- 1Department of Pharmacognosy, Semmelweis University, Budapest, Hungary
- 2Centre for Translational Medicine, Semmelweis University, Budapest, Hungary
- 3Institute of Clinical Pharmacy, University of Szeged, Szeged, Hungary
- 4Institute of Pancreatic Diseases, Semmelweis University, Budapest, Hungary
- 5Institute for Translational Medicine, University of Pécs, Pécs, Hungary
Volatile organic compounds (VOCs) and essential oils of conifers are widely used in the pharmaceutical industry. This work aimed to analyze the VOCs of 30 conifer species representing the Pinaceae and Cupressaceae families. Samples were collected from arboreta in Hungary, and their chemical composition was determined by gas chromatography (SPME-GC/MS); then, chemometric analyses were performed using multivariate methods to identify characteristic VOCs of conifers. Here, we present results for monoterpene and sesquiterpene profiles of the examined conifer samples. The most abundant compounds detected were α-pinene, bornyl acetate, limonene, β-pinene, β-caryophyllene, β-myrcene, δ-3-carene, and β-phellandrene. The results showed that the following volatiles were characteristic of the conifer groups: sabinene (RRT=6.0) for the cupressoid group (which includes the Cupressaceae species), longifolene (RRT=15.0) and β-pinene (RRT=6.1) were characteristic of the pinoid group (including Picea, Pinus, and Pseudotsuga species), and camphene (RRT=5.5) and bornyl acetate (RRT=12.6) were characteristic of the abietoid group (including Abies, Cedrus, and Tsuga species). Our results on VOCs in the Pinaceae and Cupressaceae families contribute to the elucidation of biodiversity patterns of conifer species and, in addition, may support the industrial application of terpenes.
1 Introduction
Conifers are distributed worldwide from the tropics to the Arctic regions. Many species are economically important because they provide essential oils (EOs) and pine extracts, which are used in cosmetics as fragrances, in aromatherapy, or as food and beverage ingredients. In addition, the pharmaceutical industry uses EOs, resin, and the bark of certain conifer species. Juniper oil has a herbal monograph in the European Union, products containing pine tar have been used to treat different skin conditions (e.g., eczema, psoriasis, and skin inflammation), pine bark extracts have been investigated in a variety of chronic diseases, and the effects of pine resin have shown promise in the treatment of malignant ulcerating wounds (Barnes and Greive, 2017; Robertson et al., 2020; Batista et al., 2022).
EOs are mainly plant metabolites of terpenoids, i.e., mixtures of volatile organic compounds (VOCs). The building block of terpenoids is the isoprene unit, which comprises an isopentenyl skeleton. Monoterpenes, the most important essential oil components, consist of two isoprene units, while sesquiterpenes comprise three isoprene units. EOs and VOCs are important candidates for drug development. For example, monoterpenes are subjected to structural modifications in medicinal chemistry, a promising way to synthesize novel biologically active substances. According to Zielinska et al., borneol-based polymers have recently been investigated to improve cell adhesion, borneol isomers improve surface bacterial resistance properties, and α-pinene derivatives have been synthesized as potential antitumor agents, among others (Zielinska-Blajet and Feder-Kubis, 2020). Nootkatone, which is a sesquiterpene and has been used as a safe and effective active substance in a commercial insect repellent, was first isolated from Chamaecyparis nootkatensis (D.Don) Spach (Dietrich et al., 2006). Other economically important sesquiterpenes (e.g., β-farnesene, santalol, β-caryophyllene) are also available on the market. They are used in the food and fragrance industries due to their pleasant flavor and smell (Jiang and Wang, 2023). Sundar and Parikh, authors of a recent publication, have summarized the available knowledge on encapsulation techniques of essential oils. This process contributes to increasing the shelf life and the bioavailability of essential oils. Therefore, it is a promising technique for the development of EO-containing drugs (Sundar and Parikh, 2023). VOCs can also serve as initial molecules for the synthesis of other substances in the chemical industry. Oxygenated terpenoids may play an important role in the synthesis of biofuels (e.g., 1,8-cineole as the potential bio-jet fuel precursor) (Xie et al., 2020). Current applications of non-wood pine products are discussed in a study by Neis et al.; for example, sustainable thermoplastic elastomers are made from terpene-derived monomers (Neis et al., 2019). The wood of Cupressaceae is durable; therefore, it is a suitable source of timber and construction materials, particularly species from the Calocedrus, Chamaecyparis, Cupressus, and Thuja genera. Additionally, within the Cupressaceae family, Chamaecyparis, Juniperus, Platycladus and Thuja species are valued as ornamental trees. Cryptomeria japonica is the major plantation tree in the Far East. Furthermore, conifers are also used in horticulture, contributing significantly to their economic importance as cultivated species (Farjon, 2018; Mao et al., 2019).
The aim of this work was to investigate the monoterpene and sesquiterpene profiles of conifer species and to explore possible chemotaxonomic relationships. According to current gymnosperm phylogeny (Yang et al., 2022), extant gymnosperms are classified into three Linnaean classes, Cycadopsida, Ginkgoopsida, and Pinopsida - the latter including three subclasses, Gnetidae, Pinidae, and the Cupressidae. Conventionally, conifers are the last two subclasses. The classification of Gymnosperm (including subclasses and families) is presented in Table 1. Our current study focuses on two families of conifers, namely Pinaceae and Cupressaceae. The classification of these two families (i.e., a list of corresponding genera) and their habitats are presented in the Supplementary Material in Supplementary Table S1 (WFO, 2024). Pinaceae is the most prominent gymnosperm family, with 11 genera and 272 species. It is widely distributed and restricted to the Northern Hemisphere (except for P. merkusii). The family is traditionally subdivided into two groups: the pinoid subfamily (Pinoideae) includes Cathaya, Larix, Picea, Pinus, and Pseudotsuga, whereas the abietoid subfamily (Abietoideae) includes Abies, Cedrus, Keteleeria, Nothotsuga, Pseudolarix, and Tsuga (Ran et al., 2018). Cupressaceae is also rich, comprising 31 genera and 169 species. It is distributed on all continents (except for Antarctica) from the sea level to the timberline. Gadek et al. identified seven subfamilies of the family: Cunninghamioideae, Taiwanioideae, Athrotaxidoideae, Sequoioideae, Taxodioideae, Callitroideae, and Cupressoideae. This classification was supported also by genome-based phylogeny studies (Gadek et al., 2000; Yang et al., 2012; Liu et al., 2022; Yang et al., 2022). Several samples investigated in the present study originate from species of the Cupressoideae subfamily, the largest subfamily within the Cupressaceae family, encompassing approximately 100 species distributed among 13 genera.
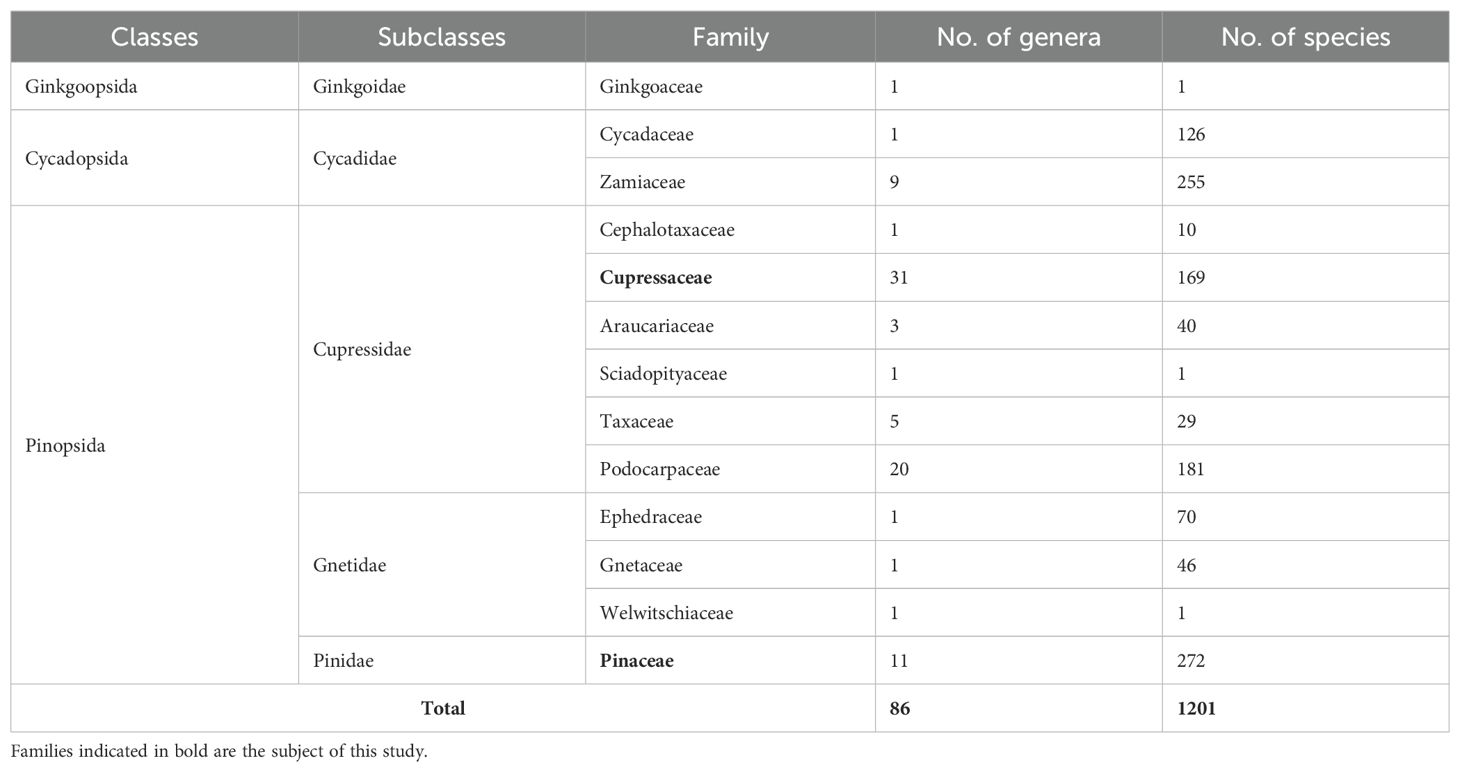
Table 1. Gymnosperm classification (Yang et al., 2022).
Most conifers are evergreen, although exceptions exist within certain genera, such as Larix and Pseudolarix, which are deciduous. The leaves of the Pinaceae are needle-like, whereas scale leaves are predominantly found in the Cupressaceae family. The morphological properties of the bark are characteristic of the species. Seeds are enclosed within cones, originating from the female cone, while the male cone produces pollens (Debreczy et al., 2011). Throughout various parts of conifer trees, oleoresin is present within resin ducts, resin vesicles, resin blisters, resin glands, and resin cells. Oleoresin is produced due to physical damage to the plant. Its primary purpose is chemical and physical defense against herbivores and to prevent the damaged part of the plant. Recent investigations have highlighted the involvement of terpenes in chemo-ecological interactions, as they influence insect behavior and serve as signal molecules for bark beetles. Additionally, alterations in the terpene profile contribute to enhanced resistance against microbial pathogens and insect-induced damage (Celedon and Bohlmann, 2019; Whitehill et al., 2019; Ninkuu et al., 2021).
In conifers, typical monoterpenes are α-pinene, β-pinene, camphene, 3-carene, myrcene, α-terpineol, bornyl acetate, and typical sesquiterpenes are β-caryophyllene and germacrene D (Bhardwaj et al., 2020). However, the similarities and differences in the essential oil composition of conifers have not been exhaustively investigated by chemometric methods. In our work, essential oil samples of 30 species of the Pinaceae and Cupressaceae families were analyzed chemically and chemometrically. Mapping the chemotaxonomic relationships of conifers can contribute to a better understanding of taxonomic relationships and provide valuable data for industrial exploitation of VOCs. In addition, this study may support other research on the terpene synthase system of conifers. A better understanding of the conifer terpene synthase system may contribute to the identification of new pathways for microbial production of terpenes and the design of key enzymes involved in the biosynthesis process (Zhang et al., 2017; Zhang and Hong, 2020).
2 Materials and methods
2.1 Plant material
Samples were collected in the Jeli Arboretum (9841 Kám, Hungary) in October 2020 and June 2021 and in the Folly Arboretum (8257 Badacsonyörs, Hungary) in May 2021. The plant material was placed in labeled polyethylene bags. The following parts of the plants were collected: needles (needles and branch tips), resin, cones, and bark. The species investigated are listed in Table 2, and the plant parts collected are listed in Supplementary Table S2 in the Supplementary Material. The following species from the Pinaceae family were collected (18 species in total): Abies concolor, Abies firma, Abies grandis, Abies holophylla, Cedrus atlantica, Picea omorika, Picea sitchensis, Pinus aristata, Pinus cembra, Pinus coulteri, Pinus heldreichii, Pinus nigra, Pinus peuce, Pinus pinaster, Pinus strobus, Pseudotsuga menziesii, Tsuga canadensis, and Tsuga heterophylla. The following species from the Cupressaceae family were collected (12 species in total): Calocedrus decurrens, Chamaecyparis pisifera, Cryptomeria japonica, Cupressus macnabiana, Juniperus chinensis, Juniperus communis, Juniperus drupacea, Juniperus rigida, Juniperus sabina, Juniperus virginiana, Sequoia sempervirens, and Thuja koraiensis. Samples were conserved immediately after collection and then analyzed in the laboratory within 1-2 days. Needles were cut into small pieces (3-4 mm in length). If necessary due to their size, resin, cones, and bark samples were cut into smaller pieces to fit inside the headspace vials. Then the plant material was placed into individual vials, sealed with caps, and loaded into the GC/MS autosampler tray. Each vial was automatically transferred from the sample tray to the heated extraction chamber by the SMPE.
