- 1Department of Horticulture, Agricultural College, Shihezi University, Shihezi, Xinjiang, China
- 2Key Laboratory of Special Fruits and Vegetables Cultivation Physiology and Germplasm Resources Utilization of Xinjiang Production and Contruction Crops, Shihezi, Xinjiang, China
- 3School of Life and Health Science, Kaili University, Kaili, Guizhou, China
Reduced glutathione (γ-glutamyl-cysteinyl-glycine, GSH), the primary non-protein sulfhydryl group in organisms, plays a pivotal role in the plant salt stress response. This study aimed to explore the impact of GSH on the photosynthetic apparatus, and carbon assimilation in tomato plants under salt stress, and then investigate the role of nitric oxide (NO) in this process. The investigation involved foliar application of 5 mM GSH, 0.1% (w/v) hemoglobin (Hb, a nitric oxide scavenger), and GSH+Hb on the endogenous NO levels, rapid chlorophyll fluorescence, enzyme activities, and gene expression related to the Calvin cycle in tomato seedlings (Solanum lycopersicum L. cv. ‘Zhongshu No. 4’) subjected short-term salt stress (100 mM NaCl) for 24, 48 and 72 hours. GSH treatment notably boosted nitrate reductase (NR) and NO synthase (NOS) activities, elevating endogenous NO signaling in salt-stressed tomato seedling leaves. It also mitigated chlorophyll fluorescence (OJIP) curve distortion and damage to the oxygen-evolving complex (OEC) induced by salt stress. Furthermore, GSH improved photosystem II (PSII) electron transfer efficiency, reduced QA- accumulation, and countered salt stress effects on photosystem I (PSI) redox properties, enhancing the light energy absorption index (PIabs). Additionally, GSH enhanced key enzyme activities in the Calvin cycle and upregulated their genes. Exogenous GSH optimized PSII energy utilization via endogenous NO, safeguarded the photosynthetic reaction center, improved photochemical and energy efficiency, and boosted carbon assimilation, ultimately enhancing net photosynthetic efficiency (Pn) in salt-stressed tomato seedling leaves. Conversely, Hb hindered Pn reduction and NO signaling under salt stress and weakened the positive effects of GSH on NO levels, photosynthetic apparatus, and carbon assimilation in tomato plants. Thus, the positive regulation of photosynthesis in tomato seedlings under salt stress by GSH requires the involvement of NO.
1 Introduction
China is the world’s largest horticultural country, Nevertheless, the escalation of secondary soil salinization, attributed to issues like high cropping intensity, excessive fertilization, and unique water transport mechanisms during production, poses a significant hurdle to the development and productivity of greenhouse crops in China (Zhu, 2016). Plant growth and development are closely linked to their photosynthesis, which is very sensitive to salt stress (Yang et al., 2022). Under salt stress, the photochemical efficiency of the plant photosystem is reduced, leading to overexcitation of the light-trapping antennae and the generation of oxidative stress. Sustaining photosynthesis under salt stress conditions hinges on regulating the photosynthetic apparatus. Moreover, salt stress induces photochemical bursts and diminishes photosynthetic quantum efficiency (Zushi and Matsuzoe, 2017), salt stress can also limit photosynthesis in plants by inactivating CO2 assimilating enzymes (Li et al., 2022a), resulting in reduced products of photosynthetic carbon assimilation and, in severe instances, potential plant death (Fatma et al., 2014). Tomato (Solanum lycopersicum L.) is a significant vegetable crop in horticulture, exhibiting moderate salt tolerance but some sensitivity to salt stress (Xu et al., 2023). Hence, exploring the photosynthetic acclimation mechanism of tomatoes under salt stress is valuable for improving their salt tolerance, reducing the damage caused by salt stress, breeding a resilient crop. Rapid chlorophyll fluorescence-induced kinetic analysis (JIP-test) is an analytical method established on the basis of biofilm energy flow. The JIP-test allows for rapid diagnosis of damage to the structure of the photosynthetic apparatus of a plant before it develops a visible pheno (Baker, 2008; Timilsina et al., 2022).
Reduced glutathione (γ-glutamyl-cysteinyl-glycine, GSH), a redox-active molecule, plays a pivotal role in the plant stress responses through redox pairs (GSH/GSSG) in the ascorbate-glutathione (AsA-GSH) cycle (Yao et al., 2021), glutathione, thioredoxin system, and other pathways. GSH can enhance the ability of plants to cope with challenges by protecting photosystem II by reducing reactive oxygen species (ROS) production (Zhou et al., 2018), protecting photosystem components, and increasing net photosynthetic rate (Mueller-Schuessele et al., 2020). Additionally, GSH collaborates with melatonin (Goodarzi et al., 2020), ascorbic acid, proline, or redox molecules (Semida et al., 2021) to collectively engage in stress-induced signaling pathways.
Nitric oxide (NO), a crucial bioactive plant molecule, regulates plant defense responses to various negative stressors. NO can effectively alleviate chlorophyll degradation, damage to the photosynthetic apparatus, reduced photochemical activity, and light energy conversion efficiency of PSII under stress, this enhances the plants’ photosynthetic adaptation (Murchie and Niyogi, 2011; Zhou et al., 2018).To date, more studies have been reported on the involvement of GSH in NO-controlled mitigation of adversity stress (Gautam et al., 2021; Wu et al., 2021; Xu et al., 2023). S-nitrosoglutathione (GSNO), a NO transporter and donor in plants, acts as a link between ROS and reactive nitrogen (RNS) signaling pathways, playing an important role in various plant signaling and defense reactions (Khan et al., 2023). GSNO/NO regulates the antioxidant system in different abiotic stress environments and improves plant resistance, suggesting that GSH participates in the NO signaling pathway to combat abiotic stresses (Hasan et al., 2016). The previous study demonstrated a synergistic role of NO and GSH in enhancing PSII activity and PSI transduction in cucumber leaves exposed to low-temperature stress (Yang et al., 2023). Currently, only a few studies have reported NO contributions to reducing abiotic stress-induced damage in plants via GSH (Hasan et al., 2016). Alamri et al. (2021) reported that GSH mitigation of arsenic toxicity in Solanum melongena requires the involvement of endogenous NO.
The authors’ previous studies demonstrated that exogenous GSH protects the photosynthetic apparatus from oxidative damage, increases PSII efficiency, and balances the uneven distribution of light energy by maintaining chloroplast redox balance and increasing ROS absorption capacity. GSH therefore effectively mitigates the inhibitory effects of salt on the growth and photosynthesis of tomato seedlings (Zhou et al., 2018). Furthermore, in another of the authors’ studies, the application of the NO scavenger Hb (hemoglobin bovine) reduced the exogenous GSH role in inducing endogenous NO production and increasing the antioxidant capacity of tomato (Wen et al., 2018). NO involvement was therefore hypothesized in the modulation of salt acclimatization by exogenous GSH. However, the role of exogenous GSH in regulating the structure and function of the photosynthetic and carbon assimilation systems in tomatoes under salinity stress, as well as the potential involvement of NO in the photoadaptative mechanism by which GSH alleviates salt stress, is still unclear. Therefore, the present study investigated the role of GSH in photosynthetic efficiency, the kinetic properties of rapid chlorophyll fluorescence induction, and carbon assimilation capacity in salt-stressed tomato seedlings via exogenous application of GSH, Hb, and Hb + GSH. NO involvement in the photosynthetic acclimatization induced by GSH was then detected and elucidated.
2 Materials and methods
2.1 Growing conditions and treatments
The hydroponics experiment was conducted in a greenhouse at Shihezi University, Xinjiang Uygur Autonomous Region,China. Tomato seeds (Solanum lycopersicum L. cv. ‘Zhongshu No. 4’) obtained from Shihezi Yaxin Seeds, China, Soak tomato seeds in warm water at 65°C for 30 minutes, stirring constantly during this time to allow them to fully soak. Subsequently, the seeds were gently rinsed with deionized water and planted in a 2:1 (v/v) charcoal and vermiculite mixture. The seedlings were grown under ambient conditions of 20–26°C, 40–60% relative humidity (RH) and approximately 500 µmol·photons·m−2·s−1 light intensity at noon. Upon reaching three fully mature leaves, uniform and healthy tomato seedlings were selected, roots were cleaned, and then transferred to a hydroponic system with a foam lid. The system was filled with 10 L of Hoagland’s nutrient solution (pH 6.2) prepared using demineralized water.
After allowing tomato seedlings to pre-culture for 7 days, five treatments were employed in the study (Table 1). The NaCl was added to the Hoagland nutrient solution, and GSH and Hb were sprayed on the leaves at 10:00 a.m every day. The concentrations of NaCl, GSH and Hb were established based on the pre-test results (Zhou et al., 2017; Wen et al., 2018). The research adopted a randomized block design with three replicates. Fully expanded functional young tomato seedling leaves in the third down from the growth point were chosen and sampled (with veins removed) at 24, 48, 72 hours post-treatment. The harvested leaves were rapidly frozen in liquid nitrogen and stored at -80°C for subsequent enzyme activity and gene expression assessments.
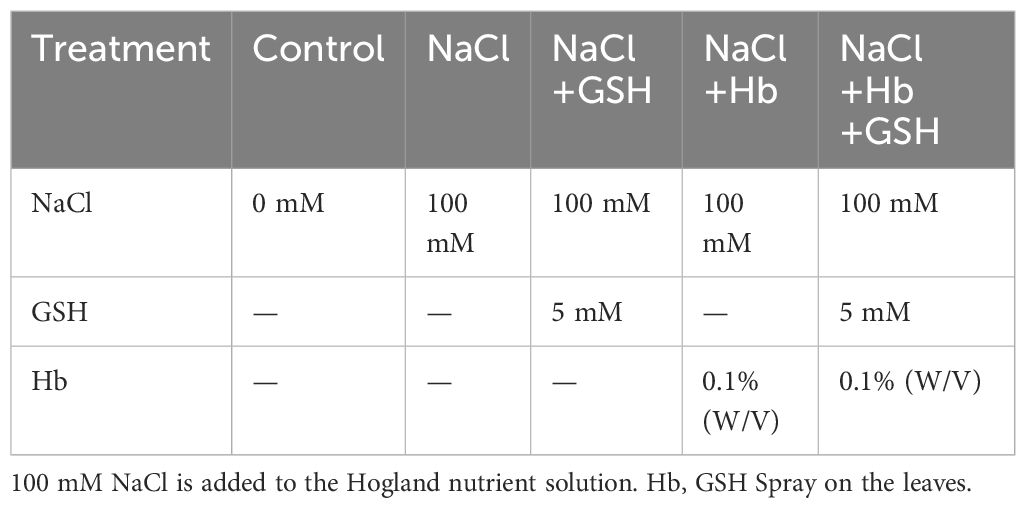
Table 1 Experimental protocols employed to investigate the impact of salt stress on tomato seedlings.
2.2 Visualization and quantification of endogenous NO signal
After 24, 48 and 72 hours of treatment, tomato leaves were sectioned into 5 x 5 mm2 pieces a dark-incubated in Tris-HCl buffer (10 mM Tris, containing 10 μM DAF-2DA, pH 7.4) for 30 min at 25°C. The excitation and emission wavelengths were 495 and 515 nm, correspondingly. Rinse 2–3 times with fluorescent dye-free buffer to remove excess fluorescent probe. Samples were examined utilizing a ZEISS LSM 510 META laser confocal scanning microscope (Zeiss, Germany). The mean fluorescence intensity of each field of view was quantified using the LSM510 software supplied with the instrument (Li et al., 2022a).
2.3 Assessment of nitrate reductase and NO Synthase activities
NR and NOS activities in tomato seedling leaves were measured using specific kits (Nanjing JianCheng Bio, China).
