- Urban Horticulture and Sustainability Laboratory, Texas Tech University, Plant and Soil Science, Lubbock, TX, United States
Soilless production systems (i.e hydroponics, aeroponics, aquaponics) have become commonplace in urban settings and controlled environments. They are efficient nutrient recyclers, space savers, and water conservers. However, they lack high levels of biological richness in the root microbiome when compared to soil production systems, which may affect plant health and nutrient uptake. To address this issue and incorporate more sustainable practices, beneficial microorganisms (i.e. Trichoderma spp., Bacillus sp.) can be added in the form of biofertilizers. However, many factors affect impacts of microorganisms and their interactions with plants. In this experiment, Black Summer Pac Choi (Brassica rapa var. Chinensis) was grown for two trials in a Deep-Water system (DWS) or a Nutrient Film Technique system (NFT) with commercial biofertilizers containing Trichoderma spp., Bacillus amyloliquefaciens, a combination of both, and a control. Plant physiology, nutrient composition, and nutrient uptake efficiency (NUE) were generally negatively affected by Trichoderma spp. both growing systems, indicating that Trichoderma may not be recommended for hydroponic production. However, Bacillus amyloliquefaciens showed promise as an effective biofertilizer in the NFT systems and had a positive influence on NUE in DWS.
1 Introduction
Various forms of hydroponics in urban food production systems are set to increase in the near future to accommodate 55-68% of the global population living in cities (Rajaseger et al., 2023). Hydroponics are often used in greenhouses and urban farms as they allow for an environment that can easily be controlled and optimized for high-value crops (i.e., nutrient additions, water conservation, biofortification, temperature) (Sambo et al., 2019). Hydroponic systems are widely used to produce leafy green vegetables that are of high value. The vast majority of these being leafy greens (Walters et al., 2020). However, most hydroponic setups lack natural levels of biodiversity in the rhizosphere. This lack of biodiversity has been shown to decrease the efficiency of nutrient uptake by plants, increase disease susceptibility, and decrease bioavailable nutrients (Lin et al., 2021; Setiawati et al., 2023; Kumar et al., 2022; Chaudhary et al., 2022). Biofertilizers can be added to hydroponic systems’ nutrient reservoirs and rhizospheres to remedy the lack of biodiversity.
Biofertilizers are generally defined as mixtures of microorganisms (e.g., fungi, bacteria) added to the rooting environment to promote a crop’s growth, plant health, and nutrient uptake (Daniel et al., 2022; Mohammadi and Sohrabi, 2012; Zhao et al., 2024). Due to the positive associations that can be formed, these can also be termed beneficial microorganisms. Various microorganisms, such as Bacillus spp., Trichoderma spp., Azospirillum spp., and Pseudomonas spp., can be used as biofertilizers (Reed and Glick, 2023). Inoculations can be made through lab-grown populations or more easily accessible commercial products. Often, mixtures are added to the rhizosphere of crops grown in soil to achieve the desired effects of increased growth, biomass, and harvestable material (Dasgan et al., 2023). However, these desired effects may not be as easily reached in hydroponics due to rhizosphere environmental differences between soil-based and soilless production systems and compatibility challenges with various crops.
Biofertilizers used in hydroponic systems have varied in composition and ranged from microalgae (Chlorella vulgaris), arbuscular mycorrhizal fungi (AMF), plant growth promoting rhizobacteria (PGPR), Bacillus spp., Pseudomonas fluorescens, Glomus spp., Gigaspora margarita, Azobacter sp., Azospirillum, sp., and many others have been explored (Dasgan et al., 2023; Kumar et al., 2022; Dasgan et al., 2022; Singh et al., 2023; Chaudhary et al., 2022). These systems have ranged from nutrient film technique (NFT), to floating raft, dutch bucket, deep water, and other systems (Dasgan et al., 2023; Ikiz et al., 2024; McClintic et al., 2024; Zhang et al., 2023). In these systems, researchers found that biofertilizers increased leaf physiological characteristics, plant yield, nutrient uptake, and higher phenol and flavonoid contents (Zhang et al., 2023; Ikiz et al., 2024; Dasgan et al., 2023). However, McClintic et al. (2024) found that the inoculation of Azopirillum and Rhizophagus sp. had limited effects on nutrient uptake and growth in tomatoes and lettuce. This variation in effects could indicate factors such as environment, storage, pH, incompatibility, nutrient competition, form, that may influence performance of biofertilizers (Karapetyan, 2023). Biofertilizers can be affected by storage, humidity, temperatures, or formulation issues that may be detrimental to live microorganisms (Karapetyan, 2023; Jain, 2019; Pirttilä et al., 2021). Hydroponic conditions may further complicate the relationship between plants and biofertilizers because of the nature of the nutrient and water solution. While liquid formulations may be preferable because of solubility and ability to apply via foliar spray, or interactions may be more efficient with root inoculation (Sarbani and Yahaya, 2022). However, several studies have shown positive impacts of foliar sprays of different biofertilizers, including increased enzyme activity, metabolites, nutrient concentration, mitigated effects of salinity, and increased biomass (Razmjooei et al., 2022; Vela Coyotl et al., 2018; Guimarâes et al., 2020). Yet one of the primary reasons for utilizing biofertilizers is to facilitate nutrient uptake or efficiency of uptake for organic nutrients. Current research has proven the efficacy of biofertilizers to facilitate uptake of organic nutrients, and sometimes inorganic nutrients is inconsistent (McClintic et al., 2024; Priadi and Nuro, 2017). This may be a function of different growing conditions, hydroponic conditions, plant species, or fertilizer, however, this must be studied further to determine exact causes and correlations between biofertilizers and their performance. Moreover, biofertilizer behavior can change based on environmental stress (Backer et al., 2018; Malusà et al., 2016). Vast environmental differences exist between soil-based production and soilless production systems, which may be a source of stress for populations of microorganisms. Some of these differences include particle porosity (which provides contact points for colonization) (Girvan et al., 2003; Vieira et al., 2020), available oxygen, leaching, and nutrient availability (i.e., affects symbiosis behavior) (Malusà et al., 2016). Yet biofertilizers have shown promise in mitigating negative plant responses to stressors (Chaudhary et al., 2022). Various biofertilizers have inhibited pathogen growth, regulated signaling pathways, increased gene expression, improved plant growth, proline synthesis, secondary metabolites, among many other responses (Chaudhary et al., 2022). For example, Guimarâes et al. (2020) found that biofertilizer mitigated some of the negative effects of salt stress in hydroponic lettuce. Further, Yasmin et al. (2020) found that priming soybean (Glycine max) seeds with Pseudomonas pseudoalcaligenes improved salt tolerance by increasing defense responses as compared to Bacillus subtilis. This contrasts with findings by Mokabel et al. (2022), who found that Bacillus subtilis had enhanced pigment concentrations, upregulated defense compounds, and enzymes compared to uninoculated eggplants (Solanum melongena). This further demonstrates the wide variability between crops, even when treated with similar stressors and the same biofertilizer.
Biofertilizers also have different compatibilities with desired crops. For example, mung beans (Vigna radiata L.) have been shown to react positively with Rhizobium spp., Trichoderma viride, and Pseudomonas putida, while rice nearly doubled in nitrogen (N), potassium (K), and phosphorus (P) concentrations after being given only Rhizobium isolates (Gangwar et al., 2013; Khanam et al., 2022). In addition, Trichoderma spp. has been documented to positively affect crops across taxonomic families and species (Kubheka and Ziena, 2022). For example, Chinese cabbage with different Trichoderma fungi applied via irrigation resulted in a significant increase in cabbage yield (by 37%) and enhanced enzyme activity in the soil (Ji et al., 2020). Trichoderma azevedoi also increased chlorophyll content in lettuce while decreasing white mold severity (Silva et al., 2021). Due to this pattern, Trichoderma was chosen as a representative commercial biofertilizer.
To further examine specific biofertilizers, Bacillus and Trichoderma, the following studies have been reviewed. For the case of Bacillus, maize given Bacillus pumilus resulted in a 30% increase in ear yield, but no significant results were documented when given Pseudomonas moraviensis (Kuan et al., 2016; Lin et al., 2018). In addition, various strains of Bacillus subtilis, Bacillus megaterium, and Bacillus pumilus have resulted in rate of growth increases, stress tolerances, and nutrient metabolites in lettuce and tomatoes (Radhakrishnan et al., 2017). Furthermore, Bacillus subtilis and Bacillus cereus positively impacted Chinese cabbage growth and nutrients (Kang et al., 2019; Ku et al., 2018). Due to this pattern, Bacillus was chosen as a representative commercial biofertilizer. Bacillus strains have not always resulted in positive impacts on plant growth, with a study by Moncada et al. (2021), showing reduced nitrate in basil leaves. Although few of these studies exploring biofertilizers have shown negative results there is significant evidence that these microorganisms can improve crop production factors and efficiency. There are indications that the consistency and benefits vary with crop and must be studied further.
