- School of Tropical Agriculture and Forest, National Key Laboratory of Tropcial Crop Breeding, Hainan University, Haikou, China
During the postharvest storage of tomatoes, they are susceptible to infection by Botrytis cinerea, leading to significant economic losses. This study evaluated the antifungal potential of 2-heptanol (2-HE), a volatile biogenic compound, against B. cinerea and explored the underlying antifungal mechanism. The results indicated that 2-HE effectively suppressed the growth of B. cinerea mycelia both in vivo and in vitro and stimulated the activities of antioxidative enzymes, including superoxide dismutase (SOD), peroxidase (POD), and catalase (CAT) in tomatoes. Furthermore, 2-HE reduced spore viability, compromised membrane integrity, and resulted in increased levels of extracellular nucleic acids, protein content, and membrane lipid peroxidation. Transcriptomic analysis revealed that 2-HE disrupted the membrane transport system and enhanced amino acid metabolism, which led to intracellular nutrient depletion and subsequent B. cinerea cell death. Additionally, the 2-HE treatment did not negatively impact the appearance or quality of the tomatoes. In conclusion, the findings of this study offer insights into the use of 2-HE as a biocontrol agent in food and agricultural applications.
1 Introduction
Postharvest decay of fruits and vegetables due to pathogen infection results in significant economic losses (Leng et al., 2022; Hosseini et al., 2023). Botrytis cinerea is a prevalent pathogen responsible for such decay, with the ability to infect over 200 plant species (Yu et al., 2018). Tomatoes, being rich in carotene and vitamin C, are one of the most vital agro-industrial crops (Szabo et al., 2021). The infection of B. cinerea is a primary factor impairing tomato quality postharvest (Williamson et al., 2007; Jiao et al., 2022). Currently, chemical fungicides are the most widespread agents used to control postharvest decay in fruits and vegetables (Chen and Ying, 2015). Nonetheless, the overuse of chemical fungicides has led to critical issues, including threats to food safety, environmental pollution, and increased resistance in fungi (Zhang X. et al., 2020; Zubrod et al., 2019). Consequently, there is an urgent need for safe biological antifungal agents to manage B. cinerea infections and mitigate the economic losses associated with postharvest tomatoes.
Recent research has highlighted the efficacy of bio-derived antifungal agents, deemed safe, in managing postharvest pathogens. Examples include magnolol (Cui et al., 2021), 3-methylthio-1-propanol (Feng et al., 2021), and curcumin (Hua et al., 2019). 2-Heptanol (2-HE), a volatile organic compound (VOC) produced by a range of microorganisms, plants, and animals (Du et al., 2022; Chang et al., 2023), is notable for its contribution to the aroma of gamma-aminobutyric acid white tea (Li et al., 2023) and has been identified in Rubus coreanus fruits (Yu et al., 2019), chicken sausages (Ozkara et al., 2019), and strains of Aureobasidium pullulans (Shi et al., 2023). In agriculture, 2-HE deters virulent green rice leafhoppers from colonizing rice plants infected with the rice dwarf virus (RDV) due to its repulsive properties (Chang et al., 2021). It also displays substantial repellent activity against pests such as Tribolium castaneum and Rhyzopertha dominica (Ukeh and Urnoetok, 2011). Moreover, 2-HE has demonstrated antifungal effects, for instance, reducing Fusarium crown and root rot incidence in tomato plants (Du et al., 2022), though its antifungal mechanisms merit further investigation. According to previous studies (Du et al., 2022; Shi et al., 2023), 2-HE has great biocontrol potential for controlling postharvest tomato diseases.
To our knowledge, the antifungal mechanisms of 2-HE have remained uncharacterized until now. This study employed both in vitro and in vivo experiments to assess the inhibitory effects of 2-HE on B. cinerea. The antifungal mechanisms underlying these effects were investigated using fluorescence microscopy, transcriptome analysis, and quantitative PCR (qPCR). The outcomes of this study will offer novel avenues for biological control and will serve as essential references for formulating ecological management strategies.
2 Materials and methods
2.1 Reagents, strains, and tomato
2-Heptanol (2-HE) (purity = 98%; CAS. No. 543–49-7) was commercially available from Macklin (Macklin Biochemical Co., Ltd., Shanghai, China). The fungal pathogen B. cinerea B05.10 was provided by Prof. Fangjie Xiong, Southwest University (Chongqing, China). Commercially mature tomatoes were harvested from Lingshui Farm (Hainan Province, China) and transported to the laboratory. Tomatoes with no damage and the same ripeness were cleaned with sterile water and then dried naturally for later use.
2.2 Antifungal activity of 2-HE against B. cinerea in vitro
The inhibitory activity of 2-HE on B. cinerea mycelium growth was evaluated using a non-contact assay. A B. cinerea mycelial plug (5-mm diameter) was placed on the side of the two-part plates containing potato dextrose agar medium, and 0, 5, 10, 15, and 20 µL of 2-HE were added to the other side of the two-part plates with filter paper (10 × 75 mm). The concentration conversion is shown in Table 1 (Su and Liang, 2015). The 0 treatment was treated with an equal volume of distilled water instead of the maximum dose of the 2-HE treatment in the control group (0.21 μL cm-3). Then the dichotomous Petri dish was quickly sealed and cultured at 25°C for 5 days. The cross method was used to measure the colony diameter and calculate the inhibition rate. At each concentration, there are three biological replicates.
2.3 Inhibitory efficacy of 2-HE against B. cinerea in vivo
Disease-free tomatoes of uniform size were cleansed with sterile distilled water and subsequently left to air-dry before further experimentation. After scraping the tomato equator with a sterile needle, the tomato wound was inoculated with a 3.0-mm B. cinerea mycelium plug. A Petri dish containing 2-HE at concentrations of 0 (distilled water as a control), 0.02, 0.05, 0.10, or 0.16 μL cm-3 was positioned at the base of a sealed glass chamber, with 20 inoculated tomatoes arranged on the perforated partition in the center of the chamber (Chen et al., 2022). After treatment, the diameter of the lesions on each tomato was recorded daily and sampled. During sampling, fruit tissue around the inoculation site (2 × 2 × 2 cm) was collected after removing the mycelium plug on the tomato surface. Each process was repeated three times.
2.4 Antioxidant enzyme activity and MDA content measurement
The samples collected in Section 2.3 were measured for antioxidant activity and malondialdehyde (MDA) content. Catalase (CAT), peroxidase (POD), superoxide dismutase (SOD) activity, and MDA content were determined by the CAT, POD, SOD, and MDA assay kit (Nanjing Jiancheng Bioengineering Institute, China). The test was performed according to the instructions of each kit, and the entire experiment was performed at 4°C. Each treatment was subjected to triplicate measurements to ensure experimental reliability.
2.5 Effects of 2-HE on tomato quality
To measure the effects of 2-HE on tomato quality, tomatoes with uniform size and maturity and without disease were washed and dried. A Petri dish containing 2-HE at concentrations of 0 (distilled water as a control), 0.02, 0.05, 0.10, or 0.16 μL cm-3 was positioned at the base of a sealed glass chamber, with 20 tomatoes arranged on the perforated partition in the center of the chamber (Chen et al., 2022). Tomatoes were collected on days 1, 2, 3, and 4 for the assessment of total soluble solids (TSS) and titratable acidity (TA). TSS content was quantified using a handheld refractometer (Atago, Japan), and the TA was ascertained through titration of tomato juice with a 0.01-M NaOH solution. Each treatment was conducted in triplicate.
2.6 Fluorescence microscopy
Cell viability and membrane integrity were assessed using fluorescein diacetate (FDA, Sigma) and propyl iodide (PI, Sigma) (Wang et al., 2020). A 10-µL spore suspension was spotted onto the inner surface of a Petri dish lid, followed by the introduction of varying 2-HE concentrations (0, 0.05, and 0.16 μL cm-3) to the base of the dish. The cultures were incubated at 25°C for 12 h. Post-incubation, spores were stained with FDA for 5 min and with PI for 10 min, both in darkness, at 25°C. The spores were then washed three times using phosphate-buffered saline (PBS) and examined under a fluorescence microscope (Zeiss, Germany). For each treatment, five random fields of view were chosen to quantify the number of stained spores.
2.7 MDA content and cellular inclusion leakage detection
Following a 5-day incubation of spores in potato dextrose broth at 25°C, 2.0 g of mycelium was harvested and resuspended in sterile water laced with 2-HE concentrations (0, 0.05, and 0.16 μL cm-3). After incubation for different times (0, 3, 6, 9, and 12 h), the supernatant and mycelium were collected, frozen with liquid nitrogen for 10 min, and then put into the refrigerator at -80°C for use. The amount of nucleic acid leakage was detected with supernatant at 260 nm (OD260), and the protein leakage was measured with a Bradford Protein Assay Kit (Nanjing Jiancheng Bioengineering Institute, China). Membrane lipid peroxidation was evaluated using MDA as an indicator (Ma et al., 2019). MDA content was determined by the MDA assay kit (Nanjing Jiancheng Bioengineering Institute, China). Each treatment was conducted in triplicate.
2.8 Transcriptomic analysis
To elucidate the global transcriptomic alterations induced by 2-HE treatment in B. cinerea, mycelia exposed to 2-HE (0.05 µL cm-3) and control groups treated with an equivalent volume of sterile distilled water were submitted to Megge Biotechnology (Shanghai, China) for transcriptome analysis. Transcriptome sequencing was performed using the Illumina sequencing platform, and differential expression genes (DEGs) were analyzed using the DESeq2 R package (screening criteria: padj ≤ 0.05). All identified DEGs were further analyzed by gene ontology (GO) and Kyoto Encyclopedia of Genes (KEGG) enrichment.
2.9 qPCR
To validate the RNA-seq results, six differentially expressed genes (DEGs) were chosen for qPCR verification. Mycelia total RNA was isolated using the Fungal RNA Kit (Omega, USA). qPCR amplification employed the RR820A and RR047A reagents from Baori Medical Technology Co., Ltd. (Beijing, China). Reference genes were selected based on Hua et al. (2019), and additional primer sequences were designed via Primer Premier 6 software (Premier, Canada), detailed in Supplementary Table S1. Bcactin served as the internal reference gene, and relative gene expression levels were calculated using the 2-ΔΔCt method. The qPCR operational parameters are provided in Supplementary Table S2. Each experimental condition was replicated three times to ensure consistency.
