- Shandong Key Laboratory of Eco-Environmental Science for the Yellow River Delta, Shandong University of Aeronautics, Binzhou, China
Human activities have increased nitrogen (N) and phosphorus (P) inputs to the Yellow River Delta and the supply level of N and P affects plant growth as well as ecosystem structure and function directly. However, the root growth, stoichiometry, and antioxidant system of plants in response to N and P additions, especially for herbaceous halophyte in the Yellow River Delta (YRD), remain unknown. A field experiment with N addition (0, 5, 15, and 45 g N m-2 yr-1, respectively) as the main plot, and P addition (0 and 1 g N m-2 yr-1, respectively) as the subplot, was carried out with a split-plot design to investigate the effects on the root morphology, stoichiometry, and antioxidant system of Suaeda salsa. The results showed that N addition significantly increased the above-ground and root biomass as well as shoot-root ratio of S. salsa, which had a significant interaction with P addition. The highest biomass was found in the treatment with 45 g N m-2 yr-1 combined with P addition. N addition significantly increased TN content and decreased C:N ratio of root, while P addition significantly increased TP content and decreased C:P ratio. The main root length (MRL), total root length (TRL), specific root length (SRL), and root tissue density (RTD) of S. salsa root were significantly affected by N addition and P addition, as well as their interaction. The treatments with or without P addition at the 45 g N m-2 yr-1 of N addition significantly increased the superoxide dismutase (SOD), peroxidase (POD), catalase (CAT) activities and soluble protein content of roots, decreased malondialdehyde (MDA) content. And there was a significant interaction between the N and P addition on SOD activity. Therefore, N and P additions could improve the growth of S. salsa by altering the root morphology, increasing the root nutrient content, and stimulating antioxidant system.
1 Introduction
Increased nitrogen (N) deposition caused by human production and activity has been an important factor that can impact the growth and development of plants, thus changing the structure and function of entire ecosystem (Liu et al., 2020). Although there may be positive, negative or neutral effects of such alterations (Xiao et al., 2022), it is widely accepted that N enrichment can increase the aboveground net primary productivity in terrestrial ecosystems, farmland, grasslands, and wetlands (Guan et al., 2019). The improvement of N availability can also increase soil phosphorus (P) availability and plant P concentration, through the stimulation of soil P activity by N. In addition, in some areas, anthropogenic changes in P availability are also increasing, especially in ecosystems receiving sediment input (Lü et al., 2015). The availability of soil N and P directly affects plant N and P concentrations and stoichiometric ratios (Li et al., 2019; Chen et al., 2020). In terrestrial ecosystems, N and P are two major elements that often restrict plant metabolic reaction and growth, and the N:P stoichiometry of plant reflects the biochemical limitations in biological activities such as protein and ribosomal RNA (Lü et al., 2015). Therefore, it is critical to understand how N and P enrichment affect the growth of plants independently and interactively, thus affecting the ecosystem function (Zhan et al., 2017).
The root system is a key organ for land plants to fix in soil and absorb water and mineral nutrients effectively (Sun et al., 2022), and also plays a critical role in the carbon (C) and N cycling (Li et al., 2015). The root structure describes the shape and spatial layout of the root system in the soil. The spatial distribution and morphological characteristics of plant roots have a certain degree of plasticity under the influence of habitat changes and genetic factors (Benlloch-González et al., 2017). Special root structures can be formed to enhance the absorption capacity of nutrients and water, and enhance the survival ability of plants (Li et al., 2020). Atmospheric N deposition can significantly affect the plant root system by changing soil available N and the absorption and transportation of N through leaves (Meng et al., 2021). The chemical, morphological, and physiological characteristics of fine root are sensitive to N application, and the increased soil N availability can also alter the C:N ratio, structure, and activity of root, thus affecting the belowground biomass of plants (Li et al., 2015). In addition, the increase in soil N availability can lead to limitation of other nutrients, such as P (Liu et al., 2020). Increased input of P also could affect the plant root system by influencing root architecture (diameter and length, etc.), biomass, and C cycle functions such as root respiration (Liu et al., 2021; Qian et al., 2023). However, the response of root morphological trait is a complex process. N and P were coupled and influenced by each other, which is important to regulate nutrient limitation and strategies for nutrient uptake in the environment (Zhang et al., 2018). Therefore, exploring the response of plant roots to N and P nutrients is necessary to understand the overall response of underground ecosystem processes to environmental changes.
The Yellow River Delta (YRD), located at the junction of land, river and sea, is a coastal wetland with unique ecosystem and important ecological functions (Zhao et al., 2020). Severe soil salinization ultimately leads to soil ecological degradation and vegetation habitat destruction in the YRD. The typical vegetation in the YRD is dominated by halophytes, which play an important role in soil desalination and restoration (Zhao et al., 2022). S. salsa is an annual true halophyte that grows in saline soil and is an ideal species for salt tolerance research (Wang et al., 2021). As a pioneer plant, S. salsa shows strong tolerance to environmental stresses such as high salinity and flooding, because of its special structure and ion transport system (Wu et al., 2012; Guo et al., 2019). S. salsa also plays an important role in improving soil quality, reducing coastal erosion, regulating climate and improving habitat quality in the YRD (Chen & Sun, 2020; Wang et al., 2021). It has been found that the N deposition has been as high as 22.64 kg hm-2 in the growing season in the YRD, which is almost one of the highest N deposition areas in China (Guan et al., 2019). In recent years, the effects of N deposition on coastal wetland ecosystems has received increasing attention, mainly involving studies on the growth and ecological characteristics of plants, soil physicochemical properties, soil microbial community diversity, etc (Guan et al., 2019; Lu et al., 2021). However, the effects of exogenous N enrichment on the root growth of regional halophyte remain unknown. It is necessary to study of the effects of N and P addition on the growth and antioxidant system of halophyte root in coastal wetland of the YRD in order to the comprehend the response of coastal wetland ecosystem functions to human activities.
In this paper, the field in situ N and P addition experiment of the YRD was conducted and the effects of exogenous nutrient enrichment on variation of S. salsa root were studied. The objectives of the study were: 1) dose N and P addition have an impact on the root growth characteristics of S. salsa? 2) how do nutrient contents of root respond to different levels of N and P addition? 3) how do N and P affect antioxidant enzyme activities of the S. salsa root system in a saline wetland ecosystem? The results could provide insight into the response of wetland plants to environmental stresses and give valuable implications for wetland vegetation restoration in the Yellow River Delta.
2 Materials and methods
2.1 Study site
The experimental site was located in the Yellow River Delta of Dongying City, Shandong province, China (N 37°55’26’’, E 118°34’37’’), which belongs to the warm temperate monsoon continental semi-humid climate zone. The mean annual temperature is 12.1°C, the mean annual precipitation is 550 mm, which 70% of rainfall concentrated between July and September (Figure 1), and the annual evaporation capacity is about 1900–2400 mm in the experimental area (Lu et al., 2021). The typical vegetation is mainly Phragmites australis, S. salsa, Tamarix chinensis, and Aeluropus sinensis, which mostly growing in sandy and argillaceous soils with poor nutrient conditions. The soil characteristics of the top 20 cm soil in the study sites include a pH of 7.83, electrical conductance (EC) of 5.38 ms·cm-1, organic matter (OM) 16.2 g kg-1, total N (TN) 0.32 g kg-1, total P (TP) 0.64 g kg-1, total potassium (TK) 0.64 g kg-1, and soil salt content 16.2 g kg-1.
2.2 Experimental design
The experiment was conducted in 2021 with a randomized block design (2 m × 2 m plots which were separated by 2 m buffers to prevent the interference caused by fertilizer movement among the experimental plots). The study was a multifactorial experiment considering N and P addition levels as two nutrient factors, including four levels of N fertilization (N0, N5, N15, and N45 was 0, 5, 15, and 45 g N m-2 yr-1, respectively) and two levels of P fertilization (P0 and P1 was 0 and 1 g P m-2 yr-1, respectively). The experiment consisted of eight treatments: N0P0, N5P0, N15P0, N45P0, N0P1, N5P1, N15P1, and N45P1, with three replicate plots. A total of 24 plots were conducted and the determination of the N and P application rates was based on previous study in the YRD (Guan et al., 2019; Li et al., 2019).
