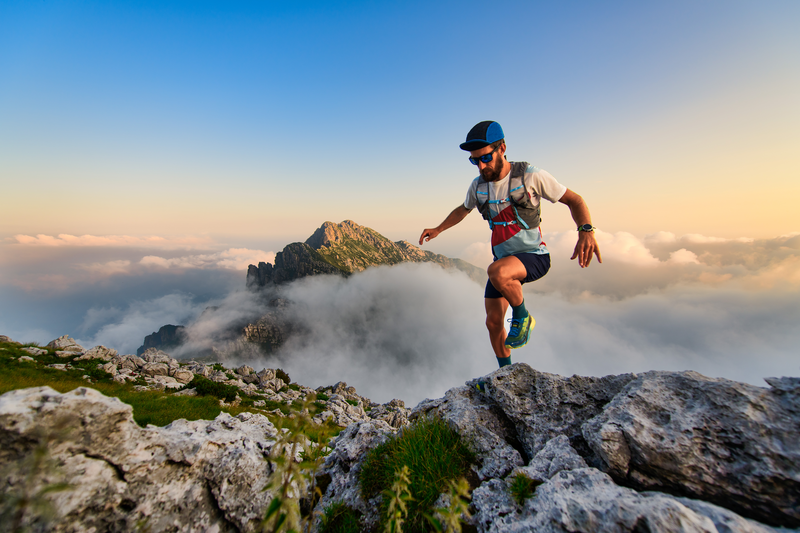
95% of researchers rate our articles as excellent or good
Learn more about the work of our research integrity team to safeguard the quality of each article we publish.
Find out more
ORIGINAL RESEARCH article
Front. Plant Sci. , 04 July 2024
Sec. Crop and Product Physiology
Volume 15 - 2024 | https://doi.org/10.3389/fpls.2024.1424665
Introduction: Indian senna (Senna alexandrina Mill.) (formerly Cassia angustifolia Vahl.) is an important medicinal plant of the family Fabaceae. The leaves and pods of Indian senna yield sennosides and rhein-based laxative. Adulteration of Indian senna is a serious issue as with most of the medicinal plants used in the Indian systems of traditional medicine. The bulk of dried leaves and pods of morphologically related species, such as Cassia fistula, Senna occidentalis, Senna sophera, and Senna tora, is usually mixed with those of the Indian senna, and the admixture is used in laxative-based formulations. The present investigation is a modest attempt at developing species-specific start codon targeted (SCoT) polymorphism- and CAAT-box-derived polymorphism (CBDP)-based sequence-characterized amplified region (SCAR) markers for the identification and authentication of Indian senna and four adulterant species (C. fistula, S. occidentalis, S. sophera, and S. tora species).
Methods: In this study, genomic DNA extracted from 44 accessions of Indian senna and four adulterant species was subjected to SCoT and CBDP PCR. The polymorphic amplicons were identified, eluted, ligated, and transformed into Escherichia coli DH5 α strain. PCR, restriction analysis, and DNA sequencing confirmed the transformed recombinant plasmid clones.
Results: Post-sequencing, the sequence of the primary SCoT and CBDP primers was analyzed and extended into the unique signature sequence of the concerned accessions. This resulted in development of one SCoT-44- and two CBDP-25-based SCARs. SCoT-44 SCAR produced a signature amplicon of 287 bp for accession DCA120, and CBDP-25 SCAR yielded signature amplicons of 575 and 345 bp for accessions DCA13 and DCA119, respectively. The developed SCAR markers were validated across 48 samples (44 accessions of Indian senna and 4 adulterant species) and produced distinct amplicons in Indian senna only, while no such amplicon was observed in the other four adulterant species.
Discussion: The information generated using these markers have been faithfully converted to single-locus, unequivocal, highly reproducible, and informative sequence-based SCAR markers. These markers will enable discrimination of individual plants on the basis of unique sequence-specific amplicons, which could be used as diagnostic markers to settle issues pertaining to the true identity of Indian senna.
Senna alexandrina Mill. (commonly known as Indian senna), an important member of the family Fabaceae (sub-family Caesalpiniaceae), is a major natural laxative-yielding medicinal plant (Reddy et al., 2015). A native to Egypt, Sudan, Nigeria, North Africa, India, Pakistan, China, and Sinai, the Indian senna is an erect perennial subshrub bearing pinnately compound leaves with lanceolate, glabrous green leaflets. The stem bears drooping branches with racemose inflorescence. The plant abounds in more than 28 bioactive compounds. The leaves and pods are the economically important parts of Indian senna and are a good source of anthraquinone-based sennosides A, B, C, and D and rhein. Sennosides, largely found in leaves (2%–3%) and pods (3%–4%) of Indian senna, are diglucosides of sennidins (Chadha and Gupta, 1995). Additionally, the roots contain rhein, chrysophanol, emodin, and aloe–emodin (Ramchander et al., 2017).
Adulteration and substitution are issues of concern in the herbal industry necessitating authentication and standardization of medicinal plants. Approaches based on powder microscopy, biochemistry, and molecular biology have been used to identify and authenticate Indian senna. Classical light microscopy coupled with scanning electron microscopy, fluorescence microscopy, and chemo profiling were employed toward establishing quality control for adulteration of Indian senna (Sultana, 2012; Shaheen et al., 2019). Authenticity of a 200-year-old “Extractum Sennae” was confirmed by reversed-phase high-performance liquid chromatography (RP-HPLC) and electrospray ionization mass spectrometry (ESI-MSn) (Nesmerak et al., 2020). Identification and authentication of medicinal plants using molecular markers is indispensable as it seeks to provide unmatched identity of the species of interest (Joseph et al., 2014). This is documented by authentication of genuine Indian senna from the adulterant species using OPC-17 and OPC-18 random amplified polymorphic DNA (RAPD) markers (Khan et al., 2011). Patent for sequence-characterized amplified region (SCAR) primer-based on RAPD has been filed for award of the same for authentication of true-to-type Indian senna (https://www.quickcompany.in/patents/scar-primers-and-a-kit-for-the-authentication-of-unani-drug-senna-acutifolia-cassia-angustifolia-from-its#documents). DNA barcoding coupled with high-resolution melting (HRM) curve analysis has been used for undisputed authentication of Indian senna (Mishra et al., 2018).