2.10 Statistical analysis
The experimental data in the manuscript were analyzed using SPSS software (version 25.0) (IBM, New York, United States). Each result was represented by means ± standard error (SE), followed by variance analysis using Duncan’s multiple range test and T-test, and a significant difference (P < 0.05) was indicated with different lowercase letters.
3 Results
3.1 Effect of 2-HE on B. cinerea mycelial growth
2-HE significantly inhibited B. cinerea mycelial growth in a dose-dependent manner (Figure 1). At a concentration of 2-HE of 0.05 µL cm-3, the inhibition rate of growth was recorded at 18.35%. Notably, mycelial expansion was entirely halted at the 0.21 µL cm-3 2-HE treatment, at which point the relative inhibition rate escalated to 100%. These findings demonstrate the potent inhibitory effect of 2-HE on B. cinerea mycelial growth.
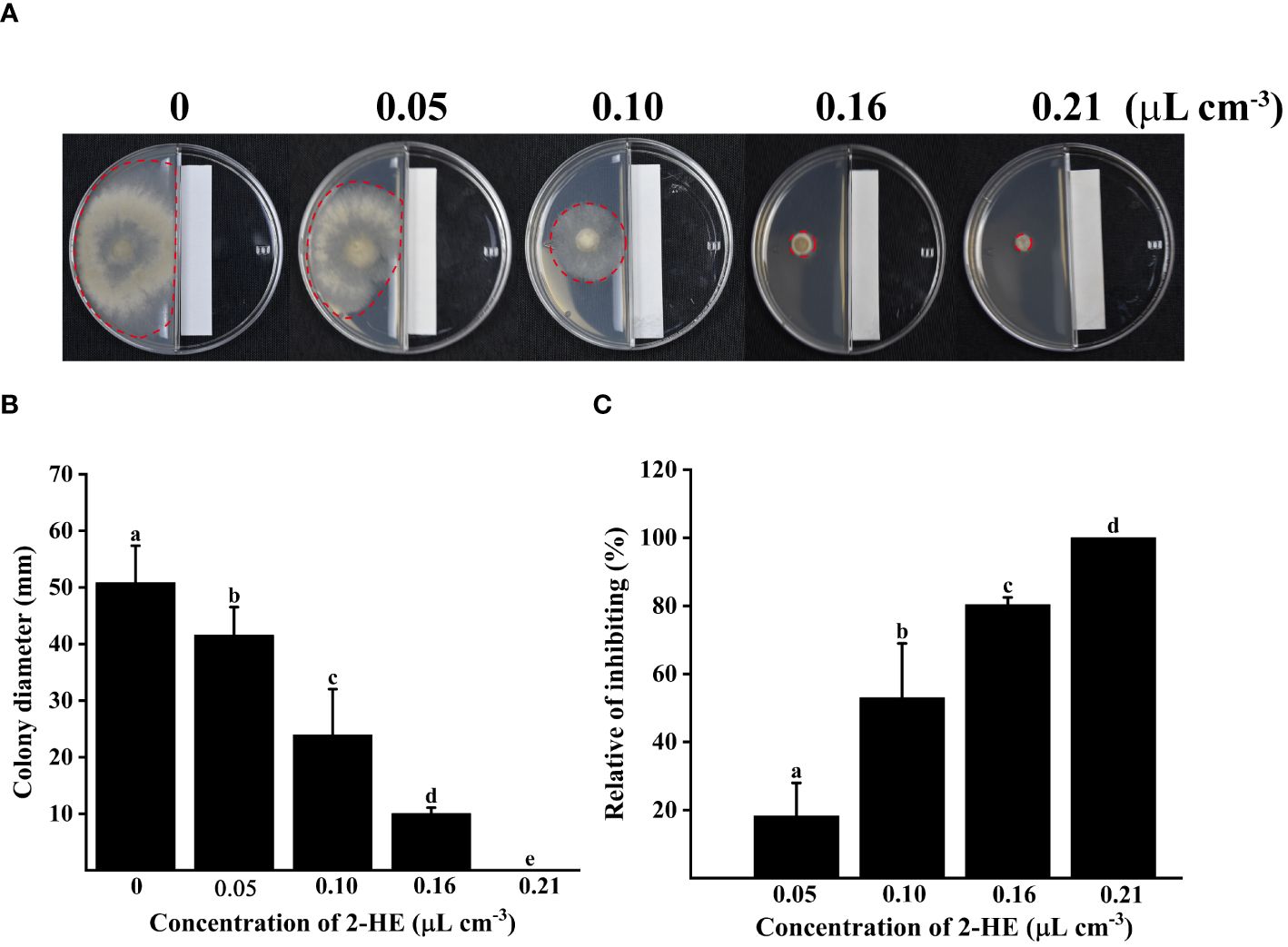
Figure 1 Varying concentrations of 2-HE (0, 0.05, 0.10, 0.16, and 0.21 μL cm-3) inhibited B. cinerea, in which 0 concentration was treated with an equal volume of distilled water instead of the maximum dose of 2-HE treatment (0.21 μL cm-3 H2O) as the control group. (A) Effect of 2-HE on the colony morphology of B. cinerea; (B) Colony diameter; (C) Relative of inhibiting. Duncan’s test was adopted, with different lowercase letters indicating significant differences (P<0.05), and the error bar in the figure represents the standard error.
3.2 Antifungal efficacy on harvested tomatoes
In this study, tomatoes were chosen as the subject to investigate the antifungal properties of 2-HE against gray mold in tomatoes. As shown in Figure 2, with increasing doses of 2-HE, the antifungal efficacy of 2-HE on tomatoes was significant in vivo, completely inhibiting gray mold at a dosage of 0.05 μL cm-3. In addition, lesions on tomatoes treated with 0.02 µL cm-3 of 2-HE were approximately 69.69% smaller in diameter than those on the control group by the fourth day. These results indicate that 2-HE possesses antifungal activity on postharvest tomatoes.
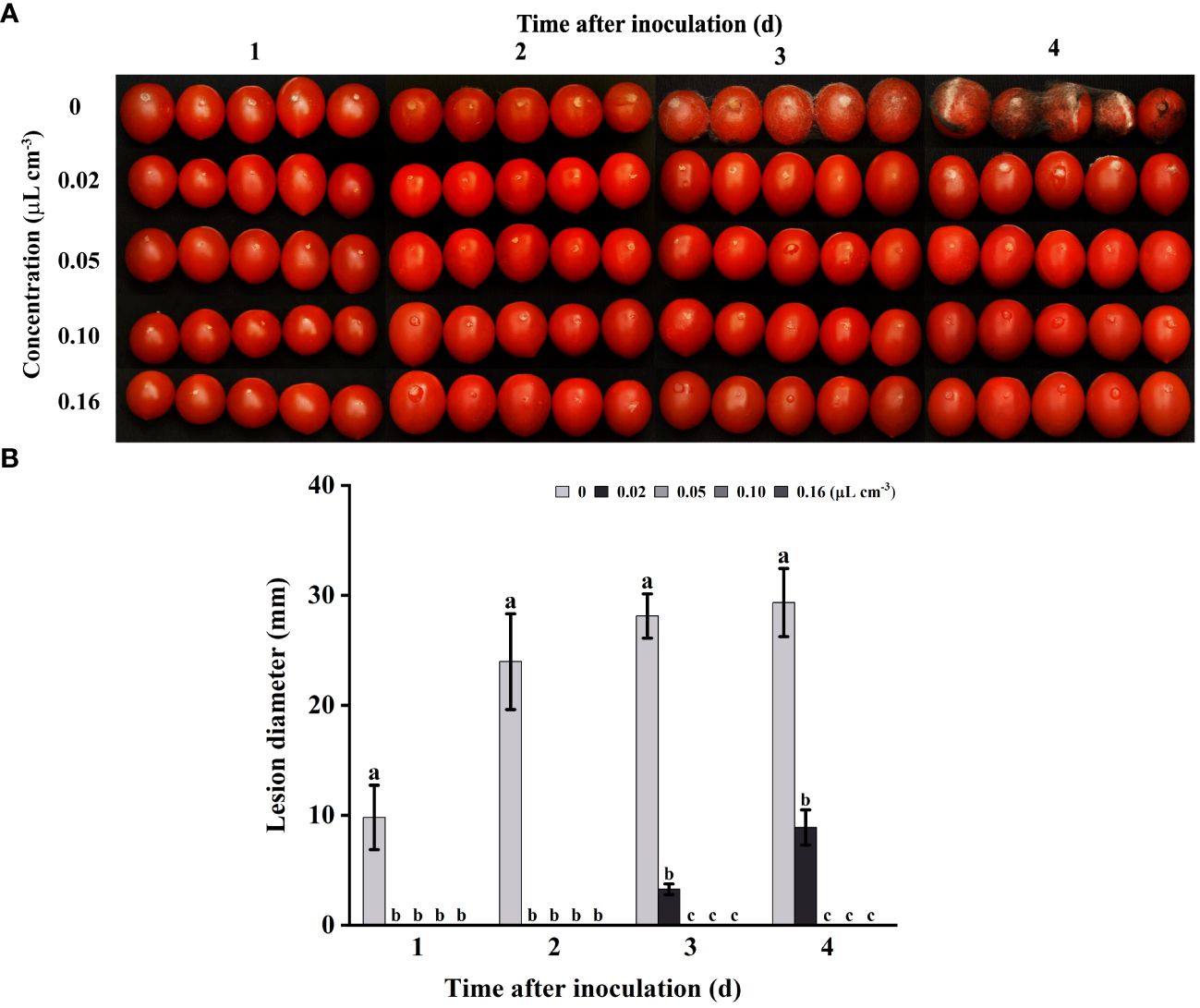
Figure 2 2-HE diminished the incidence of gray mold on tomatoes. (A) Tomatoes inoculated with B. cinerea and subsequently treated with varying concentrations of 2-HE (0, 0.02, 0.05, 0.10, and 0.16 μL cm-3, with the 0 µL cm-3 serving as the control); (B) Lesion diameter. Duncan’s test was adopted, with different lowercase letters indicating significant differences (P<0.05) and the error bar in the figure represents the standard error.
