- 1Laboratory of Entomology and Phytopathology, International Center for Agricultural Research in the Dry Areas (ICARDA), Rabat, Morocco
- 2AgroBioSciences Program, College of Agriculture and Environmental Sciences, Mohammed VI Polytechnic University, Ben Guerir, Morocco
- 3Plant and Microbial Biotechnology Center, Moroccan Foundation for Advanced Science, Innovation and Rescarch (MAScIR), Mohammed VI Polytechnic University, Ben Guerir, Morocco
Cool season legumes (Faba bean, chickpea, lentil, pea, and grass pea) are important protein harvests for food and nutrition security in many countries. They play key roles in sustainable cereal production through their ecological benefits. However, diseases and pests attack continue to have a substantial impact on crop yield and quality. Although growers used different control options to manage these biotic stresses such as pesticide application, cultural practices, and resistant varieties, there is a pressing need for the development of new, more cost-effective and environmentally friendly solution to help farmers in facing the existing environmental issues. Recently, there is a growing interest among researchers in exploiting Volatile Organic Compounds (VOCs) for the elaboration of disease and pest control strategies in food legumes and other crops. These compounds have important functions in ecological relationships occurring between plants and their surrounding environment, as well as plants and others species, such as pests and pathogens. Due to their unique properties, VOCs can be employed in improving management alternatives for food legume diseases and pests. In this assessment, we investigated the role of VOCs in plant-pest and plant-pathogen interactions and their present applications in pest and diseases control strategies. We emphasized the ecological importance of employing plant VOCs in legume farming and crop breeding. Additionally, we highlighted the potential of microbial VOCs in facilitating microbe-microbe, microbe-plant and microbe-plant-pest interactions, along with their role in food legume protection.
1 Introduction
Cool-season food legumes such as faba bean, chickpea, lentil, field pea, and grass pea, play a vital role in food production, animal feed, and revenue generation in many regions (Semba et al., 2021). Globally, they occupy huge cultivated areas and are mainly grown for their edible protein-rich seed, and thus are named grain legumes (Calles, 2016).
In cropping systems that mostly rely on cereals, food legume crops are essential for enhancing soil fertility by fixing nitrogen. In Morocco, farmers practice wheat rotation with faba bean and benefit 48% over wheat monocropping (Yigezu et al., 2019).
However, in many regions of the world, their production exhibits significant yield fluctuations over the years due to abiotic and biotic factors. Pathogen invasions and insect pest infestations have damaging impact on both crop production and ecosystem worldwide. The surveillance and management of these biotic stresses are critical to increase crop yields and maintain food security for the expanding global population.
Farmers employ diverse strategies to cope with diseases and pests in food legume crops, involving agricultural approaches such as diverse crop rotation and adapting sowing schedules. Additionally, the planting of resistant varieties is a common approach (Sharma et al., 2016); however, its effectiveness is sometimes compromised by the emergence of new pathogen races and insect biotypes that can overcome the resistance in cultivated varieties.
Moreover, the application of fungicides and insecticides allow to reduce the pathogen and pest infestation, but their excessive use can induce resistance in target organisms, disrupt the soil’s microbial community, result in environment pollution with harmful chemicals (Li et al., 2012). Consequently, there is a need to develop new eco-friendly options to enhance pest management effectiveness (Haware and Nene, 1982; Brilli et al., 2019).
Over the recent decades, Volatile Organic Compounds produced by living beings have received growing attention in agricultural, environmental and ecological researches due to their various properties, and their potential applications in the biocontrol of plant pests and pathogens, as well as plant growth promotion (Choudhary et al., 2008; Pickett and Khan, 2016; Gualtieri et al., 2022; Devrnja et al., 2022; Russo et al., 2022; Patel et al., 2023).
Plants and microorganisms produce a wide range of Volatile Organic Compounds appertain to various chemical families with distinct biochemical origins like alcohols, aldehydes, aromatic compounds, esters, furans, monoterpenes, sesquiterpenes, hydrocarbons, and ketones (Kramer and Abraham, 2012). These secondary metabolic products typically possess low molecular weight (averaging below 300 Da), reduced boiling temperatures, and elevated vapor pressure (evaporating at 0.01 kPa around 20°C) (Morath et al., 2012). Consequently, they can diffuse into the air, ground, and fluids, exerting their effects over both short and long distances to ensure interactions between the organism and its environment, including connections among plants themselves (Delory et al., 2016; Sakurai et al., 2023), as well as exchanges between plant and pests or pathogens (Baldwin, 2010; Piechulla et al., 2017; Pérez-Hedo et al., 2024).
Due to their significant attributes and role as signaling compounds, VOCs provide several ecological and agricultural roles, and contribute to plant defense against pests and diseases (Piesik, 2011; Bezerra et al., 2021; Annaz et al., 2023).
This revise outlines the various applications of VOCs as natural and eco- friendly solution in food legume protection, highlighting their specific properties that make them effective as traps for managing different insect pest species. It indicates also the role of plant VOCs in inhibiting disease development, controlling insect pests and attracting natural enemies with a specific focus on their use in cropping systems. Furthermore, it demonstrates the exploitation of VOCs potential in plant breeding as non-invasive and rapid phenotypic tool for pest and disease resistance. Additionally, it details the different interactions mediated by microbial VOCs and their function in disease and pest management.
This review utilized a bibliometric analysis to gather data from Scopus, Google Scholar, and Web of Science databases. The search was guided using the keywords “Volatile Organic Compounds AND food legume AND insect and disease management” resulting in a total of 224 documents. These documents comprised articles (80.4%), reviews (16.1%), book chapters (2.2%), conference papers (0.9%), and short surveys (0.4%) (Figure 1C).
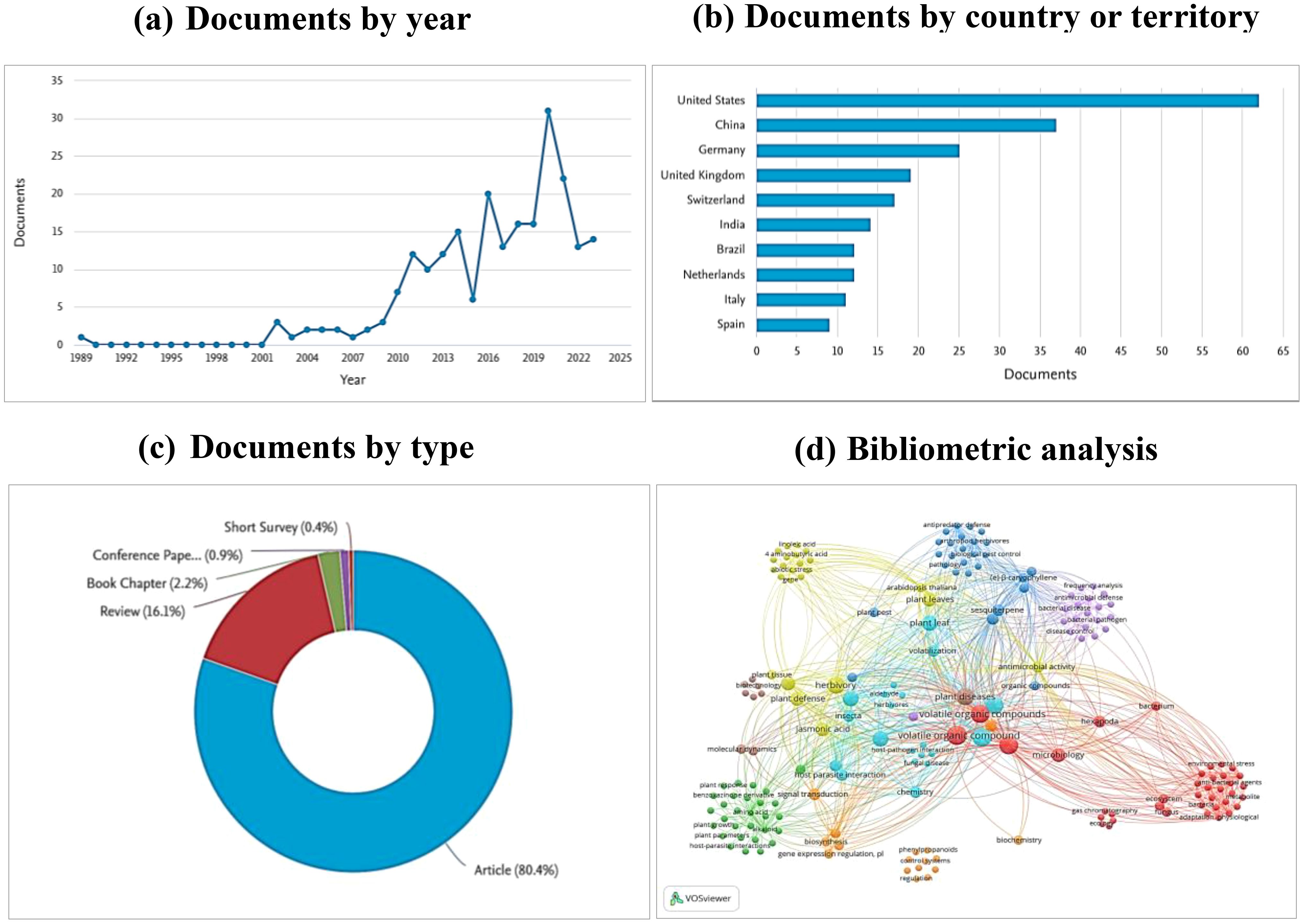
Figure 1. (A) the number of publications per year, (B) leading countries in publications on the role of VOCs in legume disease and pest management, (C) different type of documents in this search, (D) bibliometric network of keywords in publications on VOCs in disease and pest management of legume. From: VOSviewer.
Several bibliometric indices, including frequently used keywords were used to perform the Network analysis. This network was conducted using the VOS viewer processing software, revealing relationships among the identified keywords and offering a comprehensive overview of current use of Volatile Organic Compounds in legume crop protection (Figure 1D).
Furthermore, these findings facilitated an assessment of the significance of the published research. The classification of leading countries in article publications on the subject revealed that United States had the highest number of documents (62), followed by China with 37 documents and Germany with 25 documents (Figure 1B).
The publication trend indicated a substantial increase in the number of articles from 2015 to 2020, a slight decrease between 2020 and 2021, and a subsequent rise between 2021and 2023, indicating a growing interest in the topic (Figure 1A).
2 Current progress in using plant VOCs for pest and disease management in food legume crops
Plants release a diverse set of volatile organic compounds (VOC), either naturally or as reaction to both biotic and abiotic stressors, to cope with pest attack or pathogen invasion (Maffei et al., 2007; Dicke and Loreto, 2010; Mutyambai et al., 2016; Castro et al., 2017; Karolkowski et al., 2021).
These chemical signals serve multiple purposes for plants, including defense against pests and diseases, attraction of pollinators and other beneficial organisms, and communication with neighbouring plants (Figure 2) (Annaz et al., 2023; Ficke et al., 2021). An increasing attention is emerged for reducing reliance on chemical pesticides by adopting new sustainable, natural, and eco-friendly solution for effective pest and disease control like exploiting the ecological and agronomic potential of VOCs to protect plants (Brilli et al., 2019).
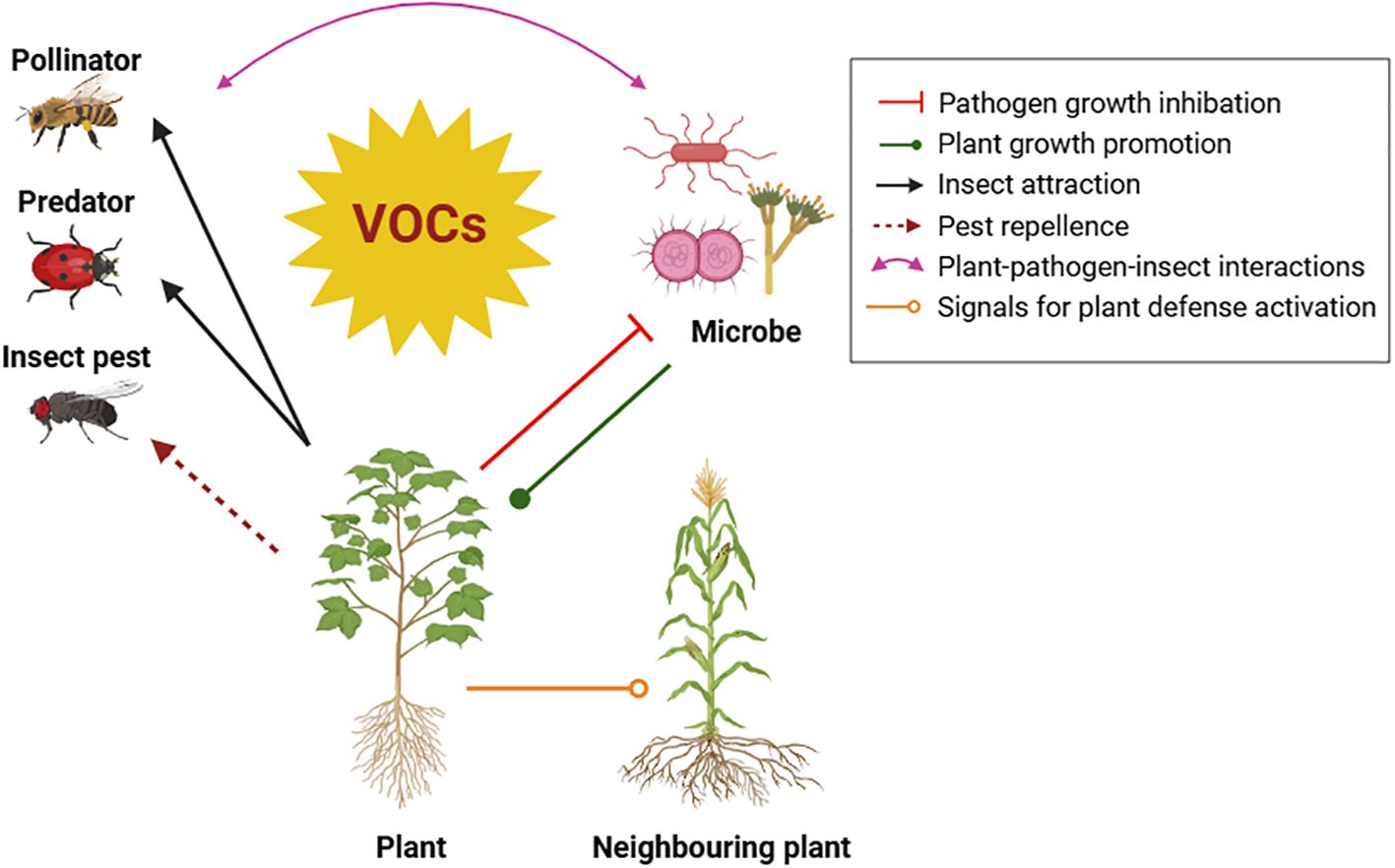
Figure 2. Diverse roles of Volatile Organic Compounds (VOCs) in mediating plant interactions with the environment.
The following briefly outlines the functions of VOCs in pest and disease management and other ecological services.
2.1 VOCs and pest management
Due to their attractive or repellent action, VOCs can influence the feeding behavior of insect pests, their reproduction, their olfactory perception, and the selection of their hosts (Hegde et al., 2011; Santos et al., 2015; Bruno et al., 2018; Singh et al., 2021). Therefore, they can be used as pest traps to control insect population dynamics.
Understanding and deciphering the chemical ecology of insects may offer opportunities for enhancing eco-friendly methods to fight against bio-aggressors. This involves the exploitation of repellent or disruptive volatile organic compounds (VOCs) to annoy harmful insects, as well as the use of attractive compounds for pest trapping (Reisenman et al., 2016; Fountain et al., 2017; Wyatt, 2018) (Table 1).
