- 1Escuela Profesional de Agronomía, Universidad Nacional de San Martín, Tarapoto, San Martín, Peru
- 2Salud Agroforestal, Instituto de Investigaciones en Salud Agroforestal (IISA), Tarapoto, Peru
- 3Escuela Profesional de Ingeniería Ambiental, Universidad César Vallejo, Tarapoto, San Martín, Peru
- 4Facultad de Ingeniería y Ciencias, Universidad Nacional Autónoma de Alto Amazonas, UNAAA, Yurimaguas, Peru
- 5Facultad de Ingeniería y Ciencias Ambientales, Universidad Nacional Intercultural de la Amazonía, Pucallpa, Peru
- 6Facultad de Zootecnica, Universidad Nacional de la Amazonia Peruana, Iquitos, Peru
- 7Área de Ciencias Forestales, Instituto Nacional de Innovación Agraria - INIA, Iquitos, Maynas, Peru
- 8Centro de Investigación e Innovación para el Cambio Climático (CiiCC), Universidad Santo Tomás, Valdivia, Chile
- 9Amsterdam Institute for Life and Environment, Section Ecology & Evolution, Vrije Universiteit Amsterdam, Amsterdam, Netherlands
Introduction: Secondary forests and coffee cultivation systems with shade trees might have great potential for carbon sequestration as a means of climate change adaptation and mitigation. This study aimed to measure carbon stocks in coffee plantations under different managements and secondary forest systems in the Peruvian Amazon rainforest (San Martín Region).
Methods: The carbon stock in secondary forest trees was estimated using allometric equations, while carbon stocks in soil, herbaceous biomass, and leaf litter were determined through sampling and laboratory analysis.
Results: The biomass carbon stock in secondary forests was 132.2 t/ha, while in coffee plantations with Inga sp. shade trees was 118.2 t/ha. Carbon stocks were 76.5 t/ha in coffee with polyculture farming, while the lowest amount of carbon was found in coffee without shade trees (31.1 t/ha). The carbon sequestered by coffee plants in all agroforestry systems examined had an average of 2.65 t/ha, corresponding to 4.63 % of the total carbon sequestered, being the highest stored in the coffee system with Inga sp. shade trees. A higher content of glomalin-related soil proteins (GRSP) was found in coffee without shade trees, with 18.5 mg/g.
Discussion: These results point to Inga sp. as a compatible model of shade system for coffee farms. However, broader-scale time-average measurements and carbon dioxide emissions should be assessed in these study systems to have a full understanding of their climate impacts.
1 Introduction
Despite multiple efforts and studies, there is still no balanced consensus on the impact of agronomic intensification on shade trees (Haggar et al., 2021). This agroforestry tool could be highly threatened due to global climate change, challenging farmers to maintain agricultural production levels in the future (Gomes et al., 2020). Nevertheless, agroforestry has great climate change mitigation potential, particularly since carbon storage and biodiversity conservation payments are of special interest to coffee farmers (Koutouleas et al., 2024). In particular, the importance of coffee agroforestry systems to preserve biodiversity and ecosystem services has been recognized in different parts of the world (Hundera et al., 2013; Solis et al., 2020). Since coffee shrubs are perennial crops, coffee-based agroforestry practices are believed to have higher biomass carbon sequestration, biodiversity, and ecosystem function than other agroforestry practices (Buechley et al., 2015; Hylander et al., 2013; Tesfay et al., 2022).
There is limited knowledge about the effects of arbuscular mycorrhizal fungi (AMF) on carbon sequestration in agroforestry systems across different agroecological settings (Tschora and Cherubini, 2020). Such a role of AMF can be mediated or explained by their production of glomalin. Nautiyal et al. (2019) highlighted the importance of glomalin in maintaining soil aggregation and its positive correlation with soil organic carbon (SOC), which not only increases total carbon stock but also binds to soil organic matter (SOM), preventing soil erosion and further improving its aggregation. At the same time, AMF contribute to a greater extent to regulating glomalin and glomalin-related soil protein (GRSP) contents, which are important indicators of SOC stock (Li et al., 2020; Santos et al., 2022), varying according to the type of agroforestry system (Santos et al., 2022). Thus, Cai et al. (2023) found that GRSP content significantly promoted SOC sequestration. Therefore, GRSP is an important component of the soil carbon pool, as it improves the structure of soil aggregates. Likewise, GRSP produced by AMF is a carbon reserve that influences the formation and stabilization of aggregates and contributes to soil carbon sequestration (Nautiyal et al., 2019). Therefore, there is an urgent need to study the potential impact of glomalin secreted by AMF in contributing to carbon sequestration in forests of the Peruvian Amazon.
Secondary forests are important carbon sinks, absorbing CO2 from the atmosphere through photosynthesis and photosynthate storage in their aboveground living biomass. Therefore, healthy secondary forests are well-adapted and constitute efficient carbon sinks whose conservation is vital to mitigate and adapt to climate change and to support biodiversity (Griscom et al., 2020; Aragón et al., 2021). They also have higher CO2 sequestration rates than costly and poorly adapted afforestation and reforestation initiatives (Chazdon and Guariguata, 2016).
Despite the potential of secondary forests and coffee agroecosystems for carbon sequestration, they are almost completely ignored in land use management in Latin America. In Peru, these Amazonian ecosystems could help to reach the country’s compromises on climate change adaptation and mitigation. Peru committed to restoring 3.2 million hectares of forests by 2030, of which 2 million hectares will be restored through commercial plantations—including naturalized and non-native species. However, in these commitments, secondary forests were not explicitly included. Such commitments were made aiming at reducing emissions by 30% (Aragón et al., 2021).
In the Peruvian Amazon, there is little research on above- and belowground carbon variations in agroforestry systems with coffee and shade trees. Overall, the Amazon rainforest has barely been investigated regarding its mycorrhizal biodiversity and functioning (Marín et al., 2022). Therefore, this exploratory study aimed to quantify carbon stocks in coffee plantations under different agricultural managements and secondary forest systems in the Peruvian Amazon rainforest. However, these measurements correspond to a single measurement in time and should be followed through several years and also include carbon dioxide emissions.
2 Materials and methods
2.1 Study area
The study was carried out in the annexes of the Tabalosos District in the province of Lamas, San Martín region in the Peruvian Amazon between September 2022 and January 2023 (Figure 1). The province is located at an altitude range between 310 and 814 m.a.s.l. The average annual rainfall and temperature in this district are 1,013 mm and 32°C, respectively, with August and December being the summer months and March to April being the winter months, very similar to the majority of districts in the region.