2.2 SPME-GC/MS measurement
The chemical composition of the samples was measured by static headspace solid-phase microextraction (sHS-SPME) using gas chromatography-mass spectrometry (GC/MS). Prior to the analysis, conifer samples were placed into 20-mL headspace vials sealed with a silicon/PTFE septum.
2.2.1 SPME
SPME, which is a fast technique that requires a low sample amount and operates without solvents, is suitable for the extraction of monoterpenes and sesquiterpenes (Knudsen et al., 1993; Szmigielski et al., 2011). SPME was performed using an automatic CTC Combi PAL multipurpose sampler (CTC Analytics AG, Zwingen, Switzerland). After a 5 minute incubation period at 100°C, a 65 μM carboxene/polydimethylsiloxane/divinylbenzene fiber (CAR/PDMS/DVB, StableFlex, Supelco, Bellefonte, PA, USA) was immersed into the headspace vial by the autosampler. The volatile components on the surface of the fiber were absorbed; the extraction was performed at 100°C for 20 minutes. Then, the fiber was transferred to the injector port of the GC/MS and desorbed (250°C, 1 minute). The injections were made in splitless mode. Then, the fiber was cleaned and conditioned in pure nitrogen (in Fiber Bakeout Station, 250°C, 15 min).
2.2.2 GC
Analyses were performed using an Agilent 6890N/5973N GC/MSD (Santa Clara, CA, USA) system with a 30 m × 250 μm × 0.25 μm SLB-5MS capillary column (Supelco, Sigma-Aldrich, Philadelphia, PA, USA). The GC oven temperature was set to increase from 60°C (3 minutes isothermal) to 250°C at a rate of 8°C/min (1 minute isothermal). High-purity helium (6.0) (Messer) was used as the carrier gas at a 1.0 mL/min (37 cm/s) flow rate in constant flow mode.
2.2.3 MS
The mass selective detector (MSD) was equipped with a quadrupole mass analyzer and operated in electron ionization mode (41–500 atomic mass units (amu) at 3.2 scan/s, at 70 eV, full scan mode).
2.2.4 Evaluation
GC/MS measurement data were evaluated using MSD ChemStation D.02.00.275 software (Agilent, Santa Clara, CA, USA). Identification of volatile components was performed by comparing the calculated Kovats indexes to those found in the literature (Adams, 2007), and the NIST (National Institute of Standards and Technology) Chemistry WebBook (Linstrom) was also taken into account for identification of spectra. Percentage evaluation was performed by area normalization. This article summarizes the findings and measurement results for monoterpenes and sesquiterpenes and their derivatives, as our GC/MS method is not suitable for the proper identification of diterpenes.
2.3 Statistical analysis
The statistical analysis was performed to investigate the correlation between the chemical profiles of conifers and other attributes (such as species and plant organs). Calculations were performed using the SYN-TAX 2000 package (Podani, 2001).
2.3.1 Principal component analysis
PCA is a dimension reduction method that helps to visualize the data structure in a few dimensions as efficiently as possible. The method creates new artificial variables (components) while the original information in the data is preserved. The object positions and the correlations between variables and components are simultaneously displayed in a coordinate system (biplot) to improve the visualization of results (Podani, 1997).
2.3.2 Canonical variates analysis
CVA is also a multivariate technique used to evaluate the separation of observations into two or more groups and to calculate the contribution of each variable to this grouping (Podani, 2000). We investigated whether the three taxonomic groups (pinoid, abietoid, cupressoid) or plant organs (needles, cones, resin, bark) were separated and, if so, which volatile components could best interpret the separation. For the analyses, based on the PCA results, we selected the variables (VOCs) with the highest correlations with the first and the second principal components (see Supplementary Material, Supplementary Tables S3; S4).
2.3.3 Analysis of variance
The total detected VOCs were analyzed by species with ANOVA, followed by a comparison of means using Tukey’s Honestly Significant Difference (HSD) test. The threshold of significance was set at p=0.05.
3 Results
3.1 GC/MS measurement results
A total of 151 conifer samples from 30 species collected from arboreta in Hungary were investigated using the SPME-GC/MS method. VOCs were analyzed in needles, bark, resin, and cone samples. All data, i.e., the identified volatile components of all samples, are presented in Supplementary Table S5 in the Supplementary Material. Our current study focuses on monoterpenes, sesquiterpenes, and related derivatives, so unknown components or diterpenes are not included. A total of 183 VOCs were identified, with an average of 18 per sample.
Considering the results from all the 151 samples, we detected the following volatiles in the largest amount: α-pinene, bornyl acetate, limonene, β-pinene, β-caryophyllene, β-myrcene, δ-3-carene, β-phellandrene, longifolene, and germacrene D. The SPME-GC/MS measurements performed unequivocally demonstrate that terpenes are abundant compounds in conifers. Of the total VOCs, the most abundant component was α-pinene, and the largest amount of about 61% was detected in the resin of Cupressus macnabiana. Representative chromatograms of samples with high amounts of the above-mentioned terpenes can be found in the Supplementary Material (Supplementary Figures S1-S5).
Major VOCs detected in our samples (α-pinene, bornyl acetate, limonene, β-pinene, β-caryophyllene, sabinene, β-myrcene, and δ-3-carene) are the subject of medicinal and pharmaceutical research. The chemical structure, the chemical classification, and biological activities of the most abundant components are presented in Table 3.
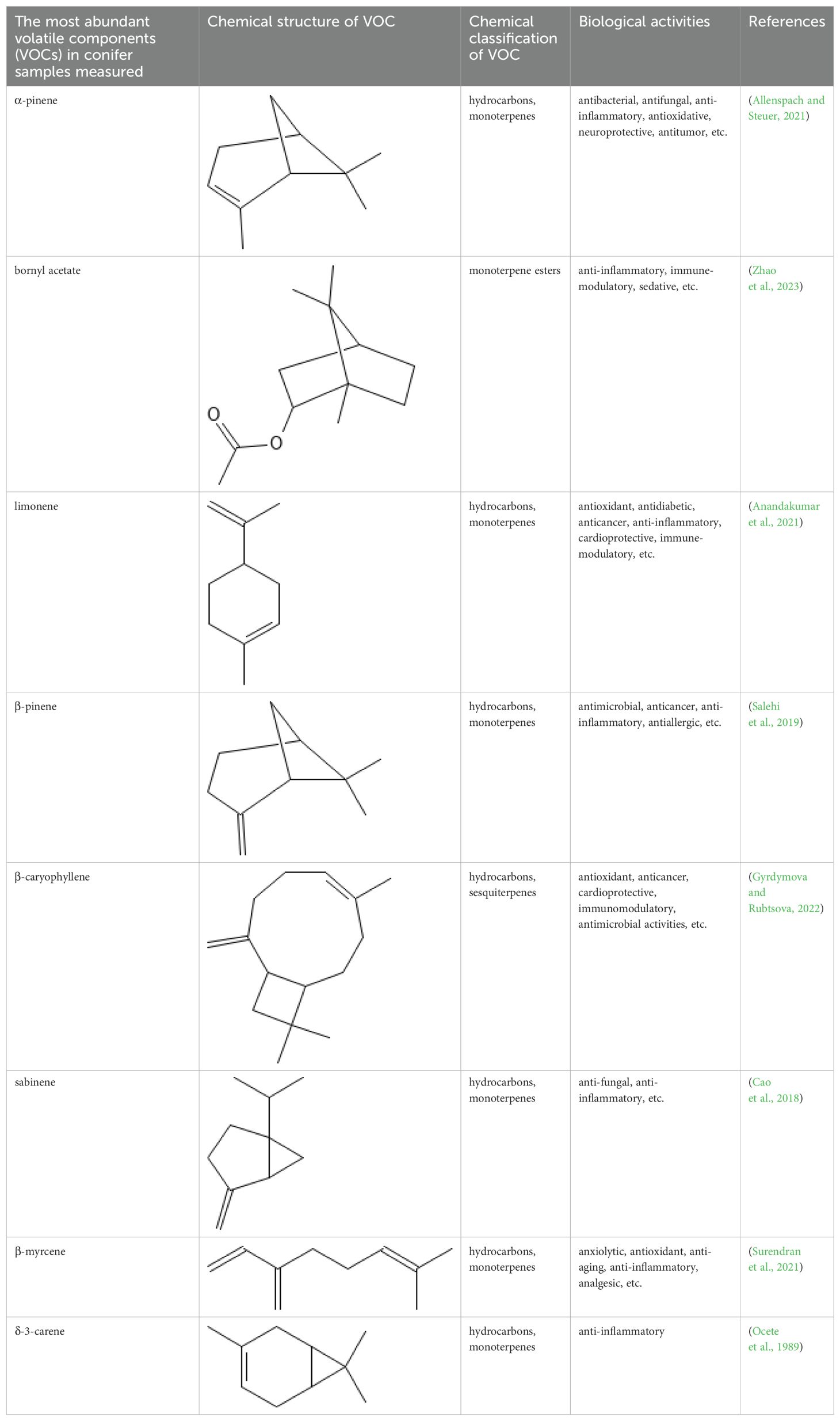
Table 3. Chemical structure, chemical classification and biological activity of the most abundant volatile organic components measured in the samples.
The samples were collected from two arboreta (Folly Arboretum and Jeli Arboretum) in two consecutive years (2020 and 2021). We investigated the influence of the location and the collection time on the distribution of the VOCs in the collected samples. No difference was detected between the terpene profile of the samples from the two years (ANOVA, p = 1.00) and the two locations (ANOVA, p = 0.999).
Based on our GC/MS analysis on 103 samples from the Pinaceae family, the most abundant components were the following (mean value): α-pinene (15%), β-pinene (7%), bornyl acetate (7%), limonene (5%), β-caryophyllene (4%), β-phellandrene (4%), δ-3-carene (3%), longifolene (3%), β-myrcene (3%), and camphene (2%).
α-Pinene was abundant in each genus (8.4%-20.7%). β-Pinene can be found in large amounts in Abies (9.5%), in Pinus (7.8%) and in Pseudotsuga (9.7%) genera. β-Phellandrene concentration was high in Picea (9.0%) and in Tsuga (5.8%), and β-myrcene content was considerable in Cedrus (7.3%) and in Picea (9.5%) genera. Bornyl acetate levels were above 10% in the Abies, Tsuga, and Pseudotsuga genera (11.2%, 15.5%, and 11.3%, respectively). β-Caryophyllene was detected in large amounts in Cedrus (13.2%) and Pinus (6.7%), and limonene was measured to be around 7% in Picea, Pinus, and Pseudotsuga genera (7.0%, 7.3%, and 6.8%, respectively). For the respective bar plot, see Figure 1.
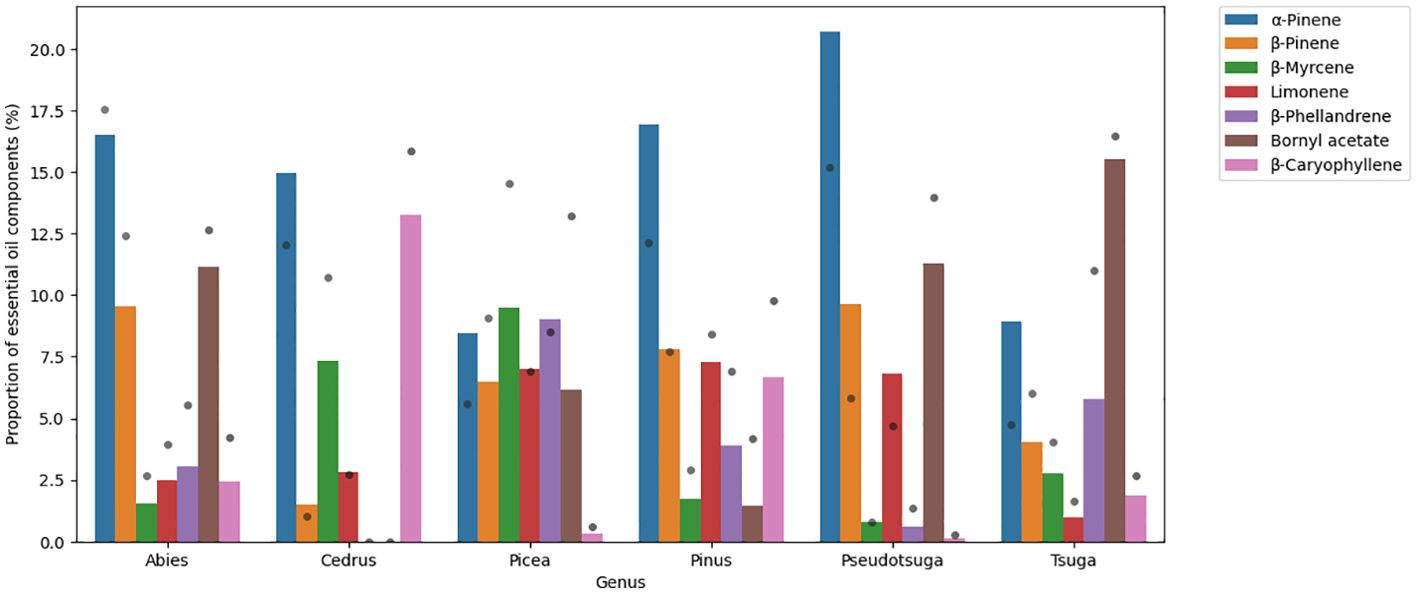
Figure 1. Bar plot showing the main volatile organic compounds of Pinaceae family. Results are mean area% measured by GC/MS. Grey dots represent standard deviation.