3.3 Effect of 2-HE on the antioxidant enzyme activity in tomatoes
As shown in Figure 3A, throughout the entire storage period monitored, catalase (CAT) activity in the tomato fruit treated with 0.16 µL cm-3 of 2-HE was significantly higher than that in the control group. In contrast, at a 2-HE concentration of 0.05 µL cm-3, the difference in CAT activity between the treated and control groups was not statistically significant. These findings suggest a concentration-dependent relationship between 2-HE and CAT activity activation in tomato fruits. In addition, peroxidase (POD) activity in samples treated with 2-HE at both 0.05 and 0.16 µL cm-3 was significantly higher than the control. Interestingly, on the first day, POD activity was significantly reduced in the 0.16 µL cm-3 2-HE treatment compared to the control (Figure 3B). With regard to superoxide dismutase (SOD) activity, treatment with 0.16 µL cm-3 of 2-HE resulted in significantly higher levels than the control at 1, 2, and 4 days; however, an unexpected decrease below control levels was observed at 2 days (Figure 3C).
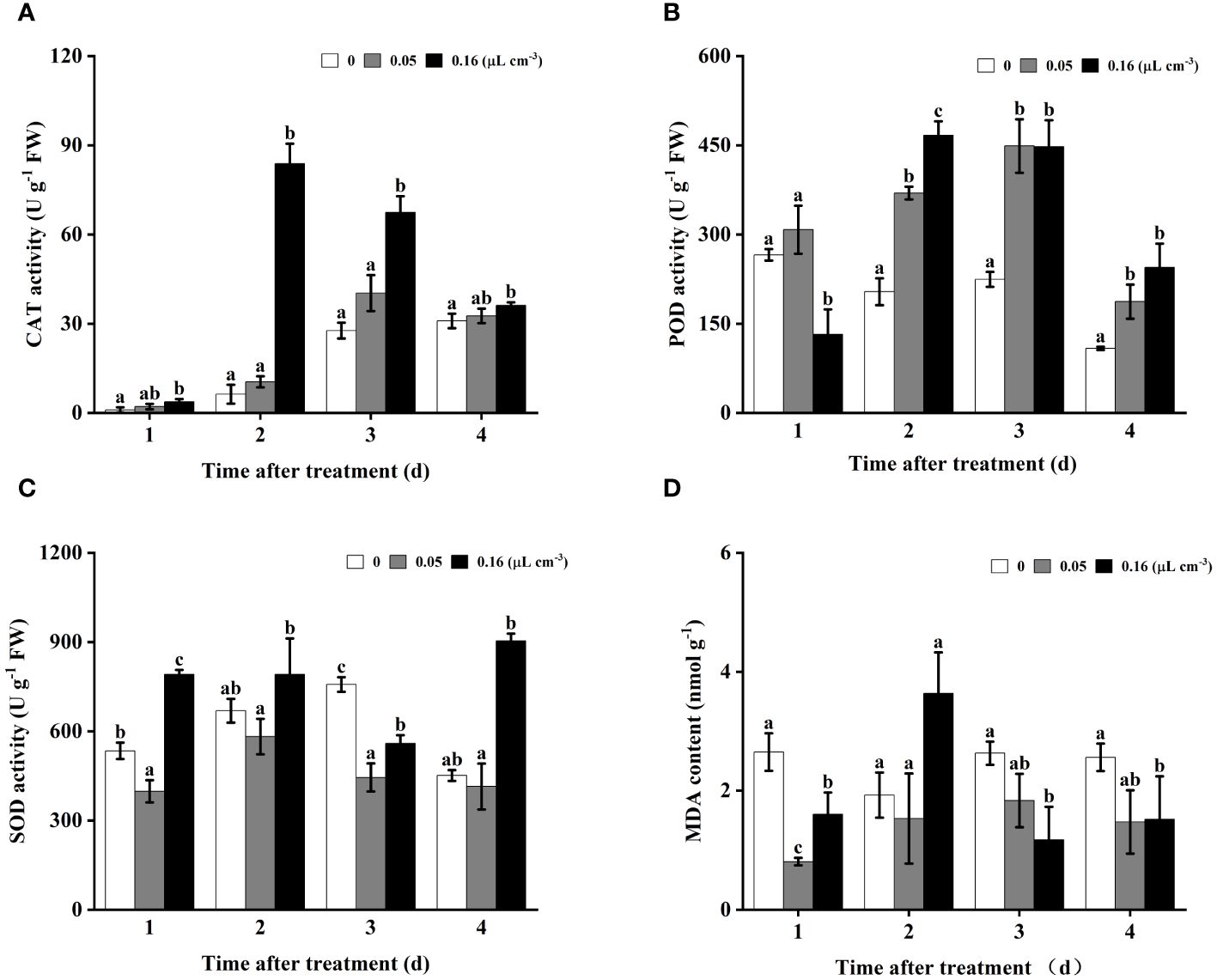
Figure 3 The antioxidant activities (SOD, POD, and CAT) and MDA content in tomatoes infected with B. cinerea were investigated at 2-HE concentrations of 0 (control), 0.05, and 0.16 μL cm-3. (A) CAT, (B) POD, (C) SOD, and (D) MDA content. Duncan’s test was adopted, with different lowercase letters indicating significant differences (P<0.05) and the error bar in the figure represents the standard error.
Malondialdehyde (MDA) content is a widely recognized marker of lipid peroxidation in cell membranes. The study revealed that at a 2-HE concentration of 0.05 µL cm-3, the MDA levels were significantly reduced compared to the control on the first day of the storage period; however, no significant differences were observed at 2–4 days. Conversely, with 2-HE at 0.16 µL cm-3, MDA levels were substantially lower than those of the control at 1, 3, and 4 days (Figure 3D).
3.4 Effects of 2-HE on tomato quality
As storage duration increased, no significant impact of 2-HE on tomato appearance was observed (Figure 4A). The influence of 2-HE on total soluble solids (TSS) content in healthy tomatoes was also evaluated. It was determined that 2-HE concentrations of 0.02, 0.05, and 0.08 µL cm-3 did not significantly affect TSS content at 1, 2, or 3 days of storage. Notably, during a 2-day storage period, varying 2-HE concentrations did not significantly alter the TSS, thereby effectively maintaining the TSS content in the tomato fruits (Figure 4B). Regarding titratable acid (TA), the content in tomatoes varied across different storage durations and 2-HE concentrations. On the first day, TA content increased significantly when treated with 2-HE at 0.02, 0.05, and 0.08 µL cm-3. Similar to TSS, TA levels did not change significantly on the second day and were not influenced by 2-HE concentration (Figure 4C).
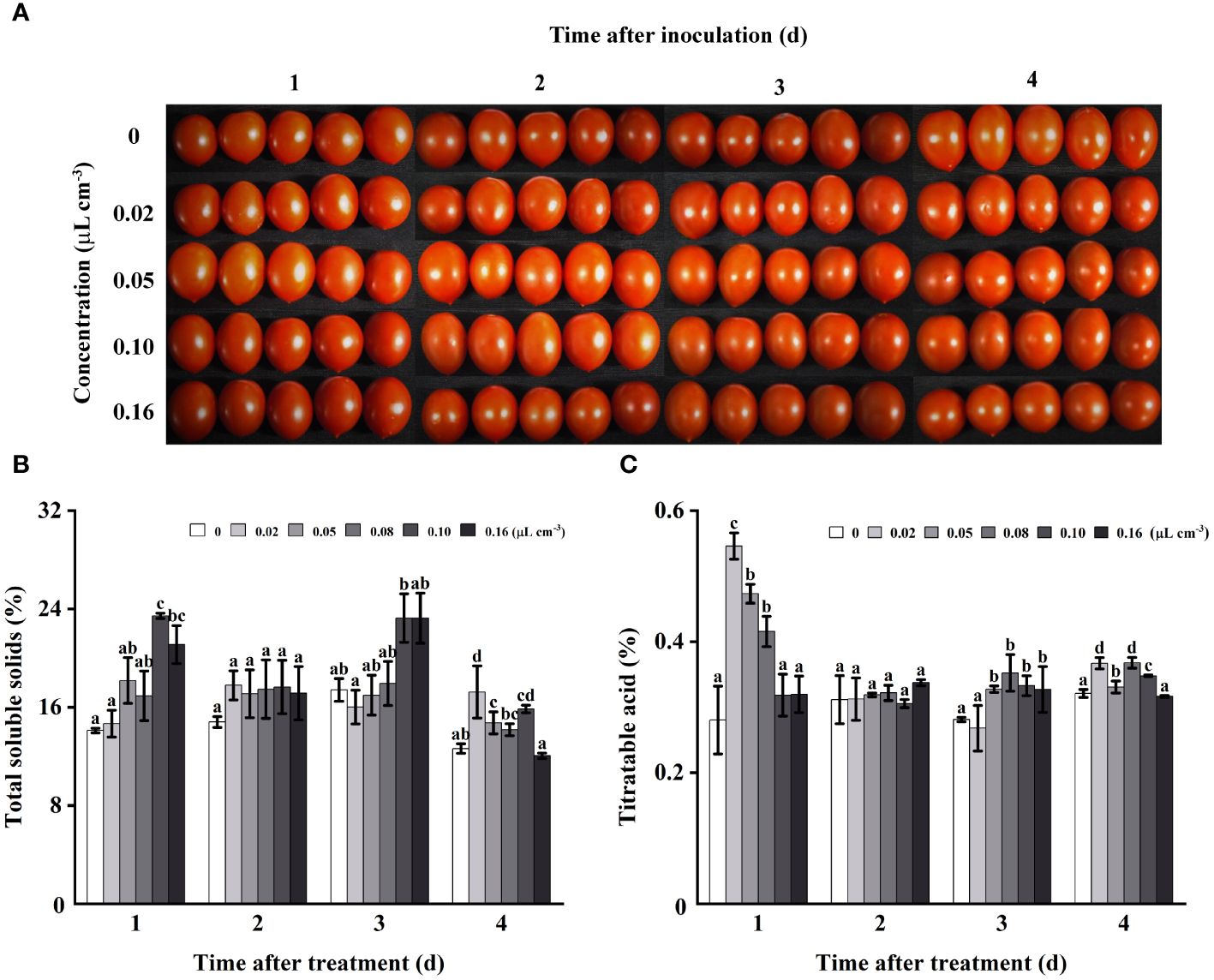
Figure 4 The appearance and contents of TSS and TA in healthy tomato fruits fumigated with varying 2-HE concentrations (0, 0.02, 0.05, 0.10, and 0.16 μL cm-3; this 0 μL cm-3 treatment used an equal volume of distilled water as the control group) were evaluated over a storage period of 1, 2, 3, and 4 days. (A) Effects of 2-HE on the tomato appearance; (B) TSS and (C) TA content in tomato. Duncan’s test was adopted, with different lowercase letters indicating significant differences (P < 0.05) and the error bar in the figure represents the standard error.