N fertilizer in the form of urea (N 46%) and P fertilizer in the form of KH2PO4 (Li et al., 2019), were dissolved in 4 L deionized water and sprayed uniformly to each plot using a sprayer (Wang et al., 2019). The whole treatments were applied once a month from May 2021 to August 2021 and the control treatment (N0P0) was sprayed with equal amounts of deionized water. The cumulative value of 4 months reached the processing value.
2.3 Sample preparation and measurements
We randomly sampled 10 live complete plants at an area of 0.5 m × 0.5 m within each plot in late August. The whole plants were collected by using the full excavation method to maintain the integrity of root system during the process (Sun et al., 2022). All the samples were placed in a cold storage box (4°C) and taken to the laboratory immediately for the further analysis.
The roots were washed with tap water carefully to remove the attached soil and rinsed thoroughly with distilled water again. Then the aboveground and belowground (root) parts were separated and one part of them were oven-dried at 105°C for 2 hour and then 75°C for 48 h to a constant weight (Li et al., 2021), and the other part were stored in a refrigerator at 4°C for the detection of antioxidant system. The aboveground biomass and root biomass were measured, and the root-shoot ratio = root biomass/aboveground biomass × 100%. Furthermore, the root samples were scanned with an Epson digital scanner and analyzed with a WinRHIZO professional root analysis system. The main root length (MRL), total root length (TRL), surface area, volume, and average diameter (AD) were measured. And the measurements were used to calculated: Specific root length (SRL, cm g-1) = root length/dry weight, specific root surface area (SRA, cm2 g-1) = surface area/dry weight, and root tissue density (RTD, g cm-3) = dry weight/volume were calculated (Zhu et al., 2021).
The oven-dried root samples were grounded with a ball mill and passed through a 0.1 mm sieve for the element analysis (Zhu et al., 2021; Xiao et al., 2022). The total carbon (TC) and TN concentrations of root were analyzed using an elemental analyzer (vario MACRO CUBE, Elementar, German). TP concentration of root samples was determined with the molybdate/ascorbic acid method after the wet digestion with H2SO4-H2O2 (Zhan et al., 2017; Zhang et al., 2018). The ratios of C:N, C:P, N:P were calculated with previous studies (Li et al., 2017b).
Three soil samples were randomly collected using a 5 cm-diameter soil drill at 0–20 cm depths from each plot, and combined as a single composite sample on the same day. All the fresh soil samples were taken back to the laboratory and extracted with 2 mol L-1 KCl, then the solution was determined using a follow injection autoanalyzer (AA3, SEAL, USA) for measuring ammonium (NH4+-N) and nitrate (NO3−-N) contents (Zhan et al., 2017). Then the samples were air-dried, ground, and sieved through a 2 mm mesh and a 0.25 mm mesh, respectively, for the determination of the following indicators. Soil pH was determined with a 1:5 (soil: water, w/v) solution by a digital pH meter (FE22-Standard, Mettler Toledo, USA). Soil salt content was measured by the weight loss of 1:5 (soil: water, w/v) extract solution after oven-drying to a constant weight at 105°C (Guan et al., 2019). Soil organic matter (OM) was measured by the potassium dichromate-sulfuric acid oxidation method (Liu et al., 2020). Soil available P (AP) was extracted with the 0.5 mol L-1 NaHCO3 and the leaching solution was measured ammonium molybdate method (Li et al., 2017b).
For antioxidant system, 0.5 g frozen root samples per replication were crushed and homogenized using a 50 mM potassium phosphate buffer (pH 7.0), containing 1% polyvinyl pyrrolidone and 1 mmol L-1 EDTA-Na2. The homogenate was centrifuged at 12,000×g for 20 min at 4°C, and then the supernatant solution was used in following assays (Aghaleh et al., 2011). Superoxide dismutase (SOD) activity was determined by the reaction of nitroblue tetrazolium using the riboflavin system under the illumination as described by Guo JM (Guo et al., 2021). Peroxidase (POD) activity was measured in the reaction mixture containing 50 mmol phosphate buffer (pH 7.0), 19 μl H2O2, 28 μl guaiacol, and 100 μl of enzyme extract at 430 nm (Zhu et al., 2020). Catalase (CAT) activity was determined by monitoring the consumption of H2O2 from the reaction mixture at 240 nm for 3 min (Ibrahim et al., 2020). Malondialdehyde (MDA) content was determined by the thiobarbituric acid reaction as described by (Azizi et al., 2022). The mixture was heated in a water bath at 98°C for 20 min, then cooled and centrifuged at 4000 × g for 20 min. The supernatant was measured at 600, 532, and 450 nm. Soluble protein (SP) concentration was determined by the Coomassie Brilliant Blue G-250 dye-binding method (Ma et al., 2019).
2.4 Data analysis
One-way ANOVA with the LSD test was used to analyze the differences in biomass, root-shoot ratios, root growth indicators (MRL, TRL, AD, SRL, SRA, and TD), nutrient concentrations (TC, TN, TP, and C:N:P ratios), antioxidant enzyme (SOD, POD, CAT) activities, MDA and SP content of S. salsa root, and soil properties and fertility (pH, salt content, TC, TN, TP). Two-way ANOVA with Duncan tests was used to examine the effects of N, P and their interactions on the indicators. The data analyses were conducted with SAS 9.2 (2010, SAS Institute Cary, NC) and were expressed as the mean ± standard error (n=3). Difference was scores as significant at the P<0.05 levels. The figures were generated using SigmaPlot 12.0, and the Pearson correlation and principal component analysis (PCA) were performed using R software (4.1.3) by Corr plot (0.92) package and prcomp function.
3 Results
3.1 Effects of different treatments on the biomass of S. salsa
There were significant effects of N, P and their interaction on above-ground biomass, below-ground biomass and root-shoot rations (Figure 2). N15 and N45 treatments significantly increased above-ground biomass and root biomass than N0 treatment by 52.0~67.7% and 151.5~177.9%, respectively, in the absences of P. In comparison to the other treatments, N45 treatment resulted in 55.4~128.7% and 63.5~279.2% higher above-ground biomass and below-ground biomass, respectively, when P was added. When the N addition was 0~15 g N m-2 yr-1, there was no effect on above-ground biomass with or without P addition. However, above-ground biomass and root biomass in N45P1 treatment were increased significantly than that in N45P0 treatment. The N1P1 treatment resulted in 94.2% and 125.0% higher root-shoot ratio than N0P0 and N0P1 treatments, respectively. When N addition was 0, 15, and 45 g N m-2 yr-1, there was no significant difference in root-shoot ratio between P0 and P1 treatments.