The most abundant components in the Cupressaceae family, based on the results of 48 samples, were (mean value): α-pinene (17%), limonene (7%), sabinene (5%), β-myrcene (4%), δ-3-carene (3%), bornyl acetate (3%), germacrene D (2%), terpinolene (2%), β-eudesmol (2%), and terpinene-4-ol (2%).
Cupressus and Calocedrus genera contain high amounts of α-pinene (41.2% and 26.1%, respectively) and the other genera contain this VOC in large amounts as well (8.3%-12.2%). Sabinene was measured at around 10% in Cryptomeria and Juniperus genera (9.5% and 7.9%, respectively). δ-3-carene is abundant in Calocedrus (9.6%) and in Chamaecyparis (10.8%) genera. The amount of α-cadinol was high in the Cupressus genus (11.6%), and bornyl acetate was above 10% in the Chamaecyparis genus only (11.7%). For the bar plot, see Figure 2.
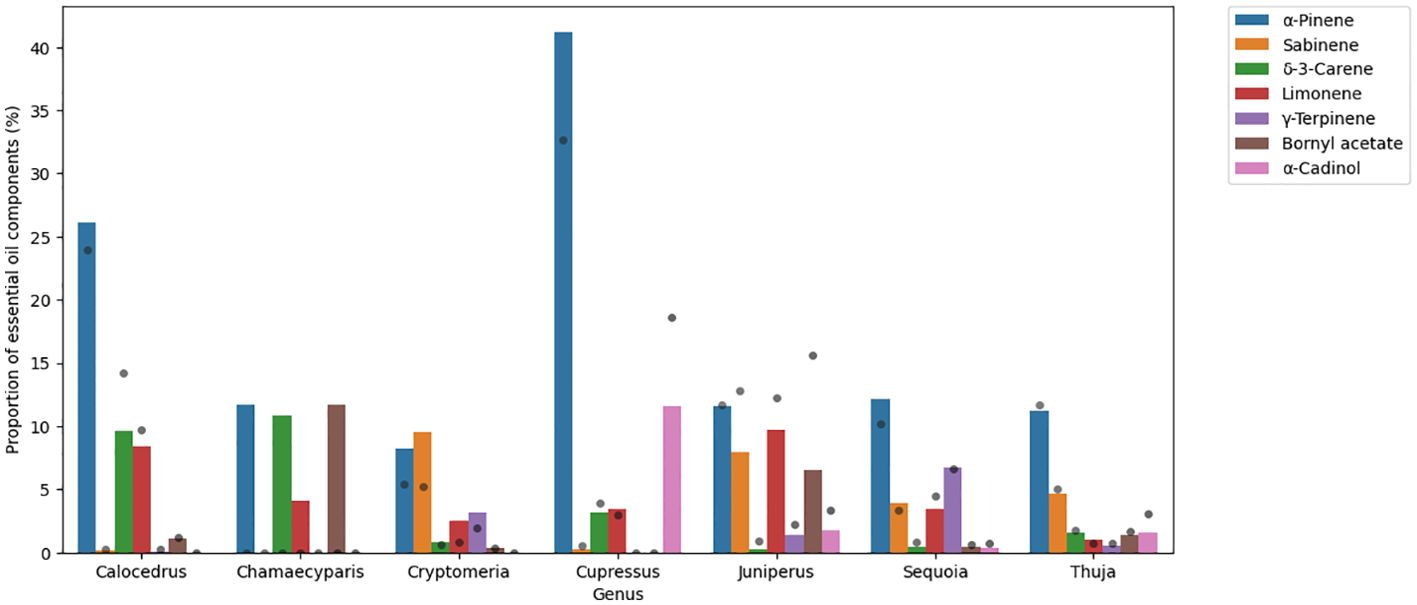
Figure 2. Bar plot showing the main volatiles of Cupressaceae family. Results are mean area% measured by GC/MS. Grey dots represent standard deviation.
In the current study, 47 needle samples from species belonging to the Pinaceae family were collected and measured. To highlight the differences, we depicted the results on a heat map alongside the phylogeny of the species, see Figure 3. The cladogram presented in Figure 3 was prepared based on the work of Stull et al. (2021). α-Pinene was detected in all samples, and its amount was considerable in all species (4%-35%). P. nigra (34.8%), P. cembra (24.9%), P. peuce (24.8%), P. coulteri (21.9%), and C. atlantica (20.8%) contained the highest percentage of α-pinene. Camphene was abundant in the species of Picea omorika (12%) and Pseudotsuga menziesii (15%). β-Pinene was also present in all species (1%-31%), with the highest percentage in Abies grandis and Abies concolor (30.8% and 24.7%, respectively). All species contained β-myrcene. C. atlantica and P. sitchensis contained the highest percentage (18% and 17%, respectively); however, the β-myrcene content remained below 8% in other species (0.5%-8%). Not all species contained δ-3-carene; only four species contained more than 10% of this VOC (Abies holophylla 19%, P. aristata 42%, P. peuce 15%, and P. strobus 11%). β-phellandrene was the major VOC of P. sitchensis, P. cembra, and Tsuga heterophylla (16%, 16%, and 17%, respectively). γ-Terpinene, terpinene-4-ol, terpinolene, and α-terpineol could not be detected in substantial amounts. Bornyl acetate was abundant in species of Abies (13%-16%), in P. omorika (33%), and in species of Tsuga and Pseudotsuga (15%-36%). Other species contained relatively low amounts of this VOC (0-3%). Almost all species contained β-caryophyllene; C. atlantica and P. pinaster had the highest levels of this VOC (30% and 34%, respectively). Germacrene D was measured to be above 10% in P. nigra, P. heldreichii, P. cembra, and P. coulteri (21%, 30%, 14%, and 13%, respectively), while other species contained lower amounts (0-9%). α-Muurolene, γ-cadinene, and δ-cadinene were not detected in high amounts (usually below 5%) but were present in most species.
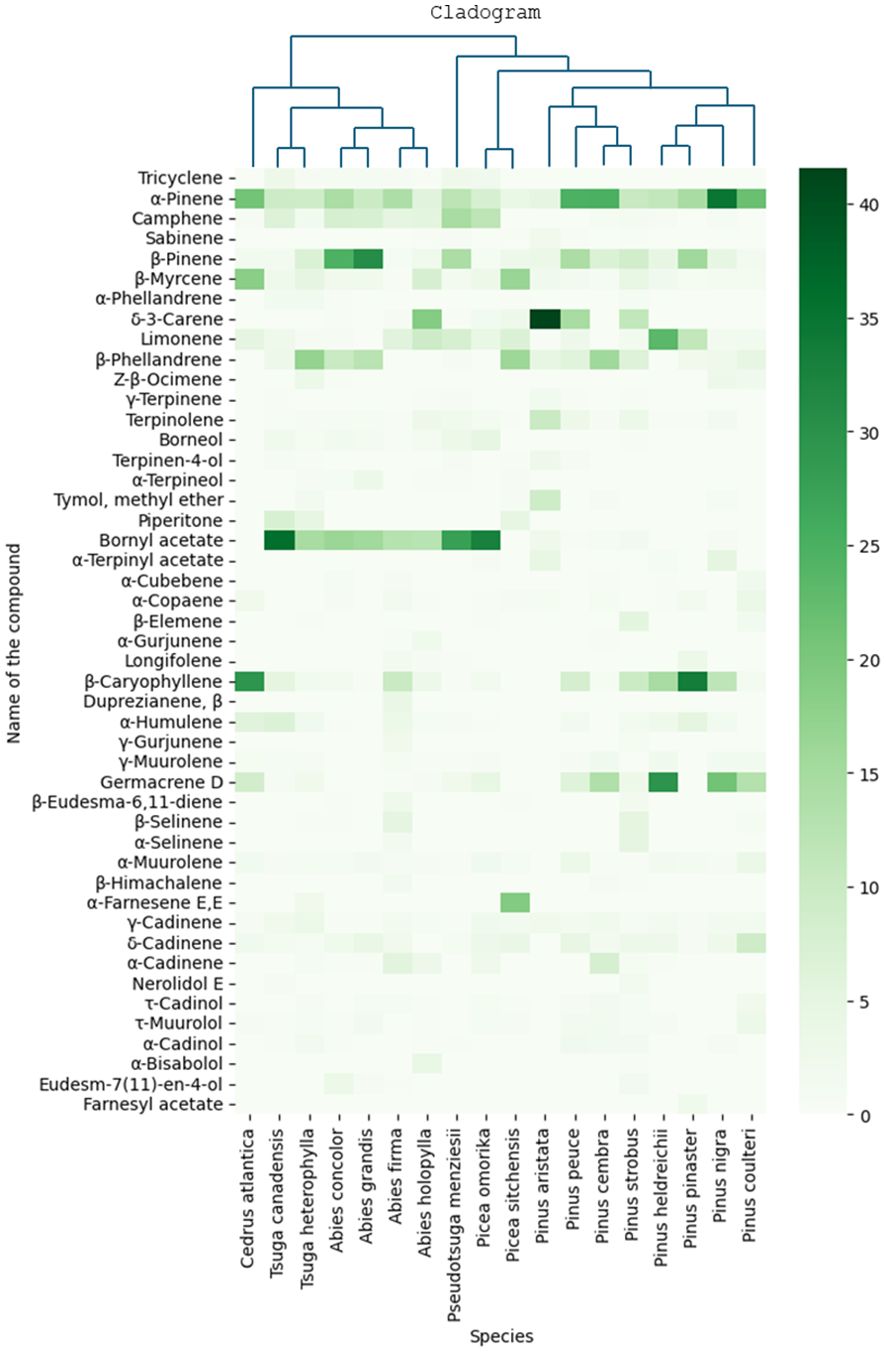
Figure 3. Heat map showing GC/MS results of all needle samples from the Pinaceae family together with the phylogeny of the species. Results are mean area% measured by GC/MS.
3.2 Investigation of the relationships between volatiles and species by PCA
PCA was performed on the GC/MS results obtained for the conifer samples investigated. The PCA biplot revealed that two compounds were characteristic of the samples and thus represent the most considerable variance: α-pinene (RRT=5.1) and bornyl acetate (RRT=12.6). α-Pinene was the most correlated component with axis 1, and samples close to it contained the largest amounts of this compound. Bornyl acetate was highly correlated with axis 2; samples around it have the highest concentration. A large group of samples were accumulated around the origin; the above two compounds were largely missing in these samples. The total variance for the first two axes was 38%. See Figures 4 and 5 (scaled for visibility).
