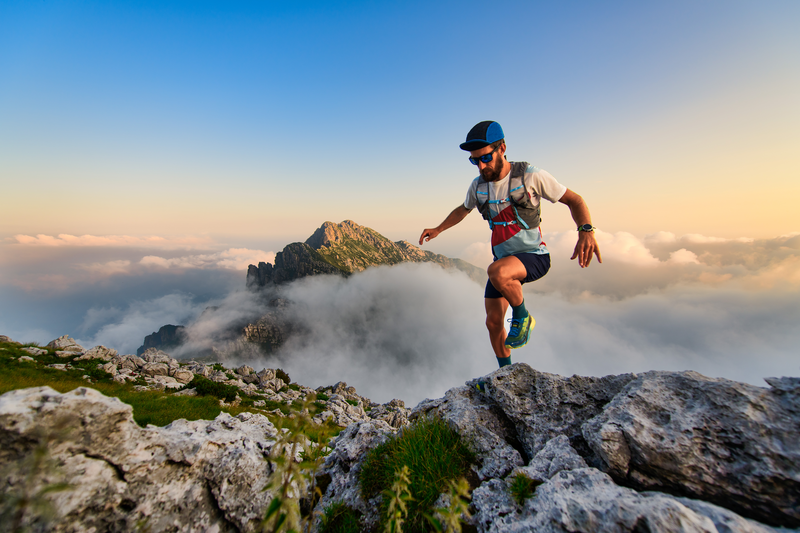
95% of researchers rate our articles as excellent or good
Learn more about the work of our research integrity team to safeguard the quality of each article we publish.
Find out more
ORIGINAL RESEARCH article
Front. Plant Sci. , 30 July 2024
Sec. Plant Nutrition
Volume 15 - 2024 | https://doi.org/10.3389/fpls.2024.1413507
Corn-soybean rotation is a cropping pattern to optimize crop structure and improve resource use efficiency, and nitrogen (N) fertilizer application is an indispensable tool to increase corn yields. However, the effects of N fertilizer application levels on corn yield and soil N storage under corn-soybean rotation have not been systematically studied. The experimental located in the central part of the Songnen Plain, a split-zone experimental design was used with two planting patterns of continuous corn (CC) and corn-soybean rotations (RC) in the main zone and three N application rates of 0, 180, and 360 kg hm-2 of urea in the secondary zone. The research has shown that RC treatments can enhance plant growth and increase corn yield by 4.76% to 79.92% compared to CC treatments. The amount of N fertilizer applied has a negative correlation with yield increase range, and N application above 180 kg hm-2 has a significantly lower effect on corn yield increase. Therefore, a reduction in N fertilizer application may be appropriate. RC increased soil N storage by improving soil N-transforming enzyme activity, improving soil N content and the proportion of soil organic N fractions. Additionally, it can improve plant N use efficiency by 1.4%-5.6%. Soybeans grown in corn-soybean rotations systems have the potential to replace more than 180 kg hm-2 of urea application. Corn-soybean rotation with low N inputs is an efficient and sustainable agricultural strategy.
A two-year crop rotation of corn and soybean is one of the most widely used crop rotation systems in single-crop areas, and this system can increase crop yield, improve soil quality, and reduce environmental pollution. In particular, the inclusion of soybean in a crop rotation enhances biological nitrogen (N) fixation (BNF) in root nodules, provides an effective source of fertilizer for the succeeding crop, and establishes a high-quality elemental cycling system, thus improving soil fertility and alleviating soil fertility degradation. In large-scale agricultural production regions in the United States (US), most farmland is managed by crop rotation (Wallander, 2013). According to the US Department of Agriculture’s Agricultural Resource Management Survey (ARMS), approximately 18% of cropland is continuously planted to corn.
By enhancing biodiversity, the corn-soybean rotation system maintains the carbon and N balance of farmland soils and promotes the synergistic development of ecosystems (Shah et al., 2021). Some scholars consider corn to be a crop that is tolerant to continuous cropping, however, maintaining high yields of corn under continuous cropping requires the continuous input of large amounts of chemical N fertilizer (Gentry et al., 2013). Soybean plants can fix N from the air into the soil for their own use or for use by succeeding crops. Some studies have shown that 60% of the N taken up by soybean plants comes from BNF in root nodules (Ohwaki and Sugahara, 1997), and the remaining N fixed in the soil by rhizobia and N from soybean plant residues can be used by succeeding crops and for the improvement of soil fertility (Hoagland et al., 2008). Beans can fix approximately 39-182 kg N ha−1 (Peoples et al., 2009), and the effect of the corn-soybean rotation system is more pronounced in low fertilizer input systems (Mahmoud et al., 2022). In the corn-soybean rotation system, the decomposition period of corn straw overlaps with the growth period of soybean. Due to the intense deposition of N during straw decomposition, plants in this system fix more N than do those in continuous cropping systems (per plant) (Xu et al., 2020), thus significantly improving the soil nutrient use efficiency (Miyazawa et al., 2010; Tang et al., 2014).
Northeast China is an important corn production base. In this region, corn has been grown continuously cultivated for a long time, the fertilizer input is high, and the N use efficiency (NUE) is significantly lower than the global average. Corn-soybean crop rotation is considered a diversified farming system (DFS) and an effective means for adjusting agricultural cropping structure and improve resource use efficiency. Based on the corn-soybean rotation system and long-term experiments of continuous corn cropping, this study investigated the effects of different N fertilizer input amounts on corn yield and soil N stocks, clarified the effect of using soybean as the preceding crop on the yield increase of subsequent corn crops and soil N stocks, and provides a reference for corn production in Northeast China.
The field experiment was conducted at the Xiangyang Experimental Practice Base (123°22′-126°50′E; 45°34′-45°46′N) of Northeast Agricultural University in Harbin city, Heilongjiang Province, China. The monthly average temperature and rainfall during the experimental period are shown in Figure 1. Located in the central part of the Songnen Plain, the region has a cool temperate continental climate with simultaneous hot and rainy seasons occurring simultaneously. During the experiment, the mean annual average temperature was 5.4°C, the frost-free period was approximately 141 days, the average annual rainfall was 687 mm, and the annual average number of days with daily average temperature ≤0°C was 129. The active cumulative temperature ≥10°C was above 2760°C. The chemical properties of the 0-30 cm soil layer in the experimental plot were as follows: soil organic matter (SOM), 34.29 g kg−1; total N (TN), 1.92 g kg−1; total phosphorus (TP), 0.51 g kg−1; total potassium (TK), 21.60 g kg−1; available N, 100.14 mg kg−1; available phosphorus, 33.82 mg kg−1; and available potassium, 246.49 mg kg−1; pH = 6.14.
The long-term experiment was arranged in the autumn of 2017, and the associated study started in 2018. The split-plot experimental design was adopted, the main plots were for continuous cropping (CC) and corn-soybean rotation cropping (RC), and the secondary plots had three N fertilization levels (N0, N180, and N360). Each plot had 10 ridges, the ridge length was 10.0 m, the ridge spacing was 0.65 m, and the area was 65.0 m2. Each plot had 3 replicates for each experiment. To exclude interannual environmental differences, two corn-soybean rotation experimental areas were established, i.e., RC (2017): soybean (Kenfeng 16) was planted in 2017, and corn (Guoyu 49) and soybean were planted every other year beginning in 2018; RC (2018): corn stubble was left in the field in 2017, and soybean and corn were planted every other year beginning in 2018. The field arrangement is shown in Figure 2. The specific fertilizer rates for the corn and soybean fields are shown in Table 1.
Figure 2 Diagram of the field arrangement used in the experiment. The red line represents the application of 15N-labeled urea in 2022.