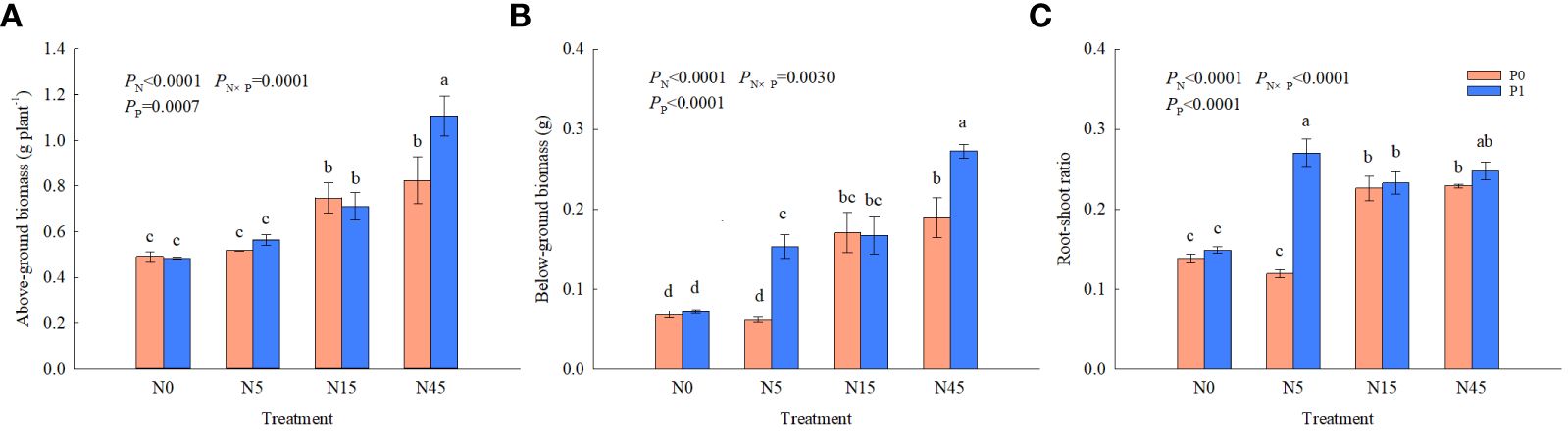
Figure 2 Biomass (A, B) and root-shoot ratios (C) of S. salsa under different treatments. Different lowercase letters show significant differences (P<0.05). The same as below.
3.2 Effects of different treatments on the root morphology of S. salsa
The MRL, TRL, AD, SRL, and RTD of S. salsa root were significantly affected by N addition and P addition, as well as their interaction except AD of root (Table 1). MRL was increased significantly with the increasing N addition whether P was added or not. N45 treatment resulted in the highest TRL with or without P addition. When the N addition was 5 and 45 g N m-2 yr-1, the P addition significantly increased MRL, TRL, AD than the treatment without P addition.
The SRL and SRA in N5 treatment were increased significantly than other treatments in the absence of P. There was no significant difference of SRL among the N5P1, N15P1 and N45P1 treatments. SRA with P addition was increased significantly than that without P addition when the N addition was 15 and 45 g N m-2 yr-1. N5P1 significantly increased RTD by 434.1%, 70.7%, and 142.8%, respectively, compared with N5P0, N0P1, and N15P1 treatment.
3.3 Effects of different treatments on the ecostoichiometry of S. salsa root
N addition significantly affected TN, C:N and N:P ratio of S. salsa root and P addition significantly affected TC, TP, C:P, and N:P ratio. While it was not significantly affected by N and P interaction on the nutrient contents and C:N:P ratios (Figure 3). N45P1 treatment increased TC content by 45.5% and 14.1% compared with N15P1 and N45P0 treatments, respectively. No significant difference was found in TC content among the N treatments without the P addition. The TN content was increased with increasing N addition rate with or without P addition. Under the same N addition, there was no significant effect on TN content and C:N ratio between P1 and P0 treatment. The treatment with P addition increased significantly TP content of root compared with no P addition. C:N ratio was decreased significantly with increasing N addition rate and P addition treatment significantly reduced C:P ratio under the same N addition. However, no significant differences were found in TP content and C:P ratio among the different N treatments. The highest N:P ratio was obtained from the N3P1 treatment, which was higher 32.2~274.0% than all the other treatments.
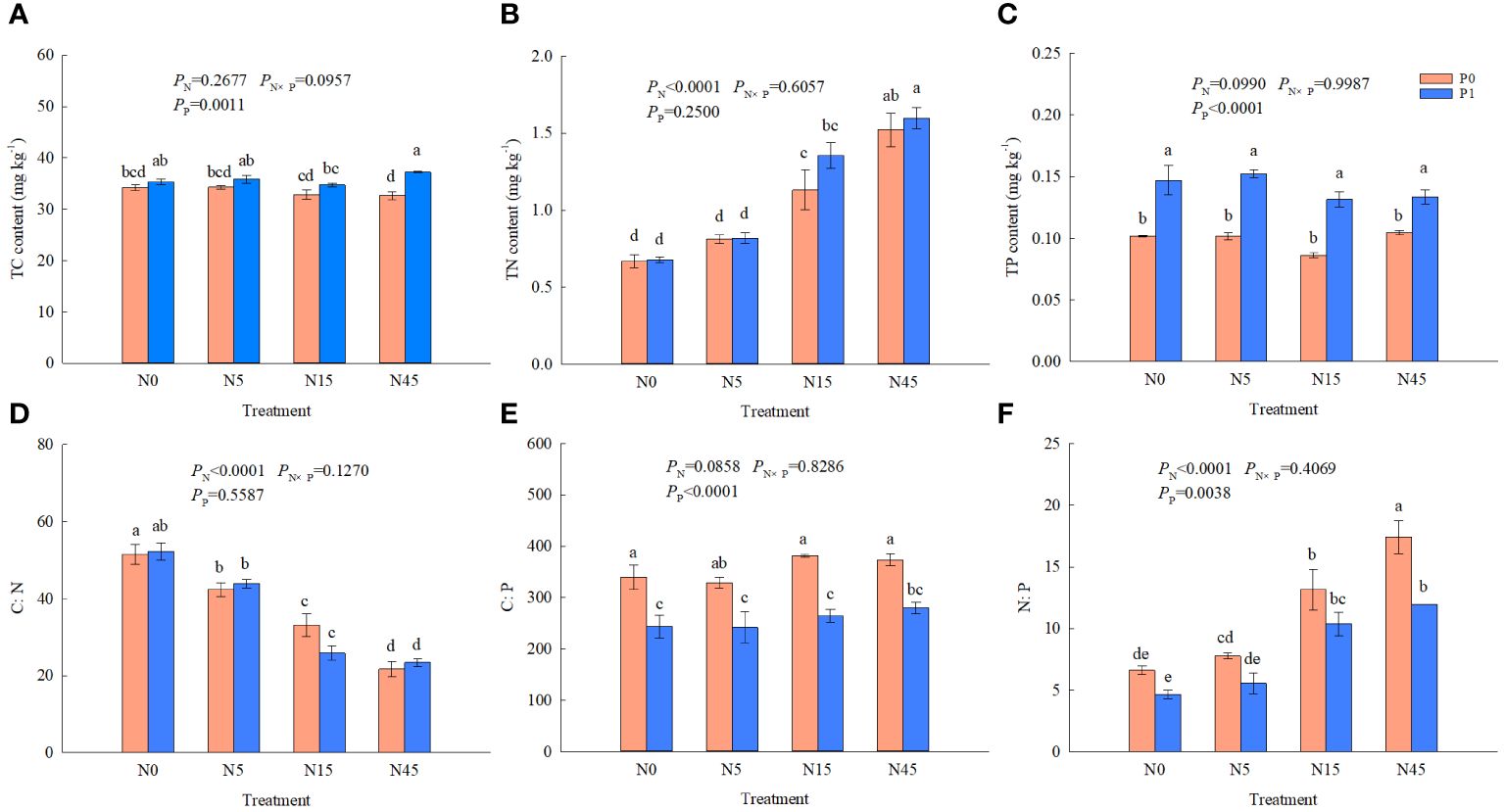
Figure 3 TC (A), TN (B), TP (C) concentrations and C:N (D), C:P (E), N:P (F) ratios of S. salsa root under different treatments. (P<0.05). Different lowercase letters in the same column show significant differences (P<0.05).
3.4 Effects of different treatments on the root antioxidant system of S. salsa
There were significant effects of N addition on SOD, POD, CAT activities and P addition on POD activities, whereas the interaction of N and P addition only significantly affected SOD activities (Figure 4). N addition significantly improved SOD activities compared with N0 treatment with or without P addition. The highest POD activity was obtained from the N45P1 treatment, which was higher 52.5% and 115.7% than N45P0 and N0P1, respectively. There was no significant difference between P1 and P0 treatment when the N addition was 0~5 g N m-2 yr-1. The N addition treatments improved CAT activities significantly compared with N0 treatment when no P addition, whereas no significant difference was found between P0 and P1 treatments with the same N addition.
