizal fungi associated with Trifolium subterraneum L. 2. Hyphal transport of 32P over defined distances. New Phytol. 120, 509–516. doi: 10.1111/j.1469-8137.1992.tb01800.x
Jia, B., Cui, X., Zhang, Z., Li, X., Hou, Y., Luo, J., et al. (2024). Arbuscular mycorrhizal fungi regulate amino acid metabolism, phytohormones and glycolysis pathway to promote the growth of Suaeda salsa under combined Cd and NaCl stresses. Plant Physiol. Biochem. 214, 108921. doi: 10.1016/j.plaphy.2024.108921
Kakouridis, A., Hagen, J. A., Kan, M. P., Mambelli, S., Feldman, L. J., Herman, D. J., et al. (2022). Routes to roots: direct evidence of water transport by arbuscular mycorrhizal fungi to host plants. New Phytol. 236, 210–221. doi: 10.1111/nph.18281
Kokkoris, V., Lekberg, Y., Antunes, P. M., Fahey, C., Fordyce, J. A., Kivlin, S. N., et al. (2020). Codependency between plant and arbuscular mycorrhizal fungal communities: what is the evidence? New Phytol. 228, 828–838. doi: 10.1111/nph.16676
Kong, L., Gong, X., Zhang, X., Zhang, W., Sun, J., Chen, B. (2020). Effects of arbuscular mycorrhizal fungi on photosynthesis, ion balance of tomato plants under saline-alkali soil condition. J. Plant Nutr. 43, 682–698. doi: 10.1080/01904167.2019.1701029
Laouane, B., Meddich, A., Bechtaoui, N., Oufdou, K., Wahbi, S. (2019). Effects of arbuscular mycorrhizal fungi and rhizobia symbiosis on the tolerance of Medicago sativa to salt stress. Gesunde Pflanzen 71, 135–146. doi: 10.1007/s10343-019-00461-x
Latef, A. A. H. A., Chaoxing, H. (2011). Effect of arbuscular mycorrhizal fungi on growth, mineral nutrition, antioxidant enzymes activity and fruit yield of tomato grown under salinity stress. Scientia Hortic. 127, 228–233. doi: 10.1016/j.scienta.2010.09.020
Li, H., Zhang, L., Wu, B., Li, Y., Wang, H., Teng, H., et al. (2023a). Physiological and proteomic analyses reveal the important role of arbuscular mycorrhizal fungi on enhancing photosynthesis in wheat under cadmium stress. Ecotoxicology Environ. Saf. 261, 115105. doi: 10.1016/j.ecoenv.2023.115105
Li, J., Wang, X., Wu, J. H., Sun, Y. X., Zhang, Y. Y., Zhao, Y. F., et al. (2023b). Climate and geochemistry at different altitudes influence soil fungal community aggregation patterns in alpine grasslands. Sci. Total Environ. 881, 163375. doi: 10.1016/j.scitotenv.2023.163375
Li, R., Shi, F., Fukuda, K., Yang, Y. (2010). Effects of salt and alkali stresses on germination, growth, photosynthesis and ion accumulation in alfalfa (Medicago sativa L.). Soil Sci. Plant Nutr. 56, 725–733. doi: 10.1111/j.1747-0765.2010.00506.x
Li, S., Li, Y., He, X., Li, Q., Liu, B., Ai, X., et al. (2019). Response of water balance and nitrogen assimilation in cucumber seedlings to CO2 enrichment and salt stress. Plant Physiol. Biochem. 139, 256–263. doi: 10.1016/j.plaphy.2019.03.028
Li, S., Yang, W., Guo, J., Li, X., Lin, J., Zhu, X. (2020). Changes in photosynthesis and respiratory metabolism of maize seedlings growing under low temperature stress may be regulated by arbuscular mycorrhizal fungi. Plant Physiol. Biochem. 154, 1–10. doi: 10.1016/j.plaphy.2020.05.025
Lin, Y., Li, B., Zhang, P., Sun, S., Cui, G. (2022). Leymus chinensis resists degraded soil stress by modulating root exudate components to attract beneficial microorganisms. Front. Microbiol. 13, 951838. doi: 10.3389/fmicb.2022.951838
Liu, S., Guo, X., Feng, G., Maimaitiaili, B., Fan, J., He, X. (2016). Indigenous arbuscular mycorrhizal fungi can alleviate salt stress and promote growth of cotton and maize in saline fields. Plant Soil 398, 195–206. doi: 10.1007/s11104-015-2656-5