A PCR-based gene-targeted functional marker, start codon targeted (SCoT) polymorphism employs a single 18-mer-long primer (which behaves both as forward and reverse primer) and is based on short-conserved region flanking the start codon (ATG) in plant genes (Collard and Mackill, 2009). SCoT marker correlates with functional genes and associated characteristics without requiring sequence information (Mulpuri et al., 2013). It generates a better fingerprint than RAPD, inter-simple sequence repeat (ISSR), and other multi-locus markers.
SCoT markers were extrapolated for authentication in rice (Collard and Mackill, 2009); Plantago (Rahimi et al., 2018), cowpea (Igwe et al., 2017), Tritordeum bergrothii L (Cabo et al., 2014), and soybean cultivars (Rayan and Osman, 2019). SCoT markers proved to be superior over RAPD and ISSR in terms of diversity index, marker index, and resolving power. SCoT yielded more polymorphism and scorable amplicons compared to RAPD (Gorji et al., 2011). Further, Amom et al. (2020) have credited SCoT with more information and higher discriminating power than RAPD and ISSR.
Another functional molecular marker, CAAT-box-derived polymorphism (CBDP), based on polymorphism due to the promoter region of genes, utilizing primers designed from promoter consensus CAAT-box region (Singh et al., 2014), has been employed in the present study to authenticate Indian senna. The CAAT-box is an essential motif in transcription and has a unique conserved nucleotide pattern with the consensus sequence GGCCAATCT. It is roughly 80 bp upstream of the start codon of eukaryotic genes. CBDP markers have been used for identification of genetic diversity in several crops such as cotton and linseed cultivars (Singh et al., 2014), jojoba genotypes (Heikrujam et al., 2015), and Andrographis paniculata (Tiwari et al., 2016). Studies that employed both SCoT and CBDP markers include examination of the genetic diversity present in various Aegilops species (Pour-Aboughadareh et al., 2019) and fidelity of the clones produced by micro-propagation of Brassica racemosa (Sharma et al., 2019).
SCAR is a polymorphic region of a known sequence, which is invariably an extension of sequence of the primary marker system. Initially, SCAR was developed for isolating downy mildew resistance genes in lettuce (Paran and Michelmore, 1993). These are mono-locus, usually co-dominant PCR-based markers that require two sequence-specific primers. SCAR may be developed from RAPD (Paran and Michelmore, 1993), amplified fragment length polymorphism (AFLP) (Liang et al., 2011), ISSR (Ghosh et al., 2011), and SCoT (Mulpuri et al., 2013). Hence, the results with these markers are more reliable and reproducible. SCoT based SCAR makers were developed to distinguish toxic and non-toxic accessions of Jatropha curcas L (Mulpuri et al., 2013). and authenticate Taxus media (Hao et al., 2018) and Physalis (Solanaceae) species (Feng et al., 2018).
With this background, the objective of the study was to develop reliable SCoT- and CBDP-based SCAR markers for the authentication of Indian senna.
The panel of plant material used in the study included 48 samples comprising 44 accessions of Indian senna (kindly provided by RNR, ICAR-DMAPR, Anand, Gujarat, India) and 4 adulterant species (C. fistula, S. occidentalis, S. sophera, and S. tora) (Table 1). Fresh young leaves from germinated seedlings of Indian senna and four adulterant Senna species were used for DNA isolation using the CTAB method (Doyle, 1991) with modification. The quality and integrity of the isolated genomic DNA was checked on 1% agarose (w/v) (Hi media MB grade) gel electrophoresis and documented by comparing it to the fluorescence yield of the standards—uncut, λ DNA (50 and 100 ng). DNA samples were appropriately diluted to 50 ng/µl in TE buffer and used for PCR amplification.
Table 1 Voucher number information of 44 accessions of Indian senna and 4 adulterant species used in the development of SCoT and CBDP based SCARs.
Based on the available primer sequences in the public domain, 16 SCoT and 25 CBDP (Singh et al., 2014) primers were custom synthesized from BioServe Biotechnologies (India) Pvt. Ltd. The primers that yielded robust SCoT and CBDP profiles were then selected, and the entire panel of 48 DNA samples (44 accessions of Indian senna + 4 adulterant species) (Table 1) were then subjected to SCoT-PCR and CBDP-PCR.
The PCR reaction was carried out in 15-µl reaction volume containing 1× PCR buffer, 50 ng of genomic DNA as template, 1.5 mM of MgCl2, 160 µM of dNTPs, 1.0 µM of SCoT and CBDP primers, and 0.5 U of Taq DNA polymerase. PCR amplifications were performed with the initial denaturation at 94°C for 4 min followed by 45 cycles of denaturation at 94°C for 1 min, annealing at 50°C for 1 min, and extension at 72°C for 2 min with a final extension at 72°C for 10 min. The PCR products were separated by electrophoresis in 3.5% (w/v) agarose gel for 2–3 h at 100 V, and 100 bp was loaded as the standard-size ladder and profiled using a gel documentation system.
SCoT-44 (5′-ACGACATGGCGACCCACA-3′) and CBDP-25 primers (5′-CTGAGCACGATCCAATGT-3′) were subjected to PCR for cloning (Tables 2 and 3). A 3.5% (w/v) agarose gel was run for 2–3 h at 100 V after PCR, and the desired amplicons were cut for further elution.