2.1.1 Legume leaf miner
The leaf miner, Liriomyza spp (Diptera: Agromyzidae), is among the most threatening insect pests to chickpea crop (Cicer arietinum L.) and other legumes in the Mediterranean region (Chrigui et al., 2020; Sabraoui et al., 2019). Adult leaf miners do not possess pheromone specific to their species; they utilize herbivore-induced plant volatiles HIPVs triggered by female punctures, which include green leaf volatile (GLVs), terpenoids and oximes, as cues for the host plant location. Also, natural enemies can exploit these HIPVs to locate their hosts.
For example, in pea plant, HIPVs such as (Z)-3-hexenyl-acetate and (Z)-3-hexenol promote the attraction of male and female flies of the pea leafminer Liriomyza huidobrensis (Figure 3A) and mating occurs on host leaves (Ge et al., 2019).
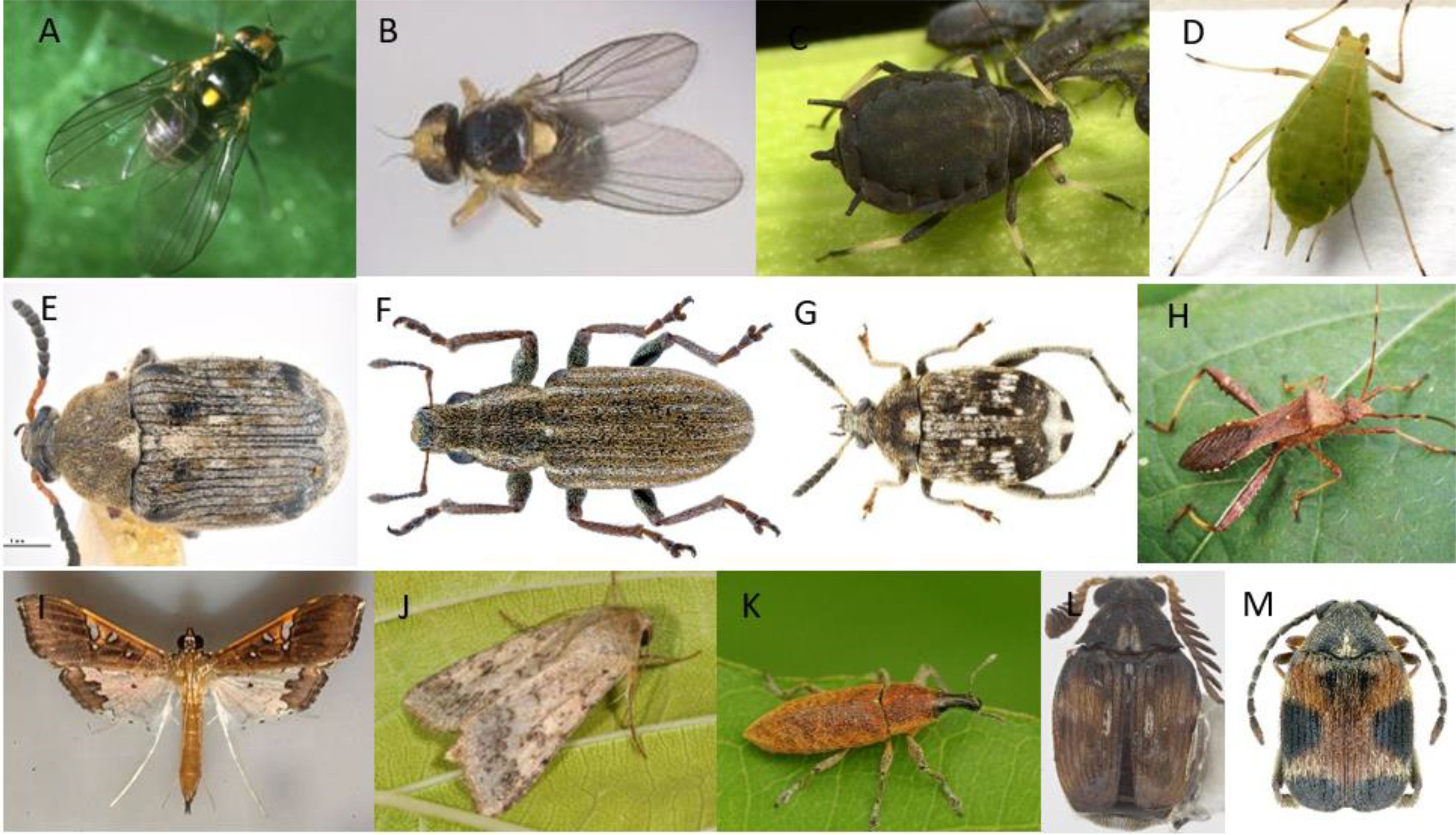
Figure 3. (A) Liriomyza huidobrensis; (B) Liriomyza sativae; (C) Aphis fabae; (D) Acyrthosiphon pisum; (E) Bruchus rufimanus; (F) Sitona lineatus L.; (G) Bruchus pisorum L.; (H) Riptortus pedestris; (I) Maruca vitrata (J) Helicoverpa armigera; (K) Lixus algirus; (L) Callosobruchus chinensis; (M) Callosobruchus maculatus.
Moreover, bean plant odors such as cis-3-hexen-1-ol and 4-hydroxy-4- methyl-2-pentanone, produced by healthy and damaged bean plants, are reported in attracting leafminer (L. sativae) (Figure 3B) and its parasitoids Diglyphus isaea (Zhao and Kang, 2002). So these herbivores induced volatiles (HIVs) can be utilized to trap the pest or to attract its natural enemy.
2.1.2 Aphids and virus on legumes
Legumes such as faba beans, chickpeas, lentils and peas are susceptible to many viruses of which 42 are identified as transmitted by vectors, mainly by insects (Makkouk, 2020; Jones and Roger, 2021; Adane and Vetten, 2022; Tatineni and Hein, 2023). The major vectors for legume viruses are aphids, leafhoppers, thrips, and beetles (Guerrieri and Digilio, 2008; Congdon et al., 2017).
For example, the black bean aphid, Aphis fabae (Hemiptera: Aphididae) (Figure 3C) poses a significant threat to faba beans (Vicia faba), inflicting harm by feeding and also by transmitting plant viruses.
The aphids is attracted to its host through a mixture of volatile compounds released by V.faba plant, which consists of (Z)-3-hexen-1-yl acetate, 1-hexanol, (Z)-3-hexen-1-ol, benzaldehyde, (E)-2-hexenal, 6-methyl-5-hepten-2-one, octanal, (R)-(-)- linalool, methyl salicylate, decanal, undecanal, (E)-β-farnesene, (E)-β-caryophyllene, (S)-(-)-germacrene D and (E,E)-4,8,12-trimethyl1,3,7,11-tridecatetraene (TMTT).
Among this blend, the molecules octanal, (R)-(-)-linalool and (S)-(-)-germacrene D can reduce the duration that aphid remains on its host. However, the presence of these compounds in VOCs blend has no effect on the aphids’ behavior which underlining the importance of the blend effect on aphid behavior over individual compounds’ effect (Webster et al., 2008).
The pea aphid, Acyrthosiphon pisum (Hemiptera: Aphididae) (Harris), (Figure 3D) can consume its host plant, Vicia faba, without causing alterations in plant VOCs release. The volatile organic compound amount emitted by affected bored beans stayed consistent or reduced compared to undamaged plants. For example, aphid feeding decreased the emission of sesquiterpene (E)-ß-ocimene and GLV (Z)-3-hexenyl acetate in comparison with intact plants. Contrarily, the GLV (E)-2-hexenal emission is not impacted by aphid attack.
Also, Pea aphid can prevent the emission of some VOCs during feeding, such as ß-caryophyllene, (E)-ß-ocimene and (E, E)-4,8,12-trimethyl-1,3,7,11-tridecatetraene (TMTT), which can attract its parasitoid, Aphidius ervi. So the suppression of some VOCs production by aphid feeding make the plant incapable to attract natural enemies and protect itself (Schwartzberg et al., 2011).
2.1.3 Legume beetle and weevil
The bean seed beetle, Bruchus rufimanus (Coleoptera: Chrysomelidae) (Figure 3E) presents a significant economic threat to beans, mainly field beans (Vicia faba) sown during the spring and winter seasons (Carrillo-Perdomo et al., 2018).
Bruce et al. (2011) showcased the significance of plant host-emitted volatile organic compounds in monitoring B. rufimanus. Nine active compounds were identified from V. faba cv. ‘Sutton dwarf’ flowers, including cinnamyl alcohol, cinnamaldehyde, myrcene, (R)-limonene, (E)-ocimene, (R)-linalool, 4-allylanisole, (E)-caryophyllene, and α-humulene.
The semiochemicals derived from host plant flowers and male bruchids induced electrophysiological and behavioral reactions in female B. rufimanus, which may be utilized to attract and trap the pests in the field (Dell'Aglio and Tayeh, 2023).
Although host plant odors are attractive as a blend, their individual exposure to insects may be less effective and even repellent. The proportion of each compound in the VOCs blend that the host plant naturally released is critical for communicating with insects. Therefore, to create an effective attractant, it’s crucial to mimic these natural ratios accurately.
In olfactometer bioassays, the chosen headspace collections and synthesized molecules were evaluated, and then incorporated into semiochemical lures for traps. During field trials, cone baits loaded with a combination of three floral volatile compounds, including (R)-linalool, cinnamyl alcohol, and cinnamaldehyde, trapped significantly more male and female of B. rufimanus compared to unbaited control traps.
Sitona weevil (PLW), Sitona lineatus L., (Coleoptera: Curculionidae) (Figure 3F) is a pest of pea (Pisum sativum L.), faba bean (Vicia faba L.) and other legume crops. Larvae that feed on root nodules reduce nitrogen fixation and cause the most damage. This pest could be managed by using an aggregation pheromone 4-methyl-3,5-heptanedione, in combination with host plant volatiles such as linalool, (Z)-3-hexenol, and (Z)-3-hexenyl acetate. The addition of plant volatiles to the aggregation pheromone can increase weevil attraction, however plant volatiles solely didn’t attract S. lineatus adults (Onge et al., 2018).
The pea weevil (Bruchus pisorum L.) (Coleoptera: Bruchidae) (Figure 3G) stands among the most damaging pests of peas (Pisum sativum L.) (Mendesil et al., 2016; Reddy et al., 2018; Aznar-Fernández and Rubiales, 2019). Ceballos et al. (2015) explored how the volatiles emitted by pea plants can affect the electrophysiological and behavioral reactions of B. pisorum, employing electroantennography (EAG) and olfactometry tests.
Volatiles produced at various developmental phases were extracted in headspace by Porapak Q tubes and identified using gas chromatography coupled to mass spectrometry (GC-MS) (Makhlouf et al., 2024). The Analysis using GC-MS showed variations, both qualitative and quantitative, in plant-emitted volatiles across different phenological stages. Terpenes were the most frequent compounds in every stage, with terpinene and 1-S-verbenone exclusively detected during the flower stage. Flowers released large amounts of all compounds, excluding myrcene and n-dodecane. Pea pods released low amount of (Z)-2-hexen-1-ol, 2,4-hexadienal, α-pinene, β-pinene, myrcene, and limonene, except for n-dodecane that was prevalent at this phase.
The highest concentration of compounds coincided with the vegetative and flower stages, consistent with Dudareva et al.’s (2004) findings, indicating that volatiles emission intensified when leaves are young and flowers are ready for pollination.
In olfactometer bioassays, volatiles produced in all growth phases prompted an attractant behavioral reaction from both male and female B. pisorum. Significantly, the female B. pisorum displayed a grater attraction to pod volatiles than other phenological stages. These volatiles released by the flowers and pods of pea plants act as natural signals directing B. pisorum to find appropriate hosts.
2.1.4 The bean bug and stink bug
The bean bug Riptortus pedestris (Fabricius) (Heteroptera: Alydidae) (Figure 3H), is a generalist pest that mainly attack legumes particularly soybean, can recognize its host through the plant’s volatile organic compounds (VOCs).
Research carried out by Song et al. (2022) revealed that both sexes of R. pedestris are able to perceive volatiles emitted from soybeans such as (Z)-3-hexen-1-ol, (Z)-3-hexenyl acetate, 4-ethylbenzaldehyde, α-farnesene, and methyl salicylate. When assessed in controlled laboratory settings, bean bugs adult showed no specific behavioral responses to single molecule; however, they did demonstrate strong preference towards a specific combination of synthetic volatile compounds of soybean.
In natural conditions, the mixture of soybean volatiles didn’t considerably lure R. pedestris. However, it showed a synergistic interaction with the aggregation pheromone, effectively attracting the bean bugs. These findings emphasize the importance of host plant volatiles in the perceptive behavior of the bean bug, shedding light on the colonization dynamics of R. pedestris in soybean plantations.
2.1.5 Legume pod borer
Zhou et al. (2015) have investigated how the female Maruca vitrata (Lepidoptera: Crambidae) (Figure 3I), a major pest in cowpea cultivation, responds to the volatiles emitted from Vigna unguiculata flowers and their role in the selection of host plants.
By employing gas chromatography coupled to electroantennography (GC-EAD) and gas chromatography-mass spectrometry (GC-MS) analysis, 17 major volatile compounds produced by V. unguiculata have been determine, involving butyl ester, butanoic acid, limonene, butanoic acid octyl ester, 4-ethylpropiophenone, 1H-indol-4-ol, and 2-methyl-3-phenylpropan.
During the field trials, six compounds from these floral volatiles successfully lured female moths and revealed notable distinctions in comparison with the control bait. These findings indicated that cowpea VOCs likely influence the scent-driven behavioral reaction of female moths, which in turn affecting their choice of egg-laying locations. This insight offers valuable understanding for investigating monitoring effectiveness and combined pest control approaches against the legume pod borer in agricultural settings.
The chickpea pod borer, Helicoverpa armigera (Lepidoptera: Noctuidae) (Figure 3J) presents a significant threat to chickpea crops. It can tunnel into the pods during the reproductive stage which resulting in major decreases in productivity (El Fakhouri et al., 2022; Boulamtat et al., 2019). Trichogramma spp., egg parasitoid, is used as biological control agents against Lepidoptera pests. Plant volatiles such as n-octadecanoic acid, n-hexadecanoic acid, and octadecane have been proven to improve the foraging function of Trichogramma spp, mainly octadecane which has been detected in several plant volatile profiles and is known to be attractive to Trichogramma spp. Pawar et al. (2023) demonstrated that applying a kairomone gel formulation of octadecane (Saturated hydrocarbon) after 24h of the Trichogramma chilonis release in chickpeas have improved the biological control capability of T. chilonis towards H. armigera larvae, leading to decreased pod damage and an increased chickpea grain yield.
Faba bean stem borer, Lixus algirus L. (Coleoptera: Curculionoidae) (Figure 3K), is regarded as one of the main insect pests affecting faba beans in the Mediterranean area (Ait Taadaouit et al., 2021a, b). Their extensive damage is primarily attributed to larval feeding within the plant stems. El Fakhouri et al. (2021) conducted experiments using both small wind tunnels and olfactometer bioassays, revealing that the volatiles emitted by healthy host plants during the flowering stage attract both male and female L. algirus significantly. The study also demonstrated that faba bean plants exhibit distinct volatile profiles based on the degree of infection, and the growth stage (VOCs of vegetative stage plants are different from those of the blooming stage). During the flowering stage of the infested plants, sixty-six compounds with notable GLV amounts such as 3-Hexen-1-ol, acetate, (Z)-, 1-Hexanol, 2-ethyl-, 3-Hexen-1-ol, 1 acetate, (E)- were released.