While work has been accomplished to build a library of crop-biofertilizer compatibility, more research must be done to expand this library and address associated with the rhizosphere environment in hydroponics. Thus, the aim and objective of this study was to determine how common and commercially available microorganisms affect the growth, physiology, nutrient concentration, and nutrient uptake efficiency (NUE) of Black Summer Pac Choi (Brassica rapa var. chinensis) grown in Deep-Water System (DWS) and Nutrient Film Technique (NFT) hydroponics. We hypothesized that Bacillus amyloliquefaciens would enhance nutrient uptake, while Trichoderma spp. had more competitive interaction effects on Pac Choi grown in different hydroponic systems.
2 Materials and methods
2.1 Experimental conditions
This experiment was conducted in two separate trials in a controlled greenhouse environment at the Texas Tech University Horticulture Gardens and Greenhouse facility. Black Summer Pac Choi (Brassica rapa var. chinensis) was grown for five weeks in two seasons (September 2022 and March 2023) in NFT and DWS hydroponic systems. During plant growth, greenhouse temperature averaged 27.3°C in trial 1 and 29.6°C in trial 2. A temperature and humidity sensor (tempi.fi, Woburn, MA, USA) was suspended in the air between two levels of the NFT system, and a separate temperature and humidity sensor (HOBO, Bourne, MA, USA) was adjusted to leaf level in the DWS hydroponics system. The university greenhouse facilities preset the temperature, and readings were collected after each second in both trials and then averaged every hour.
2.2 Experimental setup
Each set up was conducted for two experimental trials. A DWS hydroponic system consisted of 12 seven L storage containers. Each container lid had six holes of 2 in diameter (5.08 cm) to accommodate net pots for plant placement (72 plants in total). Plastic tubing was connected to air pumps and fed through the lid to provide aeration to the roots. Supplemental lighting was provided by two LumiGrow TopLight Node light fixtures (42.6 in × 4.1 in) (LumiGrow, Emeryville, CA, USA) (λ: 400-700 nm) for six hours a day to achieve photosynthetically active radiation (PAR) between 405-407 µmol m-2 s-1 and an average DLI of 12 (mol m-2 day-1). DWS hydroponic containers were organized in a random block design with a treatment assigned randomly to each container. Three containers in total represented each treatment with a total of 18 sample replicates per treatment. Black Summer Pac Choi was also grown in an NFT hydroponic system that consisted of two towers with two levels per tower. Supplemental lighting was given for each level with KEGIAN light fixtures (23.6 in × 9.5 in)(Amazon, Seattle, WA, USA)(λ: 400-750 nm). Light PPFD output was adjusted at the bottom levels to reach approximately the same light intensity across all treatments and levels. After adjustments, average PAR and DLI were recorded as 405-407 µmol m-2 s-1 and 12 mol m-2 day-1, respectively. Each NFT tower level was assigned with an experimental treatment at random based on a random block design. Each treatment was assigned a matching sample replicate amount (18) as the DWS. Treatments described below were applied to the reservoirs of each system throughout the trials at the rates specified by the commercial manufacturer. The growth of a mycelial mat in all Trichoderma spp. treatment reservoirs during Trial 1 resulted in weekly reservoir changes. Trial 2 reservoirs were not completely drained and only filled when EC or water levels dipped below optimum. Both deep-water and NFT hydroponic systems received a 1:1 ratio of two liquid fertilizers Floragro (2-1-6) and Floramicro (5-0-1) (General Hydroponics, Santa Rosa, CA, USA) to maintain an EC (electrical conductivity) (Groline, Hanna Instruments, Smithfield, RI, USA) range of 1.5-2.5 ppm as well as received pH stabilizers to maintain a pH range of 5.5-7.5.
2.3 Microorganism treatments and inoculation
Experimental treatments consisted of three unique microorganism inoculations and a non-inoculated control. A commercial biofertilizer containing Bacillus amyloliquefaciens (Botanicare, Vancouver, WA, USA) was added directly to both DWS and NFT reservoirs at the inoculation rate of 2 mL/gal (1.0×104 CFU/mL). A separate commercial biofertilizer blend containing Trichoderma harzianum and Trichoderma viride (Mikrobs, Suwanee, GA, USA) was added directly to both DWS and NFT reservoirs at the inoculation rate of 2.5 g/gal (2.0x107 CFU/g). A third treatment consisted of a combination of Bacillus amyloliquefaciens and Trichoderma spp. commercial biofertilizers at an inoculation rate of 2 mL/gal and 2.5 g/gal, respectively. Inoculation rates were applied according to the biofertilizer manufacturer’s instructions. Both of the biofertilizers are known to associate with many plants and are endophytic in nature (Zalila-Kolsi et al., 2023). Bacillus amyloliquefaciens has also been associated with a wide variety of plants and is commonly found in the environment (Zalila-Kolsi et al., 2023). Further, Trichoderma has shown plant growth promotion effects on a wide variety of crops, due to production of auxin compounds and capacity for solubilizing nutrients like phosphate (Zin and Badaluddin, 2020; Tyśkiewicz et al., 2022). Research by Poveda and Eugui (2022) has also shown beneficial and synergistic effects on plants inoculated with both Trichoderma and Bacillus spp. Because both biofertilizers have been used on Pac choi successfully in other studies (Hirst et al., 2024) and are known to associate with many species, no compatibility tests were performed.
2.4 Physical analysis
2.4.1 Growth index
Growth Index (GI) was calculated using Equation 1 according to the cylindrical shape of Pac Choi. GI measurements were collected and calculated every week of growth during Trial 2. GI was collected and calculated for all experimental replicates. Results were than plotted to show change in growth index over the period of five weeks.
W1 = Width from north-south
W2 = Width from east-west
h = plant height from base of stem to tip of leaves
2.5 Chemical analysis
2.5.1 Nutrient uptake efficiency
After the fifth week of growth, all replicate Black Summer Pac Choi leaf and stem samples were harvested, freeze-dried (Harvest Right, Salt Lake City, UT, USA) and shipped to Waters Agricultural Laboratories Inc. (Camilla, GA, USA) for a general nutrient analysis. All sample replicates were tested for concentrations of Nitrogen (N), Potassium (K), Phosphorus (P), Magnesium (Mg), Calcium (Ca), Sulfur (S), Boron (B), Zinc (Zn), Manganese (Mn), Iron (Fe), and Copper (Cu). After analysis, nutrient uptake efficiency (NUE) was calculated using Equation 2 according to (Dobermann, 2007)
gS = grams NPK in shoots
gA = grams of NPK applied
2.6 Statistics
JMP 16.0.0 (SAS, Cary, NC, USA) software was used for standard least squares factorial analysis. Each trial performed was analyzed separately, and a single plant was considered one replication for each analysis. After each trial, 72 replicates were analyzed for physical and chemical analysis. Criteria for significant differences were set at p ≤ 0.05, and a student’s t-test further tested significant differences to determine mean separation.
3 Results
3.1 Physiology and growth
3.1.1 Harvest results
In trial 1, the control and Bacillus amyloliquefaciens treatments resulted in significantly greater fresh shoot weight and root length in both systems (Table 1). However, when systems were analyzed separately, Pac Choi grown in NFT during trial 1 resulted in significantly higher fresh shoot weight in the control and Bacillus treatments, followed by the Trichoderma and combination treatments (p ≤ 0.0001; Table 1). Differences in root weight were seen between treatments, which yielded a significantly greater root weight in the Trichoderma spp. treatment in the first trial (p=0.0068). Root length was similar in both the DWS and NFT systems, where the control and Bacillus treatments had longer roots than those in the Trichoderma and combination treatments. This was further supported by the root:shoot analysis, which showed greater values in the Trichoderma containing treatments, particularly in the NFT system.