The DNA from the respective SCoT-44 and CBDP-25 gel profiles were eluted by following the manufacturer’s instructions as given in the QIAgen Gel Extraction kit. The eluted DNA was then subjected to electrophoresis on 1.2% (w/v) agarose gel to know the integrity and quantity of the eluted DNA. The eluted polymorphic amplicons were subjected to T/A cloning. The pGEM®-T Easy Vector (Promega, USA) has been used for cloning the eluted polymorphic fragments. The ligated product was transformed in competent (CaCl2 treated) E. coli DH5α following the heat-shock method. Blue-white screening was undertaken to select the recombinant from the non-recombinant colonies. Positive clones of Indian senna accessions were identified using PCR with M13 forward and reverse primers and restriction digestion of recombinant clones using Not I. The positive clones were then sequenced.
The online site for basic local alignment search tool (BLAST) (https://blast.ncbi.nlm.nih.gov/Blast.cgi) was searched for exploring similarity of the obtained sequences with any reported sequences. Separation of the vector sequences were undertaken, and the trimmed sequences were subjected to BLAST analysis.
The trimmed sequences were then fed to online primer design software, OligoCalc (Kibbe, 2007). A primer pair (SCAR forward and reverse) was then designed for each of the sequences by extending the length of the original primer of the primary marker systems (SCoT and CBDP) into the sequence of the accession of interest.
For validation of the designed SCoT–SCAR and CBDP–SCAR, PCR was carried out in a total volume of 15 µl, which included 1× PCR buffer with 1.5 mM of MgCl2, 100 µM of dNTPs, 1 µM of forward primer, 1 µM of reverse primer, 50 ng of DNA, 0.5 U of Taq DNA polymerase, and MilliQ H2O to make up the reaction volume. The amplification was performed by following a PCR thermal profile: 94°C for 3 min followed by 35 cycles of 94°C for 1 min, annealing (SCoT PCR: 64°C; CBDP PCR: 64.5°C) for 45 s, 72°C for 45 s, and a final extension at 72°C for 10 min. The SCAR markers were validated across 44 accessions and 4 adulterant species. PCR products were separated in 2% (w/v) agarose gel and electrophoresed for 1–2 h at 100 V and documented using gel documentation system.
The genomic DNA extracted from all 48 samples underwent initial primer screening. From a pool of 16 SCoT and 25 CBDP primers exhibiting distinct and consistent polymorphism, one SCoT-44 and one CBDP-25 primer were chosen for subsequent analysis across all samples. SCoT-44 and CBDP-25 primers (Table 4) were selected for PCR amplification of 48 samples. The criterion for choosing SCoT-44 and CBDP-25 primers out of 16 SCoT and 25 CBDP primers was that not all primers yielded robust banding pattern across all the 48 samples tested. Only those primers that gave at least one band across all the 48 samples were chosen, and thus, SCoT-44 and CBDP-25 primers were selected. The amplified loci were between 100 and 3,000 bp in size. SCoT-44 (Figures 1A–D) yielded polymorphic amplicons with DCA60 (Figures 1A, B), and DCA120 (Figure 1C) and CBDP-25 (Figures 2A–D) gave polymorphic amplicons with accessions DCA13 (Figure 2A) and DCA119 (Figure 2C). SCoT-44 and CAAT-25 primers generated amplicons that were chosen as potential Indian senna-specific markers.
Figure 1 PCR profile of 48 samples with SCoT-44 primer. Lane M—100-bp DNA ladder. (A) SCoT PCR of four adulterant species (Cassia fistula (F), Senna occidentalis (O), Senna sophera (S), and Senna tora (T)) and accessions of Indian senna (DCA9 to DCA56); (B) (DCA60 to DCA109) accessions; (C) (DCA112 to DCA130) accessions; (D) (DCA131 to DCA156) accessions of Indian senna. Polymorphic amplicons were obtained in DCA60 and DCA120 accessions of Indian senna. Arrows represent polymorphic amplicons in DCA60 and DCA120 accessions of Indian senna.
Figure 2 PCR profile of 48 samples with CBDP-25 primer. Lane M—100-bp DNA ladder. (A) CBDP PCR of four adulterant species (Cassia fistula (F), Senna occidentalis (O), Senna sophera (S), and Senna tora (T)) and accessions of Indian senna (DCA9 to DCA56); (B) (DCA60 to DCA109) accessions; (C) (DCA112 to DCA127) accessions; (D) (DCA128 to DCA156) accessions of Indian senna. Polymorphic amplicons were obtained in DCA13 and DCA119 accessions of Indian senna. Arrows represent polymorphic amplicons in DCA13 and DCA119 accessions of Indian senna.
Identified polymorphic amplicons were cut out from the gel and eluted using the Qiagen Gel Extraction kit. The amplicons were then cloned in Promega pGEM-T Easy vector. The positive clones were identified initially by blue-white screening. Plasmid DNA from the putative transformed recombinant clones was isolated and subjected to PCR with M13 forward and reverse primers (Figures 3A, B) and restriction digestion (Figure 4) using Not I. Restriction of the putative recombinant clones with Not I adds 22 bp to the size of the amplicon. One positive recombinant clone of SCoT-44 (DCA 120) and two clones of CBDP-25 (DCA13; DCA119) were then sequenced. Post-sequencing, the sequences were subjected to BLAST analysis. No significant similarities were found with any other sequences in the GenBank database. These sequences are unique to the Indian senna species and did not give any significant hit to any known sequences in the public domain. With SCoT-44, a signature amplicon of 287 bp was obtained, which is species specific for DCA120 (Figure 5) and other Indian senna accessions. With CBDP-25, signature amplicons of approximately 575 and 345 bp were obtained, which are species specific for DCA13 (Figure 6) and DCA119 (Figure 7), respectively, and other Indian senna accessions.