3.5 Effects of 2-HE on spore activity and membrane integrity of B. cinerea
The proportion of conidia stained with fluorescein diacetate (FDA) declined as the 2-HE concentration increased. At a 2-HE concentration of 0.16 µL cm-3, only 2.45% of the conidia were stained with FDA, suggesting a significant loss of viability (Figures 5A, B). Conversely, the proportion of conidia stained with propidium iodide (PI) increased inversely with the FDA results. With the 2-HE concentration at 0.16 µL cm-3, 49.9% of conidia were stained with PI, indicative of most conidia membrane disruption (Figures 5C, D). These findings demonstrate that 2-HE treatment reduces conidial viability and compromises membrane integrity.
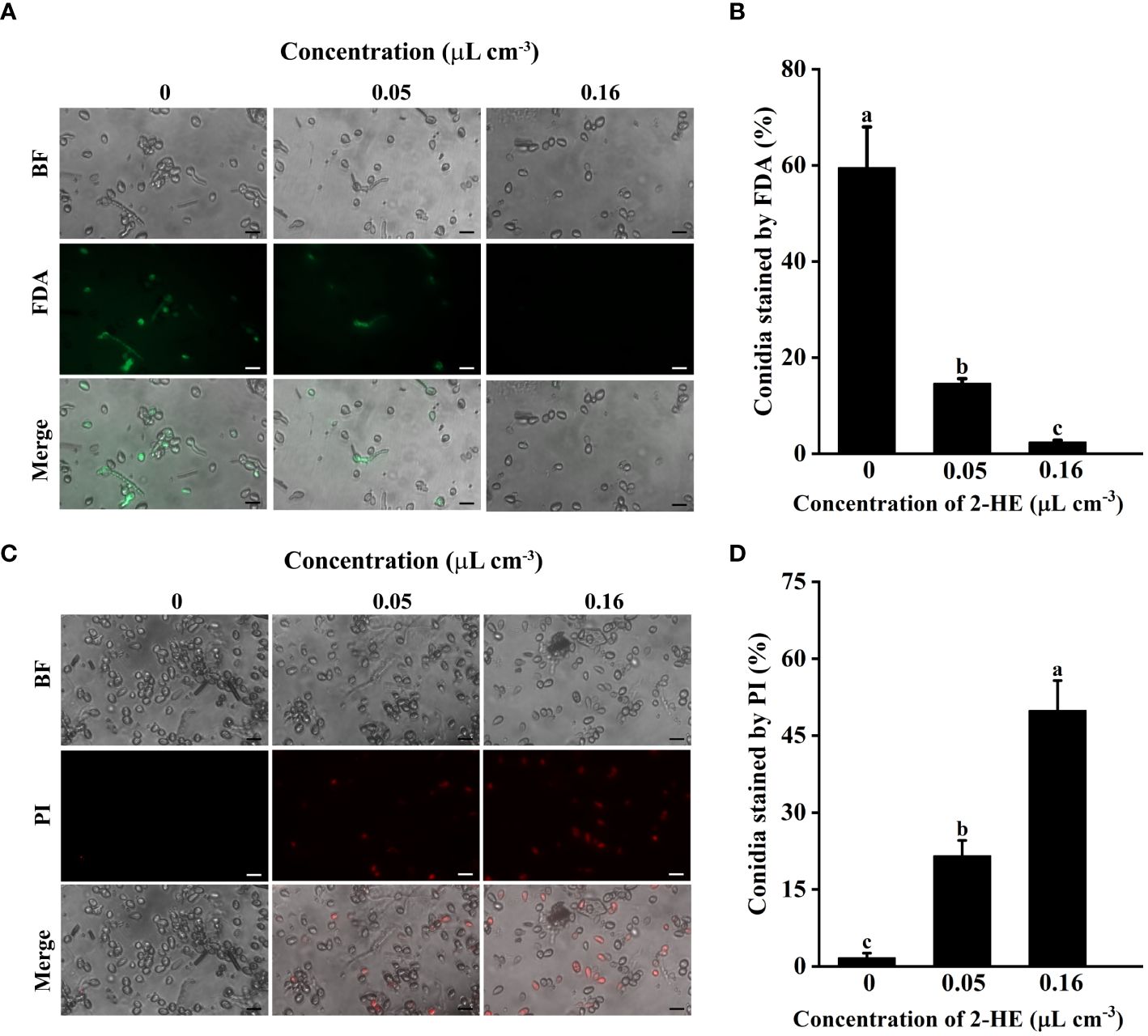
Figure 5 This study assessed conidial viability and membrane integrity following 2-HE treatment. (A) Effect of 2-HE treatment on the conidial activity of B. cinerea. Bar = 20 µm; (B) Conidia stained by FDA; (C) Effect of 2-HE treatment on the conidia membrane integrity of B. cinerea. Bar = 20 µm; (D) Conidia stained by PI. Duncan’s test was adopted, with different lowercase letters indicating significant differences (P<0.05) and the error bar in the figure represents the standard error.
3.6 Effect of 2-HE on cytoplasmic leakage and membrane lipid peroxidation
Given the high proportion of conidia stained by PI, we investigated nucleic acid and protein leakage as well as membrane lipid peroxidation in B. cinerea mycelia. As shown in Figure 6A, the control group exhibited lower levels of nucleic acid leakage than the 2-HE-treated group throughout the observation period. Comparatively, the content of nucleic acid leakage was positively correlated with the concentration of 2-HE; specifically, the group treated with 0.05 µL cm-3 had significantly less leakage than the 0.16 µL cm-3 group. Protein leakage was consistently higher in all 2-HE-treated groups than in the control group and peaked at 9 h of treatment (Figure 6B). Similarly, MDA levels in 2-HE-treated B. cinerea were significantly elevated compared to the control across all time points (Figure 6C). These findings suggest that 2-HE compromises the membrane integrity of B. cinerea, causing cytoplasmic leakage and membrane lipid peroxidation.
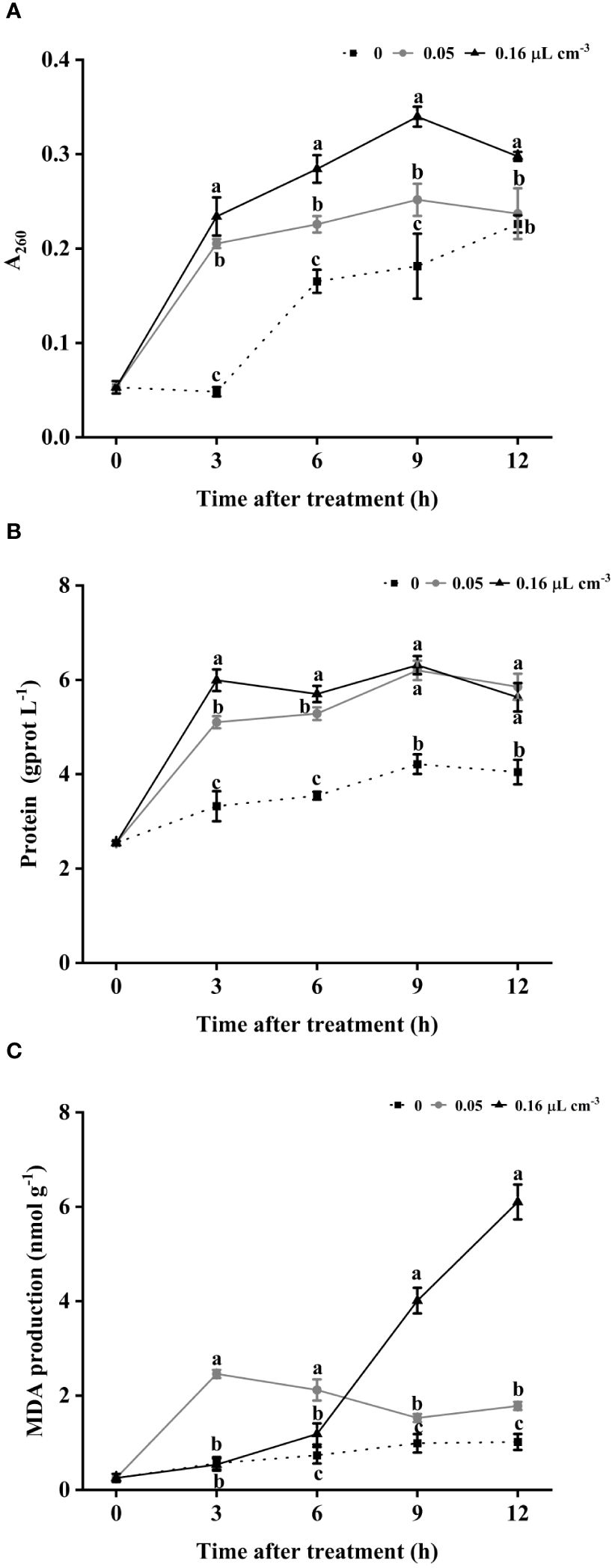
Figure 6 2-HE-induced cytoplasmic leakage and lipid peroxidation. (A) Nucleic acids; (B) soluble proteins; and (C) MDA content. Duncan’s test was adopted, with different lowercase letters indicating significant differences (P<0.05) and the error bar in the figure represents the standard error.
3.7 RNA-Seq analysis of B. cinerea under 2-HE stress
To elucidate the molecular mechanism of 2-HE antifungal activity, the transcriptome method was used to analyze the whole gene of B. cinerea under 2-HE stress. Following treatment with 0.05 μL cm-3 of 2-HE, various RNA data for B. cinerea were obtained by RNA-Seq analysis. Compared to the control, 1,642 transcripts (622 upregulated and 1,020 downregulated) exhibited significant differential expression in the 2-HE-treated group (Figure 7A), with the top 20 DEGs shown in Supplementary Table S3. Through GO analysis, it was revealed that these DEGs were predominantly associated with biological processes, including metabolic and cellular processes. Within the cellular component category, the DEGs were related to cell part, membrane part, membrane, and organelle. In terms of molecular function, a significant proportion of the DEGs were involved in catalytic activity, binding, and transporter activity (Figure 7B). These findings indicate that the DEGs of B. cinerea treated with 2-HE primarily regulate cellular processes, metabolic processes, cell parts, membrane parts, membranes, organelles, catalytic activity, binding, and transporter activity.