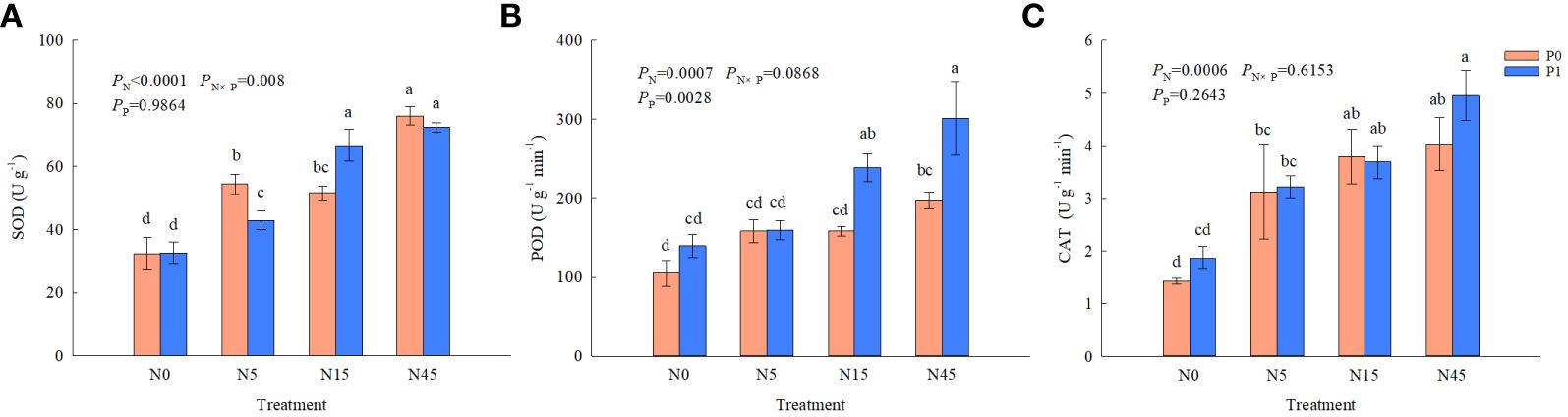
Figure 4 Antioxidant enzyme (A–C) activities of S. salsa root under different treatments. (P<0.05). Different lowercase letters in the same column show significant differences (P<0.05).
The MDA contents of S. salsa root were significantly affected by N addition (Figure 5). Whether P is added or not, N5, N15 and N45 treatments decreased the MDA content significantly compared with N0 treatment. However, the MDA content was not significantly affected by P addition. There were significant effects of N addition, and the interaction of N and P on the soluble protein of S. salsa root. N addition (N5, N15, and N45 treatments) significantly increased the soluble protein by 52.3~70.6% than N0 treatment with P addition. The SP contents in the treatments with P addition were increased significantly than that without P addition when the addition was 5~45 g N m-2 yr-1.
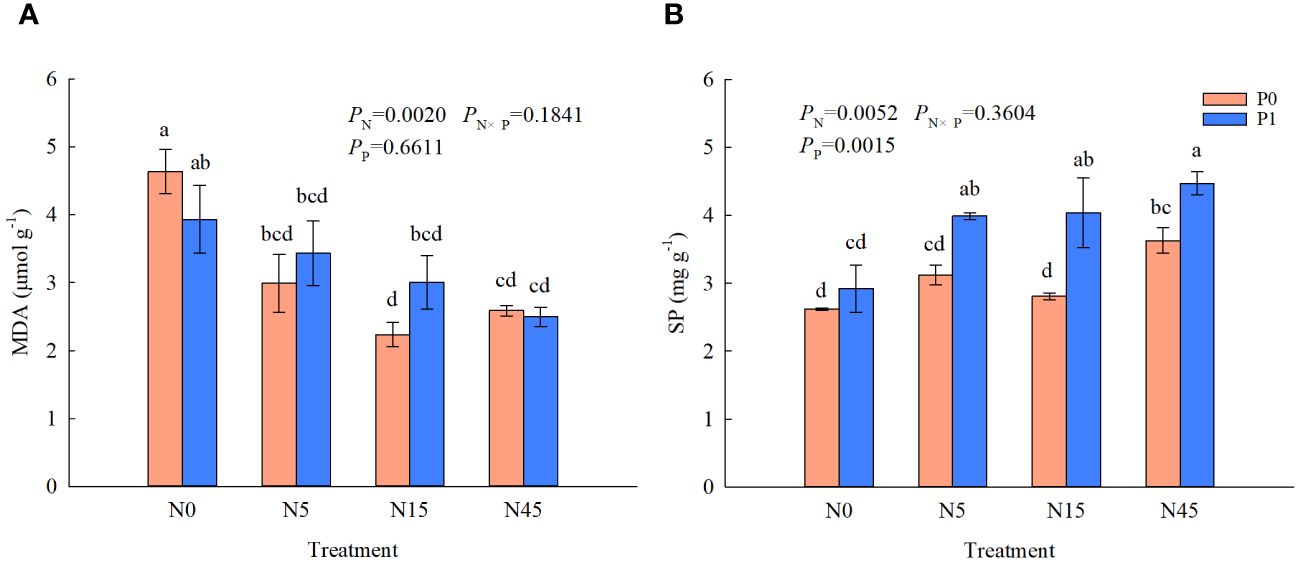
Figure 5 MDA (A) and SP (B) content of S. salsa root under different treatments. (P<0.05). Different lowercase letters in the same column show significant differences (P<0.05).
3.5 Effects of different treatments on soil properties and fertility
There were significant effects of N addition on soil salt, NH4+-N, and NO3–N content, whereas the interaction of N and P addition only significantly affected soil organic carbon (Table 2). The soil salt contents in N45P0 and N45P1 treatments were decreased by 29.6% and 26.1% than that in N0P0 treatment, respectively. Compared with N0P0 treatment, N5P0, N15P0 and N45P0 treatments significantly increased NH4+-N content by 46.9~94.4% and NO3–N content by 59.1~169.36%, respectively. N15P1 and N45P1 treatments significantly increased NH4+-N content than N0P1 treatment by 49.4~70.2%, NO3–N content by 70.1~203.8%. There was no significant effect on soil NH4+-N and NO3–N content with or without phosphorus addition at the same N addition level. Soil AP content was significantly affected by P addition. The N0P1, N15P1, and N45P1 treatments significantly increased AP content by 55.8~72.2% compared with N0P0 treatment. All the treatments showed no significant difference in soil pH and OM content.
3.6 Relationship among soil property, root morphology, nutrient content, and antioxidant system of S. salsa root
Significant correlations were found among growth characteristics, nutrient concentration, antioxidant system of S. salsa root and soil property and fertility (Figure 6). The TN of root was positively correlated with soil NH4-N and NO3-N content, MRL, TRL and AD of root (P<0.01), SRA (P<0.05), and negatively correlated with soil salt content, RTD (P<0.01), SRL (P<0.05). The TP content of root was positively correlated with soil AP content, root TC content (P<0.01), and negatively correlated with soil NH4-N content (P<0.05). SOD, POD, and CAT activities were positively correlated with soil NH4-N, NO3-N content, MRL, TRL, AD and TN content of root (P<0.01). However, the correlation of MDA content was opposite to that of SOD, POD, and CAT activity. SP content was positively correlated with soil NO3-N and AP content, root TC content (P<0.05), MRL, TRL, AD, root TN content (P<0.01), and negatively correlated with SRL and MDA content (P<0.05).
