- 1Tropical Crops Genetic Resources Institute, Chinese Academy of Tropical Agricultural Sciences, Haikou, China
- 2Key Laboratory of Biology and Cultivation of Herb Medicine (Haikou), Ministry of Agriculture and Rural Affairs, Haikou, China
- 3School of Agriculture, Forestry and Food Engineering, Yibin University, Yibin, China
- 4Guangxi Key Laboratory of High-Quality Formation and Utilization of Dao-di Herbs, Guangxi Botanical Garden of Medicinal Plants, Nanning, China
- 5Genetics Department, Faculty of Agriculture, Zagazig University, Zagazig, Egypt
Introduction: Blumea balsamifera L. (Ainaxiang) DC. is a perennial herb of the compositae family. It is also the primary source of natural borneol. Endo-borneol, the principal medical active element in B. balsamifera, is anti-inflammatory, antioxidant, and analgesic; enhances medicine absorption; refreshes; and is used as a spice and in cosmetic. Industrialization of B. balsamifera is limited by its low L-borneol concentration. Thus, understanding the accumulation pattern of the secondary metabolite endo-borneol and its synthesis process in secondary metabolism is critical for increasing B. balsamifera active ingredient content and cultivation efficiency.
Methods: In this work, B. balsamifera was treated with varying concentrations (1.00 and 10.00 mmol/L) of methyl jasmonate (MeJA) as an exogenous foliar activator. The physiological parameters and L-borneol concentration were then assessed. Transcriptome sequencing of B. balsamifera-induced leaves was used to identify key genes for monoterpene synthesis.
Results: The treatment effect of 1 mmol/L MeJA was the best, and the leaves of all three leaf positions accumulated the highest L-borneol after 120 h, correspondingly 3.043 mg·g−1 FW, 3.346 mg·g−1 FW, and 2.044 mg·g−1 FW, with significant differences from the control. The main assembly produced 509,285 transcripts with min and max lengths of 201 and 23,172, respectively. DEG analysis employing volcano blots revealed 593, 224, 612, 2,405, 1,353, and 921 upregulated genes and 4, 123, 573, 1,745, 766, and 763 downregulated genes in the treatments D1_1vsCK, D1_10vsCK, D2_1vsCK, D2_10vsCK, D5_1vsCK, and D5_10vsCK. Interestingly, when exposed to MeJA treatments, the MEP pathway’s unigenes express themselves more than those of the MVA route. Finally, when treated with 1 mmol/L, the genes DXR, DXS, and GPS showed increased expression over time. At the same time, a 10 mmol/L therapy resulted in elevated levels of ispH and GGPS.
Discussion: Our preliminary research indicates that exogenous phytohormones can raise the level of L borneol in B. balsamifera (L.) DC when given in the appropriate amounts. The most significant discovery made while analyzing the effects of different hormones and concentrations on B. balsamifera (L.) DC was the effect of 1 mmol/L MeJA treatment.
1 Introduction
Blumea balsamifera (L.) DC, a half-woody plant that can easily grow in tropical and sub-tropical zones of Asia (Pang et al., 2014), belongs to the family Asteraceae, and the herbal medicine plant has been widely used for thousands of years in Southeast Asian countries, such as China, Philippines, Vietnam, and Thailand (Phumthum et al., 2018; Sadia et al., 2018; Lee et al., 2019). The most significant member of the Blumea genus, sambang, is an endemic plant to tropical and subtropical Asia, particularly China (Pang et al., 2014). This plant grows on the borders of woods, in river bottoms, valleys, and among grasslands. It is often a widely used plant in China, particularly in the provinces of Hainan, Guizhou, Yunnan, and Guangdong, and in Taiwan (Pang et al., 2010; Guan et al., 2012; Pang et al., 2014) and the Li-nationality People take baths in boiled leaves to improve various women’s problems, especially postpartum (Shirota et al., 2011). People frequently refer to B. balsamifera as “Ainaxiang” and “Dafeng’ai” in Chinese because of its high concentration of essential oils, and they burn it as incense (Pang et al., 2014). It was first written down in “Bei Ji Qian Jin Yao Fang” by Sun Simiao in the year 652 (Pang et al., 2014). Primitive Chinese traditional medicine uses the whole plant or its leaves to cure eczema, dermatitis, beriberi, lumbago, menorrhagia, rheumatism, skin injuries, and insecticides (Chen et al., 2010). Plants are the source of traditional Chinese medicines (TCMs), such as Bing Pian and Aipian, which are identical in potency and primarily contain borneol (Pang et al., 2014). In today’s Chinese pharmaceutical market, they are interchangeable. Before 2010, Sambong was one of Bing Pian’s most important plant sources. But since 2010, the Pharmacopoeia of the People’s Republic of China lists B. balsamifera as the only plant source for Aipian (Pang et al., 2014), with B. balsamifera medicinal materials that could bring back to life, clear heat, and ease pain. Recent studies (Nessa et al., 2004; Hasegawa et al., 2006; Kubota et al., 2009; Pang et al., 2014) have confirmed the anticancer, antifungal, radical scavenging, and anti-obesity effects of extracts of its leaves. In Chinese medicine, the decoction of leaves taken as an expectorant is common and can also be found in Malaysia, India, and Mizoram (Pang et al., 2014). In Vietnam, the vapor from boiling sambong leaves can be used as an inhalation for the treatment of cough and influenza (Ahmad, 2021). Recently, research has shown that B. balsamifera DC has new applications as a diuretic or in the dissolution of renal stones (Widhiantara and Jawi, 2021). A phytochemical investigation of B. balsamifera DC revealed that it contained volatile oil, and flavonoids (Pang et al., 2014; Masyudi et al., 2022). A total of 28 compounds were identified from the main essential oils, and the main forms are L-borneol and L-camphor (Masyudi et al., 2022). Borneol is a terpene derivative and exists as two enantiomers for chiral. Both D-borneol and L-borneol are found in nature. L-Borneol is the base of the volatile oil from B. balsamifera (L.) DC (Wang et al., 2014). Pharmacological studies show L-borneol has activities of inhibiting bacteria, antioxidants, anti-inflammatory, and analgesia, and also contributes to pharmaceutical absorption and deodorizing competitiveness (Moghtader et al., 2011; Ma et al., 2023). L-Borneol is a secondary metabolite of B. balsamifera (L.) DC and its active pharmaceutical ingredients (Bhuiyan et al., 2009; Widhiantara and Jawi, 2021).
B. balsamifera DC has grown abundantly for medicine and cosmetics recently (Pang et al., 2014; Jirakitticharoen et al., 2022). The metabolite accumulation pattern of B. balsamifera DC could be affected by cultivating conditions and the external environment (Wang et al., 2023). Research revealed that the content of L-borneol was different in different tissues at different developmental stages (Pang et al., 2017; Guan et al., 2022). Mei et al. (2023) observed that tender leaves have the highest L-borneol compared with other tissues of B. balsamifera. Much research focuses on accumulating more secondary metabolites in medicinal plants (Al-Gabbiesh et al., 2015; Pant et al., 2021). Jasmonic acid (JA) and its derivative are widely distributed in plants as signal molecules and can also be inducers to regulate the biosynthesis of metabolites (Turner et al., 2002; Ruan et al., 2019). The preliminary study of our group demonstrated that the treatment of exogenous phytohormones with a suitable concentration range can improve the content of L-borneol in B. balsamifera (L.) DC, with the effect of 1 mmol/L methyl jasmonate (MeJA) treatment on B. balsamifera (L.) DC being the most outstanding when compared to other hormones and other concentrations. To understand the accumulation mechanisms of L-borneolin B. balsamifera (L.) DC with the MeJA treatment, we screen the key regulation gene, identify its function, and detect the transcriptome and metabolome.
2 Materials and methods
2.1 Experimental materials and exogenous hormone treatments
Individual B. balsamifera plants were selected for vegetative propagation at the germplasm repository of the tropical medicinal plant greenhouse (19°30′N, 109°20′E; temperature, 28°C; humidity, 60%; light application time, 9 h) of Ministry of Agriculture in Danzhou city, Hainan province, which is linked with the Tropical Crops Genetic Resources Institute, Chinese Academy of Tropical Agricultural Sciences. The nursery garden has red clay soil, partly sandy. Every plant was grown for 3 months. The identical plants were separated into five groups based on leaf count and phenotype, each with 20 plants. Each MeJA solution was produced in 1 L of ddH2O, which provided sufficient volume for foliar applications. Each plant was continuously sprayed with 20 mL of a surface-treating chemical. In addition to the control (distal water), each foliar treatment received equal exposure to MeJA doses of 1 mmol/L and 10 mmol/L. A volume of 20 ml of surface-treating chemicals were added to the spray bottle and equably sprayed at 20 cm from the plants. Each treatment was given three times in total. After the treatment, mature leaves were gathered at 24 h, 48 h, and 120 h. The samples were immediately stored in liquid nitrogen and kept at −80°C, until they were used for qPCR, RNA sequencing (RNA-seq), and metabolite analysis.
2.2 Determination of L-borneol
The collected B. balsamifera leaves were immediately placed in a mortar and ground with liquid nitrogen, and ground into a powder. We accurately weighed 2 g of the ground powder into a 50-mL centrifuge tube and added 25 mL of ethyl acetate, operating at 40 kHz and 400 W. We performed an ultrasonic extraction for 30 min, left it standing, and filtered it. Add 1 mL of the filtrate to a 10-mL volumetric flask, along with 1 mL of methyl salicylate internal standard solution. Next, fix the volume with ethyl acetate and filter through a 0.22-μm microporous filter membrane. The content of L-borneol was determined by GC-MS, HP-5 quartz capillary column (0.32 mm × 30 m, 0.25 μm), starting at 80°C for 2 min, then heating at 5°C/min to 100°C, 20°C/min to 200°C. The temperatures of the inlet and FD detector were 220°C and 240°C, respectively. The injection volume is 0.6 μL, without shunt.
