- 1Institute of Plant Protection, Beijing Academy of Agriculture and Forestry Sciences, Beijing, China
- 2Beijing Key Laboratory of Environment Friendly Management on Fruit Diseases and Pests in North China, Beijing Academy of Agriculture and Forestry Sciences, Beijing, China
- 3College of Life Sciences, Yangtze University, Jingzhou, Hubei, China
- 4School of Environmental Sciences, University of Guelph, Guelph, ON, Canada
As an evergreen shrub, Euonymus japonicus plays a crucial role in urban landscape construction, and its growth is affected by severe foliar anthracnose caused by Colletotrichum spp. However, the biodiversity of Colletotrichum species associated with anthracnose on E. japonicus remains undetermined. This study involved a two-year collection of E. japonicus leaf samples with typical anthracnose symptoms from 9 districts in Beijing, China. A total of 194 Colletotrichum isolates were obtained, and eight Colletotrichum species were subsequently identified using morphological characteristics and molecular identification with the ACT, GADPH, CHS, TUB2, and CAL genes, as well as the rDNA-ITS region. These species included Colletotrichum aenigma, C. fructicola, C. gloeosporioides, C. grossum, C. hebeiense, C. karstii, C. siamense, and C. theobromicola with C. siamense being the most prevalent (57%), followed by C. aenigma and C. theobromicola. Furthermore, C. fructicola, C. grossum and C. hebeiense are reported for the first time as causal agents of anthracnose on E. japonicus worldwide, and C. karstii is newly reported to be associated with E. japonicus anthracnose in China. Pathogenicity tests revealed that all tested isolates exhibited pathogenicity in the presence of wounds, emphasizing the need to avoid artificial or mechanical wounds to prevent infection in E. japonicus management. The EC50 values of five fungicides, namely difenoconazole, flusilazole, tebuconazole, hexaconazole, and prochloraz, were found to be less than 10 mg/L, indicating their strong potential for application. Notably, the EC50 of prochloraz was less than 0.05 mg/L for C. theobromicola. These findings offer valuable insights for the management of anthracnose on E. japonicus.
1 Introduction
Euonymus japonicus Thunb. plays an important role in urban greening because of its high aesthetic and economic value (Huang et al., 2016). The water retention capacity of E. japonicus leaves surpasses that of Ligustrum vicaryi among broad-leaved shrub species, thereby enhancing both the aesthetic and functional aspects of landscape through improved soil water storage (Yin et al., 2008). In Beijing, E. japonicus is extensively cultivated as a shrub due to its robust resistance to the dry and cold climatic conditions prevalent in the region, effectively reducing particulate matter during winter (Cai et al., 2022). However, E. japonicus is highly susceptible to fungal diseases such as powdery mildew and anthracnose (Yao et al., 2018; Lin et al., 2023). The infection of E. japonicus caused by Colletotrichum spp. leads to the formation of numerous necrotic spots on the leaves, resulting in leaf drying, shedding, and ultimately plant death, significantly impacting its greening effect and landscape aesthetics (Mahoney, 1979; Tan et al., 2023).
Colletotrichum spp. have been identified as one of the primary fungal pathogens responsible for anthracnose on a wide range of plants (Dean et al., 2012). The occurrence of anthracnose on Mangifera indica in China is caused by C. fructicola, C. gloeosporioides, C. karstii, and C. siamense, resulting in damage to leaves and fruits (Li et al., 2019). The occurrence of anthracnose on Capsicum spp., caused by C. viniferum, results in the formation of sunken, water-soaked necrotic lesions (Diao et al., 2017). However, the identification of Colletotrichum is complicated due to the variability in characteristics under different environmental conditions. The traditional identification of Colletotrichum species is based on morphological characteristics, including the size and shape of conidia, appressoria, setae, or sclerotia, the presence of sexual structures, as well as cultural attributes such as growth rate and colony color (Hyde et al., 2009). The morphological distinction of Colletotrichum species poses a challenge due to their phenotypic similarity and the potential influence of environmental factors on morphological traits. Therefore, a comprehensive integration of available morphological characteristics is commonly employed for systematic discrimination among species (Cai et al., 2009). Currently, a total of 16 Colletotrichum species complexes and 15 singleton species have been identified (Sun et al., 2019; Liu et al., 2022). The internal transcribed spacer region (ITS) of ribosomal DNA has been wildly employed for distinguishing Colletotrichum species (Freeman et al., 2000). However, relying solely on ITS sequence information is insufficient for resolving species boundaries within the genus (Cannon et al., 2012). Therefore, additional genes such as ACT, CHS-1, GAPDH, and TUB2 have been employed to elucidate phylogenetic relationships among diverse Colletotrichum species (Weir et al., 2012). The integration of molecular sequencing information and morphological data is currently recommended as a comprehensive approach for precise species identification in Colletotrichum spp (Cannon et al., 2012; Weir et al., 2012).
In recent years, ten Colletotrichum species have been reported to be associated with anthracnose on E. japonicus, including C. aenigma (Tan et al., 2023), C. boninense (Lee et al., 2005), C. euonymi, C. euonymicola (Lin et al., 2023), C. gigasporum (Alizadeh et al., 2015), C. gloeosporioides (Huang et al., 2016), C. karstii (Alizadeh et al., 2015), C. siamense (Wu, 2019), C. theobromicola (Qin et al., 2022) and C. trichellum (Wu, 1992) in various geographical locations. Anthracnose on E. japonicus may be caused by a variety of Colletotrichum species, but the specific dominant pathogen and its virulence are still unknown. Recent studies have demonstrated that chemical fungicides (i.e. mancozeb, thiram, ziram, captan, and chlorothalonil) effectively inhibit Colletotrichum spp., the causal agents of anthracnose in strawberries (Dowling et al., 2020). However, few data are available about the activity of fungicides in controlling anthracnose of E. japonicus, and research is necessary to find the most effective one.
In this study, to elucidate the Colletotrichum spp. responsible for anthracnose of E. japonicus in a single region, Colletotrichum strains were isolated from numerous anthracnose samples of E. japonicus in Beijing, China, and identified through morphological analysis, multilocus phylogenetic analysis, and pathogenicity. Through assessing the sensitivity of Colletotrichum spp. to multiple fungicides, our objective is to screen and identify efficacious fungicides for anthracnose control in E. japonicus. This study will provide a foundation for the development of effective management strategies against this disease.
2 Materials and methods
2.1 Sampling and fungal isolation
E. japonicus leaves with typical anthracnose symptoms were collected from 2020 to 2022 in nine districts of Beijing, China. The tissues were cut into 5 × 5 mm pieces, soaked in 75% ethanol for 10 s and 5% hypochlorite for 1 min, and then washed three times with distilled water for 3 min. The samples were placed on potato dextrose agar (PDA) and incubated at 25°C under a 12 h light/dark cycle. After colonies grew out, the single-spore cultures were obtained and placed on fresh PDA for subsequent analysis. The obtained isolates were deposited in the culture collection of the Institute of Plant Protection, Beijing Academy of Agriculture and Forestry Sciences, China.
2.2 DNA extraction, PCR amplification and sequencing
To extract DNA, all the isolates were incubated at 25°C for 6 days on PDA, after which mycelia were collected in 1.5 mL centrifuge tubes. Genomic DNA was extracted using the Solarbio® Fungi Genomic DNA Extraction Kit (Solarbio, China) according to the manufacturer’s instructions. DNA was subsequently detected by electrophoresis with SYBR® Safe DNA gel stain and the qualified DNA were used as templates for PCR amplification. The following primer pairs were used for amplification ITS4/ITS5 (White et al., 1990) for rDNA-ITS; GDF1/GDR1 (Cannon et al., 2012) for glyceraldehyde-3-phospate dehydrogenase (GADPH); ACT-512F/ACT-783R (Carbone and Kohn, 1999) for partial actin (ACT); T1/Bt2b (Glass and Donaldson, 1995) for β-tubulin (TUB2); CHS-79F/CHS-345R (Carbone and Kohn, 1999) for partial chitin synthase (CHS); and CL1C/CL2C (Weir et al., 2012) for calmodulin (CAL).
The PCR cycles were performed in a C1000 thermal cycler (Bio-Rad) with a total volume of 25 μL. Each PCR system contained 10 μL of 2 × Taq PCR MasterMix, 1 μL of genomic DNA (50 ng/μL), 1 μL each of forward and reverse primers (10 mM) and 7 μL of double-deionized water. The PCR conditions for ITS were as follows: 5 min at 95°C; 35 cycles of 95°C for 30 s, 55°C for 30 s, and 72°C for 45 s; and 10 min at 72°C. The annealing temperatures for the target genes were as follows: ACT and TUB2, 58°C; CHS-1, 56°C; CAL, 55°C; and GAPDH, 61°C. The remaining PCR conditions for the target genes were the same as those for the ITS region (Li et al., 2023). The genes were sequenced at SinogenoMax Company, Beijing, and aligned with sequences available in the National Center for Biotechnology Information (NCBI) database. Sequences from type or ex-type isolates were selected for further analysis.
2.3 Phylogenetic analysis of Colletotrichum spp.
The sequences of the ACT, GADPH, CHS, TUB2, and CAL genes along with the ITS region were combined for the analyses to determine the taxonomic positions of Colletotrichum spp. associated with E. japonicus. The isolate numbers and the corresponding GenBank accession numbers used for this study were listed in Supplementary Tables S1 and S2. Sequences were assembled and aligned with BioEdit 7.0.5.3 (Hall, 1999). NEXUS files were generated with Clustal X 1.81 (Thompson et al., 1997). Maximum parsimony (MP) analysis was performed using PAUP 4.0b10. The analysis involved running 1000 replicates of a heuristic search, which utilized random sequence addition for initial tree construction followed by tree bisection reconnection branch swapping (Swofford, 2002). All the data were treated as unordered or unweighted, and gaps were treated as missing data. The topological confidence of trees was assessed by the maximum parsimony bootstrap proportion (MPBP) with 1000 replications, each consisting of 10 replicates of randomly adding taxa. The consistency index (CI), homoplasy index (HI), rescaled consistency index (RC), retention index (RI), and tree length (TL) were computed for trees generated using different optimality criteria. The robustness of the most parsimonious trees was assessed by 1,000 bootstrap replications resulting from MP analysis (Hillis and Bull, 1993). The resulting trees were subjected to Kishino-Hasegawa tests to assess their statistical significance (Kishino and Hasegawa, 1989).