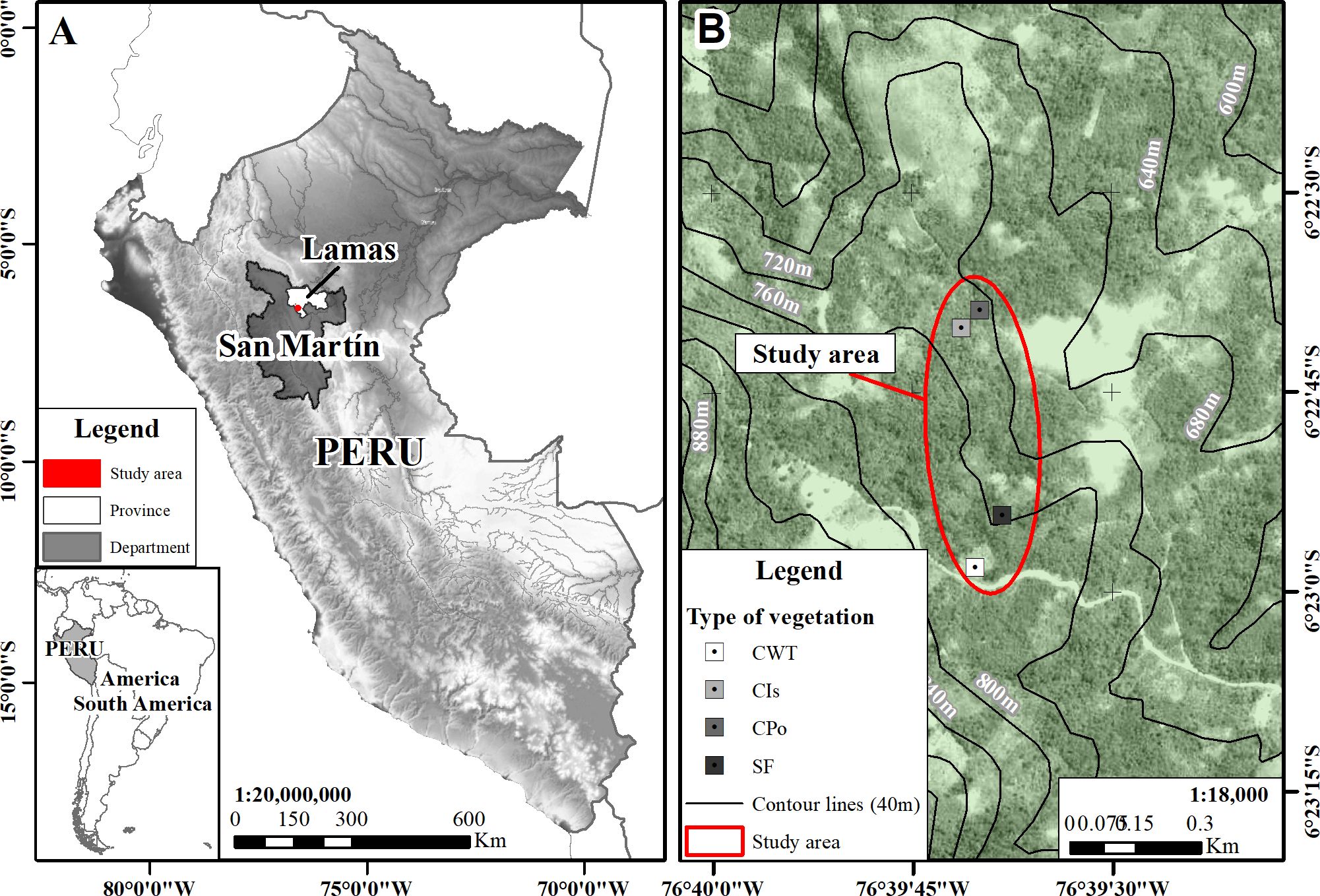
Figure 1. Study site location. (A) San Martín region in the Peruvian Amazon. (B) Plots of studied coffee agroforestry and secondary forest.
2.2 Sampling design
Four vegetation cover types were identified in three zones with coffee and one zone of secondary forest (Table 1). These were as follows: 1) coffee without shade trees (monoculture), that is, coffee plantations with a higher planting density than the other systems (2,000 coffee plants per hectare), made up of the Caturra, Pache, and Catimore varieties (all growing together); 2) coffee with Inga sp. shade trees, with a density of 1,500 plants per hectare of the Caturra, Catimore, and Pache varieties (all growing together); 3) coffee with polyculture farming, with a density of 1,450 plants per hectare of the Caturra and Pache varieties, composed of a diversity of agroforestry crops (specified in Table 1); and 4) a secondary forest with a diversity of trees, which were more than 15 years old (Table 1). Organic coffee farms between 9 and 13 years old (age of coffee trees) were considered, with similar agronomic management between them.
Following Grünzweig et al. (2003) and Roxburgh et al. (2006) regarding plot size, the biomass and carbon of the trees were evaluated in four plots (one plot per cover type) with an area of 30 m × 30 m with slopes between 0° and 40°. Four subplots of 4 m × 25 m (100 m2) were established in each plot to facilitate the evaluation of trees and coffee shrubs, for a total of 16 subplots. The height and diameter of the woody components were measured using a Suunto clinometer and tape measure, respectively. Table 1 shows the density of the different plants per treatment, per 100 m2 subplot. The diameter of coffee plants was measured at 15 cm above the ground (d15) and for trees at 1.3 m above the ground [diameter at breast height (DBH)]. In the same subplots, herbaceous material and litter were measured in a 0.5 × 0.5 m grid according to Siarudin et al. (2021). After removing litter and organic debris, soil was sampled between 0 cm and 15 cm of soil depth, making soil pits in each management system and considering four subplots per system (for a total of 16 soil samples).
2.3 Estimation of aboveground carbon
Allometric equations were used to estimate aboveground biomass based on DBH and d15 (Supplementary Table 1). Specifically, two allometric equations were used for tropical rainforest species (Supplementary Table 1), as one (Nascimento and Laurance, 2002) applies to trees with a DBH of between 2 cm and 5 cm, while the other (Chave et al., 2014) applies to trees with a DBH higher than 5 cm. The wood density value for each identified species was obtained from the “Global Wood Density Database” (Chave et al., 2009). Root biomass was estimated using the regression equations developed by Cairns et al. (1997) (Supplementary Table 1). The aboveground and root biomass values obtained for each tree within the same plot were summed to calculate the total tree biomass of the plot, and the result was extrapolated to obtain the biomass stock of 1 ha. Herbaceous biomass was obtained by determining the wet weight of a sample of approximately 500 g; the samples were then taken to the laboratory and dried at 70°C for 48 h to determine the dry weight. With this information, the moisture content and dead biomass were determined using the following equations:
Leaf litter biomass was determined in the same way as dead wood biomass. It was assumed that the carbon present in the biomass could be 50% (Intergovernmental Panel on Climate Change - IPCC, 2003). The C content obtained in each sampled component was extrapolated to estimate C stocks per hectare.
2.4 Estimation of soil organic carbon
The cylinder method of 5.5-cm diameter and 5-cm height proposed by Blake and Hartge (1986) was used to calculate the bulk/apparent density (AD) of the soil in g/cm3, determined by the following formula:
where AD is the apparent density (g/cm3), Wd is the weight of the oven-dried soil sample (g), and V is the volume of the sampled soil (cm3). Therefore, SOC was determined using the method developed by Walkley and Black (1934) in the laboratory, using the following formula:
where OC is soil organic carbon content (%), Pf is soil sampling depth (cm), and AD is the apparent density (g/cm3).
Thus, we determined the weight of sequestered carbon dioxide in the tree by multiplying the tree’s carbon weight by 3.67 (Paniagua-Ramirez et al., 2021).
2.5 Estimation of arbuscular mycorrhizal abundance and glomalin content in soils
The quantification of AMF spores was performed by wet sieving and decanting, as proposed by Gerdemann and Nicolson (1963), with some modifications. Likewise, in order to determine GRSP content, soil samples were taken following the methodology of Solis et al. (2022). The total GRSP fraction was extracted according to Wright and Upadhyaya (1998) and quantified by the method of Bradford (1976).
2.6 Statistical analyses
The statistical analyses were performed through R Studio (R Core Team, 2023). To analyze the effect of the vegetation cover factor on carbon content, AMF spores, and GRSP content, non-parametric statistics were used to evaluate carbon reserves. The Mann–Whitney test was used to compare two medians with a probability of error of 5%. A principal component analysis (PCA) biplot was performed to evaluate the correlations between variables. The variables were standardized, and the fviz_pca_biplot function of the Factoextra package of R (Kassambara and Mundt, 2022) was used for the PCA. A correlogram was also performed to test these correlations, using Pearson’s correlation coefficient (p < 0.05), using the ggpairs function of the GGally package in R (Schloerke et al., 2022).
3 Results
3.1 Carbon storage capacity in coffee and secondary forest
Carbon stocks varied according to the type of vegetation cover (Figure 2). The overall mean storage of carbon stocks in the different types of vegetation cover was 89.5 t C/ha. The total carbon storage capacity (t C/ha) in the different cover types decreased in the following order: SOC (38.74 C/ha) > aboveground carbon (AGC; 37.96 C/ha) > belowground carbon (BGC; 6.06 C/ha) > litter carbon (LC; 3.93 C/ha) > herbaceous carbon (HC; 2.84 C/ha).
