- 1Key Laboratory of Soil Environment and Nutrient Resources in Shanxi Province, Shanxi Agricultural University, Taiyuan, China
- 2State Key Laboratory of Herbage Improvement and Grassland Agro-ecosystems, College of Ecology, Lanzhou University, Lanzhou, China
- 3College of Biology and Agricultural Resources, Huanggang Normal University, Huanggang, China
Alfalfa (Medicago sativa L.) grassland is prone to degradation following multi-year maintenance. Yet, its mechanism regarding the stoichiometry of carbon (C) and nitrogen (N) across plant–soil system is still unclear. To address this issue, the method of space-for-time sampling was employed to investigate alfalfa grasslands with five planting years (5-, 8-, 10-, 15-, and 20-year periods) in the semiarid Loess Plateau. The results showed that the alfalfa above- and underground biomass decreased steadily decrease after the fifth to eighth years, showing a degradation tendency with the extension of planting duration. The mean weight diameter of aggregate registered an increase with planting years. However, the C and N stocks decreased with planting years in five soil aggregate fractions. Specifically, they were the highest in the fifth year and then started to gradually decrease along the 8th, 10th, 15th, and 20th year. Redundancy and correlation analysis confirmed that the C and N stocks of soil aggregates were closely positively associated with those of plant. Overall, the highest stability of soil physical structure was found during the period from the fifth to eighth year, and, afterward, the stability declined. In conclusion, alfalfa plantation improved soil structure stability but aggravated soil C and N stocks, and biomass and soil aggregate indicators accounted for alfalfa field degradation after a certain year of plantation.
Highlights
● There exists a steady degradation with planting years in perennial alfalfa field.
● Field productivity and soil aggregate quality declined after 5- to 8-year planting.
● C and N stocks in aggregate fractions were the highest at the fifth year and then declined.
● C and N stocks of soil aggregates were positively linked with that of alfalfa (p < 0.05).
● Steady deterioration of C and N stocks from soil to plant explains system degradation.
1 Introduction
The Loess Plateau is one of the most seriously degraded soil regions, which is located in northern China (Li et al., 2021; Yuan et al., 2024). Soil degradation is related to the climatic, environmental, and geological features of this region (Gong et al., 2020); it is also strongly tied to unreasonable land-use management (Fan et al., 2016). To restrain soil degradation, alfalfa (Medicago sativa L.) was extensively planted until the 1960s in the Loess Plateau due to the characteristics of the nutrient-rich forage crop with high biological yield potential, high resistance ability, and wide adaptability in extreme environments (Zhang X. L. et al., 2021; Song et al., 2024). Alfalfa has the potential to enhance nitrogen (N) input into soil ecosystems while promoting soil carbon (C) and N accumulation (Vitousek et al., 2013). However, alfalfa forage biomass is gradually reduced with the duration of planting years, and continuous planting for many years will degrade the alfalfa biomass and soil quality (Gu et al., 2018; Fang et al., 2021; Qi et al., 2023). Therefore, it is essential to study the optimum cultivation period and the degradation mechanism of alfalfa grasslands.
Ecological stoichiometry was used to explore the degradation mechanism for plant responses to environmental change (Austin and Vitousek, 2012). The ecological stoichiometry of C and N across ecosystem components can provide a new strategy for elucidating the nutrient cycle process (Du and Gao, 2021; Tang et al., 2022). Carbon is an essential energy source for above- and underground ecosystem biogeochemical processes (Wang et al., 2021b), whereas N is an essential plant nutrient as a key limiting factor determining primary production in ecosystems (Wang et al., 2021a). The C and N stoichiometry of plants and their interaction with the soil significantly affect ecosystem functions (Yang et al., 2019; Xu et al., 2024). Soil C and N availability affects plant nutrient absorption and assimilation (Zhang et al., 2019); In turn, plant litter and root exudates will provide substrates to enhance soil C and N cycling processes (Zhou et al., 2019; Dan et al., 2023). Overall, the variation in C and N cycles in ecosystems can change the stoichiometry of plants and soils (Wang et al., 2021a). C and N stoichiometric flexibility might affect terrestrial ecosystem biogeochemical cycling, a changing the ecosystem productivity and terrestrial degradation (Sardans et al., 2017; Li et al., 2023). Therefore, it is necessary to determine the C and N stoichiometry in plants and soil to explore the degradation mechanism of perennial alfalfa grasslands.
Soil aggregates are composed of granular or small clumped structures, and soil aggregate stability is a key index for characterizing soil degradation (Zhu et al., 2018; Tan et al., 2024). Soil aggregates are affected by many factors, such as the soil physical and chemical properties, soil microbes, plant root distribution, and artificial cultivation (Bodner et al., 2014; Shen et al., 2024). Based on soil size, aggregates are classified into three parts: macroaggregates (>0.25 mm), microaggregates (0.053–0.25 mm), and the silt-clay fraction (<0.053 mm) (Lu et al., 2021). The soil aggregate size distribution often influences soil aeration, corrosion resistance, and water permeability (Bissonnais and Arrouays, 1997). Soil aggregation is a process driven the biotic and abiotic factors that plant-derived organic matter input, microorganisms, and soil conditions combination (Liu et al., 2023). Increasing plant cover to prevent the surface erosion and to protect soil physical disturbance promotes the plant-derived organic matter input and contributes soil aggregation formation (Wang et al., 2022). In addition, more plant residue input also promotes the microbial grower, regulate microbial activity, and microbial-derived C and ultimately affects the formation of soil aggregate (Zhao et al., 2024). Higher C concentrations and mineralization rates are often found to be associated with macroaggregates (Wang et al., 2018; Araujo et al., 2024). This is because macroaggregates are easily disintegrated and broken by external interference, whereas microaggregates may be more physically protected and are, therefore, more biochemically recalcitrant to C mineralization (Kubar et al., 2021). Previous studies have investigated the soil aggregates in various land-use types, including forests, cultivated lands, grazing lands, and woodlands. In fact, most of above investigations on soil aggregates are mainly aimed at the stability and C and N characteristics (Wang et al., 2018; Bhatt et al., 2023), and few studies have focused on the relationship between alfalfa aggregates and plant C and N stoichiometry. Therefore, knowledge of C and N stoichiometry is crucial for understanding the biochemical mechanisms of plant and soil aggregates.
Plant–soil interactions via both positive and negative feedbacks can constrain or promote plants development in the novel environment, and plant–soil interaction is strongly influenced by the rhizosphere microbiota (Lustenhouwer et al., 2024). Plant–soil interaction plays an important role in global C and N biogeochemical cycles, and previous study found that rhizobacteria symbiotic with legumes can drive positive effect, and promote nutrient uptake and plant growth (Yahui et al., 2023). However, there is still controversy about how multi-year alfalfa planting affects the C and N in soil aggregates and plant organs in the semiarid regions. We hypothesized that the dynamics of C and N sequestration might be key indicators to explain soil degradation following continuous alfalfa plantation. To clarify this issue, the method of space for time was employed to investigate 5-year succession of alfalfa grasslands in the semiarid region. The purposes of this study were 1) to determine the changes in the production of alfalfa with planting years, 2) to identify the stocks dynamics of C and N in soil aggregate distribution with planting years, 3) to reveal the changes C and N across plant–soil system in the multi-year alfalfa grassland, and 4) to explore the degradation mechanisms of perennial alfalfa. The findings will help formulate an appropriate management strategy for ecological restoration and sustainable soil development.
2 Materials and methods
2.1 Description of the study site
The study area is located in Yuzhong County, Gansu Province, China (36°02′N, 104°25′E, 2,400 m) (Figure 1). The average annual temperature is 6.5°C, and the monthly average maximum and minimum temperatures are 19.0°C and −8.0°C, respectively. The mean annual precipitation from 2000 to 2018 was 323.5 mm, the maximum year was 2018 (458.8 mm), and the minimum precipitation occurred in 2004 (202.0 mm). The average annual water evaporation is approximately 1,450 mm. The local soil type is Calcic Kastanozems (Siltic) or rusty dark loess soil with a pH of 8.2 (Guo et al., 2010). The rainfall data were derived from the perennial positioning automatic meteorological recording instrument (Figure 1).
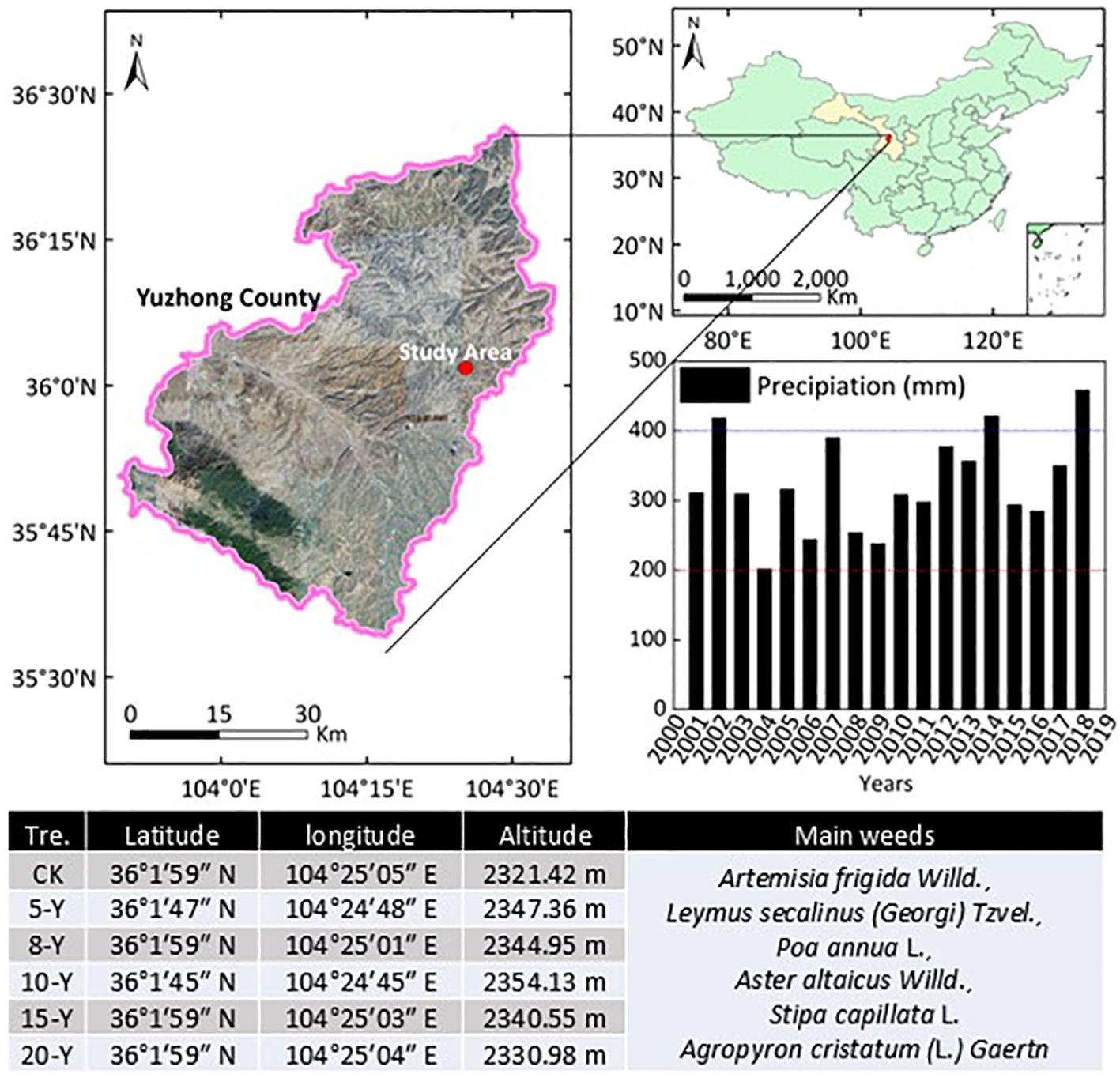
Figure 1. Location of study area and its precipitation and biodiversity background. CK, abandoned farmland. 5-Y, 8-Y, 10-Y, 15-Y, and 20-Y planting indicate the variations across 5, 8, 10, 15, and 20 years, respectively.