2.3 RNA isolation, library construction, and transcriptome sequencing
TRIzol reagent (Invitrogen) was used to extract total RNA, and the manufacturer’s instructions were followed (El-Sappah et al., 2023). Using the NanoPhotometer1 spectrophotometer (IMPLEN, CA, USA) and the Qubit1 RNA Assay Kit in Qubit1 2.0 Flurometer (Life Technologies, CA, USA), total RNA purity and concentration were assessed. RNA integrity was evaluated using the Agilent Bioanalyzer 2100 system (Agilent Technologies, CA, USA) and the RNA Nano 6000 Assay Kit. The RNA sample preparations employed a total of 1.5 g of RNA as input material for each sample. Utilizing the NEBNext1 UltraTM RNA Library Prep Kit for Illumina1 (NEB, USA) in accordance with the manufacturer’s instructions, sequencing libraries were created, and index codes were applied to assign sequences to specific samples. The TruSeq PE Cluster Kit v3-cBot-HS (Illumina) was used on the acBot Cluster Generation System to cluster the index coded sample data in accordance with the manufacturer’s recommendations. Using an Illumina Hiseq 4000 platform, the library preparations were sequenced after cluster creation, and paired-end reads were produced. Fastq format raw readings were processed using internal perl scripts as part of the quality control stage. By eliminating adapter- and ploy-N-containing reads and low-quality reads from the raw data in this stage, clean reads were produced. At the same time, it was determined what the clean data’s Q20, Q30, GC content, and sequence duplication level were. On clean, high-quality data, all subsequent analyses were performed. During the transcriptome assembly process, all libraries’ and samples’ left files (read1 files) were merged into a single big left.fq file, and all libraries’ and samples’ right files (read2 files) into a single large right.fq file. Using Trinity (Grabherr et al., 2011), transcriptome assembly was completed with min_kmer_cov and all other parameters set to their default settings. Right.fq and left.fq were used as the foundation for this. Gene function was conducted as described before. The alignments of unigenes to the Nt database were carried out using NCBI blast 2.2.28C with an E-value threshold of 1E−5. The program diamond (v0.8.22) was used for the comparison with the Nr (E-value, 1E−5), KOG/COG (E-value, 1E−3), and SwissProt (E-value, 1E−5) databases. Using an E-value limit of 0.01 and hmmscan from HMMER 3.0, Pfam was searched. With an E-value threshold of 1E−6 based on the Nr and Pfam annotations, the GO (Gene Ontology) annotations were carried out in Blast2GO (v2.5) (Wan et al., 2024). Pathway analysis was carried out to ascertain the crucial pathways of DEGs using the databases of the Kyoto Encyclopedia of Gene and Genomes (KEGG) (http://www.genome.jp/kegg). The statistical enrichment of differentially expressed genes in KEGG pathways was evaluated using the KOBAS program. Genes that exhibit substantial levels of differential expression are found in the pathways with an FDR value of 0.05 (Wan et al., 2023).
2.4 Quantitative real-time PCR validation
Five DEGs were chosen for quantitative real-time PCR (qRT-PCR) to validate the transcriptome results. All cDNAs were generated using the Prime Script RT reagent Kit with gDNA Eraser (TaKaRa, Kyoto, Japan), and qRT-PCR experiments were performed using the ABI 7500 Fast Real-Time Detection System and the TaKaRa SYBR Green Mix kit (TaKaRa, Kyoto, Japan). According to Li et al. (2021), the amplification system and process were used. The reference genes were Primer Premier 5.0-designed primers and 18S rRNA. Supplementary Table S1 lists all of the primers utilized. The 2CT approach was used to calculate the relative expression level.
2.5 Statistical analysis
The data were examined using Microsoft Excel 2007. The Statistical Analysis System v. 9.2 was used for significance testing (Duncan’s test) and principal component analysis (PCA). Heatmaps and Venn diagrams were drawn using Tbtools, while other figures were drawn using Origin 2022.
3 Result
3.1 L-Borneol concentration under different MeJA treatments
Different concentrations of MeJA affect the accumulation of L-borneol. Overall, different concentrations of MeJA can both promote L-borneol accumulation in the plant’s leaves. Except for the 10 mmol/L MeJA of 120-h treatment, moxa under other concentration treatments, there is accumulation of L-borneol in the leaves of the three leaf positions of Ainaxiang over time, especially when the concentration was 1 mmol/L on 48-h treatment. The treatment effect of 1 mmol/L MeJA was the most effective, resulting in the highest accumulation of L-borneol at 120 h in leaves at different stages of maturity (young, mature, and old leaves), with concentrations of 3.043 mg·g−1 FW, 3.346 mg·g−1 FW, and 2.044 mg·g−1 FW, respectively. These values showed significant differences compared to the control. Figure 1 clearly demonstrates the positive impact of 1 mmol/L MeJA treatment on the accumulation of L-borneol in the leaves of Ainaxiang.
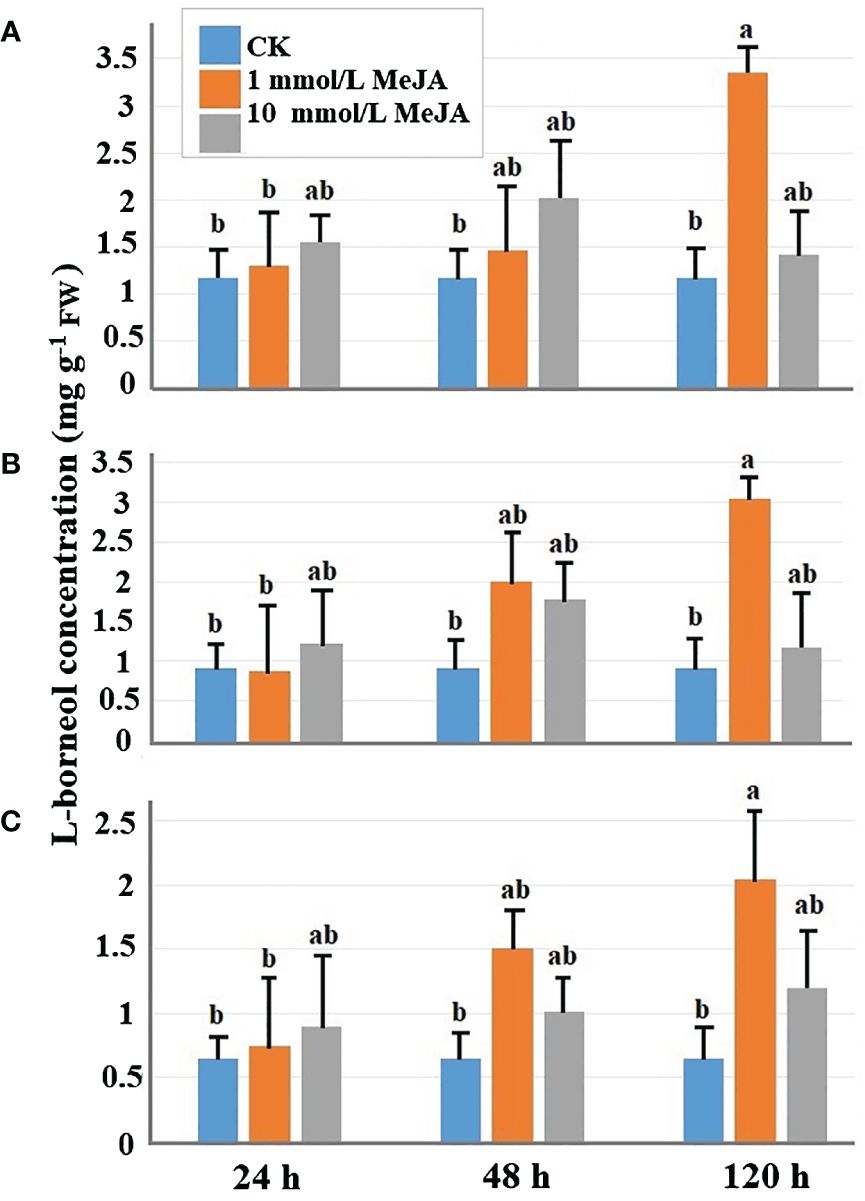
Figure 1 The L-borneol concentration of B. balsamifera mature leaves at three different times (24 h, 48 h, and 120 h) under different MeJA treatments: (A) the young leaves, (B) mature leaves, and (C) old leaves. The P <0.05 was denoted by the lowercase letters.
3.2 Sequencing and differentially expressed genes analysis of transcriptomes
To explore MeJA’s regulation mechanism on terpenoid synthesis in the leaves of Ainaxiang, non-induced and induced conditions were selected. To obtain the transcriptome, Illumina high-throughput next-generation sequencing was performed on the mature leaves of Ainaxiang under different conditions. The clean data screening requirements are tightly regulated in order to assure the caliber of the subsequent analysis. The reads with adapters should be eliminated first, and then, the reads that make up more than 10% but whose base information cannot be identified. The gathered clean reads for the non-reference genome were spliced using Trinity before the sequence was examined. The primary assembly yielded a total of 509,285 transcripts with minimum and maximum lengths of 201 and 23,172, respectively, and most of them (280,106) are between 200 bp and 500 bp, followed by those in the 501–1,000 bp range (82,683 transcripts) (Supplementary Table S2). In addition, 68,679 transcripts were observed in the range of 1,001–2,000 bp, and 77,817 transcripts were in the range of more than 2,000 bp, with average transcript length of 989 bp and N50 of 2,088 bp.