2.4 Determination of photosynthetic gas exchange parameters
Functional tomato leaves were harvested 24, 48, 72 hours post-treatment. Photosynthetic parameters were assessed with the CIRAS-3 photosynthesis system (PP Systems, USA) (Diao et al., 2014).
2.5 Polyphasic fluorescence transients and JIP-test parameters
Chlorophyll-a fluorescence kinetics (OJIP curves) and modulated reflectance kinetics at 820 nm (I/Io curves) were measured in tomato plants under various treatments using a multifunctional plant efficiency analyzer (Hansatech Instrument Ltd, Lynn, UK). After a two-hour dark acclimatization period, the leaves were exposed to saturating pulsed light (3,000 μmol photons m-2 S-1). The recorded fluorescence signals ranged from 0.01 ms to 3 S. The average OJIP measurement value of the OJIP measurement was calculated for the leaves (three tomato plants per treatment, n=3) in each treatment, and the fast OJIP curve was plotted. The spider plot data were presented as Ctest/CControl, where Ctest and CControl represented the data from the treatment and control groups, respectively. The JIP-test analysis of the OJIP curve provided parameters listed in Table 2.
2.6 Calvin cycle key enzymes
Rubisco activity was measured spectrophotometrically following the method of Lilley and Walker (1974), with some modifications. The total activity was assayed after the crude extract had been activated in a 0.1 ml activation mixture containing 33 mM Tris-HCl (pH 7.5), 0.67 mM EDTA, 33 mM MgCl2 and 10 mM NaHCO3 for 15 min. Initial Rubisco activity measurements were carried out in 0.1 ml of reaction medium containing 5 mM HEPES-NaOH (pH 8.0), 1 mM NaHCO3, 2 mM MgCl2, 0.25 mM dithiothreitol (DTT), 0.1 mM EDTA, 1 U of glyceraldehyde 3-phosphate dehydrogenase, 0.5 mM ATP, 0.015 mM NADH2, 0.5 mM phosphocreatine, 0.06 mM ribulose-1,5-bisphosphate (RuBP), and 10 μl of extract. The change in absorbance at 340 nm was monitored for 90 s (YuPing et al., 2012). Activities of RCA, PGK, GAPDH, FBPase, and TK were measured using specific biochemical spectrophotometric kits (Yuchun Bio, China). SBPase activity was determined following the method of Harrison et al. (1998) method. FBA activity was measured using biochemical kits (COMIN Bio, China).
2.7 Quantitative real-time PCR
The total RNA was extracted from tomato leaves using the Trizol method. Primers used in this study were designed with Primer 6.0 based on the NCBI database (Supplementary Table 2). The tomato actin gene served as an internal control (Li et al., 2022a; Zhang et al., 2023). All primers were synthesized by Biotech Bioengineering Co. (Shanghai, China).
High-purity and integrity total RNA was reverse transcribed into cDNA using the Hyper ScriptTM III RT SuperMix for qPCR with gDNA Remover (NovaBio, China) following the manufacturer’s instructions. qRT-PCR amplification was conducted using the 2 x S6 Universal SYBR qPCR Mix (NovaBio, China). Real-time PCR was performed using a CFX96™ Real-Time PCR System (BIO-RAD, America), following the methods in the ChamQ Blue Universal SYBR qPCR Master Mix (Vazyme Biotech Co., Ltd, Nanjing, China). Three parallel replicates were prepared for each sample stored at -80°C, and biological replicates were performed for each gene. The 2−ΔΔCt method Livak and Schmittgen (2001) was used to calculate the relative gene expression.
2.8 Statistical analysis
Data were analyzed using IBM SPSS 25 statistical software with one-way ANOVA. Differences’ significance was assessed using the Duncan test (P<0.05). Graphs were created using OriginPro 2023. Values in tables and graphs represent the mean ± standard deviation (SD). Three parallel replicates were prepared for each sample.
3 Results
3.1 Endogenous NO accumulation in tomato leaves under different treatments
As shown in Figure 1, in comparison with Control, the average NO fluorescence intensity, NR and NOS activity during the three measurement periods of NaCl treatment were significantly reduced by 41.3–42.7%, 59.1–69.9%, and 43.5–54.3%, respectively. Conversely, treatment with NaCl+GSH led to a significant increase in NO content, NR and NOS activity by 15.62–39.42%, 51.8–121.4% and 7.6–44.1%, respectively, compared to NaCl treatment. Treatment with NaCl+Hb resulted in a significant decrease in NO content and NR activity at 48 and 72 hours, as well as NOS activity at 72 hours. On the other hand, NaCl+Hb+GSH treatment significantly enhanced NO content, NR and NOS activity throughout the treatment period compared to NaCl+Hb treatment, with increases of 38.3–49.4%, 21.8–87.3% and 21.2–44.1%, respectively.
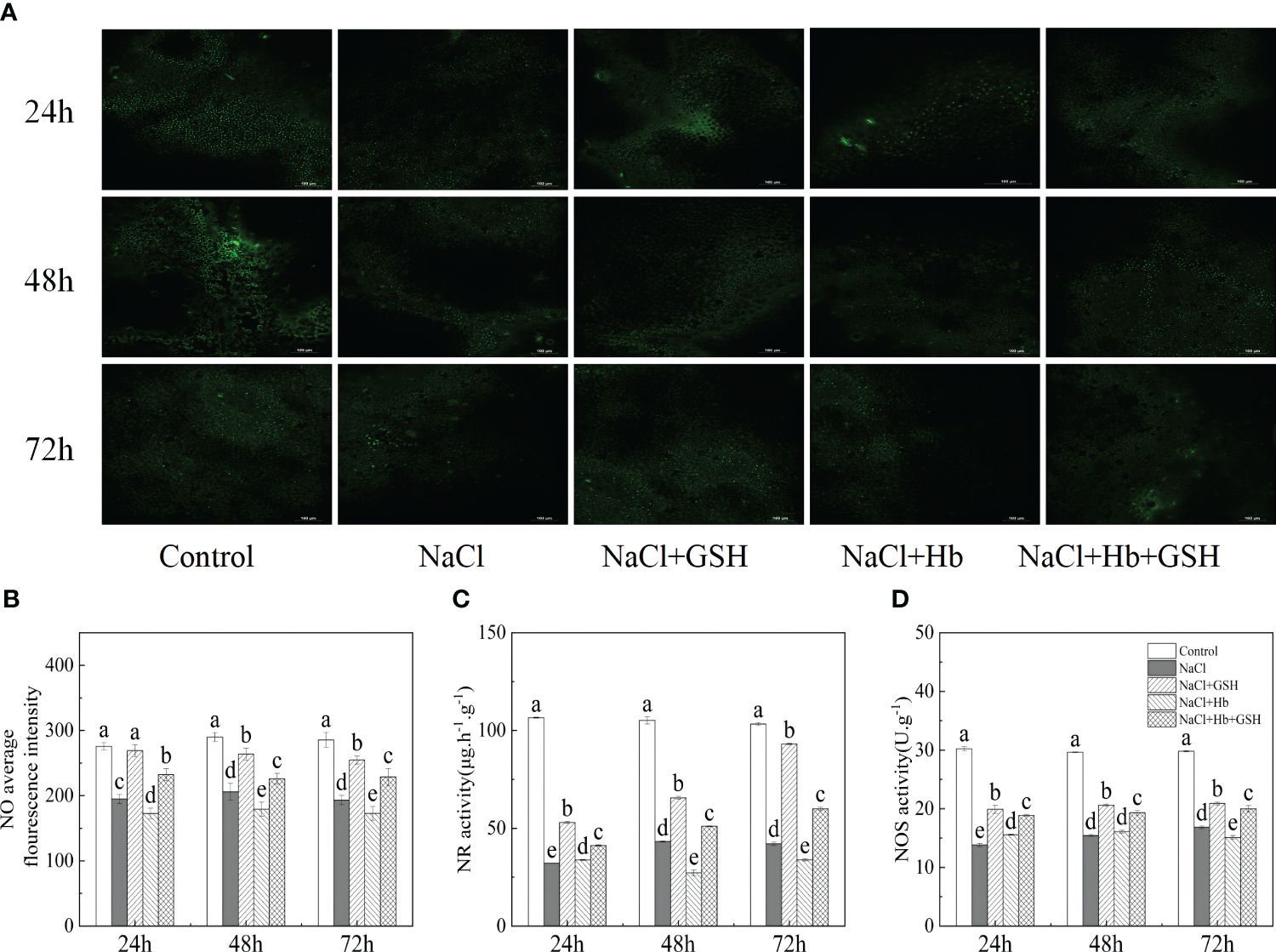
Figure 1 Effects of GSH (γ-glutamyl-cysteinyl-glycine), Hb (Hemoglobin, NO scavenger), Hb+GSH on NO fluorescence imaging (A), average fluorescence intensity (B), nitrate reductase (NR) activity (C), and nitric oxide synthase (NOS) activity (D) in leaves of salt-stressed tomato seedlings. Notes: The green spots in figure A are the NO signal, and the short white line in the lower right corner is the 100-μm scale. Control, no added NaCl and sprayed with distilled water; NaCl, addition of 100 mM NaCl and sprayed distilled water; NaCl+GSH, added 100 mM NaCl, and sprayed 5 mM GSH; NaCl+Hb, with added 5 mM GSH. The results are presented as the mean ± SD (standard deviation) (n=3).Different lowercase letters represent significant differences, and the same lowercase letters represent no significant differences (p < 0.05, Duncan's range test).
3.2 Photosynthetic gas exchange in tomato leaves under different treatments
The application of NaCl significantly reduced the net photosynthetic rate (Pn), stomatal conductance (Gs), intercellular CO2 concentration (Ci) and transpiration rate (Tr) across all three treatment periods in comparison to Control (Figure 2). Conversely, the NaCl+GSH treatment led to a substantial increase in Pn, Ci, Gs and Tr values throughout the treatment period, showing enhancements 24.3–30.5%, 21.4–32%, 6.0–15.5% and 29.4–41.9%, respectively, when compared to the NaCl treatment. On the other hand, the NaCl+Hb treatment resulted in a notable reduction in Pn, Gs, and Tr throughout the treatment duration, while Ci values experienced at 48 and 72h. In comparison to the NaCl+GSH treatment, Pn, Gs and Tr values over the treatment period, along with Ci at 24 and 72 h, exhibited significant decreases under NaCl+Hb+GSH treatment.