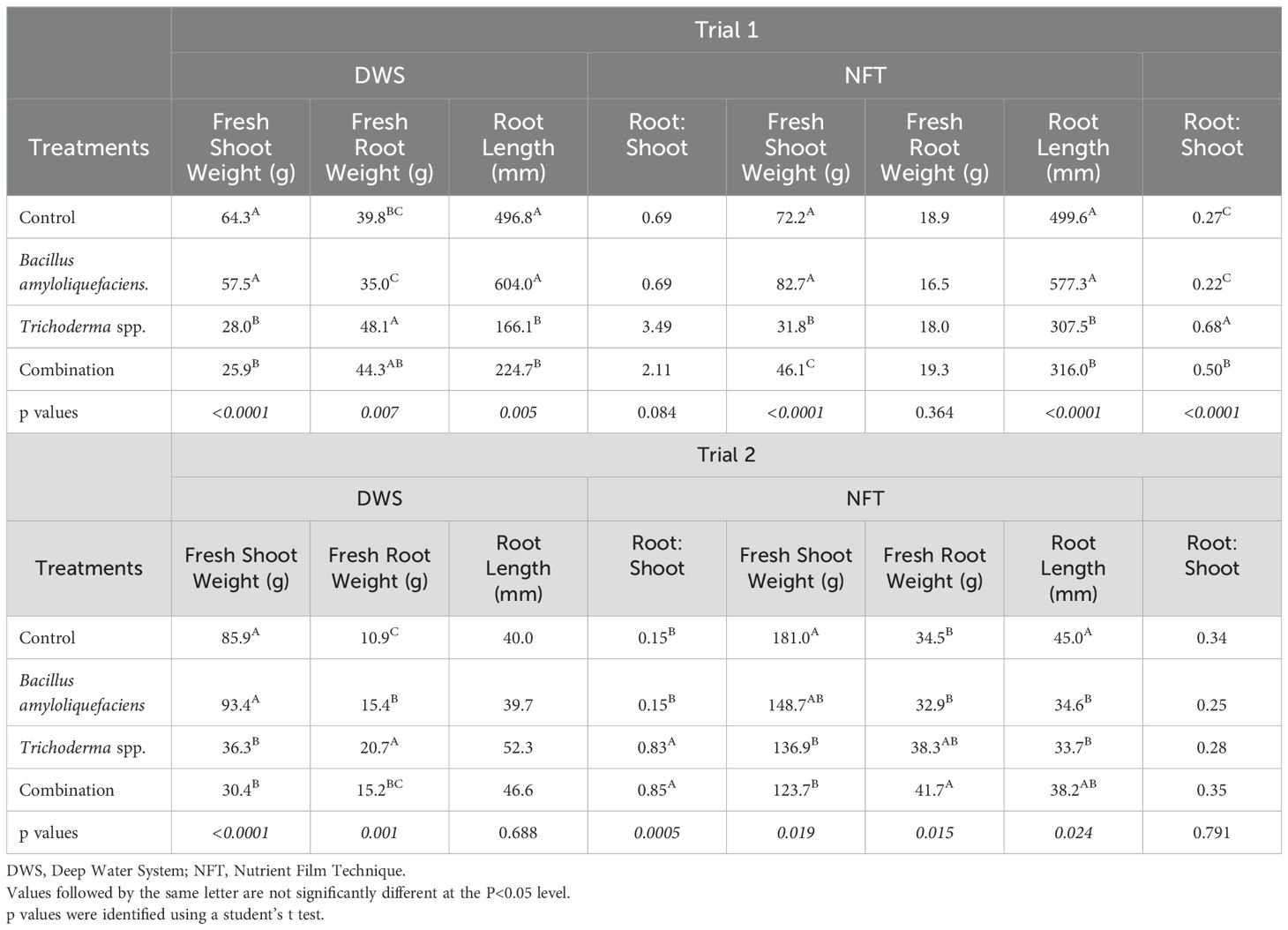
Table 1. Measurements of the fresh shoot and root weight (g) and root length (mm) of microorganism treatments during Trial 1 and Trial 2.
In trial 2, pac choi grown in DWS culture yielded significantly greater fresh shoot weight in the control and Bacillus amyloliquefaciens treatments (p ≤ 0.0001; Table 1). Additionally, trial 2 Pac Choi grown in NFT yielded significantly greater fresh weight and root length in the control treatments (p=0.0190, p=0.0237, respectively). The root weight of Pac Choi grown in DWS was significantly greater in the Trichoderma spp., followed by the Bacillus, combination, and control treatments (Table 1). In the NFT system, the root weight of plants was significantly greater in the combination treatment, followed by the Trichoderma treatment; however, there was no significant difference between the control and Bacillus treatments (p=0.0010, p=0.0150). The root:shoot analysis also reflected similar findings with Trichoderma containing treatments having higher values, except in trial 2, these were only significant in the DWS units.
3.1.2 Growth index
In the trial 2 DWS treatment, Pac Choi, in week 3 of growth, had a significantly higher growth index in the Bacillus amyloliquefaciens and control treatments (p ≤ 0.0001, p ≤ 0.0001, respectively; Figure 1), while the Trichoderma and combination treatments were statistically similar but lower than the other treatments. By the fourth week of growth, plants in the Bacillus amyloliquefaciens treatment had a greater growth index in the NFT culture only (p ≤ 0.0001). After the fifth and final weeks of growth, no significant differences existed between treatments for growth index in NFT culture (p=0.1812). However, in DWS culture, Bacillus amyloliquefaciens and control-treated Pac Choi had a significantly greater growth index, followed by Trichoderma and the combination treatment DWS (p ≤ 0.0001).
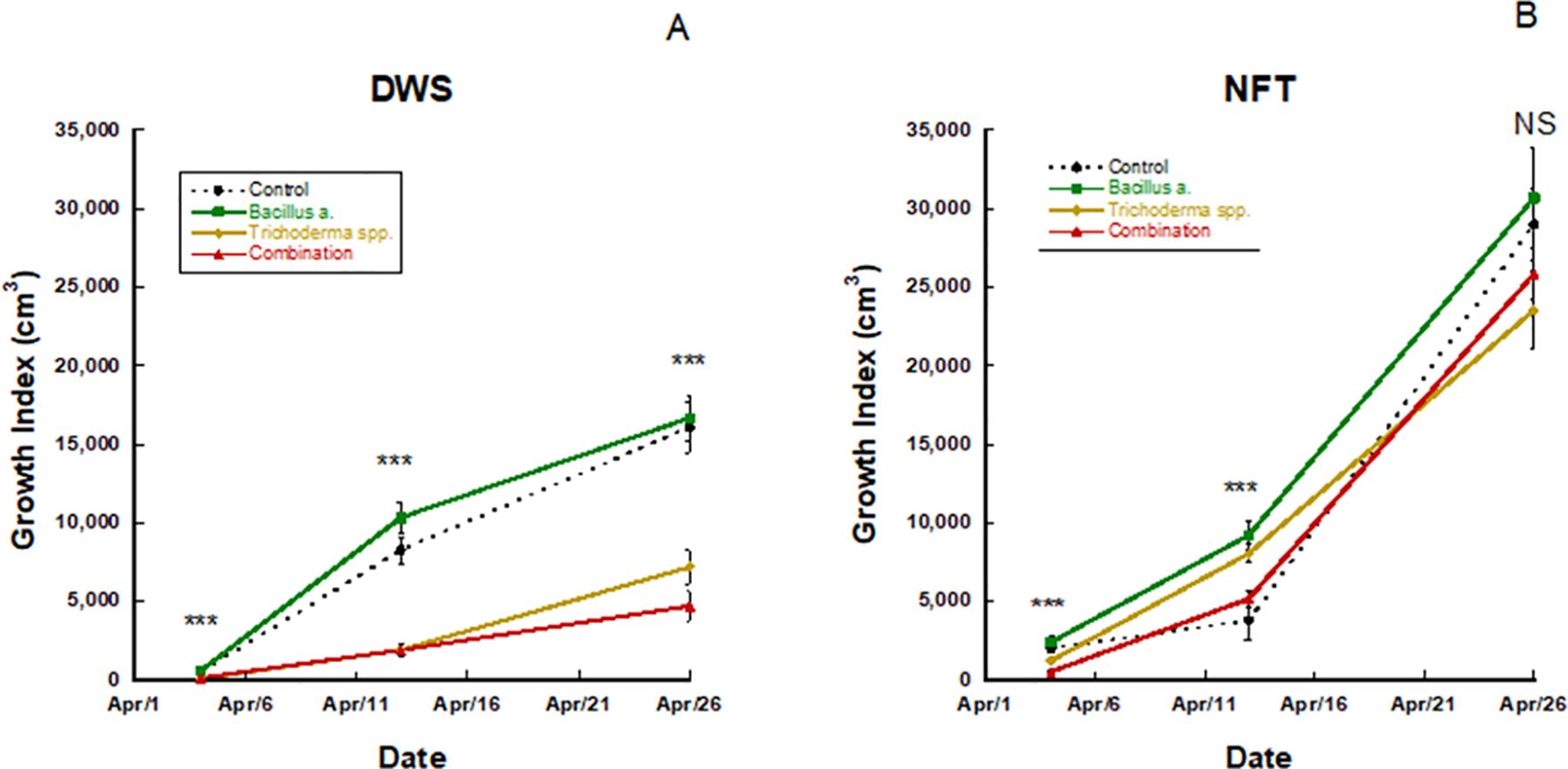
Figure 1. Pac choi growth index for (A) deep water (DWS) and (B) nutrient film technique (NFT) systems treated with different biofertilizers. The order of significance is represented by p<0.0001 (***). NS denotes no significant differences found. P-values were identified using a student's t-test. Bars represent ±1 standard error of the mean.
3.2 Plant nutrition
3.2.1 Nutrient content
After the first trial, the control treatment yielded significantly greater concentrations of K in Pac Choi tissues in both hydroponic systems (Table 2). Furthermore, the control plants contained significantly greater Fe in the DWS-grown Pac Choi (p=0.023, Table 2). The control and Bacillus amyloliquefaciens treatments behaved similarly with increased Cu and P concentrations in Pac Choi grown in DWS (p=0.0004 for Cu, p=0.0003 for P, Table 2) as well as significantly greater N, Ca, and Zn concentrations in NFT grown Pac Choi (p<0.0001 for N, p=0.0006 for Ca, p=0.0093 for Zn, Table 2). For Pac Choi given the Trichoderma spp. treatment, plants contained significantly greater B concentrations in both systems (p=0.0006 for DWS, p ≤ 0.0001 for NFT, Table 2) but only significantly increased S concentrations in NFT (p=0.0002, Table 2). At the same time, the combination-treated plants contained significantly greater Mg concentrations in both systems (p=0.0006 for DWS, p=0.0008 for NFT, Table 2). Finally, DWS-grown Pac Choi contained significantly greater nutrient concentrations for all nutrients significantly affected by treatment than NFT-grown Pac Choi.