Bayesian inference analysis was conducted using MrBayes 3.1.2 (Ronquist and Huelsenbeck, 2003) and a Markov chain Monte Carlo algorithm. The optimal models for nucleotide substitution were determined via statistical selection using MrModeltest v. 2.3 (Nylander, 2004). Simultaneous Markov chains were run for a total of 1,000,000 generations, with each chain initiated from random trees and sampled every 100 generations. After excluding the initial burn-in phase of the analysis consisting of 2,500 trees, the remaining trees were used to compute the Bayesian inference posterior probability. The phylogenetic trees were visualized via TreeviewX v. 0.5.0. The alignments and trees were deposited in TreeBASE.
2.4 Morphological identification
Representative isolates of Colletotrichum species were selected for morphological identification. Before morphological examination, single-spore isolates were cultured on PDA for 5 days at 25°C. Mycelial discs (6 mm diameter) were cut from colony margins and transferred onto 9-cm-diam Petri dishes, with four plates per isolate. The colony characteristics (color of the upper and lower surfaces) and growth rate (diameters in two perpendicular directions) were recorded after 6 days. The hyphal growth rate (cm/day) was calculated based on the colony diameter. The conidial production, shape and size were examined up to 10 days on PDA incubated at 25°C. The length and width of conidia (60 per isolate) from three-week-old PDA cultures were measured. Conidial appressoria were induced by dropping a conidial suspension (106 conidia/mL; 50 μL) on a concavity slide, which was then placed inside plates containing moistened filter papers, and incubated at 25°C in the dark (Cai et al., 2009). After 48 h, the morphology and dimensions of 50 appressoria were observed microscopically (NIS-Elements F3.0; Nikon) and measured by Image J 1.54d.
2.5 The prevalence of Colletotrichum species
After morphological and molecular identification, the prevalence of Colletotrichum species sampled in different districts in Beijing, China was calculated. The isolation rate (IR) was calculated for each species with the formula, IR % = (NS/NI) × 100, where NS is the number of isolates from the same species, and NI is the total number of isolates from each district (Vieira et al., 2014; Wang et al., 2016). The overall IR was calculated using the NI value equal to the total number of isolates obtained from E. japonicus leaves.
2.6 Pathogenicity tests of Colletotrichum spp.
Representative isolates displaying typical characteristics of Colletotrichum species were selected for pathogenicity tests by inoculating mycelial discs and conidial suspensions on healthy, detached leaves of E. japonicus. Each treatment included three replicates, with three leaves per replicate. The healthy apical leaves of E. japonicus were collected and washed three times with sterile water at the tip of the blade, followed by air-drying on sterilized filter paper. After sterilization, the leaves were punctured three times with a sterile insect pin (0.5 mm in diameter) for inoculation or left untreated (unwounded). To assess pathogenicity, a 6 mm diameter disc with either 20 μL of a 6-day-old culture or a conidial suspension at a concentration of 106 conidia/mL was inoculated onto the left side of each leaf. An equivalent volume of sterile water was used as a parallel control. Individual leaves were placed in plastic cases with distilled water to maintain > 90% relative humidity. They were then incubated at 25°C under a 12/12 h light/dark photoperiod. Daily observations were made to track symptom development. To assess the pathogenicity of various Colletotrichum spp., we measured the mean lesion length on E. japonicus leaves at 7 days post-inoculation. Infection rates were determined using the following formula: infection rate = (number of infected leaves/number of inoculated leaves) (Fu et al., 2019). The fungus was re-isolated from lesions and recognized by morphological characteristics and multi-gene phylogenetic analysis to fulfill Koch’s postulates.
2.7 Sensitivity assessments of the dominant Colletotrichum species to fungicides
Mycelial discs of the dominant Colletotrichum species (C. siamense, C. aenigma, and C. theobromicola) were inoculated onto PDA, both with and without fungicide amendments. Mycelial discs (6 mm diameter, from PDA culture grown at 25°C and incubated for up to 5 days) were placed onto PDA with or without (control) fungicide amendments. The final concentrations of eight fungicides (difenoconazole, flusilazole, tebuconazole, hexaconazole triadimefon, prochloraz, captan, and chlorothalonil) in the media are shown in Supplementary Table S3. Each treatment was tested four times, and the entire experiment was repeated twice. The mean colony diameter was measured at 25°C and incubated up to 5 days under a 12/12 h light/dark photoperiod. The formula for percent inhibition was [(radial growth of the control – radial growth at fungicide concentration)/radial growth of the control- diameter of the control] × 100% (Yan et al., 2014). The log10 probability conversion of the percentage of inhibition of fungicide concentrations was regressed to estimate half of the maximal effective concentration (EC50).
2.8 Statistical analysis
Data are expressed as mean ± standard deviation unless otherwise mentioned. Data were analyzed and imaged using GraphPad Prism 8.0 and OriginPro 2021 software. Differences between groups were analyzed with one-way ANOVA. The significant difference was defined at P < 0.05.
3 Results
3.1 Field survey, fungal isolation and prevalence of Colletotrichum species
A total of 308 isolates were obtained from 274 leaf samples collected from 9 districts spanning over 55 locations in Beijing, China, between 2020 and 2022. Through the integrated recognition of morphological characteristics and basic sequences blast and analysis, 194 Colletotrichum isolates were obtained. Combined with leaves and isolates characteristic identification, symptoms of anthracnose on E. japonicus leaves were classified into ten main types (Figure 1). Notably, some symptoms in the field may be attributable to the combination of two or three different Colletotrichum species (Supplementary Table S4), indicating the diversity of anthracnose pathogens of E. japonicus and the interactions of multiple Colletotrichum species in co-infections.
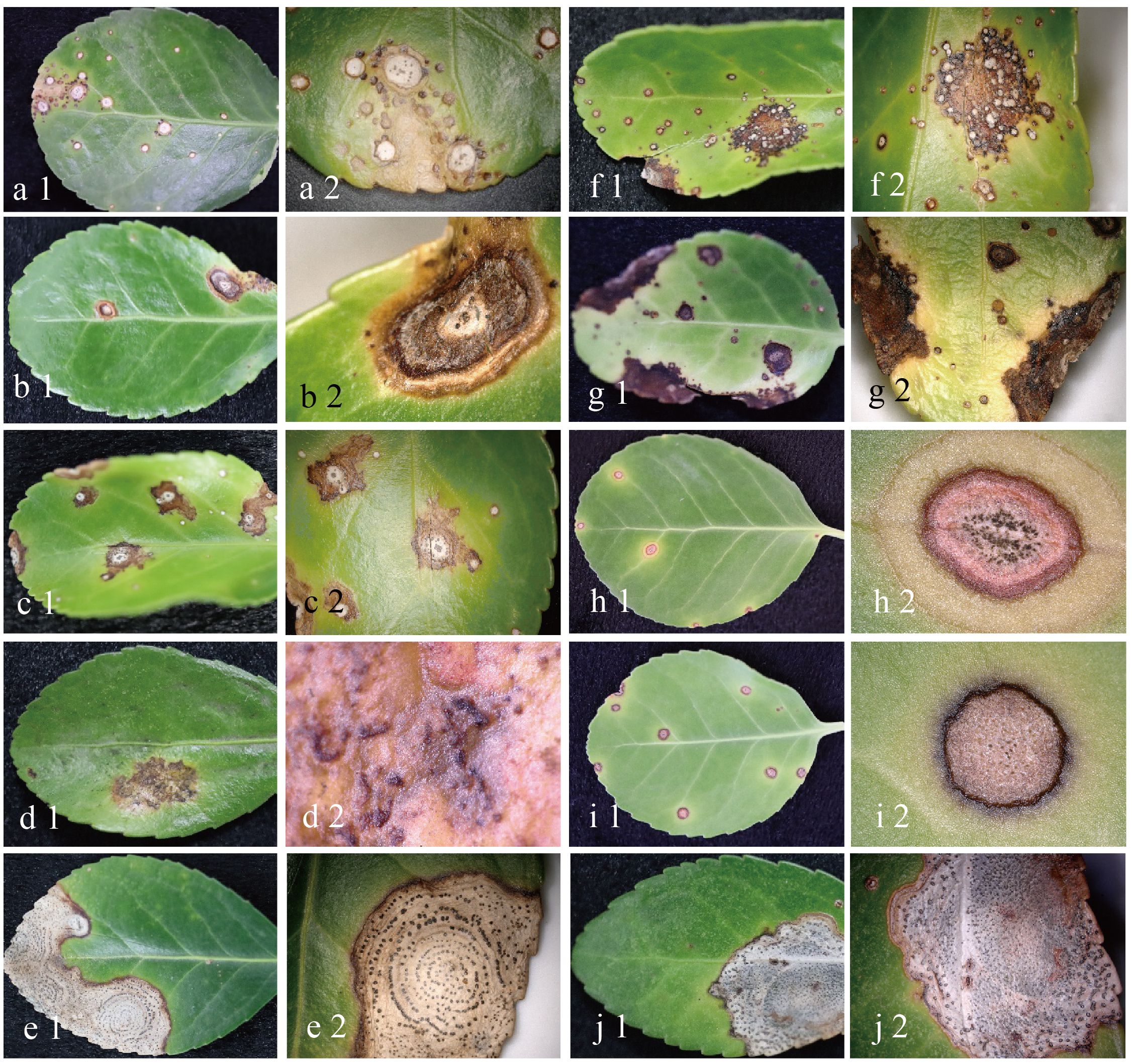
Figure 1 Typical symptoms of anthracnose on leaves of E. japonicus at early and late stage in the field. (A1–2) symptom I; (B1–2) symptom II; (C1–2) symptom III; (D1–2) symptoms IV; (E1–2) symptom V; (F1–2) symptom VI; (G1–2) symptom VII; (H1–2) symptoms VIII; (I1–2) symptom VIIII; (J1–2) symptom X.
The 194 isolates were identified as eight Colletotrichum species (Figure 2), including 110 isolates of C. siamense (56.7%), 32 isolates of C. aenigma (16.5%), 27 isolates of C. theobromicola (13.9%), 9 isolates of C. gloeosporioides (4.6%), 8 isolates of C. grossum (4.1%), 4 isolates of C. karstii (2.1%), 2 isolates C. fructicola (1.0%) and 2 isolates of C. hebeiense (1.0%). Analysis of the diversity and prevalence of Colletotrichum species revealed that C. siamense, with a prevalence of 56.7%, was the most widespread Colletotrichum spp. across eight districts, including Haidian, Chaoyang, Dongcheng, Xicheng, Fengtai, Pinggu, Huairou and Shijingshan districts (Figure 2). C. aenigma and C. theobromicola were less dominant than C. siamense, whereas C. gloeosporioides, C. grossum, C. karstii, C. fructicola, and C. hebeiense were found to be least prevalent. The diversity of Colletotrichum species associated with anthracnose of E. japonicus exhibited local variations within Beijing. For example, C. fructicola and C. hebeiense were exclusively isolated from diseased leaves of E. japonicus in Huairou district, while C. karsti was solely found from Pinggu district. Additionally, C. grossum was exclusively identified from Haidian and Chaoyang districts.