The seeds were sown around 5 May every year. Sowing was done manually. Fertilizer was applied in a single pass after ridges were ploughed open and then the ridges were closed to bury the fertilizer There were 60,000 corn plants/hm2 and 300,000 soybean plants/hm2. The harvest occurred around October 5. The management measures used were consistent with those used in local field production. In 2022, two main plots, CC and RC (2017), were selected for the 15N labeling test, which was done by arranging a 15N labeling trial in a randomly selected ridge with a ridge length of 2.5 m within the plots of the two fertilizer treatments (N180 and N360) and applying 15N labelled urea (abundance: 3.603%). Figure 2 shows the detailed field arrangement.
After the 2022 harvest, soil samples were collected from two soil layers, 0-15 cm (upper layer) and 15-30 cm (lower layer), within a ridge using a 3 cm diameter soil auger. For each plot, soil samples were collected from 5 sampling points, mixed into one sample, and residues such as plant roots were manually removed. Each treatment had 3 replicates; the soil samples were thoroughly mixed and then divided into two parts: one was air dried and used for the determination of soil organic N fractions and TN; and the other was stored in a -20°C freezer for the determination of soil inorganic N. In addition, undisturbed soil from two soil layers, 0-15 cm (upper layer) and 15-30 cm (lower layer), was collected with a 100 cm3-ring-knife soil sampler for analysis of soil bulk density.
One gram of air-dried soil was passed through a 100-mesh sieve, concentrated sulfuric acid and a mixed catalyst were added for digestion at 360-410°C, and the TN content was measured by the Kjeldahl N analyzer.
According to the methods described by Bremner (Bao, 2000), an air-dried soil sample containing approximately 10.00 mg of N was weighed, mixed with 2 drops of n-octanol and 20 mL of 6 mol/L HCl, and subsequently hydrolyzed at 120 ± 3°C for 12 h on a hot plate. After hydrolysis, filtration was performed, the filtrate was neutralized until the pH reached 6.5 ± 0.1, and the solution was diluted to 100 mL. MgO was added to the acidolysis solution and distillation was carried out to obtain the acid hydrolysed ammonia N (AN) content. The acidolysis solution was added to borate-phosphate buffer for distillation, and by subtracting the AN content from the obtained result, the acid amino sugar N (ASN) content was obtained. Concentrated sulfuric acid and catalyst were added to the acidolysis solution for digestion at 200°C in the digestion furnace, followed by distillation, and the total soil acid hydrolyzed N (THAN) content was obtained. The alkaline hydrolysis solution was treated with NaOH and then placed in a water bath at 100°C after adding citric acid and ninhydrin. Finally, the borate-phosphate buffer was added for distillation to obtain acid hydrolyzed amino acid N (AAN); the acid hydrolyzed unknown N (HUN) content was obtained by subtracting AN, ASN and AAN from THAN; and the non-acid hydrolyzed N content was obtained by subtracting THAN from soil TN.
The KCl extraction-distillation method was used. First, 10.00 g of fresh soil was weighed and extracted with 50 mL of 2 mol/L-1 KCl; 0.72 g of MgO was added to part of the extract and the soil NH4+-N concentration was determined by distillation using a Kjeldahl N analyser; the mixture of 0.72 g of MgO, 0.6 g of FeSO4 and zinc powder was added to another part of the extract, and the soil mineral N concentration was measured using a Kjeldahl N analyzer. The soil NO3--N concentration was calculated by subtracting the NH4+-N concentration from the mineral N concentration.
The chloroform fumigation method (Vance et al., 1987) was used. After the extraction of soil samples fumigated with chloroform for 24 h, the organic N content was determined by a Kjeldahl N analyzer. Soil MBN content was obtained by dividing the difference in organic N content between fumigated and unfumigated soil by kEN (0.57).
Soil enzyme activity was determined using a soil enzyme kit from Solarbio Biotech Co., Ltd., Beijing, China (Ref.). The kit included tests for urease (product No. BC0125), nitrate reductase (product no. BC3105), nitrite reductase (product no. BC2995), leucine aminopeptidase (product no. BC4025), and N-acetyl-β-D-glucosidase (product no. BC4005).
On 19 June (seedling stage), 8 July (jointing stage), 1 August (tasseling stage), 17 August (silking stage), 27 August(grain-filling stage), 13 September (milk stage) and 3 October (maturity stage) of 2022, plant samples were collected, fixed at 105°C for 30 min, dried at 80°C, weighed for dry matter weight, and subsequently crushed and digested with concentrated sulfuric acid. The N content of the plant was determined by a Kjeldahl N analyzer. The titrated sample solution was concentrated and reacted with lithium hypobromite under refrigerated vacuum conditions to produce the N2, and the 15N abundance was determined by isotope ratio mass spectrometer (Thermo-Fisher, USA) using dual channel (DI) measurements.
During the corn maturity stage, an area of 5 m long and 1.3 m wide (2 ridges wide) was selected as the sampling area in each plot. All ears in the sampling area were harvested. After air-drying, the water coefficient was measured, and the yield was converted with the water content being 14%.
The calculation method of the TN stocks and increase for a corresponding soil layer refers to Yan et al. (2023). The TN stocks was calculated as:
where NStock indicates the TNstock (kg hm−2) and BDi and Hi indicate the soil bulk density (g cm−3) and the thickness (m) of the corresponding soil layer, respectively. The increase in soil N stock was calculated as:
ΔNStock is the increase in soil N stock, NStock(RC) is the soil N stock under the RC treatment, NStock(CC) is the soil N stock under the CC treatment, and t is the year. The calculation method of nitrogen use efficiency was referred to Rocha et al. (2019). The proportion of N in the sample from fertilizer was calculated as:
Ndff: proportion of N in the sample from fertilizer; ft: 15N abundance of the treated sample; f0: natural abundance of 15N, 0.365%; fN: 15N abundance in the fertilizer, 3.603%. The N accumulation was calculated as:
where W is the N accumulation in the sample in mg; m is the sample dry weight in g; and ρ is the N content in the sample in mg g-1. The plant N accumulation from the fertilizer was calculated as:
where NCf is the plant N accumulation from the fertilizer in mg plant-1, Wi is the N accumulation in different organs of the plant in mg, and Ndffi is the proportion of N from the fertilizer in the corresponding organ. The N use efficiency was calculated as:
NUE: N use efficiency; Nf: N fertilizer rate, unit: mg plant-1. The straw-grain ratio was calculated as:
where, m1 is the dry weight of the plant stalk in g plant-1, m2 is the dry weight of the plant leaves in g plant-1, and M is the dry weight of the plant grains in g plant-1.
SPSS 18.0 software (IBM Software, Chicago, IL, USA) was used for data management and multivariate analysis. The means were compared by one-way analysis of variance (ANOVA), P ≤ 0.05. The Pearson correlation coefficient was calculated to determine the correlations between indicators. Origin 2022 software (OriginLab, Northampton, MA, USA) was used to plot figures. Nomenclature
Based on the analysis of five years of yield data, both N application and preceding soybean cultivation significantly increased corn yield (Table 2), and corn yield varied significantly among years, ranging from 4,468.26 to 11,040.52 kg hm-2. Under the CC treatment, the yield increase under the N180 fertilization treatment was 32.07%-87.85%, with an average yield increase of 55.72% compared with that under the N0 fertilization treatment. Similarly, the yield increase under the N360 fertilizer treatment compared to the N0 fertilizer treatment was 38.25%-93.19%, with an average yield increase of 65.50%. Under the same N application rate, compared with the CC treatment, the RC treatment significantly increased the corn yield. Under the N0 fertilization, the yield increase with preceding soybean plants was 31.13%-79.92%, with an average yield increase of 43.27%. The yield increase of the crop rotation system under the N180 fertilization treatment was in the range of 4.76%-39.03%, with an average yield increase of 14.12%. The rotation yield increase under the N360 fertilizer treatment ranged from 7.06% to 36.67%, with an average yield increase of 16.85%. Analysis of yield components showed that the yield increase resulting from crop rotation was mainly due to a significant increase in the diameter of corn ears, while the effect of fertilization on yield components was manifested as a significant increase in ear length, ear diameter and 100-kernel weight (Supplementary Figure 1). A comprehensive analysis of the yield-enhancing effect of crop rotation and N fertilization showed that the corn yield in the treatment with preceding soybean plants and no N application was equivalent to that of continuously cropped corn at the N180 level.