2.2 Experimental design
We selected five alfalfa grasslands in October 2018 and formed a time series of 5, 8, 10, 15, and 20 years, which represented successional series with similar topographic conditions. Where fields planted with alfalfa were recorded in 2013, 2010, 2008, 2003, and 1998, respectively. Abandoned farmland was chosen as the control group (CK; abandoning 15 years). The aspect and slope of each selected alfalfa field remained relatively consistent (Figure 1). In all the selected plots, the alfalfa variety Algonquin was planted, each plot was sown with alfalfa seeds of 35 kg ha−1, the depth was approximately 2 cm, and each row was almost 20 cm. There was no irrigation throughout the growing season. Fertilization was only applied in the first year of planting (N, 185.6 kg ha−1; P, 48 kg ha−1), and no fertilization was conducted afterward. There was no grazing treatment for the alfalfa grassland, which was harvested in June and October of each year (weeds were also mowed at the same time). The control group had no fertilization and mowing treatment. The only difference in the selected alfalfa grassland was in planting years. In the alfalfa grassland, weed biomass increased with prolonged plantation years. The dominant vegetation species are Artemisia frigida Willd., Leymus secalinus (Georgi) Tzvel., Poa annua L., Aster altaicus Willd., Stipa capillata L., and Agropyron cristatum L. Gaertn. The weeds were periodically cleared by hand when needed to avoid interspecific disturbance.
2.3 Sampling
In October 2018, 15 plots of 1 m × 1 m of each field were randomly selected and marked to measure the biomass of each field. Soil samples of 0-cm to 20-cm depth were randomly collected from the field using a soil auger (diameter of 5 cm) with five replicates for each planting period, and each alfalfa field was mixed in three samples. Before air-drying, the soil was sieved through 2.0-mm screens for available nutrient analyses, and, after drying, it was sieved through 0.15-mm screens for total nutrient analyses. The soil aggregate sample was collected with a hard Polyvinyl Chloride (PVC) pipe with a diameter of 10 cm and a height of 20 cm. After sampling, the aggregate sample was divided into small clods a diameter of 1 cm for air-drying.
2.4 Physical and chemical analysis
Soil bulk density (SBD) was determined using cutting ring (0–20 cm). Soil water storage (SWC) was measured gravimetrically (0–20 cm, 20–40 cm, 40–60 cm, 60–80 cm, and 80–100 cm). The soil aggregate-size fraction was measured using a dry- and wet-sieving method (Su et al., 2009). For the dry sieving method, we used 100 g of air-dried soil samples in a stainless-steel vibrating sieve divided into five sizes: >2 mm, 2–1 mm, 1–0.5 mm, 0.5–0.25 mm, and <0.25 mm. The wet sieving method used 50 g of air-dried soil, which was weighed according to dry sieving and moistened for 10 min with distilled water. The apparatus specifications were an oscillation of 10 min at a frequency of 30 cycle min−1. Aggregates retained in the sieve were air dried at 65°C, weighed, and stored for C and N content measurements. The soil aggregate distribution is calculated with Equation 1. The mean weight diameter (MWD) and percentage of aggregate destruction (PAD) are calculated using Equations 2 and 3, respectively.
where AD is the aggregate distribution of each fraction (%), ADi is the i size aggregate weight (g), and TO is the total weight of the soil (g). Xi is the mean diameter of the aggregate, and ADd and ADw are the proportions of dry and water stable aggregates (>0.25 mm), respectively.
The SOC content and C of aggregate fractions are determined by Puget et al. (2000). The soil total nitrogen (TN) content and TN of the aggregate fractions were measured by Bremner (1997). The above- and underground biomass of alfalfa and weeds (soil depth up to 40 cm) were collected from a 1 m × 1 m area of each plot (collected twice in late June and early October). The above- and underground biomass were dried at 105°C for 30 min and then at 65°C to constant weight (Wilson et al., 2009). The C and N contents of alfalfa plants were measured following Yu et al. (2021); aggregate C and N stocks were measured according to Equation 4 (Zhang L. Q. et al., 2015).
where C and N stocks are stocks of soil carbon and nitrogen [Mg ha−1; where M is soil quality per unit area (Mg ha−1)], SOCi and TNi are different size aggregates of soil carbon and nitrogen content (g kg−1), SBD is the soil bulk density (g cm−3), and H is 20 cm.
2.5 Statistical analysis
All statistical data were tested for normality and homogeneity of variance before further analysis. One-way ANOVA was used to compare the differences among the six treatments. The difference was tested by Tukey’s honestly significant difference (HSD) to check the statistical significance of the treatments between different planting years. Mean comparisons were performed using the least significant difference at a probability level of 0.05. Redundancy analysis (RDA) and correlation analysis were used to evaluate the relationship between aggregate C and N stocks, plant biomass, and the C and N concentrations of alfalfa plants. RDA was plotted using Canoco 5.0. Graphs were prepared using ArcGis 10.0 and Origin 2021.
3 Results
3.1 Dynamics of aboveground and belowground biomass with planting years
Above- and underground biomass varied significantly among alfalfa cultivation years (Table 1). Throughout various planting for alfalfa, a significant change in above- and underground biomass was occurred. In the aboveground biomass, alfalfa yields reached the highest after approximately 5 years, whereas those of the remaining years declined approximately 20 years after alfalfa planting. Conversely, underground biomass was at a maximum value after alfalfa planting for 8 years but then decreased with the duration years. Therefore, the above- and underground biomass of alfalfa grassland declined with the number of years of planting.
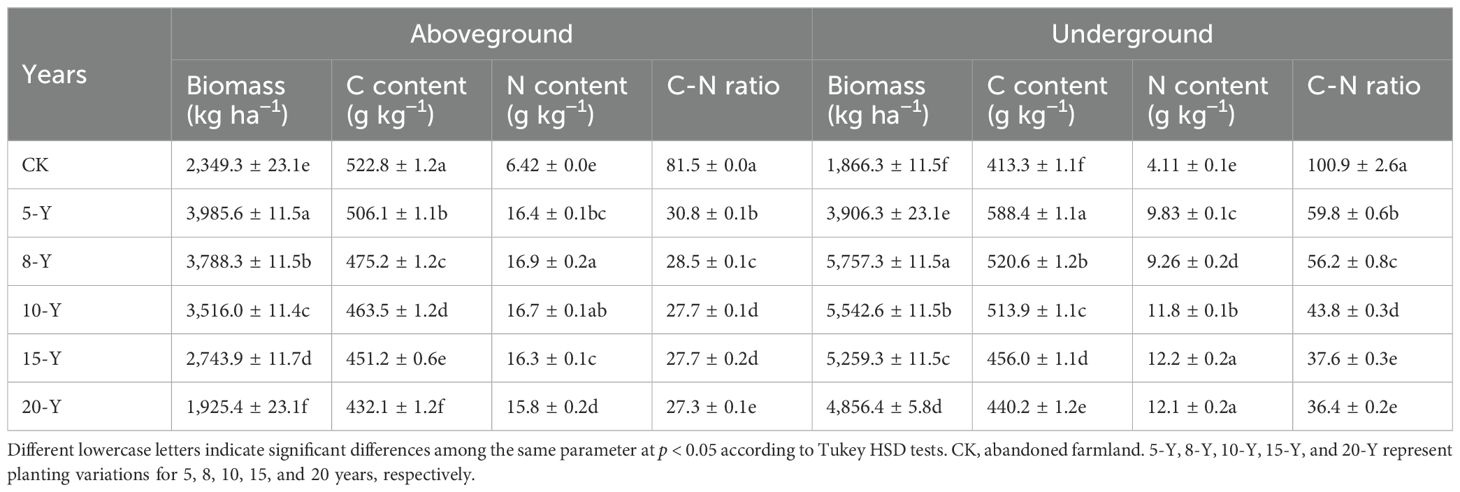
Table 1. Variations of above- and belowground biomass and the C and N stocks in alfalfa along varying planting durations.
3.2 Dynamics of the organic carbon and total nitrogen concentrations of plants with planting years
The C and N contents and C-N ratio of alfalfa varied with planting years (Table 1). The C contents of alfalfa above- and underground decreased after continuous planting 5 years. The N content of the aboveground also showed significant change; however, the N content of underground significantly increased with planting years. Similarly, the C-N ratio of alfalfa above- and underground also declined under cultivated 5 years.
3.3 The responses of soil water content and soil bulk density to planting years
As the depth of the soil layer increases, the SWC of the 0-cm to 100-cm soil layer decreases significantly, and the soil moisture content of alfalfa grassland is significantly lower than that of the control (Figure 2A). At different planting times, the SWC was the highest after 10 years of planting and the lowest after 8 years of planting. The SBD of alfalfa grassland ranged from 1.04 g cm−3 to 1.28 g cm−3, and there was a significantly variable trend in 5 years, and there is less change after cultivated 8 to 20 years (Figure 2B). The change in SBD after 8, 15, and 20 years of planting was significantly higher than that after 5 years of planting, followed by 10 years of planting.