2.1.6 Storage pests
Storage losses caused by insect pests in food legumes contribute significantly to food and nutrition insecurity, leading to reduced incomes for growers. Smallholder farmers, relying on traditional storage methods, often experience substantial losses, surpassing 70%. Reports indicate storage damages reaching up to 50% in certain essential legume crops including chickpea, faba bean, lentil, and pea (Keneni et al., 2011). Smallholder farmers employ various pest management strategies, including insecticide seed treatment, the use of botanicals, the adoption of improved storage structures and bags, and fumigation, which is the most common method for grain protection against insect pests. However, the two primary fumigants methyl bromide and phosphine, have encountered challenges due to environmental concerns such as decreasing ozone levels and biological factors like insect resistance, which highlight the need for urgent development of eco-friendly alternatives (Rajendran and Sriranjini, 2007). Volatile organic compounds emitted from different plants serve as an efficient tool for the control of many storage pests (Singh et al., 2021).
The adzuki bean weevil, Callosobruchus chinensis (L.) (Coleoptera: Chrysomelidae) (Figure 3L) is a highly harmful insect of stored legumes due to its highly destructive nature. In a study conducted by Mario et al. (2023), the application of plant powder fumigants emerged as a sustainable and economical strategy for managing pests of stored product. The effectiveness of powder fumigants of four plants including clove, holy basil, lemongrass, and turmeric was assessed. These plants exhibited substantial efficacy, causing mortality rates of up to 100% in C. chinensis. Additionally, they demonstrated oviposition deterrence, antifeedant activity, suppression of F1 offspring, and repellent efficacy for adults. Consequently, this multifaceted impact resulted in a reduced percentage of bean damage and weight loss.
Examining of the volatile organic compounds found in the four plant powder fumigants revealed key constituents responsible for their anti-pest properties. Clove was characterized by eugenol and caryophyllene, holy basil by estragole, lemongrass by α-Citral and β-Citral, and turmeric by α-zingiberene and β-sesquiphellandrene. Notably, the powder fumigant from clove plant has proved outstanding effectiveness across all observed variables, displaying remarkable bioefficacy despite the minimal quantity applied.
Callosobruchus maculatus (F.) (Coleoptera: Bruchidae) (Figure 3M) is a threatening stored grain pest affecting Lathyrus sativus L. (Leguminosae), widely referred to as khesari, in India, Bangladesh, and Ethiopia. This pest has the ability to locate its host by detecting the volatile organic compounds released from the host seeds (Hamdi et al., 2017).
A total of 23 volatiles were identified and quantified in different types of healthy khesari seeds including Bio L 212 Ratan, Nirmal B-1, WBK-13-1, and WBK-14-7, using gas chromatography coupled to mass spectrometry and gas chromatography-flame ionization detector analyses. Nonanal emerged as the predominant compound in the seeds of the two varieties Bio L 212 Ratan and WBK-13-1, then we found farnesyl acetone. Conversely, Nirmal B-1 and WBK-14-7 khesari seeds were characterized by farnesyl acetone as the predominant volatile, followed by nonanal.
The Y-shaped glass tube olfactometer bioassays were used to study the olfactory reactions of female C. maculatus towards volatile blend released by different varieties of khesari seed, as well as distinct synthetic compounds, and their mixture. The results indicated that this pest preferred the entire volatile mixture emitted by Bio L 212 Ratan seeds compared to those from the other three types. C. maculatus was attracted to five different synthetic compounds including 3-octanone, linalool oxide, 3-octanol, 1-octanol, and nonanal.
Notably, a synthesized mixture consisting of linalool oxide, 3-octanone, 3-octanol, 1-octanol, and nonanal at concentrations of 448, 390, 1182, 659, and 8114 ng/20 μl methylene chloride, respectively, proved to be the greater appealing to C. maculatus. This mix holds promise for potential use in C. maculatus pest control programs, like lured traps (Adhikary et al., 2015).
Furthermore, Ajayi et al. (2015) have investigated the host selectivity of C. maculatus female regarding seeds from three legume cultivars, Ife-brown and black-eyed cowpeas (Vigna unguiculata L.) and soybean (Glycine max L.). Results revealed that C. maculatus selects its host based on the odor and 2-ethyl hexanol has been identified as a possible attractant for this pest, which is more produced in Ife-brown cowpea.
2.2 VOCs and disease management
Plant overcomes pathogen attack by producing a set of volatile organic compounds (VOC) from different organs including seeds, leaves, roots, and nodules (Singh et al., 2022).
These VOCs serve as chemical signals that mediate interactions between plant and pathogen by reducing disease development, as well as, plant and their neighbouring plants by inducing resistance against pathogen attack (Kasote et al., 2023; Kong et al., 2024).
2.2.1 VOCs in plant-pathogen interaction
Fusarium species that are associated with chickpea plants are considered one of the most serious biotic stresses that affect chickpeas in the main growing regions (Lokesh et al., 2020; Sampaio et al., 2020). Chickpea plants can defend themselves against Fusarium attacks (Figure 4) by producing different volatile organic compounds (Table 2). Research conducted by Cruz et al. (2012) showed that chickpea VOCs, such as 1-hexanol, trans-2-hexenal, 1-penten-3-ol, cis-3- hexen-1-ol, and trans-2-hexen-1-ol, have negatively impacted both Fusarium avenaceum and F. graminearum development, however the intensity of their impact differed. For instance, trans-2-hexenal and trans-2-hexen-1-ol were highly effective, fully inhibiting F. avenaceum development even at the minimum dosage (2µl). Every VOCs have totally suppressed the pathogen growth at the highest dosage (50 µL), except for 1-penten-3-ol that decreased development by about 50% in comparison with the control without VOCs.
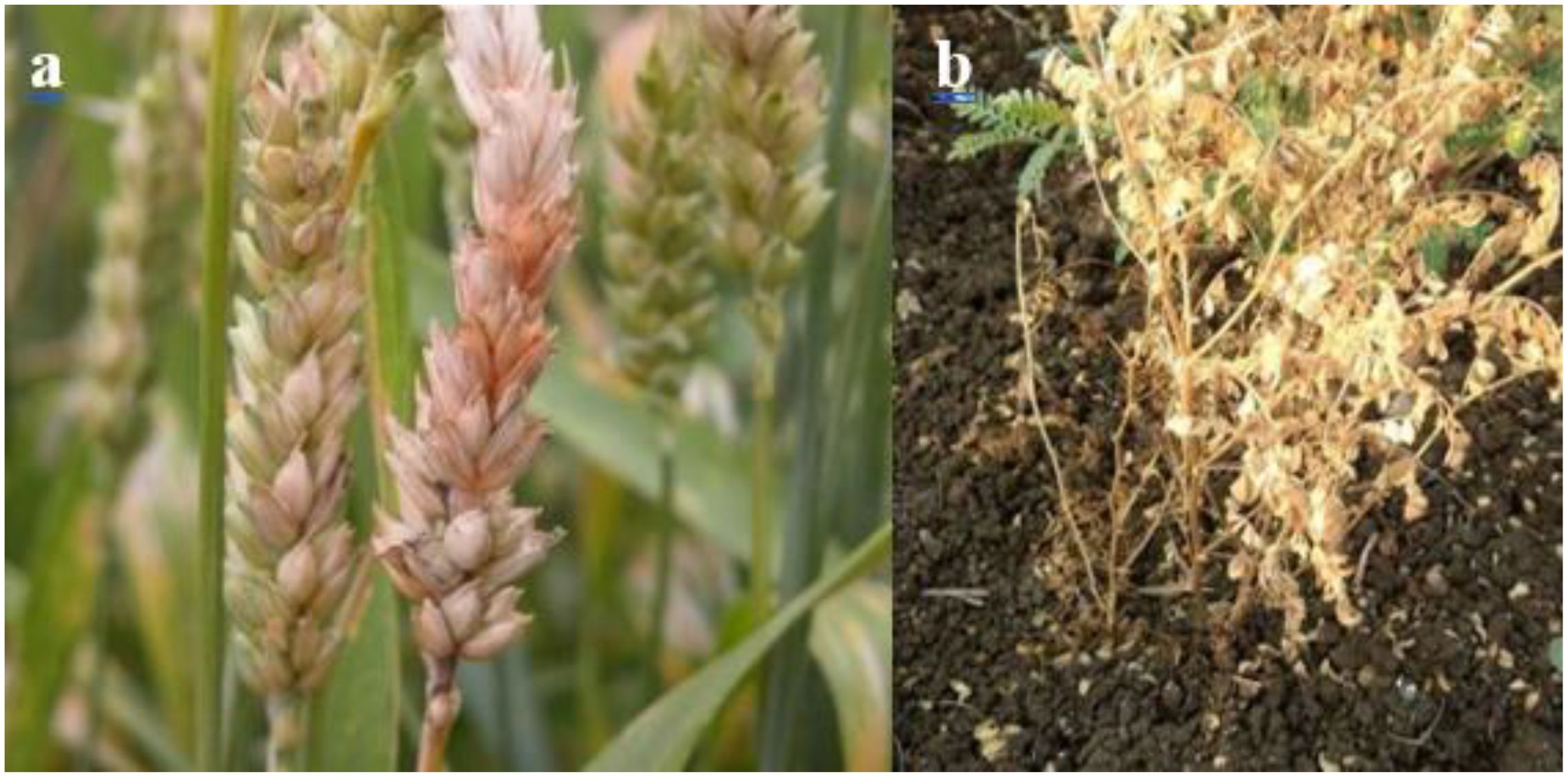
Figure 4. Fusarium symptoms (A) Fusarium head blight (FHB), caused by Fusarium graminearum, (B) Fusarium wilt on chickpea caused by Fusarium oxysporum (f) sp. Ciceris (InsectImages, 2018).
Bioassays performed in Petri plates revealed that volatile organic compounds (VOCs) produced by chickpeas, when tested individually, exhibited greater efficacy toward F. graminearum and F. avenaceum compared to VOCs produced by wheat (Cruz et al., 2012).
However, VOCs released from plants can exhibit harmful effects if they are found in high concentrations. For example, 2-ethyl-1-hexanol produced by chickpea may have an adverse effect on plant growth and also can prevent Fusarium development (Horiuchi et al., 2007; Barney et al., 2009).
Additionally, Ascochyta blight, caused by Ascochyta rabiei (Class: Coelomycetes, order: Sphaeropsidales) (Telomorph: Didymella rabiei; class: Dothideomycetes, order: Pleosporales) (Krimi et al., 2022) (Figure 5), stands as the major devastating foliar disease that affects chickpea plant (Gaur et al., 2012). It represents a serious obstacle to winter chickpea production (Houasli et al., 2021). To protect themselves from A. rabiei invasion, chickpea plants release a set of VOCs that can affect the pathogen’s development. According to Cruz et al. (2012), the release of the two volatile compounds 1-penten-3-ol and cis-3-hexen-1-ol, was triggered by the A. rabiei fungus. Besides, chickpea VOCs production was related to Ascochyta blight severity. However, research carried out by Oliva et al. (1999) has shown that the application of fungicides can reduce the production of volatile organic compounds in chickpea. Furthermore, volatile organic compounds (VOCs) can be employed for the evaluation of Ascochyta blight disease severity in chickpea crops (Kashyap and Kumar, 2021).
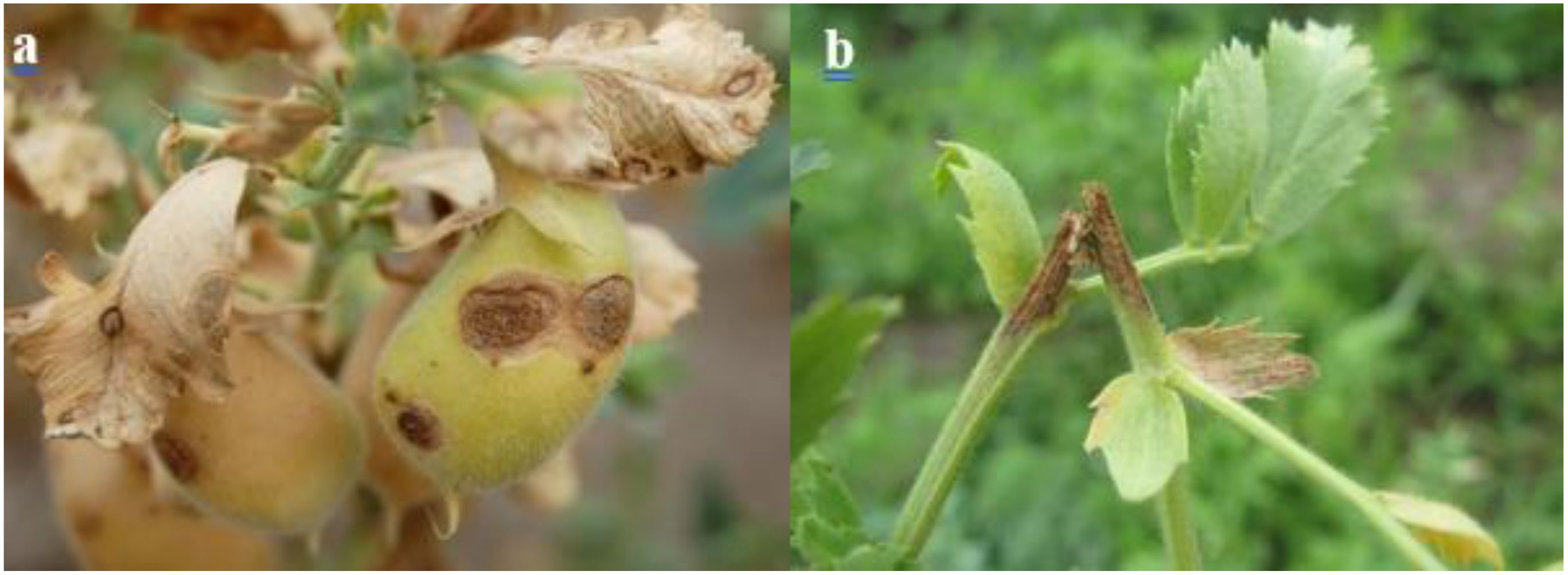
Figure 5. Ascochyta blight symptoms on chickpea plant (A) Brown lesions on leaves and pods, (B) Breakage of the stem (InsectImages, 2018).
2.2.2 VOCs in plant-plant interaction
Plants exhibiting resistance to herbivores and pathogens release volatile organic compounds (VOCs) that can prompt resistance reactions in nearby healthy plants. Latest investigations suggests that these VOCs may also induce resistance to pathogens through various mechanisms: either by priming the stimulated expression of resistance genes in the receiver plant or by inhibiting directly microbial pathogens, leading to a passive resistance in the plant subjected to VOCs (Sakurai et al., 2023). Research of Quintana-Rodriguez et al. (2015) revealed that VOCs released by infected resistant bean plants (Phaseolus vulgaris) offered resistance to bean anthracnose caused by Colletotrichum lindemuthianum in a susceptible cultivar after being exposed over 6 hours to volatile compounds collected from the headspace of resistant plants. Furthermore, individual VOCs like limonene, linalool, nonanal, methyl salicylate, and methyl jasmonate, at normal levels, could directly inhibit conidia development and can also suppress the conidia production using an active mycelium in vitro. VOCs from infected plants displayed a more potent inhibitory effect on conidial germination compared to VOCs from uninfected plants. This inhibitory effect was found to be correlated with the abundance of b-linalool, limonene, or methyl jasmonate. In conclusion, VOCs are pivotal in enhancing bean resistance to fungal pathogens, both by directly reinforcing the emitting plant’s resistance, and indirectly by influencing the resistance traits of nearby receiver plants through induced and associational resistance. Several VOCs like, linalool, limonene, nonanal, methyl salicylate, and methyl jasmonate, exhibit antimicrobial activity (Fernando et al., 2005; Arroyo et al., 2007; Neri et al., 2007). For instance, nonanal has been found to suppress fungal germination in leaves through direct fungistatic actions (Zeringue et al., 1996) and also conferred resistance in lima bean to Pseudomonas syringae (Yi et al., 2009). Notably, the dominant volatile component cis-hexenyl acetate in the volatile blend of the susceptible cultivar, is more probable to be linked to herbivory than pathogen resistance mechanism.
