- 1College of Agriculture, Tarim University, Alar, China
- 2Key Laboratory of Tarim Oasis Agriculture, Ministry of Education, Tarim University, Alar, China
Global warming causes heat and drought stress in plants, which affects crop production. In addition to osmotic stress and protein inactivation, reactive oxygen species (ROS) overaccumulation under heat and drought stress is a secondary stress that further impairs plant performance. Chloroplasts, mitochondria, peroxisomes, and apoplasts are the main ROS generation sites in heat- and drought-stressed plants. In this review, we summarize ROS generation and scavenging in heat- and drought-stressed plants and highlight the potential applications of plant nanobiotechnology for enhancing plant tolerance to these stresses.
1 Introduction
Water scarcity is a constraint on agriculture, and global warming is exacerbating the problem. Drought caused by high temperature has become one of the major abiotic stresses threatening crop yield globally (Lippmann et al., 2019; Dietz et al., 2021). The United Nations report shows, the global surface temperature over 2011–2020 was about 1.1°C higher than that over 1850–1900, and the land surface temperature was 0.71°C higher than the sea surface temperature (Calvin et al., 2023).According to a report released by the Food and Agriculture Organization of the United Nations (FAO) and World Meteorological Organization (WMO), the number of people threatened by food security worldwide has increased significantly from 25.3% in 2019 to 29.6% in 2022 (FAO, 2023), and the heat and drought in 2023 have affected the food security of millions of people (Climate change indicators reached record levels in 2023: WMO, n.d). Lifting the constraints imposed on agriculture by high temperatures and drought can further unleash the potential of agricultural production to effectively respond to the food crisis.
Reactive oxygen species (ROS) in plant cells mainly include superoxide anion radical (O2•−), hydrogen peroxide (H2O2), hydroxyl radical (•OH), and singlet oxygen (1O2) (Dat et al., 2000; Halliwell, 2006; Mittler, 2017). Normally, ROS are key signaling molecules in plant cell cycle regulation and programmed death (Gapper and Dolan, 2006; Livanos et al., 2012; Petrov et al., 2015; Qi and Zhang, 2020), and they interact with signaling molecules such as salicylic acid, jasmonic acid, ethylene, and abscisic acid and are the upstream or downstream regulators of many metabolic pathways in plants (Kwak, 2003; Jammes et al., 2009; Denness et al., 2011; Steffens, 2014; Herrera-Vásquez et al., 2015). The excessive accumulation of ROS under high temperature and drought stress can have toxic effects on plants (Suzuki and Mittler, 2006; Djanaguiraman et al., 2018b; Hussain et al., 2019). High levels of ROS can lead to DNA breakage as well as the inactivation of photosystem II (PSII) in the chloroplasts, thereby inhibiting photosynthesis (Kruk et al., 2005). Excessive ROS levels can impair plant growth and development, while maintaining ROS homeostasis is beneficial to plant growth (Dat et al., 2000; Mittler, 2017). The ROS in plant cells are mainly formed in the chloroplasts, mitochondria, peroxisome, and apoplasts. Plants also have antioxidant systems (Mittler et al., 2004; Impa et al., 2012), including both enzymatic and non-enzymatic systems (Hernández et al., 2012; Rajput et al., 2021), that protect cells from the excessive accumulation of ROS. The antioxidant enzyme system includes superoxide dismutase (SOD), peroxidase (POD), catalase (CAT), ascorbate peroxidase (APX), and glutathione peroxidase (GPX) (Alscher et al., 2002; Laxa et al., 2019)
Non-enzymatic systems include small organic molecules with antioxidant capabilities, such as polyphenols, carotenoids, vitamin C, glutathione, melatonin and polyamines (Hernández et al., 2012; Kolupaev et al., 2023). Maintaining ROS homeostasis can improve crop resistance to high temperature and drought stress (Awasthi et al., 2015; Verma et al., 2019).
In recent years, it has been reported that nanoparticles improve plant abiotic stresses tolerance. For example, iron oxide nanoparticles ameliorated the cadmium and salinity stresses in wheat plants (Manzoor et al., 2021). Application of ZnO nanoparticles could alleviate the low temperature damage on the early growth of rice (Mai et al., 2024). Emerging studies focuse on the use of plant nanobiotechnology to regulate plant ROS homeostasis to improve plant resistance to stress (Zhao et al., 2020; Liu et al., 2021a).To date, several nanomaterials, such as CeO2 (Khan et al., 2021), and Mn3O4 (Liu et al., 2023) were reported as an ROS scavenger in plant abiotic stress tolerance. For example, cerium oxide nanoparticles enable plants to withstand drought and saline-alkali stress (Djanaguiraman et al., 2018b; Liu et al., 2021). The high temperature tolerance of plants is improved with nano-selenium treatment (Djanaguiraman et al., 2018a). In addition, some nanomaterials can maintain ROS homeostasis and enhancing plants heat and drought tolerance (Wu et al., 2017).
Nanobiotechnology can effectively maintain ROS homeostasis and improve plant resistance to heat and drought stress. Therefore, in this paper, we presented a overview of how plants generate and scavenge ROS during heat and drought stress. Also show the role of nanobiotechnology in maintaining ROS homeostasis in crops, the underlying potential mechanism in enhancing crop resistance and ensuring sustainable agriculture.
2 Effect of heat and drought stress in plants
Drought and heat stress affect the plant morphology and physiological functions. For instance, both stresses retard the photosynthetic machinery and limit photosynthesis (Carmo-Silva et al., 2012), cause ROS over-accumulation (Das and Roychoudhury, 2014), and hinder plant growth and development (Yadav et al., 2022).
Combined heat and drought exacerbates the adverse effects on plants compared to individual stresses. Drought typically causes stomatal closure (Buckley, 2019), whereas heat stress results in stomatal opening (López et al., 2022). During concurrent heat and drought stress, drought can exacerbate thermal damage during heatwaves (Marchin et al., 2022). Both stresses lead to ROS over-accumulation. For example, it was reported that heat and drought stress cause ROS accumulation and suppress seed germination and growth in rice (Liu et al., 2019). Therefore, maintaining ROS homeostasis is a must to do physiological process in plants to ensure survival. This could be achieved through modulating the activity of ROS scavenging pathways and recruiting antioxidant enzyme activities. However, heat and drought significantly decrease the activities of antioxidant enzymes such as SOD, POD, and CAT (Hu et al., 2023), which is detrimental to maintaining ROS homeostasis in plants. Hence, investigating options to assist plants performance under harsh conditions it is particularly important task to explore.
3 ROS generation in plants
3.1 ROS in the mitochondria
The mitochondria are one of the ROS production sites. In plant cells, most of the ROS produced under light come from the chloroplasts or peroxisomes, but in non-green tissues or darkness, mitochondrial ROS production dominates (Navrot et al., 2007) (Figure 1). This production is an inevitable result of aerobic metabolism, and about 1–5% of the oxygen consumption of plants is due to H2O2 production (Černý et al., 2018). The main site of ROS formation in the mitochondria is the respiratory electron transport chain (RETC), which involves the reduction of oxygen to H2O. Different pathways are involved in this reduction process. Some enzyme systems, such as cytochrome c oxidase (COX), directly reduce oxygen, while others reduce oxygen in steps, i.e., oxygen accepts only one electron, resulting in the formation of O2•−. Nicotinamide adenine dinucleotide (NAD+) is an important coenzyme in RETC in the inner mitochondrial membrane. Also, it is an important electron acceptor in the RETC. NADH, ubiquinone oxidoreductase (Complex I), and the cytochrome C reductase complex (Complex III) is the main site of ROS production, Complex I mainly produces a small amount of O2•−, and Complex III produces O2•− which is then converted to H2O2 (Gakière et al., 2018; Dourmap et al., 2020).
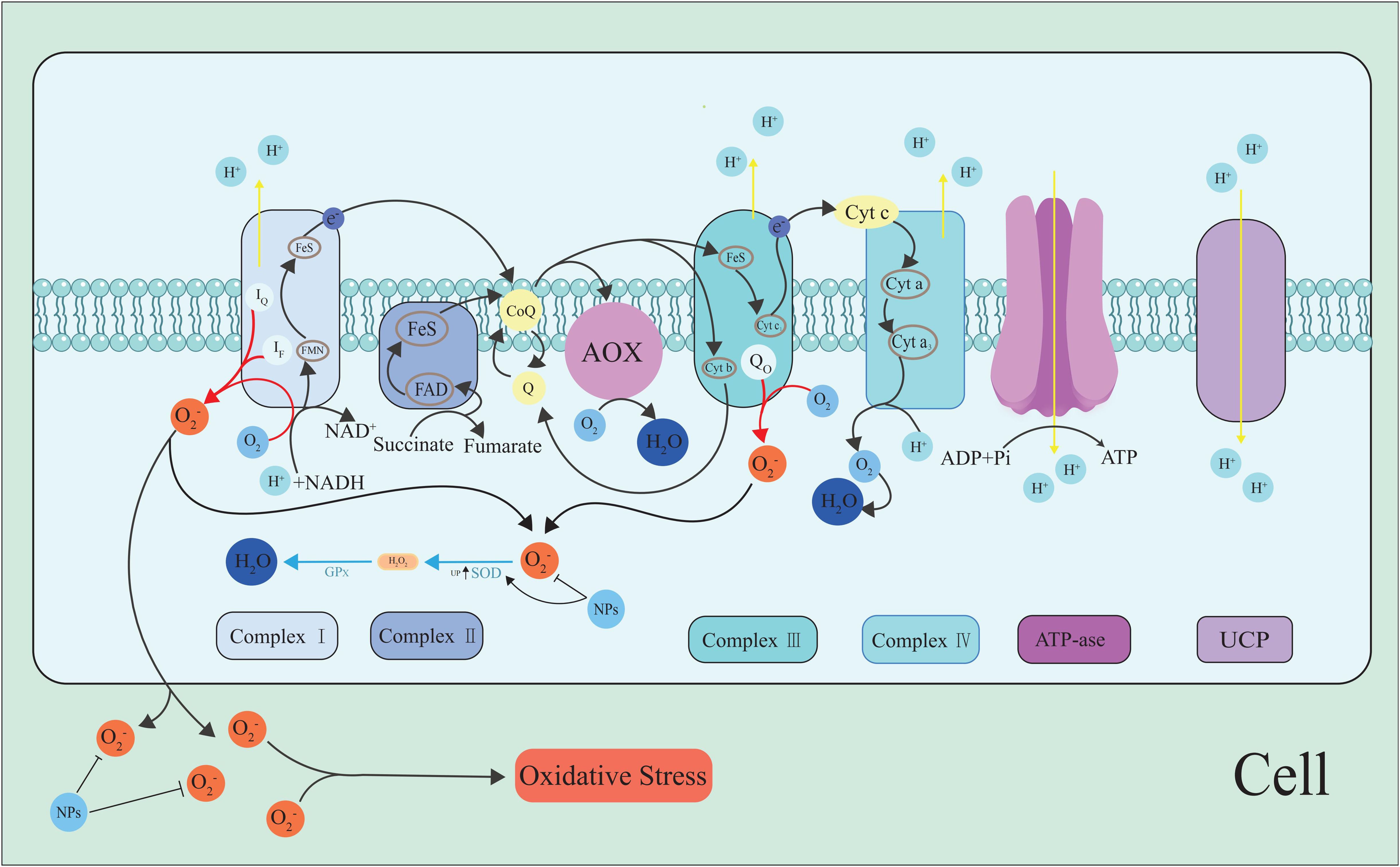
Figure 1. ROS generation along the RETC pathway. O2•− is formed upon single-electron reduction of O2 (Chenna et al., 2022). Complex I and III in the RETC are the major sites of O2•− production. UCP and AOX in RECT. The energy of the proton gradient drives the ATP synthesis of or can be consumed by UCPs. NPs inhibit the ROS generation and scavenge ROS, maintain cell ROS homeostasis. The black arrow indicates the electron pathway or ROS transfer, and the red arrows represent O2•− generation.
Mitochondrial damage caused by oxidative stress can trigger the release of metals in mitochondrial proteins (Mittler et al., 2004). In the presence of high levels of O2•− and H2O2, the release of metals accelerates the reaction to generate •OH, thus causing membrane damage through lipid peroxidation (Tan et al., 2010; Mittler, 2017; Suzuki, 2023). Some studies have shown that the content of Cu and Fe in the mitochondria is reduced by 40% after oxidative stress, which indicates that the complex containing these two metals in the electron transport chain on the mitochondrial membrane is damaged (Tan et al., 2010). This may lead to an increase in free ferrous ions, with H2O2 and Fe2+ forming highly toxic •OH, which damages plants (Mittler, 2017). As these complexes are sensitive to abiotic stresses, their protection is important for maintaining ROS homeostasis.
Uncoupling protein (UCP) is a membrane albumen located in the inner membrane of the mitochondria that belongs to the mitochondrial anion operating family. One study found that the increased expression of UCP in the tobacco mitochondria may lead to greater tolerance of drought stress (Begcy et al., 2011). Further research on the mechanism of UCP in maintaining mitochondrial ROS homeostasis may unearth the great potential of plants in the face of abiotic stresses.
Alternation oxidase (AOX) is another mitochondrial protein involved in maintaining cellular metabolism and energy balance, and its role is even more important under stress (Kumari et al., 2019; Garmash, 2022).AOX reduces excess oxygen in mitochondria by providing additional electron consumption pathways, thereby reducing ROS production and maintaining ROS homeostasis (Maxwell et al., 1999; Analin et al., 2023).In addition, AOX plays an important role in ethylene-induced drought resistance and improves drought resistance in tomato seedlings by balancing ROS levels in autophagy production (Zhu et al., 2018).Under osmotic and temperature stress, AOX pathway regulation of ROS through redox couples related to malate valve and antioxidant system (Dinakar et al., 2016). A study found that a lack of AOX under drought stress led to an increase in cell damage and a decrease in the ability of tobacco plants to recover quickly once the water supply was restored (Wang and Vanlerberghe, 2013). In another study AOX metabolites alongside the activation of several AOX enzymes relieve impact of combined heat and salt stresses on tomato (Sousa et al., 2022).