Samples of corn were taken in 2022 during the seven main growth periods. Analysis of the dry matter weight showed that the treatment with preceding soybean cultivation and N fertilization significantly increased the dry matter weight of the corn plants to different degrees (Figure 3), and the dry matter weight increased gradually with increasing fertilization application rate. On 19 June (corn seedling stage), the dry weights of the shoots and underground parts of corn plants under the N360 and N180 treatments were significantly greater than those under the N0 treatment, and there was no significant difference between the RC and CC treatments under the same N level (Figure 3A). After 8 July, the dry weight in the RC treatment was significantly greater than that in the CC treatment at the same N fertilization level. During this period, the preceding cultivation of soybean plants promoted the growth and dry matter accumulation of corn plants, and the dry weight increased with increasing fertilizer application rate (Figures 3B–G). As shown in Figures 3D, E, the dry weight of the ears of corn in the RC treatment was between 70.85 g and 107.97 g, accounting for 34.65%-38.96% of the dry weight of the whole plant; the dry weight of the ears in the CC treatment was between 24.21 g and 58.51 g, accounting for 20.18%-28.50% of the dry weight of the whole plant. On 13 September (the milk stage), there was no significant difference between the different N levels in the RC treatment, and the dry weight of plants at the N360 level of the CC treatment was significantly higher than that of plants at the N180 and N0 levels, with the difference in dry weight ranging from 95.43g to 107.07g (Figure 3F). On 3 October (the maturity stage), the dry matter weight in the RC treatment was 59.6-125.8 g/plant greater than that in the CC treatment at the same fertilization level, with an average increase of 85.0 g/plant, and notably, the seed dry weight at the N0 level under the RC treatment was comparable to that at the N360 level under CC treatment. As is seen with Equations (7) In the RC treatment, differences in the straw-grain ratio among different N fertilizer levels were not significant; in the CC treatment, the straw-grain ratio gradually decreased with increasing N application rate, and that at the N0 level was significantly higher than that at the N360 level, suggesting that more dry matter accumulated in the stalks when the N supply was insufficient (Figure 3H).
Figure 3 Changes in the dry matter weight and grass-grain ratio of maize plant. (A–G) are the dry matter weight of June 19, 2022 (seedling stage), July 8, 2022 (jointing stage), August 1, 2022 (tasseling stage), August 17, 2022 (silking stage), August 27, 2022 (grain-filling stage), September 13, 2022 (milk stage), October 3, 2022 (maturity stage), (H) is the grass-to-grain ratio of mature plants. The lowercase letters are the significant differences between different fertilization gradients in the same stubble, p < 0.05.
The leaves and grains of the corn plants had the highest N contents, and the preceding cultivation of soybean and N fertilization significantly increased the N contents in different parts of the corn plants (Figure 4), but did not change the proportion of N in each organ. The N content of each organ of the corn plant increased with increasing N application rate, and at the same N level, the N content in the same plant part was significantly higher in RC treatment than in CC treatment. Nitrogen fertilization and crop rotation had greater effects on leaf and grain N contents. In the CC treatment, the N content in kernels was higher than that in leaves at the N0 level, and the N content in the parts of the corn plant in the other treatments was in the order of leaves, kernels, rootstalks, and ears, suggesting that there was a transfer of N from the leaves to the kernels in the CC treatment. In the RC treatment, the differences in the N content of the corn leaves between the three N fertilization levels were significant, while the N contents at the other locations were not significantly different between the N360 and N180 treatments and were significantly greater than those at the N0 level. In the CC treatment, the differences in the N contents in the corn roots, stalks and grains between the three N fertilization levels were significant, while the N contents in the leaves and ears were not significantly different between the N360 and N180 treatments and were significantly higher than those in the N0 treatment.
Figure 4 N contents in plants. The lowercase letters are the significant differences between different fertilization gradients in the same stubble, p < 0.05.
As corn grew, the abundance of 15N in the plants gradually decreased, indicating that corn absorbed more N in the early growth phase (Table 3). Between 19 June and 1 August, the difference in 15N abundance in the same part of the corn plants under the different N levels in the same treatment was not significant. However, on 19 June, the 15N abundance in the shoots of the RC treatment was significantly lower than that of the CC treatment, indicating that the uptake of soil N by the corn plants occurred earlier in the RC treatment than in the CC treatment. On 27August, the 15N abundance in the corn roots at the N360 level was significantly higher than that at the N180 level, and under the same N application level, the 15N abundance in the corn roots and stems in the CC treatment was significantly higher than that in the RC treatment. On 13 September, the pattern of 15N abundance in corn roots, stems and ears was consistent among treatments, showing that the 15N abundance at the N360 level was significantly higher than that at the N180 level for the same treatments, and the 15N abundance in the CC treatment was significantly higher than that in the RC treatment at the same N application level. At the mature stage and at the N180 and N360 levels, the 15N abundance in each part of the corn in the RC treatment was significantly lower than that in the CC treatment.
The nitrogen accumulation in plant samples was calculated by Equation (4). The N accumulation and N content in the corn plants were similar between the treatments. Under the same N fertilization level, N accumulation in the RC treatment was significantly greater than that in the CC treatment, and N accumulation in each part gradually increased with increasing N fertilization rate (Figure 5A). The N accumulation in plants followed the order of grain > leave > stalk > root > ear, and the grain N accumulation accounted for 56.7-65.0% of the total accumulation. Under the CC treatment, grain N accumulation at the N0 level accounted for the highest proportion (65.0%), indicating that when N is insufficient, the N accumulated in the plant is preferentially supplied to the grains. As is seen with Equations (3), (5) and (6). The NUE calculated by 15N labelling technology (Figure 5B) showed that the NUE ranged from 24.36%-36.45%. Under the N180 and N360 levels, the NUE in the RC treatment was 5.6% and 1.4% greater than that in the CC treatment, respectively. Crop rotation can improve soil NUE, especially in cropping systems with low N inputs.
Figure 5 Plant N accumulation and NUE. (A) is the nitrogen accumulation in different parts of the maize plant at the mature stage, (B) is the NUE of the plant under the nitrogen application treatment, and the lowercase letters are the significant differences in the nitrogen utilization rate under the four treatments, p < 0.05.
Crop rotation and N fertilization significantly increased the soil N components and the mineral N and TN contents, and these increased with increasing the N fertilizer application rates (Table 4). The soil N content in the 0-15 cm soil layer was significantly greater than that in the 15-30 cm soil layer. The soil TN and non-acid hydrolyzed N contents in the RC treatment increased by 0.25-0.44 g kg-1 and 0.14-0.31 g kg-1, respectively, in the 0-15 cm soil layer, and increased by 0.28-0.39 g kg-1 and 0.12-0.24 g kg-1, respectively, in the 15 cm-30 cm layer, compared to those in the CC treatment. The amino acids N, ASN, AN, and HUN in the RC treatment increased by -11.49-91.96 mg kg-1, 5.02-39.42 mg kg-1, 67.4-127.24 mg kg-1, and -53.66-13.85 mg kg-1, respectively, in the 0-15 cm soil layer, and increased by 5.5-106.76 mg kg-1, -34.81-8.18 mg kg-1, 60.22-74.8 mg kg-1, 20.5-59.18 mg kg-1, and 116.11-233.63 mg kg-1, respectively, in the 15 cm-30 cm layer, compared with those in the CC treatment. The proportions of soil organic N components in different soil layers changed little. Overall, the stability of the organic N components in the 0-15 cm soil layer was greater than that in the other layers (Supplementary Figure 2). The MBN in the 0-15 cm soil layer was significantly greater than that in the 15-30 cm layer, the soil MBN in the RC treatment was greater than that in the CC treatment, and the patterns in the upper and lower soil layers were consistent, indicating that there was no significant pattern in the effect of N fertilization on MBN.