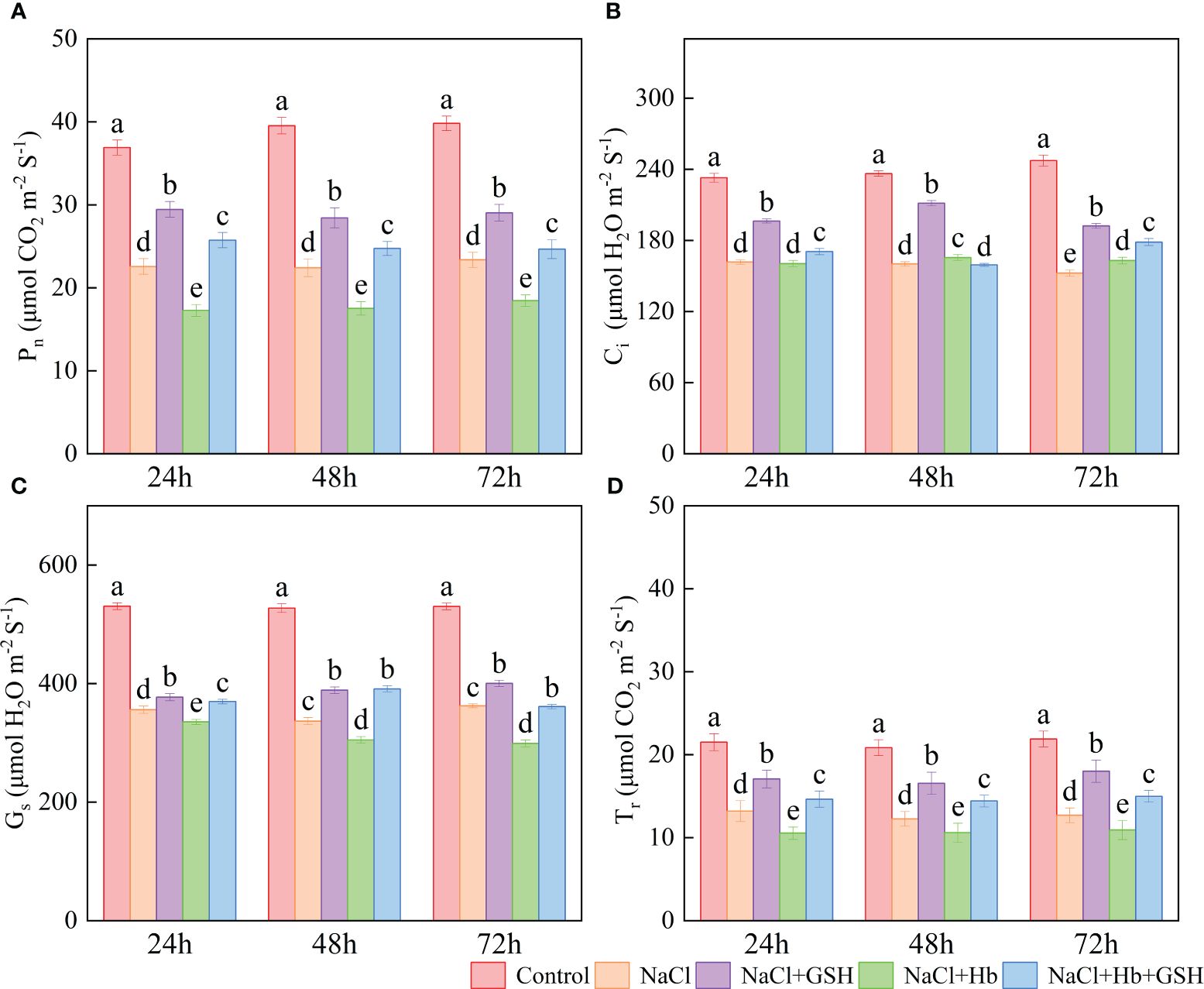
Figure 2 Effects of GSH, Hb, and Hb+GSH on the net photosynthetic rate (Pn, A), intercellular CO2 concentration (Ci, B), transpiration rate (Tr, C), and stomatal conductance (Gs, D) in leaves of salt-stressed tomato seedlings. Notes: Control, no added NaCl and sprayed with distilled water; NaCl, addition of 100 mM NaCl and sprayed distilled water; NaCl+GSH, added 100 mM NaCl, and sprayed 5 mM GSH; NaCl+Hb, with added 5 mM GSH. The results are presented as the mean ± SD (standard deviation) (n=3). Different lowercase letters represent significant differences, and the same lowercase letters represent no significant differences ( p < 0.05, Duncan's range test).
3.3 Impact of various treatments on the kinetic properties of transient fluorescence induction (JIP-test)
3.3.1 OJIP curves
Under NaCl stress, the OJIP curves displayed deformations compared to Control, indicating a reduced amplitude of the I-P phase (Figure 3A-C). The entire curve flattened, and the amplitudes of the I and P phases, as well as the I-P phase of the OJIP curves, gradually decreased with prolonged treatment time. In contrast to the NaCl treatment, the shape of the OJIP curves underwent significant alterations during NaCl+Hb treatment, leading to a notable decrease in the amplitude of the I-P phase. However, the amplitudes of the I, P and I-P phases were significantly heightened throughout the three periods of NaCl+GSH treatment. However, the amplitudes of the I, P and I-P phases were significantly enhanced during the three periods of NaCl + GSH treatment. I-P phase amplitude significantly decreased, while the amplitudes of the I and P phases as well as the I-P phase were significantly higher in the three periods under NaCl+GSH treatment. Furthermore, the amount of phase I and P in OJIP curves treated with NaCl+Hb+GSH was lower than in those treated with NaCl+GSH during the three measurement periods. Normalization of the OJIP curves (Figure 3D-F) demonstrated a significantly higher J phase under NaCl stress compared with the control. This suggested an inhibition of QA to QB electron transfer on the PSII receptor side and a large accumulation of QA-. Exogenous GSH application reduced the J phase under NaCl stress to varying degrees. The Hb application further increased the J phase under NaCl stress, weakening the effect of GSH. These findings indicate that GSH mitigated the partial damage to the PSII reaction center and decreased the ability of the PSII donor side to transfer electrons downstream under salt stress conditions, independently of NO.
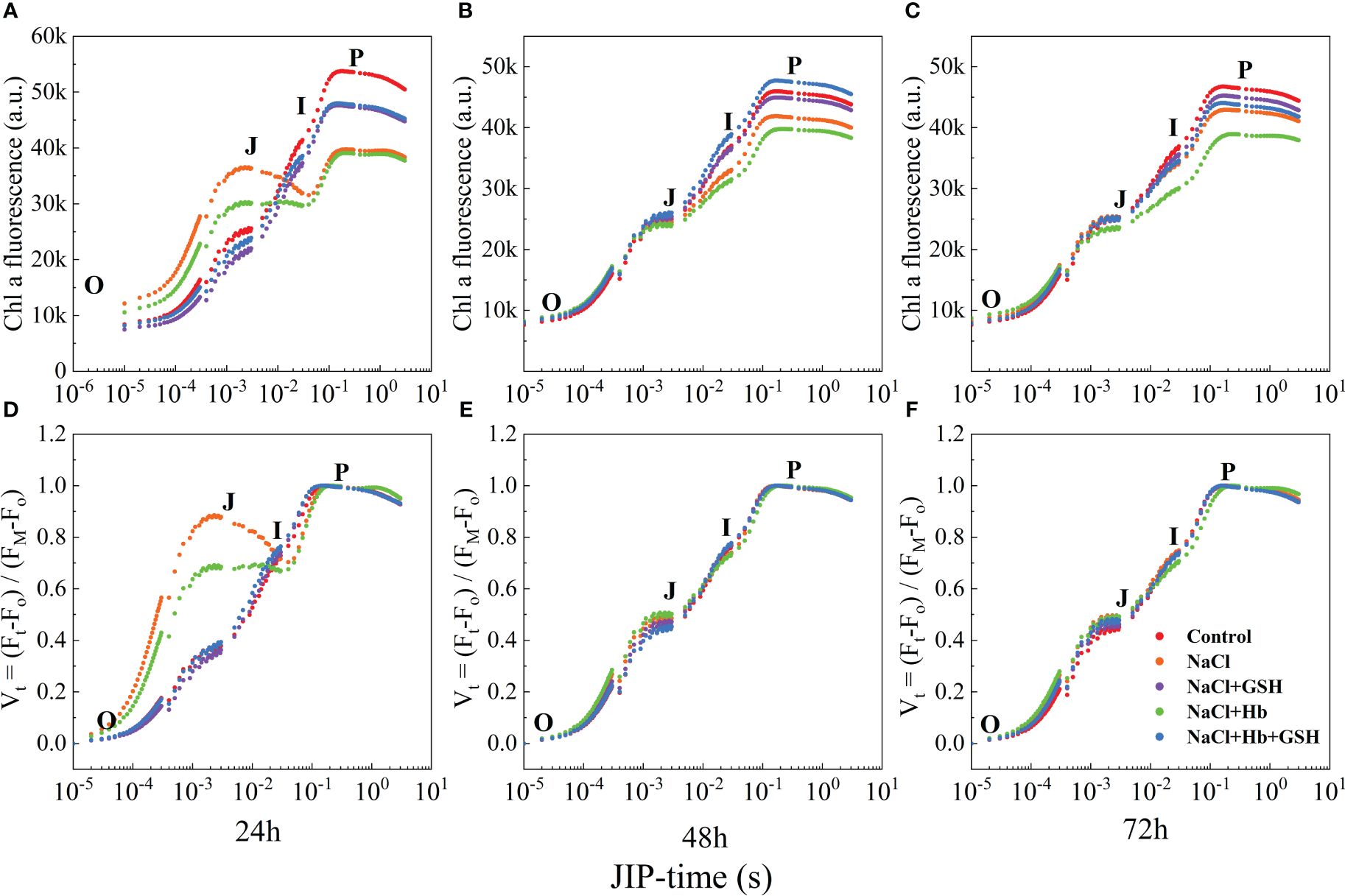
Figure 3 The fast chlorophyll a fluorescence induction (OJIP) curves (A–C) and normalized transients between O- and P-step expressed as Vt = [(Ft – Fo)/(FM – Fo] (D–F) in leaves of salt-stressed tomato seedlings as affected by GSH, Hb, and Hb+GSH.
The presence of L and K-bands at 0.15 and 0.3 ms and the rise in ΔWL and ΔWK values were recognized as specific markers of vesicle-like dissociation and damage to the PS II donor-side complex (OEC), respectively. ΔWL at 24 and 72 h and ΔWK at 48 and 72 h were significantly higher under NaCl treatment compared to the control, while ΔWL and ΔWK were significantly lower under NaCl+GSH treatment compared with NaCl treatment to varying degrees (Figure 4). These results suggested that GSH application attenuates salt stress-induced vesicle dissociation and OEC damage. In addition, the Hb application further enhanced ΔWL at 48 and 72 h of salt stress treatment and ΔWK at the three measurement periods. The Hb application also enhanced ΔWK under NaCl+GSH treatment.
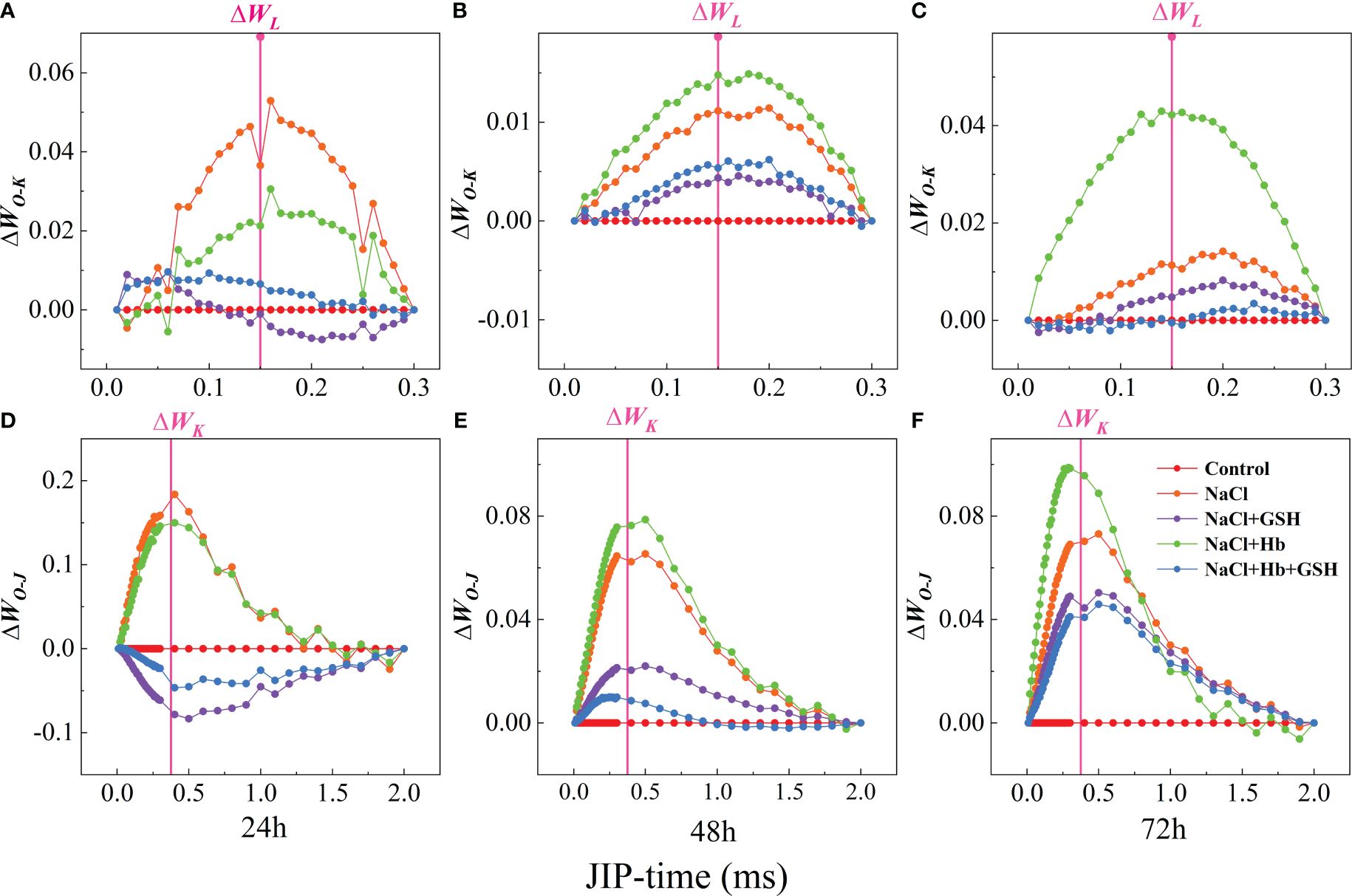
Figure 4 Difference kinetics ΔWO-K = WO-K(stress) - WO-K(control) (A–C) in a linear time scale from 0 to 300 μs and ΔWO-J = WO-J(stress) - WO-J(control) (D–F) in a linear time scale from 0 to 2 ms in leaves of salt-stressed tomato seedlings as affected by GSH, Hb, and Hb+GSH. ΔWO-K = WO-K(stress) - WO-K(control) (A–C), ΔWO-J = WO-J(stress) - WO-J(control) (D–F).