Figure 3 Confirmation of cloning of polymorphic amplicons obtained from SCoT-44 and CBDP-25 by PCR with M13 forward and reverse primers. (A) Recombinant clones (W1–W3); non-recombinant clone (B1) of DCA120; Lane M—100-bp DNA ladder; (B) Recombinant clones (W1–W3); non-recombinant clone (B1) of DCA119; recombinant clones (W1–W4); non-recombinant clone (B1) of DCA13.
Figure 4 Restriction analysis of recombinant clones of SCoT-44 (DCA120 accession of Indian senna) and CBDP-25 (DCA13 and DCA119 accessions of Indian senna) using Not I; Lane M—100-bp DNA ladder; C, cut/restricted; UC, uncut/unrestricted.
Figure 5 Nucleotide sequence (287 bases) (GenBank accession No. OR060948) of SCoT-44 SCAR specific for Indian senna accession DCA120. The underlined sequences indicate newly developed SCoT-44 based SCAR forward and reverse primers (CA120SSF2/CA120SSR2).
Figure 6 Nucleotide sequence (575 bases) (GenBank accession No. OR060949) of CBDP-25 SCAR for DCA13. The underlined sequences indicate newly developed CBDP-25 based SCAR forward and reverse primers (CA13CSF1/CA13CSR1).
Figure 7 Nucleotide sequence (345 bases) (GenBank accession No. OR060950) of CBDP-25 SCAR specific for DCA119. The underlined sequences indicate newly developed CBDP-25 based SCAR forward and reverse primers (CA119CSF2/CA119CSR2).
Primers for SCAR (both SCoT and CBDP based) markers were designed in line with the standard conventions of primer design such as ensuring maintenance of GC content of the primers at 50% and then further ensuring that the primers end either with a G or C. The SCAR sequences specific for the accessions of Indian senna were deposited in GenBank, and accession numbers were obtained for these SCoT- and CBDP-based SCARs. Based on these sequences, SCoT–SCAR (Figure 5, GenBank accession no. OR060948) and CBDP–SCARs (Figure 6, GenBank accession no. OR060949 and Figure 7 GenBank accession no. OR060950) primer pairs were designed. Based on these sequences, a SCoT–SCAR primer pair, CA120SSF2 (5′ACGACATGGCGACCCACACCCGGTG3′), CA120SSR2 (3′ACGACATGGCGACCCACAATGGAACTGGG5′) and two CBDP–SCAR primer pairs were designed: CA13CSF1 (CTGAGCACGATCCAATGTATGCTTACAAGTC), CA13CSR1 (3′CTGAGCACGATCCAATGTTCAGCACACAATCC5′) and CA119CSF2 (5′CTGAGCACGATCCAATGTTCAGCACACAATCC3′), CA119CSF2 (3′CTGAGCACGATCCATAGTATATAGCTATGGAATTGTCC5′), respectively (Table 5).
All 44 samples of Indian senna and its 4 adulterant species were amplified using the developed SCAR primers. SCAR was standardized at an annealing temperature of 64°C in the case of the SCoT-44 SCAR marker (Figure 8). In the case of the CBDP-25 SCAR marker, annealing temperature of 64.5°C was standardized for DCA13 (Figure 9) and DCA119 (Figure 10). All the developed and validated SCoT- and CBDP-based SCAR markers are specific to the accessions of Indian senna but not to the other four species (Table 5).
Figure 8 Validation of the newly designed SCoT-44 SCAR across panel of DCA120 and other Indian senna accessions along with the four adulterant species. Lane M—100-bp DNA ladder. (A) DCA120, Cassia fistula (F), Senna occidentalis (O), Senna sophera (S), Senna tora (T), DCA09–DCA42 accessions; (B) DCA45–DCA96; (C) DCA97–DCA117; (D) DCA119–DCA156.
Figure 9 Validation of the newly designed CBDP-25 SCAR across panel of DCA13 and other accessions of Indian senna along with the four adulterant species. Lane M—100-bp DNA ladder. (A) DCA13, Cassia fistula (F), Senna occidentalis (O), Senna sophera (S), Senna tora (T), DCA09–DCA60 accessions; (B) DCA70–DCA109; (C) DCA112–DCA142; (D) DCA150–DCA156.
Figure 10 Validation of the newly designed CBDP-25 SCAR across panel of DCA119 and other Indian senna accessions and the four adulterant species. Lane M - 100-bp DNA ladder. (A) DCA119, Cassia fistula (F), Senna occidentalis (O), Senna sophera (S), Senna tora (T), DCA09–DCA52; (B) DCA56–DCA107; (C) DCA108–DCA156.
Authentication of true-to-type medicinal plants is the imminent need of the hour to preserve the uniqueness and individuality of the plant under question. This exercise of authentication has been fairly facilitated using various techniques such as morphological and biochemical analysis (Bekbolatova et al., 2018; Seethapathy et al., 2018), DNA barcoding (Hebert et al., 2003; Mishra et al., 2016), DNA barcoding coupled with HRM curve analysis (Mishra et al., 2018), and molecular markers (Collard and Mackill, 2009). Approaches, such as powder microscopy, enhance taxonomic recognition of a particular genus by projecting micro morphological and anatomical characters (Nesmerak et al., 2020). But these characters need not be unique and may lead to spurious identification and authentication of a species and thus diminish the very purpose of employing the same. Biochemical approach of identification and authentication of a species is very much influenced by age, physiological condition, and environmental factors (Chan, 2003). DNA-based markers have proven to be the best for deciphering authenticity and genetic diversity among plants as they are highly discriminatory, environmental neutral, are more objective and reliable, and unlimited in number. DNA barcoding in conjunction with HRM curve analysis has been used for undisputed authentication of Indian senna (Mishra et al., 2018). Gene-based markers, such as SCoT, proved to be superior over non-genic markers, such as RAPD and ISSR, in terms of diversity index, marker index, and resolving power. SCoT yielded more polymorphism and scorable amplicons compared to RAPD in tetraploid potato (Gorji et al., 2011). SCoT is highly informative with pronounced discriminating power than RAPD and ISSR as reported for bamboo (Amom et al., 2020).