3 VOCs application on food legume crops
3.1 Exploring the role of VOCs in cropping systems
Smallholder farmers adopt diverse cropping systems to promote sustainable crop production (Brilli et al., 2019; Murphy-Bokern, 2022). Legume crops are integrated into crop rotation and multiple cropping strategies to reduce fertilizer consumption, minimize pesticide use, and enhance various ecosystem services (Agegnehu et al., 2008; Gaba et al., 2015; Wahbi et al., 2016; Ajal and Weih, 2022). Some major multiple cropping systems (Figure 6), focusing on volatile organic compounds (VOCs) role in pest management and other ecological services are briefly described (Figure 7).
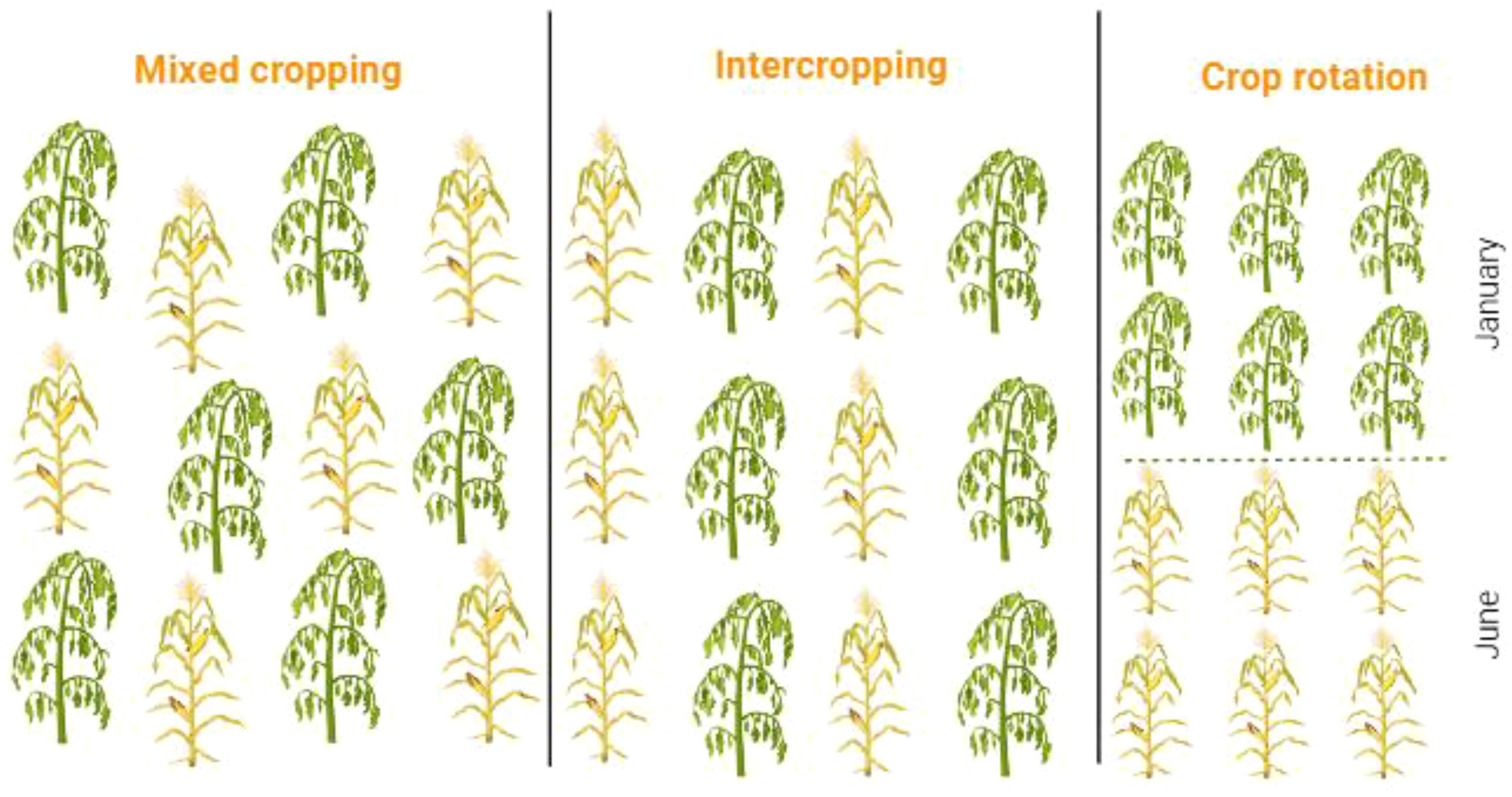
Figure 6. Different cropping systems adapted in legume crops as cultural practices to manage pest attack.
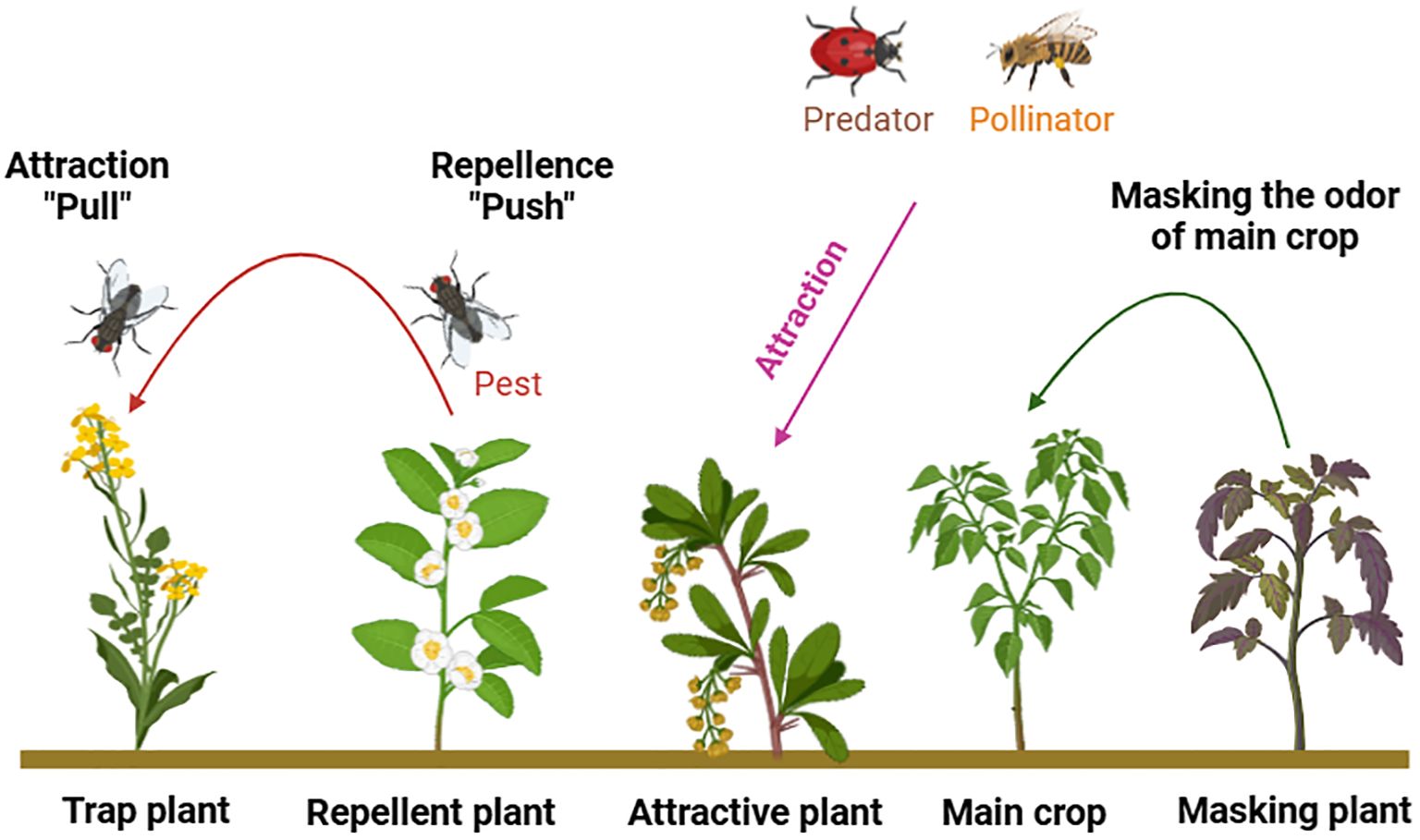
Figure 7. Various roles of service plants using different cropping systems for pest management and for other ecological services.
3.1.1 Crop rotation
Crop rotation is an agricultural strategy that involves the sequential planting of various crops in one parcel for a set period of time to enhance soil fertility and control pests and diseases.
The rotation of wheat with chickpea has proven beneficial in reducing Fusarium head blight, through volatile organic compounds (VOCs) released from legumes. Cruz et al. (2012) showed that VOCs produced from both wheat and rotation crops, particularly chickpea, appear to have a negative impact on pathogenic Fusarium which cause significant financial deficits in crop rotations based on wheat.
The findings further indicate that in chickpea-wheat rotation systems, Fusarium inoculum rates could be minimized by cultivating chickpea genotypes with elevated concentrations of 1-hexanol and trans-2-hexen-1-ol in both roots and shoots (Cruz et al., 2012).
3.1.2 Polyculture
Farmers grow different crops in the same area to exploit resources and avoid risks due to pest outbreaks or weather variability. As opposed to monocropping, polyculture increases crop diversity that influences insect populations and diseases by reducing the population density of herbivores and promoting beneficial insects like pollinators and natural enemies, especially parasitoids. Growing diverse plant species in close proximity can contribute to insect control through various mechanisms, which differ according to the particular volatile proprieties of each plant (Shrivastava et al., 2010).
3.1.2.1 Inter - and mixed cropping
These cropping practices are a type of polyculture in which crop varieties or different plant species are planted, in the same space at same time, as mixed or arranged in rows or strips (Ghaley et al., 2005; Pelzer et al., 2012). Cereal-legume inter/mixed cropping practices contribute positively to insect pests’ control (Mweke et al., 2020; Emery et al., 2021).
In maize-legume intercropping, the overall system dynamics can influence the presence of legume pests (Bukovinszky et al., 2004). For instance, intercropping bean plants with older and taller maize plants can significantly reduced black aphids (Aphis fabae) infestations (Ogenga-Latigo et al., 1992). Similarly, cowpea crops experienced a lower population of pod-sucking bugs when grown alongside with maize at particular percentages, in contrast to being cultivated alone (Olufemi et al., 2001). The intercropping of cereals and legumes has the potential to impact the insect diversity and population by changing foliage nitrogen concentration and modifying the plant taste for herbivores (Pierre et al., 2023).
3.1.2.2 Companion crops
The cultivation of both non-commercial and main crops is a common practice that supports nutrient uptake, pollination, and various other benefits. Push-pull is the most popularized innovation in managing insect pests through companion cropping (Murage et al., 2015; Eigenbrode et al., 2016; Isgren et al., 2023).
Trap cropping is a sort of companion planting where specific plants are strategically grown around the field to protect the main crop by reducing pest pressure. Typically, trap crops exhibit higher attractiveness to insects compared to the main crops, and the allocated area for trap crops should be relatively small (Sarkar et al., 2018).
These companion plants (CP) are recognized to emit compounds that may impact the aphid behavior through various ways, including mobility, feeding, and reproductivity (Moreno and Racelis, 2015).
Firstly, volatile organic compounds (VOCs) released by CPs can entice aphids far from their primary host plants. Additionally, they have the ability to modify the perception of the host plant by masking its aroma which renders it undesirable host for aphids, or by emitting repellent volatiles. Thirdly, CP can attract natural enemies by releasing VOCs to enhance and maintain biological pest management (Ben-Issa et al., 2017).
In a research investigation on the black bean aphid (A. fabae) conducted by Nottingham et al., showed that combining host plant volatiles with α-pinene identified in rosemary oil (Rosmarinus officinalis) obscured the host plant odors and make them unattractive in olfactory tests, which disrupted the aphid’s behavioral response. Additionally, the individual volatile compound from CP, when tested alone, was repellent to the aphids.
The 3-butenyl or 4-pentenyl isothiocyanate released by savory (Satureja hortensis) and thyme (Thymus vulgaris) exhibited a similar obscuring impact on enticing host odors for the black bean aphid, but when tested independently, they proved to be repellent.
Repulsive plants, which are non-host plants nearby that disrupt the insect activity and deter feeding on host plants, have been identified in laboratory tests. The compounds myrtenal and isothiocyanates released from the Brassicaceae family, demonstrated repulsing effects on the black bean aphid (A. fabae). Moreover, many terpenoids present in CP-emitted VOCs (e.g., rosemary), like 1,8 cineole, α-pinene, or camphor were found to have a repellent impact on aphids.
Basedow et al. (2006) noticed that the intercropping arrangement of bean plants (V. faba) alongside basilic (Ocimum basilicum) and summer savory (Satureja hortensis) plants (Lamiaceae family) significantly reduced the A. fabae population in wind tunnels, greenhouses, and fields. Tests conducted by Nottingham et al. (1991), employing a straight-line pathway olfactometer and a flight cage to exhibit the deterrent impact of Satureja hortensis and Tanacetum vulgare on A. fabae. Ocimum basilicum also exhibited repellent activity against the black bean aphid.
3.2 The use of VOCs in crop breeding
In most cases, plant VOCs are genetically regulated and highly species specific (Splivallo et al., 2012; Niederbacher et al., 2015). Improved crop varieties, landraces and their wild relatives release various VOCs as a defense mechanism against biotic and abiotic stressors (Moayeri et al., 2007; Thomas et al., 2023). The variability in VOCs content can be an important trait to be exploited in developing germplasm for effective deployment in insect pest and disease management (Keneni et al., 2011; War et al., 2012; Araus and Cairns, 2014; Valencia-Ortiz et al., 2022).
Limited research showed that cultivated crops have less complex inducible volatiles due to modern breeding that make them less ecologically competitive comparing to their wild relatives (Palmgren et al., 2015; Rowen and Kaplan, 2016).
3.2.1 VOCs and breeding for pest resistance
Differences in volatile organic compounds (VOCs) among genotypes offer insights into insect preferences and the degree of damage caused by insects. These findings help breeders to select genotypes that demonstrate higher tolerance or resistance to pest attacks, based on their VOCs profile. They can target crop germplasm that emits fewer herbivore-attractant volatile organic compounds (VOCs) or that increases the release of VOCs known to repel pests.
In cowpea, females of C. maculatus preferred some genotypes over the others in oviposition due to variation in volatile compounds. Ahuchaogu and Ojiako (2021) demonstrated how the mated female C. maculatus responded to odor signals released by multiple bean cultivars (Pinto beans, borno-brown beans and adzuki beans) employing both two-arm and four-arm olfactometers. The study of volatile organic compounds by gas chromatography coupled to mass spectrometry (GCMS) revealed variations in the abundance profile of these compounds. This implies that the nature and amounts of volatile compounds detected in beans impact the female C. maculatus behaviors related to host finding and preference. The volatile compounds like benzyl alcohol, nonanal, and limonene were identified as potential compounds capable to influence the behavioral attractiveness of beetles to specific bean types.