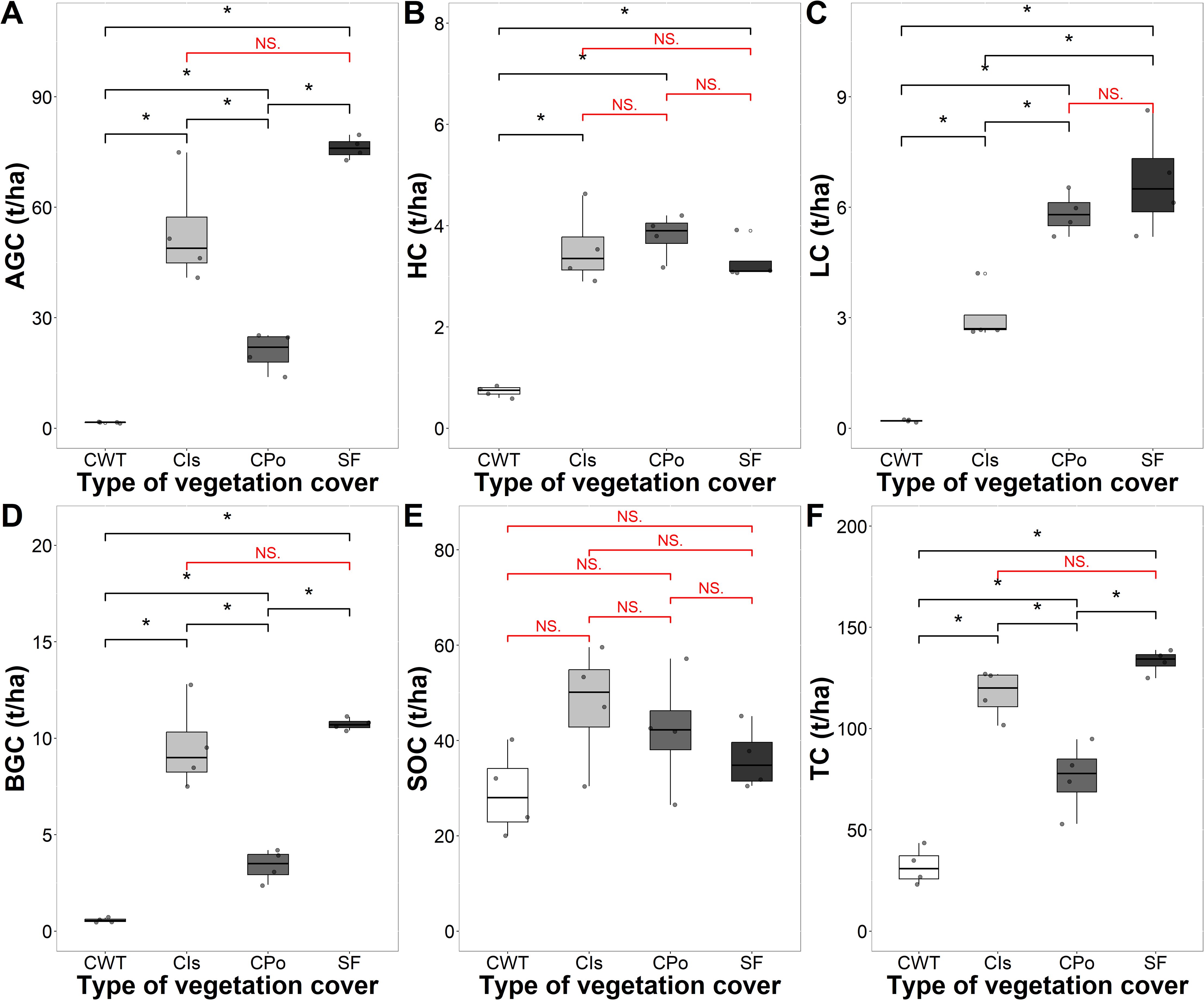
Figure 2. Carbon storage of coffee agroforestry and secondary forest systems by carbon component. (A) Carbon storage in the aboveground component (AGC). (B) Carbon storage in the herbaceous component (HC). (C) Carbon storage in leaf litter (LC). (D) Carbon storage in the belowground (BGC). (E) Carbon storage in soil organic carbon (SOC). (F) Total carbon storage. CWT, coffee without shade trees; Cis, coffee with Inga sp. shade trees; Cpo, coffee with polyculture farming; SF, secondary forest. Mann–Whitney test: * at p < 0.05 and NS. (non-significant) at p > 0.05.
The total carbon stock in the different vegetation cover types ranged from 32.1 t C/ha in coffee without shade trees to 133.08 t C/ha in the secondary forest (Figure 2F). The highest carbon content was found in the secondary forest and coffee with Inga sp. shade trees with a total of 133.08 and 117.13 t/ha, respectively, being statistically higher than those of the other covers (p < 0.05).
Aboveground carbon stocks varied from 1.58 t C/ha in coffee without shade trees to 76.13 t/ha in the secondary forest (Figure 2). Vegetation covers significantly influenced carbon stocks in the different components with the exception of SOC (p < 0.05) (Figure 2). The secondary forest and the coffee system with Inga sp. shade trees had the highest AGC (76.13 and 53.38 t C/ha) and BGC (10.7 t C/ha) carbon stocks (Figures 2A, D). Likewise, coffee without shade trees had the lowest HC and LC at 0.73 and 0.20 t C/ha, respectively (Figures 2B, C). With respect to SOC, we found values ranging from 29.05 t C/ha in coffee without shade trees to 45.7 t C/ha in coffee with Inga sp. shade trees (Figure 2E).
The proportions of carbon stored in the different vegetation and soil compartments were affected by the different vegetation cover types (p < 0.05). SOC presented the highest proportion of carbon in the three coffee covers (CWT, coffee without shade trees; Cis, coffee with Inga sp. shade trees; Cpo, coffee with polyculture farming), while with respect to the secondary forest (SF), the highest proportion was obtained in AGC (Figure 3). The SF sequestered 57.27% of carbon as AGC, 8.06% as BGC, 27.19% as SOC, and the rest as LC and HC (Figure 3D). The coffee with Inga sp. shade trees sequestered 45.17% of carbon as AGC, 41.08% as SOC, and 13.75% as LC, HC, and BGC (Figure 3B). Coffee with polyculture farming sequestered 54.81% of carbon as SOC, 27.30% as AGC, 5.32% as LC, and 12.57% as HC and BGC (Figure 3C), whereas the coffee without shade trees sequestered 89.90% of carbon as SOC, followed by AGC, HC, LC, and BGC with 5.22%, 0.58%, 2.42%, and 1.88%, respectively (Figure 3A).
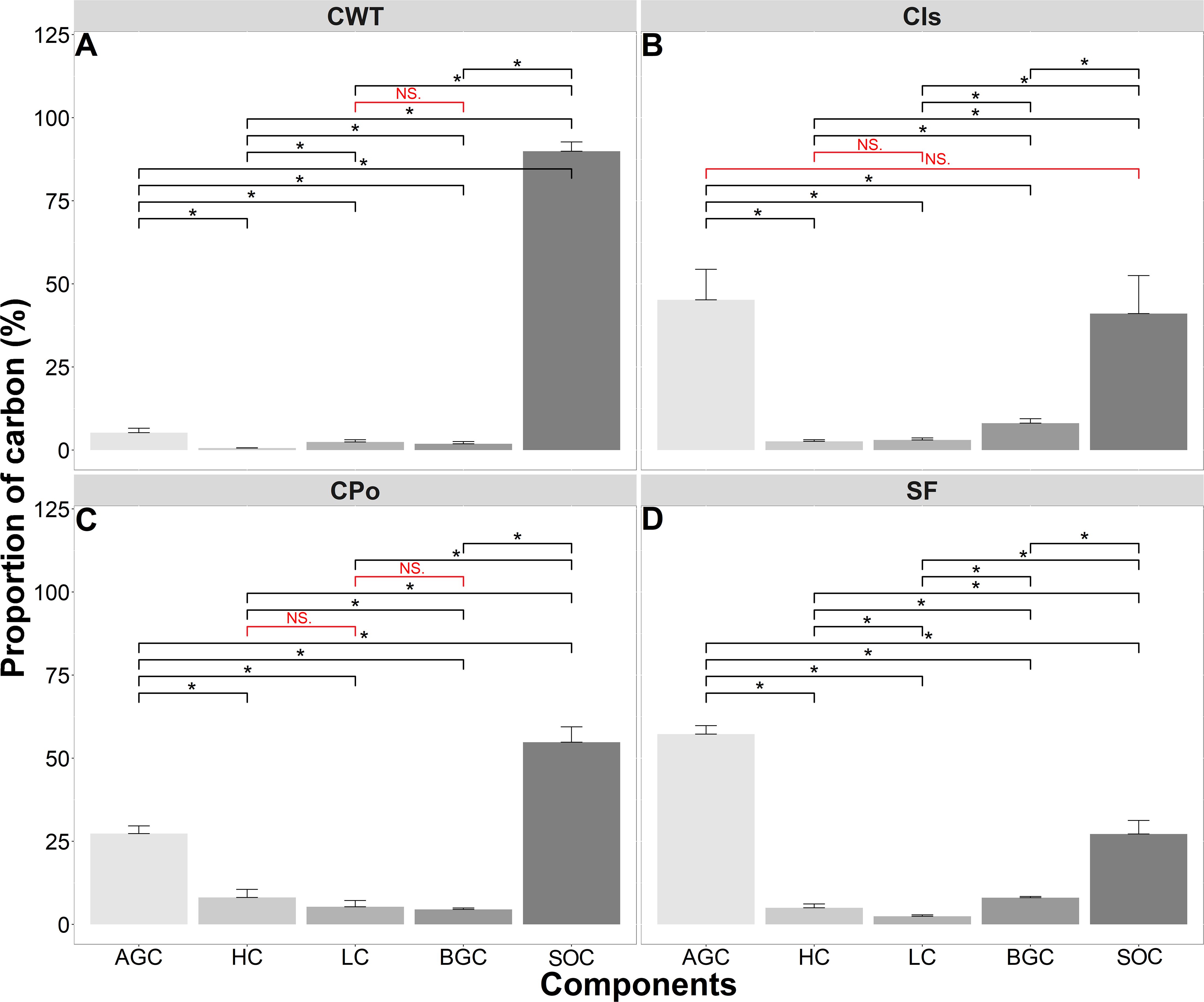
Figure 3. The proportion of carbon components stored in the different types of vegetation cover. (A) Proportion of carbon in coffee without shade trees (CWT). (B) Proportion of carbon in Coffee with Inga sp. shade trees (CIs). (C) Proportion of carbon in coffee with polyculture farming (CPo). (D) Proportion of carbon in secondary forest (SF). AGC, aboveground carbon; HC, herbaceous carbon; LC, leaf litter carbon; BGC, belowground carbon; SOC, soil organic carbon. Mann–Whitney test: * at p < 0.05 and NS. (non-significant) at p > 0.05.