3.3.2 JIP-test parameters
NaCl treatment had a significant impact on tomato plants compared to Control (refer to Figure 5A-C). There was a notable increase in the relative variable fluorescence (VI) at point I, the relative variable fluorescence (VJ) at point J, the maximum rate at which QA was fully reduced (Mo), the quantum ratio used for heat dissipation (φDo) in tomato seedling leaves. Furthermore, this increase was observed in the ratio of exciton-driven electron transfer by excitons captured by the PSII active reaction center (Ψo), the quantum efficiency of the electron transfer from QA- to the electron transfer chain (φEo), the photosynthetic performance index (PIabs), the efficiency of electron transfer from QB to the PSI receptor side (δRo), the quantum yield of the terminal electron acceptor on the reduced PSI receptor side (φRo). These findings indicate that NaCl stress resulted in a decrease in the electron transfer capacity on the PSII receptor side and in the activation of the active PSII reaction centers. In contrast, NaCl+Hb treatment further significantly increased Mo and φDo, while significantly decreasing Ψo, φEo, PIabs and φRo. GSH application effectively alleviated Mo and φDo increases under NaCl treatment to varying degrees and restored Ψo, φEo, PIabs, δRo and φRo decreases to the control group levels (72 h) with extended treatment time. However, the Hb application weakened the effect of GSH on the above parameters under NaCl stress.
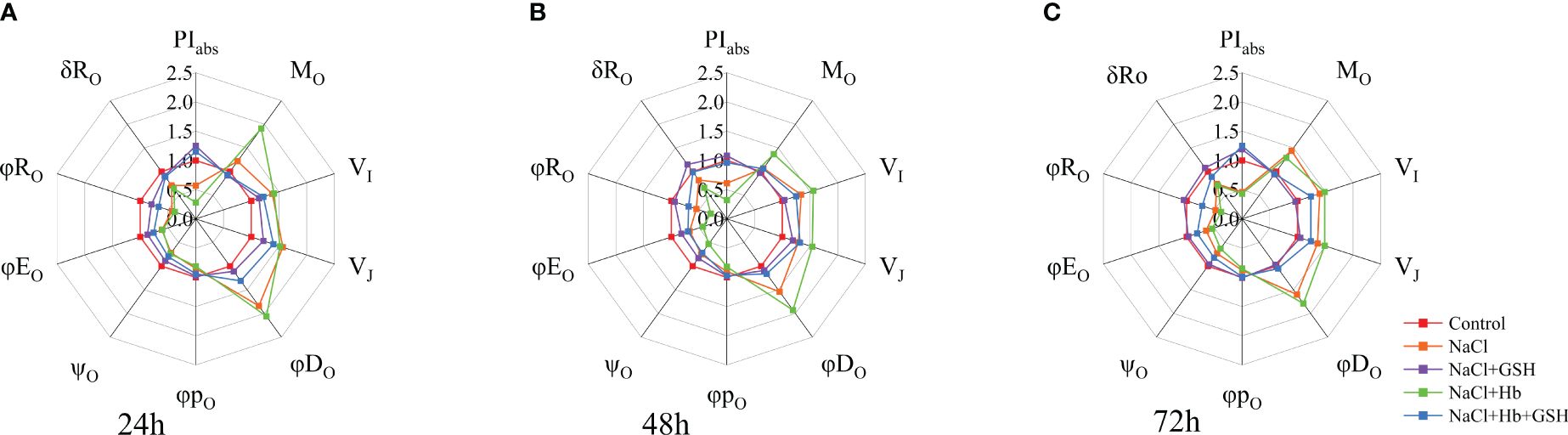
Figure 5 Spider plots were used to assess GSH, Hb, and Hb+GSH treatment influence on JIP-test parameters derived from chlorophyll a fluorescence OJIP transient curves (A–C) in salt-stressed tomato seedling leaves.
The 820 nm fluorescence reflectance kinetic curve (I/Io) is used to determine PSI complex activity due to adversity stress (Figure 6A-C), which demonstrated that NaCl stress could severely deform the leaf I/Io curve compared to the control. This was expressed by the elevation of the lowest point of the descending phase and the decrease of the highest point of the ascending phase, suggesting that the PSI redox capacity was inhibited by salt stress. In contrast, the GSH application restored I/Io curve deformation under NaCl treatment to varying degrees. The Hb application further heightened I/Io curve deformation under NaCl stress and weakened the effect of exogenous GSH in alleviating the deformation.
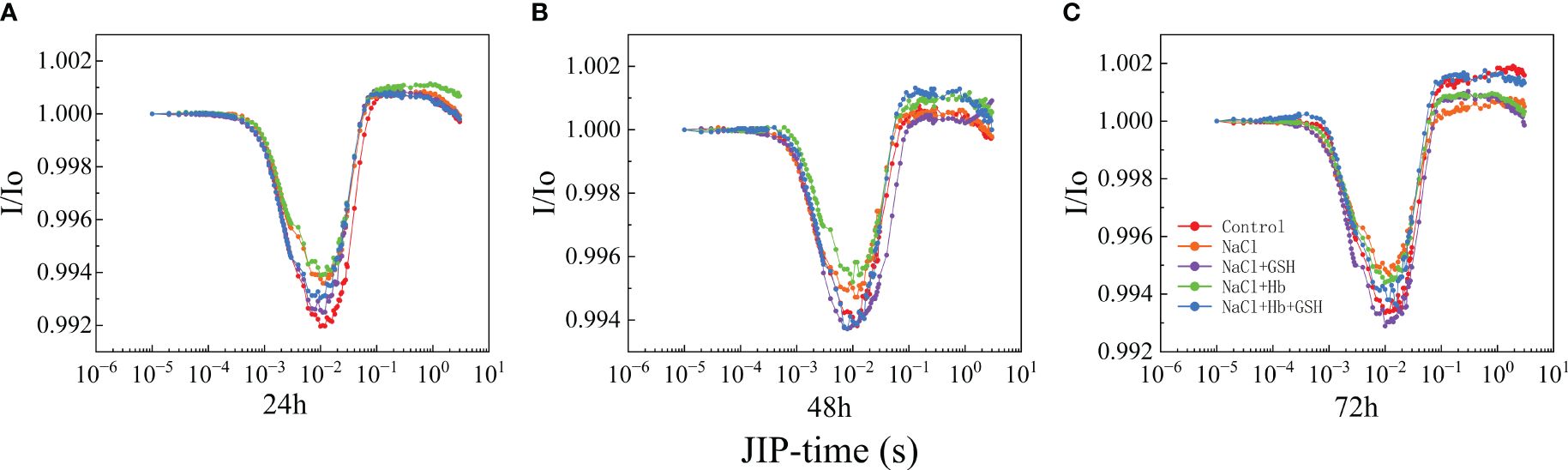
Figure 6 Curves of light-induced modulated 820 nm reflection kinetics (I/Io) (A–C) in leaves of salt-stressed tomato seedlings as affected by GSH, Hb, and Hb+GSH.
3.3.3 PSII reaction center activity and excited cross section phenomenological energy fluxes
In tomato seedling leaves, the unit activity of the PSII reaction center (RC) showed a significant increase in the energy absorbed and thermally dissipated by RC (ABS/RC and DIo/RC), along with a notable decrease in the energy transferred by RC (ETo/RC) under NaCl treatment compared to the Control conditions (Figure 7A-C). Notably, NaCl+GSH treatment led to a significant increase in ETo/RC and a decrease in ABS/RC, DIo/RC and TRo/RC. Conversely, NaCl+Hb treatment increased ABS/RC, DIo/RC and TRo/RC values. Additionally, the ABS/RC, DIo/RC and TRo/RC values were significantly reduced in the NaCl+Hb+GSH treatment compared to the NaCl+Hb treatment. For the unit leaf cross-sectional area (CSm), the number of RCs (RC/CSm) and the energy absorbed and captured by the CSm (ABS/CSm and TRo/CSm) were significantly lower in the CSm under NaCl stress compared to the Control. Although NaCl+GSH treatment significantly increased ABS/CSm, TRo/CSm, ETo/CSm and RC/CSm, both NaCl+Hb and NaCl+Hb+GSH treatments significantly decreased ABS/CSm, TRo/CSm, ETo/CSm and RC/CSm values to varying degrees compared to NaCl and NaCl+GSH treatments (Figure 7A-C). The energy flux model is depicted in Figure 7D, E.