Soil mineral N is the main form of N taken up by crops. The nitrate N content was significantly higher than the ammoniacal N content, which increased with increasing N application rate. In the 0-15 cm soil layer and at the N360 level, the ammoniacal N content in the RC treatment was 2.96 mg kg-1 higher than that in the CC treatment, and the difference was significant. The differences between the other treatments were not significant. The nitrate N content in the RC treatment was significantly higher than that in the CC treatment, and the increase in nitrate N was in the range of 0.92-6.79 mg kg-1. In the N180 and N360 treatments and in the 15-30 cm soil layer, the ammoniacal N content in the RC treatment was significantly greater than that in the CC treatment, with increases of 2.26 mg kg-1 and 1.96 mg kg-1, respectively. At the N0 level, the nitrate N content in the RC treatment increased by 5.09 mg kg-1 compared to the CC treatment, and the difference was significant.
The activities of soil N-transforming enzymes increased gradually increased with N fertilizer (Table 5). At the same N fertilization level and in the same soil layer, the soil enzyme activities in the RC treatment were significantly higher than those in the CC treatment, indicating that preceding soybean cultivation significantly increased the activities of enzymes related to soil N transformation and improved the N supply capacity in the soil. Moreover, the enzyme activity in the 0-15 cm soil layer was greater than that in the 15-30 cm soil layer, indicating that the N turnover efficiency of the surface soil was greater. The enzyme activities of NAG, urease, NR, NiR, and LAP in the RC treatment increased by 0.005-2.72U g-1, 38.58-147.94U g-1, 0.28-0.54U g-1, 5.89-18.49U g-1 and 0.19-0.47U g-1, respectively, in the 0-15 cm soil layer, and increased by 0.13-1.63U g-1, 44.17-119.53U g-1, 0.38-0.48U g-1, 7.55-10.47U g-1 and 0.10-0.37U g-1, respectively, in the 15 cm-30 cm layer, compared to those in the CC treatment.
An analysis of the N content and N transformation related enzyme activities in the two soil layers and two crops showed that with the exception of MBN, most soil N components and enzymes were significantly correlated (Figure 6). In particular, in the 0-15 cm soil layer of the RC treatment, a significant positive correlation was detected between the N components and enzyme activities (Figure 6A). In the 0-15 cm soil layer of the CC treatment, four indicators, AN, NO3−-N, NH4+-N, and NiR, correlated poorly with the other components (Figure 6B). In the 15-30 cm soil layer of the RC treatment, except for ASN, UN, and MBN, the correlations between the other indicators were greater (Figure 6C). In the 15-30 cm soil layer of the CC treatment, AN, UN, MBN, and NO3−-N were poorly correlated with the other indicators (Figure 6D).
Figure 6 Plant N accumulation and NUE. (A) shows the 0-15 cm soil layer in the RC treatment, (B) shows the 0-15 cm soil layer in the CC treatment, (C) shows the 15-30 cm soil layer in the RC treatment, and (D) shows the 15-30 cm soil layer in the CC treatment.
Based on the differences in the soil N indicators and yield differences between the RC and CC treatments, a random forest model was used to analyze the contributions of changes in the different indicators to yield changes (Figure 7). Specifically, the soil indicators in the 0-15 cm soil layer whose changes contributed more than 10% to the yield were AN, UN, NO3−-N, ASN, AAN and urease (Figure 7A), while in the 15-30 cm soil layer, they were ASN, NAG, NO3−-N, and urease (Figure 7B). In the two soil layers, NO3−-N, ASN and urease were important indicators of corn yield increase, and the average contribution rates to corn yield increase were 12.75%, 15.30% and 10.35%, respectively.
Figure 7 Contribution rates of the N components to yield. (A) is the contribution rate of changes in soil N indicators in the 0-15 cm soil layer to yield increase; (B) is the contribution rate of changes in soil N indicators in the 15-30 cm soil layer to yield increase.
Based on the soil TN content (Table 4) and soil bulk density (Supplementary Table 1), the increase in N fixation per unit area under the same N level between the RC and CC treatments was estimated. In this study, the RC treatment was applied for a total of 3 years, with the rotation cycle starting with soybean cultivation in 2017, the increase in soil N stock per unit area in each rotation cycle (2 years) was calculated by the Equations (1) and (2). In each rotation cycle (2 years), the N fixation per unit area of N0, N180 and N360 in the RC treatment increased by 149.7 kg hm-2, 66.75 kg hm-2 and 242.7 kg hm-2, respectively, in the 0-15 cm soil layer, and by 158.3 kg hm-2, 106.45 kg hm-2 and 102.5 kg hm-2, respectively, in the 15 cm-30 cm layer, compared to that in the CC treatment. The N fixation per unit area in the 0-30 cm tillage layer of corn and soybeans planted alternately was 86.6-172.6 kg hm-2. Excessive N fertilizer application in corn-soybean rotation significantly increased N stock in the 0-15 cm soil layer (Figure 8).
Crop rotation is considered an environmentally friendly strategy for sustainable agriculture. It effectively manages nutrients, water, weeds, and pests, while maintaining soil structure and fertility, thereby enhancing crop yields (Tilman et al., 2002; Castellazzi et al., 2008; Bender et al., 2016; German et al., 2017). In particular, cereal-legume rotations (nutrient-rich preceding crops) are the most favored, significantly boosting the yield of subsequent crops by up to 27%. This is well-supported by previous studies involving quantitative meta-analyses based on local field experiment data (Preissel et al., 2015; Cernay et al., 2018; Franke et al., 2018). In this study, under two nitrogen application levels (N180 and N360), the yield increased from planting soybeans as the previous crop compared to continuous maize cropping ranged from 4.76% to 39.03%, further confirming the yield-enhancing effect of soybean as a precursor crop for maize. This further confirms the yield-boosting effect of soybean as a preceding crop for maize. Research by Evans et al. (1991) and Zang et al. (2015) has demonstrated that legume-based rotation systems yield 14% more than systems without legumes, and it is mainly due to the nitrogen-fixing ability of legumes and the nitrogen-rich residues they leave behind, which significantly enhance nitrogen input for subsequent crops. Moreover, in this study, the impact of preceding crops on the yield and nutrient content of subsequent crops varied significantly. Soybean as a preceding crop notably increased the dry matter weight and nitrogen content of maize plants. This rotation system significantly reduced the stover-to-grain ratio in maize, leading to differences in dry matter distribution among grains, stems, and leaves.
The maize-soybean rotation improves the biochemical properties of the soil. Biological nitrogen fixation (BNF) is a significant way through which soybean plants increase soil nitrogen content (Thorup-Kristensen et al., 2003) and is crucial for stabilizing soil nutrient supply (Jantalia et al., 2007). In this study, the maize-soybean rotation significantly increased total nitrogen (TN) content in the soil, ensuring an adequate supply of available nitrogen. In the 0-15 cm and 15-30 cm soil layers, the TN content in the rotation treatment (RC) was significantly higher than in the continuous cropping treatment (CC). Additionally, the RC treatment reduced the proportion of acidolysis unknown nitrogen (UN) and the difficulty of soil organic nitrogen mineralization, while increasing the proportion of amminia nitrogen (Supplementary Figure 2). This is important because crop nitrogen uptake primarily relies on mineral nitrogen, which is a key indicator of soil nitrogen supply capacity. Soybeans in the rotation system exhibit high recovery capabilities for NH4+ and NO3- (Zhang et al., 2008; Shen et al., 2018). In this study, the content of ammonium nitrogen and nitrate nitrogen in the 0-15 cm soil layer was significantly higher in the maize-soybean rotation treatment compared to continuous maize cropping. This increase provided more soil-based mineral nitrogen for the subsequent maize crop, reducing the need for nitrogen fertilizer input. Peoples et al. (2009) and Barcelos et al. (2022) also found that legumes could fix approximately 39-182 kg N ha−1, and the increase in soil nitrogen reserves could regulate nitrogen fertilizer application. When fertilizer input is low during production, the rotation treatment demonstrates a stronger nutrient supply capacity due to the high nutrient demand of cereals. This aligns with our findings that maize yields were relatively high even when no nitrogen fertilizer was applied to the preceding soybean crop (Figure 3) (Xu et al., 2020; Mahmoud et al., 2022).