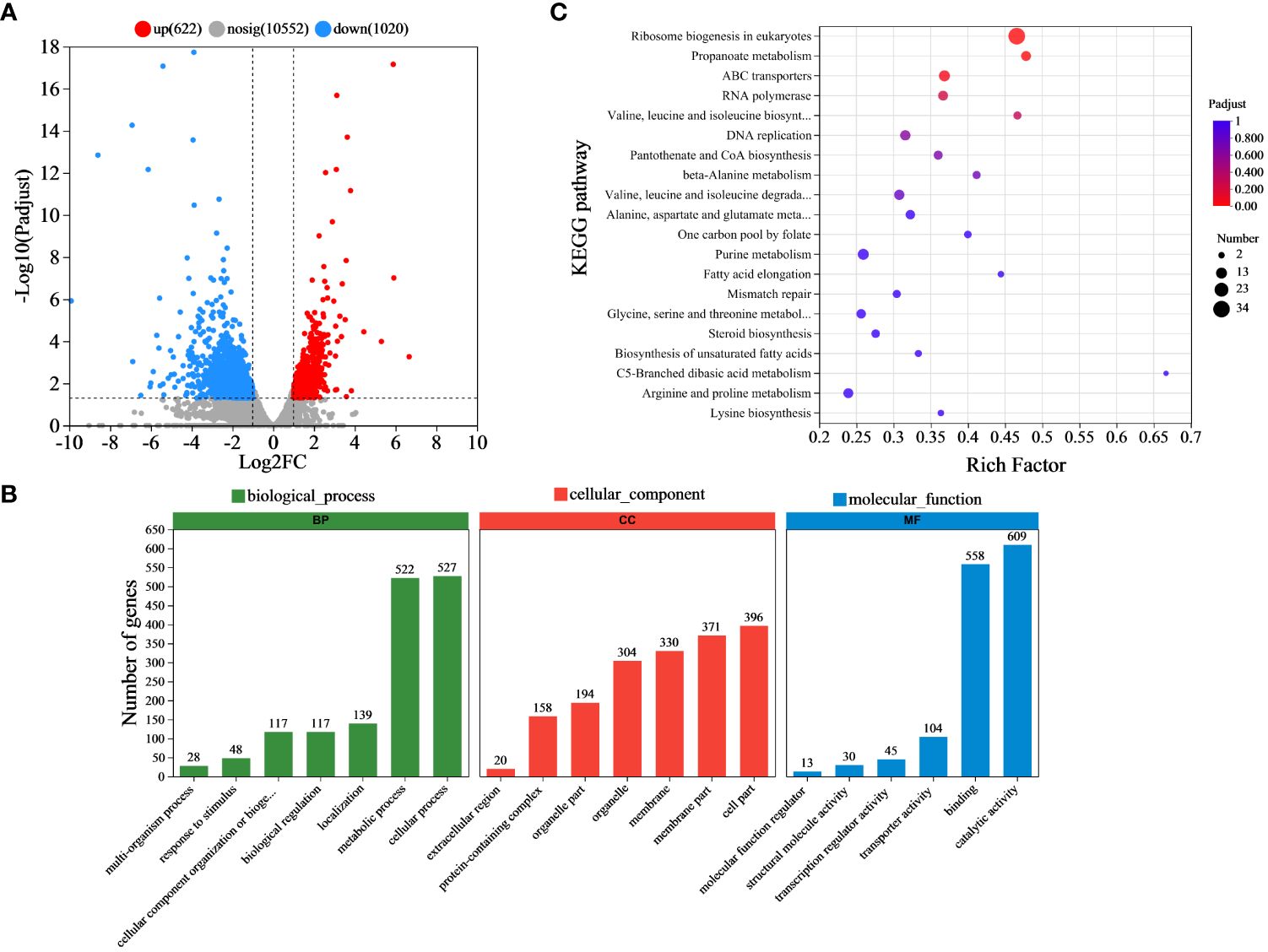
Figure 7 DEGs analysis. (A) Differential gene expression was analyzed by a volcano map, including 622 upregulated genes and 1,020 downregulated genes. (B) GO function classification statistics. (C) KEGG enrichment pathway.
The KEGG pathway enrichment analysis was performed to categorize the DEGs and further investigate the molecular mechanisms underlying the antifungal activity of 2-HE against B. cinerea (Figure 7C). The analysis identified the top 20 significantly enriched pathways (Supplementary Table S4), which predominantly included ribosome biogenesis in eukaryotes (map03008), propanoate metabolism (map00640), ABC transporters (map02010), and RNA polymerase (map03020). Additionally, several amino acid-related metabolic pathways were enriched, notably those involved in the biosynthesis and degradation of valine; leucine and isoleucine biosynthesis (map00290); valine, leucine, and isoleucine degradation (map00280); beta-alanine metabolism (map00410); alanine, aspartate, and glutamate metabolism (map00250); lysine biosynthesis (map00300); glycine, serine, and threonine metabolism (map00260); and arginine and proline metabolism (map00330). Validation of the relative expression levels of selected genes corroborated the transcriptomic findings, as indicated by a high correlation coefficient of 0.96668, thus confirming the reliability of the transcriptome data (Supplementary Figure S1).
3.8 DEGs related to amino acid metabolism and ABC transporters
Analysis of the top 20 enriched metabolic pathways via KEGG revealed that six pathways were associated with amino acid metabolism. To further elucidate the molecular mechanism of 2-HE in B. cinerea, TBtools was employed to construct a heatmap of differential gene expression across these six amino acid metabolism pathways. The valine, leucine, and isoleucine biosynthesis pathway (map00290) exhibited enrichment for seven DEGs, six of which (Bcin10g05310, Bcin01g00210, Bcin04g02200, Bcin04g03100, Bcin16g02820, and Bcin04g01520) were upregulated, suggesting an acceleration of these biosynthetic processes by 2-HE treatment (Figure 8A; Supplementary Table S5). Concomitantly, the pathway for degradation of valine, leucine, and isoleucine (map00280) was also activated, as evidenced by 12 upregulated DEGs (Figure 8B; Supplementary Table S6). Similarly, the pathways for alanine, aspartate, and glutamate metabolism (map00250); lysine biosynthesis (map00300); and glycine, serine, and threonine metabolism (map00260) were enriched with 10, 4, and 10 upregulated DEGs, respectively (Figures 8C–E; Supplementary Tables S7-S10). Under 2-HE treatment, 11 DEGs contributing to arginine and proline metabolism (map00330) were identified, with seven (Bcin11g06270, Bcin04g00260, Bcin05g08050, Bcin04g03360, Bcin02g03180, Bcin13g05810, Bcin09g03430) showing significant upregulation and four (Bcin07g00340, Bcin13g00080, Bcin01g06600, Bcin02g05730) being significantly downregulated (Figure 8F; Supplementary Table S10). In summary, these findings indicate that 2-HE treatment enhances amino acid metabolism in B. cinerea.
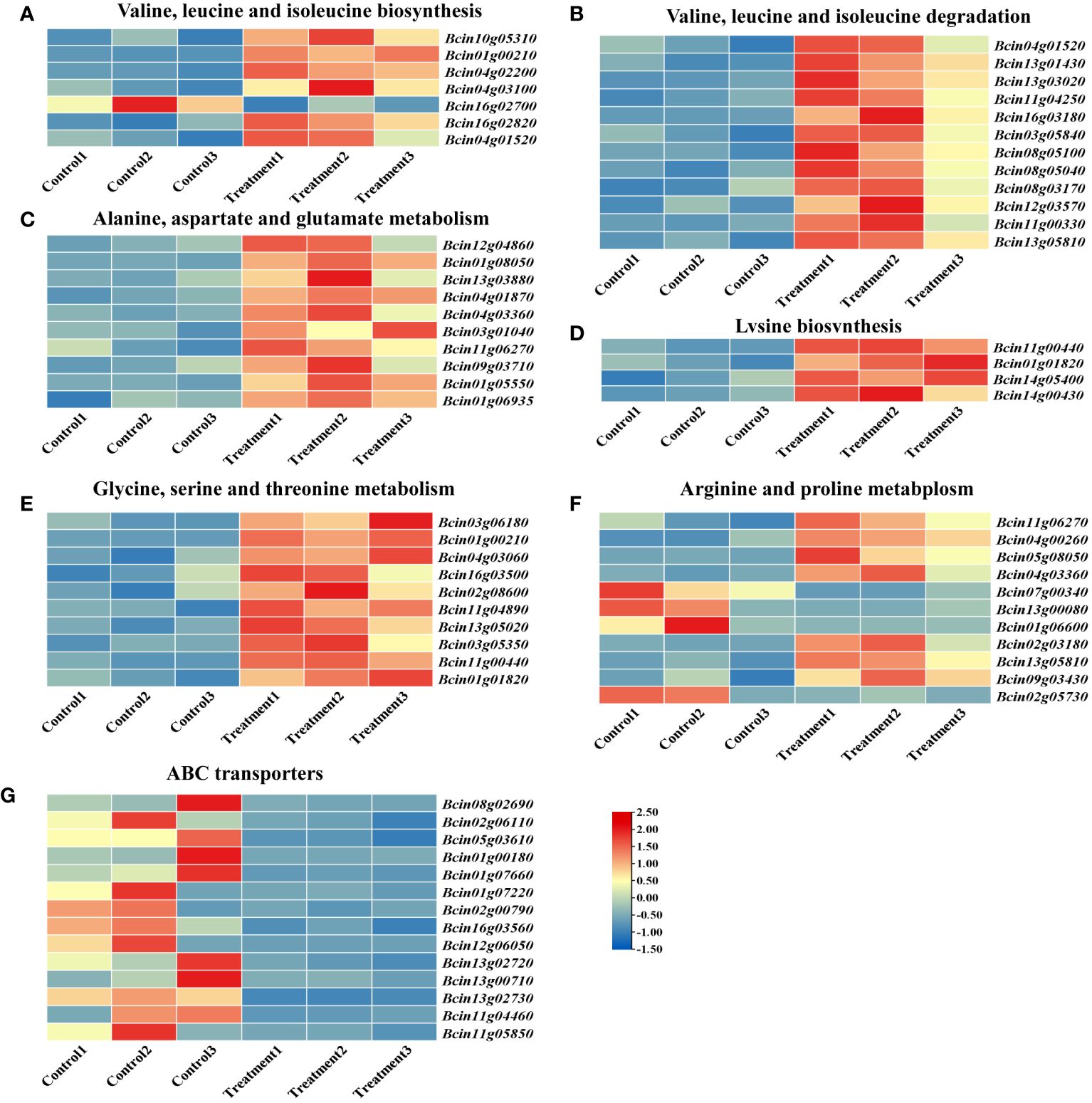
Figure 8 Heatmap of the gene expression for KEGG pathway-enriched amino acid metabolism. (A) valine, leucine, and isoleucine biosynthesis; (B) valine, leucine, and isoleucine degradation; (C) alanine, aspartate, and glutamate metabolism; (D) lysine biosynthesis; (E) glycine, serine, and threonine metabolism; (F) arginine and proline metabolism; and (G) ABC transporters.