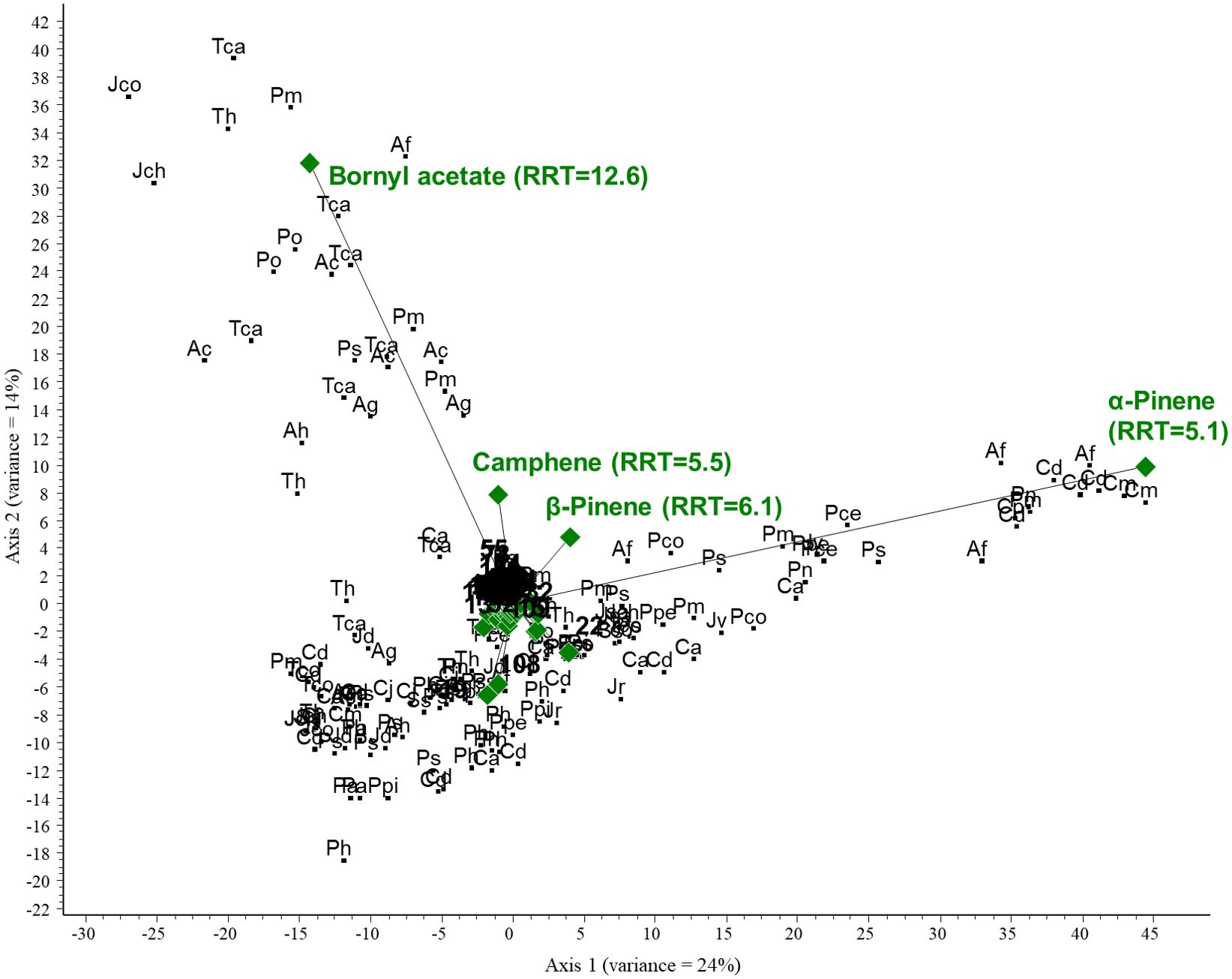
Figure 4. PCA biplot investigating the relationships between volatile organic compounds and plant species. Green diamonds are volatile components (RRT, relative retention time) and black dots are species. Ac, Abies concolor; Af, Abies firma; Ag, Abies grandis; Ah, Abies holophylla; Ca, Cedrus atlantica; Po, Picea omorika; Ps, Picea sitchensis; Pa, Pinus aristata; Pce, Pinus cembra; Pco, Pinus coulteri; Ph, Pinus heldreichii; Pn, Pinus nigra; Ppe, Pinus peuce; Ppi, Pinus pinaster; Ps, Pinus strobus; Pm, Pseudotsuga menziesii; Tca, Tsuga canadensis; Th, Tsuga heterophylla; Cd, Calocedrus decurrens; Cp, Chamaecyparis pisifera; Cj, Cryptomeria japonica; Cm, Cupressus macnabiana; JCh, Juniperus chinensis; Jco, Juniperus communis; Jd, Juniperus drupacea; Jr, Juniperus rigida; Jsa, Juniperus sabina; Jv, Juniperus virginiana; Ss, Sequoia sempervirens; and Tco, Thuja koraiensis.
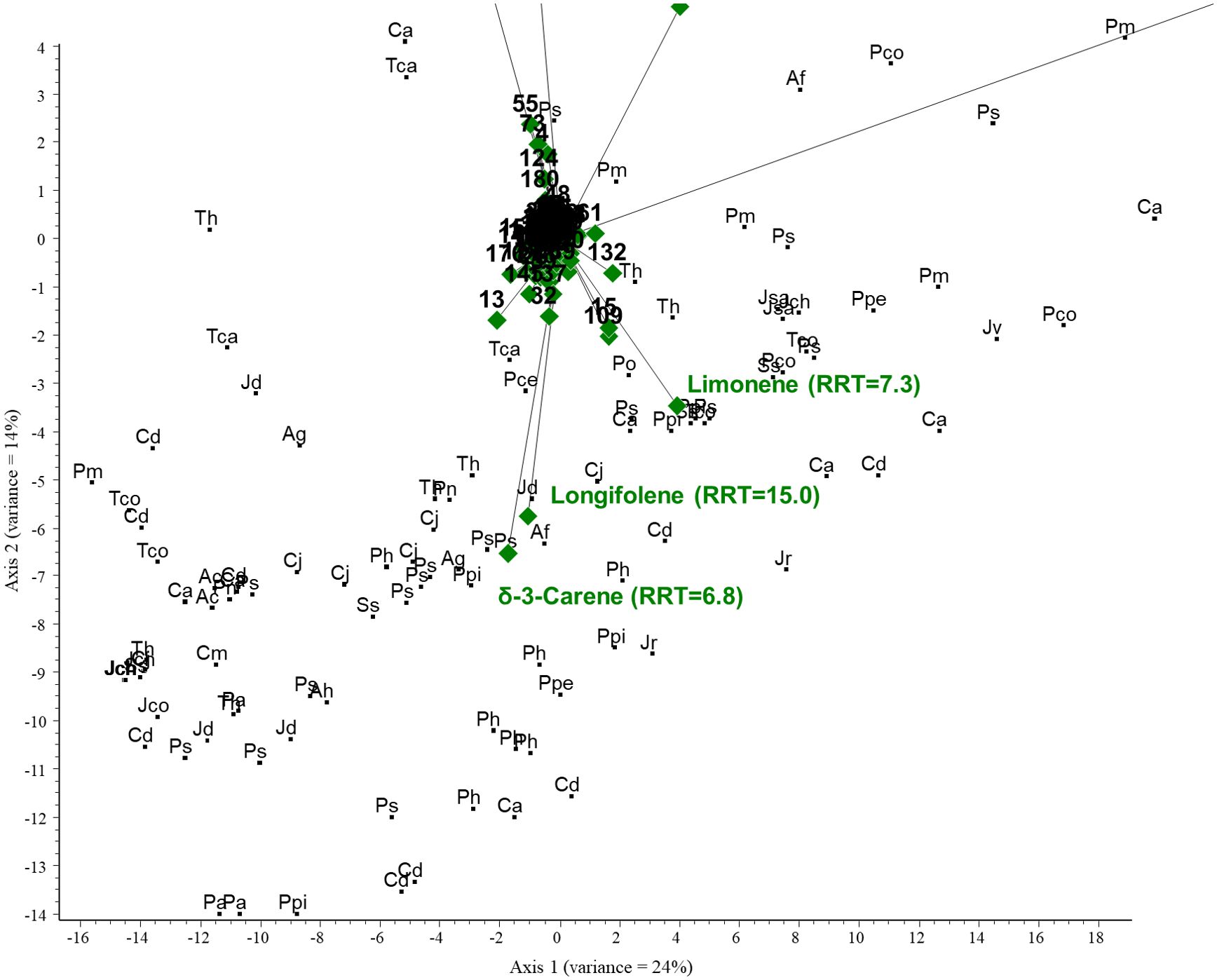
Figure 5. PCA biplot (scaled for visibility) investigating the relationships between volatile organic compounds and plant species. Green diamonds are volatile components (RRT, relative retention time) and black dots are species. Ac, Abies concolor; Af, Abies firma; Ag, Abies grandis; Ah, Abies holophylla; Ca, Cedrus atlantica; Po, Picea omorika; Ps, Picea sitchensis; Pa, Pinus aristata; Pce, Pinus cembra; Pco, Pinus coulteri; Ph, Pinus heldreichii; Pn, Pinus nigra; Ppe, Pinus peuce; Ppi, Pinus pinaster; Ps, Pinus strobus; Pm, Pseudotsuga menziesii; Tca, Tsuga canadensis; Th, Tsuga heterophylla; Cd, Calocedrus decurrens; Cp, Chamaecyparis pisifera; Cj, Cryptomeria japonica; Cm, Cupressus macnabiana; JCh, Juniperus chinensis; Jco, Juniperus communis; Jd, Juniperus drupacea; Jr, Juniperus rigida; Jsa, Juniperus sabina; Jv, Juniperus virginiana; Ss, Sequoia sempervirens; and Tco, Thuja koraiensis.
Then, according to the PCA results, we selected the variables (VOCs) with the highest variance. To investigate the correlation between these VOCs and the three conifer groups, we performed CVA. The three groups were created based on the classification of the species: abietoid (containing Abies, Cedrus, and Tsuga species), pinoid (containing Picea, Pinus and Pseudotsuga species), and cupressoid groups (containing the Cupressaceae species). For the CVA plot, see Figure 6.
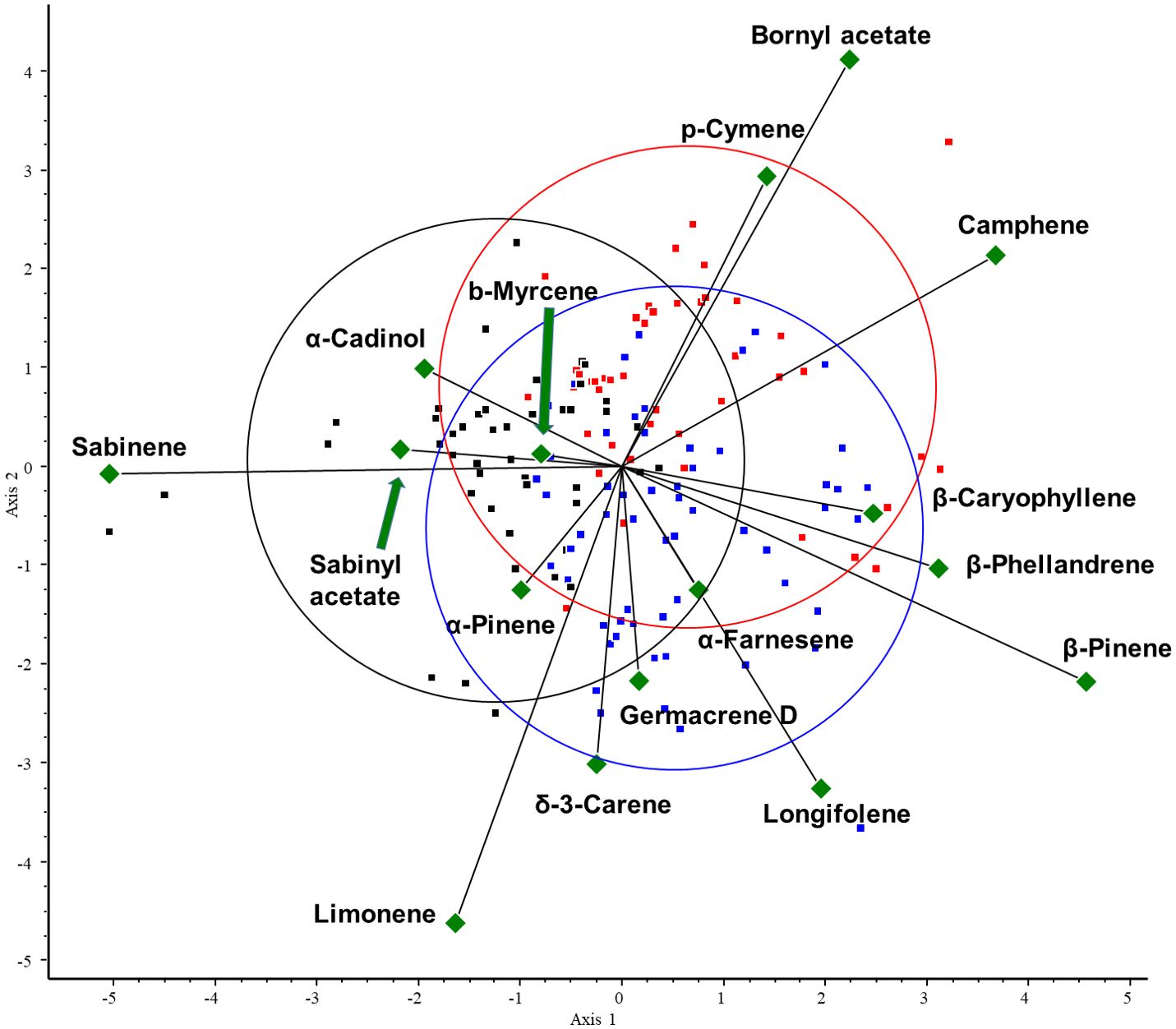
Figure 6. CVA biplot investigating the relationships between volatiles and species. CVA analysis was performed on all samples. Different groups are shown in different colors. Green triangles: volatile components. Red dots: abietoid group (containing Abies, Cedrus, and Tsuga species); blue dots: pinoid group (containing Picea, Pinus, and Pseudotsuga species); and black dots: cupressoid group (containing the Cupressaceae species).