SCoT and CBDP marker-based studies have been used for the discrimination of genuine and adulterant samples of crop plants. Both SCoT and CBDP markers use of a single primer, which acts as both forward and reverse. Further, the polymorphism, which arises based on their application, is essentially either in the coding region (SCoT) or regulatory region such as a promoter (CBDP). The polymorphic amplicons act as markers and have a pivotal role in species authentication. However, the use of a single primer in SCoT and CBDP PCR results in a multitude of amplicons across the panel of accessions being investigated, thus generating profiles which could be non-reproducible. This drawback of the non-reproducibility and multi-locus nature of SCoT and CBDP markers is resolved by cloning, sequencing, and extending the length of the initial primer used and thus generating a single-locus sequence-specific signature SCAR. SCAR is a secondary, sequence-specific (either co-dominant or dominant) marker (Paran and Michelmore, 1993).
In the present study, efforts were made to develop SCoT- and CBDP-based SCAR markers for the identification of Indian senna to facilitate its fool-proof authentication. Forty-four accessions of Indian senna and four adulterant species were included in the study. The work reports the development of highly sensitive, effective, and reproducible PCR-based SCoT–SCARs and CBDP–SCARs for identifying true-to-type Indian senna. SCAR markers have been developed for the authentication of apple (De Clercq et al., 2003), papaya (Deputy et al., 2002), rose (Bashir et al., 2014), cowpea (Boukar et al., 2004), strawberry (Rugienius et al., 2006), buffelgrass (Dwivedi et al., 2007), and brassica (Piquemal et al., 2005). SCAR markers have been used for molecular verification of medicinal plant species such as Ashoka (Saraca asoca (Roxb.) de Wild) and its adulterant Polyalthia longifolia Benth (Kumar, 2016), Artemisia, Phyllanthus, Panax (Kiran et al., 2010), and Dimocarpus longan varieties (Yang et al., 2013). Germplasm and cultivar-specific SCoT-based SCAR was identified in Dendrobium officinale (Zheng et al., 2021) and Chrysanthemum morifolium Ramat (Cai et al., 2022). Deng (2021) developed SCoT-based SCAR to detect smut resistance in sugarcane.
Parallel to this, CBDP-based SCAR markers have been developed in the present study. Deciphering the true identity of an individual plant species can be facilitated using CBDP-derived markers as they are species neutral (Singh et al., 2014). Cost effectiveness, high polymorphism, high reproducibility, and detailed genetic information can be made available using the CBDP marker system (Etminan et al., 2018). The present study is the first of its kind to use CBDP-based SCARs for the authentication of Indian senna.
A cloning-based RAPD–SCAR marker was developed by Yang et al. (2013) to distinguish D. longan from Dimocarpus confinis. Cheng et al. (2016) developed a SCAR marker to authenticate litchi species and resolved issues pertaining to naming and identification of several litchi cultivars grown internationally. Jose et al. (2021) developed an ISSR-based SCAR marker toward the identification of cardamom Malabar (prostrate panicle) variety (Elettaria cardamomum L. Maton). Using ISSR, SCoT and CBDP markers, the genetic diversity of Iranian Aegilops triuncialis accessions was evaluated by Khodaee et al. (2021). Singh et al. (2014) validated the utility of the CBDP marker across cultivars of cotton (Gossypium species), jute (Corchorus capsularis and Corchorus olitorius), and linseed (Linum usitatissimum).
All these reported findings further strengthen the application of SCoT and CBDP either singly or in combination with SCAR, which takes it to a further higher level of discriminating the true-to-type species.
The diagnostic markers developed in the present study facilitate identification and authentication of true-to-type Indian senna and intend to unambiguously resolve the issue of poor quality control, and thus help in sustainable exploitation of Indian senna.
Starting from multi-locus, PCR-based markers, such as SCoT and CBDP, single locus, highly reproducible, sequence-specific SCAR markers have been developed in the present study to resolve the identity of true-to-type Indian senna accessions against the four adulterant species. In this study, one SCoT-44 SCAR species-specific primer pair (CA120SSF2/CA120SSR2) and two CBDP-25 SCAR species-specific primer pairs (CA13CSF1/CA13CSR1 and CA119CSF2/CA119CSR2) have been developed for Indian senna. The SCoT- and CBDP-derived SCAR markers developed in the present study can be seen as a translational tool to wedge the distance between the lab and field and facilitate rapid, unequivocal, and effective identification of Indian senna paving way for its conservation and sustainable utilization.
The datasets presented in this study can be found in online repositories. The names of the repository/repositories and accession number(s) can be found below: https://www.ncbi.nlm.nih.gov/genbank/, OR060948; https://www.ncbi.nlm.nih.gov/genbank/, OR060949; https://www.ncbi.nlm.nih.gov/genbank/, OR060950.
SC: Data curation, Formal analysis, Investigation, Methodology, Software, Validation, Visualization, Writing – original draft, Writing – review & editing. MA: Data curation, Formal analysis, Investigation, Methodology, Software, Validation, Visualization, Writing – original draft, Writing – review & editing, Conceptualization, Funding acquisition, Project administration, Resources, Supervision. PK: Data curation, Formal analysis, Investigation, Methodology, Software, Validation, Visualization, Writing – original draft. SF: Data curation, Formal analysis, Investigation, Methodology, Visualization, Writing – original draft. MP: Formal analysis, Resources, Software, Visualization, Writing – original draft. CK: Conceptualization, Formal analysis, Visualization, Writing – review & editing. RNR: Formal analysis, Resources, Visualization, Writing – original draft.
The author(s) declare financial support was received for the research, authorship, and/or publication of this article. MA acknowledges the financial support extended by UGC under UGC Start-Up Research Grant (SRG) (No. F.30–520/2020(BSR)). The work reported in this manuscript is a part of the sanctioned UGC SRG.