Liu, M.-Y., Li, Q.-S., Ding, W.-Y., Dong, L.-W., Deng, M., Chen, J.-H., et al. (2023a). Arbuscular mycorrhizal fungi inoculation impacts expression of aquaporins and salt overly sensitive genes and enhances tolerance of salt stress in tomato. Chem. Biol. Technol. Agric. 10, 5. doi: 10.1186/s40538-022-00368-2
Liu, J., Li, Z., Ghanizadeh, H., Kerckhoffs, H., Sofkova-Bobcheva, S., Wu, W., et al. (2020). Comparative genomic and physiological analyses of a superoxide dismutase mimetic (SODm-123) for its ability to respond to oxidative stress in tomato plants. J. Agric. Food Chem. 68, 13608–13619. doi: 10.1021/acs.jafc.0c04618
Liu, Y., Lu, J., Cui, L., Tang, Z., Ci, D., Zou, X., et al. (2023c). The multifaceted roles of Arbuscular Mycorrhizal Fungi in peanut responses to salt, drought, and cold stress. BMC Plant Biol. 23, 36. doi: 10.1186/s12870-023-04053-w
Liu, X.-Q., Xie, M.-M., Hashem, A., Abd-Allah, E. F., Wu, Q.-S. (2023b). Arbuscular mycorrhizal fungi and rhizobia synergistically promote root colonization, plant growth, and nitrogen acquisition. Plant Growth Regul. 100, 691–701. doi: 10.1007/s10725-023-00966-6
Malik, J. A., AlQarawi, A. A., Dar, B. A., Hashem, A., Alshahrani, T. S., AlZain, M. N., et al. (2022). Arbuscular mycorrhizal fungi isolated from highly saline “Sabkha Habitat“ soil alleviated the NaCl-induced stress and improved Lasiurus scindicus Henr. growth. Agriculture 12, 337. doi: 10.3390/agriculture12030337
Marro, N., Grilli, G., Soteras, F., Caccia, M., Longo, S., Cofré, N., et al. (2022). The effects of arbuscular mycorrhizal fungal species and taxonomic groups on stressed and unstressed plants: a global meta-analysis. New Phytol. 235, 320–332. doi: 10.1111/nph.18102
McKenney, M. C., Lindsey, D. L. (1987). Improved method for quantifying endomycorrhizal fungi spores from soil. Mycologia 79, 779–782. doi: 10.1080/00275514.1987.12025458
Mehta, D., Vyas, S. (2023). Comparative bio-accumulation of osmoprotectants in saline stress tolerating plants: A review. Plant Stress 9, 100177. doi: 10.1016/j.stress.2023.100177
Mei, L., Zhang, P., Cui, G., Yang, X., Zhang, T., Guo, J. (2022b). Arbuscular mycorrhizal fungi promote litter decomposition and alleviate nutrient limitations of soil microbes under warming and nitrogen application. Appl. Soil Ecol. 171, 104318. doi: 10.1016/j.apsoil.2021.104318
Mei, L., Zhang, N., Wei, Q., Cui, G. (2022a). Alfalfa modified the effects of degraded black soil cultivated land on the soil microbial community. Front. Plant Sci. 13, 938187. doi: 10.3389/fpls.2022.938187
Moradi, A. (2016). Effect of mycorrhizal inoculation on growth, nitrogen fixation and nutrient uptake in alfalfa (Medicago sativa) under salt stress. Cercetări Agronomice în Moldova XLIX 1(165), 67–80. doi: 10.1515/cerce-2016-0006
Navarro, J. M., Pérez-Tornero, O., Morte, A. (2014). Alleviation of salt stress in citrus seedlings inoculated with arbuscular mycorrhizal fungi depends on the rootstock salt tolerance. J. Plant Physiol. 171, 76–85. doi: 10.1016/j.jplph.2013.06.006
Ortega-Villasante, C., Rellán-Alvarez, R., Del Campo, F. F., Carpena-Ruiz, R. O., Hernández, L. E. (2005). Cellular damage induced by cadmium and mercury in Medicago sativa. J. Exp. Bot. 56, 2239–2251. doi: 10.1093/jxb/eri223
Qin, W., Yan, H., Zou, B., Guo, R., Ci, D., Tang, Z., et al. (2021). Arbuscular mycorrhizal fungi alleviate salinity stress in peanut: Evidence from pot-grown and field experiments. Food Energy Secur. 10, e314. doi: 10.1002/fes3.314