The results of the chemometric analyses indicate that the following volatiles are characteristic of certain groups: sabinene (RRT=6.0) is characteristic of the cupressoid group, longifolene (RRT=15.0) and β-pinene (RRT=6.1) are characteristic of the pinoid group, and camphene (RRT=5.5) and bornyl acetate (RRT=12.6) are characteristic of the abietoid group. The results show a negative correlation between the amount of bornyl acetate and limonene in the samples investigated (see Figure 7). Considering these 5 VOCs (sabinene, longifolene, β-pinene, camphene, and bornyl acetate), statistical analysis (Tukey HSD) showed a significant difference between the volatile profile of cupressoid and pinoid (p=0.023) and cupressoid and abietoid groups (p=0.0057). However, the difference was not statistically significant between the abietoid and cupressoid groups (p=0.776).
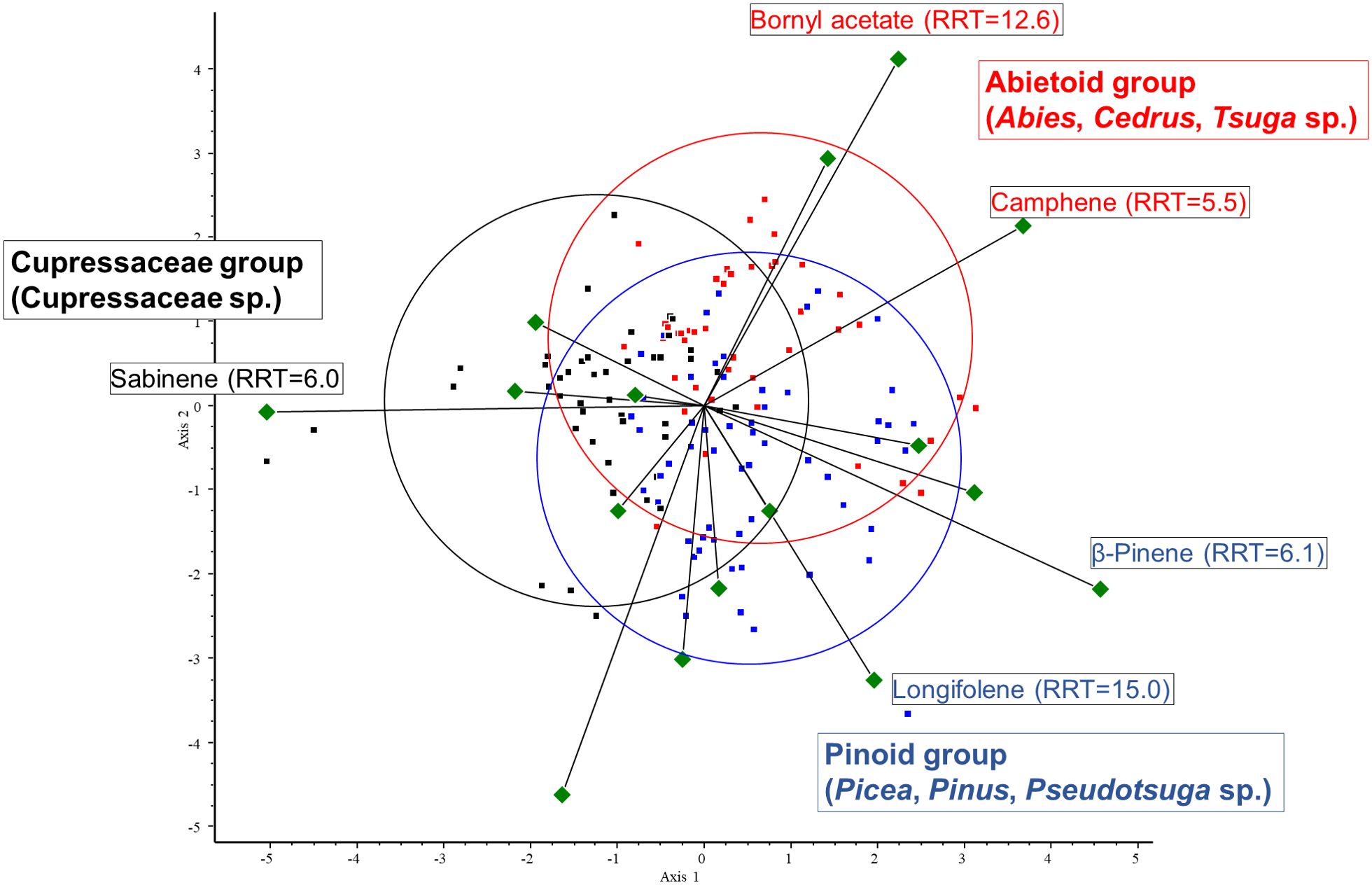
Figure 7. Main results of canonical variate analysis (CVA). Sabinene (RRT=6.0) is characteristic of the cupressoid group, longifolene (RRT=15.0) and β-pinene (RRT=6.1) are characteristic of the pinoid group, and camphene (RRT=5.5) and bornyl acetate (RRT=12.6) are characteristic of the abietoid group. RRT: relative retention time.
3.3 Investigation of the relationships between volatiles and collected plant organs of the Pinaceae family by PCA
We performed centered PCA and then CVA to investigate the relationships between VOCs and collected plant organs of the Pinaceae family. The following plant organs were considered: needles, cones, bark, and resin. PCA was performed on all GC/MS data of the Pinaceae family. The first two ordination axes account for 40% of the total variance. Two compounds are responsible for a large portion of the variance in the data: α-pinene (RRT=5.1) and bornyl acetate (RRT=12.6). α-Pinene was present in large quantities in the samples. Samples near the arrowheads contained large amounts of the respective compound. Camphene and β-pinene are positively correlated, whereas longifolene is negatively correlated with axis 2. The results show a negative correlation between the amount of bornyl acetate and limonene in the samples investigated. For the PCA biplot, see Figure 8.
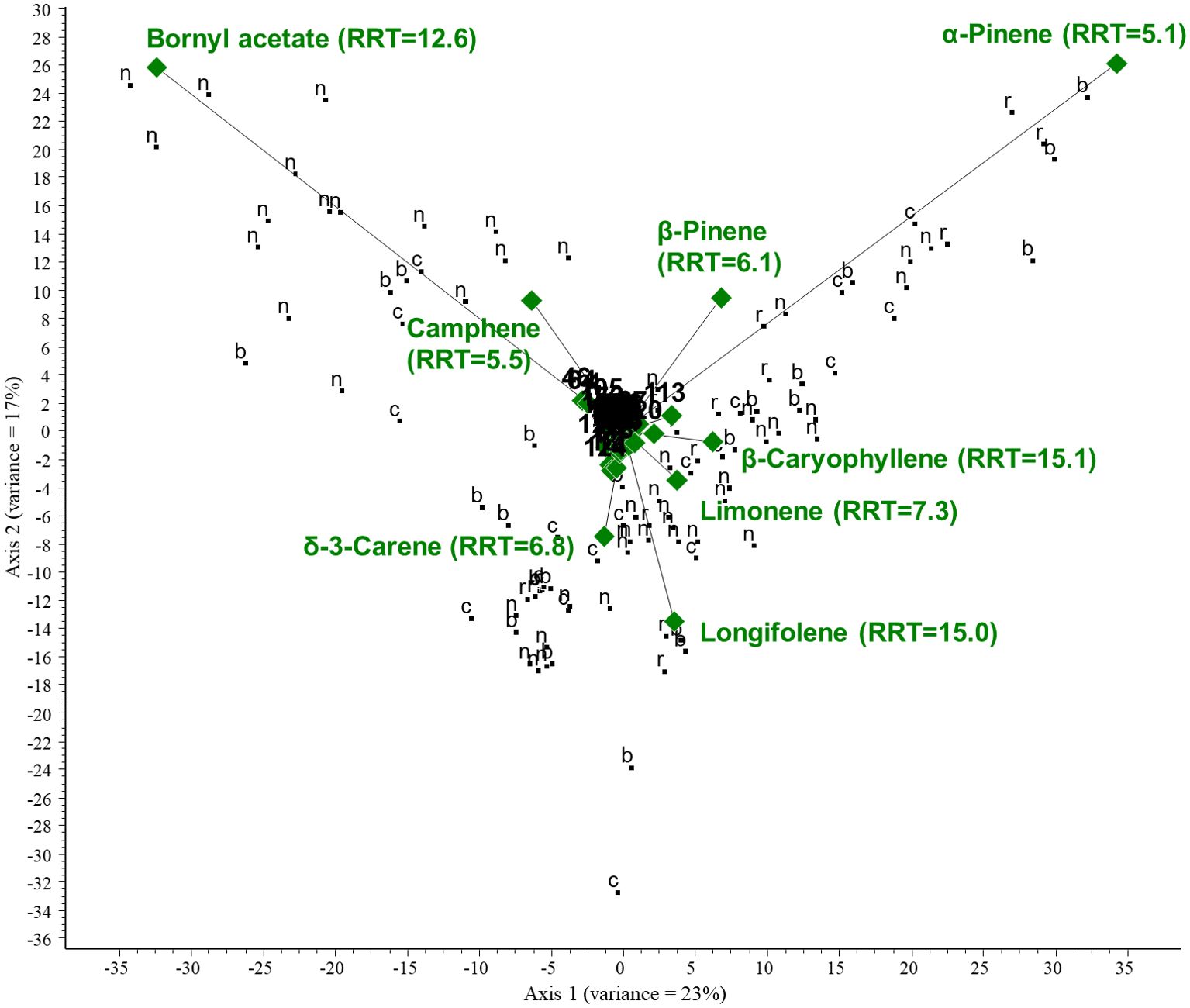
Figure 8. PCA biplot investigating the relationships between volatiles and collected plant organs of the Pinaceae family. Green diamonds are volatile components (RRT, relative retention time) and black dots are plant organs. n, needles; c, cones; r, resin; b, bark.
Then, to investigate the correlation between the VOCs with the highest variance and the plant organs, CVA was performed on the data. The results of the chemometric analyses show that β-caryophyllene (RRT=15.1) and germacrene D (RRT=16.2) are characteristic of needles, and their amounts are negatively correlated with α-pinene. Longifolene and α-pinene are characteristic of bark samples. There is also a negative correlation between the amounts of p-cymene and β-phellandrene or β-myrcene. For the CVA plot, see Figure 9. The chemical composition (mean area%) of samples of the Pinaceae family categorized by plant organs is presented in Table 4 (only the highest values are shown).
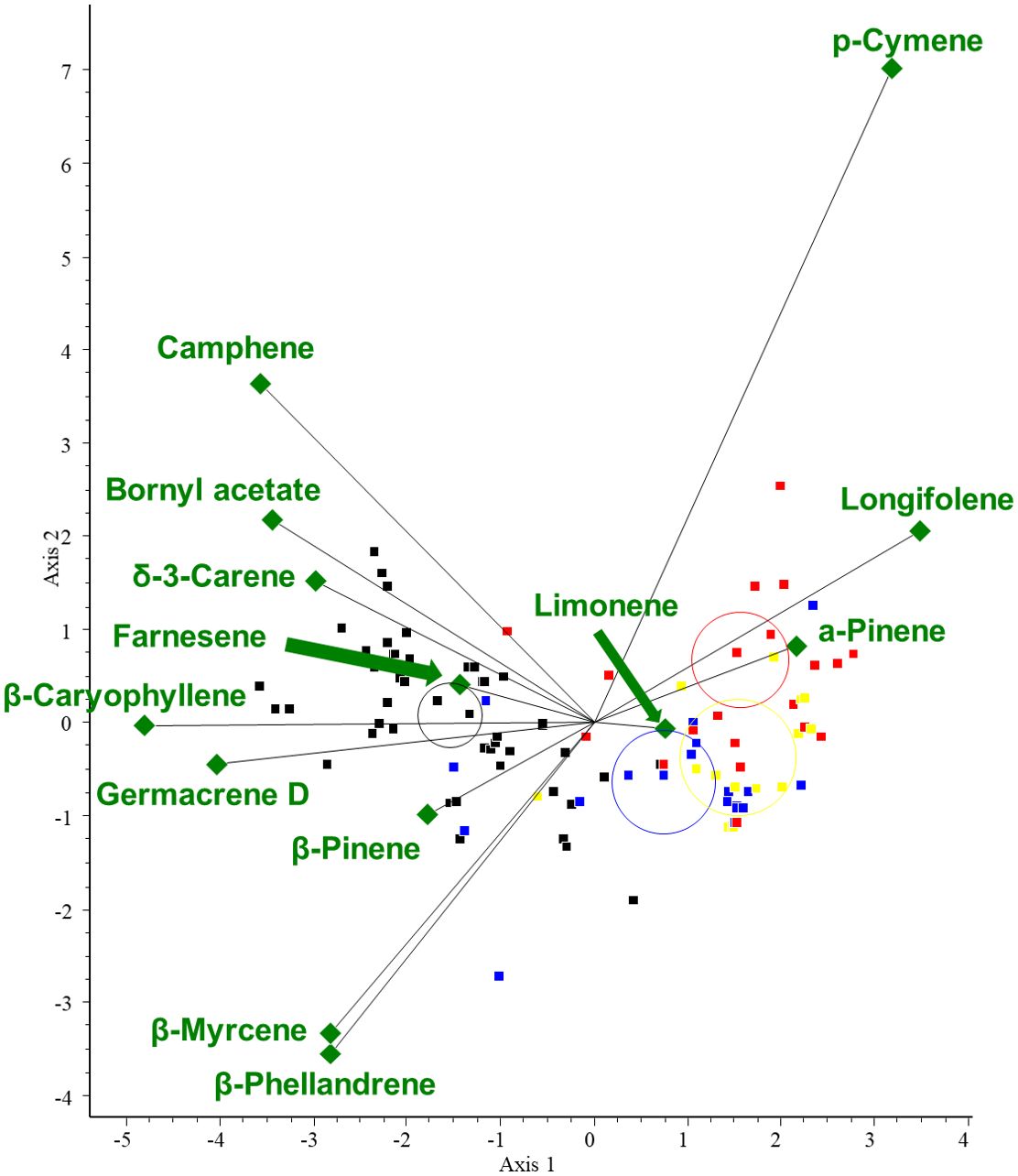
Figure 9. CVA biplot investigating the relationships between volatiles and collected plant organs of the Pinaceae family. Different groups are shown in different colors. Green diamonds: volatile components. Red dots: bark; blue dots: cones; black dots: needles; yellow dots: resin.