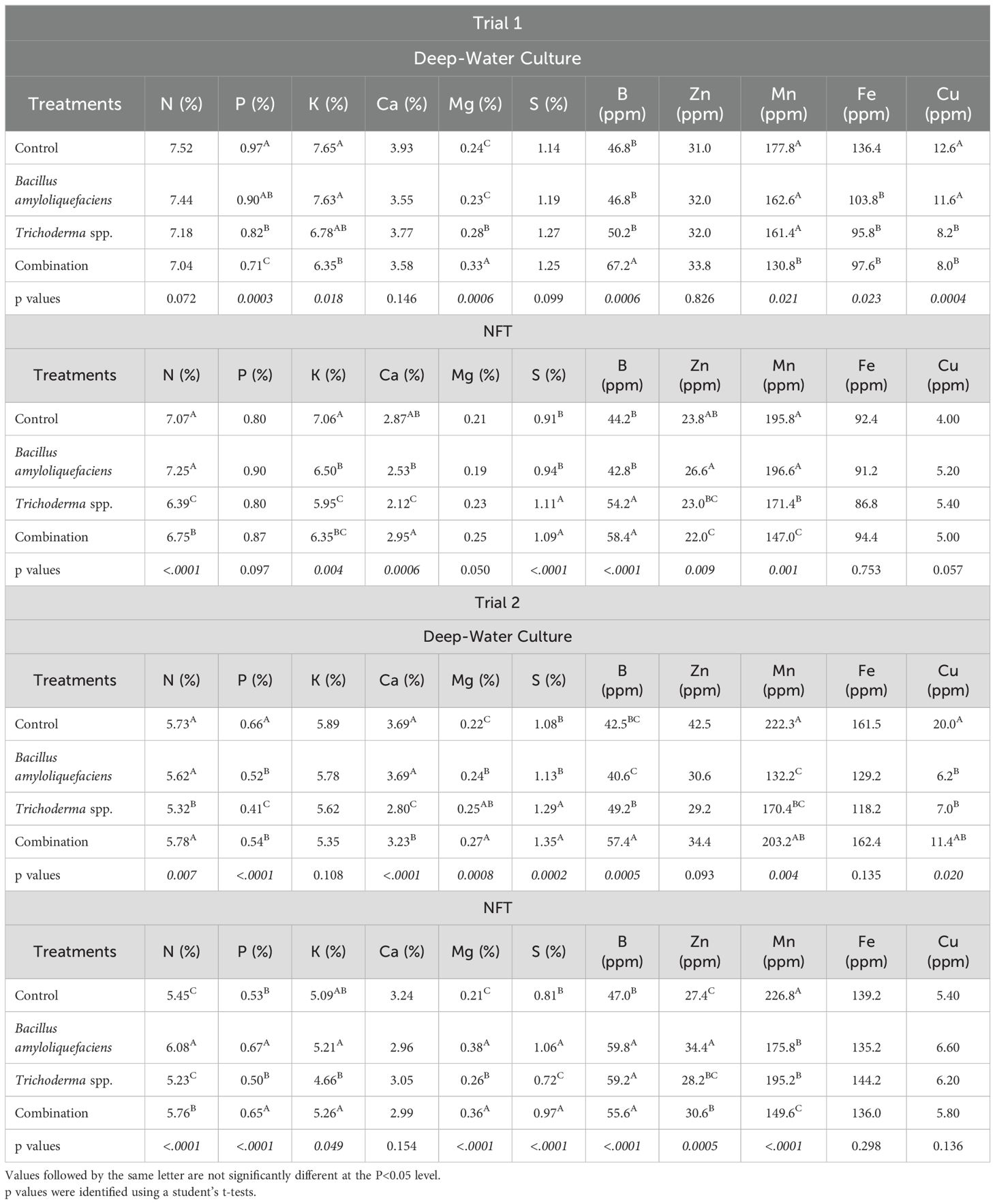
Table 2. Nutrient concentrations of Pac Choi in different treatments and production systems for Trial 1 and Trial 2.
After the second trial, the control treatment yielded Pac Choi with significantly greater concentrations of Mn in both systems (Table 2). Additionally, trial 2 DWS Pac Choi had greater concentrations of P and Cu when given the control treatment (p ≤ 0.0001 for P, p=0.02 for Cu, Table 2) but had significantly greater B concentrations in NFT (p ≤ 0.0001, Table 2). The Bacillus amyloliquefaciens and combination treatments showed higher Mg levels in plants grown in both systems during trial 2 (p=0.004 for DWS, p ≤ 0.0001 for NFT, Table 2), but the two treatments also showed higher P and K in the NFT culture only (p=0.0001 for P, p=0.049 for K, Table 2). Pac Choi grown with the combination treatment contained significantly greater Ca and B concentrations in DWS culture only (p=0.0006 for Ca, p=0.0005 for B, Table 2).
3.2.2 Nutrient uptake efficiency
During the first trial, Pac Choi grown with the control treatment had the greatest P and K uptake efficiency in both systems (p=0.0013, Table 3). However, the Bacillus amyloliquefaciens treated plants did not significantly differ in P and K nutrient uptake efficiency in the DWS. However, Pac Choi grown under the control and Bacillus amyloliquefaciens treatments contained significantly greater N uptake efficiency in NFT culture only (p=0.004, Table 3). In trial 1, lower nutrient uptake efficiencies were generally seen in the combination or Trichoderma spp. treatments. In trial 2, Pac Choi grown with Bacillus amyloliquefaciens and the combination treatment contained significantly greater N uptake efficiency in DWS (p=0.0002, Table 3). Bacillus amyloliquefaciens containing cultures yielded plants with greater K uptake efficiency (p=0.0003, Table 3) but were not significantly greater than the combination treatments. The combination treatment yielded significantly greater P uptake efficiency (p=0.0058, Table 3) followed by the control, Bacillus amyloliquefaciens., and Trichoderma spp. treatments. The NFT system had no significant differences between treatments for any nutrient uptake efficiencies in trial 2.
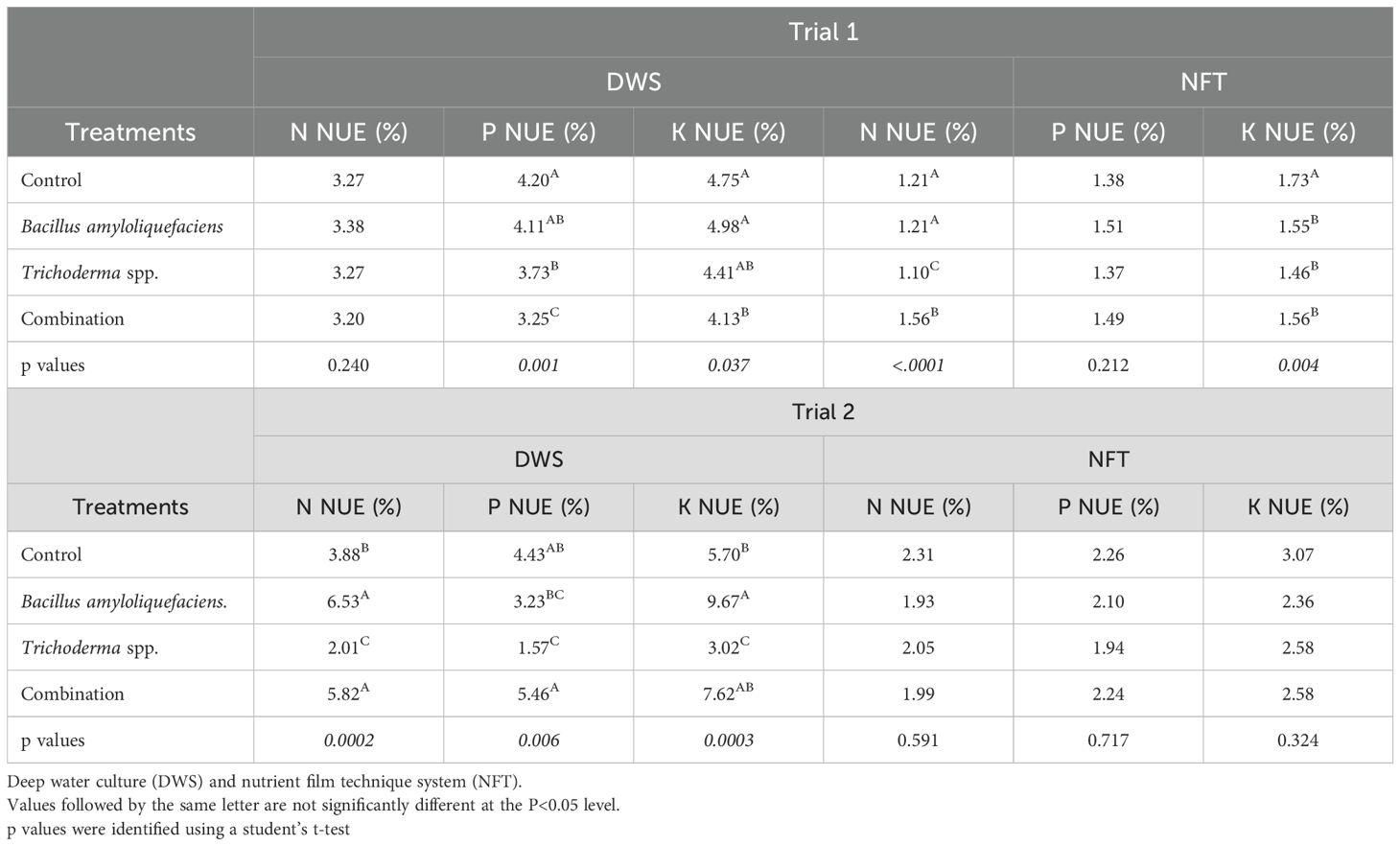
Table 3. Nitrogen (N), Phosphorous (P), and Potassium (K) Nutrient Uptake Efficiency (NUE) (%) of microorganism treatments during Trial 1 and Trial 2.