3.3 Gene function annotation and classification
We aligned the 267,178 unigenes to the Nr (NCBI non-redundant protein sequence), Nt (NCBI non-redundant nucleotide sequence), Pfam (protein family), KOG (protein ortholog source clusters), Swiss-Prot (manually annotated and reviewed protein sequence database), and KO (KEGG Ortholog database), achieving a match of 23.47% × 65.36, for example. In Figure 2, comparing DEG using a volcano blot showed that 593, 224, 612, 2,405, 1,353, and 921 genes were upregulated, and 4, 123, 573, 1,745, 766, and 763 genes were downregulated in the D1_1vsCK, D1_10vsCK, D2_1vsCK, D2_10vsCK, D5_1vsCK, and D5_10vsCK treatments, in that order. We used GO enrichment analysis to anticipate the activities of the DEGs. We found that the DEGs enriched for three primary ontologies: biological process, cellular component, and molecular function. We assigned the 666,068 unigenes to three major GO terms: cellular component (198,296 unigenes across all samples), molecular function (148,057 unigenes), and biological process (319,733 unigenes). Finally, we annotated the 62,720 DEGs of all samples to 25 GO terms, including posttranslational modification, protein turnover, chaperones category, which contained 6,248 unigenes, and translation, which had 2383 unigenes related (Figure 3; Supplementary Table S3).
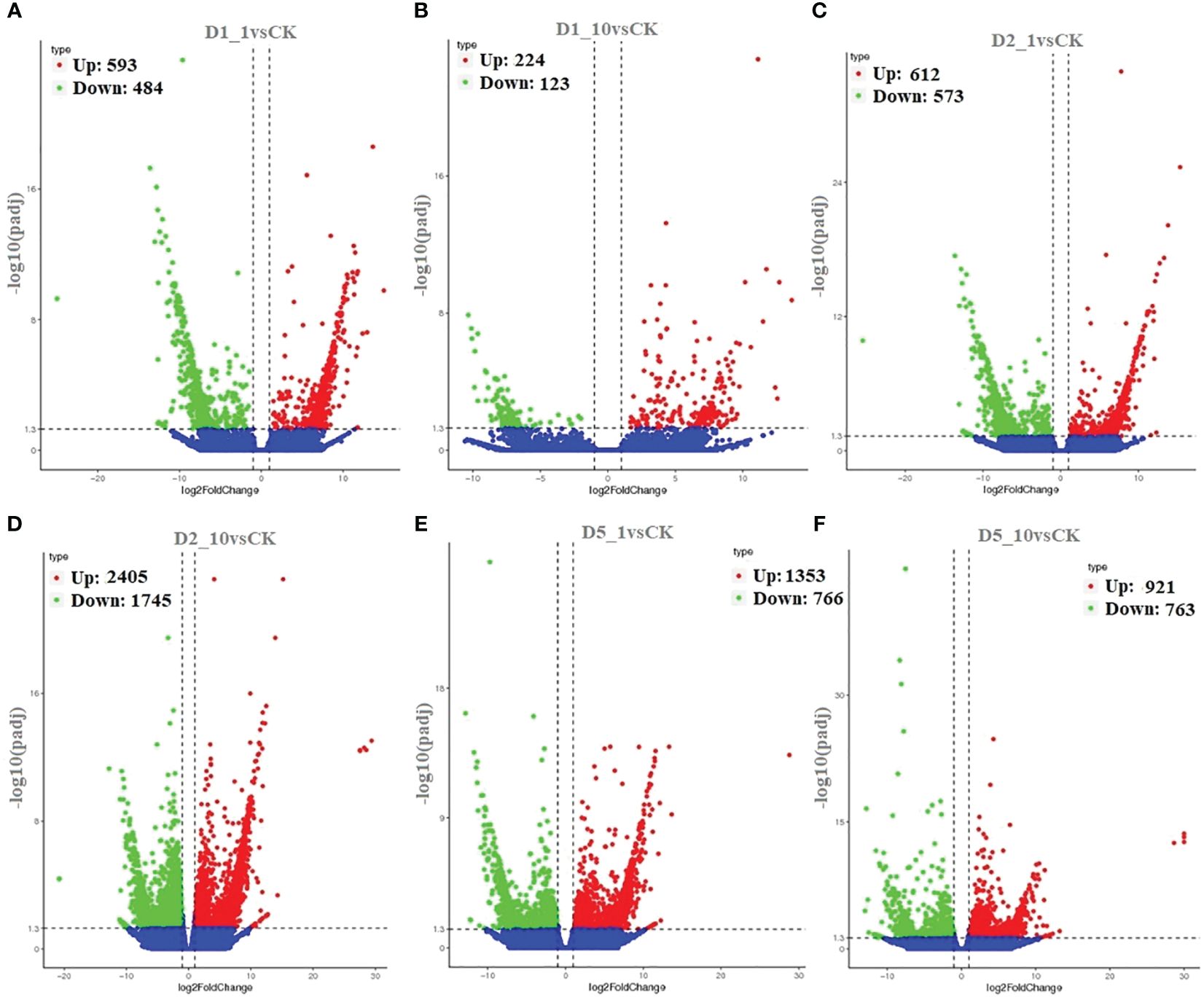
Figure 2 Identification of differentially expressed genes (DEG). Volcano plot showing the differentially expressed genes of (A) D1_1vsCK, (B) D1_10vsCK, (C) D2_1vsCK, (D) D2_10vsCK, (E) D5_1vsCK, and (F) D5_10vsCK.
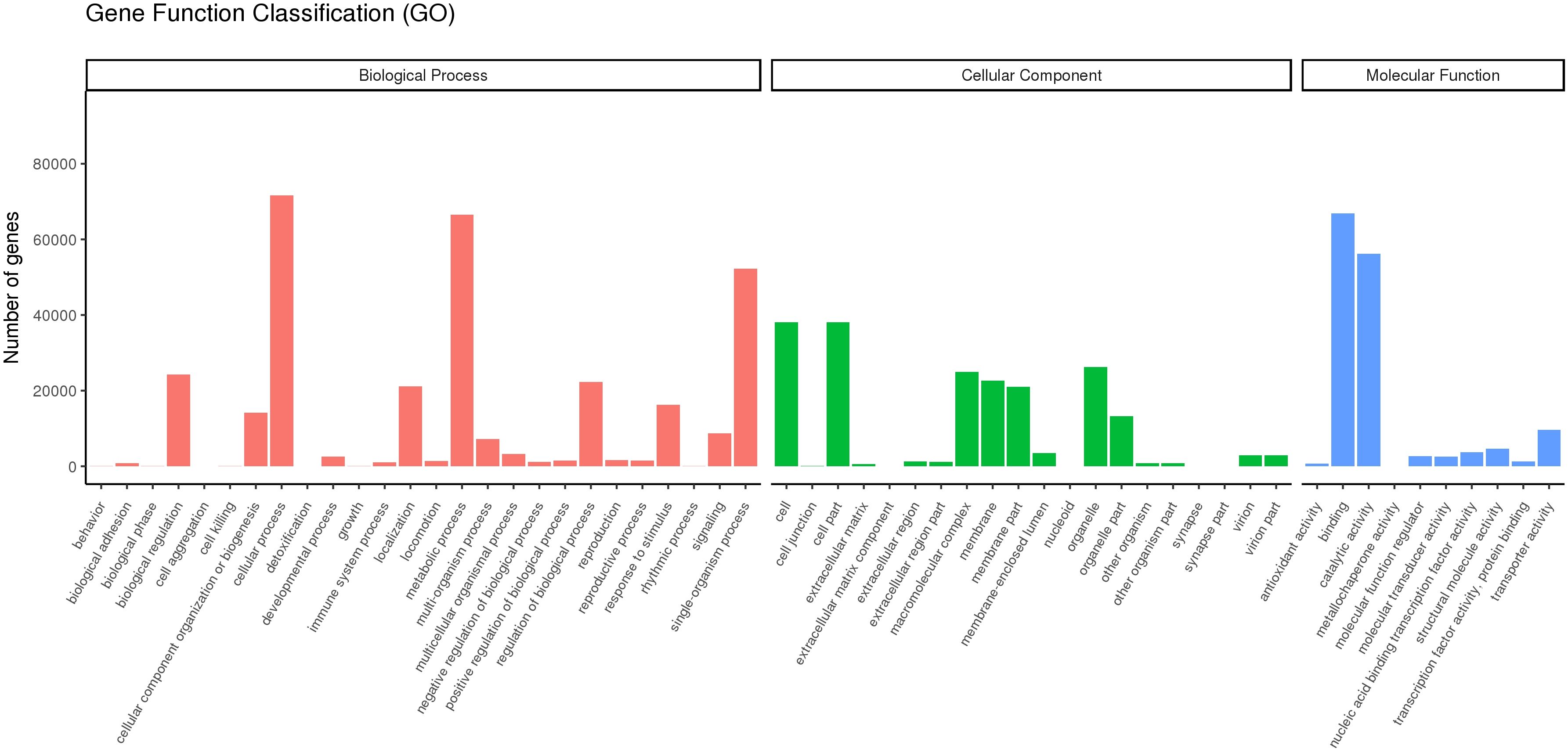
Figure 3 Unigene classification based on gene ontology (GO). These unigenes were classified into three groups based on functional annotation: biological process, cellular component, and molecular function.