3.2 Glomalin and spore content of arbuscular mycorrhizal fungi
The lowest number of AMF spores was found in coffee with polyculture and secondary forest with an average of 110 and 102 spores per 25 g of soil, respectively, showing significant differences compared to coffee without trees (Figure 4A). The highest GRSP content was found in coffee without shade trees with an average of 18.50 mg/g, and the lowest content was found in the secondary forest with 7.08 mg/g on average, showing significant differences for both covers (p < 0.05) (Figure 4B). In contrast, coffee with Inga sp. shade trees and coffee with polyculture farming did not show significant differences in GRSP content among them (Figure 4B).
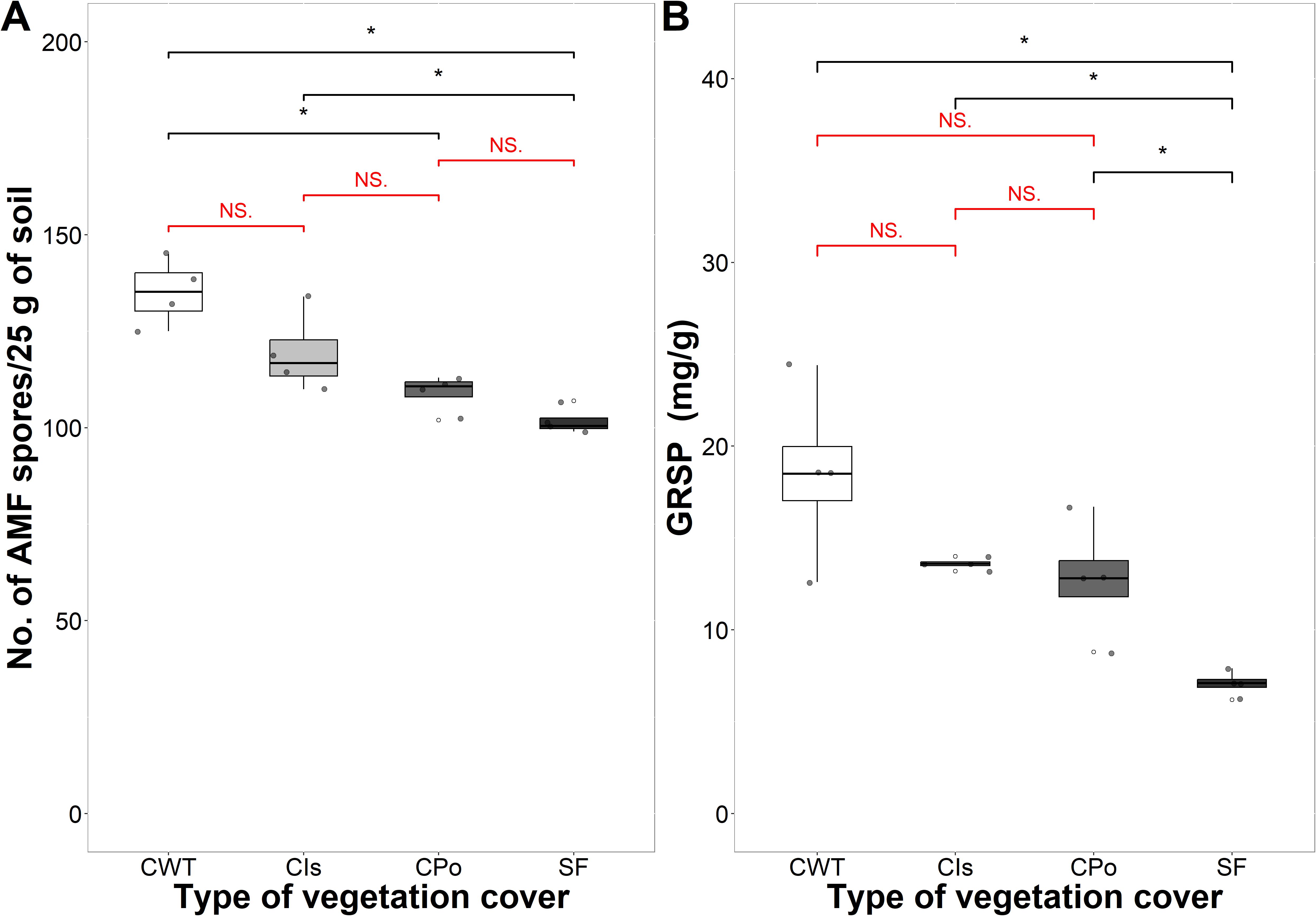
Figure 4. Glomalin in different types of vegetation cover. (A) Number of arbuscular mycorrhizal fungal (AMF) spores per 25 g of soil. (B) Glomalin-related soil protein (GRSP) (mg/g). CWT, coffee without shade trees; CIs, coffee with Inga sp. shade trees; CPo, coffee with polyculture farming; SF, secondary forest. Mann–Whitney test: * at p < 0.05 and NS. (non-significant) at p > 0.05.
The variation of carbon stocks, number of AMF spores, and GRSP content in the different vegetation covers was explained by 75.8% in the first two principal components of a PCA (Supplementary Figure 1). Coffee without shade trees was characterized by the highest values of GRSP and number of AMF spores, variables that were positively correlated (Supplementary Figures 1, 2). Likewise, the secondary forest was characterized by the highest values for AGC, BGC, and LC, variables that showed positive correlations. That is, carbon in aboveground biomass increases as underground biomass and litter biomass increase in the secondary forest cover.
GRSP ranged from 6.22 to 24.36 mg/g, and the number of AMF spores was between 99 and 145 spores per 25 g of soil, variables that presented a significant and positive correlation with an r2 value of 0.805. GRSP and the number of AMF spores presented significant and negative correlations with all carbon pools except SOC (Supplementary Figure 2). The number of AMF spores and glomalin concentration were influenced by the amounts of carbon stored, mainly by that sequestered in LC (r2 of −0.748 and −0.824 for GRSP and the number of AMF spores, respectively).
3.3 Carbon sequestration
Coffee plants also contributed to the total carbon stock in the different types of vegetation cover (Figure 5). The coffee plants growing with Inga sp. and polyculture presented greater carbon aboveground, belowground, and total carbon compared to coffee without trees (p < 0.05) (Figures 5A–C). The mean values of carbon sequestered by coffee plants with Inga sp. shade trees and polycultures were significantly different from those of coffee without shade trees but did not differ significantly from each other. The carbon sequestered by coffee plants was 2.98, 2.81, and 2.16 t C/ha in coffee with Inga sp. shade trees, coffee with polyculture farming, and coffee without shade trees, respectively (Table 2). Coffee plants contributed approximately 7.16%, 3.93%, and 2.57% of the carbon sequestered in coffee without shade trees, coffee with polyculture farming, and coffee with Inga sp. shade trees, respectively (Figure 5D). The overall average carbon sequestered by coffee plants in the different types of vegetation cover was 2.65 t C/ha (average of 4.63% of total sequestered carbon).