3.2 ROS in the chloroplasts
As the main site of photosynthesis, chloroplasts are important organelles in plants, and the photosynthetic electron transport chain (PETC) on the thylakoid membrane is crucial for plant photosynthesis. Chloroplasts not only carry out photosynthesis but also sense and transmit stress signals under abiotic stresses such as high temperature and drought (Foyer and Shigeoka, 2011; Hu et al., 2020b; Somal et al., 2023). They are also the most important plant parts for producing ROS under stress. Photosystem I (PSI) and PSII in the PETC on the thylakoid membrane are the main sites for ROS production (Takahashi and Asada, 1988; Roach and Krieger-Liszkay, 2014) (Figures 2, 3).
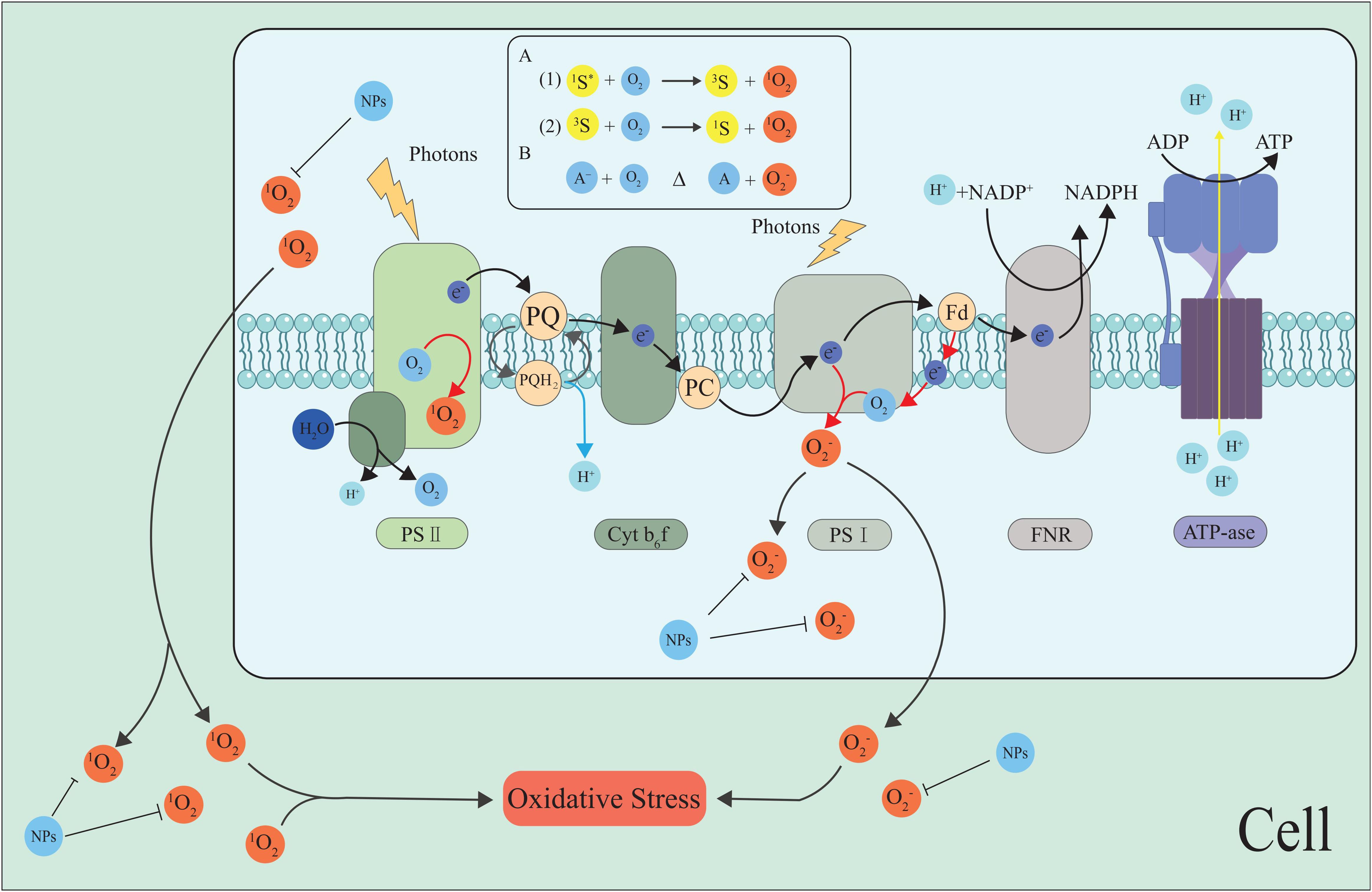
Figure 2. The generation of ROS in the photosynthetic electron transport chain. Photoexcitation of chlorophyll at PSII and PSI drives electron transport, but in the absence of acceptors, excitation may transfer to O2 at the PSII reaction center, forming a 1O2 and O2•−. NPs inhibit the ROS generation and scavenge ROS, maintain cell ROS homeostasis. The most common mechanism of 1O2 generation is photosensitization, i.e., the reaction of O2 with a photoexcited sensitizer dye (S*). Produced by spin-conserved Reactions (A1) and (A2). O2•− is mainly formed by the interaction of O2 with reducing compounds (A) with low redox potential (B) (Khorobrykh et al., 2020).The black arrow indicates the electron pathway or ROS transfer, and the red arrows represent O2•− generation. Fd, ferredoxin; FNR, ferredoxin-NADP reductase; PC, plastocyanin; PQ, plastoquinone; and Cytb6f, Cytochromeb6fcomple.
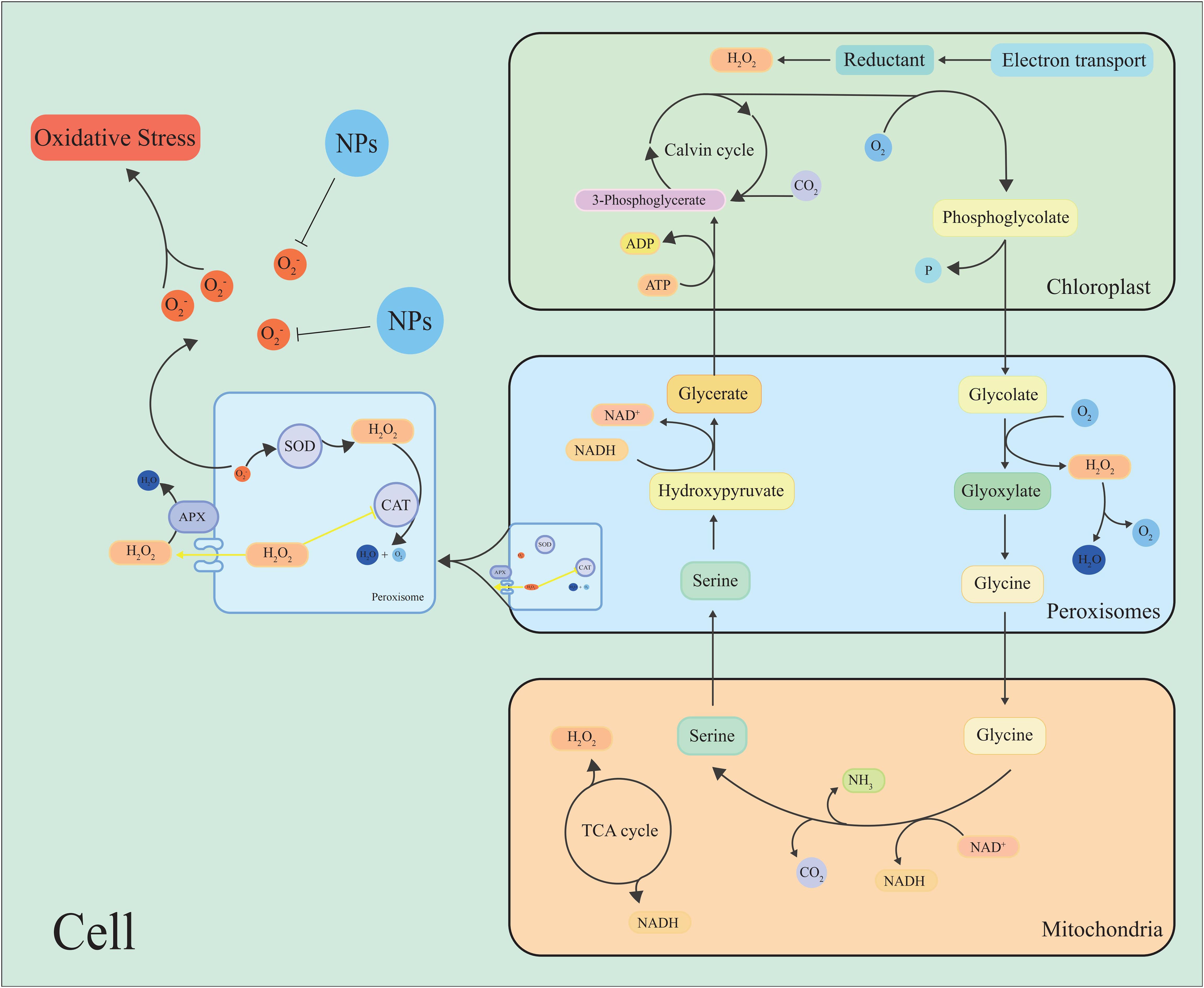
Figure 3. Simple model for the production or removal of H2O2 by plants through photorespiration and peroxisomal metabolism. During photorespiration, when oxalates are oxidized to glyoxylate by enzymes called glycolates, H2O2 is produced as a by-product. NPs inhibit the scavenge ROS, maintain cell ROS homeostasis.
The Mehler reaction is the main pathway of ROS production in the chloroplasts. During plant stress, the Mehler reaction reduces O2 to O2•− by PETC in PSI (Kozuleva et al., 2020a, Kozuleva et al., 2020b). PSII also produces ROS such as H2O2 (Pospíšil, 2009). Water-water cycling (WWC) is a highly efficient photoprotection strategy that can effectively scavenge O2•− and H2O2 from the thylakoids to protect plants from oxidative damage (Asada, 1999, Asada, 2000; Rizhsky et al., 2003; Awad et al., 2015; Huang et al., 2019). Additional findings suggest that WWC enhancement at high temperatures protects PSI, while cyclic electron flow enhancement at low temperatures compensates for WWC deactivation, both of which together protect plants from ROS damage (Sun et al., 2020; Jiang et al., 2021). Maintaining chloroplast ROS homeostasis by regulating WWC may be an effective way to improve plant stress resistance.
Nicotinamide adenine dinucleotide phosphate (NADP) is one of the end electron acceptors in the electron transport chain of photosynthesis. The reduced nicotinamide adenine dinucleotide phosphate (NADPH) is an important reducing force in chlorophyll synthesis and the Calvin cycle. NAD kinases (NADK) are involved in many plant life activities, such as maintaining intracellular REDOX balance and responding to environmental stress. Studies have shown that NADK activity mediates response mechanisms to regulate PSI biosynthesis (Ji et al., 2022). Because NADP can provide the main reduction power for the ROS scavenging system (Mittler, 2002).In addition, NADK deficiency results in decreased ROS stress and drought tolerance in plants (Sun et al., 2017; Wang et al., 2020; Chaomurilege et al., 2023).
Non-photochemical Chlorophyll fluorescence quenching (NPQ) is a process that occurs in plants where excess absorbed light energy is converted into heat energy (Demmig-Adams et al., 2014). The relationship between NPQ and ROS homeostasis is complex. When the NPQ generation process is inhibited, such as by thermal inactivation of APX, the formation of ROS increases, which can lead to plant damage. It was found that heat treatment could significantly stimulate NPQ, while increasing the temperature almost completely inhibited NPQ, and was accompanied by the generation of ROS (Hideg et al., 2008). This indicates that the NPQ generation and ROS clearance processes are closely linked. According to Liu et al. (2022) the significantly higher non-photochemical quenching (NPQ) in bundle sheath chloroplasts compared to mesophyll chloroplasts under drought stress could be a primary reason for the lower accumulation of ROS in bundle sheath chloroplasts. Studying the relationship between NPQ and ROS homeostasis and the light damage resistance of chloroplasts is important for improving plant stress resistance.
3.3 ROS in the apoplasts
Apoplasts are composed of intercellular space, a cell wall, and an extracellular matrix, and they play an important role in the transport of water, mineral nutrients, and organic nutrients, as well as the production of signaling molecules in response to stress (Hoson, 1998; Sattelmacher, 2001; Koch et al., 2003; Qi et al., 2017). Although the level of ROS production in the apoplasts is much lower than that of the chloroplasts and mitochondria, as the site of ROS production, it is no less important to plants than the chloroplasts and mitochondria (Jubany-Mari et al., 2008; Barceló and Laura, 2009). It plays an important role in the plant perception of external stresses (Mittler, 2017; Mittler et al., 2022).
In the apoplasts, ROS are mainly produced by NADPH oxidase (Podgórska et al., 2017). Respiratory burst oxidase homologs (RBOHs) are the main sites of ROS production in apoplasts, and they transfer electrons from cytosolic NADPH to O2 and then produce O2•− in apoplast bodies, following which they generate relatively stable H2O2 catalyzed by SOD and then enter the cell as a signaling molecule (Sagi and Fluhr, 2006; Marino et al., 2012; Hu et al., 2020a). NADPH acts as a fuel for RBOHs and provides sufficient substrate for ROS production when plants encounter stress (Wu et al., 2023a). ROS waves are crucial to the acquired adaptation of plant systems (Zandalinas et al., 2020). It was reported that the dependent D(rbohD) gene inhibits signal propagation by inhibiting ROS accumulation away from the start site, suggesting that ROS waves are RBOHD-dependent (Miller et al., 2009; Mittler and Blumwald, 2015).