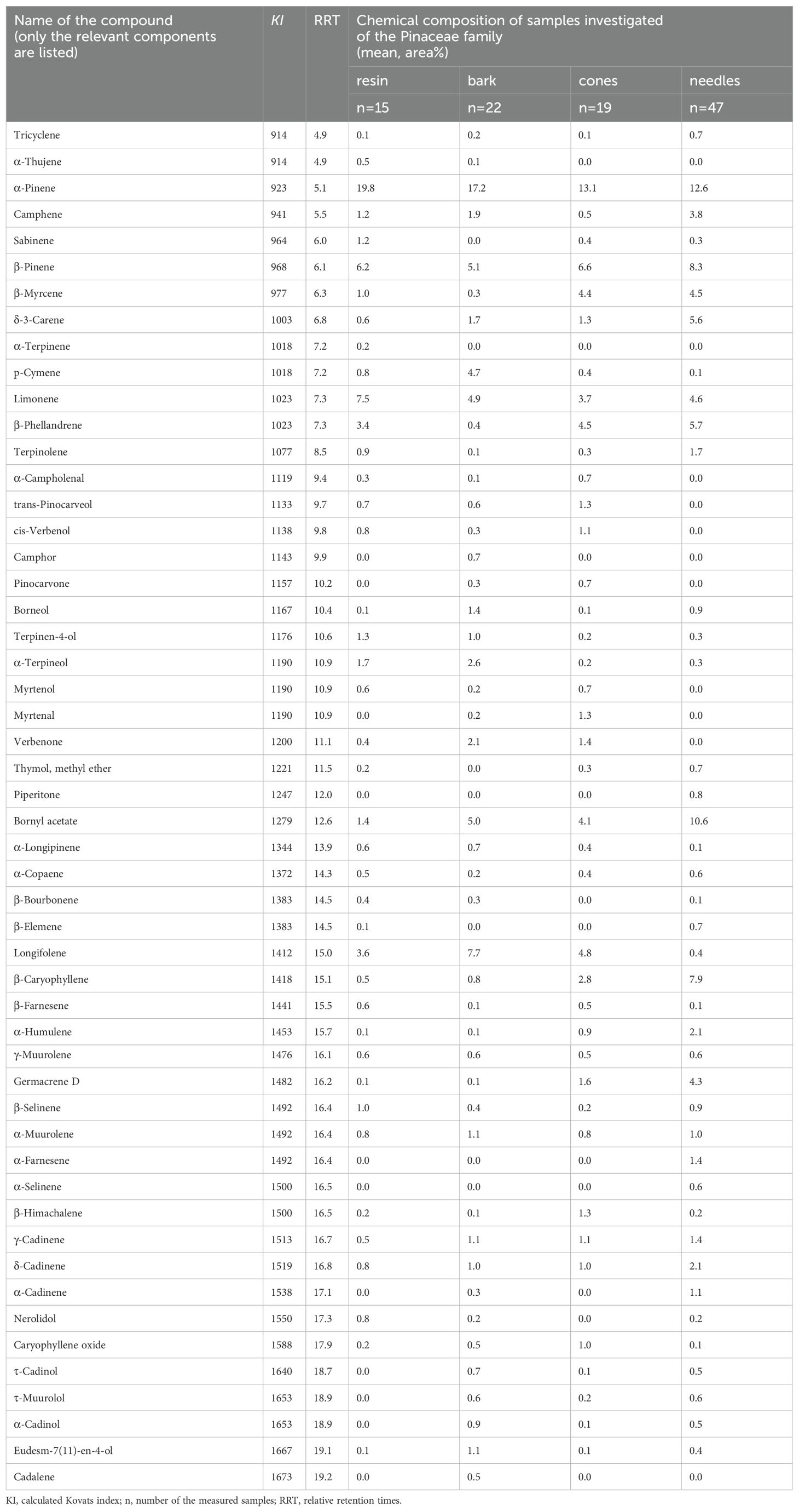
Table 4. Volatile organic compounds expressed in the mean area% of samples measured by the SPME-GC/MS method in the Pinaceae family and categorized by plant organs.
4 Discussion
Terpenes represent a major group of plant metabolites in conifers. Monoterpenes and sesquiterpenes are essential oil components, whereas terpenoids with higher molecular weight, such as diterpenes, are usually not volatile. The volatile profiles of species are mainly determined by the terpene synthase (TPS) family (Alicandri et al., 2020); however, the actual amounts of volatiles in the plant part depend on different factors. These factors include developmental factors, stress, and other stimuli (temperature, biotic stress, pollution, season, etc.) (Kopaczyk et al., 2020).
We acknowledge that there are certain constraints on the results and conclusions because the sample tissues were collected from two artificially managed arboreta in the same region (Hungary). Whereas such a sampling scheme is not suitable for evaluating overall geographic variability in the volatile oil composition of any plant, it ensures that the trees were grown under very similar environmental conditions, thereby guaranteeing that our observations are less dependent on the environment. In this way, differences among species are more likely attributable to their own metabolism.
We reviewed the literature to compare our GC/MS results with the previously reported analyses. The major VOCs identified by our study in the abietoid group were α-pinene, β-pinene, β-caryophyllene, β-phellandrene and bornyl acetate, which is consistent with the literature. The major components found in our samples of Abies concolor (needles) were α-pinene, camphene, β-pinene, and bornyl acetate. In contrast to the analysis by Swor et al., the amount of δ-3-carene (0-1%) and limonene (0-2%) were negligible in our samples, but the other VOCs were found in similar amounts (Swor et al., 2022). In this analysis by Swor et al., the authors also provided the main chemical compounds of A. grandis, such as α-pinene, camphene, β-pinene, β-phellandrene, and bornyl acetate. They concluded that the investigated compositions were qualitatively similar to other Abies essential oils examined in the literature. The main components from our A. grandis samples were qualitatively similar. Sarria et al. identified an α/β pinene synthase in A. grandis, which produces about 40% α-pinene and about 60% of β-pinene (Sarria et al., 2014). This can also be a reasonable cause of the larger amount of β-pinene (31%) compared to α-pinene (10%) in our samples. According to Na-Hyun Lee at al., the major compounds of Abies firma were bornyl acetate, δ-3-carene, α-pinene, β-caryophyllene, and camphene (Lee et al., 2015). According to Satou at al. the main constituents of A. firma were α-pinene and δ-3-carene (Satou et al., 2011). The main VOCs of our Abies firma samples were consistent with the reported analyses, however a major difference was our samples containing only a low amount of δ-3-carene (0.7%). Based on the reported analysis of Lee and Hong, the main components in A. holophylla essential oil were bicyclo[2.2.1]heptan-2-ol (28%), δ-3-carene, α-pinene, camphene, limonene, β-myrcene (Lee and Hong, 2009). Bornyl acetate (bicyclo[2,2,1]-heptan-2-ol-1,7,7-trimethyl-acetate) was not detected by Lee and Hong, however our samples contained a considerable amount of this VOC (13%). Based on the above detailed chemical profile data of previous studies we can conclude that our results of Abies species align with those found in the literature. According to Boudarene et al., the main VOCs of Cedrus atlantica oils were α-pinene and β-caryophyllene. Our results are in line with these data, however we detected β-myrcene in considerable amount (18%). In contrast to other analyses reported in the literature, himachalenes were negligible in our samples (Boudarene et al., 2004; Ainane et al., 2019; Ailli et al., 2023). Bornyl acetate and α-pinene were reported as the most abundant VOCs of Tsuga heterophylla and T. canadensis species in the study of Lagalante (Lagalante and Montgomery, 2003). Correspondingly, our Tsuga samples contained a high percentage of α-pinene and bornyl acetate, however, the ratio of β-phellandrene was also high (17%) in our T. heterophylla samples. A study by Ankney et al. reported that one of the major components in T. heterophylla essential oil is β-phellandrene (6.6–19.3%) (Ankney et al., 2024). In summary, our findings are consistent with the literature.
The major VOCs in the pinoid group identified by us were α-pinene, β-pinene, bornyl acetate, β-phellandrene, β-caryophyllene and β-myrcene, which is consistent with the literature. Nikolic et al. reported that the main compounds of Picea omorika were bornyl acetate (29.2%), camphene (18.7%), and a-pinene (12.9%) (Nikolic et al., 2009). In our samples, the main components were the same, and their quantity closely matches the results of Nikolic et al. Furthermore, our results are consistent with the findings of Hall et al., as the main components of P. sitchensis were α-pinene, β-myrcene, limonene, β-phellandrene (Hall et al., 2011). However, in our samples, the amount of α-farnesene was high (19%). Farnesenes are reported to influence the behavior of insects, for example, Picea sitchensis, when attacked by pine weevils, releases α- and β-farnesenes (Kännaste et al., 2008). The main components of our P. nigra samples were α-pinene, germacrene D, and β-caryophyllene, which is in accordance with the results of Sarac et al. (2013); Koutsaviti et al. (2015), and Mitic et al. (2018), however Mitic et al. identified a relatively high percentage of β-pinene (10%) when compared to that of our samples (5%). Koutsaviti reported that their analyses were in line with the previous studies on the chemical composition of P. nigra needle oils. The most abundant VOCs of our P. cembra needle samples were α-pinene, β-pinene, and β-phellandrene, which is in line with the findings of Dormont et al. (1998). The major components of our samples in P. heldreichii were limonene, α-pinene, germacrene D, and β-caryophyllene. These data align with the results of Mitic et al. (2019) and Basholli-Salihu et al. (2017). Pinus aristata proved to contain the highest amount of δ-3-carene among our samples (42%), which is in good agreement with the findings described by Faiola et al. (2015). Despite the high variability, their analysis also suggests that the same seven compounds can be found in the largest amount in Pinus aristata, Abies grandis, and Pseudotsuga menziesii. The major components, as stated in the report, were: α-pinene, limonene, δ-3-carene, β-pinene, β-myrcene, camphene, and β-phellandrene. Our results do not confirm this statement, as P. aristata also contained terpinolene (10%) while P. menziesii and A. grandis contained bornyl acetate (28% and 16%, respectively). Major VOCs in our P. peuce samples were α-pinene, germacrene D, β-phellandrene and β-pinene and their quantities were similar as described in the literature (Nikolic et al., 2008; Basholli-Salihu et al., 2017; Mitic et al., 2018). However, δ-3-carene and β-caryophyllene were also considerable in our samples (15% and 8%, respectively). Corresponding to our results P. strobus contains high percentage of α-pinene, β-pinene, δ-3-carene, and β-caryophyllene. According to Koutsaviti et al. a considerable amount of β-pinene, α-pinene, and β-caryophyllene were identified in P. strobus. They concluded that quantitative differences were observed between their analysis and other analyses in the literature; however, qualitative similarities were observed (Krauze-Baranowska et al., 2002; Koutsaviti et al., 2015). The primary VOCs of our P. menziesii samples were bornyl acetate, camphene, and β-pinene. Based on the findings of Swor et al. (2023), the chemical profile of P. menziesii var. glauca is characterized by high levels of bornyl acetate and camphene, which is consistent with our results.