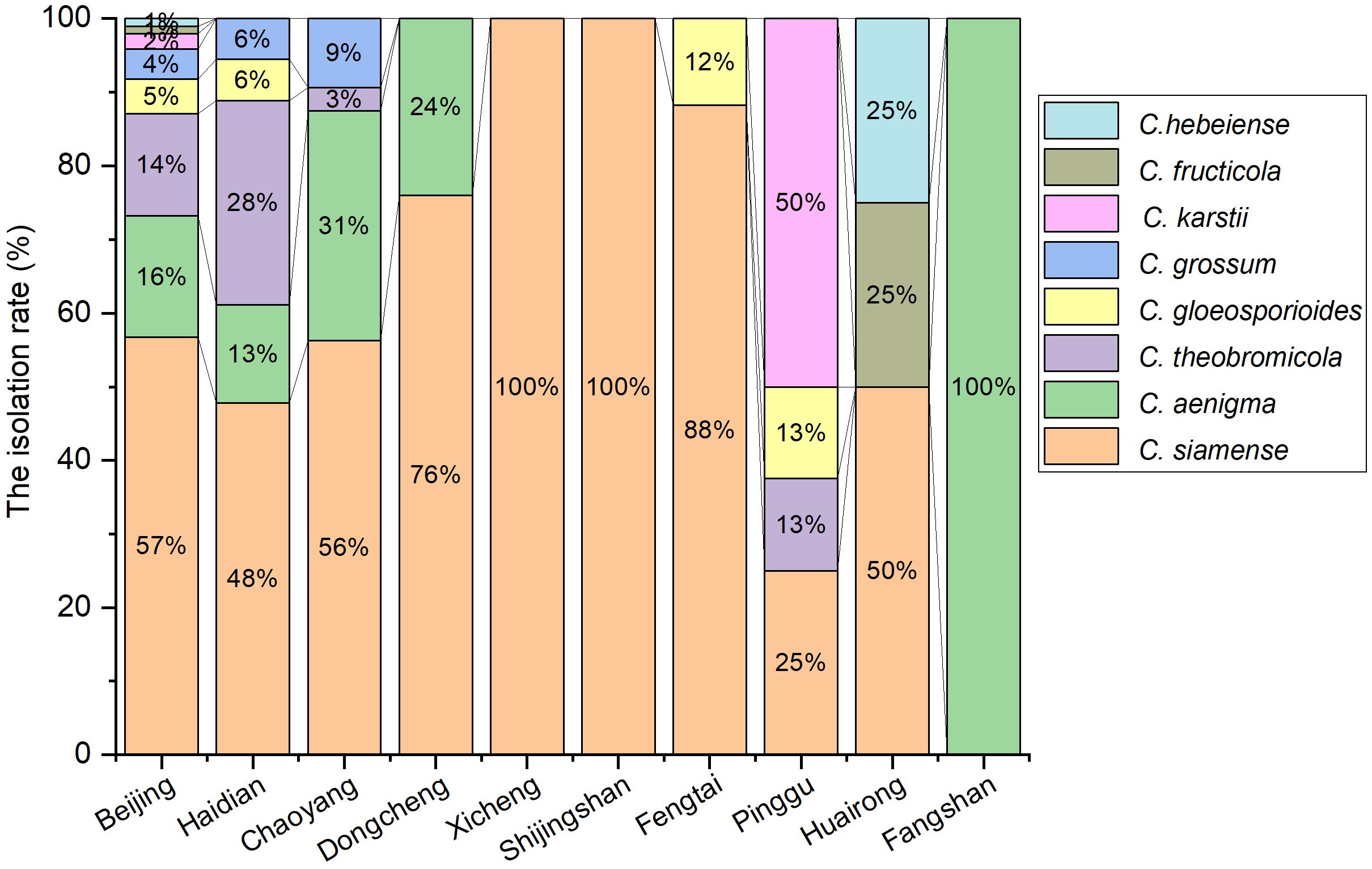
Figure 2 Isolation rate (%) of Colletotrichum spp. from the anthracnose leaves of E. japonicus in different districts of Beijing, China.
3.2 Phylogenetic analysis
Representative 53 isolates were chosen for phylogenetic analysis based on various sources and Colletotrichum species to determine the taxonomic status of Colletotrichum isolates. The isolates, along with 66 reference isolates, were selected for analysis based on their combined sequences of the ACT, GADPH, CHS, TUB2, and CAL genes and rDNA-ITS using maximum parsimony and Bayesian inference methods (Supplementary Table S1, S2). The isolates could be classified into two Colletotrichum species complexes, C. gloeosporioides species complex (49 isolates) and C. boninense species complex (4 isolates). The C. gloeosporioides species complex contained seven species, including C. aenigma, C. fructicola, C. gloeosporioides, C. grossum, C. hebeiense, C. siamense and C. theobromicola. A phylogenetic tree was constructed for C. gloeosporiodes complex species using C. boninense CBS 123755 as an outgroup (tree length = 1217, CI = 0.7527, HI = 0.2473, RC = 0.6859 and RI = 0.9113), which formed four major clades (Figure 3). The phylogenetic tree of the C. boninense species complex was constructed using C. gloeosporioides ICMP 17821 as an outgroup (tree length = 1182, CI = 0.7783, HI = 0.2217, RC = 0.6211, and RI = 0.7980), which also formed four clades (Figure 4). The above findings suggest that the majority of pathogenic fungi causing E. japonicus anthracnose belong to the C. gloeosporioides species complex and exhibit a close evolutionary relationship.
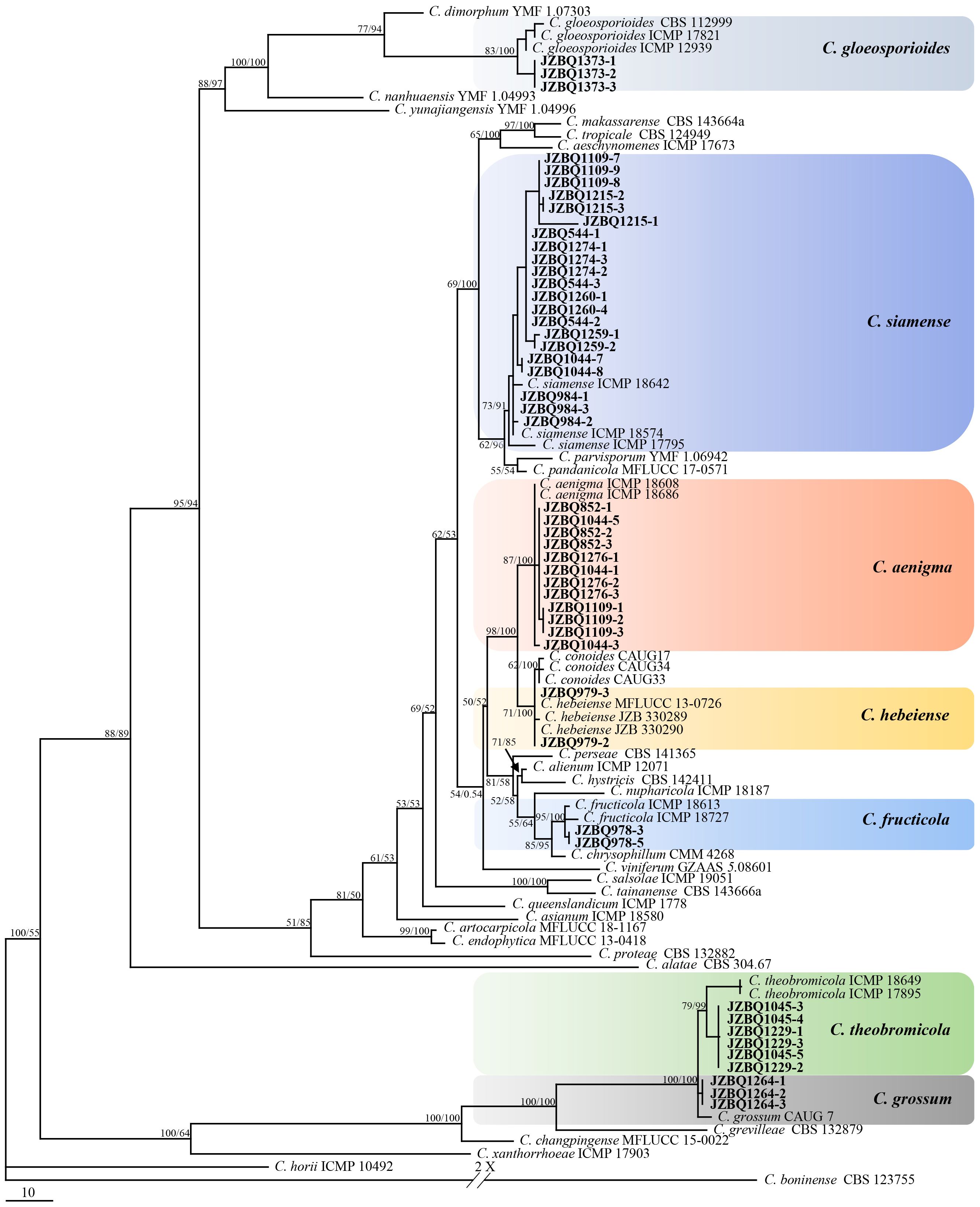
Figure 3 Maximum-parsimony (MP) phylogram reconstructed from the combined sequences of ITS, GAPDH, CAL, ACT, CHS-1 and TUB2. Colletotrichum boninense (CBS 123755) was selected as the outgroup. MP bootstrap support values (MP ≥ 50%) and bayesian posterior probability (PP ≥ 90%) were shown above the nodes (MP/PP). The scale bar indicates 10 expected changes per site. Isolates in this study were indicated in bold.