The H2O2 produced by NADPH oxidase acts as a secondary messenger to activate the MAPK, a conserved intracellular pathway in plants consisting of MAP kinase kinase kinase (MAPKKK), MAP kinase kinase (MAPKK), and MAP kinase (MAPK). These kinases are activated step by step through phosphorylation and dephosphorylation. In this process, MAPKKK is first activated; it then activates the downstream MAPKK, and finally, MAPKK will activate the most downstream MAPK, this cascade reaction allows the signal to be amplified and transmitted within the cell (Jonak, 2002). The MAPK cascade pathway is highly correlated with abiotic stresses such as high temperature and drought; for example, the expression level of MAPK was significantly correlated with the relative biomass of plants in response to drought (Liu et al., 2015). Another study showed that PlMAPK1 plays a positive regulatory role in the plant response to heat stress (Qian et al., 2023). ROS can induce MAPK activation, and interfering with the MAPK cascade can modulate ROS production and response. With further climate change, it is important to investigate the relationships and interaction mechanisms of this pathway with ROS, phytohormones, and other substances in the apoplasts to improve plant resistance to unfavorable conditions. Extracellular peroxidase (ECPOX) is a protein that induces ROS production and detoxification in apoplasts, It is closely related to the formation of extracellular O2•− (Li et al., 2010; Gautam et al., 2017).Studies have found that the activity of ECPOX is related to the production of ROS, in the apical cell wall of pea seeds, the activity of ECPOX increases simultaneously with the production of O2•− (Kranner et al., 2010).In wheat roots, the production of O2•− sensitive to peroxidase inhibitors was taken as evidence indicating that ECPOX was involved in ROS production (Minibayeva et al., 2009).
Polyamines are important regulatory factors of ROS homeostasis (Saha et al., 2015; Kolupaev et al., 2019). H2O2 in plant cell walls can be produced by the metabolism and recovery of polyamines, of which diamine oxidase (DAO) and polyamine oxidase (PAO)are key to polyamine metabolism (Moschou et al., 2008). PAOs used polyamine as substrate to catalyze the formation of smaller polyamines, as well as a molecule of amino aldehyde and H2O2. The other was DAO dependent on Cu2+ and using pyridoxal phosphate as coenzyme. DAO catalyzes putrescine to produce H2O2, ammonia and 4-aminobutyraldehyde (Kusano et al., 2007, Kusano et al., 2008). Another characteristic of tomato slpao3 mutant was found to be tolerant to drought stress, indicating polyamine helps to improve drought resistance (D’Incà et al., 2024).
Oxalate oxidase (OXO) in the cell wall catalyzes the oxidation of oxalic acid with molecular oxygen to form CO2 and H2O2 (Verma and Kaur, 2021). Studies have shown that high levels of OXO expression produce a large amount of H2O2 that also has a direct harmful effect on plants, inducing cell death (Delisle et al., 2001).
3.4 ROS in the peroxisomes
Peroxisomes are important sites to produce ROS by oxidative metabolism in plant cells, such as O2•−, H2O2, and 1O2, which participate in different physiological processes and play an important role in maintaining cellular redox homeostasis (Río et al., 2006; Choudhury et al., 2016) (Figure 3). Photorespiration has a substantial impact on plant metabolism and is the pathway of ROS production and clearance in peroxisomes (Noctor, 2002; Foyer et al., 2009; Del Río, 2020; Timm and Hagemann, 2020) (Figure 3).
Hydroxy pyruvate reductase 1 (HPR1), the main metabolic enzyme in the photorespiration cycle, can be inhibited by high light intensity, which exacerbates the production of ROS (Wang et al., 2022d). It has been found that the ROS metabolism of photorespiration has a dual role, and xanthine oxidase can mediate the production of O2•− (Tewari et al., 2009). Xanthine dehydrogenase 1 (XDH1) produces ROS with NADPH oxidase in leaf epidermal cells, and the clearance of H2O2 in mesophyll cells by XDH1 protects plants from oxidative damage (Ma et al., 2016). Researchers have found that photorespiratory metabolism was downregulated in Arabidopsis after hypoxia or the addition of aminooxyacetic acid (a photorespiratory inhibitor), while ROS levels were significantly elevated (Saini, 2023). Another study found that the photorespiration metabolism of rice was upregulated under high temperature and iron stress to ensure survival under stress (De Souza et al., 2024). These studies suggest that the regulation of photorespiration is an important way to maintain ROS homeostasis.
It was found that the H2O2 content in quinoa increased under high temperature and drought conditions, and Peroxisome abundance was positively correlated with H2O2 content in the leaves (Hinojosa et al., 2019). The OsAPX4 gene encoding peroxisomes in rice is thought is thought to affect plant development and resilience by regulating ROS signaling, suggesting that the peroxisome plays a significant role in maintaining ROS homeostasis in plant cells (Ribeiro et al., 2017).
4 ROS detoxification
4.1 Enzymatic system
Antioxidant enzymes such as CAT, SOD, APX, and glutathione reductase (GR) constitute the ascorbate-glutathione cycle (ASA-GSH cycle), which can effectively scavenge ROS such as H2O2 in plants. This pathway plays a vital role in helping plants cope with stresses such as high temperature and drought (Tiwari et al., 2017; Del Río, 2020). There are other antioxidant enzymes, such as GPX, glutathione S-transferase (GST), monodehydroascorbate reductase (MDHAR), and dehydroascorbate reductase (DHAR), which together form a complex component of the antioxidant defense system and can effectively scavenge ROS to maintain ROS homeostasis in plants (Zhou et al., 2017, Zhou et al., 2018; Sarker and Oba, 2018; Hasanuzzaman et al., 2019).
SOD can be divided into Fe-SOD, Mn-SOD, and Cu/Zn-SOD isoenzymes according to the different metal co-groups, which can catalyze O2•− to H2O2 (Alscher et al., n.d.). H2O2 can be further broken down by other antioxidant enzymes such as CAT or APX; CAT can split H2O2 into water and oxygen, and APX uses ascorbic acid to scavenge H2O2 under drought stress (Hasanuzzaman et al., 2012; Tiew et al., 2015). A study showed that CAT activity increased significantly under severe drought conditions, while mild and severe drought altered the expression patterns of CAT1 and CAT2. These results suggest that complex regulation at the level of CAT mRNA translation controls the accumulation of H2O2 in leaves (Luna, 2004). At high temperatures, SmAPX2 with APX activity can catalyze the oxidation of ascorbic acid by H2O2 in vitro and reduce the accumulation of H2O2 under high temperature stress in vivo through the temporary expression of SmAPX2. These results indicate that SmAPX2 plays an active role in plant responses to high temperature stress (Shen et al., 2024).
The GR-mediated ASA-GSH cycle in the peroxisome has special significance in ROS clearance and tolerance to abiotic stress (Foyer and Noctor, 2011). One study showed that the accumulation of GR gene transcripts under heat stress was 3.1 times that of untreated samples, and the gene was upregulated by various stresses (Chanda Roy and Chowdhary, 2023). OsNAC092 mutant rice had higher ROS clearance ability and maintained a higher GSH/GSSG ratio and reduction level under drought conditions, thus protecting cells from oxidative stress (Wang et al., 2022a).
A study by explored the response of maize varieties with different heat tolerances to the ASA-GSH cycle under high temperature stress. The results showed that high temperature resulted in increased ROS and increased levels of reduced glutathione (GSH) and oxidized glutathione (GSSG) in all varieties and significantly increased GST activity. The enzyme activities of APX, MDHAR, and DHAR were decreased in sensitive varieties, while the GR enzyme activities were significantly increased in heat-resistant varieties, and the AsA levels were increased in heat-resistant varieties and decreased in sensitive varieties (Tiwari and Yadav, 2020). Another study on wheat under high temperature stress found that both the GR and APX activities increased with increasing heat stress. The GR and APX activities of wheat varieties with higher heat resistance were the highest (Mohi-Ud-Din et al., 2021). Furthermore, when cotton was stimulated by H2O2, the activity of MDHAR significantly increased. Additionally, SOD, APX and CAT activities also increased significantly (Madhu et al., 2024). These results suggest that GhMDHAR can participate in the H2O2 response and plays an important role in maintaining ROS homeostasis. Recent studies have shown that the TaMDHAR gene was significantly upregulated in wheat under drought, high temperature, and high salt conditions. The activity of TaMDHAR increased significantly under high temperature and drought stress, while that of MDHAR decreased. It was also found that the MDHAR protein mainly interacted with Asc, DPN, NADP+, NADPH, CoA, FAD+, MgATP, H2O2, FMN, MgADP, and GSSG (Madhu et al., 2024). The researchers found that high temperatures increased MDHAR activity in two rice varieties, IR-64(heat sensitivity) and Huanghua Station (heat tolerance), and CAT, SOD, and POD activities were also affected. Moreover, the contents of H2O2 and MDA in rice were increased under high temperature, and the concentrations of cytokinin and auxin in different plant sites in the tested heat-sensitive and heat-tolerant cultivars were also affected. The study also found that the MDHAR activity was increased following the application of plant growth regulators such as methyl jasmonate and ascorbic acid (Al-Zahrani et al., 2022).
In conclusion, the ASA-GSH cycle plays an important role in plants during stress, and MDHAR has a non-negligible effect on the ASA-GSH cycle and even the antioxidant system of the entire plant. Studies on the ASA-GSH cycle, the enzyme system in the peroxisome, and the regulatory effects of exogenous substances on the ASA-GSH cycle are of great significance for improving crop stress resistance.
4.2 Non-enzymatic system
Small-molecule antioxidants exist in plant cells. Some highly toxic ROS, such as 1O2 and •OH, that cannot be scavenged by the antioxidant enzyme system can neutralize free radicals or produce a relatively harmless free radical by donating electrons or hydrogen atoms by antioxidants, which are later effectively scavenged by other antioxidant systems (Hernández et al., 2009). Antioxidants in plants include AsA, GSH, flavonoids, tocopherols, and carotenoids, among others (Hernández et al., 2009, Hernández et al., 2012; Dangles, 2012; Shen et al., 2018).
Tapetum development and functional defects1 (TDF1) can inhibit tapetum cell division. The downstream target of TDF1, SKS18, was found to encode a polycopper oxidase-like protein with ascorbate oxidase activity. Moreover, TDF1 negatively controls an AsA biosynthetase VTC1 to regulate AsA biosynthesis and maintain appropriate AsA content. SKS18 knockdown or VTC1 overexpression can increase AsA concentration and reduce ROS accumulation, thereby balancing cell division and cell differentiation in the felt layer (Wu et al., 2023b). Flavonol synthetase (FLS) is one of the key enzymes in the synthesis of flavonoids. Under drought stress, overexpression of EkFLS in Arabidopsis resulted in the increased accumulation of flavonoids and significantly enhanced POD and SOD activities (Wang M. et al., 2021).
Carotenoids and α-tocopherol, as components of the non-enzymatic antioxidant system, can also scavenge ROS. Studies have shown that NtCCD1 is a negative regulator of carotenoid content in tobacco, and silencing NtCCD1 by virus-induced gene silencing (VIGS) can increase carotenoid contents and decrease ROS levels (Du et al., 2023). Another study in tomatoes identified a VTE5 gene that plays a key role in alpha-tocopherol production. When VTE5 is silenced, the production of α-tocopherol is affected, which reduces the plant’s ability to resist high temperature and light stress. However, the content of α-tocopherol almost doubled under combined stress compared to exposure to high temperatures or high light alone (Spicher et al., 2017).
With the furthering of biological and agronomic research in recent years, the application of exogenous substances for improving the antioxidant capacity and enhancing the stress resistance of plants has become increasingly prevalent. For example, the exogenous application of AsA and α-tocopherol is used to maintain ROS homeostasis and improve drought resistance (El-Beltagi et al., 2022; Zafar et al., 2024). Melatonin was first discovered in the pineal gland of animals, and it is thought to be a free radical scavenger that can be synthesized in vivo (Hevia et al., 2014; Vielma et al., 2014; Hardeland, 2017; Zhao et al., 2019). The application of exogenous melatonin in plants has also gained increasing research interest. Studies have shown that exogenous melatonin can effectively reduce the oxidative stress caused by iron deficiency in sweet pepper and enhance antioxidant defense mechanisms. In addition, the chlorophyll content and photosynthetic efficiency of plants under salt stress can be improved (Kaya et al., 2020). Melatonin and hydrogen sulfide regulate the antioxidant defense system under chromium stress, and the supplementation of melatonin to tomato seedlings under stress eliminates excess ROS, thus maintaining ROS homeostasis and improving the stress resistance of tomato seedlings (Khan et al., 2023). Studies on the effects of exogenous melatonin under high temperature and drought stress showed that supplementation with exogenous melatonin could regulate the ASA-GSH system and affect the activity of antioxidant enzymes, thereby protecting plants from oxidative damage caused by drought (Khan, 2023).Other studies have shown that exogenous melatonin can alleviate the negative effects of excess ROS, significantly increase the activity of antioxidant enzymes, stabilize chloroplast structure, and improve the drought resistance of maize seedlings (Muhammad et al., 2023). Moreover, melatonin can enhance the heat resistance of rice seeds by enhancing the activity of antioxidant enzymes and significantly reducing the content of malondialdehyde (Yu et al., 2022). In addition, some studies have shown that the application of exogenous melatonin can increase the activity of antioxidant enzymes and the concentration of metabolites involved in osmoregulation and ion homeostasis in mung bean under drought and high temperature stress, as well as improve the physiological and yield traits of mung bean under combined stress in the reproductive stage (Kuppusamy et al., 2023).
5 Nanobiotechnology regulate ROS homeostasis and heat and drought tolerance in plants
5.1 Reported nanomaterials maintain ROS homeostasis
Maintaining plant ROS homeostasis can improve plant stress tolerance. With increased research interest in nanotechnology in recent years, the relationship between nanomaterials and plants has attracted increasing attention.