In addition, the KOG database showed that 50,632 unigenes had strong functional prediction and categorization matches (Figure 4; Supplementary Table S4). The 25 KOG categories that were looked at were translation, ribosome structure and biogenesis (5963), general function prediction alone (5544), and signal transduction pathways (3005). The largest groups were posttranslational modification, protein turnover, and chaperones (6248). The smallest categories were extracellular structures (46) and cell motility (40). We also examined the unigenes’ KEGG pathways. We found that 25 projected metabolic pathways (Figure 5; Supplementary Table S5), grouped into five categories: metabolism, genetic information processing, environmental information processing, cellular activities, and organismal systems, involved 64803 unigenes. The most numerous category in its gene content was metabolism, including carbohydrate metabolism (6244), followed by overview (5105), energy metabolism (4814), amino acid metabolism (3764), and lipid metabolism (3195). As demonstrated in Figure 5 and Supplementary Table S5, the smallest categories were organismal systems (1772) and environmental information processing (1861).
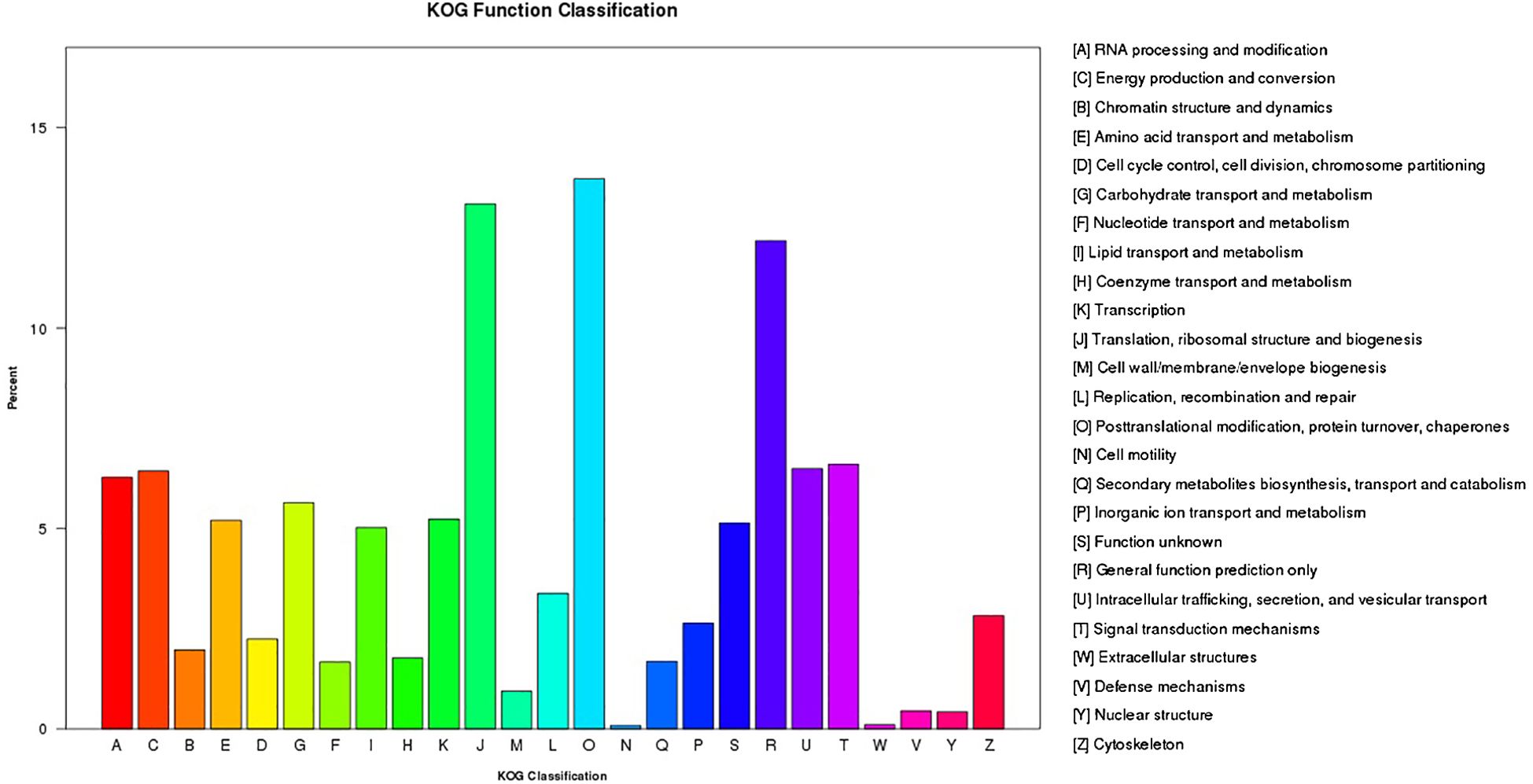
Figure 4 Unigene classification by eukaryotic orthologous groups (KOG). According to the KOG database, functional predictions of unigenes were grouped into at least 25 function classes.
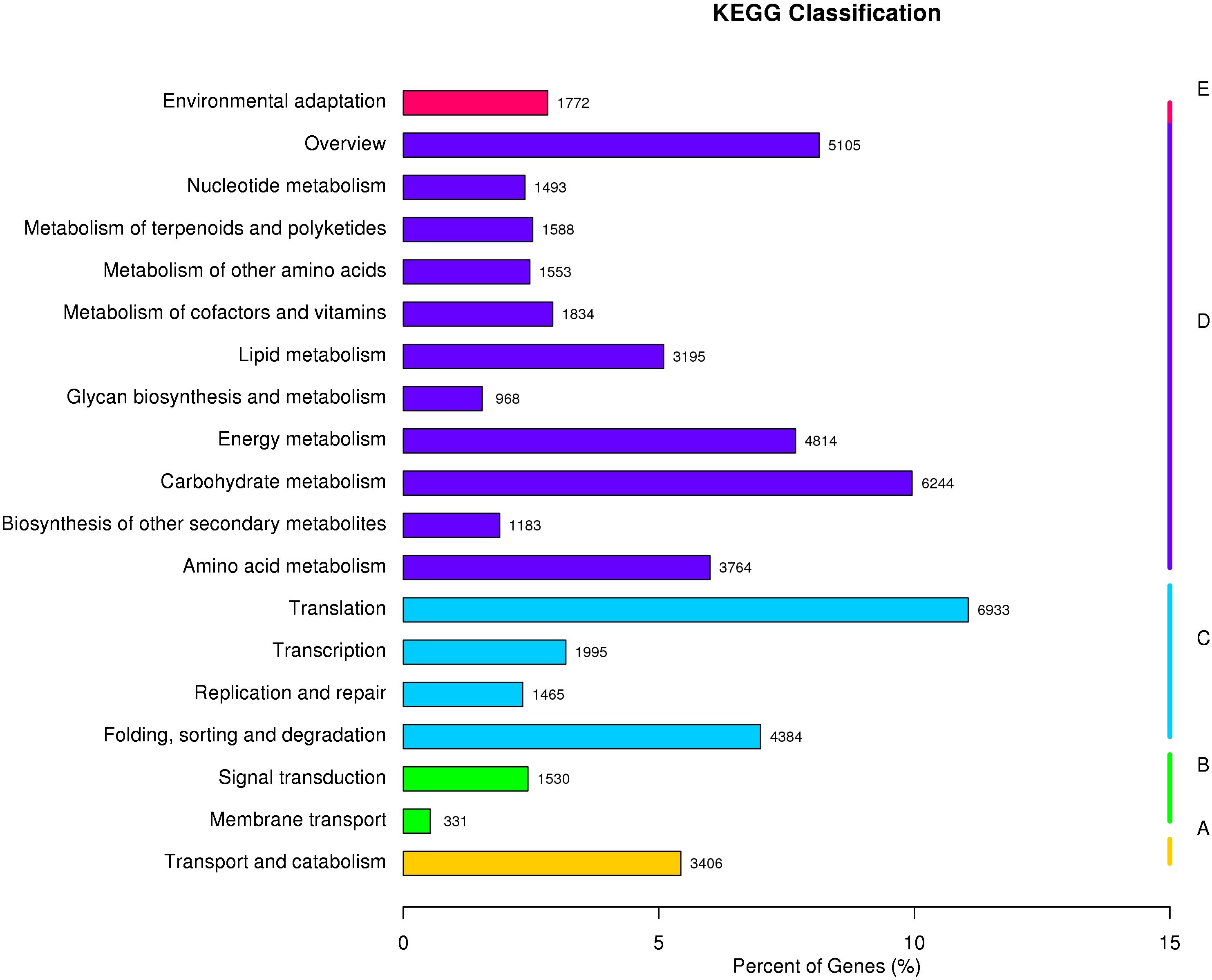
Figure 5 Unigene metabolic pathway classification according to the Kyoto Encyclopedia of Genes and Genomes (KEGG). The data were divided into five categories: (A) cellular processes, (B) environmental information processing, (C) genetic information processing, (D) metabolism, and (E) organismal systems. The number of B. balsamifera unigene matches in each category is represented by the bars.