Sabinene is a typical component of the Cupressaceae family (Foster et al., 2013), and our results support this. In addition, α-thujone and β-thujone were detected in the Cupressaceae family but were absent in the samples of the Pinaceae family. Thujone is formed from sabinene, and sabinol is an intermediate product in thujone biosynthesis (Nemeth and Nguyen, 2020). In most cases where thujone was detected in our samples, sabinol was also present; see Table 5 for all samples containing thujone.
Our research indicates differences in the terpene profiles of the plant organs of species from Pinaceae family (see Figure 9), which is in line with the literature (Dormont et al., 1998; Chizzola and Müllner, 2021; Butnaru et al., 2022). According to previous studies, the proportional amounts of several terpenes show strong positive correlations among different plant parts in conifers, or the same main VOCs could be identified in them (Dormont et al., 1998; Manninen et al., 2002). In addition, terpenoid production might be controlled not only by genetics, but also by environmental factors or plant organ development (de Simon et al., 2021; Bai, 2022). It is important to mention that although some research has observed significant differences in diterpenes (Chizzola and Müllner, 2021; de Simon et al., 2021; Nantongo et al., 2022), we did not investigate them in our study. The limitation of our study is that the collection of samples was not completely consistent (i.e., we did not always collect and analyze the same plant parts and did not consider the developmental stages of the plant organ). For future trials, we recommend using standardized and more comprehensive sample collections to improve the reliability of the data and reflect a more accurate composition of VOCs.
Terpenoid metabolites are synthesized in plants by specific terpene synthase enzymes. This is an enormous and intensively researched area (Karunanithi and Zerbe, 2019; Alicandri et al., 2020), and it is particularly important to investigate the relationships between the phylogeny and the terpene profiles of the species. This knowledge is necessary to refine metabolic engineering and synthetic biology tools to optimize the efficient production of economically valuable terpenoid compounds in the future. Our data on conifer VOCs can provide valuable scientific information for further research in this area.
5 Conclusion
In this comprehensive study, monoterpenes, sesquiterpenes, and their derivatives were analyzed by SPME-GC/MS from samples of 30 conifer species collected from arboreta in Hungary. A total of 151 samples (needles, cones, barks, and resin samples) were measured. Our gas chromatographic analyses in the Pinaceae family (103 samples) reveal that the most abundant components are α-pinene, β-pinene, bornyl acetate, limonene, and β-caryophyllene. In the Cupressaceae family (50 samples), the most important components are α-pinene, limonene, sabinene, β-myrcene, and δ-3-carene. In addition, further multivariate analyses (PCA and CVA) were performed on the analytical results to explore the correlation between chemical composition and species or plant organs. The detected VOCs showed similarities and differences within species and also among organs or higher taxonomic order levels such as genera. Characteristic chemical components were identified, such as sabinene for the cupressoid group, camphene and bornyl acetate for the abietoid group, and longifolene and β-pinene for the pinoid group.
Our present research performed on several conifer species provides comprehensive data on VOCs in the Pinaceae and Cupressaceae families. Furthermore, these data can complement our understanding of the biodiversity of conifer species and support other research on the production of terpenes by synthetic biology.
Data availability statement
The raw data supporting the conclusions of this article will be made available by the authors, without undue reservation.
Author contributions
EB: Conceptualization, Investigation, Visualization, Writing – original draft. AB: Conceptualization, Supervision, Writing – review & editing. BVSz: Writing – review & editing, Formal analysis, Visualization. MAE: Conceptualization, Methodology, Writing – review & editing. PH: Conceptualization, Supervision, Writing – review & editing. AV: Conceptualization, Supervision, Writing – review & editing. DCs: Conceptualization, Supervision, Writing – review & editing.
Funding
The author(s) declare that financial support was received for the research, authorship, and/or publication of this article. AV gratefully acknowledges the support of the Semmelweis Fund for Science and Innovation (STIA-OTKA-2022) and the National Research, Development and Innovation Office, Hungary (OTKA NKFIH FK-146487).
Acknowledgments
The authors thank Tamás Ruborits (Jeli Arboretum) and Zsuzsanna Rónai (Folly Arboretum) for their support and assistance in sample collection and plant identification. Special thanks to Réka Folly, owner of Folly Arboretum; our cooperation would not have been possible without her consent. We are also grateful to János Podani, professor at Eötvös Loránd University, for his advice and teaching on multivariate analyses and János Rácz, founder of the International Dendrological Foundation, for reviewing the manuscript.
Conflict of interest
The authors declare that the research was conducted in the absence of any commercial or financial relationships that could be construed as a potential conflict of interest.
The author(s) declared that they were an editorial board member of Frontiers, at the time of submission. This had no impact on the peer review process and the final decision.
Publisher’s note
All claims expressed in this article are solely those of the authors and do not necessarily represent those of their affiliated organizations, or those of the publisher, the editors and the reviewers. Any product that may be evaluated in this article, or claim that may be made by its manufacturer, is not guaranteed or endorsed by the publisher.
Supplementary material
The Supplementary Material for this article can be found online at: https://www.frontiersin.org/articles/10.3389/fpls.2024.1392539/full#supplementary-material
References
Adams, R. P. (2007). Identification of essential oil components by gas chromatography/mass spectroscopy (Carol Stream: Allured Publishing Corporation).
Ailli, A., Handaq, N., Touijer, H., Gourich, A. A., Drioiche, A., Zibouh, K., et al. (2023). Phytochemistry and biological activities of essential oils from six aromatic medicinal plants with cosmetic properties. Antibiotics-Basel 12. doi: 10.3390/antibiotics12040721
Ainane, A., Khammour, Charaf, S., Elabboubi, M., Elkouali, M., Talbi, M., et al. (2019). Chemical composition and insecticidal activity of five essential oils: Cedrus atlantica, Citrus limonum, Rosmarinus officinalis, Syzygium aromaticum and Eucalyptus globules. Mater. Today Proceedings 13, 474–485. doi: 10.1016/j.matpr.2019.04.004
Alicandri, E., Paolacci, A. R., Osadolor, S., Sorgonà, A., Badiani, M., Ciaffi, M. (2020). On the evolution and functional diversity of terpene synthases in the species: A review. J. Mol. Evol. 88, 253–283. doi: 10.1007/s00239-020-09930-8
Allenspach, M., Steuer, C. (2021). alpha-Pinene: A never-ending story. Phytochemistry 190, 112857. doi: 10.1016/j.phytochem.2021.112857
Anandakumar, P., Kamaraj, S., Vanitha, M. K. (2021). D-limonene: A multifunctional compound with potent therapeutic effects. J. Food Biochem. 45, e13566. doi: 10.1111/jfbc.13566
Ankney, E., Swor, K., Poudel, A., Satyal, P., da Silva, J. K. R., Setzer, W. N. (2024). Chemical Compositions and Enantiomeric Distributions of Foliar Essential Oils of Chamaecyparis lawsoniana (A. Murray bis) Parl, Thuja plicata Donn ex D. Don, and Tsuga heterophylla Sarg. Plants (Basel) 13. doi: 10.3390/plants13101325
Bai, Q. S. (2022). Comparative transcriptomics of Pinus massoniana organs provides insights on terpene biosynthesis regulation. Physiol. Plant. 174. doi: 10.1111/ppl.13791
Barnes, T. M., Greive, K. A. (2017). Topical pine tar: History, properties and use as a treatment for common skin conditions. Australas. J. Dermatol. 58, 80–85. doi: 10.1111/ajd.12427
Basholli-Salihu, M., Schuster, R., Hajdari, A., Mulla, D., Viernstein, H., Mustafa, B., et al. (2017). Phytochemical composition, anti-inflammatory activity and cytotoxic effects of essential oils from three Pinus spp. Pharm. Biol. 55, 1553–1560. doi: 10.1080/13880209.2017.1309555
Batista, J. V. C., Uecker, A., Holandino, C., Boylan, F., Maier, J., Huwyler, J., et al. (2022). A scoping review on the therapeutic potential of resin from the species Larix decidua mill. [Pinaceae] to treat ulcerating wounds. Front. Pharmacol. 13. doi: 10.3389/fphar.2022.895838
Bhardwaj, K., Islam, M. T., Jayasena, V., Sharma, B., Sharma, S., Sharma, P., et al. (2020). Review on essential oils, chemical composition, extraction, and utilization of some conifers in Northwestern Himalayas. Phytother. Res. 34, 2889–2910. doi: 10.1002/ptr.6736
Boudarene, L., Rahim, L., Baaliouamer, A., Meklati, B. Y. (2004). Analysis of Algerian essential oils from twigs, needles and wood of Cedrus atlantica G.Manetti by GC/MS. J. Essential Oil Res. 16, 531–534. doi: 10.1080/10412905.2004.9698790
Butnaru, E., Pamfil, D., Stoleru, E., Brebu, M. (2022). Characterization of bark, needles and cones from silver fir (Abies alba mill.) towards valorization of biomass forestry residues. Biomass Bioenergy 159. doi: 10.1016/j.biombioe.2022.106413
Cao, Y., Zhang, H., Liu, H., Liu, W., Zhang, R., Xian, M., et al. (2018). Biosynthesis and production of sabinene: current state and perspectives. Appl. Microbiol. Biotechnol. 102, 1535–1544. doi: 10.1007/s00253-017-8695-5
Celedon, J. M., Bohlmann, J. (2019). Oleoresin defenses in conifers: chemical diversity, terpene synthases and limitations of oleoresin defense under climate change. New Phytol. 224, 1444–1463. doi: 10.1111/nph.15984
Chizzola, R., Müllner, K. (2021). Variability of volatiles in Pinus cembra L. within and between trees from a stand in the Salzburg Alps (Austria) as assessed by essential oil and SPME analysis. Genet. Resour. Crop Evol. 68, 567–579. doi: 10.1007/s10722-020-01006-2
de Simon, B. F., Aranda, I., López-Hinojosa, M., Miguel, L., Cervera, M. T. (2021). Scion-rootstock interaction and drought systemic effect modulate the organ-specific terpene profiles in grafted Pinus pinaster Ait. Environ. Exp. Bot. 186. doi: 10.1016/j.envexpbot.2021.104437
Dietrich, G., Dolan, M. C., Peralta-Cruz, J., Schmidt, J., Piesman, J., Eisen, R. J., et al. (2006). Repellent activity of fractioned compounds from essential oil against nymphal (Acari: Ixodidae). J. Med. Entomol. 43, 957–961. doi: 10.1603/0022-2585(2006)43[957:Raofcf]2.0.Co;2
Dormont, L., Roques, A., Malosse, C. (1998). Cone and foliage volatiles emitted by Pinus cembra and some related conifer species. Phytochemistry 49, 1269–1277. doi: 10.1016/S0031-9422(98)00105-8
Faiola, C. L., Jobson, B. T., VanReken, T. M. (2015). Impacts of simulated herbivory on volatile organic compound emission profiles from coniferous plants. Biogeosciences 12, 527–547. doi: 10.5194/bg-12-527-2015
Foster, A. J., Hall, D. E., Mortimer, L., Abercromby, S., Gries, R., Gries, G., et al. (2013). Identification of genes in Thuja plicata foliar terpenoid defenses. Plant Physiol. 161, 1993–2004. doi: 10.1104/pp.112.206383
Gadek, P. A., Alpers, D. L., Heslewood, M. M., Quinn, C. J. (2000). Relationships within Cupressaceae sensu lato: A combined morphological and molecular approach. Am. J. Bot. 87, 1044–1057. doi: 10.2307/2657004
Gyrdymova, Y. V., Rubtsova, S. A. (2022). Caryophyllene and caryophyllene oxide: a variety of chemical transformations and biological activities. Chem. Papers 76, 1–39. doi: 10.1007/s11696-021-01865-8
Hall, D. E., Robert, J. A., Keeling, C. I., Domanski, D., Quesada, A. L., Jancsik, S., et al. (2011). An integrated genomic, proteomic and biochemical analysis of (+)-3-carene biosynthesis in Sitka spruce (Picea sitchensis) genotypes that are resistant or susceptible to white pine weevil. Plant J. 65, 936–948. doi: 10.1111/j.1365-313X.2010.04478.x
Jiang, H., Wang, X. (2023). Biosynthesis of monoterpenoid and sesquiterpenoid as natural flavors and fragrances. Biotechnol. Adv. 65. doi: 10.1016/j.bioteChadv.2023.108151
Kännaste, A., Vongvanich, N., Borg-Karlson, A. K. (2008). Infestation by a Nalepella species induces emissions of α- and β-farnesenes, (–)-linalool and aromatic compounds in Norway spruce clones of different susceptibility to the large pine weevil. Arthropod Plant Interact. 2, 31–41. doi: 10.1007/s11829-008-9029-4
Karunanithi, P. S., Zerbe, P. (2019). Terpene synthases as metabolic gatekeepers in the evolution of plant terpenoid chemical diversity. Front. Plant Sci. 10. doi: 10.3389/fpls.2019.01166
Knudsen, J. T., Tollsten, L., Bergström, L. G. J. P. (1993). Floral scents—a checklist of volatile compounds isolated by head-space techniques. Phytochemistry 33(22), 253–280. doi: 10.1016/0031-9422(93)85502-I
Kopaczyk, J. M., Wargula, J., Jelonek, T. (2020). The variability of terpenes in conifers under developmental and environmental stimuli. Environ. Exp. Bot. 180. doi: 10.1016/j.envexpbot.2020.104197
Koutsaviti, K., Giatropoulos, A., Pitarokili, D., Papachristos, D., Michaelakis, A., Tzakou, O. (2015). Greek Pinus essential oils: larvicidal activity and repellency against Aedes albopictus (Diptera: Culicidae). Parasitol. Res. 114, 583–592. doi: 10.1007/s00436-014-4220-2
Krauze-Baranowska, M., Mardarowicz, M., Wiwart, M., Poblocka, L., Dynowska, M. (2002). Antifungal activity of the essential oils from some species of the genus Pinus. Z Naturforsch. C J. Biosci. 57, 478–482. doi: 10.1515/znc-2002-5-613
Lagalante, A. F., Montgomery, M. E. (2003). Analysis of terpenoids from hemlock (Tsuga) species by solid-phase microextraction/gas chromatography/ion-trap mass spectrometry. J. Agric. Food Chem. 51, 2115–2120. doi: 10.1021/jf021028s
Lee, J. H., Hong, S. K. (2009). Comparative analysis of chemical compositions and antimicrobial activities of essential oils from Abies holophylla and Abies koreana activities of essential oils from Abies holophylla and Abies koreana. J. Microbiol. Biotechnol. 19, 372–377. doi: 10.4014/jmb.0811.630
Lee, N. H., Lee, S. M., Lee, T. M., Chung, N., Lee, H. S. (2015). GC-MS analyses of the essential oils obtained from pinaceae leaves in Korea. J. Essential Oil Bearing Plants 18, 538–542. doi: 10.1080/0972060x.2013.764194
Linstrom, P.J.M., W.G. NIST chemistry WebBook, NIST standard reference database number 69. Available online at: https://webbook.nist.gov/ (Accessed August 29, 2023).