Thanks to RNR, ICAR-DMAPR for kindly providing accessions of Indian senna as reported in this study.
The authors declare that the research was conducted in the absence of any commercial or financial relationships that could be construed as a potential conflict of interest.
All claims expressed in this article are solely those of the authors and do not necessarily represent those of their affiliated organizations, or those of the publisher, the editors and the reviewers. Any product that may be evaluated in this article, or claim that may be made by its manufacturer, is not guaranteed or endorsed by the publisher.
RAPD, random amplified polymorphic DNA; AFLP, amplified fragment length polymorphism; CBDP, CAAT-box-derived polymorphism; HRM, high-resolution melting; ISSR, inter-simple sequence repeat; SCAR, sequence-characterized amplified region; SCoT, start codon targeted polymorphism.
Amom, T., Tikendra, L., Apana, N., Goutam, M., Sonia, P., Koijam, A. S., et al. (2020). Efficiency of RAPD, ISSR, iPBS, SCoT and phytochemical markers in the genetic relationship study of five native and economical important bamboos of North-East India. Phytochemistry 174, 112330. doi: 10.1016/j.phytochem.2020.112330
Bashir, K. M. I., Awan, F. S., Khan, I. A., Khan, A. I., Usman, M. (2014). Identification and authentication of Rosa species through development of species-specific SCAR marker(s). Genet. Mol. Res. 13, 4130–4139. doi: 10.4238/2014.May.30.8
Bekbolatova, E. N., Kurbatova, N. V., Sakipova, Z. B., Ibragimova, L. N, Alpysbayeva, S. I., Kabdenova, A. T., et al. (2018). Macroscopic and Microscopic Diagnostic Features of the potential Herbal Drug Crataegus almaatensis Pojark Endemic in Kazakhstan: potential Herbal Drug Crataegus Almaatensis Pojark Endemic. Iranian J. Pharm. Sci. 14, 39–50. doi: 10.22037/ijps.v14.40659
Boukar, O., Kong, L., Singh, B.B., Murdock, L., Ohm, H.W. (2004). AFLP and AFLP-derived SCAR markers associated with Striga gesnerioides resistance in cowpea. Crop Sci. 44, 1259–1264. doi: 10.2135/cropsci2004.1259
Cabo, S., Ferreira, L., Carvalho, A., Martins-Lopes, P., Martín, A., Lima-Brito, J. E. (2014). Potential of Start Codon Targeted (SCoT) markers for DNA fingerprinting of newly synthesized tritordeums and their respective parents. J. Appl. Genet. 55, 307–312. doi: 10.1007/s13353-014-0211-3
Cai, Y., Gao, Y., Zhang, Z., Liu, H., Wang, Y., Ma, Y., et al. (2022). ‘Development and application of a cultivar-specific sequence-characterized amplified region (SCAR) marker for the detection of chrysanthemum morifolium ramat. “Daboju”’. Plants 11, 604. doi: 10.3390/plants11050604
Chadha, K. L., Gupta, R. (1995). Advances in horticulture. Vol. 11, Medicinal and aromatic plants (New Delhi: Malhotra Publishing House).
Chan, K. (2003). Some aspects of toxic contaminants in herbal medicines. Chemosphere 52, 1361–1371. doi: 10.1016/S0045-6535(03)00471-5
Cheng, J. L., Li, J., Qiu, Y. M., Wei, C. L., Yang, L. Q., Fu, J. J. (2016). Development of novel SCAR markers for genetic characterization of Lonicera japonica from high GC-RAMP-PCR and DNA cloning. Genet. Mol. Res. 15 (2). doi: 10.4238/gmr.15027737
Collard, B. C. Y., Mackill, D. J. (2009). Start codon targeted (SCoT) polymorphism: A simple, novel DNA marker technique for generating gene-targeted markers in plants. Plant Mol. Biol. Rep. 27, 86–93. doi: 10.1007/s11105-008-0060-5
De Clercq, D., Cognet, S., Pujol, M., Lepoivre, P., Jijakli, M.H. (2003). Development of a SCAR marker and a semi-selective medium for specific quantification of Pichia anomala strain K on apple fruit surfaces’. Postharvest Biol. Technol. 29, 237–247. doi: 10.1016/S0925-5214(03)00044-9
Deng, Q. (2021). Development of a SCAR marker based on SCoT polymorphisms for sugarcane smut resistance. Int. J. Agric. Biol. 25, 1272–1280. doi: 10.17957/IJAB/15.1789
Deputy, J. C., Ming, R., Ma, H., Liu, Z., Fitch, M. M. M., Wang, M., et al. (2002). Molecular markers for sex determination in papaya (Carica papaya L.). Theor. Appl. Genet. 106, 107–111. doi: 10.1007/s00122-002-0995-0
Doyle, J. (1991). DNA protocols for plants. Mol. techniques taxonomy 57, 283–293. doi: 10.1007/978-3-642-83962-7_18
Dwivedi, K., Bhat, S., Bhat, V., Bhat, B., Gupta, M. G. (2007). Identification of a SCAR marker linked to apomixis in buffelgrass (Cenchrus ciliaris L.). Plant Sci. 172, 788–795. doi: 10.1016/j.plantsci.2006.12.006
Etminan, A., Pour-Aboughadareh, A., Mohammadi, R., Noori, A., Ahmadi-Rad, A. (2018). Applicability of CAAT box-derived polymorphism (CBDP) markers for analysis of genetic diversity in durum wheat. Cereal Res. Commun. 46, 1–9. doi: 10.1556/0806.45.2017.054
Feng, S., Zhu, Y., Yu, C., Jiao, K., Jiang, M., Lu, J., et al. (2018). Development of species-specific SCAR markers, based on a SCoT analysis, to authenticate physalis (Solanaceae) species. Front. Genet. 9. doi: 10.3389/fgene.2018.00192
Ghosh, M., Chezhian, P., Sumathi, R., Yasodha, R. (2011). Development of SCAR marker in Casuarina equisetifolia for species authentication. Trees 25, 465–472. doi: 10.1007/s00468-010-0522-x