Moreover, the attractiveness of phytophagous alate Aphis craccivora (Hemiptera: Aphididae) in cowpea varies among cultivars of the same species depending on VOCs profile of each genotype. Diabate et al. (2019) identified 23 volatile compounds from four cowpea cultivars with just 4 volatiles (hexanal, 1‐octen‐3‐ol, (E)‐2‐hexenal, and p‐xylene) showing significant differences in emission quantities across the cultivars.
In the olfactometer assays, the aphids exhibited a notable preference for odors from cultivar Ex‐Luanda and significant non‐preference to Katumani 80. Machakos and Ken Kunde generated unbiased reactions.
The Ex‐Luanda cowpea cultivar appeared more attractive than Katumani 80 based on plant odor. The unattractant cultivar Katumani released significant amount of Hexanal and (E)‐2‐ hexenal, while the attractant cultivar Ex‐Luanda emitted large quantities of 1‐octen‐3‐ol and p‐xylene.
The composition and proportions of volatile organic compounds in cowpea varied among varieties. The attractant cowpea cultivar releases the adequate combination and particular proportion of compounds recognized by A. craccivora to identify the host plant, whereas the unattractive cultivar is distinguished via the unsuitable mixture and proportions of compounds.
In tests using standard compounds, A. craccivora responded neutrally once the attractant cowpea cultivar (Ex‐Luanda) was mixed with hexanal and (E)‐2‐hexenal compared to purify air. These two compounds may mask the volatile profile of Ex‐Luanda, making it less attractive.
In contrast, the appellant cultivar Ex‐Luanda was characterized by the presence of 1‐octen‐3‐ol compound. Although the existence of the two compounds 1‐octen‐3‐ol and p‐xylene with the less favored cultivar Katumani, A. craccivora didn’t display attraction.
In grass peas (Lathyrus sativus L.), Mitra et al. (2020) characterized the volatile organic compounds (VOCs) profile of two grass pea genotypes, namely BIO L 212 Ratan and Nirmal B-1, in response to infestation by the viviparous aphid A. craccivora.
The aphids attack led to an elevation in the VOCs emission in comparison to undamaged plants of each cultivar. Nevertheless, the overall quantity of VOCs was grater in the NIR cultivar in comparison with the BIO cultivar, for both undamaged or damaged plants. The overall quantity of VOCs released reflects the extent of insect damage (a greater number of aphids resulting in increased VOCs emission). The GC-MS analysis of the VOCs profile in damaged plants of the NIR cultivar revealed the presence of 4 compounds including thymol, benzyl alcohol, 1,3-diethylbenzene, and 1-hexadecene. In contrast, BIO grass peas cultivar plants exhibited 11 compounds such as benzyl alcohol, diacetone alcohol, p-cymene, linalool oxide, 1,3-diethylbenzene, acetophenone, 1-nonanol, ethylacetophenone, p-cymen-7-ol, thymol, and 1-hexadecene, in their volatile extracts. This blend of VOCs functioned as a lure for A. craccivora. In olfactometer bioassays, female aphids displayed a preference for an artificial mixture comprising 1,3-diethylbenzene, benzyl alcohol, 1-hexadecene, and thymol. This finding suggests the potential use of these compounds in developing lures for an effective control of this insect pest.
In chickpea, trichomes provide a potential resistance mechanism against the pod borer Helicoverpa armigera (Hübner), as highlighted by Brar and Signh (2017). Glandular trichomes, specialized hairs on plant surfaces, serve as physical barriers against herbivores and act as essential chemical defenses. These trichomes produce various secondary chemicals, including terpenoids, phenylpropenes, flavonoids, methyl ketones, acyl sugars, and defensive proteins. Terpenoids, a major component of volatile mixtures triggered by herbivore activity, serve a crucial function in attracting predators and parasitoids to plants infested by herbivore. Additionally, terpenoids contribute to direct defenses by acting as deterrents or repellents, with higher concentrations often proving toxic. For instance, the sesquiterpene (E)-β-farnesene released by glandular trichomes was found to drive away aphids (Myzus persicae), whereas their parasitoids, such as the hymenopteran Diaeretiella rapae, were drawn to (E)-β-farnesene.
Phenylpropenes play double functions, acting as defense mechanism against herbivores, and also serving as attractants for pollinators. Notably, eugenol and methylchavicol are mainly produced and retained in glandular trichomes. The use of synthesized eugenol resulted in morality and deterrence in Coleoptera species. Methyl ketones, a form of volatile compounds derived from fatty-acid, are particularly effective in defending plants against pests. Plants typically contain Methyl ketones with 7 to 15 carbons consist of 2-heptanone, 2-nonanone, 2-undecanone, 2-tridecanone and 2-pentadecanone (Glas et al., 2012; Xing et al., 2017; Kaur and Kariyat, 2020).
3.2.2 VOCs and crop breeding for disease resistance
Evaluation of disease severity is a crucial phase in developing disease-resistant cultivars. Visual evaluation and classical plant phenotyping techniques is a common practice in plant breeding; nevertheless, these methods requires intensive training, destructive harvesting during precise phenological periods, and are both time consuming and expensive.
Due to their particular properties, plant VOCs are a promising tool that could be used as a rapid and non-destructive assessment of plant phenotypic traits (Jud et al., 2018).
Research carried out by Zhang et al. (2023) showed that sensing methods utilizing volatile organic compounds (VOCs) such as a field asymmetric ion mobility spectrometry (FAIMS) system could be an alternative solution for disease monitoring. This method was evaluated as a non-invasive and fast VOC-based phenotyping approach for the surveillance of Ascochyta blight disease intensity in chickpeas.
In addition, the high variability of VOC emissions between plant genotypes and species makes them great phenotypic markers to distinguish between different levels of disease resistance and resilience, which in consequence will contribute to enhance the effectiveness and productivity of crops (Niederbacher et al., 2015).
To evaluate resistance for Aphanomyces root rot disease, caused by Aphanomyces euteiches Drechs., which is a serious soil-borne disease affecting various crops, such as pea plant (Pisum sativum L.). Marzougui et al. (2022) investigated the reaction of two cultivars, Ariel (susceptible) and Hampton (elevated degrees of partial resistance). Disease progression was assessed non-intrusively at three distinct intervals (15, 20, and 30 days post-inoculation). VOCs emitted by both infested and healthy plants were gathered employing dynamic headspace sampling. Analysis via GC-FID and GC-MS revealed a profile of sixteen volatile compounds including 2-Propanone, 2-Butanone, 3-Pentanone, 3-Hexanone, Hexanal, (E)-2-Hexanal, (Z)-3-Hexen-1-ol, (E)-2-Hexen-1-ol, 1-Hexanol, 3-Heptanone, 2-Heptanone, 2-Octanone, (Z)-3-Hexenyl acetate, (E)-2-Hexenyl acetate, Nonanal, and Decanal. At 20 DPI, the Ariel cultivar exhibited a significant increase in hexanal emissions compared to the Hampton cultivar. Concurrently, the standardized proportional peak strength of the Ariel cultivar showed elevated emissions of (Z)-3-hexen-1-ol and (Z)-3-hexenyl acetate (Marzougui et al., 2022).
Several VOCs detected through non-destructive sampling presented significant correlations with visual disease ratings and relative chlorophyll level.
This study indicated that pea varieties with varied level of resistance and susceptibility to Aphanomyces root rot displayed differences in VOC blends across several time intervals and development phases. The observed differences in VOC emissions between cultivars underscore the potential of VOCs as biomarker-based phenotyping tools for distinguishing resistance levels in evaluated pea cultivars. Integrating VOC profiles with high-throughput VOC detection methods presents a promising unique approach for characterizing disease reactions in plants.
Plants exhibiting resistance to herbivores and pathogens release volatile organic compounds (VOCs) that can prompt resistance reactions in nearby healthy plants. Latest investigations suggests that these VOCs may also induce resistance to pathogens through various mechanisms: either by priming the stimulated expression of resistance genes in the receiver plant or by inhibiting directly microbial pathogens, leading to a passive resistance in the plant subjected to VOCs.
Research of Quintana-Rodriguez et al. (2015) revealed that VOCs released by infected resistant bean plants (Phaseolus vulgaris) offered resistance to bean anthracnose caused by Colletotrichum lindemuthianum in a susceptible cultivar after being exposed over 6 hours to volatile compounds collected from the headspace of resistant plants. Furthermore, individual VOCs like limonene, linalool, nonanal, methyl salicylate, and methyl jasmonate, at normal levels, could directly inhibit conidia development and can also suppress the conidia production using an active mycelium in vitro. VOCs from infected plants displayed a more potent inhibitory effect on conidial germination compared to VOCs from uninfected plants. This inhibitory effect was found to be correlated with the abundance of b-linalool, limonene, or methyl jasmonate (Quintana-Rodriguez et al., 2015).
In conclusion, VOCs are pivotal in enhancing bean resistance to fungal pathogens, both by directly reinforcing the emitting plant’s resistance, and indirectly by influencing the resistance traits of nearby receiver plants through induced and associational resistance. Several VOCs like, linalool, limonene, nonanal, methyl salicylate, and methyl jasmonate, exhibit antimicrobial activity (Fernando et al., 2005; Arroyo et al., 2007; Neri et al., 2007). For instance, nonanal has been found to suppress fungal germination in leaves through direct fungistatic actions (Zeringue et al., 1996) and also conferred resistance in lima bean to Pseudomonas syringae (Yi et al., 2009). Notably, the dominant volatile component cis-hexenyl acetate in the volatile blend of the susceptible cultivar, is more probable to be linked to herbivory than pathogen resistance mechanism.
4 Microbial VOCs in food legume crops
During plant-pathogen or plant-pest interactions, microbial antagonists can interrupt the developmental process of pest or pathogen. This may take place via parasitic activity, conflict for area and food, the secretion of hydrolytic enzymes (Punja and Utkhede, 2003), and the release of antimicrobial compounds, including volatiles (Vinale et al., 2006). These microbial VOCs emitted from microorganisms like bacteria and fungi (Korpi et al., 2009; Francis et al., 2010; Thorn and Greenman, 2012) have the ability to travel via both air and ground (Aochi and Farmer, 2005). Therefore, they present an ideal “infochemicals” that mediate interactions of microorganisms with their natural environment (Weisskopf et al., 2021; Morath et al., 2012), including regulation of symbiotic associations, phytotoxicity, enhancement of plant development, activation of plant defense responses against pathogen attack, and insect attractant or repellent activities (Morath et al., 2012; Lee et al., 2016). These characteristics could be utilized to create eco-friendly solutions such as biofertilizers and biopesticides to enhance plant productivity and protection (Kaddes et al., 2020; Soto et al., 2021).
Over a 100 bacteria and fungi emit microbial VOCs in the ground (Effmert et al., 2012), with around 250 fungal VOCs were reported to date (Duc et al., 2022).
Five classes of microbial volatiles (alcohols, ketones, aromatic compounds, terpenes, and organic acids) each comprised at least 10% of the volatiles, reaching 64% of the total diversity. Minor groups including aldehydes, alkanes, alkenes, furans, ester, sulfur and nitrogen combining substances, and ethers, contributing together to over 37% of the total diversity (Schenkel et al., 2015; Bennett and Inamdar, 2015).
Fungi produce various mixtures of volatile organic compounds (VOCs) that are originate from both primary and secondary metabolism processes (Korpi et al., 2009). The composition of these volatile blends can vary based on the fungi species, the intra- and interspecific interactions (Schulz-Bohm et al., 2015; Piechulla et al., 2017), and the growth conditions (temperature, pH, moisture level, substrate, nutrients, and duration of incubation) (Morath et al., 2012; Bennett et al., 2013; Plaszkó et al., 2020). For example, according to Savelieva et al. (2016), Fusarium fungi grown on potato sucrose agar medium (PSA) release a broader range and greater amounts of VOCs than those cultivated on autoclaved wheat kernels (WK). Schenkel et al. (2018) have also reported considerable variances in the VOC composition of the same fungal species when cultivated in soil or malt extract medium. Moreover, the work of Li et al. (2016) showed that VOC retention in soils is affected by various environmental parameters including, temperature, moisture content, and pH that determine the polarity of VOCs and alter their evaporation pressure.
Microbial VOCs can reduce disease severity by inhibiting mycelial growth, creating unfavorable conditions for the development of diseases, stimulating soil-borne biocontrol agents, activating defense responses, and priming plants against future pathogen or pest attacks (Köhl et al., 2019).
One of the initial instances illustrating the inhibitory impact of microbial VOCs on plant pathogens were those generated by Pseudomonas isolates obtained from soybean and canola, which engaged in restraining and minimizing mycelial development of Sclerotinia sclerotiorum (Fernando et al., 2005).
Besides, VOCs released by two endophytic Bacillus have notably decreased both the mass and quantity of S. sclerotiorum persistent forms (sclerotia) (Massawe et al., 2018; Li et al., 2019). Similarly, research conducted by Fialho et al. (2011) demonstrated that the VOCs mixture emitted by S. cerevisiae, comprises alcohols like 3-methyl-1-butanol, ethanol, 2-methyl-1-butanol, and phenylethyl alcohol, and esters such as ethyl acetate and ethyl octanoate, have an effective control over S. sclerotiorum both in vitro and on bean seeds.
The substances 2-methyl-1-butanol, and 3-methyl-1-butanol showed the highest efficacy towards S. sclerotiorum, completely reducing its mycelial development at a concentration of 0.8 µL mL-1, followed by ethyl acetate, at 1.2 µL mL-1. Fumigating bean seeds with S. cerevisiae VOCs at 3.5 µL mL-1 resulted in 75% decrease in S. sclerotiorum occurrence post 4 days.
Trichoderma species have long been considered one of the most promising biocontrol agents; they are capable to generate a wide range of secondary metabolites that could influence the process of their biological function (Li et al., 2016). The research work of Kumar et al. (2019) showed that VOCs emitted by three Trichoderma species Trichoderma harzianum, Trichoderma viride, and Trichoderma konigii have hindered the mycelium development of Fusarium oxysporum f. sp. ciceri by 79.25%, 62.27%, and 50%, respectively. Also, species of Streptomyces have exhibited great potential for controlling plant fungal diseases by producing antifungal compounds (Li et al., 2012). Research carried out by Amini et al. (2016) showed that the antagonistic bacteria Streptomyces spp. has strong inhibitory effects against chickpea wilt. The isolated strains of Streptomyces (KS55, KS58, KS112, KS62, and KS31) produced a mixture of VOCs that can reduce disease severity by inhibiting mycelial growth of the pathogen, ranging from 20.2 to 33.4%.
Moreover, Elbouazaoui et al. (2022) revealed that volatile compounds produced by Bacillus can inhibit the development of Fusarium oxysporum f. sp. ciceri, with a high percentage of inhibition exceeding 30% obtained from B. subtilis.
Concerning the role of VOCs in inducing plant defenses, Ballhorn et al. (2013) investigated the impact of rhizobial symbiosis on volatile production in lima bean plants (Fabaceae: Phaseolus lunatus L.) and their role in legume defenses against herbivores.
They have demonstrated that the volatile organic compounds stimulated by jasmonic acid in rhizobia-colonized lima bean plants have repellent effects on the specialist herbivore pest (Mexican bean beetle; Coccinellidae: Epilachna varivestis). Results revealed that rhizobial symbiosis can induce plant defense via volatile production and can affect the choice behavior of beetles, so it can serve as a fundamental component of legume defenses against herbivores.
Additionally, VOCs produced by Lima bean plants colonized by rhizobia, a nitrogen-fixing bacteria, showed a repellent action on the Mexican bean beetles (Epilachna varivestis), which resulted in less damage from this pest (Bustos-Segura et al., 2024).