The content of soil organic nitrogen (SON) is of great significance for maintaining soil nitrogen fertility and determining soil nitrogen supply capacity. It is an important indicator of soil fertility (Tang et al., 2021). The composition, content, and ease of mineralization of soil organic nitrogen are closely related to soil nitrogen supply characteristics (Wu et al., 2021). Numerous studies have shown that the amount of nitrogen applied is a significant factor affecting the content of soil organic nitrogen components (He et al., 2018; Li et al., 2019; Sebastian et al., 2023). This finding aligns with our study, which also demonstrates that different levels of nitrogen fertilizer application in both rotation and continuous cropping systems significantly alter the composition of soil organic nitrogen components (Table 4). However, the relationship between nitrogen application rates and various soil organic nitrogen components is not entirely consistent with literature reports. Many studies suggested that acid-hydrolyzable non-protein nitrogen (ANN) and acid-hydrolyzable ammonium nitrogen (AN) were readily consumed during soil total nitrogen depletion (He et al., 2018; Ma et al., 2020; Tang et al., 2021), while other studies reported no impact of fertilization on ANN and AN content. For instance, Gao et al. (2009) found no significant effect of chemical fertilizers on the content of organic nitrogen and its components in a 29-year maize-maize-soybean rotation system in Shenyang. Similarly, Zhang et al. (2015) reported no significant effect of nitrogen application rates (180, 225, 270 kg/hm²) on soil total nitrogen and organic nitrogen components in a 7-year wheat-maize rotation system in Hebi, Henan.
In contrast, this study found that nitrogen application rates directly affect the content of ANN and amino sugar nitrogen (ASN) in the maize-soybean rotation system. This phenomenon may be attributed to factors such as the saturation level of adsorption sites on soil particles for organic nitrogen components and the nitrogen fertilizer application level. ANN primarily originates from soil fixed ammonium, while ASN mainly derives from the degradation of soil microbial cell walls. The content of these components is related to the retention capacity of soil colloids. In this study, long-term high fertilization in arable land accumulated high levels of soil organic nitrogen components. The stronger interactions among different components in competition for adsorption sites might be influenced by the adsorption retention capacity of soil colloids, playing a key role in the changes in component content.
ASN is a major component of total acid-hydrolyzable nitrogen, and its content responds significantly to changes in nitrogen application rates. Experimental results indicated that ASN content in the soil could reach 157.69-214.29 mg/kg. This finding is not entirely consistent with some literature reports. For example, Tang et al. (2021) and Jiao et al. (2020) reported that ASN accounted for a relatively low proportion of TNex (total nitrogen extractable) and showed a low response to changes in nitrogen application rates. However, other studies suggested that ASN could have higher concentrations in the soil. Hao et al. (2015) reported that ASN content could reach 175.1 mg/kg when organic and inorganic fertilizers were used in combination on black soil in Northeast China. Similarly, Ji et al. (2020) found that ASN content could reach 152.62 mg/kg in black soil surveys. Wu et al. (2021) also reported that ASN could reach 137.95 mg/kg in no-tillage maize fields in Northeast China. These results indicate that ASN can accumulate in high-yield, high-input systems. High crop yields result in more organic matter residues in the soil and higher microbial activity, leading to the accumulation of ASN derived from microbial residues in the soil (Zhao et al., 2014; Ma et al., 2018).
Crop rotation can improve soil conditions, promote the proliferation of beneficial microorganisms, and increase nitrogen mineralization and nitrification processes, thereby enhancing the availability of nitrogen in the soil (Yuan et al., 2022). This study found that the content of soil nitrogen components was significantly higher under the soybean rotation treatment compared to continuous maize cropping, consistent with the findings of Yuan et al. (2022). This is because crop rotation helps to promote soil microbial diversity and activity. Nitrogen forms such as acid hydrolyzable ammonium nitrogen and acid hydrolyzable unknown nitrogen contribute to the structural and functional composition of plants, support microbial activity in the soil, and enhance soil fertility and health (Li et al., 2021).
Crop rotation significantly increased soil nitrogen reserves (Supplementary Table 2), although the annual average increased in soil nitrogen reserves varied significantly under different nitrogen levels. Notably, under the N0 treatment, the nitrogen reserve in the 0-30 cm soil layer was 67.4 kg/ha higher than that under the N180 treatment. This finding aligns with the results of Lin et al. (2021) and Salvagiotti et al. (2008) which indicate that low nitrogen input conditions enhance the nitrogen-fixing effect of preceding soybean crops. The main difference in nitrogen reserves between the N360 and N180 levels was observed in the 0-15 cm soil layer. We believe that the continuous high nitrogen fertilizer application is the primary cause of this difference. Returning crop residues to the field further adjusted the soil carbon-to-nitrogen ratio, triggering the mineralization of natural organic carbon in the short term and enhancing the soil’s nitrogen fixation capacity (Guenet et al., 2010; Kirkby et al., 2014; Li et al., 2022).
This study measured the microbiological characteristics of the 0-30 cm soil layer and found that urease and microbial biomass nitrogen (MBN) significantly increased under the rotation treatment. This might be due to the soluble carbon (C) and nitrogen (N) released from crop residues, which enhanced microbial activity, thereby improving nitrogen mineralization and supply capacity (Yang et al., 2021). We observed that in the maize-soybean rotation system, the activity of nitrogen transformation-related enzymes increased correspondingly with higher nitrogen fertilizer application rates. Similarly. The experiments with different tillage methods and crop residue incorporation of Yang (2023) showed significant enhancement in nitrogen transformation-related enzyme activity in the wheat-peanut rotation system. These findings further reflect the positive impact of crop rotation and nitrogen fertilizer application on soil enzyme activity. The practice of crop rotation combined with crop residue incorporation can alter the source of soil nutrients, leading to enhanced microbial communities and soil enzyme activity (Yang et al., 2021). The growth of certain microbial groups in the soil, such as oligotrophic bacteria, may help utilize nutrients from soil organic matter (SOM) and, following crop rotation and residue incorporation, promote microbial utilization of soil nutrients (Fierer et al., 2007; McDaniel and Grandy, 2016). In this study, enzyme activity in the RC treatment was greater than in the CC treatment, indicating that residues from preceding soybean crops favor microbial proliferation and the production of more enzymes (Lian et al., 2019). The increase in soil microbial and enzyme activity can promote the transformation of crop residues into soil organic matter (SOM), enhance nutrient use efficiency, and partially replace nitrogen fertilizers. This improvement is reflected in soil nutrient content and maize yield (Wei et al., 2019).
The nitrogen fertilizer applied to the soil has three possible fates: being absorbed by the crop, remaining in the soil, and being lost from the system (Ruisi et al., 2016). This study found that the dry matter weight of maize plants increased with the amount of fertilizer applied, and the abundance of 15N showed a similar trend. The highest 15N abundance was observed at the seedling stage of maize, gradually decreasing as the plants grew. This indicates that maize plants rely more on fertilizer nitrogen during the seedling stage (Jiang et al., 2011). After the tasseling stage, plants absorbed more soil nitrogen, as evidenced by the rapid accumulation of plant dry matter and the gradual decrease in 15N abundance.