KEGG pathway enrichment analysis indicated that 2-HE treatment also affected the membrane transport system of B. cinerea. ABC transporters, which utilize the energy derived from ATP hydrolysis to facilitate the uptake of nutrients such as amino acids and sugars, are instrumental in promoting cell growth (Locher, 2016). Heatmap analysis showed that in cells treated with 2-HE, 14 DEGs corresponded to ABC transporters, and critically, all of these DEGs were significantly downregulated (Figure 8G). This evidence suggests that 2-HE treatment impedes the membrane transport system of B. cinerea.
3.9 2-HE inhibited ABC transporters in B. cinerea
The heatmap presented in Figure 8G reveals that 2-HE treatment leads to the downregulation of genes associated with ABC transporters. As shown in Table 2, Bcin01g00180, Bcin12g06050, and Bcin13g02730 exhibit markedly higher downregulation, at 3.80, 3.72, and 3.89 times, respectively. Notably, genes implicated in B. cinerea multidrug resistance, such as BcatrA (Bcin11g04460), BcatrB (Bcin13g00710), and BcatrD (Bcin13g02720), also trended downward, with respective fold changes of 2.43, 2.71, and 1.16.
4 Discussion
Biogenic secondary metabolites offer a sustainable alternative to synthetic pesticides for controlling plant diseases (Valotteau et al., 2017; Chen et al., 2021). 2-HE, a VOC, presents a less toxic, safer, and more reliable option than traditional fungicides for managing diseases in tomatoes (Gong et al., 2022). For instance, Du et al. (2022) observed that 2-HE effectively reduced the incidence of Fusarium crown and root rot in tomatoes. These findings suggest that 2-HE has great potential to inhibit tomato disease.
Mycelium growth represents a critical way through which B. cinerea infects hosts, and targeting this process is a primary strategy for preventing grey mold (Bi et al., 2023; Liu K. et al., 2023). Song et al. (2024) reported that 2-nonanol, produced from Bacillus aryabhattai, markedly suppresses Penicillium expansum mycelia growth. In vivo studies demonstrate that biogenic secondary metabolites reduce postharvest diseases in fruits and vegetables; for example, reveromycin E, isolated from Streptomyces, shows substantial inhibition of tomato B. cinerea and Penicillium italicum (Nguyen et al., 2021). In addition, 3-methylbutan-1-ol, produced by endophytic bacteria, significantly reduces the incidence of tomato gray mold (Chaouachi et al., 2021). Investigations have confirmed that such metabolites curtail the mycelial growth of pathogenic fungi both in vivo and in vitro and exhibit a dose–response relationship, with an increase in metabolite concentration leading to heightened fungal suppression. Notable compounds displaying this effect include epsilon-polylysine (Li et al., 2019), L-cysteine (Wang et al., 2023), and β-glucan (Zhao et al., 2020). This study demonstrates that 2-HE substantially curtails B. cinerea mycelial growth under both in vivo and in vitro conditions in a dose-dependent manner. These findings suggest that 2-HE has the potential to manage B. cinerea infection by hindering mycelium growth.
The conidium causes the long-distance transmission of gray mold, so the inactivation of conidia is also one of the ways to prevent gray mold (Dai et al., 2021). Studies have shown that the use of biological bacteria and their secondary metabolites also reduces the conidial activity of pathogenic fungi (Russi et al., 2024), for example, hinokitiol (Wang et al., 2020) and magnolol (Cui et al., 2021). In this study, we also discovered that 2-HE markedly reduces the conidial activity of B. cinerea. This suggests that 2-HE reduces the possibility of long-distance transmission of B. cinerea by inhibiting spore activity.
Inducing host resistance is a central strategy for controlling postharvest fruit and vegetable diseases (Kesel et al., 2021; Prusky and Romanazzi, 2023). Augmenting the activity of defense-related enzymes constitutes a significant aspect of this resistance against pathogenic fungi (Raynaldo et al., 2021). POD, CAT, and SOD are the pivotal antioxidant enzymes in fruits and vegetables; their heightened activity signals an enhanced capacity for reactive oxygen species detoxification (Jing et al., 2020; Dou et al., 2021). Relevant studies indicate that the application of bioderived secondary metabolites activates these enzymes activities, thereby fortifying host resistance to fungal pathogens (Wu et al., 2023; Xing et al., 2023). Our study demonstrated that the activities of CAT, SOD, and POD were related to the concentration of 2-HE. Notably, compared to the control, 2-HE activated the antioxidant enzyme activity in the tomato host, substantially mitigating the invasion by gray mold. Malondialdehyde (MDA), a primary product of membrane lipid peroxidation, is commonly used to gauge oxidative damage (Ling et al., 2023). Throughout storage, MDA content at 0.05 μL cm-3 of 2-HE was lower than that in the control group, whereas at 0.16 μL cm-3, MDA content surpassed the control on the second day of storage. The results indicated that 2-HE diminishes tomato cell membrane peroxidation at low concentrations (0.05 μL cm-3), identifying its potential as a preservative. In general, 2-HE acts as an antifungal agent by reducing membrane peroxidation damage in tomato fruits, activating the defense response, inducing resistance, and ultimately suppressing the incidence of tomato gray mold at an optimal concentration.
In managing postharvest decay, preserving the appearance, flavor, texture, and nutritional value of fruits and vegetables is of paramount importance (Chen et al., 2021; Hosseini et al., 2023). Wang et al. (2024) demonstrated that natamycin does not adversely impact the quality attributes of fruits, such as grape stem appearance, berry hardness, total soluble solids (TSS), and titratable acidity (TA). Similar results were also reported in thymol (Ding et al., 2023), dimethyl disulfide, and dimethyl trisulfide (Wang et al., 2021). Additionally, 2-ethyl-5-methylpyrazine was found to enhance mango postharvest quality by increasing TSS, total phenol, total proline, total carotenoid, total flavonoid, and fruit firmness (Archana et al., 2021). Our study indicates that 2-HE neither compromises the external appearance of tomato fruit nor diminishes its postharvest quality.
The plasma membrane plays a pivotal role in sustaining normal cellular function in fungal pathogens, rendering it a frequent target for antifungal agents (Liu R. et al., 2023; Wei et al., 2021). Numerous studies demonstrate that various antifungal compounds compromise membrane integrity, result in the leakage of cytoplasmic contents, and lead to spore mortality (Ma et al., 2019; Zhang M. et al., 2020). Our findings reveal that 2-HE treatment notably reduces spore activity and impairs membrane integrity. Moreover, elevated levels of soluble carbohydrates, nucleic acids, and MDA were detected in B. cinerea subjected to 2-HE treatment. These outcomes suggest that 2-HE disrupts membrane permeability, triggers cytoplasmic leakage, and induces membrane lipid peroxidation, findings that align with those from studies on 3-Octanol (Zhang et al., 2023), 2-Phenylethanol (Zou et al., 2022), and 2,3-Butanedione (Li et al., 2022).
ABC transporters facilitate the translocation of not only a wide array of nutrients but also exogenous drugs, with certain transporters playing a pivotal role in cell growth and antifungal resistance (Viglas and Olejnikova, 2021). To date, 14 ABC transporters (BcatrA to BcatrN) have been characterized, and of these, the BcatrB, BcatrD, and BcatrK genes are implicated in the multidrug resistance of B. cinerea, primarily functioning in the expulsion of toxic fungi compounds (Stefanato et al., 2009; Wu et al., 2024). Research has revealed that antifungal agents impinge on the functionality of pathogen ABC transporters by modulating ABC gene expression (Wu et al., 2024). Our investigations indicate that BcatrB and BcatrD genes were significantly downregulated following 2-HE treatment by 2.71 and 1.16 times, respectively, suggesting impaired B. cinerea ability to extrude 2-HE as a toxic substance. Moreover, other genes associated with ABC transporters were also substantially downregulated. Such findings resonate with observations of certain antifungal substances, including resveratrol (Vermeulen et al., 2001), psoralen, and eugenol (Hayashi et al., 2002). These RNA-Seq data demonstrate that 2-HE impedes the ABC transporters pathway, corroborating the notion that 2-HE compromises the membrane structure of B. cinerea and consequently disrupts membranal transport functionality.
Amino acids play a crucial role in promoting cell growth and survival by serving as vital nutrients (Li and Zhang, 2023). Disrupted amino acid metabolism often results in cell death (Jin et al., 2020). Our research found that most DEGs related to amino acid metabolism were upregulated, suggesting that 2-HE contributes to nutrient depletion in B. cinerea cells. Consequently, treatment with 2-HE not only obstructs the membrane transport pathway but also hastens intracellular amino acid metabolism within B. cinerea cells. This destruction of the membrane structure, along with the inhibition of the membrane transport pathway and the acceleration of intracellular amino acid metabolism, culminates in the cells’ inability to assimilate nutrients, leading to eventual nutrient depletion.
5 Conclusion
In summary, 2-HE exhibits a potent antifungal effect in both in vitro and in vivo conditions, enhancing the activity of defense enzymes in tomatoes. At the same time, 2-HE does not adversely affect the appearance or quality of tomatoes. The antifungal mechanism of 2-HE against B. cinerea involves the acceleration of intracellular amino acid metabolism, disruption of membrane structure, and inhibition of the membrane transport system, resulting in the depletion of intracellular nutrients and the eventual demise of B. cinerea. Consequently, 2-HE represents a promising natural agent for the control of postharvest gray mold in tomatoes.
Data availability statement
The data presented in study are deposited in the NCBI repository, accession number PRJNA114723.
Author contributions
FW: Writing – review & editing, Writing – original draft, Methodology, Conceptualization. HW: Writing – original draft, Data curation. YL: Writing – review & editing, Visualization, Data curation. ZQ: Writing – original draft, Visualization. BZ: Writing – original draft, Software, Writing – review & editing. SF: Conceptualization, Writing – review & editing, Supervision, Funding acquisition. XL: Writing – review & editing, Supervision, Funding acquisition, Data curation.