Nanomaterials (NMs) are defined as materials with at least one dimension in the nanoscale (1–100 nm) (Auffan et al., 2009). Due to their small size effect, NMs have unique properties and can penetrate through cell walls and enter plant cells to regulate the complex physiological and biochemical processes of plants, thus regulating plant growth and development. Researchers are taking advantage of the special properties of NMs to study the interaction between NMs and plants (Shi et al., 2014; Ajmal et al., 2023). Researchers alter the properties of NMs by applying different modifications to their surfaces. As a nano-mimetic enzyme, polyacrylic acid-modified manganous tetroxide nanoparticles (PMO) have both SOD and CAT activities, and they can effectively scavenge excess ROS, including H2O2 (Liu et al., 2023). Studies have shown that under drought stress conditions, PMO can effectively scavenging excess ROS through their nanase activity and alleviate the mitotic activity inhibited by drought by maintaining ROS homeostasis (Sun et al., 2023). Mn3O4 NPs can enhance the endogenous antioxidant defense ability of plants and relieve the oxidative stress of cucumber plants (Lu et al., 2020). Cerium oxide nanoparticles are also known to be an effective ROS scavenger. The principle of ROS scavenge is to catalyze ROS decomposition by utilizing the oxygen vacancy in their lattice structure (Giraldo et al., 2014). Researchers have found that Poly(acrylic acid) nanoceria (PNC) can scavenge a variety of ROS, including •OH, protect plant photosynthesis, and reduce the damage to plants caused by abiotic stress (Wu et al., 2017). CeO2 nanoparticles can also improve the SOD activity and salt tolerance of cotton and rape, Se-NPs scavenge ROS in sorghum under drought stress, relieve oxidative stress, and protect the photosynthesis and grain yield of sorghum (Djanaguiraman et al., 2018b; Liu et al., 2021; Li et al., 2022).
Nanozymes are nanomaterials with enzyme characteristics. Nanoparticles not only directly scavenge ROS but also indirectly improve the ability of plants to scavenge ROS by affecting the activity of antioxidant enzymes (Singh, 2019; Wu et al., 2019). Spraying nano-selenium under drought and high temperature stress increases the antioxidant enzyme activity of wheat seedlings, thereby improving the ability of wheat to resist high temperature and drought stress (Omar et al., 2023). Other nanoparticles such as Ag NPs can improve wheat resistance to high temperatures, while Si NPs help wheat mitigate drought stress, and TiO2 NPs alleviate the effects of drought stress on tomato (Iqbal et al., 2019; Boora et al., 2023; Cevik, 2023). Currently, Mn NPs, Ce NPs, Si NPs, Se NPs, Ag NPs, Ti NPs, and other nanoparticles have been applied to wheat, cucumber, rape, sorghum, and other plants to circumvent the negative effects of high temperature and drought stress on plants (Djanaguiraman et al., 2018b; Iqbal et al., 2019; Boora et al., 2023; Cevik, 2023; Omar et al., 2023; Sun et al., 2023; Nauman Khan et al., 2024) (Figure 4; Table 1).
Research shows that effects of NMs on plants under stress are diverse. Research shows, that PNC catalytic scavenging of •OH influences the activity of key plasma membrane channels controlling plant cell K+ retention, thereby alleviating the damage of salt stress to Arabidopsis (Wu et al., 2018). In addition, carbon dots NMs also have ROS scavenging functions (Dong et al., 2022; Innocenzi and Stagi, 2023). Salvia miltiorrhiza-derived the synthesized carbon dots have a scavenging effect on intracellular ROS in plants (Wang et al., 2022d) (Table 1). Soil application of carbon dots enhanced the N-fixing ability, the expression of genes related to nitrogen transport and water absorption was enhanced of nodules enhanced N and water uptake in soybeans under drought stress, promoted the growth and nutritional quality of soybeans (Wang et al., 2022c).
Recently, researchers synthesized manganese oxide nanoparticles (MnO2 MFs) and cerium oxide nanoparticles (CeO2 NPs) and regulated the interaction between the two by polydiallyldimethylammonium chloride surface modification, forming Ce−PMn and Mn−PCe composite materials. The formed composites exhibited a variety of enzymatic activities, such as SOD and CAT functions, and the composites Ce−PMn and Mn−PCe retained the nanozymatic activities of individual metal oxides to a certain extent (Alsharif et al., 2023). This suggests that maintaining ROS homeostasis to improve stress resistance may not be limited to a single nanomaterial. The application of composite materials is also an important focus.
In addition to maintaining ROS homeostasis and improving plant stress resistance, the special properties of NMs can also be used as tools for conveying agricultural chemicals, such as nano-fertilizers, and nano-pesticide (Ahmadian et al., 2021; Wang et al., 2022d, Wang et al., 2023; Farajollahi et al., 2023). Based on the special properties of NMs, nano fertilizers also show better utilization efficiency. For example, the application of nano-silica and nano-iron oxide fertilizers under drought stress can improve the activity of antioxidant enzymes and reduce the harmful effect of drought stress on wheat and soybeans, and the application of nano-iron oxide can also increase the activity of soybean nitrogenous enzyme (Ahmadian et al., 2021; Farajollahi et al., 2023). The use of NMs to improve the ability of biological nitrogen fixation not only uses the properties of NMs to target specific organelles for precise and effective delivery but also avoids the risks associated with transgenic methods (Li et al., 2023). In addition to nano fertilizers, nano pesticides show a better control effect than commercial synthetic pesticides in field trials, providing a new and sustainable approach for the control of plant diseases (Wang et al., 2022b). Recent studies have used nanotechnology to design a self-assembled nano bioprotectant based on double-stranded RNA and plant inducers, which enhances endocytosis and amplifies plant system defense responses, effectively helping plants cope with potato late blight infection (Wang et al., 2023).
For a long time, researchers try to investigate the possible mechanisms of nanomaterials enhance plant abiotic stress tolerance. Maintaining ROS homeostasis is the main idea of plant nanobitechology, and the downstream signaling pathway is also being studied. However, few attention has been paid to the material science mechanism.
Nanomedicine has some similarities with plant nanobitechology, and its material science mechanism can be used as reference. Cerium oxide nanoparticles (nanoceria), for instantance, was widely reported could enhance crop abiotic tolerance. Because the surface of NC has two valence states of Ce3+ and Ce4+, creating an oxygen vacancy (Boghossian et al., 2013), nanoceria can scavenge ROS effectively and enhance the salt resistance ability of plants. Further study on the nanoceria crystal structure shows that the catalytic efficiency of nanoceria with different crystal structures on ROS is different (Wang Z. et al., 2021; Yuan et al., 2023)The functional groups modified on the surface may also affect nanomaterials biological effects (Cheng et al., 2021). But there are no standardized guidelines for agricultural nanomaterials synthesis. Thus, in general, the mechanisms of nanomaterials maintaining plant ROS homeostasis is through Redox reaction from botany, but material science mechanisms need further study.
5.2 Issues in nanomaterials and plants interaction
The first step in the interaction between nanomaterials and plants is the cell. The cell wall and membrane are hindrances for nanomaterials entering plant cells. The cell wall has been reported to have pores (Zeng et al., 2017) and negative potential (Fry, 2011). Normally, a particle size smaller than the pore’s diameter and with positive potential can cross the cell wall more easily. Different sizes and potentials affect how nanomaterials enter plant cells. By exploring different leaf structures, researchers have found that they are dependent on a suitable size. However, in both cotton and maize, positive potential nanomaterials have better delivery efficiency. Problems usually have a secondary solution. The cell wall consists of pectin and cellulose; thus, cellulase can damage the cell wall and enable the entry of nanomaterials into the cell (Serag et al., 2012). Groups and ions in nanomaterials are likely to have a decisive influence on the interaction between nanomaterials and plants. Pectin was reported as an key factor for nanomaterials access cell wall (Zhu et al., 2023).
As mentioned above, nanomaterials with a positive potential may have better delivery efficiency, but bio-functions can be quite the opposite. Nanoceria is considered to have a good ROS-scavenging ability but with uncertain bio-functions. Wu et al. (2017) studied different potential ceria bio-functions and indicated that negative potential ceria had the best performance in Arabidopsis. This means that the design of nanomaterials also needs to consider their application.
The effects of nanomaterials on plants have been reported with both negative and positive implications. With the development of nanotechnology research interest, too many nanoparticles are emitted into the environment and have imposed a negative impact on plants (Hossain et al., 2015). The way NPs are used need further understanding and determination. Existing application methods, such as foliar sprays and root applications, result in a significant release of nanomaterials to the environment. For food production, a large amount of NPs is absorbed by arable land (Prakash et al., 2021). It is reported that the soil microbial community’s diversity and composition have been altered upon exposure to 100 mg/kg tungsten disulfides nanomaterials (Shi et al., 2022). Another study quantified the movement of NPs through the food chain, starting with NPs adhering to algae, then transferring to Daphnia, and ultimately ending up in fish. The study revealed that NPs were present in fish tissues, with the highest levels found in the brain (Abdolahpur Monikh et al., 2021). It is an important question to study how NPs are applied and how their concentrations affect plants and the environment to create a balance. When NPs are constantly being discharged, concerns about the potential accumulation of nanomaterials in edible parts of crops and subsequent accumulation in the food chain becomes a huge concern, which can pose risks to human health.
Achieving precise delivery of nanomaterials to plants and reducing the impact on the environment is the way forward. Moreover it is essential to establish thorough regulatory frameworks for the utilization of nanomaterials in agriculture and the environment. Creating regulatory guidelines and standardized procedures can help mitigate potential risks and ensure the safe use of nanotechnology for environmental sustainability.
6 Conclusion and prospects
The aim of the research and development of plant nanobitechology is to improve plant tolerance to stress and improve crop yield and quality. Murali et al. reviewed the uptake, transport, and bioaccumulation of NMs in plants, as their bioaccumulation has an impact on the food chain via their transportation and accumulation in the edible tissues of plants (Murali et al., 2022). While NMs have the potential to improve agricultural sustainability in crop production, their widespread manufacture and release into the environment are a growing concern. It is necessary to further explore the mechanism by which plant resistance is improved through plant nanobiology and to develop NMs whose production cost is affordable and on par with agricultural products. In addition, it is important that more environmentally friendly NMs are explored to determine the best NMs for application in different crops. The promotion of NMs from the experimental stage to real production applications is also a key issue.
Author contributions
LB: Conceptualization, Writing – original draft, Writing – review & editing. JL: Validation, Writing – review & editing. TM: Methodology, Writing – review & editing. LZ: Software, Writing – review & editing. DW: Conceptualization, Writing – review & editing. YZ: Validation, Writing – review & editing.
Funding
The author(s) declare that financial support was received for the research, authorship, and/or publication of this article. This work was supported by the National Natural Science Foundation of China (32060259; 32360547), Bingtuan Southern Xinjiang Key Industries Development Foundation (2022DB015), President’s fund of Tarim University (TDZKBS202408) and Bingtuan Natural Science Foundation (2024DB024).
Acknowledgments
We thank LetPub (www.letpub.com) for its linguistic assistance during the preparation of this manuscript.
Conflict of interest
The authors declare that the research was conducted in the absence of any commercial or financial relationships that could be construed as a potential conflict of interest.
Publisher’s note
All claims expressed in this article are solely those of the authors and do not necessarily represent those of their affiliated organizations, or those of the publisher, the editors and the reviewers. Any product that may be evaluated in this article, or claim that may be made by its manufacturer, is not guaranteed or endorsed by the publisher.