At the seedling stage, the impact of nitrogen fertilization on root 15N abundance was relatively small, while the 15N abundance in the stems and leaves of the CC treatment was significantly higher than that of the RC treatment. This indicates that continuous maize cropping requires more fertilizer nitrogen (Gentry et al., 2013). The maize-soybean rotation altered the form and proportion of nitrogen in the soil and increased the soil’s nitrogen supply capacity (Li et al., 2013), as reflected by higher stem and leaf nitrogen content and lower 15N abundance in the RC treatment. Notably, in the RC treatment, the 15N abundance in the roots at the seedling stage was significantly higher than in the stems and leaves (Table 2). Combined with soil nitrogen composition data, this study suggests that the soil nitrogen supply in the preceding soybean crop treatment is more conducive to promote plant growth and increase yield (Armstrong et al., 1997).
Nitrogen fertilizer plays a crucial role in promoting soybean and maize growth and increasing grain yield (Jing et al., 2009). However, in the black soil regions of Northeast China, nitrogen fertilizer application exceeding 250 kg ha-1 for maize leds to decrease nitrogen use efficiency (NUE) as nitrogen application increased (Li et al., 2022), especially with additional basal nitrogen fertilization aftered maize straw return, which could significantly reduce NUE (Tian et al., 2018). Therefore, in high-input agricultural areas, it is recommended to control nitrogen fertilizer application to improve fertilizer efficiency. However, reducing nitrogen fertilizer application while maintaining high yields remains a global challenge.
In suitable ecological zones, crop rotation involving legumes like soybeans is an effective strategy to improve fertilizer efficiency (Ju et al., 2009). In Northeast China, excessive pursuit of crop yields by producers has led to severe overuse of fertilizers in the past 20 years (Geng et al., 2023). In this study, planting maize after preceding soybeans at the N180 level achieved both high yield and high nitrogen use efficiency. Importantly, nitrogen fertilizer use efficiency in continuous maize cropping was significantly lower compared to the preceding soybean treatment. Similar findings by Wu et al., 2022 suggested that for maintaining high yields and improving NUE, rational nitrogen fertilization under crop rotation with straw return was advisable.
The preceding soybean crop significantly increased the yield of the subsequent corn crop, and the yield increase was significantly influenced by the amount of N applied. Both preceding soybean plants and N fertilization increased the activities of soil N-transforming enzymes, which in turn increased the soil N content, improved the proportions of soil organic N components, and increased the soil N stocks; allowing N fertilizer inputs for the succeeding crop to be reduced. A corn-soybean rotation with low N input is an effective and sustainable strategy for increasing soil NUE, improving soil N supply characteristics and maintaining the stability of agricultural production.
The original contributions presented in the study are included in the article/Supplementary Material. Further inquiries can be directed to the corresponding author.
CY: Writing – review & editing, Data curation, Formal analysis, Funding acquisition, Methodology, Visualization, Writing – original draft. YY: Data curation, Writing – original draft, Investigation, Validation. JS: Data curation, Investigation, Validation, Writing – original draft. FS: Data curation, Validation, Writing – original draft, Visualization. XL: Data curation, Validation, Visualization, Writing – review & editing. SY: Validation, Visualization, Writing – review & editing. CW: Validation, Visualization, Writing – review & editing. QS: Writing – review & editing, Conceptualization, Project administration, Resources. CM: Writing – review & editing, Conceptualization, Project administration, Resources.
The author(s) declare financial support was received for the research, authorship, and/or publication of this article. This research was funded by National Key R&D Program of China (No. 2023YFD1501600) and Natural Science Foundation of Heilongjiang (YQ2022C031).
We want to thank all the members of our team who have contributed by involving in the experiment process.
The authors declare that the research was conducted in the absence of any commercial or financial relationships that could be construed as a potential conflict of interest.
All claims expressed in this article are solely those of the authors and do not necessarily represent those of their affiliated organizations, or those of the publisher, the editors and the reviewers. Any product that may be evaluated in this article, or claim that may be made by its manufacturer, is not guaranteed or endorsed by the publisher.
The Supplementary Material for this article can be found online at: https://www.frontiersin.org/articles/10.3389/fpls.2024.1413507/full#supplementary-material
Armstrong, R. D, McCosker, K., Walsh, K., Millar, G. D., Johnson, S. B., Probert, M. E. (1997). Improved nitrogen supply to cereals in Central Queensland following short legume leys. Aust. J. Exp. Agr 37, 359–369. doi: 10.1071/ea96129
Barcelos, J. P. Q., Souza, M., Nascimento, C. A. C., Rosolem, C. A. (2022). Soil acidity amelioration improves N and C cycles in the short term in a system with soybean followed by maize-Guinea grass intercropping. Geoderma 421, 115909. doi: 10.1016/j.geoderma.2022.115909
Bender, S. F., Wagg, C., van der Heijden, M. G. A. (2016). An underground revolution: biodiversity and soil ecological engineering for agricultural sustainability. Trends Ecol. Evol. 31, 440–452. doi: 10.1016/j.tree.2016.02.016
Castellazzi, M. S., Wood, G. A., Burgess, P. J., Morris, J., Conrad, K. F., Perry, J. N. (2008). A systematic representation of crop rotations. Agric. Syst. 97, 26–33. doi: 10.1016/j.agsy.2007.10.006
Cernay, C., Makowski, D., Pelzer, E. (2018). Preceding cultivation of grain legumes increases cereal yields under low nitrogen input conditions. Environ. Chem. Lett. 16, 631–636. doi: 10.1007/s10311-017-0698-z
Evans, J., Fettell, N. A., Coventry, D. R., Connor, G. E., Walsgott, D. N., Mahoney, J., et al. (1991). Wheat response after temperate crop legumes in south-eastern Australia. Aust. J. Agric. Res. 42, 31–43. doi: 10.1071/AR9910031
Fierer, N., Bradford, M. A., Jackson, R. B. (2007). Toward an ecological classification of soil bacteria. Ecology 88, 1354–1364. doi: 10.1890/05-1839
Franke, A. C., van den Brand, G. J., Vanlauwe, B., Giller, K. E. (2018). Sustainable intensification through rotations with grain legumes in Sub-Saharan Africa: a review. Agric. Ecosyst. Environ. 261, 172–185. doi: 10.1016/j.agee.2017.09.029
Gao, X. N., Han, X. R., Liu, N., Zuo, R. H., Wu, Z. C., Yang, J. F. (2009). Effects of long-term fertilization on organic nitrogen forms and their distribution in profile of a brown soil. Scientia Agricultura Sin. 42, 2820–2827. doi: CNKI:SUN:ZNYK.0.2009-08-028