4 Discussion
Interest in biofertilizers and beneficial fungi has grown in recent years. They have been widely shown to improve plant yields and performance through associations with different crops. These biofertilizers are an essential element to sustainable horticulture and urban agriculture, whether the intended results are increased yield, decreased plant pathogens, or both (Gül et al., 2008; Kıdoğlu et al., 2009; Lee and Lee, 2015). For example, Dasgan et al. (2022) found that Basil (Ocimum basilicum L. ‘Dino’) had increased N, P, K, Mg, and Fe concentrations along with yield and leaf area when given bacterial, mycorrhizal, and micro-algae biofertilizers in a hydroponic setting. Tyśkiewicz et al. (2022) also explored the current uses and limitations of Trichoderma spp. as a biocontrol agent for plant pathogens and the properties associated with plant growth promotion. Further, Reed and Glick (2023) discussed the variable relationship between biofertilizers and vegetable crops while Backer et al. (2018) discussed how the root environment can alter compatibility and behavior. This discrepancy between biofertilizer and plant associations has not been exhaustively studied due to the wide variety of plants and biofertilizers available. Furthermore, there are limited studies on their impacts and efficacy in hydroponic production systems. To achieve optimal beneficial effects from biofertilizers, compatibility between microorganisms and plants and the rhizosphere environment must be studied.
4.1 Growth promotion
Biofertilizers are added to the soil environment of vegetable crops for their plant growth-promoting properties. For example, adding a Bacillus amyloliquefaciens strain increased tomatoes’ fresh shoot and root growth (Samaras et al., 2021). Furthermore, the growth-promoting properties of Bacillus amyloliquefaciens have been recorded in tea plants, where bud weight was increased by 22% in plants that had been inoculated (Bai et al., 2014). Results from our study show similar impacts of Bacillus amyloliquefaciens on hydroponically grown Black Summer Pac Choi, where growth-promoting properties were seen in fresh weight, root length, and growth index. However, these results vary with the microorganism and crop studied. Interestingly, there were few interaction effects of production system and biofertilizer treatment on plant growth factors. This could indicate that patterns may be independent of systems producing the plants or that the effects of the treatments are similar in each system.
In this study, Trichoderma spp. negatively impacted fresh weight, root length, and growth index of Pac Choi. This contrasts with other experiments where Trichoderma biofertilizers increased plant growth. These conflicting results may be due to the difference in production systems used. Trichoderma has shown primarily beneficial impacts in soil-based studies, while there were few if any benefits in hydroponic systems. For example, Bader et al. (2020) demonstrated that Trichoderma harzianum promoted soil-grown tomato (Solanum lycopersicum L.) shoot growth and increased the fresh weight of both shoots and roots. In addition, Dabire et al. (2016) concluded that soil-grown onion root tissues, fresh weight, and the number of leaves increased up to 30 days after inoculating seeds and seedling beds with a native strand of Trichoderma harzianum. In a hydroponic system, Moreira et al. (2022) also showed that lettuce yield was increased after applying Trichoderma harzianum to a reservoir with reduced EC. Similarly, cucumbers grown in a gnotobiotic hydroponic system experienced as much as a 40% increase in dry weight of shoots after inoculation with Trichoderma harzianum (Yedidia et al., 2001). However, our data conflict with these findings and show that applying multiple Trichoderma spp. reduced Black Summer Pac Choi growth. This could have been a function of species found in the commercial blend, application rates, and/or other factors that affect the growth of Trichoderma in the hydroponic systems. The presence of a mycelial mat attached to the root system of both DWS and NFT could also explain this behavior. The closed-loop recirculation of nutrient solutions in the hydroponics system is likely to encourage the growth of Trichoderma spp., potentially leading to larger colonies that could compete with plants for nutrients. As Trichoderma is a very aggressive and opportunistic fungus (Schuster and Schmoll, 2010), the large population likely outcompeted the Black Summer Pac Choi for nutrients and oxygen. Large colonies were observed to surround and become attached to the roots of plants in both the NFT and DWS hydroponics. This observation is further quantified in Table 1 where fresh root weight was significantly greater in treatments containing Trichoderma. This could also explain why plants, given this biofertilizer, grew smaller overall. This could possibly be mitigated by using lower inoculation rates, because those provided by the manufacturer may not be conducive for hydroponic production. However, future research should expand on this to determine what factors affect Trichoderma colonization in hydroponic systems.
4.2 Impacts on nutrition of Black Summer Bac Choi
After a complete nutrient evaluation, the control and Bacillus amyloliquefaciens treatments behaved similarly with few exceptions. According to the results shown in Table 2, Bacillus amyloliquefaciens had the potential to facilitate the increase concentrations of certain nutrients (N, P, K, Cu, Zn, Mg) in DWS and NFT hydroponics but may not perform better than a system without biofertilizers. This information differs from other sources that claim Bacillus spp. increased plant nutrition in both soil systems and hydroponics (Luo et al., 2022; Oliveira et al., 2023; Sharma et al., 2013; Wang et al., 2022). However, Luo et al. (2022); Sharma et al. (2013), and Wang et al. (2022) all argue that the mechanisms for increasing shoot nutrition occur through increasing nutrient availability during environmental stress. One reason for the similarity between the control treatment and the Bacillus amyloliquefaciens treatment could be that there was already an abundance of bioavailable nutrients before the biofertilizer was applied, and thus no additional benefits could be achieved. As a second argument, the inoculation rates or compatibility between Bacillus amyloliquefaciens would show similar results to those seen in this study. However, evidence suggests compatibility between Bacillus spp. and Chinese cabbage. Ku et al. (2018) demonstrated that field inoculations of Bacillus cereus resulted in an increased yield of Chinese cabbage. Kang et al. (2019) also demonstrated that soil inoculation of Bacillus subtilis increased nutrient uptake in Chinese cabbage. Nevertheless, inadequate inoculations would also provide evidence for the results demonstrated in this study and the use of inorganic nutrients instead of organic nutrients. Inorganic nutrients are often used in hydroponics as they allow for better pH control, easily adjustable rates for plant growth stage, and limit phytotoxic organic compounds (Torres and Somera, 2023). However, the commercial blend recommended pairing the inoculation with organic nutrients as the biofertilizer aided in providing bioavailable nutrients.
On the other hand, Trichoderma spp. resulted in negative impacts on most nutrient concentrations in the Black Summer Pac Choi shoot tissue. While there was no sign of any nutrient insufficiency (Uchida, 2000), concentrations were significantly reduced compared to the control treatment. Even when combined with Bacillus amyloliquefaciens, only B, S, and Mg concentrations were higher than control. Moreira et al. (2022) document similar results where inoculation of Trichoderma harzianum in hydroponically grown lettuce resulted in no effects on nutrient concentration. The results continue to indicate that Trichoderma is likely competing with the Pac Choi plants for nutrients in the nutrient solutions, as discussed in the previous section. Since fewer nutrients were taken into the shoots and roots, less growth could occur in the Pac Choi. The benefits of Trichoderma spp. seen in soil systems may be due to more soil microorganisms that compete or the natural soil structure that allows for more beneficial associations with plant roots (Chewapanich et al., 2021; Giurgiu et al., 2018; Promwee and Intana, 2022).
Black Summer Pac Choi treated with Bacillus amyloliquefaciens did not have a significantly greater NUE than the control in either trial of DWS and NFT. One suggestion is that there was no aid in nutrient uptake from Bacillus amyloliquefaciens This is a direct contradiction to de Sousa et al. (2021) and Wang et al. (2023), where nutrient uptake was increased by Bacillus spp. in both soil and hydroponic systems. However, these results match nutrient concentration results. The lack of significant effects of Bacillus amyloliquefaciens on nutrient concentrations in Pac Choi could be due to the abundance of bioavailable nutrients already possessed in the fertilizer additions. Since there were no nutrient deficiencies, Bacillus amyloliquefaciens likely had little effect on nutrient uptake. Mourouzidou et al. (2023) support this process and present that Bacillus spp. can increase nutrient uptake during N and Fe deficiencies and saline environments. This is also supported by the manufacturer’s recommendations for use with organic fertilizers, as discussed previously. Alternatively, the potential compatibility, or lack thereof, could explain these effects between species. If Black Summer Pac Choi is incompatible with Bacillus amyloliquefaciens, similar results would be shown between the control treatment and Bacillus amyloliquefaciens treatment in nutrient concentration and NUE.