3.4 Analysis of DEGs involved in the (−)-borneol biosynthesis pathway
The expression profiles of genes involved in borneol production were studied to investigate the regulatory mechanisms for the accumulation patterns of diverse borneol in B. balsamifera under different MeJA treatments. B. balsamifera was found to include 4,848 expressed unigenes that encode terpenoid biosynthesis enzymes. At 24 h after 1 mmol/L MeJA treatment, there are 1,077 DEGs, 1,185 DEGs at 48 h, and 2,119 DEGs at 120 h. At each sample point, there were 593, 484, and 612 upregulated genes. They also had 347, 4,150, and 1,684 DEGs at each point under 10 mmol/L MeJA treatment; upregulation genes were 224, 123, and 2,405 at three sample points, respectively. Figure 6 displays the FPKM values and expression data for each unigene. Most genes that code for important enzymes in the borneol backbone pathway (MEP and MVA pathways, KEGG entry ko00900) did have a high transcriptome expression level. However, the deoxy-d-xylulose 5-phosphate synthase (DXS), ispH, geranylgeranyl diphosphate synthase (GGPS), geranyl diphosphate synthase (GPS), and 1-deoxy-d-xylulose-5-phosphate reductoisomerase (DXR) unigenes did not. Under the low concentration of MeJA treatment, DXS (Cluster-28937.97079) exhibits high expression on all treatment days. At the same time, every ispH (Cluster-28937.127564), GGPS (Cluster-28937.10564), and GPS (Cluster-28937.47046) showed upregulation only after 120 h treatment. On the other hand, under 10 mmol/L MeJA treatment, the expression of GGPS (Cluster-28937.10564) and GPS (Cluster-28937.47046) showed upregulation in all treatment days, and DXR (Cluster-28937.158907) showed upregulation at the first 48 h, while DXS (Cluster-28937.97079) exhibited a high expression level after 120 h of treatment. Interestingly, unigenes in the MEP route have greater expression levels than those in the MVA pathway.
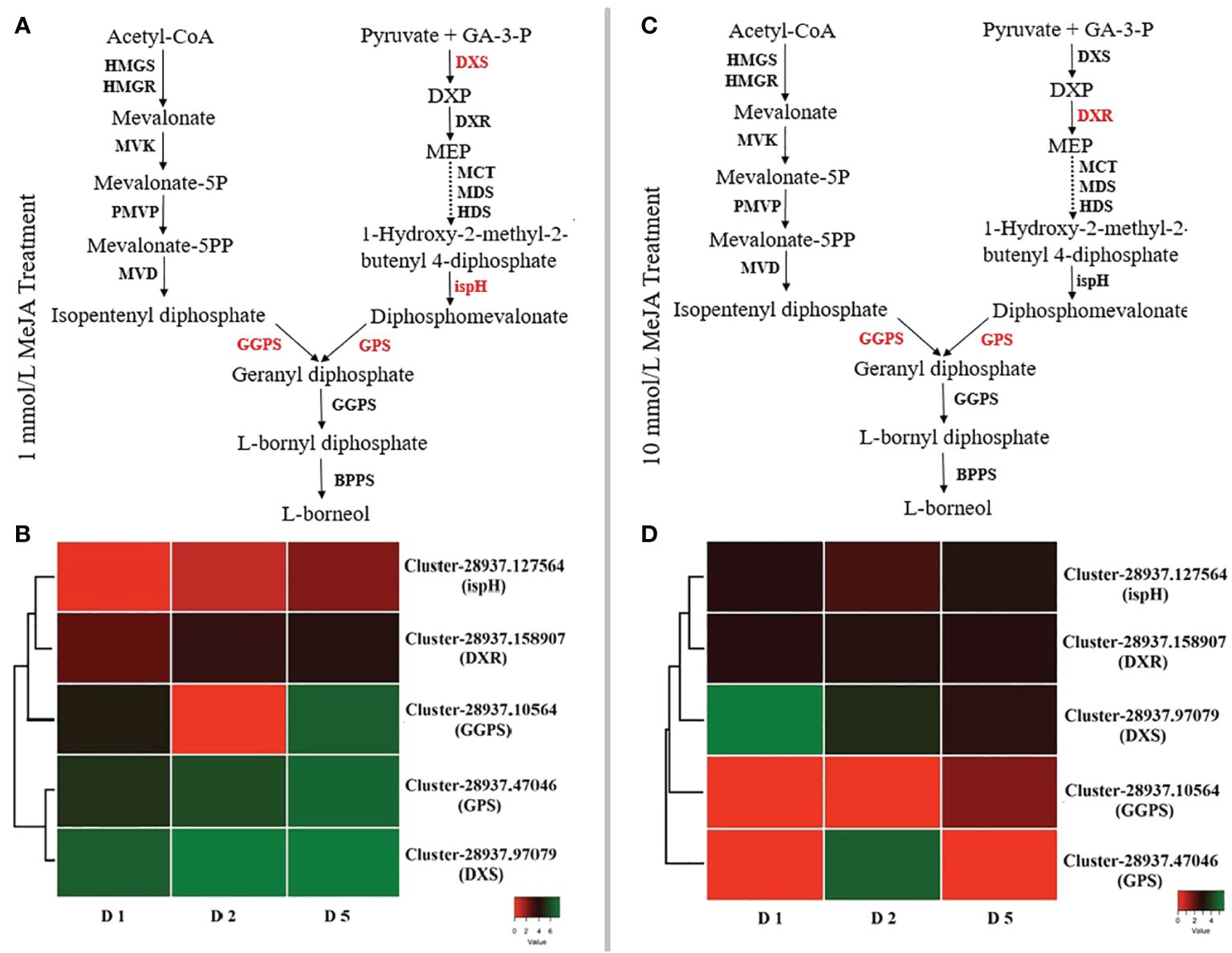
Figure 6 DEGs responsible for the (–)-borneol biosynthesis pathway in B. balsamifera: (A) the borneol biosynthesis pathway at 1 mmol/L MeJA; (B) a heat map of genes involved in borneol biosynthesis at 1 mmol/L MeJA; (C) the borneol biosynthesis pathway at 10 mmol/L MeJA; and (D) a heat map of genes involved in borneol biosynthesis at 10 mmol/L MeJA. D1 (Day 1) represents 24 hours, D2 48 hours, and D5 120 hours. Every pathway’s red-colored genes indicate an upregulated expression level.
3.5 Gene expression of the L-borneol biosynthesis pathway related genes
The expression analysis related to borneol biosynthesis genes was conducted at both 1 and 10 mmol/L MeJA treatments, as shown in Figure 7. The gene expression results are consistent with the transcriptomic analysis results. Genes DXR, DXS, and GPS showed high expression over time at 1 mmol/L treatment. At the same time, ispH and GGPS showed high expression with a 10 mmol/L treatment.
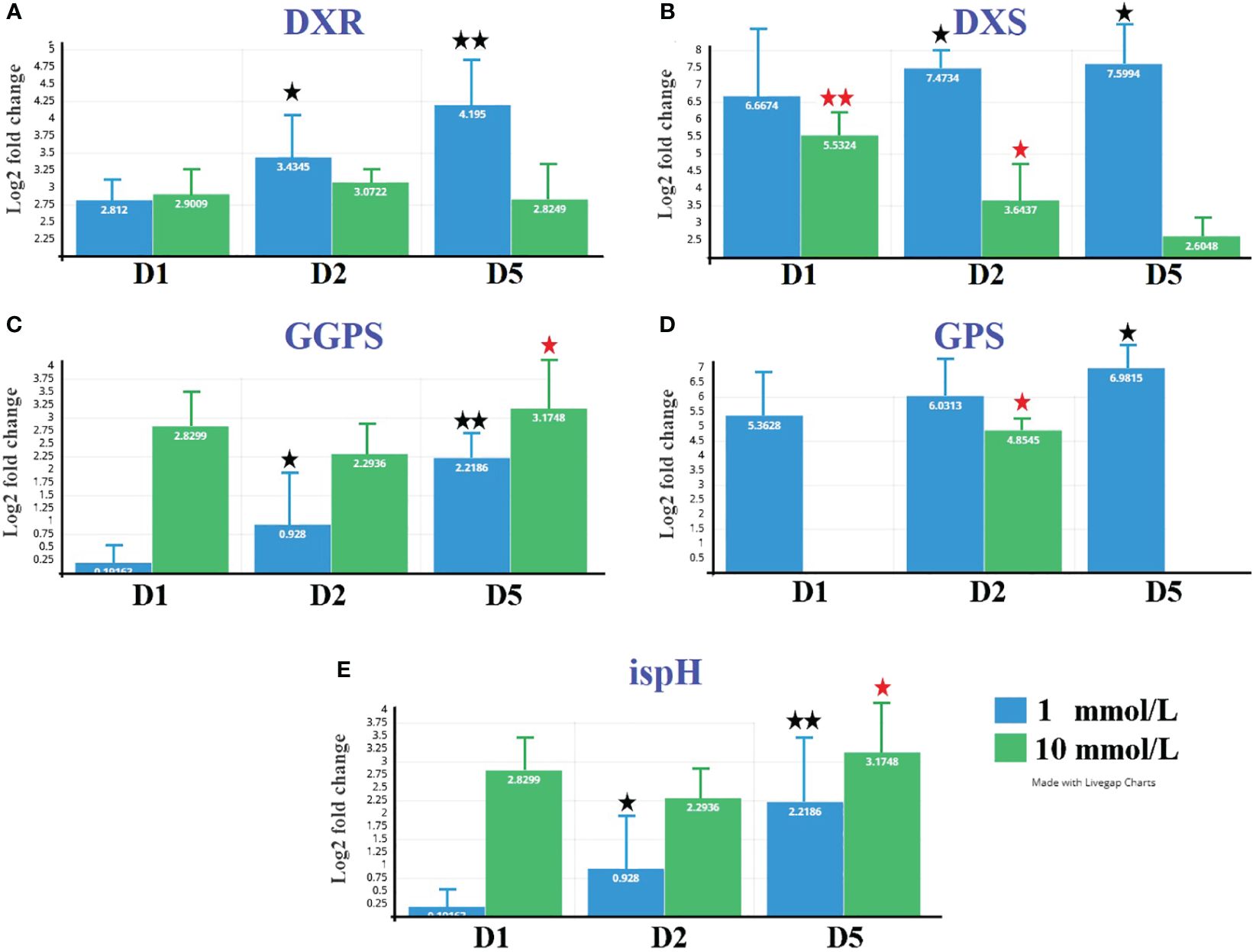
Figure 7 Expression profile of five selected unigenes related to (−)-borneol biosynthesis pathway in B. balsamifera under both MeJA concentrations. Unigene expression was analyzed by qRT-PCR using Actin gene as an internal reference. Data represent means ± standard deviation (SD) from three biological replicates (three technical replicates per biological replicate). Repetition of the experiment produced similar results. * Different than pre (P < 0.05).