Geng, S. N., Li, L. T., Miao, Y. H., Zhang, Y. J., Yu, X. N., Zhang, D., et al. (2023). Nitrogen rhizodeposition from corn and soybean, and its contribution to the subsequent wheat crops. J. Integr. Agr. 23, 2446–2457. doi: 10.1016/j.jia.2023.11.018
Gentry, L. F., Ruffo, M. L., Below, F. E. (2013). Identifying factors controlling the continuous corn yield penalty. Agron. J. 105, 295–303. doi: 10.2134/agronj2012.0246
German, R. N., Thompson, C. E., Benton, T. G. (2017). Relationships among multiple aspects of agriculture’s environmental impact and productivity: a meta-analysis to guide sustainable agriculture. Biol. Rev. 92, 716–738. doi: 10.1111/brv.12251
Guenet, B., Neill, C., Bardoux, G., Abbadie, L. (2010). Is there a linear relationship between priming effect intensity and the amount of organic matter input. Appl. Soil. Ecol. 46, 436–442. doi: 10.1016/j.apsoil.2010.09.006
Hao, X. Y., Ma, X. Z., Gao, Z. C., Chen, M. M., Zhou, B. K. (2015). Variation characteristics of fractions of active nitrogen and organic nitrogen under different long term fertilization practices in black soil. Scientia Agricultura Sin. 48, 4707–4716. doi: 10.3864/j.issn.0578-1752.2015.23.012
He, J. F., Hu, Y. F., Yang, Z. P., Duan, H., Ling, J., Xiao, H. H., et al. (2018). Effects of cultivation on soil organic nitrogen components in alpine-cold grassland in Northwest Sichuan. J. Soil Water Conserv. 32, 246–251. doi: 10.13870/j.cnki.stbcxb.2018.05.040
Hoagland, L., Carpenter-Boggs, L., Granatstein, D. (2008). Orchard floor management effects on nitrogen fertility and soil biological activity in a newly established organic apple orchard. Biol. Fertil. Soils 45, 11–18. doi: 10.1007/s00374-008-0304-4
Jantalia, C. P., Resck, D. V., Alves, B. J., Zotarelli, L., Urquiaga, S., Boddey, R. M. (2007). Tillage effect on C stocks of a clayey Oxisol under a soybean-based crop rotation in the Brazilian Cerrado region. Soil Till. Res. 95, 97–109. doi: 10.1016/j.still.2006.11.005
Ji, J. H., Liu, S. Q., Li, Y. Y., Hao, X. Y. (2020). Content and distribution of soil organic nitrogen in different types of farmland in Heilongjiang Province. Heilongjiang Agric. Sci. 9, 38–41. doi: 10.11942/j.issn1002-2767.2020.09.0038
Jiang, L. L., Han, L. S., Han, X. R., Zhan, X. M., Zuo, R. H., Wu, Z. C., et al. (2011). Effects of nitrogen on growth,root morphological traits,nitrogen uptake and utilization efficiency of maize seedlings. J. Plant Nutr. Fertilizer 17, 247–253. doi: 10.11674/zwyf.2011.0134
Jiao, Y. P., Qi, P., Wang, X. J., Wu, J., Yao, Y. M., Cai, L. Q., et al. (2020). Effects of different nitrogen application rates on soil organic nitrogen components and enzyme activities in farmland. Scientia Agricultura Sin. 53, 2423–2434. doi: 10.3864/j.issn.0578-1752.2020.12.010
Jing, Q., Van Keulen, H., Hengsdijk, H., Cao, W., Bindraban, P. S., Dai, T., et al. (2009). Quantifying N response and N use efficiency in rice-wheat (RW) cropping systems under different water management. J. Agric. Sci. 147, 303–312. doi: 10.1017/S0021859609008466
Ju, X. T., Xing, G. X., Chen, X. P., Zhang, S. L., Zhang, L. J., Liu, X. J., et al. (2009). Reducing environmental risk by improving N management in intensive Chinese agricultural systems. Proc. Natl. Acad. Sci. Unit. S. Am. 106, 3041–3046. doi: 10.1073/pnas.0813417106
Kirkby, C. A., Richardson, A. E., Wade, L. J., Passioura, J. B., Batten, G. D., Chris, B. (2014). Nutrient availability limits carbon sequestration in arable soils. Soil Biol. Biochem. 68, 402–409. doi: 10.1016/j.soilbio.2013.09.032
Li, J., Han, G., Wang, G., Liu, X., Zhang, Q., Chen, Y., et al. (2022). Imbalanced nitrogen–phosphorus input alters soil organic carbon storage and mineralisation in a salt marsh. Catena 208, 105720. doi: 10.1016/j.catena.2021.105720
Li, S., Liu, J. J., Yao, Q., Yu, Z. H., Li, Y. S., Jin, J., et al. (2021). Short-term lime application impacts microbial community composition and potential function in an acid black soil. Plant Soil 470, 1–16. doi: 10.1007/s11104-021-04913-0
Li, S. X., Wang, Z. H., Stewart, B. A. (2013). Responses of crop plants to ammonium and nitrate N. Adv. Agron. 118, 205–397. doi: 10.1016/B978-0-12-405942-9.00005-0
Li, Z. Q., Li, D. D., Ma, L., Yu, Y. Y., Zhao, B. Z., Zhang, J. B. (2019). Effects of straw management and nitrogen application rate on soil organic matter fractions and microbial properties in North China Plain. J. Soil Sediment. 19, 618–628. doi: 10.1007/s11368-018-2102-4
Lian, T. X., Yu, Z. H., Li, Y. S., Jin, J., Wang, G. H., Liu, X. B., et al. (2019). The shift of bacterial community composition magnifies over time in response to different sources of soybean residues. Appl. Soil Ecol. 136, 163–167. doi: 10.1016/j.apsoil.2019.01.001
Lin, J. S., Roswanjaya, Y. P., Kohlen, W., Stougaard, J., Reid, D. (2021). Nitrate restricts nodule organogenesis through inhibition of cytokinin biosynthesis in Lotus japonicus. Nat. Commun. 12, 6544. doi: 10.1038/s41467-021-26820-9
Ma, F. X., Wang, Y. Y., Yan, P., Wei, F., Sun, X. Z., Liu, J. G., et al. (2018). Effects of cotton straw incorporation on organic nitrogen fractions in long-term continuous cropping cotton field. Ecol. Environ. Sci. 27, 1459–1465. doi: 10.16258/j.cnki.1674-5906.2018.08.011
Ma, X., Xie, Z. Y., Luo, Z. Z., Li, L. L., Niu, Y. N., Cai, L. Q., et al. (2020). Characterization of soil organic nitrogen component in different succeeding crops of lucerne on the Loess Plateau of central Gansu. Soil Fertilizer Sci. China 2, 17–23. doi: 10.11838/sfsc.1673-6257.19154
Mahmoud, R., Casadebaig, P., Hilgert, N., Alletto, L., Freschet, G. T., de Mazancourt, C., et al. (2022). Species choice and N fertilization influence yield gains through complementarity and selection effects in cereal-legume intercrops. Agron. Sustain. Dev. 42, 12. doi: 10.1007/s13593-022-00754-y
McDaniel, M. D., Grandy, A. S. (2016). Soil microbial biomass and function are altered by 12 years of crop rotation. Soil 2, 583–599. doi: 10.5194/soil-2016-39
Miyazawa, K., Murakami, T., Takeda, M., Murayama, T. (2010). Intercropping green manure crops: Effects on rooting patterns. Plant Soil 331, 231–239. doi: 10.1007/s11104-009-0248-y
Ohwaki, Y., Sugahara, K. (1997). Active extrusion of protons and exudation of carboxylic acids in response to iron deficiency by roots of chickpea (Cicer arietinum L.). Plant Soil. 189, 49–55. doi: 10.1023/A:1004271108351
Peoples, M. B., Brockwell, J., Herridge, D. F., Rochester, I. J., Alves, B. J. R., Urquiaga, S., et al. (2009). The contributions of nitrogen-fixing crop legumes to the productivity of agricultural systems. Symbiosis 48, 1–17. doi: 10.1007/BF03179980
Preissel, S., Reckling, M., Schlaefke, N., Zander, P. (2015). Magnitude and farm-economic value of grain legume pre-crop benefits in Europe: a review. Field Crop Res. 175, 64–79. doi: 10.1016/j.fcr.2015.01.012
Rocha, K. F., Mariano, E., Grassmann, C. S., Trivelin, P. C. O., Rosolem, C. A. (2019). Fate of 15N fertilizer applied to maize in rotation with tropical forage grasses. Field Crops Res. 238, 35–44. doi: 10.1016/j.fcr.2019.04.018