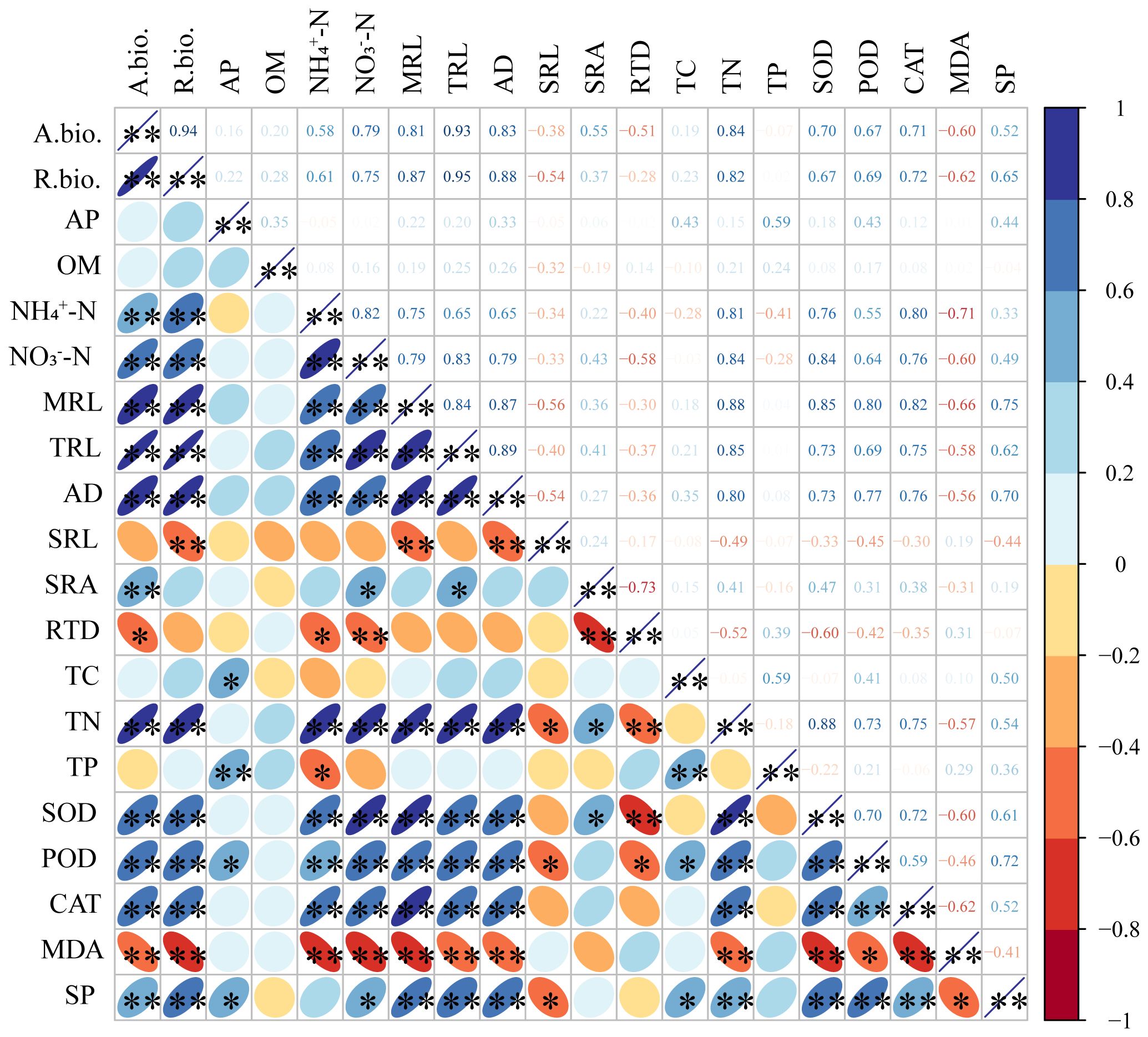
Figure 6 Correlation analysis of the S. salsa root system indexes and soil property. *, P ≤ 0.05; **, P ≤ 0.01; A.bio., aboveground biomass; R.bio., root biomass; OM, organic matter; AP, available phosphorus; NH4+-N, ammonium; NO3−-N, nitrate; MRL, main root length; TRL, total root length; AD, average diameter; SRL, specific root length; SRA, specific root surface area; RTD, root tissue density; TC, total carbon; TN, total nitrogen; TP, total phosphorus; SOD, superoxide dismutase; POD, peroxidase; CAT, catalase; MDA, malondialdehyde; SP, soluble protein.
The PCA revealed that the two major PC components together explained 69.74% of the data variability, which could reflect the expression information of the 14 indicators well (Figure 7). TRL, MRL, AD, TN and SP content, and antioxidant enzyme activity of S. salsa root had positive loads in the PC1, which explain 52.55% of the data variability. The negative load of TP, TC content and RTD and the positive load of SRA and SRL were all found in the PC2, which explained 17.19% of the observed variability. The N45P1, N45P0, and N15P1 treated groups were clearly separated from N0P0, N0P1 treated groups along PC1. N15P0 and N5P0 treated groups were separated from N5P1 treated group along PC2.
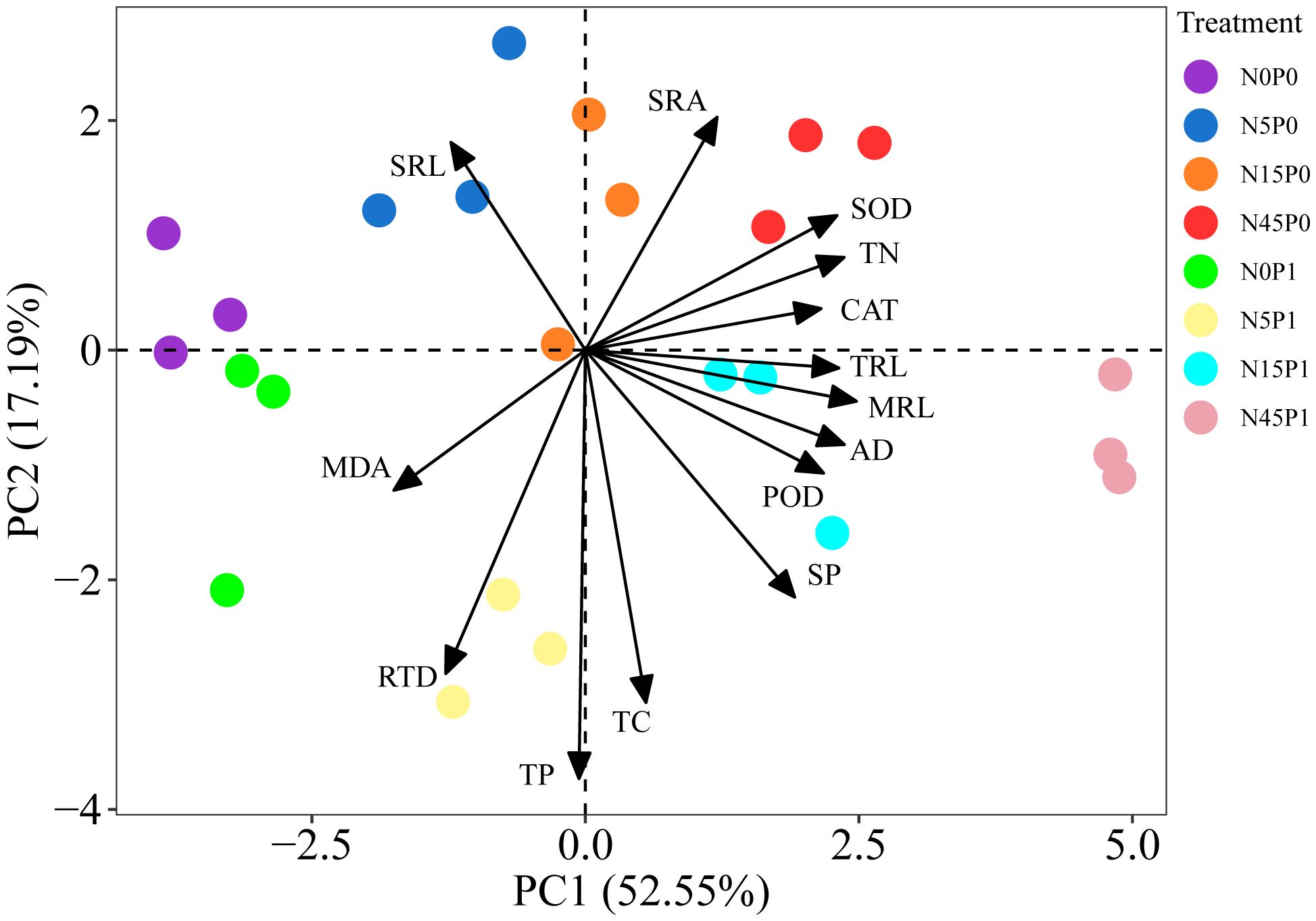
Figure 7 PCA of nutrient content, root morphology, and antioxidant system of S. salsa root. MRL, main root length; TRL, total root length; AD, average diameter; SRL, specific root length; SRA, specific root surface area; RTD, root tissue density; TC, total carbon; TN, total nitrogen; TP, total phosphorus; SOD, superoxide dismutase; POD, peroxidase; CAT, catalase; MDA, malondialdehyde; SP, soluble protein.