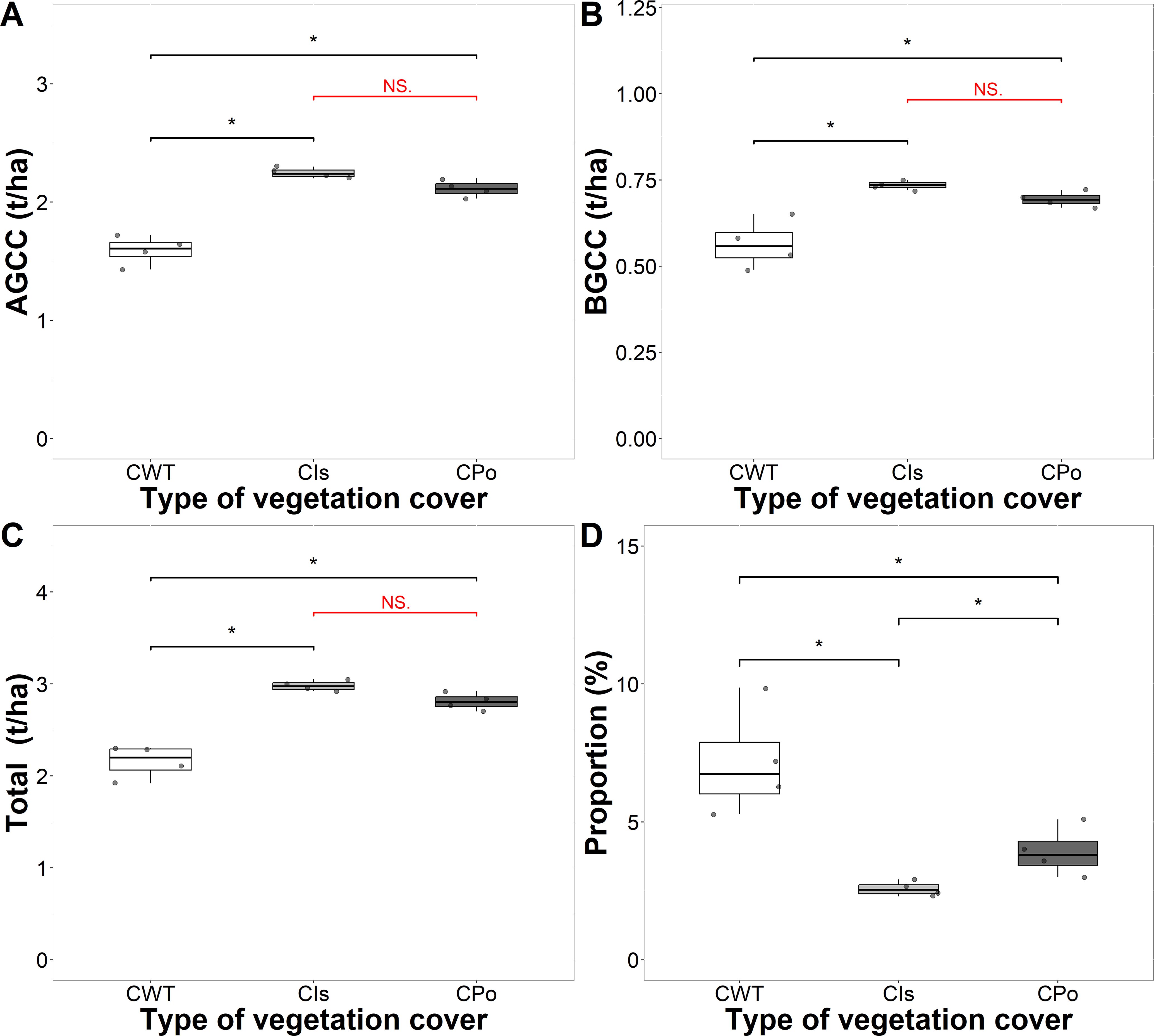
Figure 5. Amount and proportion of carbon stored in coffee plants in the coffee agroforestry systems by carbon component. (A) Carbon stored in aboveground coffee (AGCC). (B) Carbon stored in belowground coffee (BGCC). (C) Total carbon stored in coffee. (D) Proportion of carbon stored in coffee plants. CWT, coffee without shade trees; CIs, coffee with Inga sp. shade trees; CPo, coffee with polyculture farming. Mann–Whitney test: * at p < 0.05 and NS. (non-significant) at p > 0.05.
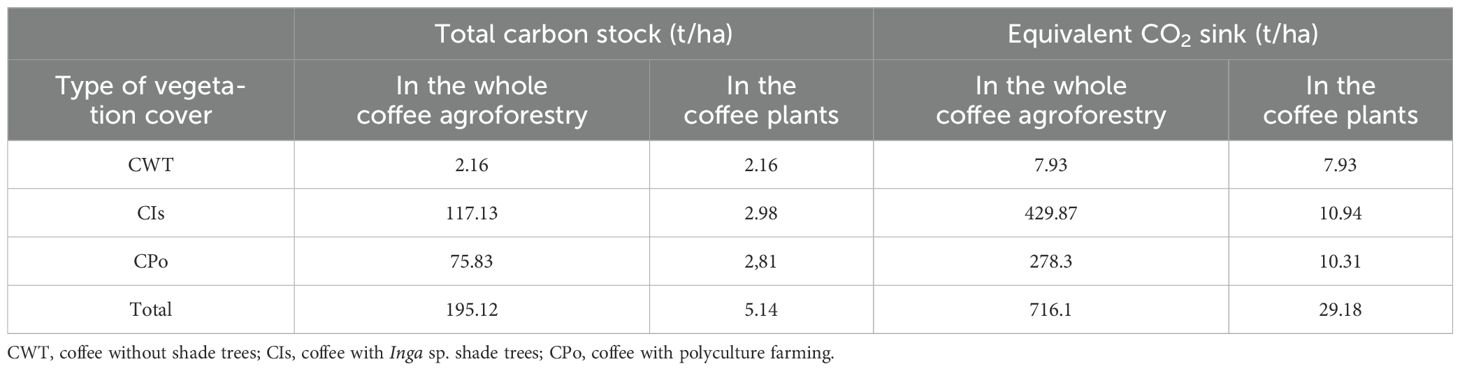
Table 2. Total carbon stock and carbon dioxide (CO2) equivalent sink in different coffee agroforestry systems and coffee plants.
In the study area, coffee agroforestry systems sequestered a large amount of carbon in vegetation, including coffee plants and soils proportional to the area of each stratum; approximately 117.13, 75.83, and 2.16 t C/ha were stored in coffee cover with Inga trees, polyculture, and coffee without shade trees, respectively (Table 2). This shows that a total of 195.12 t C/ha was stored in all coffee agroforestry systems in the study area. Particularly, coffee plants added a total of 7.93 t C/ha in the coffee agroforestry systems (Table 2). All coffee agroforestry systems in the current study area trapped 716.1 t of CO2 from the atmosphere, while coffee plants captured 29.18 t of CO2 from the atmosphere and stored it as carbon in the agroforestry systems.
4 Discussion
The higher carbon content present in the secondary forest was due to higher aboveground biomass and population density of the tree species present, as also found by Birhane et al. (2020), who observed that the least disturbed plant community, which had a higher density of trees and shrubs, had the highest SOC stocks compared to more disturbed communities. Secondary forests are of great importance due to the conservation and greater diversity of timber and non-timber trees present, storing more carbon than coffee crops. Soils that form under forests tend to accumulate high levels of organic carbon near the surface and have lower levels of carbon in the subsoil (Girmay et al., 2008). Cover types affected the proportion of carbon stocks stored in different compartments. The results indicate that the highest carbon content was stored as AGC followed by SOC.
Interestingly, regarding the different proportions of carbon components, the secondary forest and coffee with Inga sp. shade threes behaved similarly. These are surprising results since in our study, coffee systems were less diverse (coffee, 15 plants; Inga sp., 10 plants) than secondary forests (12 trees of different species; Table 1). However, coffee plots had higher total tree density and double the basal area (Table 1). This may be explained because both are ecosystems in a restoration phase with semi-woody species such as Inga sp. and forest species from the secondary forest. Also, both cover types had the same nitrogen content (0.18%) in their soils, which contributes to or may explain their similar SOC contents (and carbon stock proportions) due to the interconnection between N and C (Mehnaz et al., 2018; Jing et al., 2021). However, this good performance of the secondary forest (i.e., the other three subplots had approximately half of the aboveground carbon than the secondary forest) is most probably because each vegetation cover had just one 100-m2 subplot.