Funding
The author(s) declare financial support was received for the research, authorship, and/or publication of this article. This work was supported by the Hainan Provincial Key Research and Development Project of China (Grant No. ZDYF2023XDNY178) and the Hainan Provincial Natural Science Foundation of China (Grant No. 323QN205).
Conflict of interest
The authors declare that the research was conducted in the absence of any commercial or financial relationships that could be construed as a potential conflict of interest.
Publisher’s note
All claims expressed in this article are solely those of the authors and do not necessarily represent those of their affiliated organizations, or those of the publisher, the editors and the reviewers. Any product that may be evaluated in this article, or claim that may be made by its manufacturer, is not guaranteed or endorsed by the publisher.
Supplementary material
The Supplementary Material for this article can be found online at: https://www.frontiersin.org/articles/10.3389/fpls.2024.1400164/full#supplementary-material
References
Archana, T. J., Gogoi, R., Kaur, C., Varghese, E., Sharma, R. R., Srivastav, M., et al. (2021). Bacterial volatile mediated suppression of postharvest anthracnose and quality enhancement in mango. Postharvest Biol. Tech. 177, 111525. doi: 10.1016/j.postharvbio.2021.111525
Bi, K., Liang, Y., Mengiste, T., Sharon, A. (2023). Killing softly: a roadmap of Botrytis cinerea pathogenicity. Trends Plant Sci. 28, 211–222. doi: 10.1016/j.tplants.2022.08.024
Chang, X., Guo, Y., Ren, Y., Li, Y., Wang, F., Ye, G., et al. (2023). Virus-induced plant volatiles promote virus acquisition and transmission by insect vectors. Int. J. Mol. Sci. 24, 1777. doi: 10.3390/ijms24021777
Chang, X., Wang, F., Fang, Q., Chen, F., Yao, H., Gatehouse, A. M. R., et al. (2021). Virus-induced plant volatiles mediate the olfactory behaviour of its insect vectors. Plant Cell Environ. 44, 2700–2715. doi: 10.1111/pce.14069
Chaouachi, M., Marzouk, T., Jallouli, S., Elkahoui, S., Gentzbittel, L., Ben, C., et al. (2021). Activity assessment of tomato endophytic bacteria bioactive compounds for the postharvest biocontrol of Botrytis cinerea. Postharvest Biol. Tec. 172, 111389. doi: 10.1016/j.postharvbio.2020.111389
Chen, J., Wei, X., Ming, R., Huang, D., Yao, Y., Li, L., et al. (2022). Burkholderia cenocepacia ETR-B22 volatile organic compounds suppress postharvest grey mould infection and maintain aroma quality of tomato fruit. Lwt-food Sci. Technol. 165, 113715. doi: 10.1016/j.lwt.2022.113715
Chen, T., Ji, D., Zhang, Z., Li, B., Qin, G., Tian, S. (2021). Advances and strategies for controlling the quality and safety of postharvest fruit. Engineering 7, 1177–1184. doi: 10.1016/j.eng.2020.07.029
Chen, Z. F., Ying, G. G. (2015). Occurrence, fate and ecological risk of five typical azole fungicides as therapeutic and personal care products in the environment: A review. Environ. Int. 84, 142–153. doi: 10.1016/j.envint.2015.07.022
Cui, X., Ma, D., Liu, X., Zhang, Z., Li, B., Xu, Y., et al. (2021). Magnolol inhibits gray mold on postharvest fruit by inducing autophagic activity of Botrytis cinerea. Postharvest Biol. Tech. 180, 111596. doi: 10.1016/j.postharvbio.2021.111596
Dai, Y., Wang, Z., Leng, J., Sui, Y., Jiang, M., Wisniewski, M., et al. (2021). Eco-friendly management of postharvest fungal decays in kiwifruit. Crit. Rev. Food Sci. 62, 8307–8318. doi: 10.1080/10408398.2021.1926908
Ding, J., Liu, C., Huang, P., Zhang, Y., Hu, X., Li, H., et al. (2023). Effects of thymol concentration on postharvest diseases and quality of blueberry fruit. Food Chem. 402, 134227. doi: 10.1016/j.foodchem.2022.134227
Dou, Y., Routledge, M. N., Gong, Y., Godana, E. A., Dhanasekaran, S., Yang, Q., et al. (2021). Efficacy of epsilon-poly-L-lysine inhibition of postharvest blue mold in apples and potential mechanisms. Postharvest Biol. Tech. 171, 111346. doi: 10.1016/j.postharvbio.2020.111346
Du, J., Ji, Y., Li, Y., Liu, B., Yu, Y., Chen, D., et al. (2022). Microbial volatile organic compounds 2-heptanol and acetoin control Fusarium crown and root rot of tomato. J. Cell. Physiol., 1–11. doi: 10.1002/jcp.30889
Feng, S., Lu, W., Jian, Y., Chen, Y., Meng, R., Deng, J., et al. (2021). Biocontrol effect and possible mechanism of food-borne sulfide 3-methylthio-1-propanol against Botrytis cinerea in postharvest tomato. Front. Plant Sci. 12. doi: 10.3389/fpls.2021.763755
Gong, D., Bi, Y., Zong, Y., Li, Y., Sionov, E., Prusky, D. (2022). Characterization and sources of volatile organic compounds produced by postharvest pathogenic fungi colonized fruit. Postharvest Biol. Tech. 188, 111903. doi: 10.1016/j.postharvbio.2022.111903
Hayashi, K., Schoonbeek, H. J., De Waard, M. A. (2002). Bcmfs1, a novel major facilitator superfamily transporter from Botrytis cinerea, provides tolerance towards the natural toxic compounds camptothecin and cercosporin and towards fungicides. Appl. Environ. Microbiol. 68, 4996–5004. doi: 10.1128/AEM.68.10.4996-5004.2002
Hosseini, A., Saba, M. K., Watkins, C. B. (2023). Microbial antagonists to biologically control postharvest decay and preserve fruit quality. Crit. Rev. Food. Sci. 6, 1–13. doi: 10.1080/10408398.2023.2184323
Hua, C., Kai, K., Bi, W., Shi, W., Liu, Y., Zhang, D. (2019). Curcumin induces oxidative stress in Botrytis cinerea, resulting in a reduction in gray mold decay in kiwifruit. J. Agr. Food Chem. 67, 7968–7976. doi: 10.1021/acs.jafc.9b00539
Jiao, W., Liu, X., Li, Y., Li, B., Du, Y., Zhang, Z., et al. (2022). Organic acid, a virulence factor for pathogenic fungi, causing postharvest decay in fruits. Mol. Plant Pathol. 23, 304–312. doi: 10.1111/mpp.13159
Jin, X., Guo, L., Jin, B., Zhu, S., Mei, X., Wu, J., et al. (2020). Inhibitory mechanism of 6-Pentyl-2H-pyran-2-one secreted by Trichoderma atroviride T2 against Cylindrocarpon destructans. Pestic. Biochem. Physiol. 170, 104683. doi: 10.1016/j.pestbp.2020.104683
Jing, J., Zhang, H., Xue, Y., Zeng, K. (2020). Effects of INA on postharvest blue and green molds and anthracnose decay in citrus fruit. J. Integr. Agric. 19, 1396–1406. doi: 10.1016/S2095-3119(20)63169-0
Kesel, J. D., Conrath, U., Flors, V., Luna, E., Mageroy, M. H., Mauch-Mani, B., et al. (2021). The induced resistance lexicon: do’s and don’ts. Trends Plant Sci. 26, 685–691. doi: 10.1016/j.tplants.2021.01.001
Leng, J., Yu, L., Dai, Y., Leng, Y., Wang, C., Chen, Z., et al. (2022). Recent advances in research on biocontrol of postharvest fungal decay in apples. Crit. Rev. Food. Sci. 63, 10607–10620. doi: 10.1080/10408398.2022.2080638
Li, G., Chen, Y., Zhang, Z., Li, B., Chen, T., Tian, S. (2022). 2,3-Butanedione suppresses gray mold of postharvest fruit by activating the autophagy of Botrytis cinerea. Postharvest Biol. Tech. 193, 112057. doi: 10.1016/j.postharvbio.2022.112057
Li, H., He, C., Li, G., Zhang, Z., Li, B., Tian, S. (2019). The modes of action of epsilon-polylysine (ϵ-PL) against Botrytis cinerea in jujube fruit. Postharvest Biol. Tech. 147, 1–9. doi: 10.1016/j.postharvbio.2018.08.009
Li, Y., Wu, T., Deng, X., Tian, D., Ma, C., Wang, X., et al. (2023). Characteristic aroma compounds in naturally withered and combined withered γ-aminobutyric acid white tea revealed by HS-SPME-GC-MS and relative odor activity value. Lwt-Food. Sci. Technol. 176, 114467. doi: 10.1016/j.lwt.2023.114467
Li, X., Zhang, H. S. (2023). Amino acid metabolism, redox balance and epigenetic regulation in cancer. FEBS J. 291, 412–429. doi: 10.1111/febs.16803
Ling, L., Pang, M., Luo, H., Cheng, W., Jiang, K., Wang, Y. (2023). Antifungal activity of diacetyl, a volatile organic compound, on Trichoderma lixii F2 isolated from postharvest Lanzhou lily bulbs. Food Biosci. 52, 102365. doi: 10.1016/j.fbio.2023.102365
Liu, K., Liu, W., Huang, X., Liu, Y., Cui, X., Zhang, Z., et al. (2023). Identification of virulence-related proteins during Botrytis cinerea–fruit interaction at early phase. Postharvest Biol. Tech. 204, 112443. doi: 10.1016/j.postharvbio.2023.112443
Liu, R., Zhang, L., Xiao, S., Chen, H., Han, Y., Niu, B., et al. (2023). Ursolic acid, the main component of blueberry cuticular wax, inhibits Botrytis cinerea growth by damaging cell membrane integrity. Food Chem. 415, 136220. doi: 10.1016/j.foodchem.2023.135753
Locher, K. P. (2016). Mechanistic diversity in ATP-binding cassette (ABC) transporters. Nat. Struct. Mol. Biol. 23, 487–493. doi: 10.1038/nsmb.3216
Ma, D., Ji, D., Zhang, Z., Li, B., Qin, G., Xu, Y., et al. (2019). Efficacy of rapamycin in modulating autophagic activity of Botrytis cinerea for controlling gray mold. Postharvest Biol. Tech. 150, 158–165. doi: 10.1016/j.postharvbio.2019.01.005