Gorji, A., Poczai, P., Polgar, Z., Taller, J. (2011). Efficiency of arbitrarily amplified dominant markers (SCOT, ISSR and RAPD) for diagnostic fingerprinting in tetraploid potato. Am. J. Potato Res. 88, 226–237. doi: 10.1007/s12230-011-9187-2
Hao, J., Jiao, K., Yu, C., Guo, H., Zhu, Y., Yang, X., et al. (2018). Development of SCoT-based SCAR marker for rapid authentication of Taxus media. Biochem. Genet. 56, 255–266. doi: 10.1007/s10528-018-9842-0
Hebert, P. D., Cywinska, A., Ball, S. L., deWaard, J. R. (2003). ‘Biological identifications through DNA barcodes’, Proceedings of the Royal Society of London. Ser. B: Biol. Sci. 270, 313–321. doi: 10.1098/rspb.2002.2218
Heikrujam, M., Kumar, J., Agrawal, V. (2015). Genetic diversity analysis among male and female Jojoba genotypes employing gene targeted molecular markers, start codon targeted (SCoT) polymorphism and CAAT box-derived polymorphism (CBDP) markers. Meta Gene 5, 90–97. doi: 10.1016/j.mgene.2015.06.001
Igwe, D. O., Afiukwa, C. A., Ubi, B. E., Ogbu, K. I., Ojuederie, O. B., Ude, G. N. (2017). Assessment of genetic diversity in Vigna unguiculata L.(Walp) accessions using inter-simple sequence repeat (ISSR) and start codon targeted (SCoT) polymorphic markers. BMC Genet. 18, 1–13. doi: 10.1186/s12863-017-0567-6
Jose, S., Anisha, C. S., Rao, Y. S. (2021). Development of an ISSR based SCAR marker to identify small cardamom Malabar (prostrate panicle) variety (Elettaria cardamomum Maton.). Scientia Horticulturae 294, 110777. doi: 10.21203/rs.3.rs-168166/v1
Joseph, K. S., Murthy, H. N., Ravishankar, K. V. (2014). Development of SCAR marker for sex identification in dioecious Garcinia gummi-gutta. Trees 28, 1645–1651. doi: 10.1007/s00468-014-1074-2
Khan, S., Mirza, K. J., Al-Qurainy, F., Abdin, M. Z. (2011). Authentication of the medicinal plant Senna angustifolia by RAPD profiling. Saudi J. Biol. Sci. 18, 287–292. doi: 10.1016/j.sjbs.2011.03.001
Khodaee, L., Azizinezhad, R., Etminan, A. R., Khosroshahi, M. (2021). Assessment of genetic diversity among Iranian Aegilops triuncialis accessions using ISSR, SCoT, and CBDP markers. J. Genet. Eng. Biotechnol. 19, 5–13. doi: 10.1186/s43141-020-00107-w
Kibbe, W. A. (2007). OligoCalc: an online oligonucleotide properties calculator. Nucleic Acids Res. 35, W43–W46. doi: 10.1093/nar/gkm234
Kiran, U., Khan, S., Mirza, K. J., Ram, M., Abdin, M. Z. (2010). SCAR markers: A potential tool for authentication of herbal drugs. Fitoterapia 81, 969–976. doi: 10.1016/j.fitote.2010.08.002
Kumar, V. (2016). Rapid molecular authentication of Ashoka [Saraca asoca (Roxb.), de Wild] vulnerable medicinal plant species and its adulterant Polyalthia longifolia Benth, by the development of SCAR markers and multiplex-PCR. Res. J. Biotechnol. 10 (11), 59–68.
Liang, W., Yu, J., Li, J., Wu, H., Wu, X. (2011). Development of a species-specific AFLP-based SCAR marker for authentication of black muntjac (Muntiacus crinifrons). Mol. Ecol. Resour. 11, 162–165. doi: 10.1111/j.1755-0998.2010.02872.x
Mishra, P., Kumar, A., Rodrigues, V., Shukla, A. K., Sundaresan, V. (2016). Feasibility of nuclear ribosomal region ITS1 over ITS2 in barcoding taxonomically challenging genera of subtribe Cassiinae (Fabaceae). PeerJ 4, e2638. doi: 10.7717/peerj.2638
Mishra, P., Shukla, A. K., Sundaresan, V. (2018). Candidate DNA barcode tags combined with high resolution melting (Bar-HRM) curve analysis for authentication of senna alexandrina mill. With validation in crude drugs. Front. Plant Sci. 9. doi: 10.3389/fpls.2018.00283
Mulpuri, S., Muddanuru, T., Francis, G. (2013). Start codon targeted (SCoT) polymorphism in toxic and non-toxic accessions of Jatropha curcas L. and development of a codominant SCAR marker. Plant Sci. 207, 117–127. doi: 10.1016/j.plantsci.2013.02.013
Nesmerak, K., Kudlacek, K., Čambal, P., Sticha, M., Kozlík, P., Červený, V (2020). Authentication of senna extract from the eighteenth century and study of its composition by HPLC–MS. Monatshefte für Chemie - Chem. Monthly 151, 1241–1248. doi: 10.1007/s00706–020-02630–5
Paran, I., Michelmore, R. W. (1993). Development of reliable PCR-based markers linked to downy mildew resistance genes in lettuce. TAG. Theor. Appl. Genet. Theoretische und angewandte Genetik 85, 985–993. doi: 10.1007/BF00215038
Piquemal, J., Cinquin, E., Couton, F., Rondeau, C., Seignoret, E., Doucet, I., et al. (2005). Construction of an oilseed rape (Brassica napus L.) genetic map with SSR markers. Theor. Appl. Genet. 111, 1514–1523. doi: 10.1007/s00122-005-0080-6
Pour-Aboughadareh, A., Etminan, A., Shooshtari, L. (2019). Comparative assessment of SCoT and CBDP markers for investigation of genetic diversity existing in different Aegilops species. Agric. Biotechnol. J. 11 (4), 153–174. https://sid.ir/paper/224546/en
Rahimi, M., Nazari, L., Kordrostami, M., Safari, P. (2018). SCoT marker diversity among Iranian Plantago ecotypes and their possible association with agronomic traits. Scientia Hortic. 233, 302–309. doi: 10.1016/j.scienta.2018.01.009
Ramchander, Jalwal, P., Middha, A. (2017). ‘Recent advances on senna as a laxative: A comprehensive review’. J. Pharmacognosy Phytochem. 6, 349–353.