The highest number of AMF spores was found in coffee without shade systems, where the soils presented the lowest values of pH, total N, and available P with averages of 5.42, 0.13%, and 1.32 mg kg−1, respectively (Table 1). The larger population of coffee plants present in the coffee system without shade trees probably favors a symbiotic association between coffee plants and AMF, promoting favorable living habitats for the survival and massive multiplication of AMF spores (Vallejos-Torres et al., 2022). In contrast to previous studies (Wang et al., 2017; Singh et al., 2022; Fall et al., 2022), we did not find significant correlations between the number of AMF spores/GRSP and SOC, but we did find such significant (negative) correlations with BGC. In response to mycorrhization, coffee plants show a clear reorganization of the main metabolic pathways, which involve nutrient acquisition, carbon fixation, and primary and secondary metabolism, particularly under low phosphorus conditions (Chialva et al., 2023). This makes coffee a highly mycotrophic plant (Hernández-Acosta et al., 2021).
Our results on higher number of AMF spores on coffee without shade are analogous to those by Polo-Marcial et al. (2023), who found that the abundance and richness of AMF, especially glomerospores, were higher in agroforestry systems than in secondary forests. The presence of AMF plays an important role in the accumulation of GRSP, a critical component of the hyphal cell wall (Wang et al., 2023). Therefore, mycorrhizal hyphae prominently promote enhanced accumulation and preservation of organic carbon in aggregates and soil C pool (Wang et al., 2023). This may have contributed to soil carbon accumulation in coffee without shade trees with 28.0 t/ha.
The carbon stock found in coffee with Inga sp. shade trees (125.3 t/ha) was lower than the average carbon stocks reported for agroforestry systems with coffee by Niguse et al. (2022) in Ethiopia (287.1 t C/ha), by Schmitt-Harsh et al. (2012) in Guatemala (259 t C/ha), and by Soto-Pinto et al. (2010) in Mexico (213.80 t C/ha). Similarly, the average SOC content found in this study for the coffee system with Inga sp. shade trees (52.8 t/ha) was lower than that reported by Niguse et al. (2022) (91.5 t C/ha) and lower than that reported by Solis et al. (2020) (123.5 t C/ha), both with different shade trees than in our study. Differences in carbon stocks observed in different parts of the world could be attributed to a variety of factors, including coffee variety, management practices, plantation ages, and site factors such as climate and soil conditions. Overall, our findings show that coffee agroforestry systems could sequester a substantial amount of carbon by trapping CO2 from the atmosphere, which may help mitigate the effects of climate change (Niguse et al., 2022).
We also investigated the relationships between glomalin and SOC stocks. Glomalin is a soil component potentially produced by AMF; in turn, glomalin is a mixture of soil organic materials that are not unique to AMF (Irving et al., 2021; Holátko et al., 2021). Despite disagreements on the nature of “glomalin” (Holátko et al., 2021), it has been consistently associated with a long list of plant and soil health benefits, including soil aggregation and aggregate stability, soil carbon storage, and improved plant growth under abiotic stress (Plaza et al., 2013; Six and Paustian, 2014; (Nautiyal et al., 2019; Irving et al., 2021). Meanwhile, AMF has a positive impact on soil by producing organic acids and glomalin, which enhance carbon sequestration and stabilize soil macroaggregation (Fall et al., 2022). Likewise, Hawkins et al. (2023) confirmed the important contribution that mycorrhizal hyphae make to global soil carbon dynamics, as they fix—at least temporarily—an equivalent to ~36% of current annual CO2 emissions from fossil fuels.
4.1 Limitations
Carbon stocks varied according to the type of vegetation cover, with the secondary forest cover storing the highest carbon content, followed by the coffee system with Inga sp. shade trees, coffee with polyculture farming, and, to a lesser extent, the coffee system without shade trees. Secondary forests growing on previously cleared land could be a low-cost climate change mitigation strategy due to their potential to sequester CO2 (Elias et al., 2022). However, our results may differ if measurements are conducted over several years or if alterations to secondary forests (such as cutting them down) increase their CO2 emissions abruptly. Also, we had just one plot (and four subplots) per study system, so an increase in replications or plot size could change the results and their interpretation, particularly since many studies use a 20 × 50 m plot size (Somarriba et al., 2013; Goodall et al., 2015). Thus, these results should be interpreted as they are: a single point in time measurements of carbon stocks is still comparable, as they were conducted at the same time and following the same methodology.
5 Conclusions
Coffee plants associated with agroforestry systems sequester substantial amounts of carbon and have AMF that generate glomalin-related soil protein since coffee is a highly mycotrophic plant. That is, coffee benefits from the mycorrhizal association, contributing greatly to the accumulation of carbon in the soil. The carbon sequestration potential of coffee plants with Inga sp. shade trees is influenced by the compatibility of the trees present in the management system. Similarly, secondary forest plantations had very high carbon sequestration potential. Thus, our results should not be interpreted as a simplistic recommendation to prioritize coffee plantations with Inga sp. over secondary forests. Previous malpractices based solely on carbon capture have had negative effects on local biodiversity, including flora, fauna, and funga. In order to fully understand carbon stocks and their variability in these endangered ecosystems, we suggest follow-up studies on carbon stocks and emissions across different land uses in the Peruvian Amazon rainforest, which can lead to proper policy recommendations.
Data availability statement
The data sets generated during and analysed during the current study are available in the figshare repository: DOI: 10.6084/m9.figshare.27897243.
Author contributions
GV-T: Conceptualization, Investigation, Writing – review & editing, Data curation, Methodology, Supervision, Writing – original draft. NG-J: Writing – review & editing, Methodology, Supervision, Writing – original draft. RP-G: Writing – review & editing, Conceptualization, Investigation. LO: Conceptualization, Investigation, Writing – review & editing. PG-G: Investigation, Writing – review & editing. AQ: Funding acquisition, Investigation, Writing – review & editing. AL: Data curation, Visualization, Writing – review & editing. JS-R: Investigation, Writing – review & editing. JT-H: Funding acquisition, Investigation, Writing – review & editing. KR: Data curation, Investigation, Writing – review & editing. WM-C: Data curation, Investigation, Writing – review & editing. JB-V: Formal analysis, Investigation, Writing – review & editing. CM: Data curation, Formal analysis, Investigation, Methodology, Supervision, Writing – original draft, Writing – review & editing.
Funding
The author(s) declare that no financial support was received for the research, authorship, and/or publication of this article.
Acknowledgments
CM thanks ANID + Convocatoria Nacional Subvención a Instalación en la Academia Convocatoria Año 2021 + Folio No. SA77210019 and the Fondecyt Regular Project No. 1240186 (ANID—Chile, Convocatoria 2024).
Conflict of interest
The authors declare that the research was conducted in the absence of any commercial or financial relationships that could be construed as a potential conflict of interest.
Publisher’s note
All claims expressed in this article are solely those of the authors and do not necessarily represent those of their affiliated organizations, or those of the publisher, the editors and the reviewers. Any product that may be evaluated in this article, or claim that may be made by its manufacturer, is not guaranteed or endorsed by the publisher.
Supplementary material
The Supplementary Material for this article can be found online at: https://www.frontiersin.org/articles/10.3389/fpls.2024.1410418/full#supplementary-material
References
Aragón, F. M., Oteiza, F., Rud, J. P. (2021). Climate change and agriculture: Subsistence farmers’ response to extreme heat. Am. J. Econ. Pol'y. 13, 1–35.
Birhane, E., Ahmed, S., Hailemariam, M., Negash, M., Rannestad, M. M., Norgrove, L. (2020). Carbon stock and woody species diversity in homegarden agroforestry along an elevation gradient in southern Ethiopia. Agrofor. Syst. 94, 1099–1110. doi: 10.1007/s10457-019-00475-4
Blake, G. R., Hartge, K. H. (1986). Particle density. Methods Soil analysis: Part 1 Phys. Mineralogical Methods 5, 377–382.