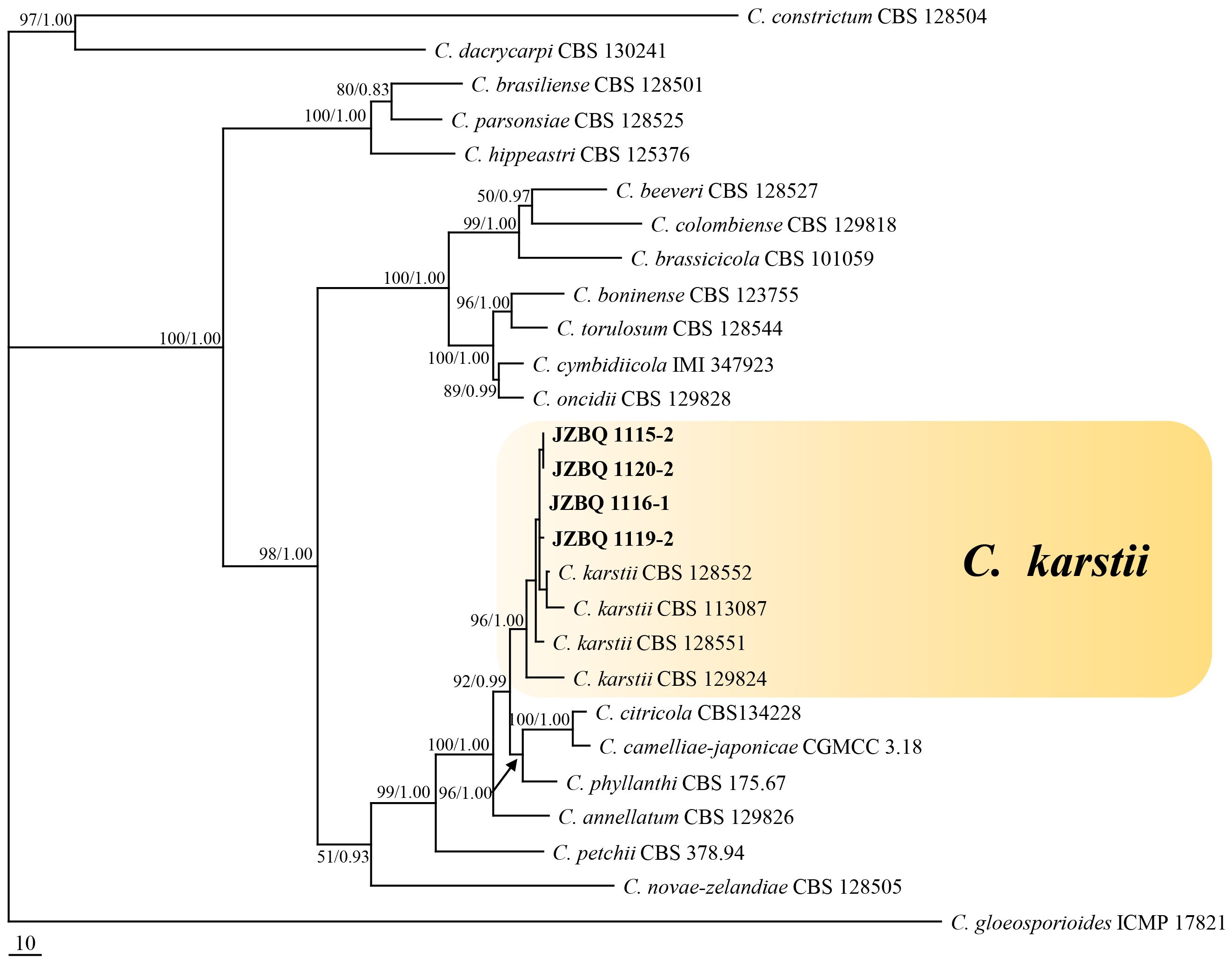
Figure 4 Maximum- parsimony (MP) phylogram reconstructed from the combined sequences of ITS, GAPDH, CAL, ACT, CHS-1 and TUB2. Colletotrichum gloeosporioides (IMI 356878) was selected as the outgroup. MP bootstrap support values (MP ≥ 50%) and bayesian posterior probability (PP ≥ 90%) were shown above the nodes (MP/PP). The scale bar indicates 10 expected changes per site. Isolates in this study were indicated in bold.
3.3 Morphological and culture characteristics
To further investigate the distinctive characteristics of the eight Colletotrichum species, representative isolates were selected from each species for morphological and biological observation (C. aenigma JZBQ1044–5, C. siamense JZBQ1044–7, C. theobromicola JZBQ1045–5, C. gloeosporioides JZBQ1373–3, C. grossum JZBQ1264–1, C. karstii JZBQ1115–2, C. fructicola JZBQ978–3 and C. hebeiense JZBQ979–2). As shown in Figure 5, seven isolates belonging to the C. gloeosporioides species complex formed grayish-white colonies on PDA plates with denser and cottony aerial mycelia. On the abaxial surface, all the isolates displayed white margins and gray-black centers, except for C. gloeosporioides JZBQ1373–3 which presented a light reddish hue at the center. Colletotrichum karstii JZBQ1115–2 exhibited sparse aerial hyphae at the margins and light orange with white flocculent hyphae attached to the center. On the back side, the plate was pale orange in the middle with white margins. The conidia of these isolates exhibited a hyaline, smooth, cylindrical morphology with obtuse rounded ends, or one end was obtusely rounded while the other was more pointed. The conidial widths of C. karstii JZBQ1115–2 were comparable to those of the isolates within the C. gloeosporioides species complex, while its lengths were significantly shorter (Table 1). The appressoria were subglobose or ellipsoid in shape, and possessed intact margins except for C. hebeiense JZBQ979–2 which displayed irregular morphology. The mycelial growth rates of Colletotrichum spp. exhibited significant variation, with C. aenigma JZBQ1044–5 and C. siamense JZBQ1044–7 displaying the highest average growth rates, followed by C. theobromicola JZBQ1045–5, C. grossum JZBQ1264–1 and C. fructicola JZBQ978–3. The above results indicate that these eight Colletotrichum species exhibited similar morphology of conidia and appressoria, while displaying variations in colony morphology and growth rate.
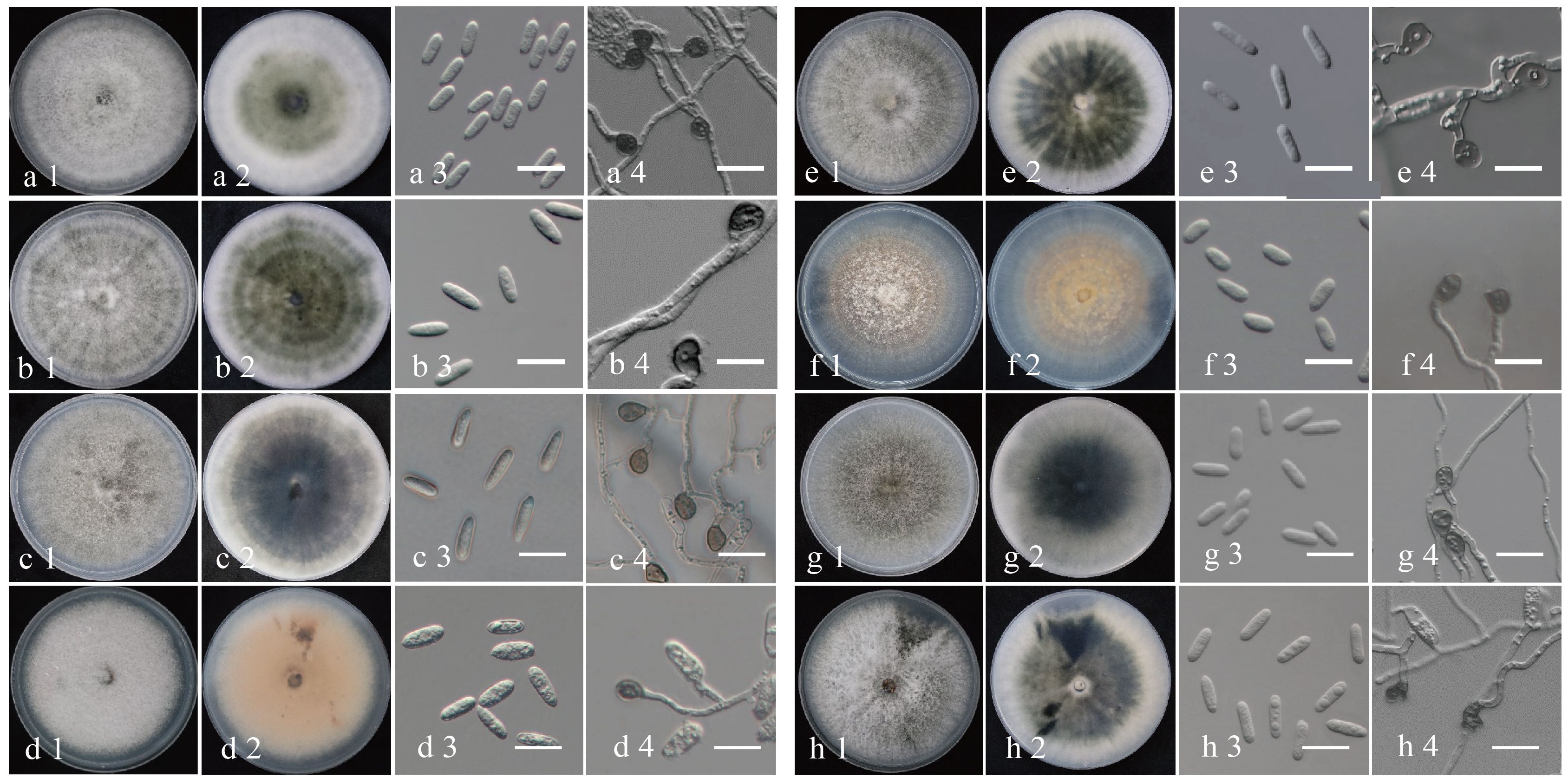
Figure 5 Morphological characteristics of eight Colletotrichum isolates from anthracnose leaves of E. japonicus in Beijing, China. The isolates were cultured on cover slips after 6 dpi at 25°C. (A) C. siamense JZBQ1044–7; (B) C. aenigma JZBQ1044–5; (C) C. theobromicola JZBQ1045–5; (D) C. gloeosporioides JZBQ1373–4; (E) C. grossum JZBQ1264–1; (F) C. karstii JZBQ1115–2; (G) C. fructicola JZBQ978–3; (H) C. hebeiense JZBQ979–2. 1–2: upper and reverse view of the colony; 3: conidia; 4: appressoria. Scale bars = 20 μm.