Microbial VOC can also control harmful plants and weed. For example, volatile compounds produced by fungi including 3-methyl-1-butanol, 1-octen-3-ol, 2-phenylethanol, 3-octanol, 1-hexanol, 3- octanone, and trans-2-octenal are categorized as toxic against plants. These compounds produced by different fungi can affect negatively the root growth and seed germination (Duc et al., 2022).
5 Conclusion and futures perspectives
In conclusion, the exploration of volatile organic compounds (VOCs) in the management of insect pests and diseases of food legumes holds significant promise and potential.
This assessment underscores the promising role of VOCs in enhancing disease and pest management strategies for cool season food legumes, offering a more sustainable and eco-friendly alternative to traditional control methods. Our research highlights several key findings and advancements in this field. The dynamic role of VOCs in mediating plant responses to biotic stresses, enabling plant to inhibit disease development, induce resistance to pathogens or herbivores, and control pest population in the field by acting as traps. Additionally, the attractive and repellent properties of plant VOCs are exploited in cropping systems of various food legumes, like bean plants, to either repel pest or attract natural enemies which reduce reliance on chemical pesticides and minimize production costs. Furthermore, traditional breeding programs that focus on genetic traits for resistance in food legumes can benefit from using VOCs as biomarkers for rapid, non-invasive plant phenotyping VOCs can also serve as diagnostic tool for an early disease or pest detection, which would allow grower to proactively intervene and select the appropriate control strategy ultimately, reducing yield losses and pesticides use. Integrating plant and microbial VOCs into crop management strategies represents a significant step and a cost-effective solution forward in sustainable agriculture, potentially improving yield quality and crop productivity.
Despite the encouraging prospects, challenges such as environmental sensitivity, inherent reactivity, and low concentrations of VOCs pose obstacles to their widespread implementation. Overcoming these challenges requires continued research to develop advanced analysis techniques, understand the factors influencing VOC production, and their mechanisms of action.
In summary, the use of VOCs in pest and disease management represents an exciting and promising area of research. While there are still many challenges to overcome, continued research has the potential to lead to the development of new, sustainable, and eco-friendly methods for protecting crops and increasing yield.
Author contributions
LM: Formal analysis, Methodology, Writing – original draft. E-FK: Formal analysis, Methodology, Writing – review & editing. S-AK: Conceptualization, Visualization, Writing – review & editing. IM: Formal analysis, Writing – review & editing. IMK: Funding acquisition, Validation, Visualization, Writing – review & editing. ME-B: Conceptualization, Writing – review & editing.
Funding
The author(s) declare financial support was received for the research, authorship, and/or publication of this article. This review is part of the “SpectraVOCS” project, which is funded by the APRD Program and sponsored by the OCP Foundation, Mohammed VI Polytechnic University, the National Center of Scientific and Technical Research (CNRST), and the Ministry of Higher Education, Scientific Research, and Innovation of Morocco.
Acknowledgments
The authors thank OCP Foundation, UM6P, CNRST and DESRS for their financial support.
Conflict of interest
The authors declare that the research was conducted in the absence of any commercial or financial relationships that could be construed as a potential conflict of interest.
Publisher’s note
All claims expressed in this article are solely those of the authors and do not necessarily represent those of their affiliated organizations, or those of the publisher, the editors and the reviewers. Any product that may be evaluated in this article, or claim that may be made by its manufacturer, is not guaranteed or endorsed by the publisher.
References
Adane, A., Vetten, H. J. (2022). Chickpea chlorotic stunt virus : a threat to cool-season food legumes. Arch. Virol. 167 (1). doi: 10.1007/s00705-021-05288-4
Adhikary, P., Mukherjee, A., Barik, A. (2015). Attraction of callosobruchus maculatus (F.) (Coleoptera: Bruchidae) to four varieties of lathyrus sativus l. seed volatiles. Bull. Entomol. Res. 105 (2), 187–201. doi: 10.1017/S000748531400087X
Agegnehu, G., Ghizaw, A., Sinebo, W. (2008). Yield potential and land-use efficiency of wheat and faba bean mixed intercropping. Agron. Sustain. Dev. 28, 257–263. doi: 10.1051/agro:2008012
Ahuchaogu, C. E., Ojiako, F. O. (2021). Host seed type and volatile compound abundance level mould host location and preference behaviours in Callosobruchus maculatus (Fabricius 1775) (Coleoptera: Chrysomelidae). Polish J. Entomol. 90, 152–163. doi: 10.5604/01.3001.0015.4380
Ait Taadaouit, N., El Fakhouri, K., Sabraoui, A., Maalouf, F., Rohi, L., El Bouhssini, M. (2021a). First sources of resistance in faba bean (Vicia faba L.) to the stem borer weevil, Lixus algirus L. (Coleoptera: Curculionidae). Phytoparasitica. 49, 349–356. doi: 10.1007/s12600-021-00885-0
Ait Taadaouit, N., El Fakhouri, K., Sabraoui, A., Rohi, L., El Bouhssini, M. (2021b). Lixus algirus L. (Coleoptera: Curculionidae): biology, population fluctuation, infestation as affected by varieties, location, and planting dates in Morocco. J. Entomol. Acarol. Res. 53, 9324. doi: 10.4081/jear.2021.9324
Ajal, J., Weih, M. (2022). Nutrient accumulation pattern in mixtures of wheat and faba bean is strongly influenced by cultivar choice and co-existing weeds. Biology 11, 630. doi: 10.3390/2Fbiology11050630
Ajayi, O. E., Balusu, R., Morawo, T. O., Zebelo, S., Fadamiro, H. (2015). Semiochemical modulation of host preference of Callosobruchus maculatus on legume seeds. J. Stored Prod. Res. 63, 31–37. doi: 10.1016/j.jspr.2015.05.003
Amini, J., Agapoor, Z., Ashengroph, M. (2016). Evaluation of Streptomyces spp. against Fusarium oxysporum f. sp. ciceris for the management of chickpea wilt. J. Plant Prot. Res. 56, (3). doi: 10.1515/jppr-2016-0038
Annaz, H., El Fakhouri, K., Ben Bakrim, W., Mahdi, I., El Bouhssini, M., Sobeh, M. (2023). Bergamotenes: a comprehensive compile of their natural occurrence, biosynthesis, toxicity, therapeutic merits and agricultural applications. Crit. Rev. Food Sci. Nutr. doi: 10.1080/10408398.2023.2184766
Aochi, Y. O., Farmer, W. J. (2005). Impact of soil microstructure on the molecular transport dynamics of 1,2-dichloroethane. Geoderma. 127, 137–153. doi: 10.1016/j.geoderma.2004.11.024
Araus, J. L., Cairns, J. E. (2014). Field high-throughput phenotyping: the new crop breeding frontier. Trends Plant Sci. 19, 52–61. doi: 10.1016/j.tplants.2013.09.008
Arroyo, F. T., Moreno, J., Daza, P., Boianova, L., Romero, F. (2007). Antifungal activity of strawberry fruit volatile compounds against Colletotrichum acutatum. J. Agric. Food Chem. 55, 5701–5707. doi: 10.1021/jf0703957
Aznar-Fernández, T., Rubiales, D. (2019). Flower and pod source influence on pea weevil (Bruchus pisorum) oviposition capacity and preference. Front. Plant Sci. 10. doi: 10.3389/fpls.2019.00491
Ballhorn, D. J., Kautz, S., Schädler, M. (2013). Induced plant defense via volatile production is dependent on rhizobial symbiosis. Oecologia. 172, 833–846. doi: 10.1007/s00442-012-2539-x
Barney, J. N., Sparks, J. P., Greenberg, J., Whitlow, T. H., Guenther, A. (2009). Biogenic volatile organic compounds from an invasive species: impacts on plant–plant interactions. Plant Ecol. 203, 195–205. doi: 10.1007/s11258-008-9529-4
Basedow, T., Hua, L., Aggarwal, N. (2006). The infestation of vicia faba l. (Fabaceae) by aphis fabae (Scop.) (Homoptera: Aphididae) under the influence of lamiaceae (Ocimum basilicum l. and satureja hortensis l.). J. Pest Sci. 79, 149–154. doi: 10.1007/s10340-006-0128-7
Ben-Issa, R., Gomez, L., Gautier, H. (2017). Companion plants for aphid pest management. Insects 8 (4), 112. doi: 10.3390/insects8040112
Bennett, J. W., Hung, R., Lee, S., Padhi, S. (2013). Fungal and bacterial volatile organic compounds; an overview and their role as ecological signaling agents. The Mycota IX Fungal Interactions (Berlin/Heidelberg, Germany: The Mycota IX Fungal Interactions), 373–393. doi: 10.1007/978-3-642-30826-0_18
Bennett, J. W., Inamdar, A. A. (2015). Are some fungal volatile organic compounds (VOCs) mycotoxins? Toxins (Basel). 7 (9), 3785–3804. doi: 10.3390/toxins7093785
Bezerra, R., Souto, L., Sant'Ana, A., Ambrogi, B. (2021). Indirect plant defenses: volatile organic compounds and extrafloral nectar. Arthropod-Plant Interact. 15 (4). doi: 10.1007/s11829-021-09837-1
Boulamtat, R., Lhaloui, S., Sabraoui, A., El-Fakhouri, K., Oubayoucef, A., Mesfioui, A., et al. (2019). Antifeedant and larvicidal activities of Mentha pulegium on chickpea pod borer Helicoverpa armigera (Lepidoptera: Noctuidae). Int. J. Trop. Insect Sci. 40, 151–156. doi: 10.1007/s42690-019-00064-z
Brar, H. S., Singh, R. (2017). Role of trichomes on leaves and pods for imparting resistance in chickpea [Cicer arientinum (L.)] genotypes against helicoverpa armigera. J. Appl. Nat. Sci. 9, 2193–2198. doi: 10.31018/JANS.V9I4.1509
Brilli, F., Loreto, F., Baccelli, I. (2019). Exploiting plant volatile organic compounds (VOCs) in agriculture to improve sustainable defense strategies and productivity of crops. Front. Plant Sci. 10. doi: 10.3389/fpls.2019.00264
Bruce, T. J. A., Martin, J. L., Smart, L. E., Pickett, J. A. (2011). Development of semiochemical attractants for monitoring bean seed beetle, Bruchus rufimanus. Pest Manage. Science. 67, 1303–1308. doi: 10.1002/ps.2186
Bruno, D., Grossi, G., Salvia, R., Scala, A., Farina, D., Grimaldi, A., et al. (2018). Sensilla morphology and complex expression pattern of odorant binding proteins in the vetch aphid megoura viciae (Hemiptera: aphididae). Front. Physiol. 9, 777. doi: 10.3389/fphys.2018.00777
Bukovinszky, T., Tréfás, H., van Lenteren, J. C., Vet, L. E. M., Fremont, J. (2004). Plant competition in pest-suppressive intercropping systems complicates evaluation of herbivore responses. Agric. Ecosyst. Environ. 102 (2), 185–196. doi: 10.1016/j.agee.2003.08.008
Bustos-Segura, C., Godschalx, A. L., Malacari, L., Deiss, F., Rasmann, S., Ballhorn, D. J., et al. (2024). Rhizobia-legume symbiosis mediates direct and indirect interactions between plants, herbivores and their parasitoids. Heliyon. 10 (6), e27815. doi: 10.1016/j.heliyon.2024.e27815
Calles, T. (2016). The International Year of Pulses: what are they and why are they important. Agric. Dev. 26, 40–42.
Carrillo-Perdomo, E., Raffiot, B., Ollivier, D. (2018). Identification of novel sources of resistance to seed weevils (Bruchus spp.) in a faba bean germplasm collection. Front. Plant Sci. 10. doi: 10.3389/fpls.2018.01914
Castro, A. M., Tapias, J., Ortiz, A., Benavides, P., Góngora, C. E. (2017). Identification of attractant and repellent plants to coffee berry borer, Hypothenemus hampei. Entomologia Experimentalis Applicata 164, 120–130. doi: 10.1111/eea.2017.164.issue-2
Ceballos, R., Fernández, N., Zúñiga, S., Zapata, N. (2015). Electrophysiological and behavioral responses of pea weevil Bruchus pisorum L. (Coleóptera: Bruchidae) to volatiles collected from its host Pisum sativum L. Chilean J. Agric. Res. 75, 202–209. doi: 10.4067/S0718-58392015000200009
Choudhary, D. K., Johri, B. N., Prakash, A. (2008). Volatiles as priming agents that initiate plant growth and defence responses. Curr. Sci. 94, 595–604.
Chrigui, N., Sari, D., Sari, H. (2020). Introgression of Resistance to Leaf miner (Liriomyza cicerina Rondani) from Cicer reticulatum Ladiz. to C. arietinum L. and Relationships between Potential Biochemical Selection Criteria. Agronomy. 11, 57. doi: 10.3390/agronomy11010057
Congdon, B. S., Coutts, B. A., Renton, M., Flematti, G. R., Jones, R. A. C. (2017). Establishing alighting preferences and species transmission differences for Pea seed-borne mosaic virus aphid vectors. Virus Res. 241, 145–155. Available at: https://www.sciencedirect.com/science/article/pii/S0168170217301764.
Cruz, A. F., Hamel, C., Yang, C. (2012). Phytochemicals to suppress Fusarium head blight in wheat–chickpea rotation. Phytochemistry. 78, 72–80. doi: 10.1016/j.phytochem.2012.03.003
Dell'Aglio, D. D., Tayeh, N. (2023). Responsiveness of the broad bean weevil, Bruchus rufimanus, to Vicia faba genotypes. Entomologia Experimentalis Applicata. 171, 312–322. doi: 10.1111/eea.13277
Delory, B. M., Delaplace, P., Fauconnier, M. L., Du Jardin, P. (2016). Root-emitted volatile organic compounds: can they mediate belowground plant-plant interactions? Plant Soil 402, 126. doi: 10.1007/s11104-016-2823-3
Devrnja, N., Milutinovic, M., Savic, J. (2022). When scent becomes a weapon— plant essential oils as potent bioinsecticides. Sustainability 14, 6847. doi: 10.3390/su14116847
Diabate, S., Deletre, E., Murungi, L. K. (2019). Behavioural response of alate Aphis craccivora Koch (Homoptera: Aphididae) to volatiles from different cowpea cultivars. J. Appl. Entomol. 143, 659–669. doi: 10.1111/jen.12633
Dicke, M., Loreto, F. (2010). Induced plant volatiles: from genes to climate change. Trends Plant Sci. 15, 115–117. doi: 10.1016/j.tplants.2010.01.007
Duc, N. G., Ha, T. N. V., van Doan, C., Hamow, H. A. (2022). Volatile organic compounds shape belowground plant–fungi interactions. Front. Plant Sci. 13. doi: 10.3389/fpls.2022.1046685
Dudareva, N., Negre, F., Aharoni, A., et al. (2004). Plant volatiles: Recent advances and future perspectives. Crit. Rev. Plant Sci. 23 (5), 539–558.