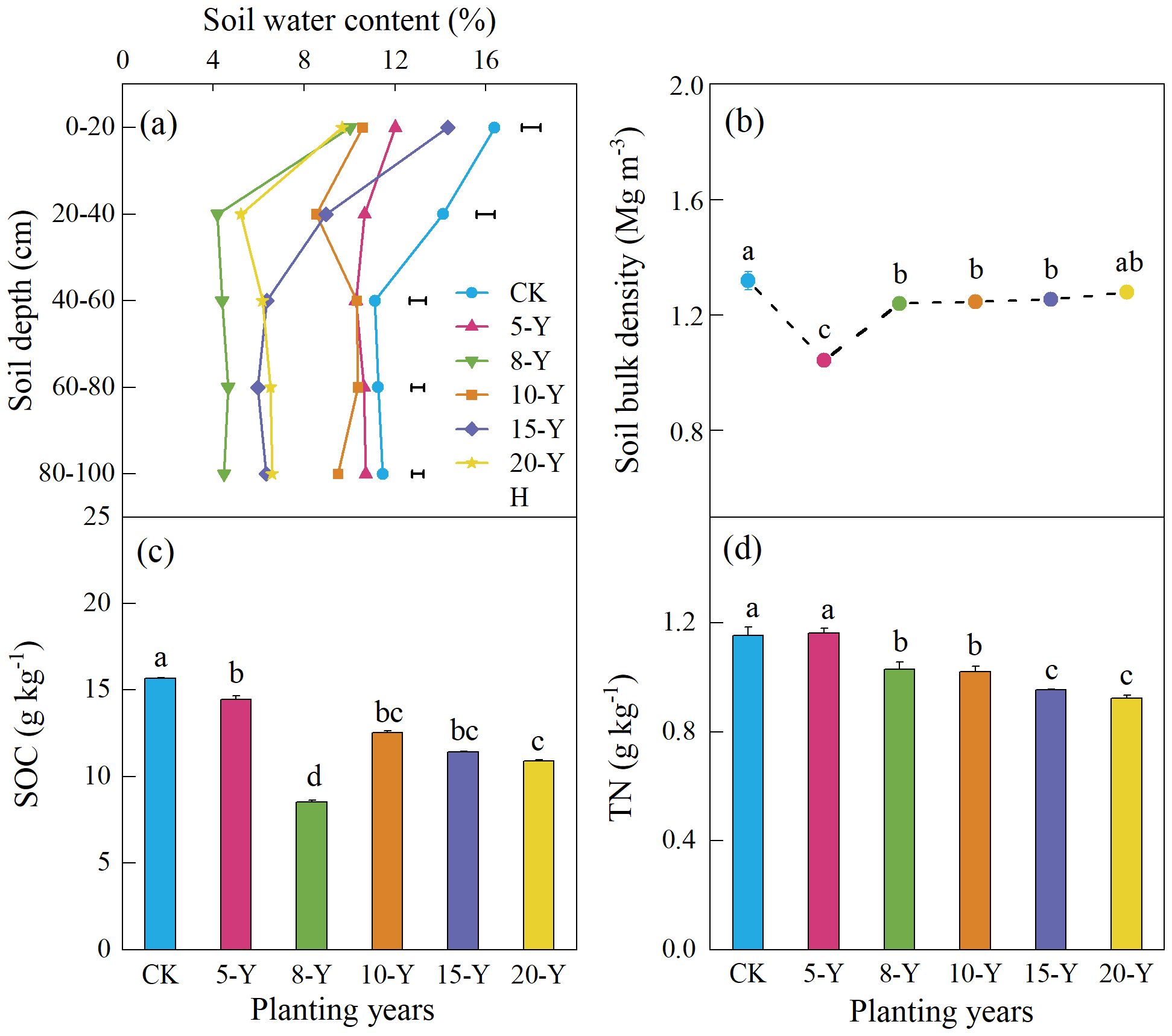
Figure 2. Dynamics of soil water content (SWC; 0–100 cm) (A), soil bulk density (B), SOC content (C), and TN content (D) at 0-cm to 20-cm soil depth in response to different durations of alfalfa planting. Different letters indicate significant differences in planting duration (p < 0.05). The values are mean + SE (error bar). CK, abandoned farmland. 5-Y, 8-Y, 10-Y, 15-Ym and 20-Y represent planting variations for 5, 8, 10, 15, and 20 years, respectively.
3.4 The dynamics of soil organic carbon and nitrogen contents under different planting years
The soil organic carbon (SOC) content of artificial alfalfa grassland at 0-cm to 20-cm soil depth showed a V-shaped trend with increasing alfalfa planting duration. The highest value of planting for 5 years was 14.4 g kg−1, and the lowest value of planting for 8 years was 8.54 g kg−1 (Figure 2C). The soil TN content was between 0.89 g kg−1 and 1.02 g kg−1, and the planting years had a significant effect on soil TN content, which showed a decreasing trend with increasing planting years (Figure 2D).
3.5 The dynamics of soil aggregate distribution with planting years
In general, the distribution of soil aggregates in size ranged considerably among various years of cultivation (Table 2). The proportion of aggregates with sizes > 2 mm and < 0.25 mm is significantly higher than that of aggregates with other sizes. After 8 years of planting, the proportion of aggregates > 2-mm size was higher than that for other planting durations, the proportions of aggregates of 1.0–2.0 mm, 0.5–1.0 mm, and 0.25–0.5 mm after 5 years of planting were higher than those for other planting durations (except for the 1.0–2.0 mm). The proportion of <0.25-mm aggregates after 20 years of planting was higher than that for other periods of cultivation.
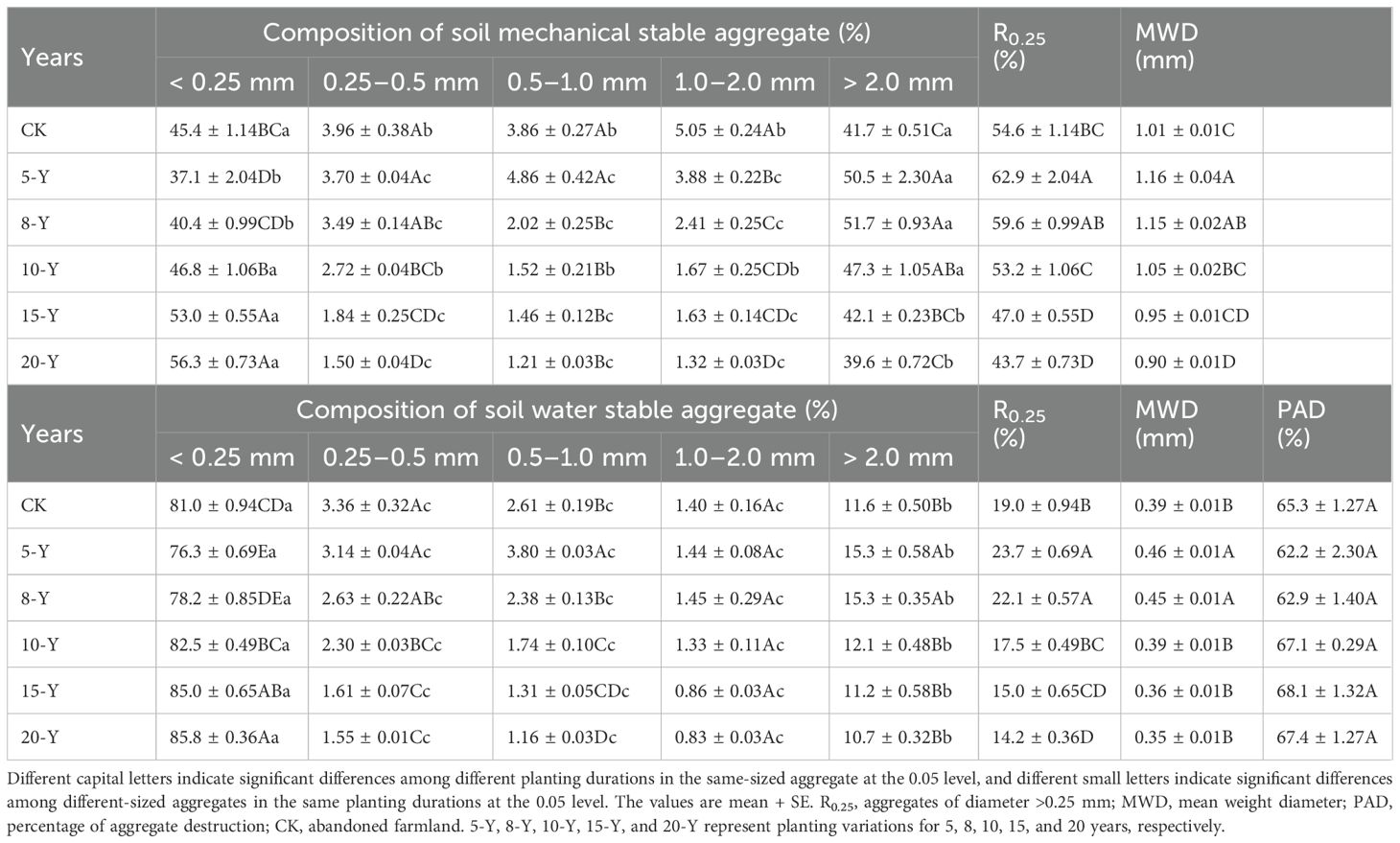
Table 2. Responses of soil mechanical and water-stable aggregate compositions to varying planting years in alfalfa field.
In addition, both the mechanical and water-stable aggregates, soil aggregate, R0.25 and MWD showed a declining trend with increase planting years. The mechanical and water-stable aggregates R0.25 ranged from 62.9% to 43.7% and from 23.7% to 14.2% accordingly. The mechanical and water-stable aggregates MWD change from 1.16 mm to 0.90 mm and from 0.46 mm to 0.35 mm, respectively. In contrast, PAD presented an increasing trend and ranged from 62.2% for planting for 5 years to 67.4% for planting for 20 years.
3.6 The soil organic carbon and total nitrogen contents of different aggregates in response to planting years
The soil organic carbon (SOC), TN, and C-N ratios in different aggregates of alfalfa grassland showed a variable trend in planting years. The SOC and TN contents of each size were the highest at 5 years of planting, but the lowest was at 8 years of planting. The C-N ratio in all five sizes showed that the highest value was in the 10-year plantation, and the lowest was in the 5-year plantation (except for the 1–2 mm) (Figure 3).
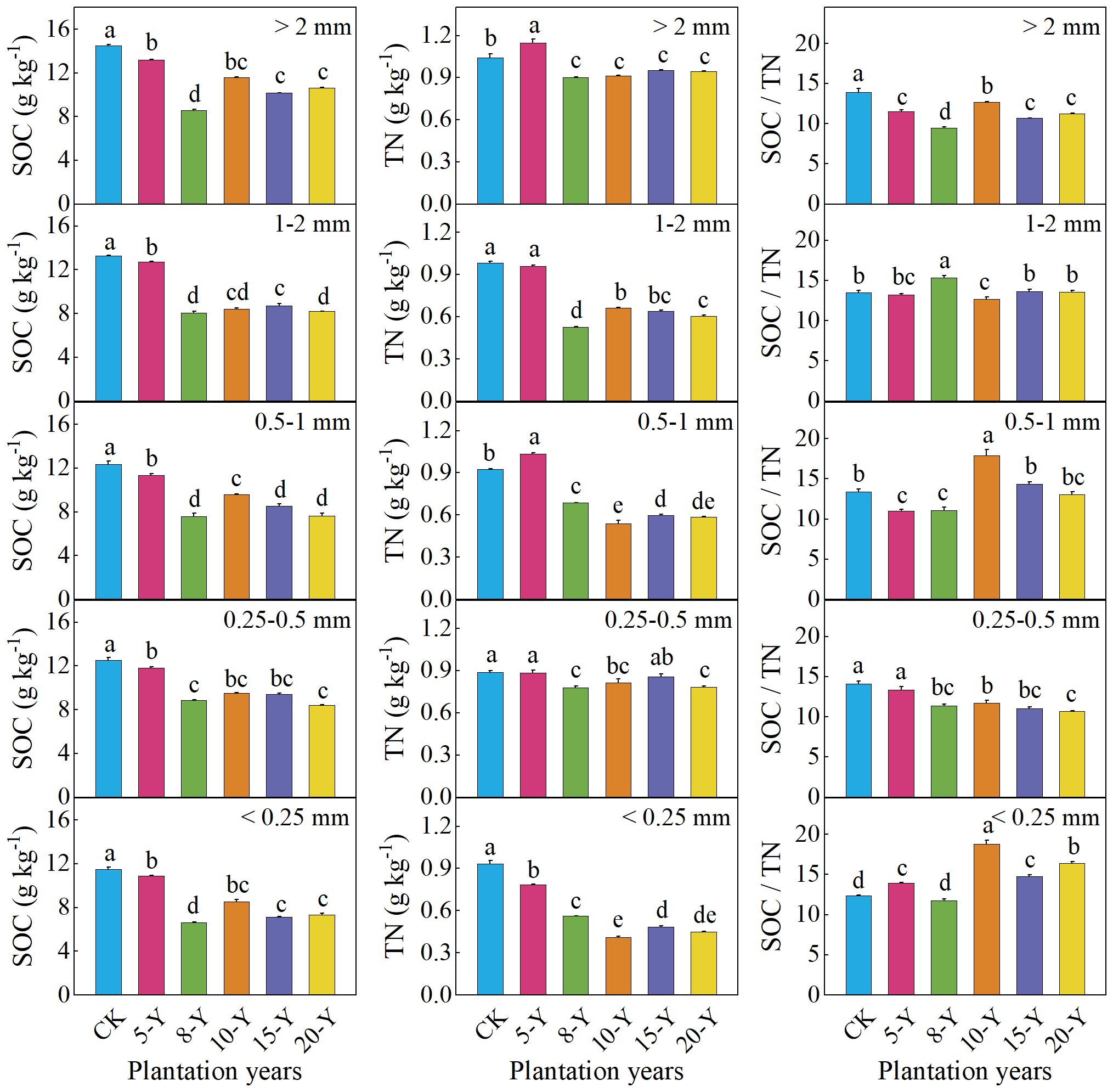
Figure 3. SOC, TN contents, and SOC/TN of different-sized aggregates at 0-cm to 20-cm soil depth in alfalfa plantations with different planting durations. Different letters indicate significant differences among different planting durations for the same-sized aggregate at the 0.05 level. The values are mean + SE (error bar). CK, abandoned farmland. 5-Y, 8-Y, 10-Y, 15-Y, and 20-Y represent planting variations for 5, 8, 10, 15, and 20 years, respectively.