Ruisi, P., Saia, S., Badagliacca, G., Amato, G., Frenda, A. S., Giambalvo, D., et al. (2016). Long-term effects of no tillage treatment on soil N availability, N uptake, and 15N-fertilizer recovery of durum wheat differ in relation to crop sequence. Field Crops Res. 189, 51–58. doi: 10.1016/j.fcr.2016.02.009
Salvagiotti, F., Cassman, K. G., Specht, J. E., Walters, D. T., Weiss, A., Dobermann, A. (2008). Nitrogen uptake, fixation and response to fertilizer N in soybeans: A review. Field Crops Res. 108, 1–13. doi: 10.1016/j.fcr.2008.03.001
Sebastian, K., Monika, S., Przemysław, T., Wojciech, L., Jacek, M. (2023). Soil organic carbon and mineral nitrogen contents in soils as affected by their pH, texture and fertilization. Agron. 13, 267–267. doi: 10.3390/agronomy13010267
Shah, K. K., Modi, B., Pandey, H. P., Subedi, A., Aryal, G., Pandey, M., et al. (2021). Diversified crop rotation: an approach for sustainable agriculture production. Adv. Agricul. 2, 1–9. doi: 10.1155/2021/8924087
Shen, Y., Sui, P., Huang, J., Wang, D., Whalen, J. K., Chen, Y. (2018). Greenhouse gas emissions from soil under maize–soybean intercrop in the North China Plain. Nutr. Cycl. Agroecosyst. 110, 451–465. doi: 10.1007/s10705-018-9908-8
Tang, X., Bernard, L., Brauman, A., Daufresne, T., Deleporte, P., Desclaux, D., et al. (2014). lncrease in microial biomass and phosphorus availability in the rhizosphere of intercropped cereal and legumes under field conditions. Soil Biol. Biochem. 75, 86–93. doi: 10.1016/j.soilbio.2014.04.001
Tang, Y. M., Ma, W. W., Li, G., Zhang, Y., Pan, P. X., Song, L. C., et al. (2021). Variations of soil organic nitrogen fractions during degradation succession in the Gahai wetland, northwest China. Chin. J. Appl. Ecol. 32, 4077–4084. doi: 10.13287/j.1001-9332.202111.035
Thorup-Kristensen, K., Magid, J., Jensen, L. S. (2003). Catch crops and green manures asbiological tools in nitrogen management in temperate zones. Adv. Agron. 79, 227–302. doi: 10.1016/S0065-2113(02)79005-6
Tian, Z. W., Liu, X. X., Gu, S. L., Yu, J. H., Zhang, L., Zhang, W. W., et al. (2018). Postponed and reduced basal nitrogen application improves nitrogen use efficiency and plant growth of winter wheat. J. Integr. Agr. 17, 2648–2661. doi: 10.1016/S2095-3119(18)62086-6
Tilman, D., Cassman, K. G., Matson, P. A., Naylor, R., Polasky, S. (2002). Agricultural sustainability and intensive production practices. Nature 418, 671–677. doi: 10.1038/nature01014
Vance, E. D., Brookes, P. C., Jenkinson, D. S. (1987). An extraction method for measuring soil microbial biomass C. Soil Biol. Biochem 19, 703–707. doi: 10.1016/0038-0717(87)90052-6
Wallander, S. (2013). While crop rotations are common, cover crops remain rare. Amber Waves 21, 1–1. doi: 10.22004/ag.econ.229284
Wei, Y. W., Wu, D., Wei, D., Zhao, Y., Wu, J. Q., Xie, X. Y., et al. (2019). Improved lignocellulose-degrading performance during straw composting from diverse sources with actinomycetes inoculation by regulating the key enzyme activities. Bioresource Technology: Biomass Bioenergy Biowastes Conversion Technologies Biotransformations Production Technol. 271, 66–67. doi: 10.1016/j.biortech.2018.09.081
Wu, G. H., Chen, Z. H., Jing, N., Jiang, H., Chen, L. J. (2021). Effects of long-term no-tillage with different residue application rates on soil nitrogen cycling. Soil Till. Res. 212. doi: 10.1016/j.still.2021.105044
Xu, Z., Li, C., Zhang, C., Yu, Y., van der Werf, W., Zhang, F. (2020). Intercropping maizeand soybean increases efficiency of land and fertilizer nitrogen use; A meta-analysis. Field Crops Res. 246, 107661. doi: 10.1016/j.fcr.2019.107661
Yang, M. (2023). Effects of no-tillage mulching on microbial biomass carbon and nitrogen contents and related enzyme activities in different soil layers of a wheat-peanut rotation system. Jiangsu Agric. Sci. 51, 216–222. doi: 10.15889/j.issn.1002-1302.2023.06.030
Yang, H., Fang, C., Meng, Y., Dai, Y., Liu, J. (2021). Long-term ditch-buried straw return increases functionality of soil microbial communities. Catena 202, 105316. doi: 10.1016/j.catena.2021.105316
Yan, Z. J., Zhou, J., Liu, C. Y., Jia, R., Mganga, K. Z., Yang, L. (2023). Legume-based crop diversification reinforces soil health and carbon storage driven by microbial biomass and aggregates. Soil Till. Res. 234, 105848. doi: 10.1016/j.still.2023.105848
Yuan, M., Bi, Y. D., Han, D. W., Wang, L., Wang, L. X., Fan, C., et al. (2022). Long-term corn-soybean rotation and soil fertilization: impacts on yield and agronomic traits. Agron. 12, 2554–2554. doi: 10.3390/agronomy12102554
Zang, H. D., Yang, X. C., Feng, X. M., Qian, X., Hu, Y. G., Ren, C. Z., et al. (2015). Rhizodeposition of nitrogen and carbon by mungbean (Vigna radiata L.) and its contribution to intercropped oats (Avena nuda L.). PloS One 10, e0121132. doi: 10.1371/journal.pone.0121132
Zhang, G. N., Chen, L. J., Chen, Z. H., Zhang, Y. L. (2008). Effects of different cropping systems of soybean on chernozem enzyme activities and kinetic parameters. Soybean Sci. 05, 795–800.
Zhang, Y. Q., Kou, C. L., Ma, Z. H., Guo, Z. L., Luo, X. S. (2015). Effects of long term combination application of organic manure and nitrogen fertilizer on organic carbon and organic nitrogen forms of Fluvo-aquic soil. Chin. J. Soil Sci. 46, 584–589. doi: 10.19336/j.cnki.trtb.2015.03.012
Keywords: rotation, straw return, corn yield, nitrogen fractions, 15N-labeled
Citation: Yan C, Yang Y, Song J, Shan F, Lyu X, Yan S, Wang C, Song Q and Ma C (2024) Analysis of the beneficial effects of prior soybean cultivation to the field on corn yield and soil nitrogen content. Front. Plant Sci. 15:1413507. doi: 10.3389/fpls.2024.1413507
Received: 07 April 2024; Accepted: 09 July 2024;
Published: 30 July 2024.
Edited by:
Fernando Carlos Gómez-Merino, Colegio de Postgraduados (COLPOS), MexicoReviewed by:
Juan Fernando Hirzel, Agricultural Research Institute, ChileCopyright © 2024 Yan, Yang, Song, Shan, Lyu, Yan, Wang, Song and Ma. This is an open-access article distributed under the terms of the Creative Commons Attribution License (CC BY). The use, distribution or reproduction in other forums is permitted, provided the original author(s) and the copyright owner(s) are credited and that the original publication in this journal is cited, in accordance with accepted academic practice. No use, distribution or reproduction is permitted which does not comply with these terms.
*Correspondence: Chunmei Ma, Y2h1bm1tQG5lYXUuZWR1LmNu; Qiulai Song, c3FsMTQyOTEzQDE2My5jb20=
Disclaimer: All claims expressed in this article are solely those of the authors and do not necessarily represent those of their affiliated organizations, or those of the publisher, the editors and the reviewers. Any product that may be evaluated in this article or claim that may be made by its manufacturer is not guaranteed or endorsed by the publisher.
Research integrity at Frontiers
Learn more about the work of our research integrity team to safeguard the quality of each article we publish.