Liu, X. Q., Xia, X. M., Chen, L., Wang, X. Q. (2022). Phylogeny and evolution of Cupressaceae: Updates on intergeneric relationships and new insights on ancient intergeneric hybridization. Mol. Phylogenet Evol. 177, 107606. doi: 10.1016/j.ympev.2022.107606
Manninen, A. M., Tarhanen, S., Vuorinen, M., Kainulainen, P. (2002). Comparing the variation of needle and wood terpenoids in Scots pine provenances. J. Chem. Ecol. 28, 211–228. doi: 10.1023/A:1013579222600
Mao, K., Ruhsam, M., Ma, Y., Graham, S. W., Liu, J., Thomas, P., et al. (2019). A transcriptome-based resolution for a key taxonomic controversy in Cupressaceae. Ann. Bot. 123, 153–167. doi: 10.1093/aob/mcy152
Mitic, Z. S., Jovanovic, B., Jovanovic, S. C., Mihajilov-Krstev, T., Stojanovi-Radic, Z. Z., Cvetkovic, V. J., et al. (2018). Comparative study of the essential oils of four Pinus species: Chemical composition, antimicrobial and insect larvicidal activity. Ind. Crops Prod. 111, 55–62. doi: 10.1016/j.indcrop.2017.10.004
Mitic, Z. S., Jovanovic, B., Jovanovic, S. C., Stojanovic-Radic, Z. Z., Mihajilov-Krstev, T., Jovanovic, N. M., et al. (2019). Essential oils of Pinus halepensis and P. heldreichii: Chemical composition, antimicrobial and insect larvicidal activity. Ind. Crops Prod. 140. doi: 10.1016/j.indcrop.2019.111702
Nantongo, J. S., Potts, B. M., Davies, N. W., Fitzgerald, H., Rodemann, T., O’Reilly-Wapstra, J. (2022). Variation in constitutive and induced chemistry in the needles, bark and roots of young Pinus radiata trees. Trees Struct. Funct. 36, 341–359. doi: 10.1007/s00468-021-02209-5
Neis, F. A., de Costa, F., de Araújo, A. T., Fett, J. P., Fett-Neto, A. G. (2019). Multiple industrial uses of non-wood pine products. Ind. Crops Prod. 130, 248–258. doi: 10.1016/j.indcrop.2018.12.088
Nemeth, E. Z., Nguyen, H. T. (2020). Thujone, a widely debated volatile compound: What do we know about it? Phytochem. Rev. 19, 405–423. doi: 10.1007/s11101-020-09671-y
Nikolic, B., Ristic, M., Bojovic, S., Marin, P. D. (2008). Variability of the needle essential oils of Pinus peuce from different populations in Montenegro and Serbia. Chem. Biodivers. 5, 1377–1388. doi: 10.1002/cbdv.200890126
Nikolic, B., Tesevic, V., Dordevic, I., Marin, P. D., Bojovic, S. (2009). Essential oil variability in natural populations of Picea omorika, a rare European conifer. Chem. Biodivers. 6, 193–203. doi: 10.1002/cbdv.200800085
Ninkuu, V., Zhang, L., Yan, J. P., Fu, Z. C., Yang, T. F., Zeng, H. M. (2021). Biochemistry of terpenes and recent advances in plant protection. Int. J. Mol. Sci. 22. doi: 10.3390/ijms22115710
Ocete, M. A., Risco, S., Zarzuelo, A., Jimenez, J. (1989). Pharmacological activity of the essential oil of Bupleurum-Gibraltaricum - anti-inflammatory activity and effects on isolated rat uteri. J. Ethnopharmacol. 25, 305–313. doi: 10.1016/0378-8741(89)90036-6
Podani, J. (1997). Bevezetés a többváltozós biológiai adatfeltárás rejtelmeibe (Budapest: Scientia Publisher).
Podani, J. (2000). Introduction to the exploration of multivariate biological data (Leiden: Backhuys Publishers).
Podani, J. (2001). Computer Programs for Data Analysis in Ecology and Systematics. User’s Manual (Budapest: Scientia Publisher).
Ran, J. H., Shen, T. T., Wu, H., Gong, X., Wang, X. Q. (2018). Phylogeny and evolutionary history of Pinaceae updated by transcriptomic analysis. Mol. Phylogenet. Evol. 129, 106–116. doi: 10.1016/j.ympev.2018.08.011
Robertson, N. U., Schoonees, A., Brand, A., Visser, J. (2020). Pine bark (Pinus spp.) extract for treating chronic disorders. Cochrane Database Syst. Rev. 9, CD008294. doi: 10.1002/14651858.CD008294.pub5
Salehi, B., Upadhyay, S., Orhan, I. E., Jugran, A. K., Jayaweera, S. L. D., Dias, D. A., et al. (2019). Therapeutic Potential of alpha- and beta-Pinene: A Miracle Gift of Nature. Biomolecules 9. doi: 10.3390/biom9110738
Sarac, Z., Bojovic, S., Nikolic, B., Tesevic, V., Dordevic, I., Marin, P. D. (2013). Chemotaxonomic significance of the terpene composition in natural populations of Pinus nigra JF Arnold from Serbia. Chem. Biodivers. 10, 1507–1520. doi: 10.1002/cbdv.201200416
Sarria, S., Wong, B., Garcia Martin, H., Keasling, J. D., Peralta-Yahya, P. (2014). Microbial synthesis of pinene. ACS Synth. Biol. 3, 466–475. doi: 10.1021/sb4001382
Satou, T., Matsuura, M., Takahashi, M., Murakami, S., Hayashi, S., Sadamoto, K., et al. (2011). Components of essential oils extracted from leaves and shoots of Abies species in Japan. Chem. Biodivers. 8, 1132–1138. doi: 10.1002/cbdv.201000254
Stull, G. W., Qu, X. J., Parins-Fukuchi, C., Yang, Y. Y., Yang, J. B., Yang, Z. Y., et al. (2021). Gene duplications and phylogenomic conflict underlie major pulses of phenotypic evolution in gymnosperms. Nat. Plants 7, 1015–101+. doi: 10.1038/s41477-021-00964-4
Sundar, S. K., Parikh, J. K. (2023). Advances and trends in encapsulation of essential oils. Int. J. Pharm. 635, 122668. doi: 10.1016/j.ijpharm.2023.122668
Surendran, S., Qassadi, F., Surendran, G., Lilley, D., Heinrich, M. (2021). Myrcene-what are the potential health benefits of this flavouring and aroma agent? Front. Nutr. 8. doi: 10.3389/fnut.2021.699666
Swor, K., Poudel, A., Rokaya, A., Satyal, P., Setzer, W. N. (2022). Foliar essential oil of white fir (Abies concolor (Gordon) Lindl. ex Hildebr.): Chemical composition and enantiomeric distribution. Natural Prod. Commun. 17. doi: 10.1177/1934578x221086901
Swor, K., Satyal, P., Poudel, A., Setzer, W. N. (2023). Gymnosperms of Idaho: Chemical Compositions and Enantiomeric Distributions of Essential Oils of Abies lasiocarpa, Picea engelmannii, Pinus contorta, Pseudotsuga menziesii, and Thuja plicata. Molecules 28. doi: 10.3390/molecules28062477
Szmigielski, R., Cieslak, M., Rudzinski, K. J., Maciejewska, B. (2011). Identification of volatiles from Pinus silvestris attractive for Monochamus galloprovincialis using a SPME-GC/MS platform. Environ. Sci. pollut. Res. Int. 19, 2860–2869. doi: 10.1007/s11356-012-0792-5
WFO (2024). World flora online. Available online at: http://www.worldfloraonline.org (Accessed 19 Apr 2024).
Whitehill, J. G. A., Yuen, M. M. S., Henderson, H., Madilao, L., Kshatriya, K., Bryan, J., et al. (2019). Functions of stone cells and oleoresin terpenes in the conifer defense syndrome. New Phytol. 221, 1503–1517. doi: 10.1111/nph.15477
Xie, S. Q., Huynh, T. H., Qin, P. Y., Tan, T. W., Lin, H. F. (2020). Facile biphasic catalytic process for conversion of monoterpenoids to tricyclic hydrocarbon biofuels. J. Energy Chem. 49, 42–50. doi: 10.1016/j.jechem.2020.01.032
Yang, Y., Ferguson, D. K., Liu, B., Mao, K. S., Gao, L. M., Zhang, S. Z., et al. (2022). Recent advances on phylogenomics of gymnosperms and a new classification. Plant Divers. 44, 340–350. doi: 10.1016/j.pld.2022.05.003
Yang, Z. Y., Ran, J. H., Wang, X. Q. (2012). Three genome-based phylogeny of Cupressaceae s.l.: further evidence for the evolution of gymnosperms and Southern Hemisphere biogeography. Mol. Phylogenet Evol. 64, 452–470. doi: 10.1016/j.ympev.2012.05.004
Zhang, C. Z., Hong, K. (2020). Production of terpenoids by synthetic biology approaches. Front. Bioeng. Biotechnol. 8. doi: 10.3389/fbioe.2020.00347
Zhang, L., Xiao, W. H., Wang, Y., Yao, M. D., Jian, G. Z., Zeng, B. X., et al. (2017). Chassis and key enzymes engineering for monoterpenes production. Biotechnol. Adv. 35, 1022–1031. doi: 10.1016/j.bioteChadv.2017.09.002
Zhao, Z. J., Sun, Y. L., Ruan, X. F. (2023). Bornyl acetate: A promising agent in phytomedicine for inflammation and immune modulation. Phytomedicine 114. doi: 10.1016/j.phymed.2023.154781
Keywords: conifers, gas chromatography, essential oils, chemotaxonomy, Pinaceae, Cupressaceae, terpenes, terpene synthases
Citation: Bakó E, Böszörményi A, Vargáné Szabó B, Engh MA, Hegyi P, Ványolós A and Csupor D (2024) Chemometric analysis of monoterpenes and sesquiterpenes of conifers. Front. Plant Sci. 15:1392539. doi: 10.3389/fpls.2024.1392539
Received: 27 February 2024; Accepted: 01 August 2024;
Published: 04 September 2024.
Edited by:
Mariam Gaid, Independent researcher, Braunschweig, GermanyReviewed by:
Lourin Malak, Assiut University, EgyptMichelle Nonato de Oliveira Melo, Federal University of Rio de Janeiro, Brazil
Copyright © 2024 Bakó, Böszörményi, Vargáné Szabó, Engh, Hegyi, Ványolós and Csupor. This is an open-access article distributed under the terms of the Creative Commons Attribution License (CC BY). The use, distribution or reproduction in other forums is permitted, provided the original author(s) and the copyright owner(s) are credited and that the original publication in this journal is cited, in accordance with accepted academic practice. No use, distribution or reproduction is permitted which does not comply with these terms.
*Correspondence: Attila Ványolós, dmFueW9sb3MuYXR0aWxhQHNlbW1lbHdlaXMuaHU=; Dezső Csupor, Y3N1cG9yLmRlenNvQHN6dGUuaHU=