4 Discussion
4.1 Effects of N and P addition on the biomass and root characteristics of S. salsa
The allocation of biomass is the result of long-term adaptation of plants to the natural environment, which could maximize the use of environmental conditions (Wang et al., 2023). Plant biomass allocation strategies will change in response to the environmental conditions (Sugiura et al., 2016). The meta-analysis of Li et al. (2015) showed that simulated N deposition significantly increased total root biomass by 20.2%. In this experiment, N addition of 15–45 g N m-2 yr-1 significantly increased aboveground and root biomass of S. salsa. There was no significant difference in aboveground biomass with phosphorus application at the N addition of 0–15 g N m-2 yr-1, while P application significantly increased aboveground and belowground biomass of the plant at 45 g N m-2 yr-1 addition. This suggested that N application stimulates plant growth and increases its demand for phosphorus (Li et al., 2016). Root-shoot ratio is an important parameter in the study of the spatial distribution of plant biomass and carbon stocks, which exhibits a high degree of plasticity to adapt to the external environment in order to optimize the allocation of resources (Sun et al., 2022). N addition (15–45 g N m-2 yr-1) was found to significantly increase the root-shoot ratio of S. salsa in our study. Allocating more biomass to underground part could maintain a higher root-shoot ratio, increasing more nutrients and water to the plant and reducing the transpiration surface area (Sugiura et al., 2016). And the high biomass allocation of below-ground organs enables greater functioning of the below-ground root system (Sainju et al., 2017). We also observed that the interaction of N and P significantly increased biomass and root-shoot ratio. The findings suggested that continued changes in human-caused inputs of N and P will significantly alter the dynamics of terrestrial biomass.
Root morphological traits are highly plastic in response to environmental changes, and the root changes are related to individual nutrient requirements and nutrient stress (Qian et al., 2023). The results of ANOVA showed that the root morphology of S. salsa was not only affected by single N or P addition, but also by the interaction of N and P addition. MRL and TRL of S. salsa were significantly increased by nitrogen addition, which suggested that increasing the root length of herbaceous plants can improve the efficiency of N uptake in soils under N application conditions (Wang et al., 2023). P application was able to significantly promote its growth with N addition of 5–45 g N m-2 yr-1, while the effect of P application without N addition was not significant. This showed that the addition of N leads to extensive P restriction (Gonzales & Yanai, 2019). SRL, SRA and RTD are relevant physiological properties of the root system, reflecting nutrient acquisition by the root system and plant growth strategies (Ahmadi et al., 2018). N0P1 treatment had higher SRL and RTD than N45P1 treatment, suggesting that plants could acquire more nutrients by altering root morphology under nitrogen deficiency. In addition, plants can optimize C inputs by controlling the AD of roots, thereby increasing SRL, which is consistent with our findings. Variation in the root morphology between the increasing N input effects on S. salsa perhaps because that root-surface phosphomonoesterase activities was stimulated by N addition, which enhances P conservation and accelerates P cycling rates (Li et al., 2019). Secondly, the input of N and P may alter the root traits by increasing plant nutrient absorption and nitrates and phosphates are both signaling molecules that stimulate root branching (Schleuss et al., 2020). Root morphology not only determines the spatial distribution characteristics of the root system, but also has an important impact on the nutrient uptake capacity of the root system as well as its immobilization, which can reflect the feeding strategy of the root system under different habitat conditions (Li et al., 2017a).
4.2 Effects of N and P addition on the root ecostoichiometry of S. salsa
C, N, and P contents of plant organs are important indicators of the plant nutritional status, which could reflect the adaptation of plants to environmental condition (Zhao et al., 2022). In our study, short-term N addition (15–45 g N m-2 yr-1) increased the TN content of S. salsa roots, which was positively correlated with above-ground and root biomass of the plant (P<0.01). However, P additions had no significant effect on TN of the roots at the same nitrogen levels. Previous studies have shown that the appropriate N addition promotes N accumulation and C fixation and then increase the plant life activities, resulting in the accumulation of more nutrients (Liu et al., 2020). We found that P addition significantly increased the TP content of the S. salsa root, while N addition had no significant effect on TC and TP of the root. This may be due to the short duration of N and P additions and the fact that roots are more insensitive to changes in NP content compared to leaves of plant (Zhan et al., 2017). Combined addition of N and P, the organic P storage, P recycling, and plant P absorption increased with the level of N addition. The addition of N stimulates the absorption of P by plants when the inorganic phosphorus is sufficient, and leads to the depletion of soil dissolved inorganic P (Schleuss et al., 2020). Plants can adjust their nutrient uptake strategies according to the amount of N and P of the environment, which affects their photosynthetic and carbon partitioning capacity, leading to changes in nutrient content and ratios among their root systems (Li et al., 2021).
The stoichiometric ratios of plant tissues can reflect the uptake and utilization of elements by plants to some extent, varying with their growth status and environmental conditions (Liu et al., 2020). The study showed that short-term N and P additions had significant effects on the C:N:P stoichiometric characteristics of S. salsa root. N addition significantly reduced the C:N ratio and increased N:P in the root system, which is consistent with the study by Lei Li (Li et al., 2019). Without P addition, N:P ratio of root ranged from 6.6 to 17.4 with increasing N level, suggesting that root growth was significantly N-limited without N addition, but gradually shifted to P-limited with the N addition. Plants can utilize N to up-regulate P uptake and transport systems. Because the dissolved inorganic P content in plant cells is higher than that in soil and plants use proton-ATPase to transport phosphate across the plasma membrane. The addition of N can increase the activity of anion/H+ co-transport pumps, as NH4+ requires the release of H+ (Schleuss et al., 2020). P is a limiting nutrient element for plant growth in the YRD, and the reason for P limitation in halophytes is mainly related to the effectiveness of soil P. The soil in the region, subjected to seawater erosion, is mainly by the calcium adsorbed form of P, which is more stable and hard to be absorbed by plants (Zhao et al., 2022). P addition could be effective in alleviating the P limitation caused by the increased N.