3.4 Pathogenicity of Colletotrichum spp. on leaves of E. japonicus
The pathogenicity of the above eight Colletotrichum species was assessed in accordance with Koch’s postulates. After seven days, the leaves inoculated with mycelial discs or conidial suspensions of the eight species under wounded conditions exhibited lesions similar to those observed in the field (Figure 6). The control leaves treated with sterile water remained devoid of symptoms. The isolates initiated the development of small brown or dark brown lesions at various time points after inoculation, with symptoms appearing as early as two days post-inoculation (dpi) for C. aenigma JZBQ1044–5, C. siamense JZBQ1044–7, C. theobromicola JZBQ1045–5, C. gloeosporioides JZBQ1373–3, and C. grossum JZBQ1264–1. These initial lesions gradually expanded into larger brown or dark brown lesions over time and eventually formed concentric rings of acervuli within 3~6 dpi. The pathogenicity of most Colletotrichum spp. toward the leaves of E. japonicus was found to be dependent on the presence of wounds, as evidenced by the infection rates caused by Colletotrichum spp. (Supplementary Table S5). Notably, only C. theobromicola JZBQ1045–5 and C. grossum JZBQ1229–2 were able to cause lesions on leaf tissues in the absence of wounds. Furthermore, the lesion sizes on the wounded leaves of E. japonicus at 7 dpi inoculated with mycelial discs of eight Colletotrichum spp. showed that C. theobromicola JZBQ1045–5 and C. grossum JZBQ1264–1 exhibited the highest virulence, while C. siamense JZBQ1044–7, C. aenigma JZBQ1044–5 and C. gloeosporioides JZBQ1373–3 demonstrated moderate levels of virulence; conversely, C. karstii JZBQ1115–2, C. fructicola JZBQ978–3, and C. hebeiense JZBQ979–2 were identified as the least virulent isolates (Figure 7). Eight Colletotrichum species were identified as potential causative pathogens for anthracnose on E. japonicus, with wounds observed to facilitate infection by most of these Colletotrichum spp.
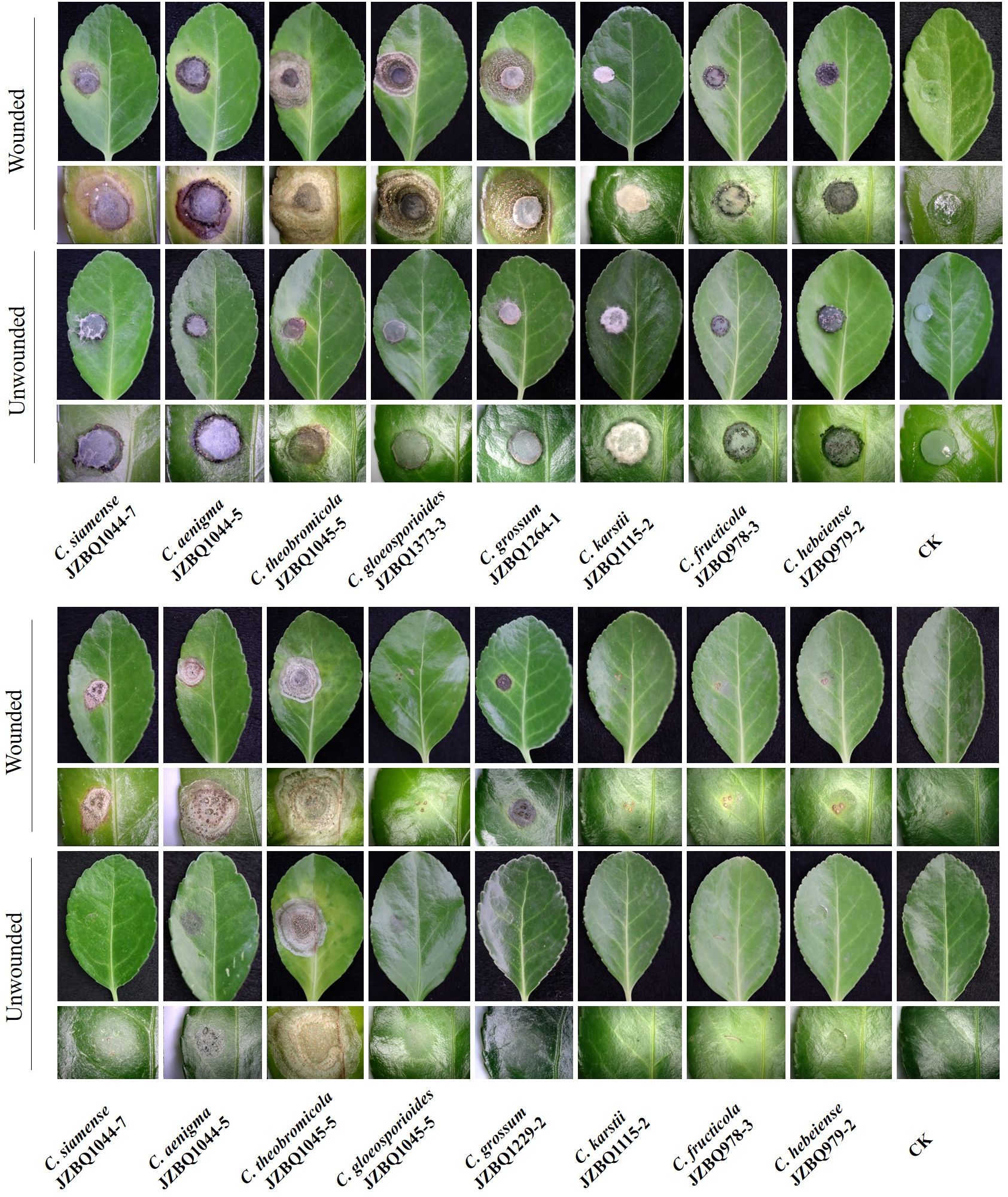
Figure 6 Symptoms on inoculated leaves of E. japonicus under wounded and unwounded conditions at 7dpi. The inocula were mycelial discs (A) or conidial suspensions (B) of eight Colletotrichum spp.
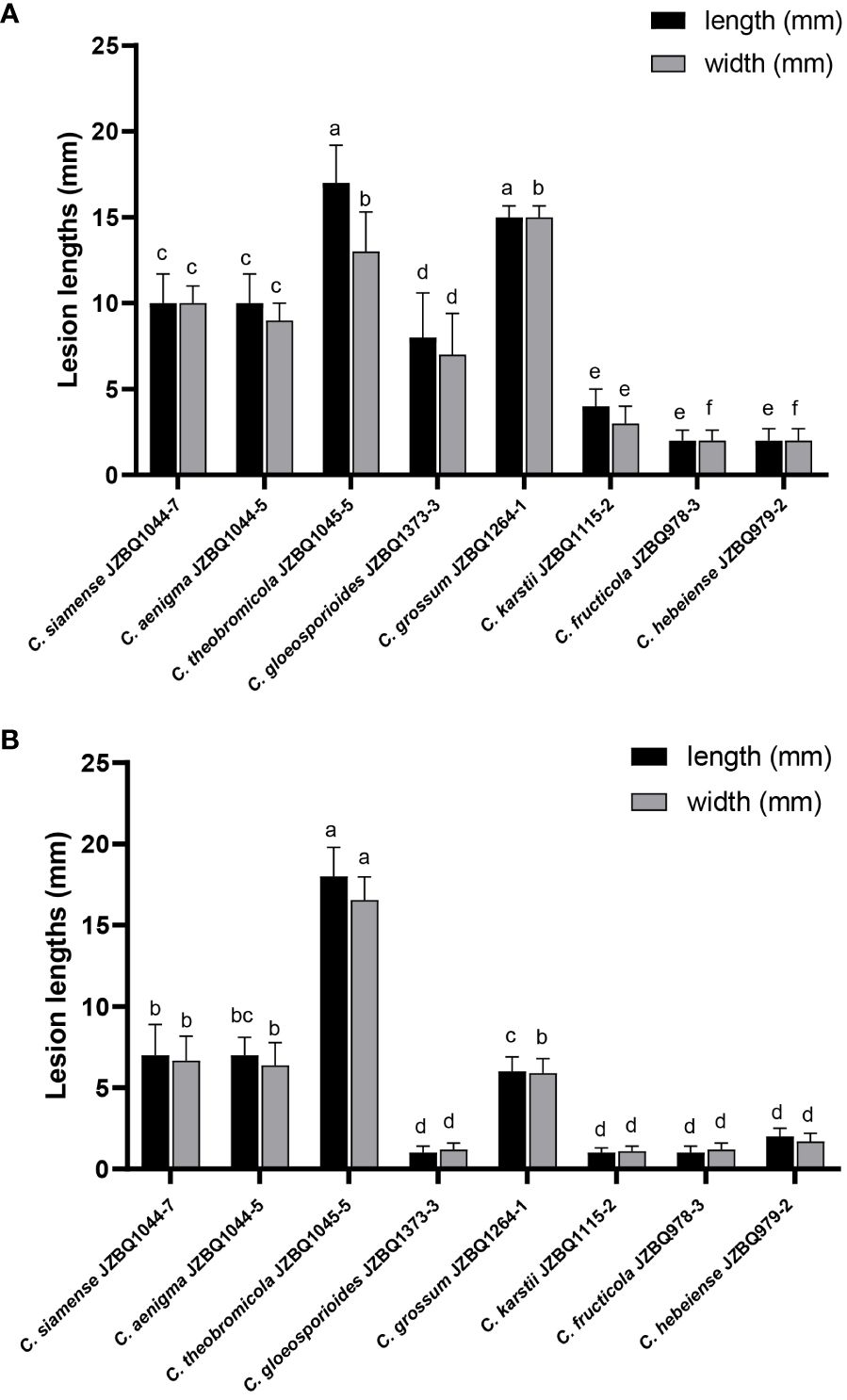
Figure 7 Lesion sizes on wounded leaves of E. japonicus at 7 dpi inoculated with mycelial discs (A) and conidial suspensions (B) of eight Colletotrichum species. Bars with different lowercase letters indicate significant differences (one-way ANOVA followed by Tukey’s multiple comparison test; p < 0.05).
3.5 Sensitivity of Colletotrichum isolates to fungicides
The EC50 values were determined by assessing the inhibitory activity of different concentrations of fungicides against Colletotrichum isolates. The virulence regression equations and associated data were placed in Supplementary Table S6. The results showed that eight fungicides exhibited significant antifungal activity against C. siamense, C. aenigma, and C. theobromicola (Table 2). The EC50 values of four triazole fungicides (difenoconazole, flusilazole, tebuconazole, and hexaconazole), as well as prochloraz, against Colletotrichum isolates were below 10 mg/L. Among them, prochloraz and difenoconazole were the most effective fungicides. The EC50 of prochloraz was less than 0.8 mg/L for the isolates of three species, and even 0.047 mg/L for C. theobromicola JZBQ1045–5. The second highly effective fungicide was difenoconazole, with an EC50 value as low as 0.2638 mg/mL for C. theobromicola JZBQ1388–10. To further observe the effect of fungicides, the colony and mycelial morphology of C. siamense JZBQ1044–7, C. aenigma JZBQ1044–5 and C. theobromicola JZBQ1045–5 treated with fungicides were observed and recorded. All the tested isolates displayed a high degree of sensitivity to various fungicides, and the inhibitory effects on mycelial growth varied significantly among different fungicides. Among them, prochloraz and difenoconazole at high concentrations caused hyphae contraction, increased apical branching, and twisting in C. siamense JZBQ1044–7 (Supplementary Figure S1). A similar situation was observed after difenoconazole treatment of C. aenigma JZBQ1044–5 and C. theobromicola JZBQ1045–5. In particular, prochloraz, at a concentration of 0.9 mg/mL, exhibited a significant increase in fungal branching and a decrease in the length of bifurcated hyphae.