References
Abdolahpur Monikh, F., Chupani, L., Arenas-Lago, D., Guo, Z., Zhang, P., Darbha, G. K., et al. (2021). Particle number-based trophic transfer of gold nanomaterials in an aquatic food chain. Nat. Commun. 12, 899. doi: 10.1038/s41467-021-21164-w
Ahmadian, K., Jalilian, J., Pirzad, A. (2021). Nano-fertilizers improved drought tolerance in wheat under deficit irrigation. Agric. Water Manage. 244, 106544. doi: 10.1016/j.agwat.2020.106544
Ajmal, M., Ullah, R., Muhammad, Z., Khan, M. N., Kakar, H. A., Kaplan, A., et al. (2023). Kinetin capped zinc oxide nanoparticles improve plant growth and ameliorate resistivity to polyethylene glycol (PEG)-induced drought stress in Vigna radiata (L.) r. wilczek (Mung Bean). Molecules. 28. doi: 10.3390/molecules28135059
Alscher, R. G., Erturk, N., Heath, L. S. (2002). Role of superoxide dismutases (SODs) in controlling oxidative stress in plants. J. Exp. Bot. 53(372), 1331–1341 doi: 10.1093/jexbot/53.372.1331
Alsharif, N. B., Viczián, D., Szcześ, A., Szilagyi, I. (2023). Formulation of antioxidant composites by controlled heteroaggregation of cerium oxide and manganese oxide nanozymes. J. Phys. Chem. C. 127, 17201–17212. doi: 10.1021/acs.jpcc.3c03964
Al-Zahrani, H. S., Alharby, H. F., Fahad, S. (2022). Antioxidative defense system, hormones, and metabolite accumulation in different plant parts of two contrasting rice cultivars as influenced by plant growth regulators under heat stress. Front. Plant Sci. 13. doi: 10.3389/fpls.2022.911846
Analin, B., Bakka, K., Challabathula, D. (2023). Exacerbation of drought-induced physiological and biochemical changes in leaves of Pisum sativum upon restriction of COX and AOX pathways of mitochondrial oxidative electron transport. J. Biosci. 49, 5. doi: 10.1007/s12038-023-00380-0
Asada, K. (1999). The water-water cycle in chloroplasts: scavenging of active oxygens and dissipation of excess photons. Annu. Rev. Plant Physiol. Plant Mol. Biol. 50, 601–639. doi: 10.1146/annurev.arplant.50.1.601
Asada, K. (2000). The water–water cycle as alternative photon and electron sinks. Philos. Trans. R Soc. Lond B Biol. Sci. 355, 1419–1431. doi: 10.1098/rstb.2000.0703
Auffan, M., Rose, J., Bottero, J. Y., Lowry, G. V., Jolivet, J. P., Wiesner, M. R. (2009). Towards a definition of inorganic nanoparticles from an environmental, health and safety perspective. Nat. Nanotechnol. 4, 634–641. doi: 10.1038/nnano.2009.242
Awad, J., Stotz, H. U., Fekete, A., Krischke, M., Engert, C., Havaux, M., et al. (2015). 2-Cysteine peroxiredoxins and thylakoid ascorbate peroxidase create a water-water cycle that is essential to protect the photosynthetic apparatus under high light stress conditions. Plant Physiol. 167, 1592–1603. doi: 10.1104/pp.114.255356
Awasthi, R., Bhandari, K., Nayyar, H. (2015). Temperature stress and redox homeostasis in agricultural crops. Front. Environ. Sci. Eng. 3. doi: 10.3389/fenvs.2015.00011
Barceló, A. R., Laura, V. G. R. (2009)Reactive oxygen species in plant cell walls, inReactive oxygen species in plant signalingEds. Rio, L. A., Puppo, A. (Springer Berlin, HeidelbergBerlin, Heidelberg), 73–93. doi: 10.1007/978-3-642-00390-5_5
Begcy, K., Mariano, E. D., Mattiello, L., Nunes, A. V., Mazzafera, P., Maia, I. G., et al. (2011). An Arabidopsis mitochondrial uncoupling protein confers tolerance to drought and salt stress in transgenic tobacco plants. PloS One 6, e23776. doi: 10.1371/journal.pone.0023776
Boghossian, A. A., Sen, F., Gibbons, B. M., Sen, S., Faltermeier, S. M., Giraldo, J. P., et al. (2013). Application of nanoparticle antioxidants to enable hyperstable chloroplasts for solar energy harvesting. Adv. Energy Mater. 3, 881–893. doi: 10.1002/aenm.201201014
Boora, R., Sheoran, P., Rani, N., Kumari, S., Thakur, R., Grewal, S. (2023). Biosynthesized silica nanoparticles (Si NPs) helps in mitigating drought stress in wheat through physiological changes and upregulation of stress genes. Silicon 15, 5565–5577. doi: 10.1007/s12633-023-02439-x
Buckley, T. N. (2019). How do stomata respond to water status? New Phytol. 224, 21–36. doi: 10.1111/nph.15899
Calvin, K., Dasgupta, D., Krinner, G., Mukherji, A., Thorne, P. W., Trisos, C., et al. (2023). IPC: climate change 2023: synthesis report. (Geneva, Switzerland: Intergovernmental Panel on Climate Change) doi: 10.59327/IPCC/AR6-9789291691647
Carmo-Silva, A. E., Gore, M. A., Andrade-Sanchez, P., French, A. N., Hunsaker, D. J., Salvucci, M. E. (2012). Decreased CO2 availability and inactivation of Rubisco limit photosynthesis in cotton plants under heat and drought stress in the field. Environ. Exp. Bot. 83, 1–11. doi: 10.1016/j.envexpbot.2012.04.001
Černý, M., Habánová, H., Berka, M., Luklová, M., Brzobohatý, B. (2018). Hydrogen peroxide: its role in plant biology and crosstalk with signalling networks. Int. J. Mol. Sci. 19, 2812. doi: 10.3390/ijms19092812
Cevik, S. (2023). TiO2 nanoparticles alleviates the effects of drought stress in tomato seedlings. Bragantia. 82, e20220203. doi: 10.1590/1678-4499.20220203
Chanda Roy, P., Chowdhary, G. (2023). Molecular cloning of glutathione reductase from Oryza sativa, demonstrating its peroxisomal localization and upregulation by abiotic stresses. Acta Biochim. Pol. 70 (1), 175–181. doi: 10.18388/abp.2020_6441
Chaomurilege, Z., Miyagi, A., Hashida, S.-N., Ishikawa, T., Yamaguchi, M., Maki, K-Y, et al. (2023). Loss of chloroplast-localized NAD kinase causes ROS stress in Arabidopsis thaliana. J. Plant Res. 136, 97–106. doi: 10.1007/s10265-022-01420-w
Cheng, L., Wu, F., Bao, H., Li, F., Xu, G., Zhang, Y., et al. (2021). Unveiling the actual catalytic sites in nanozyme-catalyzed oxidation of o-phenylenediamine. Small. 17, 2104083. doi: 10.1002/smll.202104083
Chenna, S., Koopman, W. J. H., Prehn, J. H. M., Connolly, N. M. C. (2022). Mechanisms and mathematical modeling of ROS production by the mitochondrial electron transport chain. Am. J. Physiol. Cell Physiol. 323, C69–C83. doi: 10.1152/ajpcell.00455.2021
Choudhury, F. K., Rivero, R. M., Blumwald, E., Mittler, R. (2016). Reactive oxygen species, abiotic stress and stress combination. Plant J. 90, 856–867. doi: 10.1111/tpj.13299
Climate change indicators reached record levels in 2023: WMO (n.d). Available online at: https://wmo.int/news/media-centre/climate-change-indicators-reached-record-levels-2023-wmo (Accessed July 9, 2024).
D’Incà, R., Mattioli, R., Tomasella, M., Tavazza, R., Macone, A., Incocciati, A., et al. (2024). A Solanum lycopersicum polyamine oxidase contributes to the control of plant growth, xylem differentiation, and drought stress tolerance. Plant J. 960–981. doi: 10.1111/tpj.16809
Dangles, O. (2012). Antioxidant activity of plant phenols: chemical mechanisms and biological significance. Curr. Org Chem. 16, 692–714. doi: 10.2174/138527212799957995
Das, K., Roychoudhury, A. (2014). Reactive oxygen species (ROS) and response of antioxidants as ROS-scavengers during environmental stress in plants. Front. Environ. Sci. Eng. 2. doi: 10.3389/fenvs.2014.00053
Dat, J., Vandenabeele, S., Vranov, E., Van Montagu, M., Inz*, D., Van Breusegem, F. (2000). Dual action of the active oxygen species during plant stress responses. Cell Mol. Life Sci. 57, 779–795. doi: 10.1007/s000180050041
Delisle, G., Champoux, M., Houde, M. (2001). Characterization of oxalate oxidase and cell death in al-sensitive and tolerant wheat roots. Plant Cell Physiol. 42, 324–333. doi: 10.1093/pcp/pce041
Del Río, L. A. (2020). “Plant peroxisomes and their metabolism of ROS, RNS, and RSS,” in Progress in botany vol. 82. Eds. Cánovas, F. M., Lüttge, U., Risueño, M.-C., Pretzsch, H. (Springer International Publishing, Cham), 171–209. doi: 10.1007/124_2020_37
Demmig-Adams, B., Garab, G., Adams Iii, W., Govindjee (2014). Non-photochemical quenching and energy dissipation in plants, algae and cyanobacteria (Dordrecht: Springer Netherlands). doi: 10.1007/978-94-017-9032-1
Denness, L., McKenna, J. F., Segonzac, C., Wormit, A., Madhou, P., Bennett, M., et al. (2011). Cell wall damage-induced lignin biosynthesis is regulated by a reactive oxygen species- and jasmonic acid-dependent process in Arabidopsis. Plant Physiol. 156, 1364–1374. doi: 10.1104/pp.111.175737
De Souza, M. A., De Andrade, L. I. F., Gago, J., Pereira, E. G. (2024). Photoprotective mechanisms and higher photorespiration are key points for iron stress tolerance under heatwaves in rice. Plant Sci. 342, 112031. doi: 10.1016/j.plantsci.2024.112031
Dietz, K. J., Zörb, C., Geilfus, C. M. (2021). Drought and crop yield. Plant Biol. J. 23, 881–893. doi: 10.1111/plb.13304
Dimkpa, C. O., Singh, U., Bindraban, P. S., Elmer, W. H., Gardea-Torresdey, J. L., White, J. C. (2019). Zinc oxide nanoparticles alleviate drought-induced alterations in sorghum performance, nutrient acquisition, and grain fortification. Sci. Total Environ. 688, 926–934. doi: 10.1016/j.scitotenv.2019.06.392
Dinakar, C., Vishwakarma, A., Raghavendra, A. S., Padmasree, K. (2016). Alternative oxidase pathway optimizes photosynthesis during osmotic and temperature stress by regulating cellular ros, malate valve and antioxidative systems. Front. Plant Sci. 7. doi: 10.3389/fpls.2016.00068
Djanaguiraman, M., Belliraj, N., Bossmann, S., Prasad, P. (2018a). High-temperature stress alleviation by selenium nanoparticle treatment in grain sorghum. ACS Omega. 3, 2479–2491. doi: 10.1021/acsomega.7b01934
Djanaguiraman, M., Nair, R., Giraldo, J. P., Prasad, P. V. V. (2018b). Cerium oxide nanoparticles decrease drought-induced oxidative damage in sorghum leading to higher photosynthesis and grain yield. ACS Omega. 3, 14406–14416. doi: 10.1021/acsomega.8b01894
Dong, C., Ma, X., Huang, Y., Zhang, Y., Gao, X. (2022). Carbon dots nanozyme for anti-inflammatory therapy via scavenging intracellular reactive oxygen species. Front. Bioeng. Biotechnol. 10. doi: 10.3389/fbioe.2022.943399
Dourmap, C., Roque, S., Morin, A., Caubrière, D., Kerdiles, M., Béguin, K., et al. (2020). Stress signalling dynamics of the mitochondrial electron transport chain and oxidative phosphorylation system in higher plants. Ann. Bot. 125, 721–736. doi: 10.1093/aob/mcz184
Du, F., Hu, Z., Qin, L., Zhang, C., Wang, Z., Shi, Y., et al. (2023). Suppression of carotenoid cleavage dioxygenase 1 (NtCCD1) increases carotenoid contents and attenuates reactive oxygen species (ROS) in tobacco leaves. Plant Growth Regul. 100, 667–679. doi: 10.1007/s10725-023-00961-x
El-Beltagi, H. S., Sulaiman, M. E. M., Ullah, S., Shah, S. (2022). Effects of Ascorbic Acid and/or α-Tocopherol on agronomic and physio-biochemical traits of oat (Avena sativa L.) under drought condition. Agronomy. 12. doi: 10.3390/agronomy12102296
FAO (2023). Tracking progress on food and agriculture-related SDG indicators 2023. Rome. doi: 10.4060/cc7088en
Farajollahi, Z., Eisvand, H. R., Nazarian-Firouzabadi, F., Nasrollahi, A. H. (2023). Nano-Fe nutrition improves soybean physiological characteristics, yield, root features and water productivity in different planting dates under drought stress conditions. Ind. Crop Prod. 198, 116698. doi: 10.1016/j.indcrop.2023.116698
Foyer, C. H., Bloom, A. J., Queval, G., Noctor, G. (2009). Photorespiratory metabolism: genes, mutants, energetics, and redox signaling. Annu. Rev. Plant Biol. 60, 455–484. doi: 10.1146/annurev.arplant.043008.091948
Foyer, C. H., Noctor, G. (2011). Ascorbate and glutathione: The heart of the redox hub. Plant Physiol. 155, 2–18. doi: 10.1104/pp.110.167569
Foyer, C. H., Shigeoka, S. (2011). Understanding oxidative stress and antioxidant functions to enhance photosynthesis. Plant Physiol. 155, 93–100. doi: 10.1104/pp.110.166181
Fry, S. C. (2011). Plant cell walls. from chemistry to biology. Ann. Bot. 108, viii–viix. doi: 10.1093/aob/mcr128
Gakière, B., Fernie, A. R., Pétriacq, P. (2018). More to NAD+ than meets the eye: a regulator of metabolic pools and gene expression in Arabidopsis. Free Radic. Biol. Med. 122, 86–95. doi: 10.1016/j.freeradbiomed.2018.01.003
Gapper, C., Dolan, L. (2006). Control of plant development by reactive oxygen species. Plant Physiol. 141, 341–345. doi: 10.1104/pp.106.079079