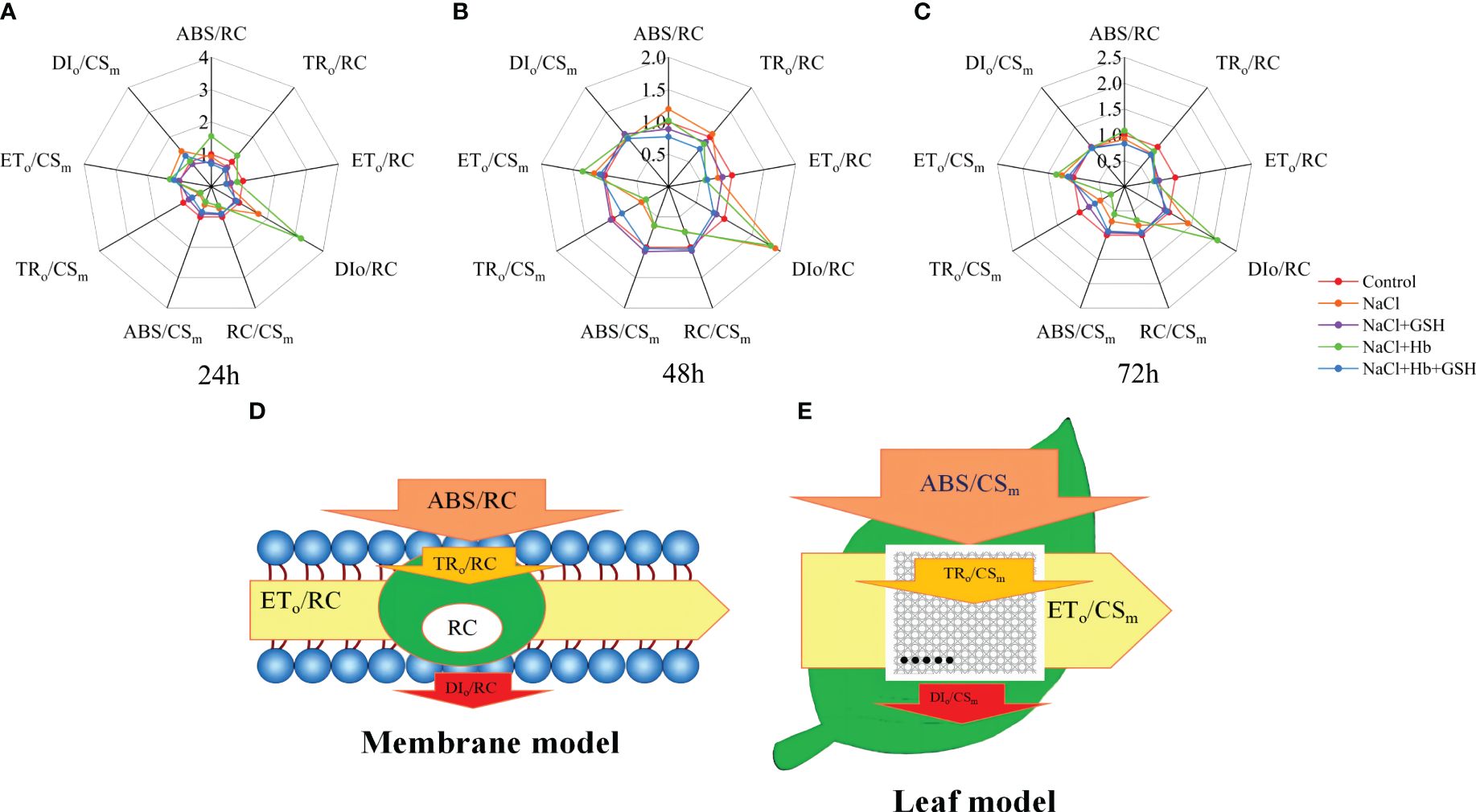
Figure 7 Spider plots of the energy distribution parameters per PSII reaction center (RC) and exciting cross-sectional area (CSm) (A–C) and the energy pipeline model of specific fluxes per RC (D) and phenomenological fluxes per excited CSm (E) in salt-stressed tomato seedlings as affected by GSH, Hb, and Hb+GSH.
3.4 The role of NO in GSH-induced CO2 assimilation under salt stress
NaCl treatment significantly reduced Rubisco (both initial and total), RCA, GAPDH, PGK, FBA, FBPase, SBPase and TK activity by 46.7–50.1%, 50.3–54.6%, 11.9–24.9%, 40.6–51.1%, 30.1–35.7%, 29.9–34.5%, 33.3–38.8%, 40.5–49.9% and 40.2–43.8%, respectively (Figure 8). However, GSH application alleviated the inhibitory effect of NaCl stress, increasing above enzyme activity by 46.1–64.1%, 50.1–73.3%, 8.1–26.0%, 44.1–75.6%, 21.2–42.75%, 32.6–44.5%, 45.1–59.9% 29.1–60.7% and 46.5–62.1%, respectively. NaCl+Hb treatment significantly decreased TK activity, 24-hour RCA activity and 48-hour SBPase activity throughout the treatment period compared to NaCl stress. NaCl+Hb+GSH treatment significantly increased RCA, FBPase, FBA SBPase and TK activity, as well as PGK at 24 and 72 h and GADPH at 48 and 72 h compared to the NaCl+Hb treatment.
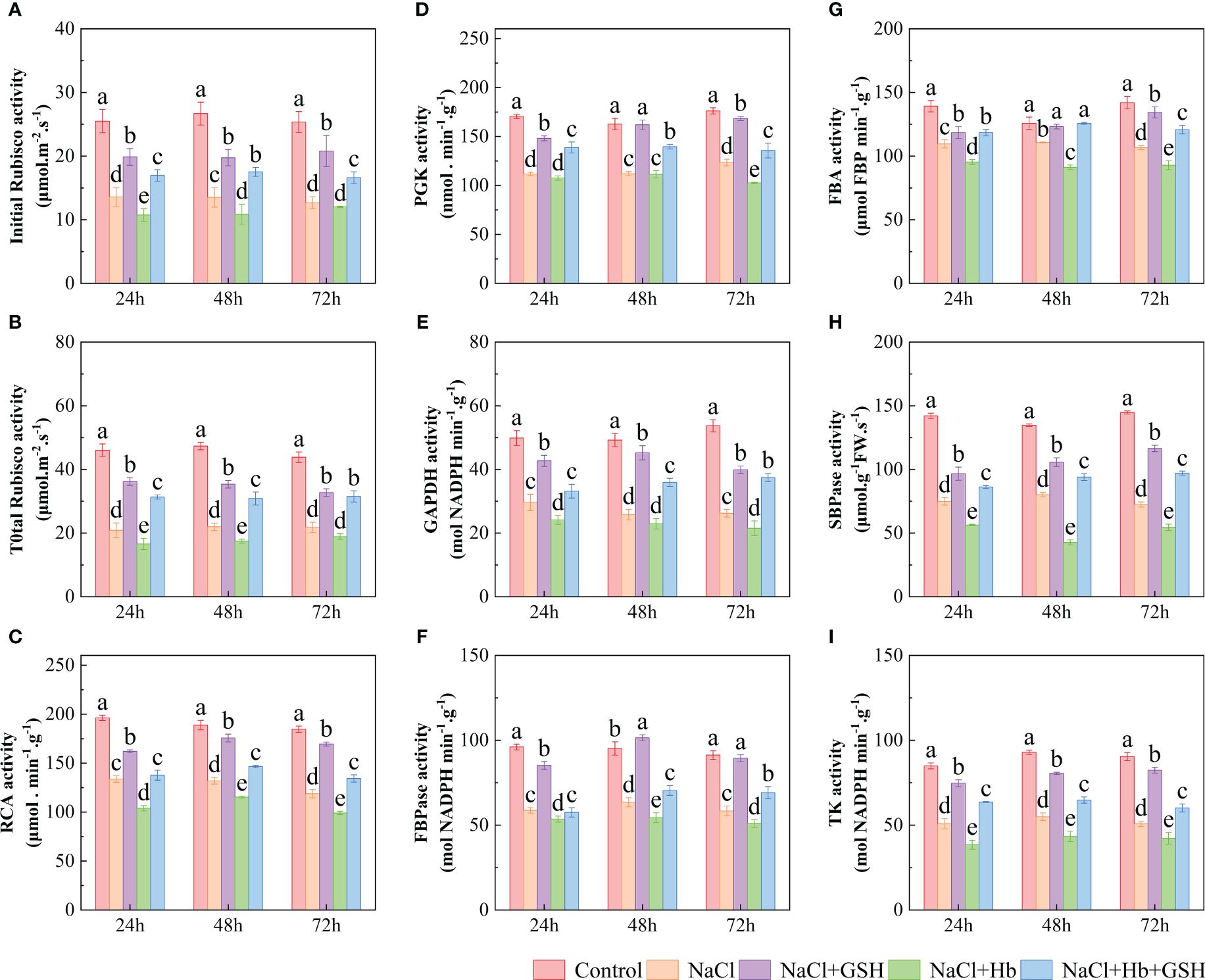
Figure 8 Effects of GSH, Hb, and Hb+GSH treatments on key enzymes of the Calvin cycle in leaves of salt-stressed tomato seedlings. Initial and total activity of Rubisco (A, B), RCA, PGK, GAPDH, FBPase, FBA, SBPase and TK (C–I). Notes: Control, no added NaCl and sprayed with distilled water; NaCl, addition of 100 mM NaCl and sprayed distilled water; NaCl+GSH, added 100 mM NaCl, and sprayed 5 mM GSH; NaCl+Hb, with added 5 mM GSH. The results are presented as the mean ± SD (standard deviation) (n=3). Different lowercase letters represent significant differences, and the same lowercase letters represent no significant differences ( p < 0.05, Duncan's range test).
The transcript levels of key enzymes involved in CO2 carboxylation and reduction (RbcS, RbcL, RCA, PGK and GADPH) and enzymes related to RuBP regeneration (SBPase, FBA, FBPase and TK) were significantly downregulated under NaCl stress (Figure 9). However, NaCl+GSH treatment resulted in a significant upregulation of these genes. Conversely, NaCl+Hb treatment led to a significant downregulation of FBA at 24 h, SBPase at 48 h, RbcL and PGK at 72 h, RbcS and RCA at 24 and 72 h. No significant effects were detected on GAPDH, FBPase, TK transcript levels.
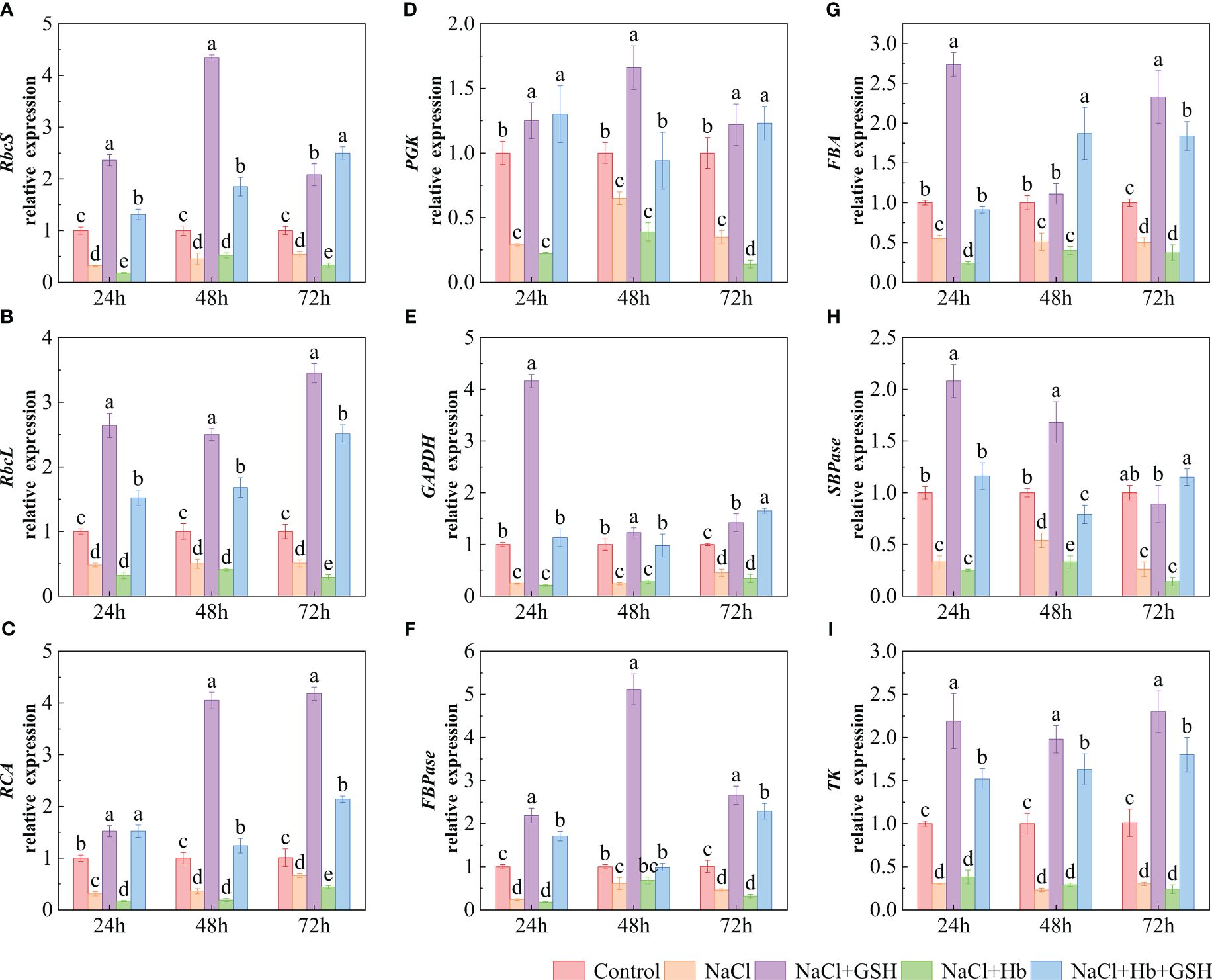
Figure 9 The relative expression of genes involved in the Calvin cycle in tomato seedling leaves under salt stress were analyzed for their response to GSH, Hb, and Hb+GSH. RbcS, RbcL, RCA, PGK, GAPDH, FBPase, FBA, SBPase and TK (A-I). Transcript levels were quantified using quantitative PCR, which were then normalized to actin expression. Notes: Control, no added NaCl and sprayed with distilled water; NaCl, addition of 100 mM NaCl and sprayed distilled water; NaCl+GSH, added 100 mM NaCl, and sprayed 5 mM GSH; NaCl+Hb, with added 5 mM GSH. The results are presented as the mean ± SD (standard deviation) (n=3). Different lowercase letters represent significant differences, and the same lowercase letters represent no significant differences ( p < 0.05, Duncan's range test).