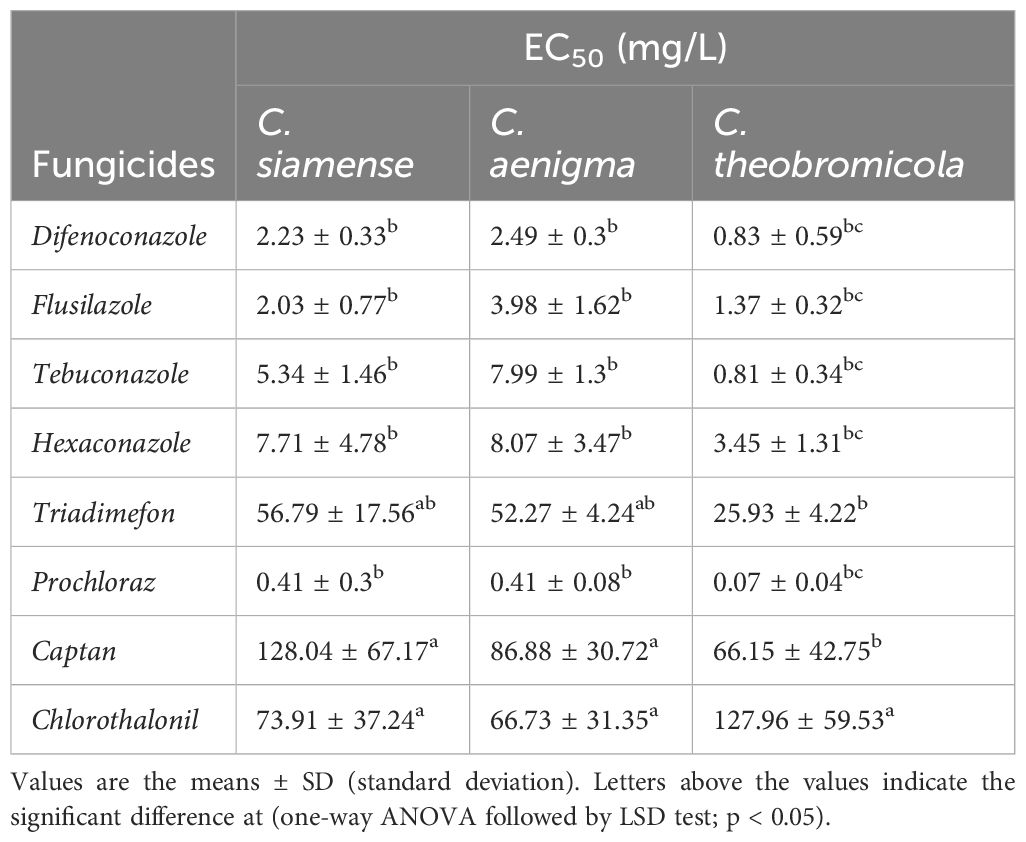
Table 2 The sensitivity of eight fungicides to the dominant pathogens C. siamense, C. theobromicola, and C. aenigma of E. japonicas.
4 Discussion
Colletotrichum spp. as a group are among the top ten fungal pathogens causing wilting and even death of plant parts, as well as post-harvest rotting of fruits worldwide (Dean et al., 2012). For the woody ornamental plant E. japonicus, currently, only a few Colletotrichum species causing anthracnose, such as C. siamense, C. theobromicola, and C. aenigma, have been reported (Qin et al., 2022; Tan et al., 2023). In this study, pathogenicity tests of eight species C. aenigma, C. siamense, C. theobromicola, C. gloeosporioides, C. grossum, C. fructicola, C. hebeiense and C. karstii revealed that all eight species significantly infected the leaves of E. japonicus. Notably, this study is the first to report that C. fructicola, C. grossum and C. hebeiense cause anthracnose in E. japonicus worldwide and that C. karstii in China causes anthracnose on E. japonicus. Moreover, most isolates belonged to the C. gloeosporioides species complex, and E. japonicus anthracnose in Beijing was primarily caused by seven species within this complex. This complex comprises 37 species and is associated with numerous plant anthracnose species (Oliveira et al., 2022). For example, various species within this complex, including C. changpingense, C. fructicola, C. siamense and C. theobromicola, were found to be responsible for strawberry anthracnose, whereas grape ripe rot in South and North America, Australasia, and Asia was associated with several species such as C. aenigma, C. fructicola, C. siamense, and C. hebeiense (Hsieh et al., 2023).
In this study, C. siamense, C. aenigma and C. theobromicola were the three dominant species causing anthracnose on E. japonicus in Beijing. Colletotrichum siamense, a significant plant pathogen previously documented to induce E. japonicus anthracnose in Jiangxi Province, China (Kuang et al., 2022), has been observed to infect numerous other plant species. For example, it can cause anthracnose of Pecan (Carya illinoinensis) and Allamanda cathartica in China (Huang et al., 2022; Zhuo et al., 2022). Furthermore, it is also the main pathogen causing strawberry crown rot, which has a high prevalence globally and is considered to be one of the most damaging species of the genus Colletotrichum (Ji et al., 2022). Similarly, C. siamense exhibited strong pathogenicity with a prevalence exceeding 50% in this study. Colletotrichum theobromicola has been reported to cause anthracnose of E. japonicus (Qin et al., 2022). In this study, pathogenicity test revealed that C. theobromicola JZBQ1045–5 exhibited the highest level of pathogenicity, causing significant infection on leaves even in the absence of wounds. This species is also known to induce dieback or anthracnose on a diverse array of plants, such as Buxus spp., a commonly used landscape shrub and Gossypium indicum (Singh and Doyle, 2017; Kang et al., 2022). The reasons for the high pathogenicity of C. theobromicola compared to other species remain to be investigated. Colletotrichum aenigma was less pathogenic and less prevalent than C. siamense and C. theobromicola, but it also exhibits broad host range and significant agricultural affect. For example, C. aenigma can cause a leaf spot disease on mulberry, affecting up to 40% of the leaves (Zhu et al., 2022). It can also cause grape anthracnose and apple leaf spot (Kim et al., 2021; Zhang et al., 2021). Therefore, the prevention and control of plant anthracnose caused by C. siamense, C. aenigma, and C. theobromicola are important in both agriculture and urban landscapes.
Most Colletotrichum spp. exhibit high pathogenicity toward wounded plants and cause low levels of infection toward unwounded plants. For example, C. siamense and C. fructicola exhibit increased pathogenicity toward various strawberry cultivars when fruits are damaged (Hu et al., 2022). Additionally, several Colletotrichum species are pathogenic to chili peppers following fruit surface injury, but most of them induce only minimal levels of infection in undamaged peppers (De Silva et al., 2019; Hu et al., 2022). The presence of wounds was conducive to the pathogenicity of most Colletotrichum spp. tested here to E. japonicus either by inoculation with mycelial discs or conidial suspensions. This situation may be explained by inefficient penetration of the pathogen into the cell wall of uninjured plants or by the cuticle acting as an anthracnose infection barrier to impede infection. As a landscape shrub, E. japonicus requires frequent pruning. As a result, damage to leaves and branches often occurs, thereby creating favorable conditions for infection by various Colletotrichum spp. Therefore, it is crucial to control anthracnose particularly after pruning of E. japonicus. As one of strongly pathogenic isolates of Colletotrichum spp., C. theobromicola exhibits pathogenicity with or without leaf injury supported by reports of C. siamense UOM 1137 being strongly pathogenic on both wounded and non-wounded chili peppers (De Silva et al., 2019). Hence, variations in pathogenicity might exist among diverse isolates of Colletotrichum spp., necessitating a focus on highly virulent strains.
The application of fungicides remains an important strategy for controlling plant anthracnose. Four triazole fungicides (difenoconazole, flusilazole, tebuconazole, and hexaconazole) had low EC50 values against C. siamense, C. aenigma, and C. theobromicola. All of these fungicides, belonging to the demethylation inhibitor (DMI) class, are widely used in agriculture for controlling fungal diseases due to their strong efficacy, and broad spectrum of activity. These fungicides could be used to control phytopathogenic fungi by inhibiting the biosynthesis of ergosterol in the cell membrane of fungi (Liu et al., 2021; Roman et al., 2021). The study revealed that difenoconazole, at a concentration of 0.0319 μg/mL, demonstrates significant inhibitory activity against various Colletotrichum species, including C. siamense, C. aenigma, and C. fructicola (Han et al., 2021). Flusilazole has been officially registered for controlling powdery mildew in cucumbers and anthracnose in apples in China (Wang et al., 2020). Tebuconazole exhibits potent inhibitory activity against Colletotrichum isolates on tea-oil trees in China, with EC50 values ranging from 0.1561 μg/mL to 1.8848 μg/mL (Zhu et al., 2023). Hexaconazole demonstrates effective control against anthracnose disease on pomegranate (Punica granatum L.) caused by C. gloeosporioides (Raj et al., 2024). These studies suggest the potential application of the four fungicides in managing anthracnose disease in E. japonicus. Among the eight fungicides, prochloraz and difenoconazole were the most effective against C. siamense, C. aenigma and C. theobromicola. They exhibit strong control effects against C. siamense and C. fructicola in the control of strawberry anthracnose (Zhang et al., 2020). Prochloraz is highly inhibitory to a large number of C. gloeosporioides species complex isolates (Mora-Aguilera et al., 2021). The imidazole fungicide prochloraz is extensively utilized in horticulture and agriculture worldwide, exerting its inhibitory effect on sterol biosynthesis in fungi (Vinggaard et al., 2006). Therefore, following treatment with prochloraz and difenoconazole, C. siamense, C. aenigma, and C. theobromicola caused twisted and deformed hyphae, as well as shortened bifurcated hyphae, possibly due to restricted cell membrane development. These two fungicides may have potential for the control of anthracnose on E. japonicus, but field testing is needed to confirm their effects. In addition, the combination of two multisite fungicides (captan and chlorothalonil) with other fungicides also have great potential applications. For instance, the combined use of captan and paclobutrazol for controlling C. gloeosporioides (Pérez, 2020). In vitro studies on C. nymphaeae and C. fioriniae have shown that combinations of two DMI fungicides can exert synergistic effects on Colletotrichum species, possibly due to differences in binding with the conserved CYP51 protein (Chen et al., 2020). Furthermore, the alternation and/or combination of single-site and multisite fungicides have been advocated to enhance control efficacy and further prevent the development of resistance in anthracnose isolates (Corkley et al., 2022).