Nutrient uptake efficiency varied with treatments, and while Trichoderma-containing treatments were, on average, lower in NUE than others, these findings were inconsistent between trials and systems. Additionally, N NUE and K NUE showed significant interaction effects between treatments and systems. This showed that the treatments did have different effects in different systems, but in general the deep water grown plants had higher NUE. Overall, there was a reduction of NUE occurring in both DWS and NFT for N, P, and K treated with Trichoderma. Both physiology and nutrient concentration results have suggested that the presence of Trichoderma spp. at the inoculation rate likely outcompeted Black Summer Pac Choi for nutrients. Moreover, results from the NUE analysis indicate that the Trichoderma fungus negatively impacted the ability of the plant to take up nutrients due to competition. At the inoculation rates used, Trichoderma spp. is not a beneficial biofertilizer for Black Summer Pac Choi grown in DWS and NFT hydroponics. In their study, Li et al. (2015) discusses both patterns of symbiosis between Trichoderma spp. and soil-grown tomatoes. Under Cu-deficient conditions, Trichoderma spp. increased the Cu intake of tomato seedlings by 42%. However, under P and Zn-deficient conditions, Trichoderma spp. was shown to compete with and negatively impact the P and Zn concentrations of tomato seedlings. This suggests that more research must examine fertilizer composition, inoculation rates and population sizes of Trichoderma spp. in hydroponics.
Commercial hydroponic production focuses on efficient and rapid production of crops, primarily leafy green vegetables. One of the primary issues with hydroponic production is that the use of organic fertilizers is not recommended because of the necessity for microorganisms to mineralize nutrients for absorption (McClintic et al., 2024). The use of microorganisms and biofertilizers that aid in this process is necessary to facilitate this but can offer other benefits as previously discussed. However, the practical application of commercial biofertilizers must be examined in different crops and production systems. This research demonstrates that manufacturers recommended rates may not be practical for use in hydroponic production due to mycelial mat formation and the proliferation of Trichoderma supplied with adequate nutrients. These studies replicated greenhouse grower conditions and, as such, showed that the results often promised are not always delivered. Consequently, future research should investigate different inoculation rates of biofertilizers, the mechanisms of the interactions between plants and biofertilizers, and different formulations or ratios of the biofertilizers or organisms.
5 Conclusion
The use of Bacillus amyloliquefaciens as a biofertilizer for Black Summer Pac Choi in a hydroponic environment has the potential for positive effects, especially for an early harvest. The effects of Bacillus were primarily seen on plant physiology and nutrient uptake but weren’t significantly different from the control in most cases. However, the use of Trichoderma spp. at the tested inoculation rate is not recommended. Trichoderma negatively affected yield and aerial tissue factors, and while root data indicate that roots were larger, it was due to mycelial mats that accumulated. While both production systems showed positive impacts on growth, NFT grown plants were generally larger.Future research should aim to refine inoculation methods and rates for biofertilizers and test additional biofertilizer types for Black Summer Pac Choi. Further research must be completed to evaluate the differences in biofertilizer behavior in organically and inorganically fertilized systems.
Data availability statement
The raw data supporting the conclusions of this article will be made available by the authors, without undue reservation.
Author contributions
GP: Conceptualization, Data curation, Investigation, Methodology, Visualization, Writing – original draft, Writing – review & editing. DR: Methodology, Writing – original draft, Writing – review & editing. CS: Conceptualization, Formal analysis, Project administration, Resources, Supervision, Validation, Visualization, Writing – original draft, Writing – review & editing.
Funding
The author(s) declare financial support was received for the research, authorship, and/or publication of this article. Funding for this project includes the USDA REEU Nutrition BEST program (REEU Project #: TEXW-2020-09346), the USDA NLGCA Award#2018-70001-32215, and the Texas Tech university TrUE Scholars Program.
Acknowledgments
We show the upmost gratitude for the members of the Urban Horticulture and Sustainability Laboratory at Texas Tech University for their support and aid in this project. We also show our thanks to the Horticulture Gardens and Greenhouse complex at Texas Tech University for the use of their facilities. Finally, we show great gratitude to the Texas Tech University TrUE Scholars program and the USDA REEU Nutrition BEST program for their support and training for this project.
Conflict of interest
The authors declare that the research was conducted in the absence of any commercial or financial relationships that could be construed as a potential conflict of interest.
Publisher’s note
All claims expressed in this article are solely those of the authors and do not necessarily represent those of their affiliated organizations, or those of the publisher, the editors and the reviewers. Any product that may be evaluated in this article, or claim that may be made by its manufacturer, is not guaranteed or endorsed by the publisher.
Supplementary material
The Supplementary Material for this article can be found online at: https://www.frontiersin.org/articles/10.3389/fpls.2024.1438038/full#supplementary-material
References
Backer, R., Rokem, J. S., Ilangumaran, G., Lamont, J., Praslickova, D., Ricci, E., et al. (2018). Plant growth-promoting rhizobacteria: context, mechanisms of action, and roadmap to commercialization of biostimulants for sustainable agriculture. Front. Plant Sci. 9, 1473. doi: 10.3389/fpls.2018.01473
Bader, A. N., Salerno, G. L., Covacevich, F., Consolo, V. F. (2020). Native Trichoderma harzianum strains from Argentina produce indole-3 acetic acid and phosphorus solubilization, promote growth and control wilt disease on tomato (Solanum lycopersicum L.). J. King Saud Univ. 32, 867–873. doi: 10.1016/j.jksus.2019.04.002
Bai, Z., Zhou, C., Cao, J., Xu, S., Wu, S., Li, D. (2014). Effects of Bacillus amyloliquefaciens Biofertilizer on Tea Yield and Quality. Agric. Sci. Technol. 15, 1883–1887. Available at: https://www.researchgate.net/publication/276273389.
Chaudhary, P., Singh, S., Chaudhary, A., Sharma, A., Kumar, G. (2022). Overview of biofertilizers in crop production and stress management for sustainable agriculture. Front. Plant Sci. 13. doi: 10.3389/fpls.2022.930340
Chewapanich, W., Charoenrak, P., Intanoo, W., Chamswarng, C. (2021). Efficacy of Trichoderma asperellum CB-Pin-01 and potassium dihydrogen phosphate to enhance growth and yield and reduce Pythium root rot of hydroponically grown lettuce. Agric. Natural Resour. 55, 601–610–601–610. doi: 10.34044/j.anres.2021.55.4.10
Dabire, T., Bonzi, S., Somda, I., Legreve, A. (2016). Evaluation of the Potential of Trichoderma harzianum as a Plant Growth Promoter and Biocontrol Agent Against Fusarium Damping-off in Onion in Burkina Faso. Asian J. Plant Pathol. 10, 49–60. Available at: https://scialert.net/abstract/?doi=ajppaj.2016.49.60.
Daniel, A. I., Fadaka, A. O., Gokul, A., Bakare, O. O., Aina, O., Fisher, S., et al. (2022). Biofertilizer: the future of food security and food safety. Microorganisms 10, 1220. doi: 10.3390/microorganisms10061220
Dasgan, H. Y., Aldiyab, A., Elgudayem, F., Ikiz, B., Gruda, N. S. (2022). Effect of biofertilizers on leaf yield, nitrate amount, mineral content and antioxidants of basil (Ocimum basilicum L.) in a floating culture. Sci. Rep. 12, 20917. doi: 10.1038/s41598-022-24799-x
Dasgan, H. Y., Kacmaz, S., Arpaci, B. B., Ikiz, B., Gruda, N. S. (2023). Biofertilizers improve the leaf quality of hydroponically grown baby spinach (Spinacia oleracea L.). Agronomy 13, 575. doi: 10.3390/agronomy13020575
de Sousa, S. M., de Oliveira, C. A., Andrade, D. L., de Carvalho, C. G., Ribeiro, V. P., Pastina, M. M., et al. (2021). Tropical bacillus strains inoculation enhances maize root surface area, dry weight, nutrient uptake and grain yield. J. Plant Growth Regul. 40, 867–877. doi: 10.1007/S00344-020-10146-9
Dobermann, A. (2007). “Nutrient use efficiency-measurement and management,” in Fertilizer Best Management Practices: General Principles, Strategy for Their Adoption and Voluntary Initiatives Versus Regulations. eds Krauss, A., Isherwood, K., Heffer, P.. (Paris, France: International Fertilizer Industry Association), 1–28. Available at: https://www.fertilizer.org//images/Library_Downloads/2007_IFA_FBMP%20Workshop_Brussels.pdf.