4 Discussion
Plant photosynthesis is essential for energy production and metabolism, and is highly susceptible to external environmental factors (Bahmani et al., 2019). Pn serves as a vital indicator of plant photosynthetic capacity (Simkin et al., 2019). During salt stress, the decrease in Pn can be attributed to both stomatal and non-stomatal limiting factors. In this investigation, the decline in Pn under salt stress coincided with reductions in Ci, Gs, Tr (Figure 2) and PIabs (Figure 5). PIabs is a comprehensive index that reflects photochemical efficiency. Previous studies have indicated that the reduction in PIabs is more sensitive than Fv/Fm, accurately indicating the impairment of the plant’s photosynthetic machinery and the decrease in photochemical efficiency under adverse condition (Chen et al., 2021; Li et al., 2022b). The decrease in Pn due to NaCl stress was influenced by both stomatal-limiting and non-stomatal-limiting factors. These results were similar to the studies of Hordeum jubatum (Shi et al., 2021) and Cucumis melo (Sarabi et al., 2019). Furthermore, a correlation was noted between the reduction in Pn under salt stress and a decrease in NO signaling intensity, along with reduced NOS and NR activity. Conversely, the application of GSH under salt stress led to an improvement in Pn, Ci, Gs and PIabs, accompanied by an increase in NO levels as well as NR and NOS enzyme activity (Figure 2).
NO is widely distributed in various tissues and organs of plants, and exerts a positive regulatory role in plant growth and stress resilience. It is involved in the regulation of diverse physiological processes in plants (Fancy et al., 2017). Its cellular concentrations are decisive for its function, as a signaling molecule at lower concentrations, but triggers nitro-oxidative stress and cellular damage when produced at higher concentrations (Wani et al., 2021; Nishat Timilsina et al., 2022; Parveen et al., 2023). Salt stress-induced fluctuations in NO levels have been documented in different plant species, potentially influenced by salt type, stress intensity, duration and plant species. The results showed that external GSH improved Pn in salt-stressed tomato leaves by promoting the production of endogenous NO (Figures 1, 2). To explore if external GSH influenced the photosynthetic capacity of tomato seedlings under salt stress through modulating endogenous NO levels, we treated tomato seedlings under salt stress with exogenous Hb, a NO scavenger. Numerous studies have previously demonstrated the ability of external Hb to efficiently decrease endogenous NO levels in plants (Shao et al., 2010; Lee and Hwang, 2015; Bahmani et al., 2019; Hu et al., 2023). In this investigation, the application of Hb not only resulted in a further reduction in Pn and endogenous NO levels under salt stress but also attenuated the beneficial impacts of GSH treatment, including the rise in Pn and endogenous NO levels. These findings strongly suggest that GSH mitigates the effects of NaCl stress by mediating endogenous NO levels, and is involved in regulating the decline of Pn in tomato plants. Hb application significantly reduced NR activity at 48 and 72 h and NOS activity at 72 h under salt stress to varying degrees, as well as NR and NOS activity under NaCl+GSH treatment. These results, similar to (Shao et al., 2010), suggested that Hb application also reduced endogenous NO levels via a down-regulation of the NO synthesis pathway.
Salt stress can significantly affect the photosynthetic apparatus, specifically the PSII located in the thylakoid lamellae, PSII is highly vulnerable to salt stress-induced photoinhibition, which can damage the overall photosynthetic efficiency (Zushi and Matsuzoe, 2017). The OJIP curve provides insights into PSII primary photochemical reactions, revealing the impact of environmental conditions on the photosynthetic apparatus, including the PSII donor and acceptor sides. The J-phase represented QA- rapid accumulation and increased J-phase fluorescence demonstrated a blockage in electron transfer from QA to QB on the PSII acceptor side. OJIP curve analysis provided insights into PSII functional status and environmental influence. The appearance of the K-band (ΔWK>0) before the OJIP curve rising to the J-phase reflected OEC damage on the PSII donor side. The appearance of the L-band with ΔWL> 0. On the other hand, indicated the dissociation of the basal vesicle-like bodies, resulting in an increased dissociation between the PSII complexes. VJ and VI reflect the number of reaction centers in the J-phase and I-phase closure, respectively. An increase in VJ was a specific marker of blocked electron transfer from the PSII receptor side QA to the secondary quinone receptor QB. However, an increase in VI indicated a decrease in the ability of the PQ pool to accept electrons. In the current study, salt stress reduced the amplitude of the I-P phase and increased the ΔWK, ΔWL, and J phases (Figures 3, 4), as well as elevated VJ and VI values in tomato leaves (Figure 5). These changes indicated that salt stress damaged the PSII donor side OEC and increased dispersion between PSII complexes. This also led to the accumulation of QA- on the PSII acceptor side, resulting in the partial closure of PSII reaction centers. Furthermore, salt stress reduced Ψo and φEo while promoting an increase in Mo (Figure 5). These results demonstrated a reduction in the openness of active PSII reaction centers and PQ pool capacity, as well as a decline in electron transfer ability from QA on the PSII acceptor side. GSH treatment however protected the PSII donor side OEC and reaction center structure under salt stress and also enhanced electron transfer and photochemical efficiency on the PSII acceptor side (Figures 3, 5).
Conversely, the application of GSH shielded both the OEC and the reaction center structure on the donor side of PSII from salt stress, enhancing electron transfer and the photochemical efficiency of the PSII acceptor side (Figures 3, 5). Furthermore, the use of Hb diminished the favorable outcomes of external GSH on electron transfer at the PSII donor side, the photosynthetic apparatus, and PSII acceptor measurements in NaCl-stressed tomato seedlings. Collectively, these findings indicate that NO is involved in mediating the advantageous effects of GSH on the structure and functionality of the PSII photosynthetic apparatus.
Under normal conditions, the PSII reaction center efficiently converts captured light energy into excitation energy. This energy is primarily utilized for carbon assimilation, while any surplus energy is dissipated as heat. However, under adversity stress, PSII reaction centers become temporarily inactive. Despite the continued absorption of light energy, PSII reaction centers fail to transfer it to the electron transport chain (Murchie and Niyogi, 2011). Additionally, the research illustrated a notable decline in RC/CSm, TRo/CSm and ETo/CSm in tomato leaves (Figure 7), indicating the degradation or deactivation of reaction centers and a reduction in light energy available for electron trapping and transfer (Li et al., 2017). The decrease in ABS/CSm and the rise in DIo/CSm under salt stress (Figure 7) indicated the activation of defense mechanisms in salt-stressed tomato plants, aligning with the observations of Yuan et al. (2014) findings. On the one hand, mitigating the overaccumulation of light energy by reducing the light energy absorption of PSII antenna pigments, and on the other hand, diminishing the buildup of excess excitation energy through the enhancement of the heat dissipation pathway. Moreover, salt stress induced an elevation in ABS/RC, TRo/RC and DIo/RC, while a decrease in RC/CSm prompted a compensatory reaction. The remaining active RCs exhibited enhanced efficiency in absorbing, converting, and dissipating light energy, leading to improved consumption of excess energy in the electron transport chain (Chen et al., 2021). In contrast, GSH treatment not only mitigated the decrease in ABS/CSm, TRo/CSm and ETo/CSm in tomato leaves under salt stress, but also sustained elevated levels of DIo/CSm, ABS/RC, TRo/RC and DIo/RC (Figure 7). This protective mechanism optimizes energy allocation in PSII, preventing over-reduction of the photosynthetic electron transport chain and safeguarding the integrity and functionality of both the electron transport chain and the PSII reaction center.
In addition to plant leaf PSII, PSI reaction centers are vulnerable to adversity stress. The I/Io curves reflect PSI primary photochemical reactions (Yuan et al., 2014). The fast descend phase in the I/Io curves represents the oxidation of P700 and PC, the nadir point is the turning point of the redox of PSI. When the electrons from PSII promote PSI reduction, the I/Io curve turns to a slow-rising phase, which represents the re-reduction of P700 and PC (corresponding to the I-P phase of the OJIP curve) (Guo et al., 2020). Therefore, the I/Io curve is simultaneously affected by PSI and PSII activity and can reflect the coordination between these photosystems. The decrease in PSI activity prevents the PSII from transferring electrons to PSI, exacerbating the degree of PSII injury, meanwhile, PSI stability can ensure the rapid repair of damaged PSII (Yuan et al., 2014). The results of this study indicated that exogenous GSH effectively alleviated the reduction of PSI redox capacity in tomato plants under salt stress (Figure 6). Combined with the significant φRo increase under NaCl+GSH treatment compared with NaCl treatment, it was hypothesized that GSH may enhance PSI activity via an increased ability or amount of electron transfer from PQ to P700+, facilitating PSI receptor-side electron transfer and coordinating the connectivity between PSII and PSI. However, the positive GSH effect on PSI in salt-stressed tomato leaves may be weakened by exogenous Hb application (Figures 5, 6). This suggested that PSI activity regulation in salt-stressed tomato leaves by GSH required NO participation.