5 Conclusions
In this study, a total of 194 Colletotrichum isolates were obtained and recognized as eight Colletotrichum species responsible for anthracnose of E. japonicus during a two-year investigation of 9 districts in Beijing, China. Among them, C. fructicola, C. grossum and C. hebeiense are reported for the first time to be associated with E. japonicus anthracnose worldwide, and C. karstii is firstly reported to be associated with E. japonicus anthracnose in China. Moreover, C. siamense, C. aenigma and C. theobromicola were dominant in the pathogens of anthracnose on E. japonicus in Beijing, China. And C. theobromicola showed the strongest pathogenicity in the tests. Additionally, the presence of wounds on E. japonicus was conducive to the pathogenicity of most Colletotrichum spp. whether through inoculation with mycelial discs or conidial suspensions. Therefore, it is crucial to minimize artificial or mechanical wounds to prevent pathogen infection in the production and management of E. japonicus. Among eight representative fungicides, four DMI fungicides and prochloraz showed more significant inhibitory effects on C. siamense, C. aenigma, and C. theobromicola, suggesting that they have potential applications toward anthracnose control. These results offer valuable insights for the prevention and treatment of anthracnose of E. japonicus.
Data availability statement
The datasets presented in this study can be found in online repositories. The names of the repository/repositories and accession number(s) can be found in the article/Supplementary Material.
Author contributions
YL: Data curation, Visualization, Writing – original draft, Writing – review & editing. XT: Data curation, Investigation, Methodology, Writing – original draft. JZ: Funding acquisition, Methodology, Writing – review & editing. YN: Data curation, Investigation, Writing – original draft. TH: Methodology, Writing – review & editing. ZY: Conceptualization, Methodology, Writing – review & editing. WQ: Conceptualization, Funding acquisition, Project administration, Writing – review & editing.
Funding
The author(s) declare financial support was received for the research, authorship, and/or publication of this article. This study was funded by the Youth Foundation of Beijing Academy of Agriculture and Forestry Sciences (QNJJ202313), the Innovation Foundation of Beijing Academy of Agriculture and Forestry Sciences (KJCX201910), and Reform and Development Project of Institute of Plant Protection, Beijing Academy of Agriculture and Forestry Sciences (ZBS202401).
Conflict of interest
The authors declare that the research was conducted in the absence of any commercial or financial relationships that could be construed as a potential conflict of interest.
Publisher’s note
All claims expressed in this article are solely those of the authors and do not necessarily represent those of their affiliated organizations, or those of the publisher, the editors and the reviewers. Any product that may be evaluated in this article, or claim that may be made by its manufacturer, is not guaranteed or endorsed by the publisher.
Supplementary material
The Supplementary Material for this article can be found online at: https://www.frontiersin.org/articles/10.3389/fpls.2024.1411625/full#supplementary-material
References
Alizadeh, A., Javan-Nikkhah, M., Zare, R., Fotouhifar, K., Stukenbrock, E. (2015). New records of Colletotrichum species for the mycobiota of Iran. Mycologia Iranica. 2, 95–109. doi: 10.22043/mi.2015.19967
Cai, H., Gu, X., Li, Y., Ren, Y., Yan, S., Yang, M. (2022). Cold resistance of Euonymus japonicus Beihaidao leaves and its chloroplast genome structure and comparison with Celastraceae species. Plants (Basel) 11, 2449. doi: 10.3390/plants11192449
Cai, L., Hyde, K., Taylor, P., Weir, B., Waller, J., Abang, M., et al. (2009). A polyphasic approach for studying Colletotrichum. Fungal Divers. 39, 183–204. doi: 10.1016/j.riam.2009.11.001
Cannon, P. F., Damm, U., Johnston, P. R., Weir, B. S. (2012). Colletotrichum – current status and future directions. Stud. Mycol 73, 181–213. doi: 10.3114/sim0014
Carbone, I., Kohn, L. M. (1999). A method for designing primer sets for speciation studies in filamentous ascomycetes. Mycologia 91, 553–556. doi: 10.1080/00275514.1999.12061051
Chen, S., Hu, M., Schnabel, G., Yang, D., Yan, X., Yuan, H. (2020). Paralogous CYP51 genes of Colletotrichum spp. mediate differential sensitivity to sterol demethylation inhibitors. Phytopathology. 110, 615–625. doi: 10.1094/PHYTO-10-19-0385-R
Corkley, I., Fraaije, B., Hawkins, N. (2022). Fungicide resistance management: Maximizing the effective life of plant protection products. Plant Pathol. 71, 150–169. doi: 10.1111/ppa.13467
Dean, R., Van Kan, J. A., Pretorius, Z. A., Hammond-Kosack, K. E., Di Pietro, A., Spanu, P. D., et al. (2012). The Top 10 fungal pathogens in molecular plant pathology. Mol. Plant Pathol. 13, 414–430. doi: 10.1111/j.1364-3703.2011.00783.x
De Silva, D. D., Groenewald, J. Z., Crous, P. W., Ades, P. K., Nasruddin, A., Mongkolporn, O., et al. (2019). Identification, prevalence and pathogenicity of Colletotrichum species causing anthracnose of Capsicum annuum in Asia. IMA Fungus 10, 8. doi: 10.1186/s43008-019-0001-y
Diao, Y. Z., Zhang, C., Liu, F., Wang, W. Z., Liu, L., Cai, L., et al. (2017). Colletotrichum species causing anthracnose disease of chili in China. Persoonia 38, 20–37. doi: 10.3767/003158517X692788
Dowling, M., Peres, N., Villani, S., Schnabel, G. (2020). Managing Colletotrichum on fruit crops: a “complex” challenge. Plant Dis. 104, 2301–2316. doi: 10.1094/PDIS-11-19-2378-FE
Freeman, S., Minz, D., Jurkevitch, E., Maymon, M., Shabi, E. (2000). Molecular analyses of Colletotrichum species from almond and other fruits. Phytopathology 90, 608–614. doi: 10.1094/PHYTO.2000.90.6.608
Fu, M., Crous, P. W., Bai, Q., Zhang, P. F., Xiang, J., Guo, Y. S., et al. (2019). Colletotrichum species associated with anthracnose of Pyrus spp. in China. Persoonia 42, 1–35. doi: 10.3767/persoonia.2019.42.01
Glass, N. L., Donaldson, G. C. (1995). Development of primer sets designed for use with the PCR to amplify conserved genes from filamentous ascomycetes. Appl. Environ. Microbiol. 61, 1323–1330. doi: 10.1128/aem.61.4.1323-1330.1995
Hall, T. A. (1999). BioEdit: a user-friendly biological sequence alignment editor and analysis. Nuclc Acids Symp 41, 95–98.
Han, Y. C., Zeng, X. G., Guo, C., Zhang, Q. H., Chen, F. Y., Ren, L., et al. (2021). Reproduction response of Colletotrichum fungi under the fungicide stress reveals new aspects of chemical control of fungal diseases. Microb. Biotechnol. 15, 431–441. doi: 10.1111/1751-7915.13754
Hillis, D. M., Bull, J. J. (1993). An empirical test of bootstrapping as a method for assessing confidence in phylogenetic analysis. Syst. Biol. 42, 182–192. doi: 10.1093/sysbio/42.2.182
Hsieh, T. F., Shen, Y. M., Huang, J. H., Tsai, J. N., Lu, M. T., Lin, C. P. (2023). Insights into Grape ripe rot: a focus on the Colletotrichum gloeosporioides species complex and its management strategies. Plants (Basel) 12, 2873. doi: 10.3390/plants12152873
Hu, S., Zhang, Y., Yu, H., Zhou, J., Hu, M., Liu, A., et al. (2022). Colletotrichum spp. diversity between leaf anthracnose and crown rot from the same strawberry plant. Front. Microbiol. 13. doi: 10.3389/fmicb.2022.860694
Huang, L., Li, Q. C., Zhang, Y., Li, D. W., Ye, J. R. (2016). Colletotrichum gloeosporioides sensu stricto is a pathogen of leaf anthracnose on evergreen spindle tree (Euonymus japonicus). Plant Dis. 100, 672–678. doi: 10.1094/PDIS-07-15-0740-RE
Huang, R. H., Zhong, F. T., Liu, Y. L., Chen, J. G. (2022). First report of Colletotrichum siamense causing anthracnose in Allamanda cathartica in China. Plant Dis. 106, 1757. doi: 10.1094/PDIS-08-21-1859-PDN
Hyde, K., Cai, L., Mckenzie, E., Yl, Y., Jz, Z., H, P. (2009). Colletotrichum: A catalogue of confusion. Fungal Divers. 39, 1–17.