4.3 Effects of N and P addition on the root antioxidant system of S. salsa
Soil salinization in the YRD has increased due to insufficient groundwater replenishment, continuous seawater intrusion and high evapotranspiration (Guan et al., 2019; Zhao et al., 2020). Massive increase of reactive oxygen species (ROS) in plants causes severe damage to the organism under salt stress (Azizi et al., 2022). The plant reduces the damage produced by ROS through increasing the activity of antioxidant enzymes, such as SOD, POD, and CAT et al (Zhu et al., 2020). The results of our study showed that N addition significantly increased SOD, POD and CAT enzyme activities of S. salsa root compared to no N addition and there was a significant positive interaction on SOD activity between N and P addition. POD and CAT can effectively reduce H2O2 decomposed from O2- by SOD with increasing salinity (Aghaleh et al., 2011). P addition significantly increased POD activity, while had no significant impact on CAT activity, at the N addition of 15–45 g N m-2 yr-1. The enzymes act synergistically to defend against cell membrane damage caused by ROS, inhibit membrane lipid peroxidation, and mitigate the damage caused by salt stress, thus keeping the production and removal of reactive oxygen species in a balanced state (Li et al., 2020; Qian et al., 2023). MDA, as a product of cellular membrane lipid peroxidation, reflects the strength of membrane lipid peroxidation and the degree of membrane lipid damage (Qian et al., 2023). MDA content was significantly reduced with N addition, which could be related to the increase of antioxidant enzyme activity. N addition significantly increased root SP content, and P addition significantly increased SP content under the same N addition level. Correlation analysis revealed that root TN content was positive correlated with root antioxidant enzymes and SP content (P<0.01), and negative correlated with MDA content (P<0.01). N and P both are the main elements involved in protein synthesis in the process of plant growth, so N and P addition could effectively improve the activity of antioxidant enzymes of S. salsa, enhancing the survival ability.
5 Conclusion
N and P addition significantly increased aboveground, belowground biomass, and root-shoot ratio of S. salsa and there was a significant positive interaction between N and P among them. The root morphology (MRL, TRL, AD, SRL, and RTD) of S. salsa varied with the level of N and P addition which promoted the nutrient absorption. N addition significantly affected TN, C:N and N:P ratio and P addition significantly affected TC, TP, C:P, and N:P ratio of S. salsa root. N treatment effectively increased antioxidant enzyme activities and SP content of root, while decreased MDA content. When the N addition was 15–45 g N m-2 yr-1, P addition significantly improved POD activity and SP content. This study suggests that moderate amount of N and P addition could effectively increase the nutrient content and antioxidant enzyme activities of the root system, promote its root development, and then increase its biomass. The results could provide a theoretical support for the response of wetland plants to the environment and vegetation restoration.
Data availability statement
The original contributions presented in the study are included in the article/supplementary material. Further inquiries can be directed to the corresponding author.
Author contributions
JM: Writing – original draft. XX: Writing – original draft, Resources, Methodology, Investigation. YC: Writing – original draft, Resources, Investigation. LZ: Writing – original draft, Resources, Investigation. ZZ: Writing – original draft, Resources, Investigation. DZ: Writing – review & editing. ZF: Writing – review & editing. JS: Writing – review & editing.
Funding
The author(s) declare financial support was received for the research, authorship, and/or publication of this article. The study was supported by the National Key Research and Development Program of China (2022YFF1303200), the National Natural Science Foundation ofChina (No. 42171059; 41871089); and the Doctoral Fund Projectof Binzhou University (No. 2019Y10).
Conflict of interest
The authors declare that the research was conducted in the absence of any commercial or financial relationships that could be construed as a potential conflict of interest.
Publisher’s note
All claims expressed in this article are solely those of the authors and do not necessarily represent those of their affiliated organizations, or those of the publisher, the editors and the reviewers. Any product that may be evaluated in this article, or claim that may be made by its manufacturer, is not guaranteed or endorsed by the publisher.
References
Aghaleh, M., Niknam, V., Ebrahimzadeh, H., Razavi, K. (2011). Effect of salt stress on physiological and antioxidative responses in two species of Salicornia (S. persica and S. europaea). Acta Physiol. Plant 33, 1261–1270. doi: 10.1007/s11738–010-0656-x
Ahmadi, S. H., Sepaskhah, A. R., Zarei, M. (2018). Specific root length, soil water status, and grain yields of irrigated and rainfed winter barley in the raised bed and flat planting systems. Agric. Water Manage. 210, 304–315. doi: 10.1016/j.agwat.2018.08.031
Azizi, F., Amiri, H., Ismaili, A. (2022). Melatonin improves salinity stress tolerance of Phaseolus vulgaris L. cv. Pak by changing antioxidant enzymes and photosynthetic parameters. Acta Physiol. Plant 44, 40–51. doi: 10.1007/s11738–022-03373-y
Benlloch-González, M., Sánchez-Lucas, R., Benlloch, M. (2017). Effects of olive root warming on potassium transport and plant growth. J. Plant Physiol. 218, 182–188. doi: 10.1016/j.jplph.2017.07.018
Chen, B., Sun, Z. (2020). Effects of nitrogen enrichment on variations of sulfur in plant-soil system of Suaeda salsa in coastal marsh of the Yellow River estuary, China. Ecol. Indic. 109, 105797. doi: 10.1016/j.ecolind.2019.105797
Chen, J., Groenigen, K. J., Hungate, B. A., Terrer, C., van Groenigen, J.-W., Maestre, F. T., et al. (2020). Long-term nitrogen loading alleviates phosphorus limitation in terrestrial ecosystems. Global Change Biol. 26, 5077–5086. doi: 10.1111/gcb.15218
Gonzales, K., Yanai, R. (2019). Nitrogen-phosphorous interactions in young northern hardwoods indicate P limitation: foliar concentrations and resorption in a factorial N by P addition experiment. Oecologia 189, 829–840. doi: 10.1007/s00442–019-04350-y
Guan, B., Xie, B., Yang, S., Hou, A., Chen, M., Han, G. (2019). Effects of five years’ nitrogen deposition on soil properties and plant growth in a salinized reed wetland of the Yellow River Delta. Ecol. Eng. 136, 160–166. doi: 10.1016/j.ecoleng.2019.06.016
Guo, J., Chen, Y., Lu, P., Liu, M., Sun, P., Zhang, Z. (2021). Roles of endophytic bacteria in Suaeda salsa grown in coastal wetlands: Plant growth characteristics and salt tolerance mechanisms. Environ. pollut. 287, 117641. doi: 10.1016/j.envpol.2021.117641
Guo, S., Tan, Y., Chu, H., Sun, M., Xing, J. (2019). Transcriptome sequencing revealed molecular mechanisms underlying tolerance of Suaeda salsa to saline stress. PloS One 14, e0219979. doi: 10.1371/journal.pone.0219979
Ibrahim, M. E. H., Ali, A. Y. A., Elsiddig, A. M. I., Zhou, G., Nimir, N. E. A., Ahmad, I., et al. (2020). Biochar improved sorghum germination and seedling growth under salinity stress. Agron. J. 112, 911–920. doi: 10.1002/agj2.20026
Li, L., Gao, X., Gui, D., Liu, B., Zhang, B., Li, X. (2017b). Stoichiometry in aboveground and fine roots of Seriphidium korovinii in desert grassland in response to artificial nitrogen addition. J. Plant Res. 130, 689–697. doi: 10.1007/s10265–017-0930–8