The Calvin-Benson cycle (CBC) is the primary CO2 fixation pathway utilized by C3 plants (Jensen et al., 2017). The CBC consists of the following three stages: CO2 fixation, CO2 reduction, and RuBP regeneration. Under adversity stress, the maintenance of high photosynthetic carbon assimilation capacity is a prerequisite for high yields (Zhao et al., 2021). Rubisco is the rate-limiting enzyme in CBC which plays a vital role in catalyzing CO2 fixation. The activity and expression of Rubisco directly influence the efficiency of CO2 carboxylation and the flow of electrons in the photosynthetic electron transfer chain (Sudhani and Moreno, 2015). RbcL, RbcS, and RCA genes encode the large subunit, small subunit and ribulose-1,5-bisphosphate carboxylase, respectively, which work closely together to regulate the structure and function of Rubisco holoenzymes. Many studies have reported that abiotic stresses (including low light, low temperature, drought, and other stresses) can negatively affect Rubisco and RCA activity and gene expression, affecting the photosynthetic rate of plants (Bi et al., 2017; Wijewardene et al., 2020). Sudhani and Moreno (2015) identified that GSH can convert Rubisco to an active state by modulating the RCA disulfide bond structure. Some reports have demonstrated that a high GSH/GSSG ratio increases the Rubisco activity and consequently CO2 assimilation via enhanced thiol and disulfide exchanges (Jiang et al., 2012). The authors’ previous study demonstrated that exogenous GSH can improve the CO2 carboxylation efficiency of tomato seedlings by up-regulating Rubisco and RCA activity as well as RbcL, RbcS and RCA expression under longer periods of salt stress (5, 10 and 15 d) (Liu et al., 2014). The results of the current study demonstrated that exogenous GSH treatment effectively counteracted the inhibitory effects of short-term salt stress (24, 48 and 72 h) on Rubisco activity, RCA activity, and RbcL, RbcS, and RCA expression. However, the beneficial effects of GSH on CO2 carboxylation efficiency under salt stress were diminished with the addition of exogenous Hb. These results suggested that exogenous GSH improved CO2 carboxylation and photosynthetic electron transfer efficiency in salt-stressed tomato plants, thereby alleviating photoinhibition caused by NADPH accumulation at the PSI receptor site. Furthermore, NO involvement in the positive regulation of GSH on CO2 carboxylation efficiency under NaCl stress was investigated. PGK and GAPDH are the main rate-limiting enzymes in the CO2 reduction phase (Zhang et al., 2011). The activity and gene expression of both directly affected CBC transport efficiency. SBPase (Ding et al., 2016), FBPase (Yu et al., 2020), TK (Bi et al., 2019) and FBA are the key enzymes in the RuBP regeneration stage. The strength of FBPase activity directly affects carbohydrate accumulation and photosynthetic efficiency. SBPase is located at the branching point between the assimilation and regeneration phases of the Calvin cycle, controlling carbon influx and regeneration. FBA catalyzes the cleavage of FBP into DHAP and G3P. These reactions play a role in plant responses to abiotic adversity such as salt stress (Lu et al., 2012). A reduction in TK activity decreases the efficiency of converting carbon-assimilated metabolites, leading to a decline in the photosynthetic rate of plants. CsTK antisense plants inhibit the growth of the capacity to assimilate carbon of Cucumis sativus under cold stress (Bi et al., 2019). This study demonstrated that exogenous GSH treatment effectively alleviated the inhibitory effects of NaCl stress on key enzyme activity and gene expression in the Calvin cycle (PGK, GAPDH, SBPase, FBPase, FBA and TK) (Figures 8, 9). Hb application attenuated GSH effects to varying degrees. These results suggested that GSH enhanced carbon assimilation efficiency, relieved feedback inhibition of photosynthetic products, and promoted CBC operation, thereby mitigating the decline in carbon assimilation capacity caused by salt stress. Furthermore, NO was shown to be involved in the regulation of GSH on CO2 reduction and RuBP regeneration stages under salt stress.
5 Conclusion
In summary, the supplementation of exogenous GSH alleviated photoinhibition and enhanced photosynthetic efficiency in tomato seedlings subjected to NaCl stress. This was achieved through optimizing energy utilization in PSII reaction centers, safeguarding the structure and functionality of photosynthetic reaction centers, enhancing photochemical reactions and energy utilization efficiency, facilitating the operation of the Calvin-Benson Cycle, and alleviating the inhibitory effects of photosynthetic products. Moreover, the application of GSH resulted in an elevation of endogenous NO levels through the induction of the NO synthesis pathway. The presence of Hb attenuated the advantageous impacts of GSH on endogenous NO levels, as well as on the structure and functionality of the photosynthetic apparatus, and the efficiency of carbon assimilation in tomato seedlings under salt stress. These findings validated the role of NO in mediating the beneficial impacts of GSH in ameliorating photosynthesis in tomato seedlings under salt stress.
Data availability statement
The original contributions presented in the study are included in the article/Supplementary Material. Further inquiries can be directed to the corresponding authors.
Author contributions
YC: Conceptualization, Data curation, Methodology, Writing – original draft, Writing – review & editing, Investigation, Visualization, Formal analysis. XC: Writing – original draft, Writing – review & editing, Formal analysis, Investigation. JX: Investigation, Writing – original draft, Writing – review & editing, Formal analysis. XL: Investigation, Writing – original draft, Writing – review & editing. SP: Conceptualization, Writing – original draft, Writing – review & editing. HL: Conceptualization, Funding acquisition, Methodology, Project administration, Resources, Supervision, Writing – original draft, Writing – review & editing.
Funding
The author(s) declare financial support was received for the research, authorship, and/or publication of this article. This work was supported by the National Natural Science Foundation of China (No. 31860550) and earmarked funds for XJARS (XJARS-07).
Conflict of interest
The authors declare that the research was conducted in the absence of any commercial or financial relationships that could be construed as a potential conflict of interest.
Publisher’s note
All claims expressed in this article are solely those of the authors and do not necessarily represent those of their affiliated organizations, or those of the publisher, the editors and the reviewers. Any product that may be evaluated in this article, or claim that may be made by its manufacturer, is not guaranteed or endorsed by the publisher.
Supplementary material
The Supplementary Material for this article can be found online at: https://www.frontiersin.org/articles/10.3389/fpls.2024.1413653/full#supplementary-material
References
Alamri, S., Kushwaha, B. K., Singh, V. P., Siddiqui, M. H., Al-Amri, A. A., Alsubaie, Q. D., et al. (2021). Ascorbate and glutathione independently alleviate arsenate toxicity in brinjal but both require endogenous nitric oxide. Physiol. Plant 173, 276–286. doi: 10.1111/ppl.13411
Bahmani, R., Kim, D., Na, J., Hwang, S. (2019). Expression of the tobacco non-symbiotic class 1 hemoglobin gene hb1 reduces cadmium levels by modulating cd transporter expression through decreasing nitric oxide and ROS level in arabidopsis. Front. Plant Sci. 10. doi: 10.3389/fpls.2019.00201
Baker, N. R. (2008). Chlorophyll fluorescence: A probe of photosynthesis in vivo. Annu. Rev. Plant Biol. 59, 89–113. doi: 10.1146/annurev.arplant.59.032607.092759
Bi, H., Li, F., Wang, H., Ai, X. (2019). Overexpression of transketolase gene promotes chilling tolerance by increasing the activities of photosynthetic enzymes, alleviating oxidative damage and stabilizing cell structure in Cucumis sativus L. Physiologia plantarum 167, 502–515. doi: 10.1111/ppl.12903
Bi, H., Liu, P., Jiang, Z., Ai, X. (2017). Overexpression of the rubisco activase gene improves growth and low temperature and weak light tolerance in Cucumis sativus. Physiol. Plant 161, 224–234. doi: 10.1111/ppl.12587
Chen, X., Zhou, Y., Cong, Y., Zhu, P., Xing, J., Cui, J., et al. (2021). Ascorbic acid-induced photosynthetic adaptability of processing tomatoes to salt stress probed by fast OJIP fluorescence rise. Front. Plant Sci. 12. doi: 10.3389/fpls.2021.594400
Diao, M., Ma, L., Wang, J., Cui, J., Fu, A., Liu, H. (2014). Selenium promotes the growth and photosynthesis of tomato seedlings under salt stress by enhancing chloroplast antioxidant defense system. J. Plant Growth Regul. 33, 671–682. doi: 10.1007/s00344-014-9416-2
Ding, F., Wang, M., Zhang, S., Ai, X. (2016). Changes in SBPase activity influence photosynthetic capacity, growth, and tolerance to chilling stress in transgenic tomato plants. Sci. Rep. 6, 32741. doi: 10.1038/srep32741
Fancy, N. N., Bahlmann, A. K., Loake, G. J. (2017). Nitric oxide function in plant abiotic stress. Plant Cell Environ. 40, 462–472. doi: 10.1111/pce.12707
Fatma, M., Asgher, M., Masood, A., Khan, N. A. (2014). Excess sulfur supplementation improves photosynthesis and growth in mustard under salt stress through increased production of glutathione. Environ. Exp. Bot. 107, 55–63. doi: 10.1016/j.envexpbot.2014.05.008
Gautam, H., Sehar, Z., Rehman, M. T., Hussain, A., AlAjmi, M. F., Khan, N. A. (2021). Nitric oxide enhances photosynthetic nitrogen and sulfur-use efficiency and activity of ascorbate-glutathione cycle to reduce high temperature stress-induced oxidative stress in rice (Oryza sativa L.). Plants Biomolecules 11, 305. doi: 10.3390/biom11020305
Goodarzi, A., Namdjoyan, S., Soorki, A. A. (2020). Effects of exogenous melatonin and glutathione on zinc toxicity in safflower (Carthamus tinctorius L.) seedlings. Ecotoxicol. Environ. Saf. 201, 110853. doi: 10.1016/j.ecoenv.2020.110853
Guo, Y., Lu, Y., Goltsev, V., Strasser, R. J., Kalaji, H. M., Wang, H., et al. (2020). Comparative effect of tenuazonic acid, diuron, bentazone, dibromothymoquinone and methyl viologen on the kinetics of Chl a fluorescence rise OJIP and the MR820 signal. Plant Physiol. Biochem. 156, 39–48. doi: 10.1016/j.plaphy.2020.08.044
Harrison, E. P., Willingham, N. M., Lloyd, J. C., Raines, C. A. (1998). Reduced sedoheptulose-1,7-bisphosphatase levels in transgenic tobacco lead to decreased photosynthetic capacity and altered carbohydrate accumulation. Planta 204, 27–36. doi: 10.1007/s004250050226
Hasan, M. K., Liu, C., Wang, F., Ahammed, G. J., Zhou, J., Xu, M.-X., et al. (2016). Glutathione-mediated regulation of nitric oxide, S-nitrosothiol and redox homeostasis confers cadmium tolerance by inducing transcription factors and stress response genes in tomato. Chemosphere 161, 536–545. doi: 10.1016/j.chemosphere.2016.07.053
Hu, L., Gao, X., Li, Y., Lyu, J., Xiao, X., Zhang, G., et al. (2023). Nitric oxide induced by ammonium/nitrate ratio ameliorates low-light stress in brassica pekinesis: regulation of photosynthesis and root architecture. Int. J. Mol. Sci. 24, 7271. doi: 10.3390/ijms24087271