Effmert, U., Kalderás, J., Warnke, R., Piechulla, B. (2012). Volatile mediated interactions between bacteria and fungi in the soil. J. Chem. Ecol. 38 (6), 665–703. doi: 10.1007/s10886-012-0135-5
Eigenbrode, S. D., Birch, A. N. E., Lindzey, S., Meadow, R., Snyder, W. E., Pocock, M. (2016). Review: A mechanistic framework to improve understanding and applications of pushpull systems in pest management. J. Appl. Ecol. 53, 202–212. doi: 10.1111/jpe.2016.53.issue-1
Elbouazaoui, A., Douira, A., Maafa, I., Ahmed, S. K. (2022). Integrating sowing date with chickpea genotypes in managing fusarium wilt in Morocco. Agriculture. 12, 773. doi: 10.3390/agriculture12060773
El Fakhouri, K., Boulamtat, R., Sabraoui, A., El Bouhssini, M. (2022). The chickpea pod borer, helicoverpa armigera (Hübner): yield loss estimation and biorational insecticide assessment in Morocco. Agronomy. 12, 3017. doi: 10.3390/agronomy1212301
El Fakhouri, K., Huang, J., Sabraoui, A., Aasfar, A., El Bouhssini, M., Gut, L. (2021). Screening of volatile compounds used in host location by the faba bean stem borer, Lixus algirus on faba bean in Morocco.
Emery, S. E., Anderson, P., Carlsson, G. (2021). The potential of intercropping for multifunctional crop protection in oilseed rape (Brassica napus L.). Front. Agron. 3. doi: 10.3389/fagro.2021.782686
Fernando, W. G. D., Ramarathan, R., Krishnamoorthy, A. S., Savchuk, S. C. (2005). Identification and use of potential bacteria organic antifungal volatile isolates in biocontrol. Soil Biol. Biochem. 37, 955–964. doi: 10.1016/j.soilbio.2004.10.021
Fialho, M. B., Heloisa, M., Moraes, D., Tremocoldi, A. R., Pascholati, S. F. (2011). Potential of antimicrobial volatile organic compounds to control Sclerotinia sclerotiorum in bean seeds. Pesq. Agropec. Bras. Brasília. 46, 137–142. doi: 10.1590/S0100-204X2011000200004
Ficke, F., Asalf, B., Norli, H. R. (2021). Volatile organic compound profiles from wheat diseases are pathogen-specific and can be exploited for disease classification. Front. Microbiol. 12. doi: 10.3389/fmicb.2021.803352
Fountain, M. T., Baroffio, C., Borg-Karlson, A. K., Brain, P., Cross, J. V., Farman, D. I., et al. (2017). Design and deployment of semiochemical traps for capturing Anthonomus rubi Herbst (Coleoptera: Curculionidae) and Lygus rugulipennis Poppius (Hetereoptera: Miridae) in soft fruit crops. Crop Prot. 99, 1–9. doi: 10.1016/j.cropro.2017.05.001
Francis, I., Holsters, M., Vereecke, D. (2010). The gram-positive side of plant-microbe interactions. Environ. Microbiol. 12, 1–12. doi: 10.1111/j.1462-2920.2009.01989.x
Gaba, S., Lescourret, F., Boudsocq, S. (2015). Multiple cropping systems as drivers for providing multiple ecosystem services from concepts to design. Agron. Sustain. Dev. 35, 607–623. doi: 10.1007/s13593-014-0272-z
Gaur, P. M., Jukanti, A. K., Varshney, R. (2012). Impact of genomic technologies on chickpea breeding strategies. Agron. J. 2, 199–221. doi: 10.3390/agronomy2030199
Ge, J., Li, N., Yang, J. (2019). Female adult puncture-induced plant volatiles promote mating success of the pea leaf miner via enhancing vibrational signals. Phil. Trans. R. Soc. B374, 20180318. doi: 10.1098/rstb.2018.0318
Ghaley, B. B., Hauggaard-Nielsen, H., Høgh-Jensen, H. (2005). Intercropping of wheat and pea as influenced by nitrogen fertilization. Nutr. Cycl Agroecosyst 73, 201–212. doi: 10.1007/s10705-005-2475-9
Glas, J. J., Schimmel, B. C. J., Alba, J. M. (2012). Plant glandular trichomes as targets for breeding or engineering of resistance to herbivores. Int. J. Mol. Sci. 13, 17077–17103. doi: 10.3390/ijms131217077
Gualtieri, L., Monti, M. M., Mele, F., Russo, A., Pedata, P. A., Ruocco, M. (2022). Volatile organic compound (VOC) profiles of different trichoderma species and their potential application. J. Fungi. 8, 989. doi: 10.3390/jof8100989
Guerrieri, E., Digilio, M. C. (2008). Aphid-plant interactions: a review. J. Plant Interactions. 3, 223–232. doi: 10.1080/17429140802567173
Hamdi, S. H., Abidi, S., Sfayhi, D. (2017). Nutritional alterations and damages to stored chickpea in relation with the pest status of Callosobruchus maculatus (Chrysomelidae). J. Asia-Pacific Entomol. 20, 1067–1076. doi: 10.1016/j.aspen.2017.08.008
Haware, M. P., Nene, Y. L. (1982). Symptomless carriers of the chickpea wilt Fusarium. Plant Dis. 66, 809–810. doi: 10.1094/PD-66-809
Hegde, M., Oliveira, J. N., da Costa, J. G., Bleicher, E., Santana, A. E. G., Bruce, T. J. A., et al. (2011). Identification of Semiochemicals Released by Cotton, Gossypium hirsutum, Upon Infestation by the Cotton Aphid, Aphis gossypii. J. Chem. Ecol. 37, 741–750. doi: 10.1007/s10886-011-9980-x
Horiuchi, J. I., Badri, D. V., Kimball, B. A., Negre, F., Dudareva, N., Paschke, M. W., et al. (2007). The floral volatile, methyl benzoate, from snapdragon (Antirrhinum majus) triggers phytotoxic effects in Arabidopsis thaliana. Planta. 226, 1–10. doi: 10.1007/s00425-006-0464-0
Houasli, C., Sahri, A., Nsarellah, N. (2021). Chickpea (Cicer arietinum L.) breeding in Morocco: genetic gain and stability of grain yield and seed size under winter planting conditions. Euphytica. 217, 159. doi: 10.1007/s10681-021-02885-x
Isgren, E., Clough, Y., Murage, A. (2023). Are agricultural extension systems ready to scale up ecological intensification in East Africa? A literature review with particular attention to the Push−Pull Technology (PPT). Food Security. 15, 1399–1420. doi: 10.1007/s12571-023-01387-z
Jones, R. A. C., Roger, A. C. (2021). Global plant virus disease pandemics and epidemics. Plants. 10, 233. doi: 10.3390/plants10020233
Jud, W., Winkler, J. B., Niederbacher, B. (2018). Volatilomics: a non-invasive technique for screening plant phenotypic traits. Plant Methods 14, 109. doi: 10.1186/s13007-018-0378-4
Kaddes, A., Fauconnier, M. L., Sassi, K., Berhal, C., Nasraoui, B., Jijakli, H. (2020). Efficacité des Composés Organiques Volatils fongiques (synthèse bibliographique). Biotechnol. Agron. Société Environ. 24, 81–98. doi: 10.25518/1780-4507.18531
Karolkowski, A., Guichard, E., Briand, L. (2021). Volatile compounds in pulses: A review. Foods 10, 3140. doi: 10.3390/foods10123140
Kashyap, B., Kumar, R. (2021). Sensing methodologies in agriculture for monitoring biotic stress in plants due to pathogens and pests. Inventions 6, 29. doi: 10.3390/inventions6020029
Kasote, D., Lee, J., Sreenivasulu, N. (2023). Editorial: Volatilomics in plant and agricultural research: recent trends. Front. Plant Sci. 14, 1289998. doi: 10.3389/fpls.2023.1289998
Kaur, J., Kariyat, R. (2020). “Role of Trichomes in Plant Stress Biology,” in Evolutionary Ecology of Plant-Herbivore Interaction. Eds. Núñez-Farfán, J., Valverde, P.. (Cham: Springer), 15–35. doi: 10.1007/978-3-030-46012-9_2
Keneni, K., Bekele, B., Getu, E., Imtiaz, M., Damte, T., Mulatu, M., et al. (2011). Breeding food legumes for resistance to storage insect pests: potential and limitations. Sustainability 3, 1399–1415. doi: 10.3390/su3091399
Köhl, J., Kolnaar, R., Ravensberg, W. J. (2019). Mode of action of microbial biological control agents against plant diseases: relevance beyond efficacy. Front. Plant Sci. 10. doi: 10.3389/fpls.2019.00845
Kong, C.-H., Li, Z., Li, F.-L., Xia, X.-X., Wang, P. (2024). Chemically mediated plant–plant interactions: Allelopathy and allelobiosis. Plants 13, 626. doi: 10.3390/plants13050626
Korpi, A., Jarnberg, J., Pasanen, A. L. (2009). Microbial volatile organic compounds. Crit. Rev. Toxicol. 39, 139–193. doi: 10.1080/10408440802291497
Kramer, R., Abraham, W. R. (2012). Volatile sesquiterpenes from fungi: What are they good for? Phytochem 11, 15–37. doi: 10.1007/s11101-011-9216-2
Krimi, S. B., Ahmed, S., Imtiaz, M., Hamwieh, A., Udupa, S. M., Sahri, A., et al. (2022). Pathogen diversity and mating types of Didymella rabiei isolates collected from Morocco. Curr. Plant Biol. 29, 100231. doi: 10.1016/j.cpb.2021.100231
Kumar, M., Kumar, V., Rana, M., Srivastava, S. (2019). Effect of volatile and nonvolatile compounds of Trichoderma spp. Against Fusarium isolates causing chickpea wilt in Punja. Plant Arch. 19, 159–162.
Lee, S., Yap, M., Behringer, G., Hung, R., Bennett, J. W. (2016). Volatile Organic Compounds emitted by Trichoderma species mediate plant growth. Fungal Biol. Biotechnol. 3, 7. doi: 10.1186/s40694-016-0025-7
Li, F., Tang, M., Tang, X., Sun, W., Gong, J., Yi., Y. (2019). Bacillus subtilis-Arabidopsis thaliana: a model interaction system for studying the role of volatile organic compounds in the interchange between plants and bacteria. Botany 97, (12). doi: 10.1139/cjb-2019-0093
Li, Q., Ning, P., Zheng, L., Huang, J., Li, G., Hsiang, T. (2012). Effects of volatile substances of Streptomyces globisporus JK-1 on control of Botrytis cinerea on tomato fruit. Biol. Control 61, 113–120. doi: 10.1016/j.biocontrol.2011.10.014
Li, G., Su, H., Li, X., Kuhn, U., Meusel, H., Hoffmann, T., et al. (2016). Uptake of gaseous formaldehyde by soil surfaces: a combination of adsorption/desorption equilibrium and chemical reactions. Atmos. Chem. Phys. 16, 10299–10311. doi: 10.5194/acp-16-10299-2016
Lokesh, B. K., Shashidhara, N., Kantharaju, V. (2020). Survey for the incidence of wilt disease and management of wilt in chickpea. Int. J. Agric. Sci. 16, 91–94. doi: 10.15740/HAS/IJAS/16.1/91-94
Maffei, M. E., Mithofer, A., Boland, W. (2007). Insects feeding on plants: rapid signals and responses preceding the induction of phytochemical release. Phytochemistry. 68, 22-24, 2946–2959. doi: 10.1016/j.phytochem.2007.07.016
Makhlouf, L., El Fakhouri, K., Kemal, S. A., Maafa, I., Meftah Kadmiri, I., El Bouhssini, M. (2024). Advances in analytical techniques for assessing volatile organic compounds in pulse crops: a comprehensive review. Front. Hortic. 3, 1394041. doi: 10.3389/fhort.2024.1394041
Makkouk, K. M. (2020). Plant pathogens which threaten food security: viruses of chickpea and other cool season legumes in West Asia and North Africa. Food Sec. 12, 495–502. doi: 10.1007/s12571-020-01017-y
Mario, M. B., Astuti, L. P., Jue-Liang, H. (2023). Bioefficacy of eight different plant powders applied as fumigants against the adzuki bean weevil, Callosobruchus chinensis. Crop Prot. 167, 6200. doi: 10.1016/j.cropro.2023.106200
Marzougui, A., Rajendran, A., Mattinson, D. S., Ma, Y., McGee, R., Garcia-Perez, M., et al. (2022). Evaluation of biogenic markers-based phenotyping for resistance to Aphanomyces root rot in field pea. Inf. Process. Agric. 9 (1), 1–10. doi: 10.1016/j.inpa.2021.01.007
Massawe, V., Hanif, A., Farzand, A., Mburu, D. K., Ochola, S., Wu, L., et al. (2018). Volatile organic compounds of endophytic Bacillus spp. have biocontrol activity against sclerotinia sclerotiorum. Phytopathology. 108 (12). doi: 10.1094/PHYTO-04-18-0118-R
Mendesil, E., Rämert, B., Marttila, S. (2016). Oviposition Preference of Pea Weevil, Bruchus pisorum L. Among Host and Non-host Plants and its Implication for Pest Management. Front. Plant Sci. 6. doi: 10.3389/fpls.2015.01186
Mitra, P., Das, S., Debnath, R. (2020). Identification of Lathyrus sativus plant volatiles causing behavioral preference of Aphis craccivora. Pest Manage. Sci. 77, 285–299. doi: 10.1002/ps.6018
Moayeri, H. R. S., Ashouri, A., Poll, L., Enkegaard, A. (2007). Olfactory response of a predatory mirid to herbivore induced plant volatiles: multiple herbivories vs. single herbivory. J. Appl. Entomol. 131, 326–332. doi: 10.1111/j.1439-0418.2007.01177.x
Morath, S. U., Hung, R., Bennett, J. W. (2012). Fungal volatile organic compounds: A review with emphasis on their biotechnological potential. Fungal Biol. Rev. 26, 73–83. doi: 10.1016/j.fbr.2012.07.001
Moreno, C. R., Racelis, A. E. (2015). Attraction, repellence, and predation: role of companion plants in regulating myzus persicae (Sulzer) (Hemiptera: aphidae) in organic kale systems of South Texas. Southwestern Entomologist. 40, 1–14. doi: 10.3958/059.040.0101
Murage, A. W., Pittchar, J. O., Midega, C. A. O. (2015). Gender specific perceptions and adoption of the climate-smart push–pull technology in eastern Africa. Crop Prot. 76, 83–91. doi: 10.1016/j.cropro.2015.06.014
Murphy-Bokern, D. (2022). Developing legume-supported cropping systems in Europe: Have we overlooked something? Ann. Appl. Biol. 181, 133–136. doi: 10.1111/aab.12764
Mutyambai, D. M., Bruce, T. J., van den Berg, J., Midega, C. A., Pickett, J. A., Khan, Z. R. (2016). An indirect defence trait mediated through igg-induced maize volatiles from neighbouring plants. PloS One 11, 0158744. doi: 10.1371/journal.pone.0158744
Mweke, A., Akutse, K. S., Ulrichs, C. (2020). Integrated Management of Aphis craccivora in Cowpea Using Intercropping and Entomopathogenic Fungi under Field Conditions. J. Fungi. 6, 60. doi: 10.3390/jof6020060
Neri, F., Mari, M., Brigati, S., Bertolini, P. (2007). Fungicidal activity of plant volatile compounds for controlling Monilinia laxa in stone fruit. Plant Dis. 91, 30–35. doi: 10.1094/PD-91-0030
Niederbacher, B., Winkler, J. B., Schnitzler, J. P. (2015). Volatile organic compounds as non-invasive markers for plant phenotyping. J. Exp. Bot. 66, 5403–5416. doi: 10.1093/jxb/erv21
Nottingham, S., Hardie, J., Dawson, G., Hick, A., Pickett, J., Wadhams, L., et al. (1991). Behavioral and electrophysiological responses of aphids to host and nonhost plant volatiles. J. Chem. Ecol. 17, 1231–1242. doi: 10.1007/BF01402946
Oliva, J., Navarro, S., Barba, A., Navarro, G., Salinas, M. R. (1999). Effect of pesticide residues on the aromatic composition of red wines. J. Agric. Food Chem. 47, 2830–2836. doi: 10.1021/jf9813135
Olufemi, O., Pitan, R., Odebiyi, J. A. (2001). The effect of intercropping with maize on the level of infestation and damage by pod-sucking bugs in cowpea. Crop Prot. 20, 367–372. doi: 10.1016/S0261-2194(00)00135-6
Ogenga-Latigo, M. W., Ampofo, J., Baliddawa, C. (1992). Influence of maize row spacing on infestation and damage of intercropped beans by the bean aphid (Aphis fabae scop.). i. incidence of aphids. Agric. Food Sci. 30, 111–121. doi: 10.1016/0378-4290(92)90060-M
Onge, A., Cárcamo, H. A., Evenden, M. L. (2018). Evaluation of semiochemical-baited traps for monitoring the pea leaf weevil, sitona lineatus (Coleoptera: curculionidae) in field pea crops. Environ. Entomol. 47, 93–106. doi: 10.1093/ee/nvx180
Palmgren, M. G., Edenbrandt, A. K., Vedel, S. E., Andersen, M. M., Landes, X., Østerberg, J. T., et al. (2015). Are we ready for back-to-nature crop breeding? Trends Plant Sci. 20 (3), 155–164. doi: 10.1016/j.tplants.2014.11.003
Patel, H. K., Gomes, E. N., Wu, Q., Patel, N., Kobayashi, D. Y., Wang, C., et al. (2023). Volatile metabolites from new cultivars of catnip and oregano as potential antibacterial and insect repellent agents. Front. Plant Sci. 14, 1124305. doi: 10.3389/fpls.2023.1124305
Pawar, P., Baskaran, R. K. M., Sharma, K. C., Marathe, A. (2023). Enhancing biocontrol potential of trichogramma chilonis against borer pests of wheat and chickpea. iScience 26. doi: 10.1016/j.isci.2023.106512
Pérez-Hedo, M., Gallego-Giraldo, C., Forner-Giner, M. Á., Ortells-Fabra, R., Urbaneja, A. (2024). Plant volatile-triggered defense in citrus against biotic stressors. Front. Plant Sci. 15, 1425364. doi: 10.3389/fpls.2024.1425364
Pelzer, E., Bazot, M., Makowski, D. (2012). Pea–wheat intercrops in low-input conditions combine high economic performances and low environmental impacts. Eur. J. Agron. 40, 39–53. doi: 10.1016/j.eja.2012.01.010
Pickett, J. A., Khan, Z. R. (2016). Plant volatile-mediated signalling and its application in agriculture: successes and challenges. New Phytologist. 212, 856–870. doi: 10.1111/nph.14274
Piechulla, B., Lemfack, M. C., Kai, M. (2017). Effects of discrete bioactive microbial volatiles on plants and fungi. In Plant Cell Environ. 40: 10, 2042–2067. doi: 10.1111/pce.v40.10
Pierre, J. F., Jacobsen, K. L., Latournerie-Moreno, L. (2023). A review of the impact of maize-legume intercrops on the diversity and abundance of entomophagous and phytophagous insects. Peer J. 11, e15640. doi: 10.7717/peerj
Piesik, A. W. (2011). Volatile Organic Compound Emissions by Winter Wheat Plants (Triticum aestivum L.) under Fusarium spp. Infestation and Various Abiotic Conditions. Pol. J. Environ. Stud. 20, 1335–1342.