Li, W., Jin, C., Guan, D., Wang, Q., Wang, A., Yuan, F., et al. (2015). The effects of simulated nitrogen deposition on plant root traits: A meta-analysis. Soil Biol. Biochem. 82, 112–118. doi: 10.1016/j.soilbio.2015.01.001
Li, Q., Liang, J., Zhang, X., Feng, J., Song, M., Gao, J. (2021). Biochar addition affects root morphology and nitrogen uptake capacity in common reed (Phragmites australis). Sci. Total Environ. 766, 144381. doi: 10.1016/j.scitotenv.2020.144381
Li, L., Liu, B., Gao, X., Li, X., Li, C. (2019). Nitrogen and phosphorus addition differentially affect plant ecological stoichiometry in desert grassland. Sci. Rep. 9, 18673. doi: 10.1038/s41598–019-55275–8
Li, Y., Niu, S., Yu, G. (2016). Aggravated phosphorus limitation on biomass production under increasing nitrogen loading: a meta-analysis. Global Change Biol. 22, 934–943. doi: 10.1111/gcb.13125
Li, S., Wan, L., Nie, Z., Li, X. (2020). Fractal and topological analyses and antioxidant defense systems of alfalfa (Medicago sativa L.) root system under drought and rehydration regimes. Agronomy 10, 805. doi: 10.3390/agronomy10060805
Li, F., Xie, Y., Yang, G., Zhu, L., Hu, C., Chen, X., et al. (2017a). Interactive influence of water level, sediment heterogeneity, and plant density on the growth performance and root characteristics of Carex brevicuspis. Limnologica 62, 111–117. doi: 10.1016/j.limno.2016.11.007
Liu, J., Liu, W., Long, X.-E., Chen, Y., Huang, T., Huo, J., et al. (2020). Effects of nitrogen addition on C:N:P stoichiometry in moss crust-soil continuum in the N-limited Gurbantünggüt Desert, Northwest China. Eur. J. Soil Biol. 98, 103174. doi: 10.1016/j.ejsobi.2020.103174
Liu, X., Zhao, J., Liu, J., Lu, W., Ma, C., Gu, X., et al. (2021). Water–phosphorus coupling enhances fine root turnover and dry matter yield of alfalfa under drip irrigation. Agron. J. 113, 4161–4175. doi: 10.1002/agj2.20782
Lü, X., Reed, S. C., Yu, Q., Han, X. (2015). Nutrient resorption helps drive intra-specific coupling of foliar nitrogen and phosphorus under nutrient-enriched conditions. Plant Soil 398, 111–120. doi: 10.1007/s11104–015-2642-y
Lu, G., Xie, B., Cagle, G. A., Wang, X., Han, G., Wang, X., et al. (2021). Effects of simulated nitrogen deposition on soil microbial community diversity in coastal wetland of the Yellow River Delta. Sci. Total Environ. 757, 143825. doi: 10.1016/j.scitotenv.2020.143825
Ma, J., Zhang, M., Liu, Z., Chen, H., Li, Y. C., Sun, Y., et al. (2019). Effects of foliar application of the mixture of copper and chelated iron on the yield, quality, photosynthesis, and microelement concentration of table grape (Vitis vinifera L.). Sci. Hortic. 254, 106–115. doi: 10.1016/j.scienta.2019.04.075
Meng, B., Li, J., Maurer, G. E., Zhong, S., Yao, Y., Yang, X., et al. (2021). Nitrogen addition amplifies the nonlinear drought response of grassland productivity to extended growing-season droughts. Ecology 102, e03483. doi: 10.1002/ecy.3483
Qian, J., Liu, Y., Wang, P., Hu, J., Lu, B., Tang, S., et al. (2023). Responses of riparian plant roots to acute combined nitrogen and phosphorus stress: changes in root morphology and antioxidant system. Aquat. Ecol. 57, 715–732 doi: 10.1007/s10452–023-10041-x
Sainju, U. M., Allen, B. L., Lenssen, A. W., Ghimire, R. P. (2017). Root biomass, root/shoot ratio, and soil water content under perennial grasses with different nitrogen rates. Field Crops Res. 210, 183–191. doi: 10.1016/j.fcr.2017.05.029
Schleuss, P. M., Widdig, M., Heintz-Buschart, A., Kirkman, K., Spohn, M. (2020). Interactions of nitrogen and phosphorus cycling promote P acquisition and explain synergistic plant-growth responses. Ecology 101, e03003. doi: 10.1002/ecy.3003
Sugiura, D., Kojima, M., Sakakibara, H. (2016). Phytohormonal regulation of biomass allocation and morphological and physiological traits of leaves in response to environmental changes in polygonum cuspidatum. Front. Plant Sci. 7. doi: 10.3389/fpls.2016.01189
Sun, J., Zhao, X., Fang, Y., Xu, W., Gao, F., Zhao, W., et al. (2022). Root growth and architecture of Tamarix chinensis in response to the groundwater level in the Yellow River Delta. Mar. pollut. Bull. 179, 113717. doi: 10.1016/j.marpolbul.2022.113717
Wang, J., Gao, Y., Zhang, Y., Yang, J., Smith, M. D., Knapp, A. K., et al. (2019). Asymmetry in above- and belowground productivity responses to N addition in a semi-arid temperate steppe. Global Change Biol. 25, 2958–2969. doi: 10.1111/gcb.14719
Wang, Q., Liu, G., Qu, P., Yan, G., Xing, Y. (2023). Long-term nitrogen addition and drought altered root trait and plant biomass allocation of five herbs in early spring in a temperate forest. Scand. J. For. Res. 38, 232–243. doi: 10.1080/02827581.2023.2216021
Wang, S., Zhao, Z., Ge, S., Peng, B., Zhang, K., Hu, M., et al. (2021). Root morphology and rhizosphere characteristics are related to salt tolerance of Suaeda salsa and Beta vulgaris L. Front. Plant Sci. 12. doi: 10.3389/fpls.2021.677767
Wu, H., Liu, X., Zhao, J., Yu, J., Pang, Q., Feng, J. (2012). Toxicological effects of environmentally relevant lead and zinc in halophyte Suaeda salsa by NMR-based metabolomics. Ecotoxicology 21, 2363–2371. doi: 10.1007/s10646–012-0992–2
Xiao, J., Dong, S., Shen, H., Li, S., Zhi, Y., Mu, Z., et al. (2022). Phosphorus addition promotes Nitrogen retention in alpine grassland plants while increasing N deposition. Catena 210, 105887. doi: 10.1016/j.catena.2021.105887
Zhan, S., Wang, Y., Zhu, Z., Li, W., Bai, Y. (2017). Nitrogen enrichment alters plant N: P stoichiometry and intensifies phosphorus limitation in a steppe ecosystem. Environ. Exp. Bot. 134, 21–32. doi: 10.1016/j.envexpbot.2016.10.014
Zhang, J., Yan, X., Su, F., Li, Z., Wang, Y., Wei, Y., et al. (2018). Long-term N and P additions alter the scaling of plant nitrogen to phosphorus in a Tibetan alpine meadow. Sci. Total Environ. 625, 440–448. doi: 10.1016/j.scitotenv.2017.12.292
Zhao, Q., Bai, J., Gao, Y., Zhao, H., Zhang, G., Cui, B. (2020). Shifts in the soil bacterial community along a salinity gradient in the Yellow River Delta. Land Degrad. Dev. 31, 2255–2267. doi: 10.1002/ldr.3594
Zhao, Y., Li, T., Liu, J., Sun, J., Zhang, P. (2022). Ecological stoichiometry, salt ions and homeostasis characteristics of different types of halophytes and soils. Front. Plant Sci. 13. doi: 10.3389/fpls.2022.990246
Zhu, G., Lu, H., Shi, X., Wang, Y., Zhi, W., Chen, X., et al. (2020). Nitrogen management enhanced plant growth, antioxidant ability, and grain yield of rice under salinity stress. Agron. J. 112, 550–563. doi: 10.1002/agj2.20013
Keywords: nitrogen and phosphorus addition, Suaeda salsa, root morphology, stoichiometry, antioxidant enzyme
Citation: Ma J, Xin X, Cao Y, Zhao L, Zhang Z, Zhang D, Fu Z and Sun J (2024) Root growth characteristics and antioxidant system of Suaeda salsa in response to the short-term nitrogen and phosphorus addition in the Yellow River Delta. Front. Plant Sci. 15:1410036. doi: 10.3389/fpls.2024.1410036
Received: 31 March 2024; Accepted: 22 May 2024;
Published: 06 June 2024.
Edited by:
Patricio Ramos, Universidad de Talca, ChileReviewed by:
Zhongsheng Zhang, Chinese Academy of Sciences (CAS), ChinaFeng Li, Chinese Academy of Sciences (CAS), China
Copyright © 2024 Ma, Xin, Cao, Zhao, Zhang, Zhang, Fu and Sun. This is an open-access article distributed under the terms of the Creative Commons Attribution License (CC BY). The use, distribution or reproduction in other forums is permitted, provided the original author(s) and the copyright owner(s) are credited and that the original publication in this journal is cited, in accordance with accepted academic practice. No use, distribution or reproduction is permitted which does not comply with these terms.
*Correspondence: Jingkuan Sun, c3VuamluZ2t1YW5AMTI2LmNvbQ==