Jensen, E., Clement, R., Maberly, S. C., Gontero, B. (2017). Regulation of the Calvin - Benson - Bassham cycle in the enigmatic diatoms: biochemical and evolutionary variations on an original theme. Philos. T R Soc. B 372, 20160401. doi: 10.1098/rstb.2016.0401
Jiang, Y., Wang, D., Wen, J. (2012). The independent prokaryotic origins of eukaryotic fructose-1, 6-bisphosphatase and sedoheptulose-1, 7-bisphosphatase and the implications of their origins for the evolution of eukaryotic Calvin cycle. BMC Evol. Biol. 12, 208. doi: 10.1186/1471-2148-12-208
Khan, M., Ali, S., Al Azzawi, T. N. I., Yun, B.-W. (2023). Nitric oxide acts as a key signaling molecule in plant development under stressful conditions. Int. J. Mol. Sci. 24, 4782. doi: 10.3390/ijms24054782
Lee, B. R., Hwang, S. (2015). Over-expression of NtHb1 encoding a non-symbiotic class 1 hemoglobin of tobacco enhances a tolerance to cadmium by decreasing NO (nitric oxide) and Cd levels in Nicotiana tabacum. Environ Exp. Bot. 113, 18–27. doi: 10.1016/j.envexpbot.2015.01.003
Li, H., Chang, J., Chen, H., Wang, Z., Gu, X., Wei, C., et al. (2017). Exogenous melatonin confers salt stress tolerance to watermelon by improving photosynthesis and redox homeostasis. Front. Plant Sci. 8. doi: 10.3389/fpls.2017.00295
Li, Z., Geng, W., Tan, M., Ling, Y., Zhang, Y., Zhang, L., et al. (2022b). Differential responses to salt stress in four white clover genotypes associated with root growth, endogenous polyamines metabolism, and sodium/potassium accumulation and transport. Front. Plant Sci. 13. doi: 10.3389/fpls.2022.896436
Li, X., Wang, S., Chen, X., Cong, Y., Cui, J., Shi, Q., et al. (2022a). The positive effects of exogenous sodium nitroprusside on the plant growth, photosystem II efficiency and Calvin cycle of tomato seedlings under salt stress. Sci. Hortic. 299, 111016. doi: 10.1016/j.scienta.2022.111016
Lilley, R. M., Walker, D. A. (1974). An improved spectrophotometric assay for ribulosebisphosphate carboxylase. Biochim. Biophys. Acta 358, 226–229. doi: 10.1016/0005-2744(74)90274-5
Liu, H., He, X., Xiao, C., Cui, J., Xu, W., Liu, H. (2014). Effects of exogenous GSH on photosynthetic characteristics and expression of key enzyme genes of CO2 assimilation in leaves of tomato seedlings under NaCl stress.(In chinese). Ying Yong Sheng Tai Xue Bao 25, 2637–2644. doi: 10.13287/j.1001–9332.2014.0210
Livak, K. J., Schmittgen, T. D. (2001). Analysis of relative gene expression data using real-time quantitative PCR and the 2-ΔΔCT method. Methods 25, 402–408. doi: 10.1006/meth.2001.1262
Lu, W., Tang, X., Huo, Y., Xu, R., Qi, S., Huang, J., et al. (2012). Identification and characterization of fructose 1,6-bisphosphate aldolase genes in Arabidopsis reveal a gene family with diverse responses to abiotic stresses. Gene 503, 65–74. doi: 10.1016/j.gene.2012.04.042
Mueller-Schuessele, S. J., Wang, R., Guetle, D. D., Romer, J., Rodriguez-Franco, M., Scholz, M., et al. (2020). Chloroplasts require glutathione reductase to balance reactive oxygen species and maintain efficient photosynthesis. Plant J. 103, 1140–1154. doi: 10.1111/tpj.14791
Murchie, E. H., Niyogi, K. K. (2011). Manipulation of photoprotection to improve plant photosynthesis. Plant Physiol. 155, 86–92. doi: 10.1104/pp.110.168831
Parveen, N., Kandhol, N., Sharma, S., Singh, V. P., Chauhan, D. K., Ludwig-Müller, J., et al. (2023). Auxin crosstalk with reactive oxygen and nitrogen species in plant development and abiotic stress. Plant Cell Physiol. 63, 1814–1825. doi: 10.1093/pcp/pcac138
Sarabi, B., Fresneau, C., Ghaderi, N., Bolandnazar, S., Streb, P., Badeck, F.-W., et al. (2019). Stomatal and non-stomatal limitations are responsible in down-regulation of photosynthesis in melon plants grown under the saline condition: application of carbon isotope discrimination as a reliable proxy. Plant Physiol. BIOCH 141, 1–19. doi: 10.1016/j.plaphy.2019.05.010
Semida, W. M., Abd El-Mageed, T. A., Abdalla, R. M., Hemida, K. A., Howladar, S. M., Leilah, A. A. A., et al. (2021). Sequential antioxidants foliar application can alleviate negative consequences of salinity stress in vicia faba L. Plants Basel 10, 914. doi: 10.3390/plants10050914
Shao, R., Wang, K., Shangguan, Z. (2010). Cytokinin-induced photosynthetic adaptability of Zea mays L. to drought stress associated with nitric oxide signal: Probed by ESR spectroscopy and fast OJIP fluorescence rise. J. Plant Physiol. 167, 472–479. doi: 10.1016/j.jplph.2009.10.020
Shi, C., Yang, F., Liu, Z., Li, Y., Di, X., Wang, J., et al. (2021). Uniform water potential induced by salt, alkali, and drought stresses has different impacts on the seedling of hordeum jubatum: from growth, photosynthesis, and chlorophyll fluorescence. Front. Plant Sci. 12. doi: 10.3389/fpls.2021.733236
Simkin, A. J., Lopez-Calcagno, P. E., Raines, C. A. (2019). Feeding the world: improving photosynthetic efficiency for sustainable crop production. J. Exp. Bot. 70, 1119–1140. doi: 10.1093/jxb/ery445
Sudhani, H. P. K., Moreno, J. (2015). Control of the ribulose 1,5-bisphosphate carboxylase/oxygenase activity by the chloroplastic glutathione pool. Arch. Biochem. Biophys. 567, 30–34. doi: 10.1016/j.abb.2014.12.032
Timilsina, A., Dong, W., Hasanuzzaman, M., Liu, B., Hu, C. (2022). Nitrate-nitrite-nitric oxide pathway: A mechanism of hypoxia and anoxia tolerance in plants. Int. J. Mol. Sci. 23, 11522. doi: 10.3390/ijms231911522
Wani, K. I., Naeem, M., Castroverde, C. D. M., Kalaji, H. M., Albaqami, M., Aftab, T. (2021). Molecular mechanisms of nitric oxide (NO) signaling and reactive oxygen species (ROS) homeostasis during abiotic stresses in plants. Int. J. Mol. Sci. 22, 9656. doi: 10.3390/ijms22179656
Wen, Z., Liu, H., Zhou, Y., Xianjun, C., Feng, Y. (2018). The involvement of nitric oxide in exogenous glutathione regulates antioxidant defense capacity against salt stress in tomato seedlings (In chinese). J. Plant Physiol. 54, 607–617. doi: 10.13592/j.cnki.ppj.2017.0432
Wijewardene, I., Mishra, N., Sun, L., Smith, J., Zhu, X., Payton, P., et al. (2020). Improving drought-, salinity-, and heat-tolerance in transgenic plants by co-overexpressing Arabidopsis vacuolar pyrophosphatase gene AVP1 and Larrea Rubisco activase gene RCA. Plant Sci. 296, 110499. doi: 10.1016/j.plantsci.2020.110499
Wu, P., Xiao, C., Cui, J., Hao, B., Zhang, W., Yang, Z., et al. (2021). Nitric oxide and its interaction with hydrogen peroxide enhance plant tolerance to low temperatures by improving the efficiency of the calvin cycle and the ascorbate-glutathione cycle in cucumber seedlings. J. Plant Growth Regul. 40, 2390–2408. doi: 10.1007/s00344-020-10242-w
Xu, J., Wei, Z., Lu, X., Liu, Y., Yu, W., Li, C. (2023). Involvement of nitric oxide and melatonin enhances cadmium resistance of tomato seedlings through regulation of the ascorbate-glutathione cycle and ROS metabolism. Int. J. Mol. Sci. 24, 9526. doi: 10.3390/ijms24119526
Yang, Z., Wang, X., Cui, J., Liu, H., Cui, H., Wu, P. (2023). Nitric oxide and glutathione act synergistically to improve PSII activity and PSI electron transfer under chilling stress in cucumber leaves. J. Plant Growth Regul. 42, 5558–5573. doi: 10.1007/s00344-023-10936-x
Yang, Y., Xie, J., Li, J., Zhang, J., Zhang, X., Yao, Y., et al. (2022). Trehalose alleviates salt tolerance by improving photosynthetic performance and maintaining mineral ion homeostasis in tomato plants. Front. Plant Sci. 13. doi: 10.3389/fpls.2022.974507
Yao, M., Ge, W., Zhou, Q., Zhou, X., Luo, M., Zhao, Y., et al. (2021). Exogenous glutathione alleviates chilling injury in postharvest bell pepper by modulating the ascorbate-glutathione (AsA-GSH) cycle. Food Chem. 352, 129458. doi: 10.1016/j.foodchem.2021.129458
Yu, A., Xie, Y., Pan, X., Zhang, H., Cao, P., Su, X., et al. (2020). Photosynthetic phosphoribulokinase structures: enzymatic mechanisms and the redox regulation of the calvin-benson-bassham cycle. Plant Cell 32, 1556–1573. doi: 10.1105/tpc.19.00642
Yuan, Y., Shu, S., Li, S., He, L., Li, H., Du, N., et al. (2014). Effects of exogenous putrescine on chlorophyll fluorescence imaging and heat dissipation capacity in cucumber (Cucumis sativus L.) under salt stress. J. Plant Growth Regul. 33, 798–808. doi: 10.1007/s00344-014-9427-z
YuPing, J., Fei, C., YanHong, Z., Xiao-Jian, X., WeiHua, M., Kai, S., et al. (2012). Cellular glutathione redox homeostasis plays an important role in the brassinosteroid-induced increase in CO2 assimilation in Cucumis sativus. New Phytol. 194, 932–943. doi: 10.1111/j.1469–8137.2012.04111.x
Zhang, W., He, X., Chen, X., Han, H., Shen, B., Diao, M., et al. (2023). Exogenous selenium promotes the growth of salt-stressed tomato seedlings by regulating ionic homeostasis, activation energy allocation and CO2 assimilation. Front. Plant Sci. 14. doi: 10.3389/fpls.2023.1206246
Zhang, X.-H., Rao, X.-L., Shi, H.-T., Li, R.-J., Lu, Y.-T. (2011). Overexpression of a cytosolic glyceraldehyde-3-phosphate dehydrogenase gene OsGAPC3 confers salt tolerance in rice. Plant Cell Tis Org 107, 1–11. doi: 10.1007/s11240-011-9950-6
Zhao, H., Tang, Q., Chang, T., Xiao, Y., Zhu, X.-G. (2021). Why an increase in activity of an enzyme in the Calvin–Benson cycle does not always lead to an increased photosynthetic CO2 uptake rate?—a theoretical analysis. silico Plants 3, diaa009. doi: 10.1093/insilicoplants/diaa009
Zhou, Y., Diao, M., Cui, J., Chen, X., Wen, Z., Zhang, J., et al. (2018). Exogenous GSH protects tomatoes against salt stress by modulating photosystem II efficiency, absorbed light allocation and H2O2-scavenging system in chloroplasts. J. Integr. Agric. 17, 2257–2272. doi: 10.1016/S2095-3119(18)62068-4
Zhou, Y., Wen, Z., Zhang, J., Chen, X., Cui, J., Xu, W., et al. (2017). Exogenous glutathione alleviates salt-induced oxidative stress in tomato seedlings by regulating glutathione metabolism, redox status, and the antioxidant system. Sci. Hortic. 220, 90–101. doi: 10.1016/j.scienta.2017.02.021
Zhu, J.-K. (2016). Abiotic stress signaling and responses in plants. Cell 167, 313–324. doi: 10.1016/j.cell.2016.08.029
Keywords: Calvin cycle, fast OJIP fluorescence rise, glutathione, nitric oxide, salt stress, tomato
Citation: Cong Y, Chen X, Xing J, Li X, Pang S and Liu H (2024) Nitric oxide signal is required for glutathione-induced enhancement of photosynthesis in salt-stressed Solanum lycopersicum L. Front. Plant Sci. 15:1413653. doi: 10.3389/fpls.2024.1413653
Received: 07 April 2024; Accepted: 31 May 2024;
Published: 17 June 2024.
Edited by:
Fernanda Fidalgo, University of Porto, PortugalReviewed by:
Suchismita Roy, University of California, San Diego, United StatesBruno Sousa, University of Porto, Portugal
Copyright © 2024 Cong, Chen, Xing, Li, Pang and Liu. This is an open-access article distributed under the terms of the Creative Commons Attribution License (CC BY). The use, distribution or reproduction in other forums is permitted, provided the original author(s) and the copyright owner(s) are credited and that the original publication in this journal is cited, in accordance with accepted academic practice. No use, distribution or reproduction is permitted which does not comply with these terms.
*Correspondence: Huiying Liu, hyliuok@aliyun.com; Shengqun Pang, pangshqok@shzu.edu.cn