In addition, the SOC and TN contents and C-N ratio of different size aggregates in the same planting years also showed a significant difference (Figure 3). In general, the SOC and TN contents of macroaggregates in the alfalfa grassland were higher than those in other particle sizes, and the < 0.25-mm particle size was the lowest. In the alfalfa grassland field, the C-N ratio of >2 mm, 0.25–0.5 mm, and <0.25 mm in 8 years was the lowest than that in the other years. After planting for 8 years, the ratios of the five size aggregates increased in all five sizes.
3.7 The dynamics of carbon and nitrogen stocks with planting years
The variation in the C and N stocks of the alfalfa succession series with different planting years is shown in Figure 4. In general, the C and N stocks of aggregates > 2 mm were the highest in each planting year (except for <0.25 mm). Among different planting years, the stock of C after 5 years of planting was the highest, and, after 8 years, it was declined. Similarly, in each planting year, the N stocks of aggregates > 2 mm were the largest. The N stocks after 5 years were the highest among the five planting years. Overall, the C and N stocks slightly decreased with prolonged planting.
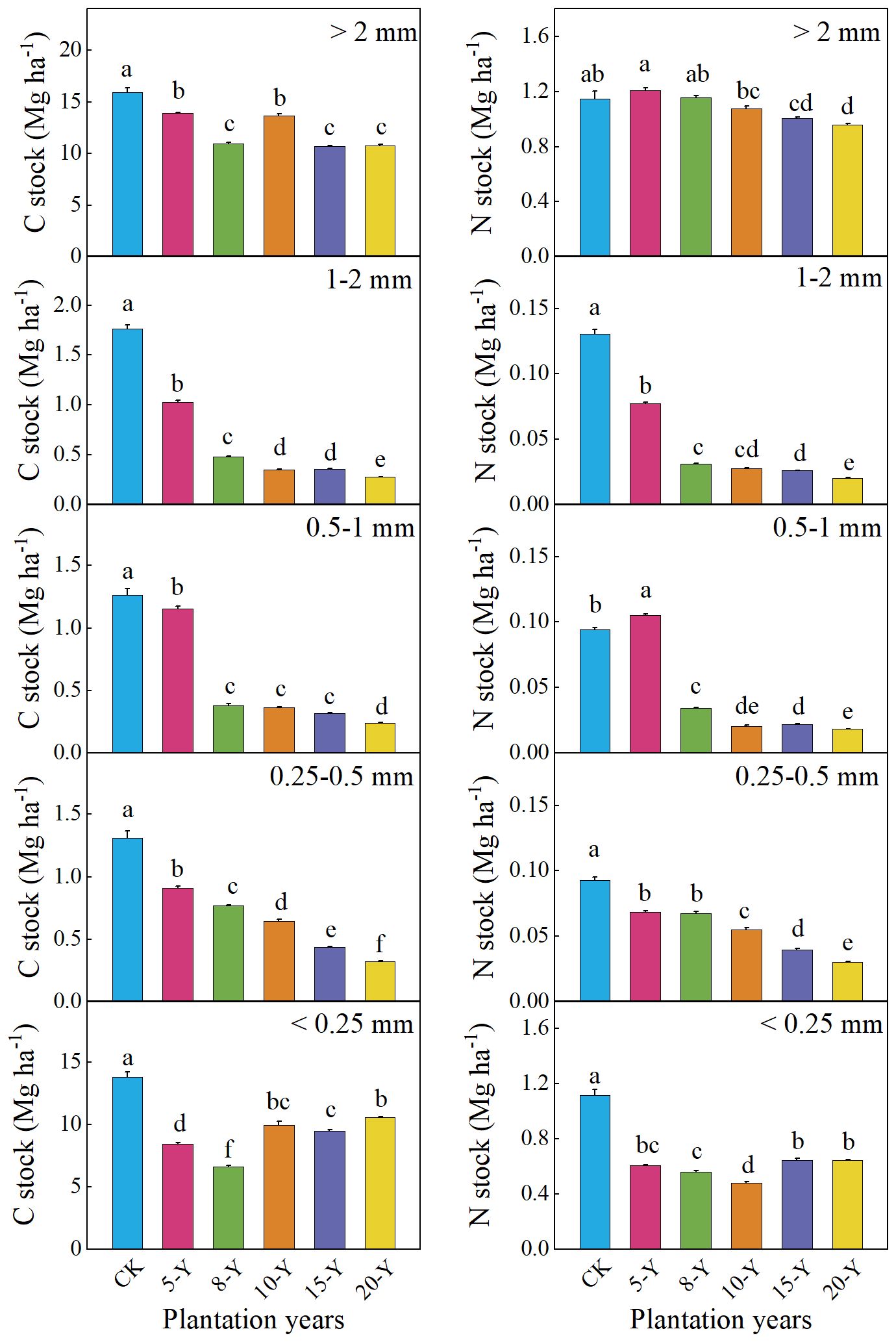
Figure 4. C and N stocks of different-sized aggregates at 0- to 20-cm soil depth in alfalfa plantations with different planting durations. Different letters indicate significant differences among different planting durations for the same-sized aggregate at the 0.05 level. The values are mean + SE (error bar). CK, abandoned farmland. 5-Y, 8-Y, 10-Y, 15-Y, and 20-Y represent planting variations for 5, 8, 10, 15, and 20 years, respectively.
3.8 Relationships of aggregate carbon and nitrogen stocks with alfalfa plants
The RDA showed that the CK treatment was concentrated in the first quadrant, and alfalfa grasslands were distributed in the other three quadrants (Figure 5). The first and second axes accounted for 84.63% (a) and 84.62% (b) of the variability explained. Aboveground biomass was negatively correlated with SBD and planting years, whereas underground biomass was positively correlated with planting years. At the same time, we found that aggregate C and N stocks were closely related to the C-N ratio of alfalfa above- and underground plants.
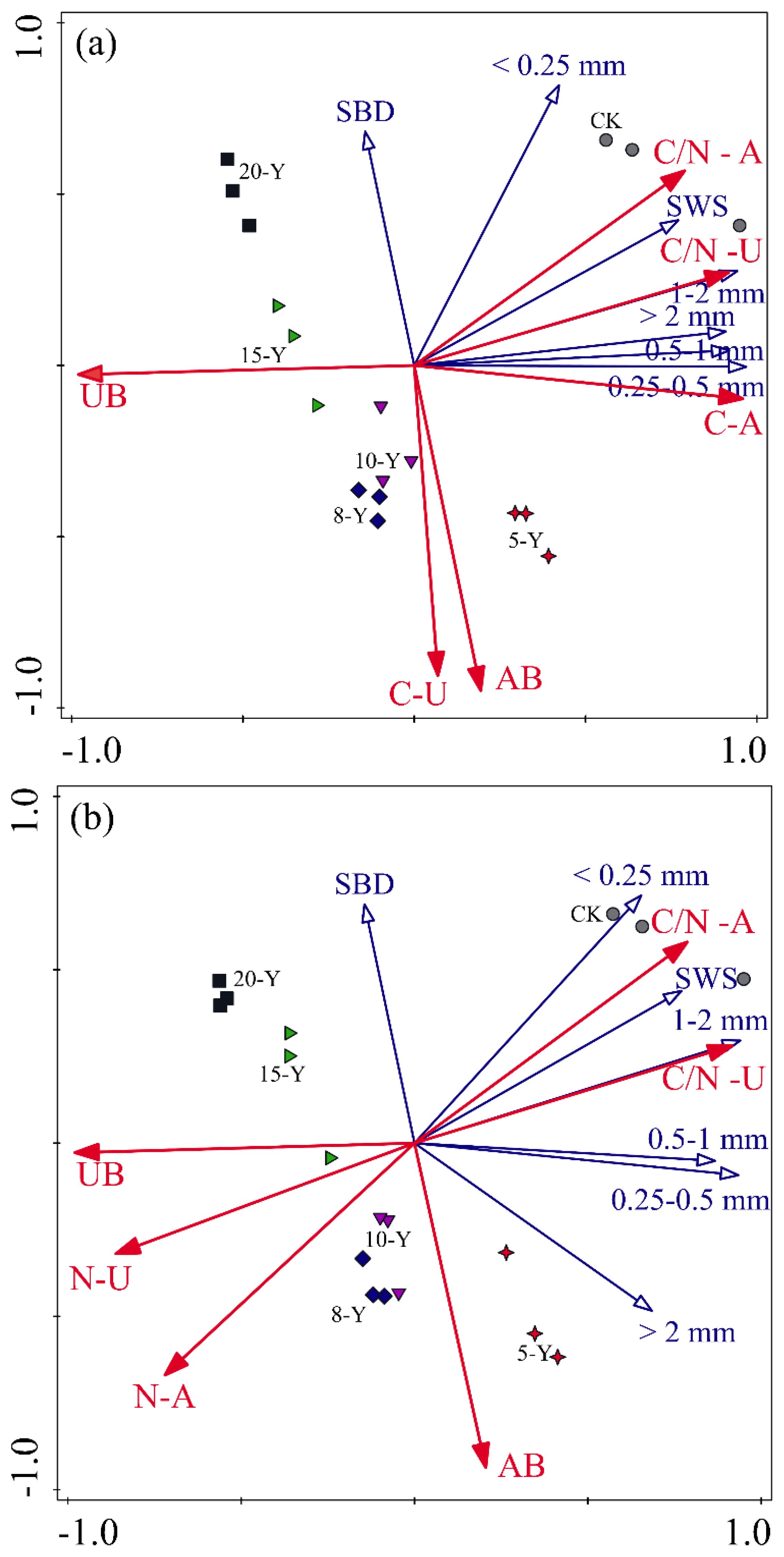
Figure 5. RDA biplot based on biomass and C and N concentrations of alfalfa plant variables in all treatments under different size aggregates C (A) and N (B) stocks. CK, abandoned farmland. 5-Y, 8-Y, 10-Y, 15-Y, and 20-Y represent planting variations for 5, 8, 10, 15, and 20 years, respectively, AB, aboveground biomass; UB, underground biomass; C-A, carbon concentration of alfalfa aboveground plant; C-U, carbon concentration of alfalfa underground plant; N-A, nitrogen concentration of alfalfa aboveground plant; N-U, carbon concentration of alfalfa underground plant; C/N-A, carbon-nitrogen ratio of alfalfa aboveground plant; C/N-U, carbon-nitrogen ratio of alfalfa underground plant; SBD, soil bulk density; SWS, soil water storage.