Rúa, M. A., Antoninka, A., Antunes, P. M., Chaudhary, V. B., Gehring, C., Lamit, L. J., et al. (2016). Home-field advantage? Evidence of local adaptation among plants, soil, and arbuscular mycorrhizal fungi through meta-analysis. BMC Evolutionary Biol. 16, 1–15. doi: 10.1186/s12862-016-0698-9
Santander, C., Sanhueza, M., Olave, J., Borie, F., Valentine, A., Cornejo, P. (2019). Arbuscular mycorrhizal colonization promotes the tolerance to salt stress in lettuce plants through an efficient modification of ionic balance. J. Soil Sci. Plant Nutr. 19, 321–331. doi: 10.1007/s42729-019-00032-z
Shahbaz, M., Ashraf, M. (2013). Improving salinity tolerance in cereals. Crit. Rev. Plant Sci. 32, 237–249. doi: 10.1080/07352689.2013.758544
Shao, L., Yan, P., Ye, S., Bai, H., Zhang, R., Shi, G., et al. (2024). Soil organic matter and water content affect the community characteristics of arbuscular mycorrhizal fungi in Helan mountain, an arid desert grassland area in China. Front. Microbiol. 15, 1377763. doi: 10.3389/fmicb.2024.1377763
Shi-Chu, L., Yong, J., Ma-Bo, L., Wen-Xu, Z., Nan, X., Hui-hui, Z. (2019). Improving plant growth and alleviating photosynthetic inhibition from salt stress using AMF in alfalfa seedlings. J. Plant Interact. 14, 482–491. doi: 10.1080/17429145.2019.1662101
Shrivastava, P., Kumar, R. (2015). Soil salinity: A serious environmental issue and plant growth promoting bacteria as one of the tools for its alleviation. Saudi J. Biol. Sci. 22, 123–131. doi: 10.1016/j.sjbs.2014.12.001
Sýkorová, Z., Wiemken, A., Redecker, D. (2007). Cooccurring Gentiana verna and Gentiana acaulis and their neighboring plants in two Swiss upper montane meadows harbor distinct arbuscular mycorrhizal fungal communities. Appl. Environ. Microbiol. 73, 5426–5434. doi: 10.1128/AEM.00987-07
Tani, E., Sarri, E., Goufa, M., Asimakopoulou, G., Psychogiou, M., Bingham, E., et al. (2018). Seedling growth and transcriptional responses to salt shock and stress in Medicago sativa L., Medicago arborea L., and their hybrid (Alborea). Agronomy 8, 231. doi: 10.3390/agronomy8100231
Tisarum, R., Theerawitaya, C., Thongpoem, P., Singh, H. P., Cha-um, S. (2020). Alleviation of salt stress in upland rice (Oryza sativa L. ssp. indica cv. Leum Pua) using arbuscular mycorrhizal fungi inoculation. Front. Plant Sci. 11, 487251. doi: 10.3389/fpls.2020.00348
Wang, J., Gao, X., Wang, J., Song, J., Zhu, Z., Zhao, J., et al. (2024a). Host plants directly determine the α diversity of rhizosphere arbuscular mycorrhizal fungal communities in the National Tropical Fruit Tree Field Genebank. Chem. Biol. Technol. Agric. 11, 20. doi: 10.1186/s40538-024-00540-w
Wang, H., Li, J., Liu, H., Chen, S., Zaman, Q. U., Rehman, M., et al. (2023). Variability in morpho-biochemical, photosynthetic pigmentation, enzymatic and quality attributes of potato for salinity stress tolerance. Plant Physiol. Biochem. 203, 108036. doi: 10.1016/j.plaphy.2023.108036
Wang, H., Liang, L., Liu, B., Huang, D., Liu, S., Liu, R., et al. (2020). Arbuscular mycorrhizas regulate photosynthetic capacity and antioxidant defense systems to mediate salt tolerance in maize. Plants 9, 1430. doi: 10.3390/plants9111430
Wang, Q., Liu, M., Huang, D. (2024b). The role of arbuscular mycorrhizal symbiosis in plant abiotic stress. Front. Microbiol. 14, 1323881. doi: 10.3389/fmicb.2023.1323881
Wang, L., Seki, K., Miyazaki, T., Ishihama, Y. (2009). The causes of soil alkalinization in the Songnen Plain of Northeast China. Paddy Water Environ. 7, 259–270. doi: 10.1007/s10333-009-0166-x