Nguyen, H. T. T., Park, A. R., Hwang, I. M., Kim, J. (2021). Identification and delineation of action mechanism of antifungal agents: Reveromycin E and its new derivative isolated from Streptomyces sp. JCK-6141. Postharvest Biol. Tech. 182, 111700. doi: 10.1016/j.postharvbio.2021.111700
Ozkara, K. T., Amanpour, A., Guclu, G., Kelebek, H., Selli, S. (2019). GC-MS-olfactometric differentiation of aroma-active compounds in turkish heat-treated sausages by application of aroma extract dilution analysis. Food. Anal. Method. 12, 729–741. doi: 10.1007/s12161-018-1403-y
Prusky, D., Romanazzi, G. (2023). Induced resistance in fruit and vegetables: a host physiological response limiting postharvest disease development. Annu. Rev. Phytopathol. 61, 297–300. doi: 10.1146/annurev-phyto-021722-035135
Raynaldo, F. A., Dhanasekaran, S., Ngea, G. L. N., Yang, Q., Zhang, X., Zhang, H. (2021). Investigating the biocontrol potentiality of Wickerhamomyces anomalus against postharvest gray mold decay in cherry tomatoes. Sci. Hortic. 285, 110137. doi: 10.1016/j.scienta.2021.110137
Russi, A., Granada, C. E., Schwambach, J. (2024). Optimization of Bacillus velezensis S26 sporulation for enhanced biocontrol of gray mold and anthracnose in postharvest strawberries. Postharvest Biol. Tech. 210, 112737. doi: 10.1016/j.postharvbio.2023.112737
Shi, Y., Zhao, Q., Xin, Y., Yang, Q., Dhanasekaran, S., Zhang, X., et al. (2023). Aureobasidium pullulans S2 controls tomato gray mold and produces volatile organic compounds and biofilms. Postharvest Biol. Tech. 204, 112450. doi: 10.1016/j.postharvbio.2023.112450
Song, C., Zhang, Y., Zhao, Q., Chen, M., Zhang, Y., Gao, C., et al. (2024). Volatile organic compounds produced by Bacillus aryabhattai AYG1023 against Penicillium expansum causing blue mold on the Huangguan pear. Microbiol. Res. 278, 127531. doi: 10.1016/j.micres.2023.127531
Stefanato, F. L., Abou-Mansour, E., Buchala, A., Kretschmer, M., Mosbach, A., Hahn, M., et al. (2009). The ABC transporter BcatrB from Botrytis cinerea exports camalexin and is a virulence factor on Arabidopsis thaliana. Plant J. 58, 499–510. doi: 10.1111/j.1365-313X.2009.03794.x
Su, Y., Liang, Y. (2015). Foliar uptake and translocation of formaldehyde with Bracket plants (Chlorophytum comosum). J. Hazard. Mater 291, 120–128. doi: 10.1016/j.jhazmat.2015.03.001
Szabo, K., Dulf, F. V., Teleky, B. E., Eleni, P., Boukouvalas, C., Krokida, M., et al. (2021). Evaluation of the bioactive compounds found in tomato seed oil and tomato peels influenced by industrial heat treatments. Foods 10, 110. doi: 10.3390/foods10010110
Ukeh, D. A., Urnoetok, S. B. A. (2011). Repellent effects of five monoterpenoid odours against Tribolium castaneum (Herbst) and Rhyzopertha Dominica (F.) in Calabar, Nigeria. Crop Prot. 30, 1351–1355. doi: 10.1016/j.cropro.2011.05.016
Valotteau, C., Banat, I. M., Mitchell, C. A., Lydon, H., Marchant, R., Babonneau, F., et al. (2017). Antibacterial properties of sophorolipid-modified gold surfaces against gram positive and gram negative pathogens. Colloid Surface. B. 157, 325–334. doi: 10.1016/j.colsurfb.2017.05.072
Vermeulen, T., Schoonbeek, H., De Waard, M. A. (2001). The ABC transporter BcatrB from Botrytis cinerea is a determinant of the activity of the phenylpyrrole fungicide fludioxonil. Pest. Manage. Sci. 57, 393–402. doi: 10.1002/ps.309
Viglas, J., Olejnikova, P. (2021). An update on ABC transporters of filamentous fungi-from physiological substrates to xenobiotics. Microbiol. Res. 246, 126684. doi: 10.1016/j.micres.2020.126684
Wang, W., Ling, Y., Deng, L., Yao, S., Zeng, K. (2023). Effect of L-cysteine treatment to induce postharvest disease resistance of Monilinia fructicola in plum fruits and the possible mechanisms involved. Pestic Biochem. Physiol. 191, 105367. doi: 10.1016/j.pestbp.2023.105367
Wang, Y., Liu, X., Chen, T., Xu, Y., Tian, S. (2020). Antifungal effects of hinokitiol on development of Botrytis cinerea in vitro and in vivo. Postharvest Biol. Tech. 159, 111038. doi: 10.1016/j.postharvbio.2019.111038
Wang, F., Saito, S., Xiao, C. L. (2024). Postharvest application of natamycin to control gray mold in table grapes. Postharvest Biol. Tech. 210, 112777. doi: 10.1016/j.postharvbio.2024.112777
Wang, Z., Zhong, T., Chen, X., Yang, B., Du, M., Wang, K., et al. (2021). Potential of volatile organic compounds emitted by Pseudomonas fluorescens ZX as biological fumigants to control citrus green mold decay at postharvest. J. Agric. Food Chem. 69, 2087–2098. doi: 10.1021/acs.jafc.0c07375
Wei, C., Zhang, F., Song, L., Chen, X., Meng, X. (2021). Photosensitization effect of curcumin for controlling plant pathogen Botrytis cinerea in postharvest apple. Food Control. 123, 107683. doi: 10.1016/j.foodcont.2020.107683
Williamson, B., Tudzynski, B., Tudzynski, P., van Kan, J. A. L. (2007). Botrytis cinerea: the cause of grey mould disease. Mol. Plant Pathol. 8, 561–580. doi: 10.1111/j.1364-3703.2007.00417.x
Wu, Z., Bi, Y., Zhang, J., Gao, T., Li, X., Hao, J., et al. (2024). Multidrug resistance of Botrytis cinerea associated with its adaptation to plant secondary metabolites. Mbio 15, 2. doi: 10.1128/mbio.02237-23
Wu, Y., Gao, Y., Zheng, X., Yu, T., Yan, F. (2023). Enhancement of biocontrol efficacy of Kluyveromyces marxianus induced by N-acetylglucosamine against Penicillium expansum. Food Chem. 404, 134658. doi: 10.1016/j.foodchem.2022.134658
Xing, M., Zhao, J., Zhang, J., Wu, Y., Khan, R. A. A., Li, R., et al. (2023). 6-Pentyl-2H-pyran-2-one from Trichoderma erinaceum is fungicidal against litchi downy blight pathogen Peronophythora litchii and preservation of litchi. J. Agric. Food Chem. 71, 19488–19500. doi: 10.1021/acs.jafc.3c03872
Yu, A. N., Yang, Y. N., Yang, Y., Zheng, F. P., Sun, B. G. (2019). Free and bound volatile compounds in the Rubus coreanus fruits of different ripening stages. J. Food. Biochem. 43, e12964. doi: 10.1111/jfbc.12964
Yu, W., Zhao, R., Sheng, J., Shen, L. (2018). SIERF2 Is Associated with Methyl Jasmonate-Mediated Defense Response against Botrytis cinerea in Tomato Fruit. J. Agr. Food Chem. 66, 9923–9932. doi: 10.1021/acs.jafc.8b03971
Zhang, M., Li, Y., Bi, Y., Wang, T., Dong, Y., Yang, Q., et al. (2020). 2-Phenylethyl isothiocyanate exerts antifungal activity against Alternaria alternata by affecting membrane integrity and mycotoxin production. Toxins 12, 124. doi: 10.3390/toxins12020124
Zhang, X., Li, B., Zhang, Z., Chen, Y., Tian, S. (2020). Antagonistic yeasts: a promising alternative to chemical fungicides for controlling postharvest decay of fruit. J. Fungi. 6, 158. doi: 10.3390/jof6030158
Zhang, X., Li, G., Zhang, Z., Tian, S. (2023). 3-Octanol controls gray mold on postharvest fruit by inducing autophagy of Botrytis cinerea. Postharvest Biol. Tech. 205, 112525. doi: 10.1016/j.postharvbio.2023.112525
Zhao, L., Wang, Y., Wang, Y., Li, B., Gu, X., Zhang, X., et al. (2020). Effect of β-glucan on the biocontrol efficacy of Cryptococcus podzolicus against postharvest decay of pears and the possible mechanisms involved. Postharvest Biol. Tech. 160, 111057. doi: 10.1016/j.postharvbio.2019.111057
Zou, X., Wei, Y., Jiang, S., Xu, F., Wang, H., Zhan, P., et al. (2022). ROS stress and cell membrane disruption are the main antifungal mechanisms of 2-Phenylethanol against Botrytis cinerea. J. Agr. Food Chem. 70, 14468–14479. doi: 10.1021/acs.jafc.2c06187
Keywords: gray mold, volatile organic compounds, antifungal mechanism, amino acid metabolism, membrane transport system
Citation: Wu F, Wang H, Lin Y, Qu Z, Zheng B, Feng S and Li X (2024) 2-Heptanol inhibits Botrytis cinerea by accelerating amino acid metabolism and retarding membrane transport. Front. Plant Sci. 15:1400164. doi: 10.3389/fpls.2024.1400164
Received: 13 March 2024; Accepted: 06 May 2024;
Published: 03 June 2024.
Edited by:
Elsherbiny A. Elsherbiny, Mansoura University, EgyptReviewed by:
Chuying Chen, Jiangxi Agricultural University, ChinaMarisabel Mecca, Oncological Center of Basilicata (IRCCS), Italy
Justyna Nawrocka, University of Łódź, Poland
Copyright © 2024 Wu, Wang, Lin, Qu, Zheng, Feng and Li. This is an open-access article distributed under the terms of the Creative Commons Attribution License (CC BY). The use, distribution or reproduction in other forums is permitted, provided the original author(s) and the copyright owner(s) are credited and that the original publication in this journal is cited, in accordance with accepted academic practice. No use, distribution or reproduction is permitted which does not comply with these terms.
*Correspondence: Shun Feng, MTU1MjAzNzY5MUBxcS5jb20=; Xinguo Li, bGl4aW5ndW8xM0AxNjMuY29t