Garmash, E. V. (2022). Signal pathways for regulation of plant alternative oxidase genes’ expression. Russ J. Plant Physl+. 69, 1. doi: 10.1134/S1021443722010058
Gautam, V., Kaur, R., Kohli, S. K., Verma, V., Kaur, P., Singh, R., et al. (2017). “ROS compartmentalization in plant cells under abiotic stress condition,” in Reactive oxygen species and antioxidant systems in plants: role and regulation under abiotic stress. Eds. Khan, M. I. R., Khan, N. A. (Singapore: Springer Singapore), 89–114. doi: 10.1007/978-981-10-5254-5_4
Giraldo, J. P., Landry, M. P., Faltermeier, S. M., McNicholas, T. P., Iverson, N. M., Boghossian, A. A., et al. (2014). Plant nanobionics approach to augment photosynthesis and biochemical sensing. Nat. Mater. 13, 400–408. doi: 10.1038/nmat3890
Halliwell, B. (2006). Phagocyte-derived reactive species: salvation or suicide? Trends Biochem. Sci. 31, 509–515. doi: 10.1016/j.tibs.2006.07.005
Hardeland, R. (2017). Taxon- and site-specific melatonin catabolism. Molecules. 22. doi: 10.3390/molecules22112015
Hasanuzzaman, M., Bhuyan, M. H. M. B., Anee, T. I., Parvin, K., Nahar, K., Mahmud, J. A., et al. (2019). Regulation of ascorbate-glutathione pathway in mitigating oxidative damage in plants under abiotic stress. Antioxidants. 8, 384. doi: 10.3390/antiox8090384
Hasanuzzaman, M., Hossain, M. A., Da Silva, J. A. T., Fujita, M. (2012). “Plant response and tolerance to abiotic oxidative stress: antioxidant defense is a key factor,” in Crop stress and its management: perspectives and strategies. Eds. Venkateswarlu, B., Shanker, A. K., Shanker, C., Maheswari, M. (Springer Netherlands, Dordrecht), 261–315. doi: 10.1007/978-94-007-2220-0_8
Hernández, I., Alegre, L., Van Breusegem, F., Munné-Bosch, S. (2009). How relevant are flavonoids as antioxidants in plants. Trends Plant Sci. 14, 125–132. doi: 10.1016/j.tplants.2008.12.003
Hernández, I., Cela, J., Alegre, L., Munné-Bosch, S. (2012). “Antioxidant defenses against drought stress,” in Plant responses to drought stress. Ed. Aroca, R. (Springer Berlin Heidelberg, Berlin, Heidelberg), 231–258. doi: 10.1007/978-3-642-32653-0_9
Herrera-Vásquez, A., Salinas, P., Holuigue, L. (2015). Salicylic acid and reactive oxygen species interplay in the transcriptional control of defense genes expression. Front. Plant Sci. 6. doi: 10.3389/fpls.2015.00171
Hevia, D., Mayo, J. C., Tan, D.-X., Rodriguez-Garcia, A., Sainz, R. M. (2014). Melatonin enhances photo-oxidation of 2′,7′-dichlorodihydrofluorescein by an antioxidant reaction that renders N1-acetyl-N2-formyl-5-methoxykynuramine (AFMK). PloS One 9, e109257. doi: 10.1371/journal.pone.0109257
Hideg, E., Kos, P. B., Schreiber, U. (2008). Imaging of NPQ and ROS formation in tobacco leaves: heat inactivation of the water-water cycle prevents down-regulation of PSII. Plant Cell Physiol. 49, 1879–1886. doi: 10.1093/pcp/pcn170
Hinojosa, L., Sanad, M. N. M. E., Jarvis, D. E., Steel, P., Murphy, K., Smertenko, A. (2019). Impact of heat and drought stress on peroxisome proliferation in quinoa. Plant J. 99, 1144–1158. doi: 10.1111/tpj.14411
Hoson, T. (1998). Apoplast as the site of response to environmental signals. J. Plant Res. 111, 167–177. doi: 10.1007/BF02507163
Hossain, Z., Mustafa, G., Komatsu, S. (2015). Plant responses to nanoparticle stress. IJMS. 16, 26644–26653. doi: 10.3390/ijms161125980
Hu, S., Ding, Y., Zhu, C. (2020b). Sensitivity and responses of chloroplasts to heat stress in plants. Front. Plant Sci. 11. doi: 10.3389/fpls.2020.00375
Hu, C. H., Wang, P. Q., Zhang, P. P., Nie, X. M., Li, B. B., Tai, L., et al. (2020a). NADPH oxidases: the vital performers and center hubs during plant growth and signaling. Cells. 9, 437. doi: 10.3390/cells9020437
Hu, J., Zhao, X., Gu, L., Liu, P., Zhao, B., Zhang, J., et al. (2023). The effects of high temperature, drought, and their combined stresses on the photosynthesis and senescence of summer maize. AGR Water Manage. 289, 108525. doi: 10.1016/j.agwat.2023.108525
Huang, W., Yang, Y. J., Zhang, S. B. (2019). The role of water-water cycle in regulating the redox state of photosystem I under fluctuating light. Biochim. Biophys. Acta Bioenerg. 1860, 383–390. doi: 10.1016/j.bbabio.2019.03.007
Hussain, S., Rao, M. J., Anjum, M. A., Ejaz, S., Zakir, I., Ali, M. A., et al. (2019). “Oxidative stress and antioxidant defense in plants under drought conditions,” in Plant abiotic stress tolerance. Eds. Hasanuzzaman, M., Hakeem, K. R., Nahar, K., Alharby, H. F. (Springer International Publishing, Cham), 207–219. doi: 10.1007/978-3-030-06118-0_9
Impa, S. M., Nadaradjan, S., Jagadish, S. V. K. (2012). “Drought stress induced reactive oxygen species and anti-oxidants in plants,” in Abiotic stress responses in plants. Eds. Ahmad, P., Prasad, M. N. V. (Springer New York, New York, NY), 131–147. doi: 10.1007/978-1-4614-0634-1_7
Innocenzi, P., Stagi, L. (2023). Carbon dots as oxidant-antioxidant nanomaterials, understanding the structure-properties relationship. A critical review. Nano Today 50, 101837. doi: 10.1016/j.nantod.2023.101837
Iqbal, M., Raja, N. I., Mashwani, Z.-U.-R., Hussain, M., Ejaz, M., Yasmeen, F. (2019). Effect of silver nanoparticles on growth of wheat under heat stress. Iran J. Sci. Technol. Trans. Sci. 43, 387–395. doi: 10.1007/s40995-017-0417-4
Jaberzadeh, A., Moaveni, P., Tohidi Moghadam, H. R., Zahedi, H. (2013). Influence of bulk and nanoparticles titanium foliar application on some agronomic traits, seed gluten and starch contents of wheat subjected to water deficit stress. NOT Bot. HORTI AGROBO. 41, 201. doi: 10.15835/nbha4119093
Jammes, F., Song, C., Shin, D., Munemasa, S., Takeda, K., Gu, D., et al. (2009). MAP kinases MPK9 and MPK12 are preferentially expressed in guard cells and positively regulate ROS-mediated ABA signaling. Proc. Natl. Acad. Sci. U.S.A. 106, 20520–20525. doi: 10.1073/pnas.0907205106
Ji, D., Li, Q., Guo, Y., An, W., Manavski, N., Meurer, J., et al. (2022). NADP+ supply adjusts the synthesis of photosystem I in Arabidopsis chloroplasts. Plant Physiol. 189, 2128–2143. doi: 10.1093/plphys/kiac161
Jiang, Y., Feng, X., Wang, H., Chen, Y., Sun, Y. (2021). Heat-induced down-regulation of photosystem II protects photosystem I in honeysuckle (Lonicera japonica). J. Plant Res. 134, 1311–1321. doi: 10.1007/s10265-021-01336-x
Jonak, C. (2002). Complexity, cross talk and integration of plant MAP kinase signalling. Curr. Opin. Plant Biol. 5, 415–424. doi: 10.1016/S1369-5266(02)00285-6
Jubany-Mari, T., Munne-Bosch, S., Lopez-Carbonell, M., Alegre, L. (2008). Hydrogen peroxide is involved in the acclimation of the mediterranean shrub, Cistus albidus L., to summer drought. J. Exp. Bot. 60, 107–120. doi: 10.1093/jxb/ern274
Kaya, C., Higgs, D., Ashraf, M., AlYemeni, M. N., Ahmad, P. (2020). Integrative roles of nitric oxide and hydrogen sulfide in melatonin-induced tolerance of pepper (Capsicum Annuum L.) plants to iron deficiency and salt stress alone or in combination. Physiol. Plant 168, 256–277. doi: 10.1111/ppl.12976
Khan, M. N. (2023). Melatonin regulates mitochondrial enzymes and ascorbate–glutathione system during plant responses to drought stress through involving endogenous calcium. S AFR J. Bot. 162, 622–632. doi: 10.1016/j.sajb.2023.09.032
Khan, M. N., Li, Y., Khan, Z., Chen, L., Liu, J., Hu, J., et al. (2021). Nanoceria seed priming enhanced salt tolerance in rapeseed through modulating ROS homeostasis and α-amylase activities. J. Nanobiotechnol. 19, 276. doi: 10.1186/s12951-021-01026-9
Khan, M. N., Siddiqui, M. H., Mukherjee, S., AlSolami, M. A., Alhussaen, K. M., AlZuaibr, F. M., et al. (2023). Melatonin involves hydrogen sulfide in the regulation of H+-ATPase activity, nitrogen metabolism, and ascorbate-glutathione system under chromium toxicity. Environ. pollut. 323, 121173. doi: 10.1016/j.envpol.2023.121173
Khorobrykh, S., Havurinne, V., Mattila, H., Tyystjärvi, E. (2020). Oxygen and ROS in photosynthesis. Plants. 9, 91. doi: 10.3390/plants9010091
Koch, W., Kwart, M., Laubner, M., Heineke, D., Stransky, H., Frommer, W. B., et al. (2003). Reduced amino acid content in transgenic potato tubers due to antisense inhibition of the leaf H+/amino acid symporter StAAP1. Plant J. 33, 211–220. doi: 10.1046/j.1365-313X.2003.01618.x
Kolupaev, Y., Karpets, V., Kabashnikova, L. F. (2019). Antioxidative system of plants: cellular compartmentalization, protective and signaling functions, mechanisms of regulation (Review). Appl. Biochem. Microbiol. 55, 441–459. doi: 10.1134/S0003683819050089
Kolupaev, Y., Yastreb, T. O., Ryabchun, N. I., Kokorev, A. I., Kolomatska, V. P., Dmitriev, A. P. (2023). Redox homeostasis of cereals during acclimation to drought. Theor. Exp. Plant Physiol. 35, 133–168. doi: 10.1007/s40626-023-00271-7
Kozuleva, M. A., Ivanov, B. N., Vetoshkina, D. V., Borisova-Mubarakshina, M. M. (2020a). Minimizing an electron flow to molecular oxygen in photosynthetic electron transfer chain: an evolutionary view. Front. Plant Sci. 11. doi: 10.3389/fpls.2020.00211
Kozuleva, M., Petrova, A., Milrad, Y., Semenov, A., Ivanov, B., Redding, K. E., et al. (2020b). The Mehler reaction site is the phylloquinone within Photosystem I. Plant Biol. (Stuttg). 2020–08. doi: 10.1101/2020.08.13.249367
Kranner, I., Roach, T., Beckett, R. P., Whitaker, C., Minibayeva, F. V. (2010). Extracellular production of reactive oxygen species during seed germination and early seedling growth in Pisum sativum. J. Plant Physiol. 167, 805–811. doi: 10.1016/j.jplph.2010.01.019
Kruk, J., Holländer-Czytko, H., Oettmeier, W., Trebst, A. (2005). Tocopherol as singlet oxygen scavenger in photosystem II. J. Plant Physiol. 162, 749–757. doi: 10.1016/j.jplph.2005.04.020
Kumari, A., Pathak, P. K., Bulle, M., Igamberdiev, A. U., Gupta, K. J. (2019). Alternative oxidase is an important player in the regulation of nitric oxide levels under normoxic and hypoxic conditions in plants. J. Exp. Bot. 70, 4345–4354. doi: 10.1093/jxb/erz160
Kuppusamy, A., Alagarswamy, S., Karuppusami, K. M., Maduraimuthu, D., Natesan, S., Ramalingam, K., et al. (2023). Melatonin enhances the photosynthesis and antioxidant enzyme activities of mung bean under drought and high-temperature stress conditions. Plants. 12. doi: 10.3390/plants12132535
Kusano, T., Berberich, T., Tateda, C., Takahashi, Y. (2008). Polyamines: essential factors for growth and survival. Planta. 228, 367–381. doi: 10.1007/s00425-008-0772-7
Kusano, T., Yamaguchi, K., Berberich, T., Takahashi, Y. (2007). Advances in polyamine research in 2007. J. Plant Res. 120, 345–350. doi: 10.1007/s10265-007-0074-3
Kwak, J. M. (2003). NADPH oxidase AtrbohD and AtrbohF genes function in ROS-dependent ABA signaling in Arabidopsis. EMBO J. 22, 2623–2633. doi: 10.1093/emboj/cdg277
Laxa, M., Liebthal, M., Telman, W., Chibani, K., Dietz, K.-J. (2019). The role of the plant antioxidant system in drought tolerance. Antioxidants. 8, 94. doi: 10.3390/antiox8040094
Li, M., Gao, L., White, J. C., Haynes, C. L., O’Keefe, T. L., Rui, Y., et al. (2023). Nano-enabled strategies to enhance biological nitrogen fixation. Nat. Nanotechnol. 18, 688–691. doi: 10.1038/s41565-023-01392-5
Li, Y., Li, W., Yang, X., Kang, Y., Zhang, H., Liu, Y., et al. (2021). Salvia miltiorrhiza -derived carbon dots as scavengers of reactive oxygen species for reducing oxidative damage of plants. ACS Appl. Nano Mater. 4, 113–120. doi: 10.1021/acsanm.0c02419
Li, Y., Liu, J., Fu, C., Khan, M. N., Hu, J., Zhao, F., et al. (2022). CeO2 nanoparticles modulate Cu–Zn superoxide dismutase and lipoxygenase-IV isozyme activities to alleviate membrane oxidative damage to improve rapeseed salt tolerance. Environ. Sci.: Nano. 9, 1116–1132. doi: 10.1039/D1EN00845E
Li, J. L. Y., Sulaiman, M., Beckett, R. P., Minibayeva, F. V. (2010). Cell wall peroxidases in the liverwort Dumortiera hirsuta are responsible for extracellular superoxide production, and can display tyrosinase activity. Physiol. Plantarum. 138, 474–484. doi: 10.1111/j.1399-3054.2009.01318.x