4 Discussion
L-Borneol, which has a high degree of volatility, is the major active substance. Furthermore, researchers discovered a variety of biological actions in essential oils, flavonoids, and terpenoids (Chen et al., 2009). These investigations may help to understand the many pharmacological actions of this plant.
The MeJA, is an important cellular regulator that controls how plants grow and defend themselves in response to both living and non-living factors (Cheong and Do Choi, 2003; Wolucka et al., 2005). MeJA, when used before harvest, is considered safe for all food products (García-Pastor et al., 2020; Dhiman et al., 2022). The major technique for raising the number of secondary metabolites in vegetables is phytohormone induction, which results in a reaction to stress in plants (Sharma et al., 2022). The most extensively researched and applied excitons for promoting the synthesis of secondary metabolites in agricultural products to improve their nutritional quality are JA and MeJA (Wang et al., 2022). Exogenous MeJA has the ability to modify the concentrations of several primary metabolites, such as plant sugars, organic acids, and amino acids (Kim et al., 2017; Meza et al., 2022). Brassica oleracea L. var. italica (Plenck) treatment with MeJA promotes the production of bioactive chemicals (Ilahy et al., 2020; Bao et al., 2022). Treatment with MeJA also encourages the synthesis of volatile molecules, the buildup of non-volatile secondary metabolites, and secondary metabolites with a longer half-life (Bourgougnon and Stiger-Pouvreau, 2011; Singh et al., 2016). The production of aromatic compounds and the buildup of β-carotene are both stimulated by exogenous JA (Fan et al., 1998; Wang et al., 2022). MeJA, according to Liu et al. (2012) enhanced the expression of genes essential for carotenoid production, which in turn increased the accumulation of lycopene in tomato (Lycopersicon esculentum) tissue. According to Shafiq et al. (2013), MeJA enhanced the formation of phenolic components in the pericarp of apples (Malus pumila Mill.), such as chlorogenic acid, anthocyanin 3-galactoside, and flavonols. Scientific studies have demonstrated a decreased incidence of chronic and degenerative illnesses, including cancer, with a diet high in naturally occurring polyphenols produced by plants (Pandey and Rizvi, 2009; Zhang and Tsao, 2016). This is mostly due to the fact that phenolic compounds rich in phenolic acids, anthocyanins, and flavonoids usually exhibit potent free radical scavenging properties (Weng and Yen, 2012). When exposed to MeJA, the bioactive components of blackberry (Rubus fruticosus Pollich) and pomegranate (Punica granatum L.) show increased antioxidant and other positive effects (Wang et al., 2008; Sayyari et al., 2011). In the present study, the plants of B. balsamifera grown for 3 months were used as materials, different concentrations (1.00 mmol/L and 10.00 mmol/L) of MeJA were exogenous activators, their physiological indexes and L-borneol were determined, and the optimal concentration and time were screened in B. balsamifera induction. To identify the crucial genes in the manufacture of monoterpene, the transcriptome of the induced leaves of B. balsamifera was sequenced. The main source of (−)-borneol is the initial geranyl diphosphate (GPP), which is made from the two common C5 building blocks isopentenyl diphosphate and dimethylallyl diphosphate using either the cytoplasmic mevalonate (MVA) or the plastidic 2-C-methyl-D-erythritol-4-phosphate (MEP) pathways (Lichtenthaler, 1999; Rehman et al., 2016; Tian et al., 2022). Numerous (+)-bornyl diphosphate synthases have been identified in plants, including Cinnamomum burmanni, Salvia officinalis, Amomum villosum, Lippia dulcis, and Lavandula angustifolia, but it is still unclear which monoterpene synthase is responsible for cycling GPP to form (−)-bornyl diphosphate (Chen et al., 2023). The analysis of the gene expression profiles for those involved in the production of borneol revealed that 1 mmol/L MeJA therapy had a positive impact on the accumulation of L-borneol.
B. balsamifera leaves exhibited a total of 4,848 expressed unigenes that encode terpenoid biosynthesis enzymes. With the exception of the deoxy-d-xylulose 5-phosphate synthase (DXS), ispH, GGPS, GPS, and DXR unigenes, the majority of the genes encoding important enzymes in the borneol backbone pathway (MEP and MVA pathways, KEGG entry ko00900) had a high transcriptome expression level. Furthermore, under the low concentration of MeJA treatment, the expression of DXS (Cluster-28937.97079) exhibits high expression at all treatment days, while every expression of ispH (Cluster-28937.127564), GGPS (Cluster-28937.10564) and GPS (Cluster-28937.47046) showed upregulation only after 120 h treatment. Previous studies revealed that the 1-deoxy-D-xylulose-5-phosphate synthase (DXS) and two monoterpene synthases, known as CbDXS9, CbTPS2, and CbTPS3, were upregulated in the high-borneol group compared to the low-borneol and borneol-free groups and may be crucial for the biosynthesis of D-borneol in C. burmannii (Yang et al., 2020). Arabidopsis IspH gene expression in both photosynthetic and nonphotosynthetic tissues lends credence to the idea that the non-MVA pathway is involved in the synthesis of a range of isoprenoids in plants (Hsieh and Goodman, 2005). The enzyme known as geranylgeranyl diphosphate synthase (GGPPS) is essential for diterpene biosynthesis because it catalyzes the synthesis of GGPP, a frequent precursor to diterpenes (Zhang et al., 2015). Additionally, GPPS catalyzes the condensation of isopentenyl diphosphate and dimethylallyl diphosphate to yield geranyl diphosphate (Gilg et al., 2005). Several earlier studies found a strong link between the DXR gene’s activity and the amount of essential oil in C. camphora (Hou et al., 2020). This suggests that the upregulated DXR gene expression in C. camphora could lead to an increase in terpenoid content (Du et al., 2022). Wang et al. (2023) indicated that the OfDXR gene is very important for making terpenoid compounds and that overexpressing it in Arabidopsis plants during the main or full blooming stage led to a rise in the total amount of terpenoid compounds. Zhang et al. (2022) found that DXS, DXR, and ispH in the MEP pathway and GGPS were significantly expressed in summer Chinese cedar (SCC) needles and increased the accumulation of terpenoids, notably diterpenoids.
Data availability statement
The original contributions presented in the study are publicly available. This data can be found at the National Center for Biotechnology Information (NCBI) using accession number PRJNA668407, as well as in the article/Supplementary Material.
Ethics statement
This article does not contain any studies with human participants or animals performed by any of the authors.
Author contributions
LG: Conceptualization, Data curation, Formal analysis, Funding acquisition, Investigation, Methodology, Project administration, Resources, Visualization, Writing – original draft, Writing – review & editing. NL: Data curation, Formal analysis, Methodology, Resources, Writing – review & editing. LYW: Investigation, Software, Validation, Visualization, Writing – review & editing. FY: Formal analysis, Methodology, Resources, Visualization, Writing – review & editing. XC: Data curation, Formal analysis, Methodology, Writing – review & editing. XX: Methodology, Resources, Software, Writing – review & editing. CY: Formal analysis, Investigation, Methodology, Writing – review & editing. LHW: Data curation, Investigation, Writing – review & editing. SS: Data curation, Formal analysis, Writing – review & editing. MA: Data curation, Formal analysis, Writing – review & editing. RH: Data curation, Formal analysis, Writing – review & editing. AE: Conceptualization, Data curation, Formal analysis, Methodology, Validation, Writing – original draft, Writing – review & editing.
Funding
The author(s) declare financial support was received for the research, authorship, and/or publication of this article. This work was funded by Sichuan Provincial Regional Innovation Cooperation Special Project (2023YFQ0097) and National Natural Science Foundation of China (No. 31870317).
Conflict of interest
The authors declare that the research was conducted in the absence of any commercial or financial relationships that could be construed as a potential conflict of interest.
Publisher’s note
All claims expressed in this article are solely those of the authors and do not necessarily represent those of their affiliated organizations, or those of the publisher, the editors and the reviewers. Any product that may be evaluated in this article, or claim that may be made by its manufacturer, is not guaranteed or endorsed by the publisher.
Supplementary material
The Supplementary Material for this article can be found online at: https://www.frontiersin.org/articles/10.3389/fpls.2024.1391042/full#supplementary-material
References
Ahmad, H. (2021). Physicochemical, Analytical and Toxicity Studies on Kushta Khabasul Hadeed (Calx of Iron Rust) Prepared by Two Methods (India: Rajiv Gandhi University of Health Sciences).