Bradford, M. M. (1976). A rapid and sensitive method for the quantitation of microgram quantities of protein utilizing the principle of protein-dye binding. Anal. Biochem. 72, 248–254. doi: 10.1016/0003-2697(76)90527-3
Buechley, E. R., Şekercioğlu, Ç.H., Atickem, A., Gebremichael, G., Ndungu, J. K., Mahamued, B. A., et al. (2015). Importance of Ethiopian shade coffee farms for forest bird conservation. Biol. Conserv. 188, 50–60. doi: 10.1016/j.biocon.2015.01.011
Cai, C., Huang, F., Yang, Y., Yu, S., Wang, S., Fan, Y., et al. (2023). Effects of glomalin-related soil protein driven by root on forest soil aggregate stability and carbon sequestration during urbanization in Nanchang, China. Plants 12, 1847. doi: 10.3390/plants12091847
Cairns, M. A., Brown, S., Helmer, E. H., Baumgardner, G. A. (1997). Root biomass allocation in the world's upland forests. Oecologia. 111, 1–11. doi: 10.1007/s004420050201
Chave, J., Coomes, D., Jansen, S., Lewis, S. L., Swenson, N. G., Zanne, A. E. (2009). Towards a worldwide wood economics spectrum. Ecol. Lett. 12, 351–366. doi: 10.1111/j.1461-0248.2009.01285.x
Chave, J., Réjou‐Méchain, M., Búrquez, A., Chidumayo, E., Colgan, M. S., Delitti, W. B., et al. (2014). Improved allometric models to estimate the aboveground biomass of tropical trees. Glob Change Biol 20, 3177–3190. doi: 10.1111/gcb.12629
Chazdon, R. L., Guariguata, M. R. (2016). Natural regeneration as a tool for large-scale forest restoration in the tropics: prospects and challenges. Biotropica. 48, 716–730. doi: 10.1111/btp.12381
Chialva, M., Patono, D. L., de Souza, L. P., Novero, M., Vercellino, S., Maghrebi, M., et al. (2023). The mycorrhizal root-shoot axis elicits Coffea arabica growth under low phosphate conditions. New Phytol. 239, 271–285. doi: 10.1111/nph.18946
Elias, F., Ferreira, J., Resende, A. F., Berenguer, E., França, F., Smith, C. C., et al. (2022). Comparing contemporary and lifetime rates of carbon accumulation from secondary forests in the eastern Amazon. For. Ecol. Manage. 508, 120053. doi: 10.1016/j.foreco.2022.120053
Fall, A. F., Nakabonge, G., Ssekandi, J., Founoune-Mboup, H., Apori, S. O., Ndiaye, A., et al. (2022). Roles of arbuscular mycorrhizal fungi on soil fertility: Contribution in the improvement of physical, chemical, and biological properties of the soil. Front. Fungal Biol. 3. doi: 10.3389/ffunb.2022.723892
Gerdemann, J. W., Nicolson, T. H. (1963). Spores of mycorrhizal Endogone species extracted from soil by wet sieving and decanting. Trans. Br. Mycol. Soc 46, 235–244. doi: 10.1016/S0007-1536(63)80079-0
Girmay, G., Singh, B. R., Mitiku, H., Borresen, T., Lal, R. (2008). Carbon stocks in Ethiopian soils in relation to land use and soil management. Land Degrad. Dev. 19, 351–367. doi: 10.1002/ldr.844
Gomes, L. C., Bianchi, F. J. J. A., Cardoso, I. M., Fernandes, R. B. A., Fernandes Filho, E. I., Schulte, R. P. O. (2020). Agroforestry systems can mitigate the impacts of climate change on coffee production: A spatially explicit assessment in Brazil. Agric. Ecosyst. Environ. 294, 106858. doi: 10.1016/j.agee.2020.106858
Goodall, K. E., Bacon, C. M., Mendez, V. E. (2015). Shade tree diversity, carbon sequestration, and epiphyte presence in coffee agroecosystems: A decade of smallholder management in San Ramón, Nicaragua. Agric. Ecosyst. Environ. 199, 200–206. doi: 10.1016/j.agee.2014.09.002
Griscom, B. W., Busch, J., Cook-Patton, S. C., Ellis, P. W., Funk, J., Leavitt, S. M., et al. (2020). National mitigation potential from natural climate solutions in the tropics. Philos. Trans. R. Soc B: Biol. 375, 20190126. doi: 10.1098/rstb.2019.0126
Grünzweig, J. M., Lin, T., Rotenberg, E., Schwartz, A., Yakir, D. (2003). Carbon sequestration arid-land forest. Glob. Change Biol. 9, 791–799. doi: 10.1046/j.1365-2486.2003.00612.x
Haggar, J., Casanoves, F., Cerda, R., Cerretelli, S., Gonzalez-Mollinedo, S., Lanza, G., et al. (2021). Shade and agronomic intensification in coffee agroforestry systems: trade-off or synergy? Front. Sustain. Food Syst. 5. doi: 10.3389/fsufs.2021.645958
Hawkins, H. J., Cargill, R. I., Van Nuland, M. E., Hagen, S. C., Field, K. J., Sheldrake, M., et al. (2023). Mycorrhizal mycelium as a global carbon pool. Curr. Biol. 33, R560–R573. doi: 10.1016/j.cub.2023.02.027
Hernández-Acosta, E., Banuelos, J., Trejo-Aguilar, D. (2021). Revisión: Distribución y efecto de los hongos micorrízicos en el agroecosistema de café. Rev. Biología Trop. 69, 445–461. doi: 10.15517/rbt.v69i2.42256
Holátko, J., Brtnický, M., Kučerík, J., Kotianová, M., Elbl, J., Kintl, A., et al. (2021). Glomalin–Truths, myths, and the future of this elusive soil glycoprotein. Soil Biol. Biochem. 153, 108116. doi: 10.1016/j.soilbio.2020.108116
Hundera, K., Aerts, R., Fontaine, A., Van Mechelen, M., Gijbels, P., Honnay, O., et al. (2013). Effects of coffee management intensity on composition, structure, and regeneration status of Ethiopian moist evergreen afromontane forests. Environ. Manag 51, 801–809. doi: 10.1007/s00267-012-9976-5
Hylander, K., Nemomissa, S., Delrue, J., Enkosa, W. (2013). Effects of coffee management on deforestation rates and forest integrity. Conserv. Biol. 27, 1031–1040. doi: 10.1111/cobi.12079
Intergovernmental Panel on Climate Change - IPCC (2003). Good practice guidance for land use, land-use change and forestry (Geneva, Switzerland: IGES).
Irving, T. B., Alptekin, B., Kleven, B., Ané., J.-M. (2021). A critical review of 25 years of glomalin research: a better mechanical understanding and robust quantification techniques are required. New Phytol. 232, 1572–1581. doi: 10.1111/nph.17713
Jing, H., Li, J., Yan, B., Wei, F., Wang, G., Liu, G. (2021). The effects of nitrogen addition on soil organic carbon decomposition and microbial C-degradation functional genes abundance in a Pinus tabulaeformis forest. For. Ecol. Manage. 489, 119098. doi: 10.1016/j.foreco.2021.119098
Kassambara, A., Mundt, F. (2022). Factoextra R package. Available online at: https://cloud.r-project.org/package=factoextra.