Lippmann, R., Babben, S., Menger, A., Delker, C., Quint, M. (2019). Development of wild and cultivated plants under global warming conditions. Curr. Biol. 29, R1326–R1338. doi: 10.1016/j.cub.2019.10.016
Liu, J., Fu, C., Li, G., Khan, M. N., Wu, H. (2021a). ROS homeostasis and plant salt tolerance: Plant nanobiotechnology updates. Sustainability 13, 3552. doi: 10.3390/su13063552
Liu, J., Gu, J., Hu, J., Ma, H., Tao, Y., Li, G., et al. (2023). Use of Mn3O4 nanozyme to improve cotton salt tolerance. Plant Biotechnol. J. 21, 1935–1937. doi: 10.1111/pbi.14145
Liu, J., Hasanuzzaman, M., Wen, H., Zhang, J., Peng, T., Sun, H., et al. (2019). High temperature and drought stress cause abscisic acid and reactive oxygen species accumulation and suppress seed germination growth in rice. Protoplasma. 256, 1217–1227. doi: 10.1007/s00709-019-01354-6
Liu, J., Li, G., Chen, L., Gu, J., Wu, H., Li, Z. (2021). Cerium oxide nanoparticles improve cotton salt tolerance by enabling better ability to maintain cytosolic K+/Na+ ratio. J. Nanobiotechnol. 19, 153. doi: 10.1186/s12951-021-00892-7
Liu, W.-J., Liu, H., Chen, Y.-E., Yin, Y., Zhang, Z.-W., Song, J., et al. (2022). Chloroplastic photoprotective strategies differ between bundle sheath and mesophyll cells in maize (Zea mays L.) Under drought. Front. Plant Sci. 13. doi: 10.3389/fpls.2022.885781
Liu, Y., Zhou, M., Gao, Z., Ren, W., Yang, F., He, H., et al. (2015). RNA-Seq analysis reveals MAPKKK family members related to drought tolerance in maize. PloS One 10, e0143128. doi: 10.1371/journal.pone.0143128
Livanos, P., Apostolakos, P., Galatis, B. (2012). Plant cell division: ROS homeostasis is required. Plant Signal Behav. 7, 771–778. doi: 10.4161/psb.20530
López, R., Ramírez-Valiente, J. A., Pita, P. (2022). How plants cope with heatwaves in a drier environment. Flora. 295, 152148. doi: 10.1016/j.flora.2022.152148
Lu, L., Huang, M., Huang, Y., Corvini, P. F. X., Ji, R., Zhao, L. (2020). Mn 3O4 nanozymes boost endogenous antioxidant metabolites in cucumber (Cucumis sativus) plant and enhance resistance to salinity stress. Environ. Sci.: Nano 7, 1692–1703. doi: 10.1039/D0EN00214C
Luna, C. M. (2004). Drought controls on H2O2 accumulation, catalase (CAT) activity and CAT gene expression in wheat. J. Exp. Bot. 56, 417–423. doi: 10.1093/jxb/eri039
Ma, X., Wang, W., Bittner, F., Schmidt, N., Berkey, R., Zhang, L., et al. (2016). Dual and opposing roles of xanthine dehydrogenase in defense-associated reactive oxygen species metabolism in Arabidopsis. Plant Cell. 28, 1108–1126. doi: 10.1105/tpc.15.00880
Madhu, S. A., Kaur, A., Singh, K., Upadhyay, S. K. (2024). Modulation in gene expression and enzyme activity suggested the roles of monodehydroascorbate reductase in development and stress response in bread wheat. Plant Sci. 338, 111902. doi: 10.1016/j.plantsci.2023.111902
Mai, Y., Ren, Y., Deng, S., Ashraf, U., Tang, X., Duan, M., et al. (2024). Influence of ZnO nanoparticles on early growth stage of fragrant rice at low temperature (LT) stress. J. Soil Sci. Plant Nutr. 24, 1301–1317. doi: 10.1007/s42729-024-01632-0
Manzoor, N., Ahmed, T., Noman, M., Shahid, M., Nazir, M. M., Ali, L., et al. (2021). Iron oxide nanoparticles ameliorated the cadmium and salinity stresses in wheat plants, facilitating photosynthetic pigments and restricting cadmium uptake. Sci. Total Environ. 769, 145221. doi: 10.1016/j.scitotenv.2021.145221
Marchin, R. M., Backes, D., Ossola, A., Leishman, M. R., Tjoelker, M. G., Ellsworth, D. S. (2022). Extreme heat increases stomatal conductance and drought-induced mortality risk in vulnerable plant species. Glob Chang Biol. 28, 1133–1146. doi: 10.1111/gcb.15976
Marino, D., Dunand, C., Puppo, A., Pauly, N. (2012). A burst of plant NADPH oxidases. Trends Plant Sci. 17, 9–15. doi: 10.1016/j.tplants.2011.10.001
Maxwell, D. P., Wang, Y., McIntosh, L. (1999). The alternative oxidase lowers mitochondrial reactive oxygen production in plant cells. Proc. Natl. Acad. Sci. U.S.A. 96, 8271–8276. doi: 10.1073/pnas.96.14.8271
Miller, G., Schlauch, K., Tam, R., Cortes, D., Torres, M. A., Shulaev, V., et al. (2009). The plant NADPH oxidase RBOHD mediates rapid systemic signaling in response to diverse stimuli. Sci. Signal. 2, ra45–ra45. doi: 10.1126/scisignal.2000448
Minibayeva, F., Kolesnikov, O., Chasov, A., Beckett, R. P., Lüthje, S., Vylegzhanina, N., et al. (2009). Wound-induced apoplastic peroxidase activities: their roles in the production and detoxification of reactive oxygen species. Plant Cell Environ. 32, 497–508. doi: 10.1111/j.1365-3040.2009.01944.x
Mittler, R. (2002). Oxidative stress, antioxidants and stress tolerance. Trends Plant Sci. 7, 405–410. doi: 10.1016/S1360-1385(02)02312-9
Mittler, R., Blumwald, E. (2015). The roles of ROS and ABA in systemic acquired acclimation. Plant Cell. 27, 64–70. doi: 10.1105/tpc.114.133090
Mittler, R., Vanderauwera, S., Gollery, M., Van Breusegem, F. (2004). Reactive oxygen gene network of plants. Trends Plant Sci. 9, 490–498. doi: 10.1016/j.tplants.2004.08.009
Mittler, R., Zandalinas, S. I., Fichman, Y., Van Breusegem, F. (2022). Reactive oxygen species signalling in plant stress responses. Nat. Rev. Mol. Cell Biol. 23, 663–679. doi: 10.1038/s41580-022-00499-2
Mohi-Ud-Din, M., Siddiqui, N., Rohman, M., Jagadish, S. V. K., Ahmed, J. U., Hassan, M. M., et al. (2021). Physiological and biochemical dissection reveals a trade-off between antioxidant capacity and heat tolerance in bread wheat (Triticum aestivum L.). Antioxidants 10, 351. doi: 10.3390/antiox10030351
Moschou, P. N., Paschalidis, K. A., Delis, I. D., Andriopoulou, A. H., Lagiotis, G. D., Yakoumakis, D. I., et al. (2008). Spermidine exodus and oxidation in the apoplast induced by abiotic stress is responsible for H2O2 signatures that direct tolerance responses in tobacco. Plant Cell. 20, 1708–1724. doi: 10.1105/tpc.108.059733
Muhammad, I., Yang, L., Ahmad, S., Farooq, S., Khan, A., Muhammad, N., et al. (2023). Melatonin-priming enhances maize seedling drought tolerance by regulating the antioxidant defense system. Plant Physiol. 191, 2301–2315. doi: 10.1093/plphys/kiad027
Murali, M., Gowtham, H. G., Singh, S. B., Shilpa, N., Aiyaz, M., Alomary, M. N., et al. (2022). Fate, bioaccumulation and toxicity of engineered nanomaterials in plants: Current challenges and future prospects. Sci. Total Environ. 811, 152249. doi: 10.1016/j.scitotenv.2021.152249
Nauman Khan, M., Fu, C., Liu, X., Li, Y., Yan, J., Yue, L., et al. (2024). Nanopriming with selenium doped carbon dots improved rapeseed germination and seedling salt tolerance. Crop J. S2214514124000734. doi: 10.1016/j.cj.2024.03.007
Navrot, N., Rouhier, N., Gelhaye, E., Jacquot, J. (2007). Reactive oxygen species generation and antioxidant systems in plant mitochondria. Physiol. Plant 129, 185–195. doi: 10.1111/j.1399-3054.2006.00777.x
Noctor, G. (2002). Drought and oxidative load in the leaves of C3 plants: a predominant role for photorespiration? Ann. Botany. 89, 841–850. doi: 10.1093/aob/mcf096
Omar, A. A., Heikal, Y. M., Zayed, E. M., Shamseldin, S. A. M., Salama, Y. E., Amer, K. E., et al. (2023). Conferring of drought and heat stress tolerance in wheat (Triticum aestivum L.) genotypes and their response to selenium nanoparticles application. Nanomaterials. 13, 998. doi: 10.3390/nano13060998
Petrov, V., Hille, J., Mueller-Roeber, B., Gechev, T. S. (2015). ROS-mediated abiotic stress-induced programmed cell death in plants. Front. Plant Sci. 6. doi: 10.3389/fpls.2015.00069
Podgórska, A., Burian, M., Szal, B. (2017). Extra-cellular but extra-ordinarily important for cells: apoplastic reactive oxygen species metabolism. Front. Plant Sci. 8. doi: 10.3389/fpls.2017.01353
Pospíšil, P. (2009). Production of reactive oxygen species by photosystem II. Biochim. Biophys. Acta 1787, 1151–1160. doi: 10.1016/j.bbabio.2009.05.005
Prakash, V., Peralta-Videa, J., Tripathi, D. K., Ma, X., Sharma, S. (2021). Recent insights into the impact, fate and transport of cerium oxide nanoparticles in the plant-soil continuum. Ecotox Environ. Safe. 221, 112403. doi: 10.1016/j.ecoenv.2021.112403
Qi, J., Wang, J., Gong, Z., Zhou, J.-M. (2017). Apoplastic ROS signaling in plant immunity. Curr. Opin. Plant Biol. 38, 92–100. doi: 10.1016/j.pbi.2017.04.022
Qi, F., Zhang, F. (2020). Cell cycle regulation in the plant response to stress. Front. Plant Sci. 10. doi: 10.3389/fpls.2019.01765
Qian, Y., Cheng, Z., Meng, J., Tao, J., Zhao, D. (2023). PlMAPK1 facilitates growth and photosynthesis of herbaceous peony (Paeonia lactiflora Pall.) under high-temperature stress. Sci. Hortic. 310, 111701. doi: 10.1016/j.scienta.2022.111701
Rajput, V. D., Harish, R. K., Verma, K. K., Sharma, L., Quiroz-Figueroa, F. R., et al. (2021). Recent developments in enzymatic antioxidant defence mechanism in plants with special reference to abiotic stress. Biology. 10, 267. doi: 10.3390/biology10040267
Ribeiro, C. W., Korbes, A. P., Garighan, J. A., Jardim-Messeder, D., Carvalho, F. E. L., Sousa, R. H. V., et al. (2017). ). Rice peroxisomal ascorbate peroxidase knockdown affects ROS signaling and triggers early leaf senescence. Plant Sci. 263, 55–65. doi: 10.1016/j.plantsci.2017.07.009
Río, L. A., Sandalio, L. M., Corpas, F. J., Palma, J. M., Barroso, J. B. (2006). Reactive oxygen species and reactive nitrogen species in peroxisomes. production, scavenging, and role in cell signaling. Plant Physiol. 141, 330–335. doi: 10.1104/pp.106.078204
Rizhsky, L., Liang, H., Mittler, R. (2003). The water-water cycle is essential for chloroplast protection in the absence of stress. J. Biol. Chem. 278, 38921–38925. doi: 10.1074/jbc.M304987200
Roach, T., Krieger-Liszkay, A. (2014). Regulation of photosynthetic electron transport and photoinhibition. Curr. Protein Pept. Sci. 15, 351–362. doi: 10.2174/1389203715666140327105143
Sagi, M., Fluhr, R. (2006). Production of reactive oxygen species by plant NADPH oxidases. Plant Physiol. 141, 336–340. doi: 10.1104/pp.106.078089
Saha, J., Brauer, E. K., Sengupta, A., Popescu, S. C., Gupta, K., Gupta, B. (2015). Polyamines as redox homeostasis regulators during salt stress in plants. Front. Environ. Sci. 3. doi: 10.3389/fenvs.2015.00021
Saini, D. (2023). Suppression of photorespiratory metabolism by low O2 and presence of aminooxyacetic acid induces oxidative stress in Arabidopsis thaliana leaves. Physiol. Mol. Biol. Plants. 29, 1851–1861. doi: 10.1007/s12298-023-01388-4
Sarker, U., Oba, S. (2018). Catalase, superoxide dismutase and ascorbate-glutathione cycle enzymes confer drought tolerance of Amaranthus tricolor. Sci. Rep. 8, 16496. doi: 10.1038/s41598-018-34944-0
Sattelmacher, B. (2001). The apoplast and its significance for plant mineral nutrition. New Phytol. 149, 167–192. doi: 10.1046/j.1469-8137.2001.00034.x
Serag, M. F., Kaji, N., Tokeshi, M., Baba, Y. (2012). Introducing carbon nanotubes into living walled plant cells through cellulase-induced nanoholes. RSC Adv. 2, 398–400. doi: 10.1039/C1RA00760B
Shen, Y., Li, J., Gu, R., Yue, L., Wang, H., Zhan, X., et al. (2018). Carotenoid and superoxide dismutase are the most effective antioxidants participating in ROS scavenging in phenanthrene accumulated wheat leaf. Chemosphere. 197, 513–525. doi: 10.1016/j.chemosphere.2018.01.036
Shen, L., Zhou, Y., Yang, X. (2024). Genome-wide identification of ascorbate peroxidase (APX) gene family and the function of SmAPX2 under high temperature stress in eggplant. Sci. Hortic. 326, 112744. doi: 10.1016/j.scienta.2023.112744
Shi, N., Bai, T., Wang, X., Tang, Y., Wang, C., Zhao, L. (2022). Toxicological effects of WS2 nanomaterials on rice plants and associated soil microbes. Sci. Total Environ. 832, 154987. doi: 10.1016/j.scitotenv.2022.154987
Shi, J., Peng, C., Yang, Y., Yang, J., Zhang, H., Yuan, X., et al. (2014). Phytotoxicity and accumulation of copper oxide nanoparticles to the Cu-tolerant plant Elsholtzia splendens. Nanotoxicology. 8, 179–188. doi: 10.3109/17435390.2013.766768
Singh, S. (2019). Nanomaterials exhibiting enzyme-like properties (Nanozymes): current advances and future perspectives. Front. Chem. 7. doi: 10.3389/fchem.2019.00046