Al-Gabbiesh, A., Kleinwächter, M., Selmar, D. (2015). Influencing the contents of secondary metabolites in spice and medicinal plants by deliberately applying drought stress during their cultivation. Jordan J. Biol. Sci. 147, 1–10. doi: 10.12816/0026941
Bao, J., Lu, X., Ma, L., Zhang, X., Tian, P., Zhang, X., et al. (2022). Transcriptome analysis of genes related to glucoraphanin and sulforaphane synthesis in methyl jasmonate treated broccoli (Brassica oleracea var. italica) hairy roots. J. Plant Res. 135, 757–770. doi: 10.1007/s10265-022-01407-7
Bhuiyan, M. N. I., Chowdhury, J. U., Begum, J. (2009). Chemical components in volatile oil from Blumea balsamifera (L.) DC. Bangladesh J. Bot. 38, 107–109. doi: 10.3329/bjb.v38i1.5132
Bourgougnon, N., Stiger-Pouvreau, V. (2011). Chemodiversity and bioactivity within red and brown macroalgae along the French coasts, metropole and overseas departements and territories. Handb. Mar. Macroalgae: Biotechnol. Appl. Phycol 58–105. doi: 10.1002/9781119977087.ch4
Chen, M., Jin, H. Z., Zhang, W. D., Yan, S. K., Shen, Y. H. (2009). Chemical constituents of plants from the genus Blumea. Chem. Biodiversity 6, 809–817. doi: 10.1002/cbdv.200800113
Chen, M., Qin, J.-J., Fu, J.-J., Hu, X.-J., Liu, X.-H., Zhang, W.-D., et al. (2010). Blumeaenes A–J, sesquiterpenoid esters from Blumea balsamifera with NO inhibitory activity. Planta Med. 76, 897–902. doi: 10.1055/s-0029-1240800
Chen, C., Wu, Y., Li, J., Wang, X., Zeng, Z., Xu, J., et al. (2023). TBtools-II: A “one for all, all for one” bioinformatics platform for biological big-data mining. Mol. Plant 16, 1733–1742. doi: 10.1016/j.molp.2023.09.010
Cheong, J.-J., Do Choi, Y. (2003). Methyl jasmonate as a vital substance in plants. Trends Genet. 19, 409–413. doi: 10.1016/S0168-9525(03)00138-0
Dhiman, A., Suhag, R., Thakur, D., Gupta, V., Prabhakar, P. K. (2022). Current status of Loquat (Eriobotrya japonica Lindl.): Bioactive functions, preservation approaches, and processed products. Food Rev. Int. 38, 286–316. doi: 10.1080/87559129.2020.1866007
Du, Y., Zhou, H., Yang, L., Jiang, L., Chen, D., Qiu, D., et al. (2022). Advances in Biosynthesis and Pharmacological Effects of Cinnamomum camphora (L.) Presl Essential Oil. Forests. 13 (7), 1020–316. doi: 10.3390/f1307102
El-Sappah, A. H., Abbas, M., Rather, S. A., Wani, S. H., Soaud, N., Noor, Z., et al. (2023). Genome-wide identification and expression analysis of metal tolerance protein (MTP) gene family in soybean (Glycine max) under heavy metal stress. Mol. Biol. Rep. 50, 2975–2990. doi: 10.1007/s11033-022-08100-x
Fan, X., Mattheis, J. P., Fellman, J. K. (1998). A role for jasmonates in climacteric fruit ripening. Planta 204, 444–449. doi: 10.1007/s004250050278
García-Pastor, M. E., Serrano, M., Guillén, F., Giménez, M. J., Martínez-Romero, D., Valero, D., et al. (2020). Preharvest application of methyl jasmonate increases crop yield, fruit quality and bioactive compounds in pomegranate ‘Mollar de Elche’at harvest and during postharvest storage. J. Sci. Food Agric. 100, 145–153. doi: 10.1002/jsfa.10007
Gilg, A. B., Bearfield, J. C., Tittiger, C., Welch, W. H., Blomquist, G. J. (2005). Isolation and functional expression of an animal geranyl diphosphate synthase and its role in bark beetle pheromone biosynthesis. Proc. Natl. Acad. Sci. U.S.A. 102, 9760–9765. doi: 10.1073/pnas.0503277102
Grabherr, M. G., Haas, B. J., Yassour, M., Levin, J. Z., Thompson, D. A., Amit, I., et al. (2011). Full-length transcriptome assembly from RNA-Seq data without a reference genome. Nat. Biotechnol. 29, 644–652. doi: 10.1038/nbt.1883
Guan, L.-L., PANG, Y.-X., WANG, D., ZHANG, Y.-B., WU, K.-Y. (2012). Research progress on Chinese Minority Medicine of Blumea balsamifera L DC. J. Plant Genet. Resour. 13, 695–698.
Guan, L., Yang, Y., Jiang, P., Mou, Q., Gou, Y., Zhu, X., et al. (2022). Potential distribution of Blumea balsamifera in China using MaxEnt and the ex situ conservation based on its effective components and fresh leaf yield. Environ. Sci. pollut. Res. 29, 44003–44019. doi: 10.1007/s11356-022-18953-1
Hasegawa, H., Yamada, Y., Komiyama, K., Hayashi, M., Ishibashi, M., Yoshida, T., et al. (2006). Dihydroflavonol BB-1, an extract of natural plant Blumea balsamifera, abrogates TRAIL resistance in leukemia cells. Blood 107, 679–688. doi: 10.1182/blood-2005-05-1982
Hou, J., Zhang, J., Zhang, B., Jin, X., Zhang, H., Jin, Z. (2020). Transcriptional Analysis of Metabolic Pathways and Regulatory Mechanisms of Essential Oil Biosynthesis in the Leaves of Cinnamomum camphora (L.) Presl. Front. Genet 11, 598714. doi: 10.3389/fgene.2020.598714
Hsieh, M. H., Goodman, H. M. (2005). The Arabidopsis IspH homolog is involved in the plastid nonmevalonate pathway of isoprenoid biosynthesis. Plant Physiol. 138, 641–653. doi: 10.1104/pp.104.058735
Ilahy, R., Tlili, I., Pék, Z., Montefusco, A., Siddiqui, M. W., Homa, F., et al. (2020). Pre-and post-harvest factors affecting glucosinolate content in broccoli. Front. Nutr. 7, 147. doi: 10.3389/fnut.2020.00147
Jirakitticharoen, S., Wisuitiprot, W., Jitareerat, P., Wongs-Aree, C. (2022). Phenolics, antioxidant and antibacterial activities of immature and mature Blumea balsamifera leaf extracts eluted with different solvents. J. Trop. Med. 2022. doi: 10.1155/2022/7794227
Kim, M. J., Chiu, Y.-C., Kim, N. K., Park, H. M., Lee, C. H., Juvik, J. A., et al. (2017). Cultivar-specific changes in primary and secondary metabolites in pak choi (Brassica rapa, Chinensis group) by methyl jasmonate. Int. J. Mol. Sci. 18, 1004. doi: 10.3390/ijms18051004
Kubota, H., Kojima-Yuasa, A., Morii, R., Huang, X., Norikura, T., Rho, S.-N., et al. (2009). Anti-obesity effect of Blumea balsamifera extract in 3T3-L1 preadipocytes and adipocytes. Am. J. Chin. Med. 37, 843–854. doi: 10.1142/S0192415X09007326
Lee, C., Kim, S.-Y., Eum, S., Paik, J.-H., Bach, T. T., Darshetkar, A. M., et al. (2019). Ethnobotanical study on medicinal plants used by local Van Kieu ethnic people of Bac Huong Hoa nature reserve, Vietnam. J. Ethnopharmacol 231, 283–294. doi: 10.1016/j.jep.2018.11.006
Li, X., Li, Y., Zhao, M., Hu, Y., Meng, F., Song, X., et al. (2021). Molecular and metabolic insights into anthocyanin biosynthesis for leaf color change in chokecherry (Padus virginiana). Int. J. Mol. Sci. 22, 10697. doi: 10.3390/ijms221910697
Lichtenthaler, H. K. (1999). The 1-deoxy-D-xylulose-5-phosphate pathway of isoprenoid biosynthesis in plants. Annu. Rev. Plant Physiol. Plant Mol. Biol. 50, 47–65. doi: 10.1146/annurev.arplant.50.1.47
Liu, L., Wei, J., Zhang, M., Zhang, L., Li, C., Wang, Q. (2012). Ethylene independent induction of lycopene biosynthesis in tomato fruits by jasmonates. J. Exp. Bot. 63, 5751–5761. doi: 10.1093/jxb/ers224
Ma, R., Lu, D., Wang, J., Xie, Q., Guo, J. (2023). Comparison of pharmacological activity and safety of different stereochemical configurations of borneol: L-borneol, D-borneol, and synthetic borneol. Biomed Pharmacother 164, 114668. doi: 10.1016/j.biopha.2023.114668
Masyudi, M., Hanafiah, M., Rinidar, R., Usman, S., Marlina, M. (2022). Phytochemical screening and GC-MS analysis of bioactive compounds of Blumea balsamifera leaf extracts from South Aceh, Indonesia. Biodiversitas J. Biol. Diversity 23. doi: 10.13057/biodiv/d230319
Mei, Y., Li, L., Fan, L., Fan, W., Liu, L., Zhang, F., et al. (2023). The history, stereochemistry, ethnopharmacology and quality assessment of borneol. J. Ethnopharmacol 300, 115697. doi: 10.1016/j.jep.2022.115697
Meza, S. L. R., de Castro Tobaruela, E., Pascoal, G. B., Magalhães, H. C. R., Massaretto, I. L., Purgatto, E. (2022). Induction of metabolic changes in amino acid, fatty acid, tocopherol, and phytosterol profiles by exogenous methyl jasmonate application in tomato fruits. Plants 11, 366. doi: 10.3390/plants11030366
Moghtader, M., Salari, H., Farahmand, A. (2011). Evaluation of the antifungal effects of rosemary oil and comparison with synthetic borneol and fungicide on the growth of Aspergillus flavus. J. Ecol. Natural Environ. 3, 210–214.