The correlation analysis indicated that aggregate MWD was positively correlated with alfalfa aboveground biomass and the above- and underground C content (Figure 6). The aggregate C stock was markedly positively correlated with the SWC, the C and N content of aggregates, the C content of alfalfa aboveground, and the C-N ratio of above- and underground. Similarly, the aggregate N stock was markedly positively correlated with the SWC, C and N content of the soil surface and each aggregate size, the aboveground C content of alfalfa, and the aboveground C-N ratio. The C and N stocks of aggregates were negatively correlated with underground biomass and the above- and underground N content. In addition, the aboveground C content of alfalfa was positively correlated with SWC and MWD. The above- and underground N content was negatively correlated with the SWC and aboveground C content.
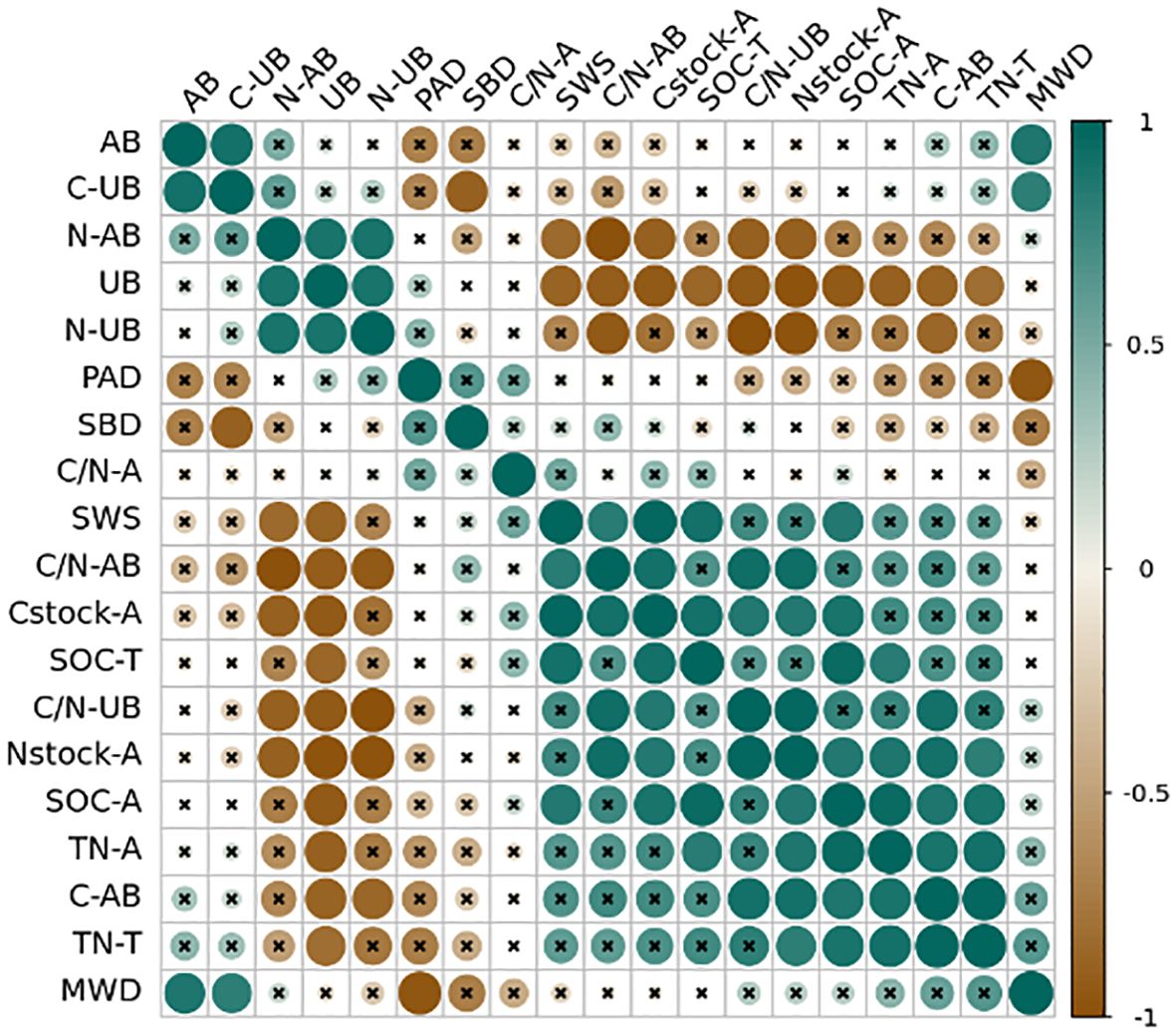
Figure 6. Correlation matrix of the study variables. Color and the size of the circles are proportional to the correlation coefficients between the variables. SWS is soil water storage, SBD is soil bulk density, SOC-T is surface soil organic carbon content of 0-cm to 20-cm depth, TN-T is surface soil total nitrogen content of 0-cm to 20-cm depth, MWD is the mean weight diameter, PAD is the percentage of aggregate destruction, SOC-A is the average SOC content of five size aggregates, TN-A is the average TN content of five size aggregates, Cstock-A is the sum of SOC stock in the five size aggregates, Nstock-A is the sum of TN stock in the five size aggregates, AB is the aboveground biomass, UB is the underground biomass, C-AB is the SOC content of aboveground plant, C-UB is the SOC content of root, N-AB is the TN content of aboveground plant, N-UB is the TN content of root, C/N-AB is the C/N content of aboveground plant, C/N-UB is the C/N content of root. The values in the figure represent correlation coefficients.
4 Discussion
4.1 The dynamic of alfalfa productivity under cultivation years
The productivity of alfalfa is affected by soil fertility, soil physical conditions, and other ecological factors (Feng et al., 2022). Soil moisture will affect the growth of roots, and excessive or limited water supply will change the distribution of crop roots, thereby affecting yields (Jia et al., 2009; Fan and Schutze, 2024). The results of this study showed that the largest aboveground biomass was found after 5 years in a cultivated field (Table 1), which is related to the precipitation in previous and subsequent years (Figure 1). Water is the main limiting factor restricting vegetation restoration and reconstruction, and it determines the water–soil ecological relationship of grassland on the Loess Plateau (Jiang et al., 2006). Soil moisture is affected by many factors, such as soil characteristics, land-use structure, topography, vegetation types, and weather, resulting in complex dynamic changes (Fan et al., 2016). In this paper, compared to the control, the soil water content of alfalfa with different planting years has a greater decrease (Figure 2A), which indicates that the soil water deficit is obvious. Because the local soil is loess soil, it is beneficial for rainwater to penetrate the soil and restore the water content. On the other hand, we found that the root biomass was negatively correlated with SWC (Figure 6), which may be because the root system of alfalfa is relatively wide, and the proliferation of the root system in the soil increases the porosity of the soil (Jia et al., 2009; Heinze, 2020). In addition, as the planting time increases, the distribution of root systems becomes wider and deeper, which often leads to more consumption and utilization of shallow soil water (Li and Huang, 2008). Moreover, as the planting time increases, the soil moisture gradually decreases, which is directly related to the root biomass. The accumulation of root biomass requires sufficient soil moisture, which leads to water depletion in the soil profile. In addition, alfalfa productivity was also affected by local rainfall (Zhang X. L. et al., 2021). In our study, we found that planting alfalfa for 5 years had the highest aboveground biomass, which may be related to the increasing rainfall from 2010 to 2013, and, after 2013 years, alfalfa biomass showed a declining trend due to the relative decreasing precipitation (Figure 1).
4.2 The driving factors of soil aggregate distribution in alfalfa grasslands
Soil aggregates are closely connected with soil physical, chemical, and biological properties (Plaza et al., 2013; Li et al., 2019). In this experiment, the particle size distribution of soil aggregates in different years of alfalfa cultivation and abandoned land showed a “V”-shaped trend, and soil aggregate size distributions of >2 mm and <0.25 mm were dominant (Table 2). This can be further explained by the fact that the stability of loess soil is generally low. Therefore, macroaggregates will decompose into microaggregates or smaller soil particles after being immersed in water (Lu et al., 2021). In addition, alfalfa planting for numerous years significantly increased the macroaggregate content in certain years, which is directly related to the proliferation of alfalfa roots. Because the root biomass can be increased by producing thick roots or multiple thin roots (Heinze, 2020), the increase in planting time will lead to more accumulation of root biomass, and this extensively proliferating root system helps to consolidate broad soil particles from smaller ones (Tisdall and Oades, 1982). However, due to the reduced physical and mechanical disturbance, the surface herbs, litter, and root biomass increase in natural-restored grassland, resulting in increased soil organic carbon, which is beneficial for aggregate formation (Xiao et al., 2019). Similar outcomes have been reported by Song et al. (2024) who found that the plant residue accelerates the soil aggregates turnover, promoting the soil macroaggregate formation. In addition, we found that dissolved organic matter that, as a substrate for microorganism, contributes to the increase in microbial activity may contribute to macroaggregate formation and stability (Sonsri and Watanabe, 2023).
4.3 The driving factors of plant–soil C and N stoichiometry of alfalfa grasslands
Plant–soil C and N concentrations and their ratios are essential in the restoration process of grasslands, not only for a better understanding of the C and N cycles but also for management practices (Du and Gao, 2021). We evaluated alfalfa above- and underground plant C and N contents and their ratios during the multiyear growing season. Our results showed that multiple planting durations decreased aboveground plant C and N concentrations and the C-N ratio (Table 1). These findings are supported by years of continuous planting, soil nutrient deficiency, gradual alfalfa grassland degradation, and plant biomass decrease due to self-toxic and self-thinning effects, which eventually results in less photosynthetic function and a lack of C assimilates in alfalfa grasslands (Zhang et al., 2017). At the same time, the results of Table 1 indicated that the N concentration of alfalfa roots increased with increasing planting years, which is similar to the findings of other studies (Cao et al., 2020). The higher N concentrations of roots in plantations than those in natural-restored grassland can be explained by the N2 fixation of leguminous forage (Song et al., 2021). Additionally, in our paper, we found that alfalfa planting duration resulted in soil C and N decreases in the topsoil layer compared to the control (Figure 2). These results were mainly attributed to decreased plant inputs due to yearly plant removal, a decrease in outside litter input, and increased decomposition rates (Zhang J. H. et al., 2015). Moreover, the degree of C and N decrease depends on many factors, such as basic soil SOC and TN contents, climate conditions, and surface erosion status (Yang et al., 2019).