Koutouleas, A., Bosselmann, A. S., Rahn, E. (2024). “Is agroforestry a sustainable management system for future coffee production?,” in Advances in Botanical Research, vol. 112 . Ed. Kuete, V. (Cambridge, Massachusetts, United States: Academic Press) Early View. doi: 10.1016/bs.abr.2024.05.001
Li, X., Han, S., Luo, X., Chen, W., Huang, Q. (2020). Arbuscular mycorrhizal-like fungi and glomalin-related soil protein drive the distributions of carbon and nitrogen in a large scale. J. Soil. Sediment. 20, 963–972. doi: 10.1007/s11368-019-02421-4
Marín, C., Godoy, R., Rubio, J. (2022). “Gaps in South American mycorrhizal biodiversity and ecosystem function research,” in Mycorrhizal Fungi in South America. Eds. Lugo, M. A., Pagano, M. C. (Springer, Cham), 445–461. doi: 10.1007/978-3-031-12994-0_22
Mehnaz, K. R., Keitel, C., Dijkstra, F. A. (2018). Effects of carbon and phosphorus addition on microbial respiration, N2O emission, and gross nitrogen mineralization in a phosphorus-limited grassland soil. Biol. Fertility Soils 54, 481–493. doi: 10.1007/s00374-018-1274-9
Nascimento, H. E., Laurance, W. F. (2002). Total aboveground biomass in central Amazonian rainforests: a landscape-scale study. For. Ecol. Manage. 168, 311–321. doi: 10.1016/S0378-1127(01)00749-6
Nautiyal, P., Rajput, R., Pandey, D., Arunachalam, K., Arunachalam, A. (2019). Role of glomalin in soil carbon storage and its variation across land uses in temperate Himalayan regime. Biocatal. Agric. Biotechnol. 21, 101311. doi: 10.1016/j.bcab.2019.101311
Niguse, G., Iticha, B., Kebede, G., Chimdi, A. (2022). Contribution of coffee plants to carbon sequestration in agroforestry systems of Southwestern Ethiopia. J. Agric. Sci. 160, 440–447. doi: 10.1017/S0021859622000624
Paniagua-Ramirez, A., Krupinska, O., Jagdeo, V., Cooper, W. J. (2021). Carbon storage estimation in a secondary tropical forest at CIEE Sustainability Center, Monteverde, Costa Rica. Sci. Rep. 11, 23464. doi: 10.1038/s41598-021-03004-5
Plaza, C., Courtier-Murias, D., Fernández, J. M., Polo, A., Simpson, A. J. (2013). Physical, chemical, and biochemical mechanisms of soil organic matter stabilization under conservation tillage systems: a central role for microbes and microbial by-products in C sequestration. Soil Biol. Biochem. 57, 124–134. doi: 10.1016/j.soilbio.2012.07.026
Polo-Marcial, M. H., Solís-Ramos, L. Y., Murillo-Cruz, R., Ávila-Arias, C., Andrade-Torres, A. (2023). Mycorrhizal and endophytic richness and colonization in Cedrela odorata L., in agroforestry systems and secondary forest from southeastern Costa Rica. Agrofor. Syst. 97, 647–658. doi: 10.1007/s10457-023-00816-4
R Core Team (2023). R: A Language and Environment for Statistical Computing (Vienna, Austria: R Foundation for Statistical Computing). Available at: https://www.R-project.org/.
Roxburgh, S. H., Wood, S. W., Mackey, B. G., Woldendorp, G., Gibbons, P. (2006). Assessing the carbon sequestration potential of managed forests: a case study from temperate Australia. J. Appl. Ecol. 43, 1149–1159. doi: 10.1111/j.1365-2664.2006.01221.x
Santos, M., Cajaiba, R. L., Bastos, R., Gonzalez, D., Petrescu Bakış, A. L., Ferreira, D., et al. (2022). Why do agroforestry systems enhance biodiversity? Evidence from habitat amount hypothesis predictions. Front. Ecol. Evol. 9. doi: 10.3389/fevo.2021.630151
Schloerke, B., Cook, D., Larmarange, J., Briatte, F., Marbach, M., Thoen, E., et al. (2022). GGally: Extensión a 'ggplot2'. Available online at: https://ggobi.github.io/ggally/ (Accessed October, 2, 2023).
Schmitt-Harsh, M., Evans, T. P., Castellanos, E., Randolph, J. C. (2012). Carbon stocks in coffee agroforests and mixed dry tropical forests in the western highlands of Guatemala. Agrofor. Syst. 86, 141–157. doi: 10.1007/s10457-012-9549-x
Siarudin, M., Rahman, S. A., Artati, Y., Indrajaya, Y., Narulita, S., Ardha, M. J., et al. (2021). Carbon sequestration potential of agroforestry systems in degraded landscapes in West Java, Indonesia. Forests. 12, 714. doi: 10.3390/f12060714
Singh, A. K., Zhu, X., Chen, C., Wu, J., Yang, B., Zakari, S., et al. (2022). The role of glomalin in mitigation of multiple soil degradation problems. Crit. Rev. Environ. Sci. Technol. 52, 1604–1638. doi: 10.1080/10643389.2020.1862561
Six, J., Paustian, K. (2014). Aggregate-associated soil organic matter as an ecosystem property and a measurement tool. Soil Biol. Biochem. 68, A4–A9. doi: 10.1016/j.soilbio.2013.06.014
Solis, R., Vallejos-Torres, G., Arévalo, L., Caceres, B. (2022). Efeitos micorrízicos nos teores de proteína do solo relacionada à glomalina e de clorofila em cafeeiro na Amazônia Peruana. Pesquisa Agropecuária Tropical. 52, e72303. doi: 10.1590/1983-40632022v5272303
Solis, R., Vallejos-Torres, G., Arévalo, L., Marín-Díaz, J., Ñique-Alvarez, M., Engedal, T., et al. (2020). Carbon stocks and the use of shade trees in different coffee growing systems in the Peruvian Amazon. J. Agric. Sci. 158, 450–460. doi: 10.1017/S002185962000074X
Somarriba, E., Cerda, R., Orozco, L., Cifuentes, M., Dávila, H., Espin, T., et al. (2013). Carbon stocks and cocoa yields in agroforestry systems of Central America. Agr. Ecosyst. Environ. 173, 46–57. doi: 10.1016/j.agee.2013.04.013
Soto-Pinto, L., Anzueto, M., Mendoza, J., Ferrer, G. J., de Jong, B. (2010). Carbon sequestration through agroforestry in indigenous communities of Chiapas, Mexico. Agrofor. Syst. 78, 39–51. doi: 10.1007/s10457-009-9247-5
Tesfay, F., Moges, Y., Asfaw, Z. (2022). Woody species composition, structure, and carbon stock of coffee‐based agroforestry system along an elevation gradient in the moist mid‐highlands of Southern Ethiopia. Int. J. For. Res. 1, 4729336. doi: 10.1155/2022/4729336
Tschora, H., Cherubini, F. (2020). Co-benefits and trade-offs of agroforestry for climate change mitigation and other sustainability goals in West Africa. Glob. Ecol. Conserv. 22, e00919. doi: 10.1016/j.gecco.2020.e00919
Vallejos-Torres, G., Tenorio-Cercado, M., Gaona-Jimenez, N., Corazon-Guivin, M., Ormeño-Luna, J., Paredes, C., et al. (2022). Multiplicación masiva de hongos micorrícicos arbusculares aislados de suelos cultivados con cacao. Bioagro. 34, 265–276. doi: 10.51372/bioagro343.6
Walkley, A., Black, C. A. (1934). An examination of the Degtajareff’s method for determining soil organic matter and a proposed modification of the chromic acid titration method. Soil Sci. 37, 29–38. doi: 10.1097/00010694-193401000-00003
Wang, W., Zhong, Z., Wang, Q., Wang, H., Fu, Y., He, X. (2017). Glomalin contributed more to carbon, nutrients in deeper soils, and differently associated with climates and soil properties in vertical profiles. Sci. Re. 7, 13003. doi: 10.1038/s41598-017-12731-7
Wang, Y. J., He, X. H., Meng, L. L., Zou, Y. N., Wu, Q. S. (2023). Extraradical mycorrhizal hyphae promote soil carbon sequestration through difficultly extractable glomalin-related soil protein in response to soil water stress. Microb. Ecol. 86, 1023–1034. doi: 10.1007/s00248-022-02153-y
Keywords: agroforestry, carbon stocks, Peruvian Amazon, secondary forests, shade trees
Citation: Vallejos-Torres G, Gaona-Jimenez N, Pichis-García R, Ordoñez L, García-Gonzales P, Quinteros A, Lozano A, Saavedra-Ramírez J, Tuesta-Hidalgo JC, Reategui K, Macedo-Córdova W, Baselly-Villanueva JR and Marín C (2024) Carbon reserves in coffee agroforestry in the Peruvian Amazon. Front. Plant Sci. 15:1410418. doi: 10.3389/fpls.2024.1410418
Received: 01 April 2024; Accepted: 12 November 2024;
Published: 12 December 2024.
Edited by:
Ana I. F. Ribeiro-Barros, University of Lisbon, PortugalReviewed by:
Goetz Schroth, Universidade Federal do Oeste do Pará, BrazilAthina Koutouleas, The University of Copenhagen, Denmark
Copyright © 2024 Vallejos-Torres, Gaona-Jimenez, Pichis-García, Ordoñez, García-Gonzales, Quinteros, Lozano, Saavedra-Ramírez, Tuesta-Hidalgo, Reategui, Macedo-Córdova, Baselly-Villanueva and Marín. This is an open-access article distributed under the terms of the Creative Commons Attribution License (CC BY). The use, distribution or reproduction in other forums is permitted, provided the original author(s) and the copyright owner(s) are credited and that the original publication in this journal is cited, in accordance with accepted academic practice. No use, distribution or reproduction is permitted which does not comply with these terms.
*Correspondence: Geomar Vallejos-Torres, Z3ZhbGxlam9zQHVuc20uZWR1LnBl