Ji, Y., Li, X., Gao, Q.-H., Geng, C., Duan, K. (2022). Colletotrichum species pathogenic to strawberry: discovery history, global diversity, prevalence in China, and the host range of top two species. Phytopathol. Res. 4, 42. doi: 10.1186/s42483-022-00147-9
Kang, D., Kim, J., Lee, Y., Balaraju, K., Jeon, Y. (2022). First report of anthracnose of Gossypium indicum caused by Colletotrichum theobromicola in Korea. Plant Dis. 106, 1068. doi: 10.1094/PDIS-07-21-1386-PDN
Kim, J. S., Hassan, O., Go, M. J., Chang, T. (2021). First report of Colletotrichum aenigma causing anthracnose of Grape (Vitis vinifera) in Korea. Plant Dis. 105, 2729. doi: 10.1094/PDIS-11-20-2458-PDN
Kishino, H., Hasegawa, M. (1989). Evaluation of the maximum likelihood estimate of the evolutionary tree topologies from DNA sequence data, and the branching order in hominoidea. J. Mol. Evol. 29, 170–179. doi: 10.1007/BF02100115
Kuang, W., Ye, L., Gao, W., Ma, J., Zhang, L., Xiong, G., et al. (2022). First report of Colletotrichum siamense causing anthracnose on Eriobotrya japonica in China. Plant Dis. 107, 2221. doi: 10.1094/PDIS-06–22-1341-PDN
Lee, H. B., Park, J. Y., Jung, H. S. (2005). First report of leaf anthracnose caused by Colletotrichum boninense on spindle trees. Plant Pathol. 54, 254–254. doi: 10.1111/j.1365-3059.2005.01130.x
Li, Q., Bu, J., Shu, J., Yu, Z., Tang, L., Huang, S., et al. (2019). Colletotrichum species associated with mango in southern China. Sci. Rep. 9, 18891. doi: 10.1038/s41598–019-54809–4
Li, F., Chen, J., Chen, Q., Liu, Z., Sun, J., Yan, Y., et al. (2023). Identification, pathogenicity, and sensitivity to fungicide of Colletotrichum species that causes walnut anthracnose in Beijing. Agronomy 13, 214. doi: 10.3390/agronomy13010214
Lin, L., Pan, M., Gao, H., Tian, C., Fan, X. (2023). The potential fungal pathogens of Euonymus japonicus in Beijing, China. J. Fungi (Basel) 9, 271. doi: 10.3390/jof9020271
Liu, R., Li, J., Zhang, L., Feng, T., Zhang, Z., Zhang, B. (2021). Fungicide difenoconazole induced biochemical and developmental toxicity in wheat (Triticum aestivum L.). Plants (Basel) 10, 2304. doi: 10.3390/plants10112304
Liu, F., Ma, Z. Y., Hou, L. W., Diao, Y. Z., Wu, W. P., Damm, U., et al. (2022). Updating species diversity of Colletotrichum, with a phylogenomic overview. Stud. Mycol 101, 1–56. doi: 10.3114/sim.2022.101.01
Mahoney, M. J. (1979). Identification, etiology and control of Euonymus fortunei anthracnose caused by Colletotrichum gloeosporioides. Plant Dis. 64, 854–856. doi: 10.7275/20483145
Mora-Aguilera, J. A., Ríos-López, E. G., Yáñez-Zúñiga, M., Rebollar-Alviter, A., Nava-Díaz, C., Leyva-Mir, S. G., et al. (2021). Sensitivity to MBC fungicides and prochloraz of Colletotrichum gloeosporioides species complex isolates from mango orchards in Mexico. J. Plant Dis. Protect 128, 481–491. doi: 10.1007/s41348-020-00412-z
Oliveira, M. S., Wang, N.-Y., Peres, N. A. (2022). Multilocus phylogenetic analyses of Colletotrichum gloeosporioides species complex causing crown rot on strawberry in Florida. Phytopathology 112, 898–906. doi: 10.1094/PHYTO-04-20-0151-R
Pérez, P. (2020). Chemical management of anthracnose-twister (Colletotrichum gloeosporioides and Fusarium fujikuroi) disease of onion (Allium cepa). Plant Pathol. quarantine. 10, 198–216. doi: 10.5943/ppq/10/1/19
Qin, W., Li, X., Huang, J. B., Qiao, G. H. (2022). First Report of Colletotrichum theobromicola Causing Anthracnose on Euonymus japonicus in China. Plant Dis. 107, 1629. doi: 10.1094/PDIS-08–22-1789-PDN
Raj, P., Ekabote, S. D., Patil, B., Ramesh, A. N., Onkarappa, S. (2024). In vitro and in vivo evaluation of fungicides against anthracnose disease on pomegranate (Punica granatum L.) caused by Colletotrichum gloeosporioides. Crop Prot. 178, 106598. doi: 10.1016/j.cropro.2024.106598
Roman, D. L., Voiculescu, D. I., Filip, M., Ostafe, V., Isvoran, A. (2021). Effects of triazole fungicides on soil microbiota and on the activities of enzymes found in soil: a review. Agriculture. 11, 893. doi: 10.3390/agriculture11090893
Ronquist, F., Huelsenbeck, J. P. (2003). MrBayes 3: Bayesian phylogenetic inference under mixed models. Bioinformatics 19, 1572–1574. doi: 10.1093/bioinformatics/btg180
Singh, R., Doyle, V. P. (2017). Boxwood dieback caused by Colletotrichum theobromicola: a diagnostic guide. Plant Hlth Prog. 18, 174–180. doi: 10.1094/PHP-04-17-0024-DG
Sun, Y. C., Damm, U., Huang, C. J. (2019). Colletotrichum plurivorum, the causal agent of anthracnose fruit rot of papaya in Taiwan. Plant Dis. 103, 1040. doi: 10.1094/PDIS-08-18-1423-PDN
Swofford, D. (2002). PAUP*. Phylogenetic analysis using parsimony (*and Other Methods), version 4.0b10, 4 ed (New England: Sinauer Associates). doi: 10.1111/j.0014–3820.2002.tb00191.x
Tan, X., Zhao, J., Qiao, G., Liu, J., Hsiang, T., Yu, Z., et al. (2023). Morphological characterization and pathogenicity of Colletotrichum aenigma and C. siamense causing anthracnose on Euonymus japonicus in Beijing, China. Plant Pathol. 72, 430–441. doi: 10.1111/ppa.13681
Thompson, J. D., Gibson, T. J., Plewniak, F., Jeanmougin, F., Higgins, D. G. (1997). The CLUSTAL_X windows interface: flexible strategies for multiple sequence alignment aided by quality analysis tools. Nucleic Acids Res. 25, 4876–4882. doi: 10.1093/nar/25.24.4876
Vieira, W. A. S., Michereff, S. J., De Morais, M. A., Hyde, K. D., Câmara, M. P. S. (2014). Endophytic species of Colletotrichum associated with mango in northeastern Brazil. Fungal Divers. 67, 181–202. doi: 10.1007/s13225-014-0293-6
Vinggaard, A. M., Hass, U., Dalgaard, M., Andersen, H. R., Bonefeld-Jørgensen, E., Christiansen, S., et al. (2006). Prochloraz: an imidazole fungicide with multiple mechanisms of action. Int. J. Androl 29, 186–192. doi: 10.1111/j.1365-2605.2005.00604.x
Wang, Y. C., Hao, X. Y., Wang, L., Bin, X., Wang, X. C., Yang, Y. J. (2016). Diverse Colletotrichum species cause anthracnose of tea plants (Camellia sinensis (L.) O. Kuntze) in China. Sci. Rep. 6, 35287. doi: 10.1038/srep35287
Wang, Y., Wang, M., Xu, L., Sun, Y., Feng, J. (2020). Baseline sensitivity and toxic action of the sterol demethylation inhibitor flusilazole against Botrytis cinerea. Plant Dis. 104, 2986–2993. doi: 10.1094/PDIS-11-19-2478-RE
Weir, B. S., Johnston, P. R., Damm, U. (2012). The Colletotrichum gloeosporioides species complex. Stud. Mycol 73, 115–180. doi: 10.3114/sim0011
Wu, W. P. (1992). Note on some Coelomycetes on Euonymus japonicus I. Melanoconiales. J. Hebei Acad. Sci. 1, 65–74. doi: 10.16191/j.cnki.hbkx.1992.01.011
Wu, M. (2019). First Report of Colletotrichum siamense causing anthracnose on Euonymus japonicus in China. Plant Dis. 104, 587. doi: 10.1094/PDIS-04–19-0824-PDN
Yan, J., Jayawardena, R., Goonasekara, I., Wang, Y., Zhang, W., Liu, M., et al. (2014). Diverse species of Colletotrichum associated with grapevine anthracnose in China. Fungal Divers. 71, 233–246. doi: 10.1007/s13225-014-0310-9
Yao, J., Yu, D., Cheng, Y., Kang, Z. (2018). Histological and cytological studies of plant infection by Erysiphe euonymi-japonici. Protoplasma 255, 1613–1620. doi: 10.1007/s00709-018-1254-9
Yin, X. J., Li, Y. L., Pu, Z. G., Shang, G. L. (2008). Evaluation of water-saving and drought resistance of landscape tree species in North China. J. Agric. Univ. Hebei 31, 22–29.
Zhang, L., Song, L., Xu, X., Zou, X., Duan, K., Gao, Q. (2020). Characterization and fungicide sensitivity of Colletotrichum species causing strawberry anthracnose in Eastern China. Plant Dis. 104, 1960–1968. doi: 10.1094/PDIS-10-19-2241-RE
Zhang, Z., Yan, M., Li, W., Guo, Y., Liang, X. (2021). First report of Colletotrichum aenigma causing apple glomerella leaf spot on the granny smith cultivar in China. Plant Dis. 105, 1563. doi: 10.1094/PDIS-10-20-2298-PDN
Zhu, Z., Dong, Z., Mo, R., Zhang, C., Zuo, Y., Yu, C., et al. (2022). First report of Colletotrichum aenigma causing anthracnose on mulberry leaves in China. Plant Dis. 107, 571. doi: 10.1094/PDIS-05–22-1107-PDN
Zhu, Y., Ma, M., Zhang, S., Li, H. (2023). Baseline sensitivity and resistance mechanism of Colletotrichum isolates on tea-oil trees of China to tebuconazole. Phytopathology. 113, 1022–1033. doi: 10.1094/PHYTO-09-22-0325-R
Keywords: Euonymus japonicus, Colletotrichum, anthracnose, fungicides, pathogenicity
Citation: Liu Y, Tan X, Zhao J, Niu Y, Hsiang T, Yu Z and Qin W (2024) Diversity of Colletotrichum species associated with anthracnose on Euonymus japonicus and their sensitivity to fungicides. Front. Plant Sci. 15:1411625. doi: 10.3389/fpls.2024.1411625
Received: 03 April 2024; Accepted: 03 June 2024;
Published: 13 June 2024.
Edited by:
Franco Nigro, University of Bari Aldo Moro, ItalyReviewed by:
Juan Manuel Tovar-Pedraza, National Council of Science and Technology (CONACYT), MexicoAbhay K. Pandey, North Bengal Regional R & D Center, India
Zhang Chuanqing, Zhejiang Agriculture and Forestry University, China
Copyright © 2024 Liu, Tan, Zhao, Niu, Hsiang, Yu and Qin. This is an open-access article distributed under the terms of the Creative Commons Attribution License (CC BY). The use, distribution or reproduction in other forums is permitted, provided the original author(s) and the copyright owner(s) are credited and that the original publication in this journal is cited, in accordance with accepted academic practice. No use, distribution or reproduction is permitted which does not comply with these terms.
*Correspondence: Zhihe Yu, emhpaGV5dUBob3RtYWlsLmNvbQ==; Wentao Qin, cWlud2VudGFvQGlwcGJhYWZzLmNu
†These authors have contributed equally to this work