The SOC content of soil aggregates is a microscopic expression of SOC balance and mineralization rate, which is of great significance to soil fertility and soil carbon sequestration, and the TN content of soil is one of the main indicators of soil fertility (Lu et al., 2021). In this experiment, alfalfa grassland planted for 5 years had higher SOC and TN contents, and the largest SOC and TN storage were found in particles >2 mm and <0.25 mm, whereas the lowest SOC and TN contents were found in particles of 0.25–2.00 mm (Figure 3). This is mainly because macroaggregates are made up of many microaggregates and because of the formation of microaggregates through the combination of organic molecules with clay and cations and not by aggregated particles (Devine et al., 2014). Therefore, soil macroaggregates and microaggregate are rich in organic matter, and soil macroaggregates have a faster turnover time than middle aggregates (Puget et al., 2000). The microaggregates and the surrounding small particles combine to form large aggregates; when the large soil aggregates decompose into microaggregates, the particulate organic matter decomposes, resulting in a greatly reduced SOC content of the microaggregates (Bissonnais and Arrouays, 1997). At the same time, the binding and bonding effects of macroaggregates also reduces the SOC of microaggregates (Tisdall and Oades, 1982). Previous studies have shown that the SOC content of aggregates mainly exists in the particle size of <0.25 mm, which is a combination of smaller organic and inorganic colloids. The smaller aggregates have a larger specific surface area after being combined, resulting in more adsorbed organic matter (He et al., 2011).
Considering the aggregate size distribution and the SOC and TN of specific aggregates, the aggregate size distribution not only represents the contribution rate of aggregates to SOC and TN, but it might also completely depict the interaction of different planting durations to the soil C and N pools (Xu et al., 2012). In different numbers of alfalfa planting years, the stocks of SOC and TN in different proportions of aggregates were mainly restricted by aggregates > 2 mm, which were significantly higher than those of aggregates of other sizes (Figure 4). After 5 years of planting, the SOC and TN reserves of the soil aggregates were the highest. This is because, after 5 years of planting, the soil formed a stable plant community, increased the biomass of underground roots, and accumulated more SOC in the soil. At the same time, alfalfa is a leguminous crop with strong nitrogen fixation ability. The roots fix atmospheric nitrogen, which is conducive to the accumulation of soil nitrogen (Liu et al., 2005; Thomas et al., 2009). In our study, the soil C and N stocks decreased after alfalfa planting duration, which may be caused by the very low C and N inputs from plants and soil. Aminiyan et al. (2015) reported higher aggregate-related SOC content in larger aggregate fractions (> 2 mm). In short, large aggregates > 2 mm are the main contributors to the SOC and TN contents in soil aggregates. This may be due to the increase in the strength or stability of the aggregates due to the humidification of crop residues, thereby increasing the SOC concentration of the large aggregates (Kushwaha et al., 2001). Therefore, increasing the number of aggregates with a particle size greater than 2 mm can enhance soil carbon and nitrogen fixation. These findings may be limited by the method of observing spatial and temporal changes used in this article. However, we will focus on research on long-term positioning to overcome these shortcomings in the future.
4.4 The degradation mechanism of perennial alfalfa grasslands
Obtaining sustainable high biomass and suitable soil nutrient management is crucial for cultivate alfalfa grassland (Fang et al., 2021); however, degraded continuous alfalfa grasslands are frequently characterized by substantially reduced of biomass output and potential losses in soil nutrient (Gu et al., 2018). In the Loess Plateau of China, the low precipitation and high evapotranspiration of alfalfa field will limit its sustainable development (Ren et al., 2010). The lack of soil water content is the mainly parameter caused the perennial alfalfa grassland degradation (Wang et al., 2019). Alfalfa is a deep root plant that gives it access to water deeper in the soil than annual pastures and crops (Cheng et al., 2005). Alfalfa used soil water from deeper soil profile than other crops and extracted more water and thus creates a large soil water deficit (Huang et al., 2018). Previous study showed that the length of the alfalfa cropping phase in the short term (2–4 years) depends on soil water replenishment, and alfalfa continuously, for 6 years, reduces a relatively desiccation layer in the soil with 2-m to 10-m depth (Li, 1983). Li and Chao (1992) also found that the biomass output of continuous alfalfa markedly decreased after 7 or 8 years due to the declined of soil water. Jia et al. (2009) reported that the alfalfa average yield reached a peak after continuous 9 years planting and, more than 11 years, cultivated had higher soil water use efficiency. In our results, we also found that the soil water content was quickly declined after continuous cultivated alfalfa for 8 years (Figure 2A), the dynamic of underground biomass (the underground biomass reached the higher value in 8 year) (Table 1), because the longer the growing years of alfalfa, the deeper its root distribution, which intensified the high water consumption at deep soil (there was no rainfall when we collected soil moisture samples during the first half of the month). On the contrary, the soil water content increased from 10 to 20 years; this was probably because the alfalfa plants’ low transpiration and low productivity made less water consumption. The above results were coincided with the found by Li and Huang (2008) and Ren et al. (2010) and Li et al. (2018). Therefore, the long alfalfa stand duration may deplete available soil water, which would negatively impact production (Li and Huang, 2008) and thus caused the alfalfa grassland degradation.
On the other hand, the deficiency of soil nutrient also limits the alfalfa grassland sustainable development, and eventually leading to alfalfa grassland degradation and, conversely, enhancing the fertilizer application in perennial alfalfa can boost biomass output and prolong the alfalfa degradation timeline (Hakl et al., 2016; Gu et al., 2018; Fang et al., 2021). The decrease of soil nutrients, especially soil nitrogen, limits the perennial alfalfa growth, which, in turn, becomes a crucial restricting factor for the alfalfa cultivation (Yuan, 2017; Fang et al., 2021). In our results, we found that the soil C concentration decreased in the eight year and then increased after the 10th year of alfalfa cultivation (Figure 2). The soil C dynamical dramatically declined mainly because the fertilizer was applied in early planting alfalfa, and the carbon source in the soil is increased. With the continuous cultivated, a large amount of aboveground biomass was removed from alfalfa grassland, and the less carbon source will add to soil. In addition, the root biomass litters were not converted to carbon source by microbe decomposition. However, after cultivation alfalfa for the 10th to 20th years, the soil C storage increased, especially in the microaggregate, and this agrees with the findings from other researchers (Li and Huang, 2008). It seems that soil C storage rose through enhancing the aggregate stability with the increased in cultivation years. However, in this study, TN content continues decreased compared to the fifth year of alfalfa cultivation (Figure 2), mainly because the N that was derived from symbiotic fixation by perennial alfalfa over 20th years does not reach the N value of farmland, and artificial nitrogenous fertilizer losses were larger than N fixation by perennial alfalfa. However, the TN storage in the microaggregate presented less declined and finally increased after continuous cultivation on the 15th to 20th years (Figure 4). This was probably because this growing stage reached an imbalance between TN fixation and loss and reduced the consumption of TN on account of decreased alfalfa plant. Similar results were reported by Ji et al. (2020), who found that the highest values of SOC and TN content were observed in the begin stage of alfalfa grassland, and, with the cultivated prolong, the above contents declined, and the decline soil nutrients restricted alfalfa growth and biomass output.
5 Conclusion
We first found the ecological indicator of C and N stoichiometry from plant to soil aggregate fractions affecting soil degradation. Alfalfa plantations can improve soil structure and promote the number of macroaggregates within a certain year. Different planting periods display different effects on the SOC and TN distribution of aggregates. In the present study, the inputs of organic C compounds via root exudates provide a material base for the rapid turnover of C and N stocks. Both low-quality resources (high C/N) and high-quality material supply (low C/N) exert their effects on sustaining soil nutrients. Thus, alfalfa production can promote larger aggregates and accordingly improve soil C and N in large aggregates. This process would help improve the soil structure under the condition of continuous alfalfa planting. However, after 5–8 years of planting, alfalfa grassland productivity and soil quality began to degrade gradually. In conclusion, a certain period of alfalfa planting strengthened the stability of the soil physical structure, yet it resulted in a steady deterioration of the C and N stocks from soil to plant. Our findings provide novel insight into perennial alfalfa grassland degradation and, therefore, help explore a potentially sustainable solution in semiarid rainfed agricultural areas.
Data availability statement
The original contributions presented in the study are included in the article/supplementary material. Further inquiries can be directed to the corresponding authors.
Author contributions
WW: Conceptualization, Data curation, Formal analysis, Funding acquisition, Investigation, Methodology, Project administration, Resources, Software, Supervision, Validation, Visualization, Writing – original draft, Writing – review & editing. TT: Conceptualization, Data curation, Formal analysis, Investigation, Methodology, Project administration, Resources, Software, Supervision, Writing – original draft, Writing – review & editing. M-YL: Conceptualization, Data curation, Formal analysis, Investigation, Methodology, Validation, Writing – original draft, Writing – review & editing. B-ZW: Conceptualization, Data curation, Formal analysis, Investigation, Methodology, Software, Writing – original draft, Writing – review & editing. QZ: Conceptualization, Data curation, Formal analysis, Resources, Software, Validation, Writing – original draft, Writing – review & editing. F-JM: Data curation, Formal analysis, Investigation, Software, Writing – original draft, Writing – review & editing. J-YL: Data curation, Investigation, Methodology, Software, Writing – original draft, Writing – review & editing. NW: Data curation, Investigation, Methodology, Software, Writing – original draft, Writing – review & editing. Y-MY: Data curation, Investigation, Methodology, Software, Writing – original draft, Writing – review & editing. H-YT: Investigation, Writing – original draft, Writing – review & editing. LZ: Conceptualization, Data curation, Formal analysis, Funding acquisition, Investigation, Methodology, Project administration, Resources, Software, Supervision, Validation, Visualization, Writing – original draft, Writing – review & editing. Y-CX: Conceptualization, Data curation, Formal analysis, Funding acquisition, Investigation, Methodology, Project administration, Resources, Software, Supervision, Validation, Visualization, Writing – original draft, Writing – review & editing.
Funding
The author(s) declare financial support was received for the research, authorship, and/or publication of this article. This research was supported by the Research Start-up Project of Shanxi Agricultural University for Recruited Talents (2023BQ120), Promotive Scientific Research Fund for New PhD Graduates working in Shanxi Province (SXBYKY2023038), Youth Science and Technology Research Program of Shanxi Province Basic Research Program (202303021222077 and 202303021212104), National Natural Science Foundation of China (32161143012), Key Research and Development Program of Gansu Province (20YF8WA083), and “111” Program (BP0719040).
Conflict of interest
The authors declare that the research was conducted in the absence of any commercial or financial relationships that could be construed as a potential conflict of interest.
Publisher’s note
All claims expressed in this article are solely those of the authors and do not necessarily represent those of their affiliated organizations, or those of the publisher, the editors and the reviewers. Any product that may be evaluated in this article, or claim that may be made by its manufacturer, is not guaranteed or endorsed by the publisher.
References
Aminiyan, M. M., Sinegani, A. A. S., Sheklabadi, M. (2015). Assessment of changes in different fractions of the organic carbon in a soil amended by nanozeolite and some plant residues: incubation study. Int. J. Recycl. Organ. Waste Agric. 4, 239–247. doi: 10.1007/s40093-015-0110-6
Araujo, R. A., Gamarodrigues, E. F., Gamarodrigues, A. C., M. Vieira, R. A. (2024). Carbon mineralization in soil aggregate classes under Leguminous tree planting in north Fluminense, Brazil. Floresta Ambient. 31, e20230033. doi: 10.1590/2179-8087-FLORAM-2023-0033
Austin, A. T., Vitousek, P. M. (2012). Introduction to a virtual special issue on ecological stoichiometry and global change. New Phytol. 196, 649–651. Available at: https://www.jstor.org/stable/newphytologist.196.3.649.