Somal, M. K., Sachan, R. S. K., Bhagat, D., Khusbhoo, B. R., Kumar, M. (2023). “An update on reactive oxygen species synthesis and its potential application,” in Reactive oxygen species. Eds. Faizan, M., Hayat, S., Ahmed, S. M. (Springer Nature Singapore, Singapore), 1–15. doi: 10.1007/978-981-19-9794-5_1
Sousa, B., Rodrigues, F., Soares, C., Martins, M., Azenha, M., Lino-Neto, T., et al. (2022). Impact of combined heat and salt stresses on tomato plants—insights into nutrient uptake and redox homeostasis. Antioxidants. 11, 478. doi: 10.3390/antiox11030478
Spicher, L., Almeida, J., Gutbrod, K., Pipitone, R., Dörmann, P., Glauser, G., et al. (2017). Essential role for phytol kinase and tocopherol in tolerance to combined light and temperature stress in tomato. J. Exp. Bot. 68, 5845–5856. doi: 10.1093/jxb/erx356
Steffens, B. (2014). The role of ethylene and ROS in salinity, heavy metal, and flooding responses in rice. Front. Plant Sci. 5. doi: 10.3389/fpls.2014.00685
Sun, G., Dong, Z., Li, G., Yuan, H., Liu, J., Yao, X., et al. (2023). Mn3O4 Nanoparticles alleviate ROS-inhibited root apex mitosis activities to improve maize drought tolerance. Adv. Biol. (Weinh). 7, 2200317. doi: 10.1002/adbi.202200317
Sun, L., Li, Y., Miao, W., Piao, T., Hao, Y., Hao, F.-S. (2017). NADK2 positively modulates abscisic acid-induced stomatal closure by affecting accumulation of H2O2, Ca2+ and nitric oxide in Arabidopsis guard cells. Plant Sci. 262, 81–90. doi: 10.1016/j.plantsci.2017.06.003
Sun, H., Yang, Y. J., Huang, W. (2020). The water-water cycle is more effective in regulating redox state of photosystem I under fluctuating light than cyclic electron transport. Biochim. Biophys. Acta Bioenerg. 1861, 148235. doi: 10.1016/j.bbabio.2020.148235
Suzuki, N. (2023). Fine tuning of ROS, redox and energy regulatory systems associated with the functions of chloroplasts and mitochondria in plants under heat stress. Int. J. Mol. Sci. 24. doi: 10.3390/ijms24021356
Suzuki, N., Mittler, R. (2006). Reactive oxygen species and temperature stresses: A delicate balance between signaling and destruction. Physiologia Plantarum 126, 45–51. doi: 10.1111/j.0031-9317.2005.00582.x
Takahashi, M., Asada, K. (1988). Superoxide production in aprotic interior of chloroplast thylakoids. Arch. Biochem. Biophys. 267 (2), 714–722. doi: 10.1016/0003-9861(88)90080-x
Tan, Y.-F., O’Toole, N., Taylor, N. L., Millar, A. H. (2010). Divalent metal ions in plant mitochondria and their role in interactions with proteins and oxidative stress-induced damage to respiratory function. Plant Physiol. 152, 747–761. doi: 10.1104/pp.109.147942
Tewari, R. K., Kumar, P., Kim, S., Hahn, E. J., Paek, K. Y. (2009). Nitric oxide retards xanthine oxidase-mediated superoxide anion generation in Phalaenopsis flower: an implication of NO in the senescence and oxidative stress regulation. Plant Cell Rep. 28, 267–279. doi: 10.1007/s00299-008-0632-8
Tiew, T. W.-Y., Sheahan, M. B., Rose, R. J. (2015). Peroxisomes contribute to reactive oxygen species homeostasis and cell division induction in Arabidopsis protoplasts. Front. Plant Sci. 6. doi: 10.3389/fpls.2015.00658
Timm, S., Hagemann, M. (2020). Photorespiration—how is it regulated and how does it regulate overall plant metabolism? J. Exp. Bot. 71, 3955–3965. doi: 10.1093/jxb/eraa183
Tiwari, S., Tiwari, S., Singh, M., Singh, A., Prasad, S. M. (2017). “Generation mechanisms of reactive oxygen species in the plant cell: an overview,” in Reactive oxygen species in plants. Eds. Singh, V. P., Singh, S., Tripathi, D. K., Prasad, S. M., Chauhan, D. K.. (Hoboken: John Wiley & Sons Inc), 1–22. doi: 10.1002/9781119324928.ch1
Tiwari, Y. K., Yadav, S. K. (2020). Effect of high-temperature stress on ascorbate–glutathione cycle in maize. Agric. Res. 9, 179–187. doi: 10.1007/s40003-019-00421-x
Verma, R., Kaur, J. (2021). Expression of barley oxalate oxidase confers resistance against sclerotinia sclerotiorum in transgenic brassica juncea cv varuna. Transgenic Res. 30, 143–154. doi: 10.1007/s11248-021-00234-1
Verma, G., Srivastava, D., Tiwari, P., Chakrabarty, D. (2019). “ROS modulation in crop plants under drought stress,” in Reactive oxygen, nitrogen and sulfur species in plants. Eds. Hasanuzzaman, M., Fotopoulos, V., Nahar, K., Fujita, M.. (Hoboken: John Wiley & Sons Inc), 311–336. doi: 10.1002/9781119468677.ch13
Vielma, J. R., Bonilla, E., Chacín-Bonilla, L., Mora, M., Medina-Leendertz, S., Bravo, Y. (2014). Effects of melatonin on oxidative stress, and resistance to bacterial, parasitic, and viral infections: A review. Acta Trop. 137, 31–38. doi: 10.1016/j.actatropica.2014.04.021
Wang, C., Ji, Y., Cao, X., Yue, L., Chen, F., Li, J., et al. (2022c). Carbon dots improve nitrogen bioavailability to promote the growth and nutritional quality of soybeans under drought stress. ACS Nano. 16, 12415–12424. doi: 10.1021/acsnano.2c03591
Wang, X., Li, B. B., Ma, T. T., Sun, L. Y., Tai, L., Hu, C. H., et al. (2020). The NAD kinase OsNADK1 affects the intracellular redox balance and enhances the tolerance of rice to drought. BMC Plant Biol. 20, 11. doi: 10.1186/s12870-019-2234-8
Wang, Y., Li, M., Ying, J., Shen, J., Dou, D., Yin, M., et al. (2023). High-efficiency green management of potato late blight by a self-assembled multicomponent nano-bioprotectant. Nat. Commun. 14. doi: 10.1038/s41467-023-41447-8
Wang, D., Saleh, N. B., Byro, A., Zepp, R., Sahle-Demessie, E., Luxton, T. P., et al. (2022b). Nano-enabled pesticides for sustainable agriculture and global food security. Nat. Nanotechnol. 17, 347–360. doi: 10.1038/s41565-022-01082-8
Wang, Z., Shen, X., Gao, X. (2021). Density functional theory mechanistic insight into the peroxidase- and oxidase-like activities of nanoceria. J. Phys. Chem. C 125, 23098–23104. doi: 10.1021/acs.jpcc.1c04878
Wang, J., Vanlerberghe, G. C. (2013). A lack of mitochondrial alternative oxidase compromises capacity to recover from severe drought stress. Physiologia Plantarum 149, 461–473. doi: 10.1111/ppl.12059
Wang, Z., Wang, Y., Wang, Y., Li, H., Wen, Z., Hou, X. (2022d). HPR1 is required for high light intensity induced photorespiration in arabidopsis thaliana. Int. J. Mol. Sci. 23. doi: 10.3390/ijms23084444
Wang, B., Wang, Y., Yu, W., Wang, L., Lan, Q., Wang, Y., et al. (2022a). Knocking out the Transcription factor OsNAC092 promoted rice drought tolerance. Biology. 11doi: 10.3390/biology11121830
Wang, M., Zhang, Y., Zhu, C., Yao, X., Zheng, Z., Tian, Z., et al. (2021). EkFLS overexpression promotes flavonoid accumulation and abiotic stress tolerance in plant. Physiol. Plant 172, 1966–1982. doi: 10.1111/ppl.13407
Wu, S.-Y., Hou, L.-L., Zhu, J., Wang, Y.-C., Zheng, Y.-L., Hou, J.-Q., et al. (2023b). Ascorbic acid-mediated reactive oxygen species homeostasis modulates the switch from tapetal cell division to cell differentiation in Arabidopsis. Plant Cell. 35, 1474–1495. doi: 10.1093/plcell/koad037
Wu, B., Qi, F., Liang, Y. (2023a). Fuels for ROS signaling in plant immunity. Trends Plant Sci. 28, 1124–1131. doi: 10.1016/j.tplants.2023.04.007
Wu, H., Shabala, L., Shabala, S., Giraldo, J. P. (2018). Hydroxyl radical scavenging by cerium oxide nanoparticles improves Arabidopsis salinity tolerance by enhancing leaf mesophyll potassium retention. Environ. Sci. Nano. 5, 1567–1583. doi: 10.1039/C8EN00323H
Wu, H., Tito, N., Giraldo, J. P. (2017). Anionic cerium oxide nanoparticles protect plant photosynthesis from abiotic stress by scavenging reactive oxygen species. ACS Nano. 11, 11283–11297. doi: 10.1021/acsnano.7b05723
Wu, J., Wang, X., Wang, Q., Lou, Z., Li, S., Zhu, Y., et al. (2019). Nanomaterials with enzyme-like characteristics (nanozymes): next-generation artificial enzymes (II). Chem. Soc Rev. 48, 1004–1076. doi: 10.1039/C8CS00457A
Yadav, L. P., Gangadhara, K., Apparao, V. V. (2022). Evaluation of moringa oleifera (drumstick) variety thar harsha under rainfed semi-arid conditions for growth, yield and quality along with antioxidant potentiality and nutrient content. S AFR J. Bot. 148, 112–122. doi: 10.1016/j.sajb.2022.04.005
Yang, K.-Y., Doxey, S., McLean, J. E., Britt, D., Watson, A., Al Qassy, D., et al. (2018). Remodeling of root morphology by CuO and ZnO nanoparticles: effects on drought tolerance for plants colonized by a beneficial pseudomonad. Botany. 96, 175–186. doi: 10.1139/cjb-2017-0124
Yu, Y., Deng, L., Zhou, L., Chen, G., Wang, Y. (2022). Exogenous melatonin activates antioxidant systems to increase the ability of rice seeds to germinate under high temperature conditions. Plants. 11, 886. doi: 10.3390/plants11070886
Yuan, B., Tan, Z., Guo, Q., Shen, X., Zhao, C., Chen, J. L., et al. (2023). Regulating the H2O2 activation pathway on a well-defined CeO2 nanozyme allows the entire steering of its specificity between associated enzymatic reactions. ACS nano 17, 17383–17393. doi: 10.1021/acsnano.3c05409
Zafar, N., Akram, N. A., Fatima, K., Noreen, S., Akram, M. S., Umer, S., et al. (2024). Drought-induced changes in plant-yield interlinked biochemistry of cauliflower (Brassica oleracea L. var. botrytis) by exogenously applied alpha-tocopherol. J. King Saud Univ. - Sci. 36, 103028. doi: 10.1016/j.jksus.2023.103028
Zandalinas, S. I., Fichman, Y., Devireddy, A. R., Sengupta, S., Azad, R. K., Mittler, R. (2020). Systemic signaling during abiotic stress combination in plants. Proc. Natl. Acad. Sci. U.S.A. 117, 13810–13820. doi: 10.1073/pnas.2005077117
Zeng, Y., Himmel, M. E., Ding, S. Y. (2017). Visualizing chemical functionality in plant cell walls. Biotechnol. Biofuels. 10, 263. doi: 10.1186/s13068-017-0953-3
Zhao, L., Lu, L., Wang, A., Zhang, H., Huang, M., Wu, H., et al. (2020). Nano-biotechnology in agriculture: use of nanomaterials to promote plant growth and stress tolerance. J. Agric. Food Chem. 68, 1935–1947. doi: 10.1021/acs.jafc.9b06615
Zhao, D., Yu, Y., Shen, Y., Liu, Q., Zhao, Z., Sharma, R., et al. (2019). Melatonin synthesis and function: evolutionary history in animals and plants. Front. Endocrinol. 10. doi: 10.3389/fendo.2019.00249
Zhou, Y., Hu, L., Wu, H., Jiang, L., Liu, S. (2017). Genome-wide identification and transcriptional expression analysis of cucumber superoxide dismutase (SOD) family in response to various abiotic stresses. Int. J. Genomics 2017, 1–14. doi: 10.1155/2017/7243973
Zhou, Y., Hu, L., Ye, S., Jiang, L., Liu, S. (2018). Genome-wide identification of glutathione peroxidase (GPX) gene family and their response to abiotic stress in cucumber. 3 Biotech. 8, 159. doi: 10.1007/s13205-018-1185-3
Zhu, L., Xu, W., Yao, X., Chen, L., Li, G., Gu, J., et al. (2023). Cell wall pectin content refers to favored delivery of negatively charged carbon dots in leaf cells. ACS Nano. 17, 23442–23454. doi: 10.1021/acsnano.3c05182
Keywords: drought stress, heat stress, plant nanobiotechnology, chloroplasts, mitochondria, apoplast, ROS homeostasis
Citation: Bao L, Liu J, Mao T, Zhao L, Wang D and Zhai Y (2024) Nanobiotechnology-mediated regulation of reactive oxygen species homeostasis under heat and drought stress in plants. Front. Plant Sci. 15:1418515. doi: 10.3389/fpls.2024.1418515
Received: 16 April 2024; Accepted: 31 July 2024;
Published: 27 August 2024.
Edited by:
Yuriy Kolupaev, Research Institute of Plant Production, Breeding and Genetics Kharkiv, UkraineReviewed by:
Mirza Hasanuzzaman, Sher-e-bangla Agricultural University, BangladeshTemesgen Assefa Gelaw, Debre Berhan University, Ethiopia
Copyright © 2024 Bao, Liu, Mao, Zhao, Wang and Zhai. This is an open-access article distributed under the terms of the Creative Commons Attribution License (CC BY). The use, distribution or reproduction in other forums is permitted, provided the original author(s) and the copyright owner(s) are credited and that the original publication in this journal is cited, in accordance with accepted academic practice. No use, distribution or reproduction is permitted which does not comply with these terms.
*Correspondence: Desheng Wang, d2RzMTg1OEAxNjMuY29t; Yunlong Zhai, enlsemt5QHRhcnUuZWR1LmNu
†These authors have contributed equally to this work