Wang, F., Zhang, L., Zhou, J., Rengel, Z., George, T. S., Feng, G. (2022). Exploring the secrets of hyphosphere of arbuscular mycorrhizal fungi: processes and ecological functions. Plant Soil 481, 1–22. doi: 10.1007/s11104-022-05621-z
Xu, X., Guo, L., Wang, S., Wang, X., Ren, M., Zhao, P., et al. (2023). Effective strategies for reclamation of saline-alkali soil and response mechanisms of the soil-plant system. Sci. Total Environ. 905, 167179. doi: 10.1016/j.scitotenv.2023.167179
Yan, F., Wei, H., Ding, Y., Li, W., Liu, Z., Chen, L., et al. (2021). Melatonin regulates antioxidant strategy in response to continuous salt stress in rice seedlings. Plant Physiol. Biochem. 165, 239–250. doi: 10.1016/j.plaphy.2021.05.003
Zhang, J., Quan, C., Ma, L., Chu, G., Liu, Z., Tang, X. (2021). Plant community and soil properties drive arbuscular mycorrhizal fungal diversity: A case study in tropical forests. Soil Ecol. Lett. 3, 52–62. doi: 10.1007/s42832-020-0049-z
Zhang, D.-J., Tong, C.-L., Wang, Q.-S., Bie, S. (2024b). Mycorrhizas affect physiological performance, antioxidant system, photosynthesis, endogenous hormones, and water content in cotton under salt stress. Plants 13, 805. doi: 10.3390/plants13060805
Zhang, C., Xiang, X., Yang, T., Liu, X., Ma, Y., Zhang, K., et al. (2024a). Nitrogen fertilization reduces plant diversity by changing the diversity and stability of arbuscular mycorrhizal fungal community in a temperate steppe community. Sci. Total Environ. 918, 170775. doi: 10.1016/j.scitotenv.2024.170775
Zhao, F., Feng, X., Guo, Y., Ren, C., Wang, J., Doughty, R. (2020). Elevation gradients affect the differences of arbuscular mycorrhizal fungi diversity between root and rhizosphere soil. Agric. For. Meteorology 284, 107894. doi: 10.1016/j.agrformet.2019.107894
Zhao, Y., Zhang, Z., Li, Z., Yang, B., Li, B., Tang, X., et al. (2023). Comprehensive study on saline-alkali soil amelioration with sediment of irrigation area in northeast China. Arabian J. Chem. 16, 104608. doi: 10.1016/j.arabjc.2023.104608
Zheng, Z., Ma, X., Zhang, Y., Liu, Y., Zhang, S. (2022). Soil properties and plant community-level traits mediate arbuscular mycorrhizal fungal response to nitrogen enrichment and altered precipitation. Appl. Soil Ecol. 169, 104245. doi: 10.1016/j.apsoil.2021.104245
Keywords: arbuscular mycorrhizal fungi, alfalfa, salinization, rhizosphere soil, growth-promoting
Citation: Xu W, Liu Q, Wang B, Zhang N, Qiu R, Yuan Y, Yang M, Wang F, Mei L and Cui G (2024) Arbuscular mycorrhizal fungi communities and promoting the growth of alfalfa in saline ecosystems of northern China. Front. Plant Sci. 15:1438771. doi: 10.3389/fpls.2024.1438771
Received: 26 May 2024; Accepted: 30 July 2024;
Published: 29 August 2024.
Edited by:
Muhammad Waseem, Hainan University, ChinaReviewed by:
Joseph George Ray, Mahatma Gandhi University, IndiaTao Zhang, Northeast Normal University, China
Copyright © 2024 Xu, Liu, Wang, Zhang, Qiu, Yuan, Yang, Wang, Mei and Cui. This is an open-access article distributed under the terms of the Creative Commons Attribution License (CC BY). The use, distribution or reproduction in other forums is permitted, provided the original author(s) and the copyright owner(s) are credited and that the original publication in this journal is cited, in accordance with accepted academic practice. No use, distribution or reproduction is permitted which does not comply with these terms.
*Correspondence: Linlin Mei, z11425@neau.edu.cn; Guowen Cui, cgw603@163.com