Gangwar, R. K., Bhushan, G., Singh, J., Upadhyay, S. K., Singh, A. P. (2013). COMBINED EFFECTS OF PLANT GROWTH PROMOTING RHIZOBACTERIA AND FUNGI ON MUNG BEAN (VIGNA RADIATA L.) | INTERNATIONAL JOURNAL OF PHARMACEUTICAL SCIENCES AND RESEARCH. Int. J. Pharm. Sci. Res. 4, 4422–4426. doi: 10.13040/IJPSR.0975-8232.4(11).4422-26
Girvan, M. S., Bullimore, J., Pretty, J. N., Osborn, A. M., Ball, A. S. (2003). Soil type is the primary determinant of the composition of the total and active bacterial communities in arable soils. Appl. Environ. Microbiol. 69, 1800–1809. doi: 10.1128/AEM.69.3.1800-1809.2003/ASSET/B8304C46-8913-4B55-B861-815CD6259234/ASSETS/GRAPHIC/AM0331429007.JPEG
Giurgiu, R. M., Dumitraș, A., Morar, G., Scheewe, P., Schröder, F. G. (2018). A Study on the Biological Control of Fusarium oxysporum Using Trichoderma spp., on Soil and Rockwool Substrates in Controlled Environment. Notulae Botanicae Horti Agrobotanici Cluj-Napoca 46, 260–269. doi: 10.15835/NBHA46110939
Guimarâes, Í.T., Oliveira, F.D.A., Leal, C. C. P., Souza, M.W.D.L., Alves, T. R. C. (2020). Foliar application of biofertilizer in semi-hydroponic lettuce fertigated with saline nutrient solution. Comunicata Scientiae 11, e3115. doi: 10.14295/cs.v11i0.3115
Gül, A., Kidoglu, F., Tüzel, Y., Tüzel, I. H. (2008). Effects of nutrition and Bacillus amyloliquefaciens on tomato (Solanum lycopersicum, L.) growing in perlite. Spanish J. Agric. Res. 6, 422–429. doi: 10.5424/SJAR/2008063-335
Hirst, A. K., Anee, S. A., Housley, M. J., Qin, K., Ferrarezi, R. S. (2024). Selected beneficial microbes alleviate salinity stress in hydroponic lettuce and pak choi. HortTechnology 34, 345–352. doi: 10.21273/HORTTECH05403-24
Ikiz, B., Dasgan, H. Y., Gruda, N. S. (2024). Utilizing the power of plant growth promoting rhizobacteria on reducing mineral fertilizer, improved yield, and nutritional quality of Batavia lettuce in a floating culture. Sci. Rep. 14, 1616. doi: 10.1038/s41598-024-51818-w
Ji, S., Liu, Z., Liu, B., Wang, Y., Wang, J. (2020). The effect of Trichoderma biofertilizer on the quality of flowering Chinese cabbage and the soil environment. Scientia Hortic. 262, 109069. doi: 10.1016/J.SCIENTA.2019.109069
Kang, S.-M., Hamayun, M., Khan, M. A., Iqbal, A., Lee, I.-J. (2019). Bacillus subtilis JW1 enhances plant growth and nutrient uptake of Chinese cabbage through gibberellins secretion Amjad Iqbal. J. Appl. Bot. Food Qual. 92, 172–178. doi: 10.5073/JABFQ.2019.092.023
Karapetyan, A. (2023). Application of biofertilizers in hydroponics: a review. J. Plant Nutr. 47, 822–836. doi: 10.1080/01904167.2023.2280159
Khanam, M., Solaiman, A. R. M., Rahman, G. K. M. M., Haque, M. M., Alam, M. S. (2022). Effect of plant growth promoting bacteria on growth and nutrient content of rice. Asian J. Adv. Agric. Res. 19, 20–29. doi: 10.9734/AJAAR/2022/V19I230242
Kıdoğlu, F., Gül, A., Tüzel, Y., Özaktan, H. (2009). Yield enhancement of hydroponically grown tomatoes by rhizobacteria. Acta Hortic. 807, 475–480. doi: 10.17660/ActaHortic.2009.807.68
Ku, Y., Xu, G., Tian, X., Xie, H., Yang, X., Cao, C. (2018). Root colonization and growth promotion of soybean, wheat and Chinese cabbage by Bacillus cereus YL6. PloS One 13, e0200181. doi: 10.1371/journal.pone.0200181
Kuan, K. B., Othman, R., Rahim, K. A., Shamsuddin, Z. H. (2016). Plant growth-promoting rhizobacteria inoculation to enhance vegetative growth, nitrogen fixation and nitrogen remobilisation of maize under greenhouse conditions. PloS One 11, e0152478. doi: 10.1371/JOURNAL.PONE.0152478
Kubheka, B. P., Ziena, L. W. (2022). Trichoderma: a biofertilizer and a bio-fungicide for sustainable crop production. IntechOpen. doi: 10.5772/intechopen.102405
Kumar, S., Sindhu, S. S., Kumar, R. (2022). Biofertilizers: An ecofriendly technology for nutrient recycling and environmental sustainability. Curr. Res. Microbial Sci. 3, 100094. doi: 10.1016/J.CRMICR.2021.100094
Lee, S., Lee, J. (2015). Beneficial bacteria and fungi in hydroponic systems: Types and characteristics of hydroponic food production methods. Scientia Hortic. 195, 206–215. doi: 10.1016/J.SCIENTA.2015.09.011
Li, R. X., Cai, F., Pang, G., Shen, Q.-R., Li, R., Chen, W. (2015). Solubilisation of phosphate and micronutrients by trichoderma harzianum and its relationship with the promotion of tomato plant growth. PLoS ONE 10, e0130081. doi: 10.1371/journal.pone.0130081
Lin, Y. P., Lin, C.-M., Mukhtar, H., Lo, H.-F., Ko, M.-C., Wang, S.-J. (2021). Temporal variability in the rhizosphere bacterial and fungal community structure in the melon crop grown in a closed hydroponic system. Agronomy 11, 719. doi: 10.3390/agronomy11040719
Lin, Y., Watts, D. B., Kloepper, J. W., Torbert, H. A. (2018). Influence of plant growth-promoting rhizobacteria on corn growth under different fertility sources. Commun. Soil Sci. Plant Anal. 49, 1239–1255. doi: 10.1080/00103624.2018.1457155
Luo, L., Zhao, C., Wang, E., Raza, A., Yin, C. (2022). Bacillus amyloliquefaciens as an excellent agent for biofertilizer and biocontrol in agriculture: An overview for its mechanisms. Microbiological Res. 259, 127016. doi: 10.1016/j.micres.2022.127016
Malusà, E., Pinzari, F., Canfora, L. (2016). “Efficacy of biofertilizers: Challenges to improve crop production,” in Microbial Inoculants in Sustainable Agricultural Productivity. (New Delhi: Springer India), vol. 2, 17–40. doi: 10.1007/978-81-322-2644-4_2/FIGURES/2
McClintic, N., Chen, Z., Park, Y. (2024). Evaluating organic fertilizers and microbial inoculation for soilless and hydroponic crop production. HortScience 59, 552–560. doi: 10.21273/HORTSCI17727-24
Mohammadi, K., Sohrabi, Y. (2012). Bacterial biofertilizers for sustainable crop production: A review. J. Agric. Biol. Sci. 7, 6145. Available at: www.arpnjournals.com.