Bhatt, R., Singh, P., Sharma, S. (2023). Changes in soil organic pool and carbon preservation capacity of macro- and micro-aggregates in response to land-use change in north-western India. J. Soil Sci. Plant Nutt. 23, 2849–2867. doi: 10.1007/s42729-023-01239-x
Bissonnais, Y. L. E., Arrouays, D. (1997). Aggregate stability and assessment of soil crustability and erodibility: II. Application to humic loamy soils with various organic carbon contents. Eur. J. Soil Sci. 48, 39–48. doi: 10.1111/j.1365-2389.1997.tb00183.x
Bodner, G., Leitner, D., Kaul, H. P. (2014). Coarse and fine root plants affect pore size distributions differently. Plant Soil 380, 133–151. doi: 10.1007/s11104-014-2079-8
Bremner, J. M. (1997). “Nitrogen-total,” in Part3. Chemical methods. Ed. Sparks, D. L. (ASA and SSSA, Madison, WI), 1085–1121.
Cao, Y., Li, Y. N., Zhang, G. Q., Zhang, J., Chen, M. (2020). Fine root C:N:P stoichiometry and its driving factors across forest ecosystems in northwestern China. Sci. Total Environ. 737, 140299. doi: 10.1016/j.scitotenv.2020.140299
Cheng, J. M., Wan, H. E., Wang, J. (2005). Alfalfa growth and its relation with soil water status in loess hilly and gully region. (In Chinese) Chin. J. Appl. Ecol. 3, 435–438.
Dan, X., He, M., Meng, L., He, X., Wang, X., Chen, S., et al. (2023). Strong rhizosphere priming effects on N dynamics in soils with higher soil N supply capacity: The ‘Matthew effect’ in plant-soil system. Soil Biol. Biochem. 178, 108949. doi: 10.1016/j.soilbio.2023.108949
Devine, S., Markewitz, D., Hendrix, P., Coleman, D. (2014). Soil aggregates and associated organic matter under conventional tillage, no-tillage, and forest succession after three decades. PloS One 9, 1–12. doi: 10.1371/journal.pone.0084988
Du, C. J., Gao, Y. H. (2021). Grazing exclusion alters ecological stoichiometry of plant and soil in degraded alpine grassland. Agric. Ecosyst. Environ. 308, 107256. doi: 10.1016/j.agee.2020.107256
Fan, J. W., Du, Y. L., Wang, B. R., Turner, N. C., Wang, T., Abbott, L. K., et al. (2016). Forage yield, soil water depletion, shoot nitrogen and phosphorus uptake and concentration, of young and old stands of alfalfa in response to nitrogen and phosphorus fertilisation in a semiarid environment. Field Crops Res. 198, 247–257. doi: 10.1016/j.fcr.2016.08.014
Fan, X. Y., Schutze, N. (2024). Assessing crop yield and water balance in crop rotation irrigation systems: Exploring sensitivity to soil hydraulic characteristics and initial moisture conditions in the North China Plain. Agri Water Mange. 300, 108897. doi: 10.1016/j.agwat.2024.108897
Fang, Y., Huang, Z., Cui, Z., He, H. H., Liu, Y. (2021). Trade-offs between forage crop productivity and soil nutrients for different ages of alfalfa grassland. Land Degrad. Dev. 32, 374–386. doi: 10.1002/ldr.3686
Feng, Y. P., Shi, Y., Zhao, M. Y., Shen, H. H., Xu, L. C., Luo, Y. K., et al. (2022). Yield and quality properties of alfalfa (Medicago sativa L.) and their influencing factors in China. Eur. J. Agron. 141, 126637. doi: 10.1016/j.eja.2022.126637
Gong, Y. H., Zhao, D. M., Ke, W. B., Fang, C., Pei, J. Y., Sun, G. J. (2020). Legacy effects of precipitation amount and frequency on the aboveground plant biomass of a semi-arid grassland. Sci. Total Environ. 705, 135899. doi: 10.1016/j.scitotenv.2019.135899
Gu, Y. J., Han, C. L., Fan, J. W., Shi, X. P., Kong, M., Shi, X. Y., et al. (2018). Alfalfa forage yield, soil water and P availability in response to plastic film mulch and P fertilization in a semiarid environment. Field Crops Res. 215, 94–103. doi: 10.1016/j.fcr.2017.10.010
Guo, Z. B., Yan, G. J., Zhang, R. H., Li, F. M., Zeng, Z. X., Liu, H. (2010). Improvement of soil physical properties and aggregate-associated C, N, and P after cropland was converted to grassland in semiarid Loess Plateau. Soil Sci. 175, 99–104. doi: 10.1097/ss.0b013e3181cda54a
Hakl, J., Kunzova, E., Konecna, J. (2016). Impact of long-term organic and mineral fertilization on lucerne forage yield over an 8-year period. Plant Soil Env. 62, 36–41. doi: 10.17221/660/2015-pse
He, S. Q., Zheng, Z. C., Gong, Y. B. (2011). Distribution characteristics and soil organic carbon of soil water-stable aggregates with different de-farming patterns. J. Soil Water Conserv. 25, 229–233. doi: 10.13870/j.cnki.stbcxb.2011.05.045
Heinze, J. (2020). Herbivory by aboveground insects impacts plant root morphological traits. Plant Ecol. 221, 725–732. doi: 10.1007/s11258-020-01045-w
Huang, Z., Liu, Y., Cui, Z., Fang, Y., He, H. H., Liu, B. R., et al. (2018). Soil water storage deficit of alfalfa (Medicago sativa) grasslands along ages in arid area (China). Field Crops Res. 221, 1–6. doi: 10.1016/j.fcr.2018.02.013
Ji, B., Wang, Z., Pan, Z., Xu, H., Han, X. S., Xie, Y. Z. (2020). Soil carbon storage characteristics of alfalfa (Medicago sativa) artificial grasslands in the semi-arid hilly gully region of the loess plateau, China. Russ J. Ecol. 51, 466–476. doi: 10.1134/S1067413620050045
Jia, Y., Li, F. M., Zhang, Z. H., Wang, X. L., Guo, R. Y., Siddique, K. H. M. (2009). Productivity and water use of alfalfa and subsequent crops in the semiarid Loess Plateau with different stand ages of alfalfa and crop sequences. Field Crops Res. 114, 58–65. doi: 10.1016/j.fcr.2009.07.004
Jiang, H. M., Jiang, J. P., Jia, Y., Li, F. M., Xu, J. Z. (2006). Soil carbon pool and effects of soil fertility in seeded alfalfa fields on the semi-arid Loess Plateau in China. Soil Biol. Biochem. 38, 2350–2358. doi: 10.1016/j.soilbio.2006.02.008
Kubar, K. A., Huang, L., Xue, B., Li, X. K., Lu, J. W. (2021). Straw management stabilizes the chemical composition of soil organic carbon (SOC): the relationship with aggregate-associated C in a rice-rape cropping system. Land Degrad. Dev. 32, 851–866. doi: 10.1002/ldr.3727
Kushwaha, C., Tripathi, S., Singh, K. (2001). Soil organic matter and water-stable aggregates under different tillage and residue conditions in a tropical dryland agroecosystem. Appl. Soil Ecol. 16, 229–241. doi: 10.1016/S0929-1393(00)00121-9
Li, Y. (1983). The properties of water cycle in soil and their effect on water cycle for land in the Loess Plateau. Acta Ecol. Sin. 3, 91–101.
Li, Q., Chao, Z. (1992). Survey and analysis to development of alfalfa in the Loess Plateau. Chin. Pasture Sci. 9, 7–11.
Li, J., Deng, L., Peuelas, J., Wu, J., Shangguan, Z., Sardans, J., et al. (2023). C:N:P stoichiometry of plants, soils, and microorganisms: Response to altered precipitation. Global Change Biol. 29, 7051–7071. doi: 10.1111/gcb.16959
Li, Y. S., Huang, M. B. (2008). Pasture yield and soil water depletion of continuous growing alfalfa in the Loess Plateau of China. Agric. Ecosyst. Environ. 124, 24–32. doi: 10.1016/j.agee.2007.08.007
Li, F. Q., Qiu, P. F., Shen, B., Shen, Q. R. (2019). Soil aggregate size modi fi es the impacts of fertilization on microbial communities. Geoderma 343, 205–214. doi: 10.1016/j.geoderma.2019.02.039
Li, J. P., Xie, Y. Z., Deng, L., Wang, K. B., Li, X. W. (2018). Soil physico-chemical property dynamics when continually growing alfalfa (Medicago sativa) in the loess plateau of China. Nat. Environ. pollut. Technol. 17, 11–20.