Plaszkó, T., Kállai, Z., Csoma, H., Vasas, G., Gonda, S. (2020). Volatile organic compounds (VOCs) of endophytic fungi growing on extracts of the host, horseradish (Armoracia rusticana). Metabolites 10, 451. doi: 10.3390/metabo10110451
Punja, Z. K., Utkhede, R. S. (2003). Using fungi and yeasts to manage vegetable crop diseases. Trends Biotechnol. 21, 400–407. doi: 10.1016/S0167-7799(03)00193-8
Quintana-Rodriguez, E., Morales-Vargas, A. T., Molina-Torres, J. (2015). Plant volatiles cause direct, induced and associational resistance in common bean to the fungal pathogen Colletotrichum lindemuthianum. J. Ecol. 103, 250–260. doi: 10.1111/1365-2745.12340
Rajendran, S., Sriranjini, V. (2007). Use of fumigation for managing grain quality. Stewart Postharvest Review. 3 (6), 1–8. doi: 10.2212/spr.2007.6.9
Reddy, G. V. P., Sharma, A., Gadi, R. L. (2018). Biology, ecology, and management of the pea weevil (Coleoptera: chrysomelidae). Ann. Entomol. Soc. Am. 111, 161–171. doi: 10.1093/aesa/sax078
Reisenman, C. E., Lei, H., Guerenstein, P. G. (2016). Neuroethology of olfactory-guided behavior and its potential application in the control of harmful insects. Front. Physiol. 7, 271. doi: 10.3389/fphys.2016.00271
Rowen, E., Kaplan, I. (2016). Eco-evolutionary factors drive induced plant volatiles: a meta-analysis. New Phytologist. 210, 284–294. doi: 10.1111/nph.13804
Russo, A., Pollastri, S., Ruocco, M., Monti, M. M., Loreto, F. (2022). Volatile Organic Compounds in the interaction between plants and beneficial microorganisms. Plant Interact. 17, 840–852. doi: 10.1080/17429145.2022.2107243
Sabraoui, A., Lhaloui, S., Bouchelta, A., El Fakhouri, K., El Bouhssini, M. (2019). Grain yield losses due to leaf miner (Liriomyza cicerina R.) in winter- and spring-planted chickpea in Morocco. Crop Prot. 117, 115–120. doi: 10.1016/j.cropro.2018.11.021
Sakurai, Y., Ishizaki, S., Izumi, S., Yoshida, T., Shiojiri, K., Takabayashi, J. (2023). The exposure of field-grown maize seedlings to weed volatiles affects their growth and seed quality. Front. Plant Sci. 14. doi: 10.3389/fpls.2023.1141338
Sampaio, A. M., Araújo, S. S., Rubiales, D., Vaz Patto, M. C. (2020). Fusarium wilt management in legume crops. Agronomy. 10, 1073. doi: 10.3390/agronomy10081073
Santos, D. R. C., Peñaflor, M. F. G. V., Sanches, P. A., Nardi, C., Bento, J. M. S. (2015). The effects of Gibberella zeae, Barley Yellow Dwarf Virus, and co-infection on Rhopalosiphum padi olfactory preference and performance. Phytoparasitica. 44, 47–54. doi: 10.1007/s12600-015-0493-y
Sarkar, S. H., Wang, E., Wu, S. (2018). Application of trap cropping as companion plants for the management of agricultural pests: A review. Insects 9, 128. doi: 10.3390/insects9040128
Savelievaa, E. I., Gustylevaa, L. K., Kessenikha, E. D., Khlebnikovaa, N. S., Leffingwellb, J., Gavrilovac, O. P., et al. (2016). Study of the vapor phase over fusarium fungi cultured on various substrates. Chem. Biodiversity. 13, 891–903. doi: 10.1002/cbdv.201500284
Schenkel, D., Lemfack, M. C., Piechulla, B. (2015). A meta-analysis approach for assessing the diversity and specificity of belowground root and microbial volatiles. Front. Plant Sci. 6. doi: 10.3389/fpls.2015.00707
Schenkel, D., Maciá-Vicente, J. G., Bissell, A., Splivallo, R. (2018). Fungi indirectly affect plant root architecture by modulating soil volatile organic compounds. Front. Microbiol. Sec. Plant Pathogen Interact. 9. doi: 10.3389/fmicb.2018.01847
Schulz-Bohm, K., Zweers, H., de Boer, W., Garbeva, P. (2015). A fragrant neighborhood: Volatile mediated bacterial interactions in soil. Front. Microbiol. 6, 1212. doi: 10.3389/fmicb.2015.01212
Schwartzberg, E. G., Böröczky, K., Tumlinson, J. H. (2011). Pea aphids, acyrthosiphon pisum, suppress induced plant volatiles in broad bean, vicia faba. J. Chem. Ecol. 37, 1055–1062. doi: 10.1007/s10886-011-0006-5
Semba, R. D., Ramsing, R., Rahman, N. (2021). Legumes as a sustainable source of protein in human diets. Global Food Security. 28, 100520. doi: 10.1016/j.gfs.2021.100520
Sharma, H. C., Manuele, T., El Bouhssini, M. (2016). “Pest Management in Grain Legumes: Potential and Limitations,” in Integrated Pest Management in the Tropics. Ed. Abrol, D. P. (New India Publishing Agency, New Delhi (India), 275–292.
Shrivastava, G., Rogers, M., Wszelaki, A. (2010). Plant volatiles-based insect pest management in organic farming. Crit. Rev. Plant Sci. 29, 123–133. doi: 10.1080/07352681003617483
Singh, R., Kumar, K., Purayannur, K. (2022). Ascochyta rabiei: A threat to global chickpea production. Mol. Plant Pathol. 23, 1241–1261. doi: 10.1111/mpp.13235
Singh, K. D., Mobolade, A. J., Bharali, R. (2021). Main plant volatiles as stored grain pest management approach: A review. J. Agric. Food Res. 4, 100127. doi: 10.1016/j.jafr.2021.100127
Soltani, A., Ben Abda, M., Amri, M., Carapelli, A., Ben Jemâa, J. M. (2020). Seasonal incidence of the leaf miner Liriomyza cicerina Rond (Diptera: Agromyzidae) in chickpea fields and effects of climatic parameters, chickpea variety, and planting date on the leaf miner infestation rate. Euro-Mediterranean J. Environ. Integration. 5), 58. doi: 10.1007/s41207-020-00198-4
Song, J., Lee, G., Jung, J., Moon, J. K., Kim, S. (2022). Effect of soybean volatiles on the behavior of the bean bug. Riptortus pedestris. J. Chem. Ecology. 48, 207–218. doi: 10.1007/s10886-021-01343-1
Soto, M. J., López-Lara, I. M., Geiger, O. (2021). Rhizobia volatiles: potential new players in the complex interkingdom signaling with legumes. Front. Plant Sci. 12. doi: 10.3389/fpls.2021.698912
Splivallo, R., Valdez, N., Kirchhoff, N., Ona, M. C., Schmidt, J. P., Feussner, I., et al. (2012). Intraspecific genotypic variability determines concentrations of key truffle volatiles. New Phytologist. 194, 823–835. doi: 10.1111/j.1469-8137.2012.04077.x
Tatineni, S., Hein, G. L. (2023). Plant viruses of agricultural importance: current and future perspectives of virus disease management strategies. Phytopathology. 113, 117–141. doi: 10.1094/PHYTO-05-22-0167-RVW
Thomas, G., Rusman, Q., Morrison, W. R., III (2023). Deciphering plant-insect-microorganism signals for sustainable crop production. Biomolecules. 13, 997. doi: 10.3390/biom13060997
Thorn, R. M., Greenman, J. (2012). Microbial volatile compounds in health and disease conditions. J. Breath Res. 6 (2), 024001. doi: 10.1088/1752-7155/6/2/024001
Valencia-Ortiz, M., Marzougui, A., Zhang, C. (2022). Biogenic VOCs emission profiles associated with plant-pest interaction for phenotyping applications. Sensors. 22, 4870. doi: 10.3390/s22134870
Vinale, F., Marra, R., Scala, F., Ghisalberti, E. L., Lorito, M., Sivasithamparam, K. (2006). Major secondary metabolites produced by two commercial Trichoderma strains active against different phytopathogens. Lett. Appl. Microbiol. 43, 143148. doi: 10.1111/j.1472-765X.2006.01939.x
Wahbi, S., Prin, Y., Thioulouse, J. (2016). Impact of wheat/faba bean mixed cropping or rotation systems on soil microbial functionalities. Front. Plant Sci. 7. doi: 10.3389/fpls.2016.01364
War, A. R., Paulraj, M. G., Ahmad, T. (2012). Mechanisms of plant defense against insect herbivores. Plant Signaling Behav. 7, 1306–1320. doi: 10.4161/psb.21663
Webster, B., Bruce, T., Dufour, S. (2008). Identification of volatile compounds used in host location by the black bean aphid, aphis fabae. J. Chem. Ecol. 34, 1153–1161. doi: 10.1007/s10886-008-9510-7
Weisskopf, L., Schulz, S., Garbeva, P. (2021). Microbial volatile organic compounds in intra-kingdom and inter-kingdom interactions. Nat. Rev. Microbiol. 19, 391–404. doi: 10.1038/s41579-020-00508-1
Wyatt, T. D. (2018). Queen pheromones, colony odors, and better science: a comment on Holman. Behav. Ecol. doi: 10.1093/beheco/ary074
Xing, Z., Liu, Y., Cai, W. (2017). Efficiency of trichome-based plant defense in phaseolus vulgaris depends on insect behavior, plant ontogeny, and structure. Front. Plant Sci. 7. doi: 10.3389/fpls.2017.02006
Yi, H. S., Heil, M., Álvarez, R. M., Ballhorn, D. J., Ryu, C. H. M. (2009). Airborne induction and priming of plant defenses against a bacterial pathogen. Plant Physiol. 151, 2152. doi: 10.1104/pp.109.144782
Yigezu, Y. A., El-Shater, T., Boughlala, M. (2019). Legume-based rotations have clear economic advantages over cereal monocropping in dry areas. Agron. Sustain. Dev. 39, 58. doi: 10.1007/s13593-019-0602-2
Zeringue, H. J., Brown, R. L., Neucere, J. N., Cleveland, T. E. (1996). Relation-ships between C6–C12 alkanal and alkenal volatile contents and resistance ofmaize genotypes to Aspergillus flavus and aflatoxin production. J. Agric. Food Chem. 44, 403–407.
Zhang, C., Chen, T., Chen, W., Sankaran, S. (2023). Non-invasive evaluation of Ascochyta blight disease severity in chickpea using field asymmetric ion mobility spectrometry and hyperspectral imaging techniques. Crop Prot. 165, 106163. doi: 10.1016/j.cropro.2022.106163
Zhao, Y. X., Kang, L. (2002). The role of plant odours in the leafminer Liriomyza sativae (Diptera: Agromyzidae) and its parasitoid Diglyphus isaea (Hymenoptera: Eulophidae): Orientation towards the host habitat. Eur. J. Entomol. 99, 445–450. doi: 10.14411/eje.2002.056
Keywords: food legumes, volatile organic compounds, microbial VOCs, pest and disease management, cropping systems, crop breeding, pest and disease resistance
Citation: Makhlouf L, El Fakhouri K, Kemal SA, Maafa I, Meftah Kadmiri I and El Bouhssini M (2024) Potential of volatile organic compounds in the management of insect pests and diseases of food legumes: a comprehensive review. Front. Plant Sci. 15:1430863. doi: 10.3389/fpls.2024.1430863
Received: 10 May 2024; Accepted: 04 September 2024;
Published: 04 October 2024.
Edited by:
Nieves Goicoechea, University of Navarra, SpainReviewed by:
Kaori Shiojiri, Ryukoku University, JapanKailash Chand Kumawat, Sam Higginbottom University of Agriculture, Technology and Sciences, India
Copyright © 2024 Makhlouf, El Fakhouri, Kemal, Maafa, Meftah Kadmiri and El Bouhssini. This is an open-access article distributed under the terms of the Creative Commons Attribution License (CC BY). The use, distribution or reproduction in other forums is permitted, provided the original author(s) and the copyright owner(s) are credited and that the original publication in this journal is cited, in accordance with accepted academic practice. No use, distribution or reproduction is permitted which does not comply with these terms.
*Correspondence: Leila Makhlouf, TGVpbGEubWFraGxvdWZAdW02cC5tYQ==