Mokabel, S., Olama, Z., Ali, S., El-Dakak, R. (2022). The role of plant growth promoting rhizosphere microbiome as alternative biofertilizer in boosting solanum melongena L. Adaptation to salinity stress. Plants 11, 659. doi: 10.3390/plants11050659
Moncada, A., Miceli, A., Vetrano, F. (2021). Use of plant growth-promoting rhizobacteria (PGPR) and organic fertilization for soilless cultivation of basil. Scientia Hortic. 275, 109733. doi: 10.1016/J.SCIENTA.2020.109733
Moreira, V. D. A., Oliveira, C. E. D. S., Jalal, A., Gato, I. M. B., Oliveira, T. J. S. S., Boleta, G. H. M., et al. (2022). Inoculation with Trichoderma harzianum and Azospirillum brasilense increases nutrition and yield of hydroponic lettuce. Arch. Microbiol. 204, 440. doi: 10.1007/S00203-022-03047-W
Mourouzidou, S., Ntinas, G. K., Tsaballa, A., Monokrousos, N. (2023). Introducing the power of plant growth promoting microorganisms in soilless systems: A promising alternative for sustainable agriculture. Sustainability 15, 5959. doi: 10.3390/su15075959
Oliveira, C.E.D.S., Jalal, A., Aguilar, J. V., de Camargos, L. S., Zoz, T., Ghaley, B. B., et al. (2023). Yield, nutrition, and leaf gas exchange of lettuce plants in a hydroponic system in response to Bacillus subtilis inoculation. Front. Plant Sci. 14. doi: 10.3389/FPLS.2023.1248044/FULL
Pirttilä, A. M., Mohammad Parast Tabas, H., Baruah, N., Koskimäki, J. J. (2021). Biofertilizers and biocontrol agents for agriculture: how to identify and develop new potent microbial strains and traits. Microorganisms 9, 817. doi: 10.3390/microorganisms9040817
Poveda, J., Eugui, D. (2022). Combined use of Trichoderma and beneficial bacteria (mainly Bacillus and Pseudomonas): Development of microbial synergistic bio-inoculants in sustainable agriculture. Biol. Control 176, 105100. doi: 10.1016/j.biocontrol.2022.105100
Priadi, D., Nuro, F. (2017). Seedling production of pak choy (Brassica rapa L.) using organic and inorganic nutrients. Biosaintifika J. Biol. Biol. Education. 9, 217–224. doi: 10.15294/biosaintifika.v9i2.85
Promwee, A., Intana, W. (2022). Trichoderma asperellum (NST-009): A potential native antagonistic fungus to control Cercospora leaf spot and promote the growth of 'Green Oak' lettuce (Lactuca sativa L.) cultivated in the commercial NFT hydroponic system. Plant Prot. Sci. 58, 139–149. doi: 10.17221/69/2021-PPS
Radhakrishnan, R., Hashem, A., Abd Allah, E. F. (2017). Bacillus: A biological tool for crop improvement through bio-molecular changes in adverse environments. Front. Physiol. 8. doi: 10.3389/FPHYS.2017.00667/ABSTRACT
Rajaseger, G., Chan, K. L., Tan, K. Y., Ramasamy, S., Khin, M. C., Amaladoss, A., et al. (2023). Hydroponics: current trends in sustainable crop production. Bioinformation 19, 925. doi: 10.6026/97320630019925
Razmjooei, Z., Etemadi, M., Eshghi, S., Ramezanian, A., Mirazimi Abarghuei, F., Alizargar, J. (2022). Potential role of foliar application of azotobacter on growth, nutritional value and quality of lettuce under different nitrogen levels. Plants 11, 406. doi: 10.3390/plants11030406
Reed, L., Glick, B. R. (2023). The recent use of plant-growth-promoting bacteria to promote the growth of agricultural food crops. Agriculture 13, 1089. doi: 10.3390/agriculture13051089
Samaras, A., Roumeliotis, E., Ntasiou, P., Karaoglanidis, G. (2021). Bacillus subtilis MBI600 promotes growth of tomato plants and induces systemic resistance contributing to the control of soilborne pathogens. Plants 10, 1113. doi: 10.3390/plants10061113
Sambo, P., Nicoletto, C., Giro, A., Pii, Y., Valentinuzzi, F., Mimmo, T., et al. (2019). Hydroponic solutions for soilless production systems: issues and opportunities in a smart agriculture perspective. Front. Plant Sci. 10. doi: 10.3389/fpls.2019.00923
Sarbani, N. M. M., Yahaya, N. (2022). Advanced development of bio-fertilizer formulations using microorganisms as inoculant for sustainable agriculture and environment: A review. Malaysian J. Sci. Health Technol. 8, 92–101. doi: 10.33102/mjosht.v8i1.228
Schuster, A., Schmoll, M. (2010). Biology and biotechnology of trichoderma. Appl. Microbiol. Biotechnol. 87, 787–799. doi: 10.1007/S00253-010-2632-1
Setiawati, M. R., Afrilandha, N., Hindersah, R., Suryatmana, P., Fitriatin, B. N., Kamaluddin, N. N. (2023). The effect of beneficial microorganism as biofertilizer application in hydroponic-grown tomato. Sains Tanah 20, 66–77. doi: 10.20961/STJSSA.V20I1.63877
Sharma, S. K., Ramesh, A., Johri, B. N. (2013). Isolation and Characterization of Plant Growth-Promoting Bacillus amyloliquefaciens Strain sks_bnj_1 and its Influence on Rhizosphere Soil Properties and Nutrition of Soybean (Glycine max L. Merrill). J. Virol. Microbiol. 2013, 446006. doi: 10.5171/2013.446006
Silva, L.R.D., Valadares-Inglis, M. C., Peixoto, G. H. S., Luccas, B. E. G., de, Muniz, P. H. P. C., Magalhães, D. M., et al. (2021). Volatile organic compounds emitted by Trichoderma azevedoi promote the growth of lettuce plants and delay the symptoms of white mold. Biol. Control 152, 104447. doi: 10.1016/J.BIOCONTROL.2020.104447
Singh, K., Guleria, V., Kaushal, S., Shubham (2023). Utilization of biofertilizers and plant growth promoters in hydroponic production system. Curr. J. Appl. Sci. Technol. 42, 13–23. doi: 10.9734/CJAST/2023/V42I374243
Torres, E. C., Somera, C. G. G. (2023). How Organic Fertilizers can be used as a Plant Nutrient Source in Hydroponics: A Review. Appl. Sci. Eng. Prog. 16, 6359. doi: 10.14416/j.asep.2022.11.002
Tyśkiewicz, R., Nowak, A., Ozimek, E., Jaroszuk-Ściseł, J. (2022). Trichoderma: the current status of its application in agriculture for the biocontrol of fungal phytopathogens and stimulation of plant growth. Int J Mol Sci. (2022) 23, 2329. doi: 10.3390/ijms23042329
Uchida, R. (2000). “Essential Nutrients for Plant Growth: Nutrient Functions and Deficiency Symptoms.” in Plant Nutrient Management in Hawaii’s Soils, Approaches for Tropical and Subtropical Agriculture, eds. Silva, J. A., Uchida, R.. (College of Tropical Agriculture and Human Resources, University of Hawaii at Manoa, Honolulu), 31–55.
Vela Coyotl, M. A. D. l., López Tecpoyotl, Z. G., Sandoval Castro, E., Tornero Campante, M. A., Cobos Peralta, M. A. (2018). The organo-mineral fertilization on the yield of faba bean in soil and hydroponics in protected agriculture. Rev. Mexicana Cienc. Agrícolas 9, 1603–1614. doi: 10.29312/remexca.v9i8.1717
Vieira, S., Sikorski, J., Gebala, A., Boeddinghaus, R. S., Marhan, S., Rennert, T., et al. (2020). Bacterial colonization of minerals in grassland soils is selective and highly dynamic. Environ. Microbiol. 22, 917–933. doi: 10.1111/1462-2920.14751
Walters, K. J., Behe, B. K., Currey, C. J., Lopez, R. G. (2020). Historical, current, and future perspectives for controlled environment hydroponic food crop production in the United States. HortScience 55, 758–767. doi: 10.21273/HORTSCI14901-20
Wang, Q., Ou, E. L., Wang, P. C., Chen, Y., Wang, Z. Y., Wang, Z. W., et al. (2022). Bacillus amyloliquefaciens GB03 augmented tall fescue growth by regulating phytohormone and nutrient homeostasis under nitrogen deficiency. Front. Plant Sci. 13, 979883. doi: 10.3389/FPLS.2022.979883/FULL
Wang, Q.-Y., Zhao, M.-R., Wang, J.-Q., Hu, B.-Y., Chen, Q.-J., Qin, Y., et al. (2023). Effects of microbial inoculants on agronomic characters, physicochemical properties and nutritional qualities of lettuce and celery in hydroponic cultivation. Scientia Hortic. 320, 112202. doi: 10.1016/j.scienta.2023.112202
Yasmin, H., Naeem, S., Bakhtawar, M., Jabeen, Z., Nosheen, A., Naz, R., et al. (2020). Halotolerant rhizobacteria Pseudomonas pseudoalcaligenes and Bacillus subtilis mediate systemic tolerance in hydroponically grown soybean (Glycine max L.) against salinity stress. PloS One 15, e0231348. doi: 10.1371/journal.pone.0231348
Yedidia, I., Srivastva, A. K., Kapulnik, Y., Chet, I. (2001). Effect of Trichoderma harzianum on microelement concentrations and increased growth of cucumber plants. Plant and Soil 235, 235–242. doi: 10.1023/A:1011990013955
Zalila-Kolsi, I., Ben-Mahmoud, A., Al-Barazie, R. (2023). Bacillus amyloliquefaciens: harnessing its potential for industrial, medical, and agricultural applications—A comprehensive review. Microorganisms 11, 2215. doi: 10.3390/microorganisms11092215
Zhang, B., Zhang, H., Lu, D., Cheng, L., Li, J. (2023). Effects of biofertilizers on the growth, leaf physiological indices and chlorophyll fluorescence response of spinach seedlings. PloS One 18, e0294349. doi: 10.1371/journal.pone.0294349
Zhao, G., Zhu, X., Zheng, G., Meng, G., Dong, Z., Hye Baek, J., et al. (2024). Development of biofertilizers for sustainable agriculture over four decades, (1980-2022). Geogr. Sustainability 5, 19–28. doi: 10.1016/j.geosus.2023.09.006
Keywords: biofertilizer, beneficial microorganisms, deep-water hydroponics, nutrient film technique, microorganism-root interactions
Citation: Plocek G, Rueda Kunz D and Simpson C (2024) Impacts of Bacillus amyloliquefaciens and Trichoderma spp. on Pac Choi (Brassica rapa var. chinensis) grown in different hydroponic systems. Front. Plant Sci. 15:1438038. doi: 10.3389/fpls.2024.1438038
Received: 24 May 2024; Accepted: 09 September 2024;
Published: 23 September 2024.
Edited by:
Dasiel Obregon, University of Guelph, CanadaReviewed by:
Arthy Surendran, Scotland’s Rural College, United KingdomHayriye Yildiz Dasgan, Çukurova University, Türkiye
Copyright © 2024 Plocek, Rueda Kunz and Simpson. This is an open-access article distributed under the terms of the Creative Commons Attribution License (CC BY). The use, distribution or reproduction in other forums is permitted, provided the original author(s) and the copyright owner(s) are credited and that the original publication in this journal is cited, in accordance with accepted academic practice. No use, distribution or reproduction is permitted which does not comply with these terms.
*Correspondence: Catherine Simpson, Y2F0aGVyaW5lLnNpbXBzb25AdHR1LmVkdQ==