Li, Y., Zhang, X., Cao, Z., Liu, Z., Lu, Z., Liu, Y. (2021). Towards the progress of ecological restoration and economic development in China’s Loess Plateau and strategy for more sustainable development. Sci. Total. Environ. 756, 143676. doi: 10.1016/j.scitotenv.2020.143676
Liu, J. X., Price, D. T., Chen, J. M. (2005). Nitrogen controls on ecosystem carbon sequestration: a model implementation and application to Saskatchewan, Canada. Ecol. Modell 186, 178–195. doi: 10.1016/j.ecolmodel.2005.01.036
Liu, Y. L., Zhang, M., Xiong, H., Li, Y., Zhang, Y. R., Huang, X. C., et al. (2023). Influence of long-term fertilization on soil aggregates stability and organic carbon occurrence characteristics in karst yellow soil of Southwest China. Front. Plant Sci. 14. doi: 10.3389/fpls.2023.1126150
Lu, X. F., Hou, E. Q., Guo, J. Y., Gilliam, F. S., Li, J. L., Tang, S. B., et al. (2021). Nitrogen addition stimulates soil aggregation and enhances carbon storage in terrestrial ecosystems of China: A meta-analysis. Glob. Change Biol. 27, 2780–2792. doi: 10.1111/gcb.15604
Lustenhouwer, N., Chanbet, T. M. R., Melen, M. K., Putten, W. H. V. D., Parker, I. M. (2024). Plant–soil interactions during the native and exotic range expansion of an annual plant. J. J. Evolution Biol. 37, 653–664. doi: 10.1093/jeb/voae040
Plaza, C., Courtier-murias, D., Fernández, J. M., Polo, A., Simpson, A. J. (2013). Physical, chemical, and biochemical mechanisms of soil organic matter stabilization under conservation tillage systems: A central role for microbes and microbial by-products in C sequestration. Soil Biol. Biochem. 57, 124–134. doi: 10.1016/j.soilbio.2012.07.026
Puget, P., Chenu, C., Balesdent, J. (2000). Dynamics of soil organic matter associated with particle-size fractions of water-stable aggregates. Eur. J. Soil Sci. 51, 595–605. doi: 10.1111/j.1365-2389.2000.00353.x
Qi, J. J., Fu, D. Q., Wang, X. Z., Zhang, F. F., Ma, C. H. (2023). The effect of alfalfa cultivation on improving physicochemical properties soil microorganisms community structure of grey desert soil. Sci. Rep. 13, 13747. doi: 10.1038/s41598-023-41005-8
Ren, X. L., Jia, Z. K., Wan, S. M., Chen, X. L. (2010). Soil water distribution on different number of growing years of alfalfa pasture in the Loess Plateau of Northwest China. Afr. J. Biotechnol. 9, 5686–5693. doi: 10.5897/AJB10.245
Sardans, J., Grau, O., Chen, H., Janssens, I. A., Ciais, P., Piao, S., et al. (2017). Changes in nutrient concentrations of leaves and roots in response to global change factors. Glob. Change Biol. 23, 3849–3856. doi: 10.1111/gcb.13721
Shen, Y., Zhang, R., Yang, Q., Liu, Z., Li, G., Han, H., et al. (2024). Long-term subsoiling and tillage rotation increase carbon storage in soil aggregates and the abundance of autotrophs. Appl. Soil Ecol. 200, 105444. doi: 10.1016/j.apsoil.2024.105444
Song, X., Fang, C., Yuan, Z. Q., Li, F. M. (2021). Long-term growth of alfalfa increased soil organic matter accumulation and nutrient mineralization in a semi-arid environment. Front. Env. Sci. 9. doi: 10.3389/fenvs.2021.649346
Song, X., Yuan, Z. Q., Fang, C., Hu, Z. H., Li, F. M., Sardans, J., et al. (2024). The formation of humic acid and micro-aggregates facilitated long-time soil organic carbon sequestration after Medicago sativa L. introduction on abandoned farmlands. Geoderma 445, 116889. doi: 10.1016/j.geoderma.2024.116889
Sonsri, K., Watanabe, A. (2023). Insights into the formation and stability of soil aggregates in relation to the structural properties of dissolved organic matter from various organic amendments. Soil Till. Res. 232, 105774. doi: 10.1016/j.still.2023.105774
Su, Y. Z., Liu, W. J., Yang, R., Chang, X. X. (2009). Changes in soil aggregate, carbon, and nitrogen storages following the conversion of cropland to alfalfa forage land in the Marginal Oasis of northwest China. Environ. Manage. 43, 1061–1070. doi: 10.1007/s00267-009-9284-x
Tan, M., Li, W., Zong, R., Li, X., Han, Y., Luo, P., et al. (2024). Long-term mulched drip irrigation enhances the stability of soil aggregates by increasing organic carbon stock and reducing salinity. Soil Till. Res. 240, 106069. doi: 10.1016/j.still.2024.106069
Tang, X., Qiu, J. C., Xu, Y. Q., Li, J. H., Chen, J. H., Li, B., et al. (2022). Responses of soil aggregate stability to organic C and total N as controlled by land-use type in a region of south China affected by sheet erosion. Catena 218, 106543. doi: 10.1016/j.catena.2022.106543
Thomas, R. Q., Canham, C. D., Weathers, K. C., Goodale, C. L. (2009). Increased tree carbon storage in response to nitrogen deposition in the US. Nat. Geosci. 3, 13–17. doi: 10.1038/ngeo721
Tisdall, J. M., Oades, J. M. (1982). Organic matter and water-stable aggregates in soils. J. Soil Sci. 33, 141–163. doi: 10.1111/j.1365-2389.1982.tb01755.x
Vitousek, P. M., Menge, D. N. L., Reed, S. C., Cleveland, C. C. (2013). Biological nitrogen fixation: rates, patterns and ecological controls in terrestrial ecosystems. Philos. Trans. R. Soc B-Biol. Sci. 368, 20130119. doi: 10.1098/rstb.2013.0119
Wang, M., Gong, Y., Lafleur, P., Wu, Y. (2021b). Patterns and drivers of carbon, nitrogen and phosphorus stoichiometry in southern China’s grasslands. Sci. Total Environ. 785, 147201. doi: 10.1016/j.scitotenv.2021.147201
Wang, S. Q., Li, T. X., Zheng, Z. C. (2018). Tea plantation age effects on soil aggregate-associated carbon and nitrogen in the hilly region of western Sichuan, China. Soil Till. Res. 180, 91–98. doi: 10.1016/j.still.2018.02.016
Wang, C. T., Sun, Y., Chen, H. Y., Ruan, H. H. (2021a). Effects of elevated CO2 on the C:N stoichiometry of plants, soils, and microorganisms in terrestrial ecosystems. Catena 201, 105229. doi: 10.1016/j.catena.2021.105219
Wang, Z. T., Yang, L., Li, G., Chai, C. S., Zhang, Y. D., Chen, R., et al. (2019). Effects of alfalfa (Medicago sativa L.) degradation on herbage distribution and diversity in the semi-arid Loess Plateau. Acta Ecol. Sin. 39, 3720–3729. doi: 10.5846/stxb201805181099
Wang, W., Zhou, R., Wang, B. Z., Zhao, L., Zhao, Z. Y., Sheteiwy, M. S., et al. (2022). Biocrust as a nature-based strategy (NbS) to restore the functionality of degraded soils in semiarid rainfed alfalfa (Medicago sativa L.) field. J. Clean. Prod. 336, 130378. doi: 10.1016/j.jclepro.2022.130378
Wilson, G. W. T., Rice, C. W., Rillig, M. C., Springer, A., Hartnett, D. C. (2009). Soil aggregation and carbon sequestration are tightly correlated with the abundance of arbuscular mycorrhizal fungi: results from long-term field experiments. Ecol. Lett. 12, 452–461. doi: 10.1111/j.1461-0248.2009.01303.x
Xiao, L., Zhang, Y., Li, P., Xu, G. C., Shi, P., Zhang, Y. (2019). Effects of freeze-thaw cycles on aggregate-associated organic carbon and glomalin-related soil protein in natural-succession grassland and Chinese pine forest on the Loess Plateau. Geoderma 334, 1–8. doi: 10.1016/j.geoderma.2018.07.043
Xu, H., Wang, M., You, C., Tan, B., Xu, L., Li, H., et al. (2024). Warming effects on C:N:P stoichiometry and nutrient limitation in terrestrial ecosystems. Soil Till. Res. 235, 105896. doi: 10.1016/j.still.2023.105896
Xu, M. X., Wang, Z., Zhang, J., Liu, G. B. (2012). Response of soil organic carbon sequestration to the “Grain for Green Project” in the hilly Loess Plateau region. Acta Ecologica Sin. 32, 5405–5415. doi: 10.5846/stxb201112312027
Yahui, J., Le, Y., Xuesong, C., Feiran, C., Jing, L., Jiangshan, Z., et al. (2023). Carbon dots promoted soybean photosynthesis and amino acid biosynthesis under drought stress: reactive oxygen species scavenging and nitrogen metabolism. Sci. Total Environ. 856, 159125–159125. doi: 10.1016/j.scitotenv.2022.159125
Yang, D., Song, L., Jin, G. (2019). The soil C:N:P stoichiometry is more sensitive than the leaf C:N:P stoichiometry to nitrogen addition: a four-year nitrogen addition experiment in a Pinus koraiensis plantation. Plant Soil 442, 183–198. doi: 10.1007/s11104-019-04165-z
Yu, H., Gao, R. Y., Yang, W. J., Yang, L. S., Li, S. Y., Lin, Y. M., et al. (2021). Contents and relationships of C, N, P of leaf, root and soil in dominant herbs in dry-hot valley. Chin. J. Appl. Environ. Biol. 28 (3), 727–735. doi: 10.19675/j.cnki.1006-687x.2021.01008
Yuan, Z. Q. (2017). Factors affecting lucerne-rich vegetation under revegetation in a semi-arid environment. Ecol. Eng. 108, 249–254. doi: 10.1016/j.ecoleng.2017.08.030
Yuan, S., Xu, Q., Zhao, K., Zhou, Q., Wang, X., Zhang, X., et al. (2024). Dynamic analyses of soil erosion and improved potential combining topography and socio-economic factors on the Loess Plateau. Ecol. Indic. 160, 111814. doi: 10.1016/j.ecolind.2024.111814
Zhang, Y., Li, C., Wang, M. (2019). Linkages of C: N: P stoichiometry between soil and leaf and their response to climatic factors along altitudinal gradients. J. Soils Sediments 19, 1820–1829. doi: 10.1007/s11368-018-2173-2
Zhang, J. H., Wang, Y., Li, F. C. (2015). Soil organic carbon and nitrogen losses due to soil erosion and cropping in a sloping terrace landscape. Soil Res. 53, 87–56. doi: 10.1071/SR14151
Zhang, L. Q., Wei, X. R., Hao, M. D., Zhang, M. (2015). Changes in aggregate-associated organic carbon and nitrogen after 27 years of fertilization in a dryland alfalfa grassland on the Loess Plateau of China. J. Arid Land 7, 429–437. doi: 10.1007/s40333-015-0003-6
Zhang, G. Q., Zhang, P., Peng, S. Z., Chen, Y. M., Cao, Y. (2017). The coupling of leaf, litter, and soil nutrients in warm temperate forests in northwestern China. Sci. Rep. 7, 11754. doi: 10.1038/S41598-017-12199-5
Zhang, X. L., Zhao, Y. Y., Gao, W. J., Song, X., Zhang, X. T., Shi, X. Y., et al. (2021). Converting alfalfa pasture into annual cropland achieved high productivity and kept soil organic carbon in a semiarid area. Land Degrad. Dev. 32, 1478–1486. doi: 10.1002/ldr.3808
Zhao, R. N., Kuzyakov, Y., Zhang, H. Y., Wang, Z. R., Li, T. P., Shao, L. Y., et al. (2024). Labile carbon inputs offset nitrogen-induced soil aggregate destabilization via enhanced growth of saprophytic fungi in a meadow steppe. Geoderma 443, 116841. doi: 10.1016/j.geoderma.2024.116841
Zhou, G., Xu, S., Ciais, P., Manzoni, S., Fang, J., Yu, G., et al. (2019). Climate and litter C/N ratio constrain soil organic carbon accumulation. Nati. Sci. Rev. 6, 746–757. doi: 10.1093/nsr/nwz045
Keywords: alfalfa field, soil aggregates, carbon and nitrogen stoichiometry, degradation, semiarid region
Citation: Wang W, Tian T, Li M-Y, Wang B-Z, Mei F-J, Li J-Y, Wang N, Yang Y-M, Zhang Q, Tao H-Y, Zhu L and Xiong Y-C (2024) Carbon and nitrogen stoichiometry across plant–soil system accounts for the degradation of multi-year alfalfa grassland. Front. Plant Sci. 15:1400261. doi: 10.3389/fpls.2024.1400261
Received: 13 March 2024; Accepted: 26 August 2024;
Published: 18 October 2024.
Edited by:
Khurram Shahzad, Lasbela University of Agriculture, Water and Marine Sciences, PakistanReviewed by:
Muhammad Qayyum, Bahauddin Zakariya University, PakistanQamar Sarfaraz, Lasbela University of Agriculture, Water and Marine Sciences, Pakistan
Muhammad Baqir Hussain, MNS University of Agriculture, Multan, Pakistan
Copyright © 2024 Wang, Tian, Li, Wang, Mei, Li, Wang, Yang, Zhang, Tao, Zhu and Xiong. This is an open-access article distributed under the terms of the Creative Commons Attribution License (CC BY). The use, distribution or reproduction in other forums is permitted, provided the original author(s) and the copyright owner(s) are credited and that the original publication in this journal is cited, in accordance with accepted academic practice. No use, distribution or reproduction is permitted which does not comply with these terms.
*Correspondence: You-Cai Xiong, eGlvbmd5Y0BsenUuZWR1LmNu; Li Zhu, emh1bGlAaGdudS5lZHUuY24=
†Present address: You-Cai Xiong, State Key Laboratory of Herbage Improvement and Grassland Agro-Ecosystems, College of Ecology, Lanzhou University, Lanzhou, China
Li Zhu, State Key Laboratory of Herbage Improvement and Grassland Agro-Ecosystems, College of Ecology, Lanzhou University, Lanzhou, China
‡These authors have contributed equally to this work