Rayan, W. A., Osman, S. A. (2019). Phylogenetic relationships of some Egyptian soybean cultivars (Glycine max L.) using SCoT marker and protein pattern. Bull. Natl. Res. Centre 43, 161–170. doi: 10.1186/s42269–019-0197–4
Reddy, N. R. R., Mehta, R. H., Soni, P. H., Makasana, J., Gajbhiye, N. A., Ponnuchamy, M., et al. (2015). Next generation sequencing and transcriptome analysis predicts biosynthetic pathway of sennosides from senna (Cassia angustifolia vahl.), a non-model plant with potent laxative properties. PloS One 10, e0129422. doi: 10.1371/journal.pone.0129422
Rugienius, R., Siksnianas, T., Stanys, V., Bendokas, V. (2006). Use of RAPD and SCAR markers for identification of strawberry genotypes carrying red stele (Phytophtora fragariae) resistance gene Rpf1. Agron. Res. 4, 335–339.
Seethapathy, G. S., Tadesse, M., Urumarudappa, S. K. J., Gunaga, S. V., Vasudeva, R, Malterud, K. E., et al. (2018). Authentication of Garcinia fruits and food supplements using DNA barcoding and NMR spectroscopy. Sci. Rep. 8, 10561. doi: 10.1038/s41598-018-28635-z
Shaheen, S., Jaffer, M., Khalid, S., Khan, M. A., Hussain, K., Butt, M. M., et al. (2019). Microscopic techniques used for the identification of medicinal plants: A case study of Senna. Microscopy Res. Technique 82, 1660–1667. doi: 10.1002/jemt.23332
Sharma, U., Rai, M. K., Shekhawat, N. S., Kataria, V. (2019). Genetic homogeneity revealed in micropropagated Bauhinia racemosa Lam. using gene targeted markers CBDP and SCoT. Physiol. Mol. Biol. Plants 25, 581–588. doi: 10.1007/s12298-018-00639-z
Singh, A., Rana, M. K., Singh, S., Kumar, S., Kumar and Singh, R. R., et al. (2014). CAAT box- derived polymorphism (CBDP): A novel promoter -targeted molecular marker for plants. J. Plant Biochem. Biotechnol. 23, 175–183. doi: 10.1007/s13562-013-0199-5
Sultana, S. (2012). Authentication of herbal drug Senna (Cassia angustifolia Vahl.): A village pharmacy for Indo-Pak Subcontinent. Afr. J. Pharm. Pharmacol. 6 (30), 2299–2308. doi: 10.5897/AJPP12.446
Tiwari, G., Singh, R., Singh, N., Choudhury, D. R., Paliwal, R., Kumar, A., et al. (2016). ‘Study of arbitrarily amplified (RAPD and ISSR) and gene targeted (SCoT and CBDP) markers for genetic diversity and population structure in Kalmegh [Andrographis paniculata (Burm. f.) Nees]’. Ind. Crops Products 86, 1–11. doi: 10.1016/j.indcrop.2016.03.031
Yang, L., Fu, S., Khan, M. A., Zeng, W., Fu, J. (2013). Molecular cloning and development of RAPD-SCAR markers for Dimocarpus longan variety authentication. SpringerPlus 2, 501. doi: 10.1186/2193-1801-2-501
Keywords: Senna alexandrina Mill., Indian senna, SCOT, CBDP, SCAR, adulterant species
Citation: Chouksey S, Ashfaq MA, Kaira P, Farhat S, Pandey M, Kumar CA and Nagaraja Reddy RR (2024) Development of highly discriminatory SCoT- and CBDP-based SCAR fingerprint for authentication of Indian senna (Senna alexandrina Mill.) formerly Cassia angustifolia Vahl.). Front. Plant Sci. 15:1424665. doi: 10.3389/fpls.2024.1424665
Received: 28 April 2024; Accepted: 10 June 2024;
Published: 04 July 2024.
Edited by:
Ravi Gupta, Kookmin University, Republic of KoreaReviewed by:
Passoupathy Rajendrakumar, ICAR-Indian Institute of Millets Research (IIMR), IndiaCopyright © 2024 Chouksey, Ashfaq, Kaira, Farhat, Pandey, Kumar and Nagaraja Reddy. This is an open-access article distributed under the terms of the Creative Commons Attribution License (CC BY). The use, distribution or reproduction in other forums is permitted, provided the original author(s) and the copyright owner(s) are credited and that the original publication in this journal is cited, in accordance with accepted academic practice. No use, distribution or reproduction is permitted which does not comply with these terms.
*Correspondence: Mohd Ashraf Ashfaq, YXNocmFmQGphbWlhaGFtZGFyZC5hYy5pbg==
Disclaimer: All claims expressed in this article are solely those of the authors and do not necessarily represent those of their affiliated organizations, or those of the publisher, the editors and the reviewers. Any product that may be evaluated in this article or claim that may be made by its manufacturer is not guaranteed or endorsed by the publisher.
Research integrity at Frontiers
Learn more about the work of our research integrity team to safeguard the quality of each article we publish.