Nessa, F., Ismail, Z., Mohamed, N., Haris, M. R. H. M. (2004). Free radical-scavenging activity of organic extracts and of pure flavonoids of Blumea balsamifera DC leaves. Food Chem. 88, 243–252. doi: 10.1016/j.foodchem.2004.01.041
Pandey, K. B., Rizvi, S. I. (2009). Plant polyphenols as dietary antioxidants in human health and disease. Oxid. Med. Cell. Longevity 2, 270–278. doi: 10.4161/oxim.2.5.9498
Pang, Y., Wang, D., Fan, Z., Chen, X., Yu, F., Hu, X., et al. (2014). Blumea balsamifera—A phytochemical and pharmacological review. Molecules 19, 9453–9477. doi: 10.3390/molecules19079453
Pang, Y., Wang, W., Zhang, Y., Mo, T., Yuan, Y. (2010). Clonal diversity and structure in natural populations of Blumea balsamifera. Guangxi Zhiwu/Guihaia 30, 209–214.
Pang, Y., Zhang, Y., Huang, L., Xu, L., Wang, K., Wang, D., et al. (2017). Effects and mechanisms of total flavonoids from Blumea balsamifera (L.) DC. @ on skin wound in rats. Int. J. Mol. Sci. 18, 2766. doi: 10.3390/ijms18122766
Pant, P., Pandey, S., Dall'Acqua, S. (2021). The influence of environmental conditions on secondary metabolites in medicinal plants: A literature review. Chem. Biodiversity 18, e2100345. doi: 10.1002/cbdv.202100345
Phumthum, M., Srithi, K., Inta, A., Junsongduang, A., Tangjitman, K., Pongamornkul, W., et al. (2018). Ethnomedicinal plant diversity in Thailand. J. Ethnopharmacol 214, 90–98. doi: 10.1016/j.jep.2017.12.003
Rehman, R., Hanif, M. A., Mushtaq, Z., Al-Sadi, A. M. (2016). Biosynthesis of essential oils in aromatic plants: A review. Food Rev. Int. 32, 117–160. doi: 10.1080/87559129.2015.1057841
Ruan, J., Zhou, Y., Zhou, M., Yan, J., Khurshid, M., Weng, W., et al. (2019). Jasmonic acid signaling pathway in plants. Int. J. Mol. Sci. 20, 2479. doi: 10.3390/ijms20102479
Sadia, S., Tariq, A., Shaheen, S., Malik, K., Ahmad, M., Qureshi, H., et al. (2018). Ethnopharmacological profile of anti-arthritic plants of Asia-a systematic review. J. Herbal Med. 13, 8–25. doi: 10.1016/j.hermed.2018.08.003
Sayyari, M., Babalar, M., Kalantari, S., Martínez-Romero, D., Guillén, F., Serrano, M., et al. (2011). Vapour treatments with methyl salicylate or methyl jasmonate alleviated chilling injury and enhanced antioxidant potential during postharvest storage of pomegranates. Food Chem. 124, 964–970. doi: 10.1016/j.foodchem.2010.07.036
Shafiq, M., Singh, Z., Khan, A. S. (2013). Time of methyl jasmonate application influences the development of ‘Cripps Pink’apple fruit colour. J. Sci. Food Agric. 93, 611–618. doi: 10.1002/jsfa.5851
Sharma, D., Shree, B., Kumar, S., Kumar, V., Sharma, S., Sharma, S. (2022). Stress induced production of plant secondary metabolites in vegetables: Functional approach for designing next generation super foods. Plant Physiol. Biochem. 192, 252–272. doi: 10.1016/j.plaphy.2022.09.034
Shirota, O., Oribello, J. M., Sekita, S., Satake, M. (2011). Sesquiterpenes from blumea balsamifera. J. Natural Prod 74, 470–476. doi: 10.1021/np100646n
Singh, A., Singh, S., Singh, I. K. (2016). Recent insights into the molecular mechanism of jasmonate signaling during insect-plant interaction. Australas. Plant Pathol. 45, 123–133. doi: 10.1007/s13313-015-0392-1
Tian, Z., Zeng, P., Lu, X., Zhou, T., Han, Y., Peng, Y., et al. (2022). Thirteen Dipterocarpoideae genomes provide insights into their evolution and borneol biosynthesis. Plant Commun. 3, 100464. doi: 10.1016/j.xplc.2022.100464
Turner, J. G., Ellis, C., Devoto, A. (2002). The jasmonate signal pathway. Plant Cell 14, S153–S164. doi: 10.1105/tpc.000679
Wan, L., Huang, Q., Ji, X., Song, L., Zhang, Z., Pan, L., et al. (2023). RNA sequencing in Artemisia annua L explored the genetic and metabolic responses to hardly soluble aluminum phosphate treatment. Funct. Integr. Genomics 23, 1–15. doi: 10.1007/s10142-023-01067-3
Wan, L., Huo, J., Huang, Q., Ji, X., Song, L., Zhang, Z., et al. (2024). Genetics and metabolic responses of Artemisia annua L to the lake of phosphorus under the sparingly soluble phosphorus fertilizer: evidence from transcriptomics analysis. Funct. Integr. Genomics 24, 26. doi: 10.1007/s10142–024-01301–6
Wang, S. Y., Bowman, L., Ding, M. (2008). Methyl jasmonate enhances antioxidant activity and flavonoid content in blackberries (Rubus sp.) and promotes antiproliferation of human cancer cells. Food Chem. 107, 1261–1269.
Wang, Q., Deng, K., Ai, J., Wang, Y., Wang, Y., Ren, Y., et al. (2023). Integrated transcriptomic and metabolomic analyses reveal the effects of grafting on special metabolites of acanthopanax senticosus leaves. Molecules 28, 4877. doi: 10.3390/molecules28124877
Wang, Y., Shi, L., Wang, A., Tian, H., Wang, H., Zou, C. (2014). Preparation of high-purity (–)-borneol and xanthoxylin from leaves of blumea balsamifera (L.) DC. Separation Sci. Technol. 49, 1535–1540. doi: 10.1080/01496395.2014.891613
Wang, C., Zhang, J., Lv, J., Li, J., Gao, Y., Patience, B. E., et al. (2022). Effect of methyl jasmonate treatment on primary and secondary metabolites and antioxidant capacity of the substrate and hydroponically grown chinese chives. Front. Nutr. 9. doi: 10.3389/fnut.2022.859035
Weng, C.-J., Yen, G.-C. (2012). Chemopreventive effects of dietary phytochemicals against cancer invasion and metastasis: phenolic acids, monophenol, polyphenol, and their derivatives. Cancer Treat Rev. 38, 76–87. doi: 10.1016/j.ctrv.2011.03.001
Widhiantara, I. G., Jawi, I. M. (2021). Phytochemical composition and health properties of Sembung plant (Blumea balsamifera): A review. Veterinary World 14, 1185. doi: 10.14202/vetworld.2021.1185-1196
Wolucka, B. A., Goossens, A., Inzé, D. (2005). Methyl jasmonate stimulates the de novo biosynthesis of vitamin C in plant cell suspensions. J. Exp. Bot. 56, 2527–2538. doi: 10.1093/jxb/eri246
Yang, Z., An, W., Liu, S., Huang, Y., Xie, C., Huang, S., et al. (2020). Mining of candidate genes involved in the biosynthesis of dextrorotatory borneol in Cinnamomum burmannii by transcriptomic analysis on three chemotypes. PeerJ 8, e9311. doi: 10.7717/peerj.9311
Zhang, M., Su, P., Zhou, Y. J., Wang, X. J., Zhao, Y. J., Liu, Y. J., et al. (2015). Identification of geranylgeranyl diphosphate synthase genes from Tripterygium wilfordii. Plant Cell Rep. 34, 2179–2188. doi: 10.1007/s00299–015-1860–3
Zhang, H., Tsao, R. (2016). Dietary polyphenols, oxidative stress and antioxidant and anti-inflammatory effects. Curr. Opin. Food Sci. 8, 33–42. doi: 10.1016/j.cofs.2016.02.002
Keywords: Ainaxiang, cytoplasmic mevalonate, exogenous treatment, RNA sequencing, geranyl diphosphate
Citation: Guan L, Lin N, Wan L, Yu F, Chen X, Xie X, Yuan C, Soaud SA, Abd Elhamid MA, Heakel RMY, Wang L and El-Sappah AH (2024) Transcriptome analysis revealed the role of moderate exogenous methyl jasmonate treatments in enhancing the metabolic pathway of L-borneol in the Blumea balsamifera. Front. Plant Sci. 15:1391042. doi: 10.3389/fpls.2024.1391042
Received: 24 February 2024; Accepted: 28 May 2024;
Published: 26 June 2024.
Edited by:
Mohammad Faisal, King Saud University, Saudi ArabiaReviewed by:
Harnish Mukesh Naik, Johns Hopkins University, United StatesTao Yi, Hong Kong Baptist University, Hong Kong SAR, China
Copyright © 2024 Guan, Lin, Wan, Yu, Chen, Xie, Yuan, Soaud, Abd Elhamid, Heakel, Wang and El-Sappah. This is an open-access article distributed under the terms of the Creative Commons Attribution License (CC BY). The use, distribution or reproduction in other forums is permitted, provided the original author(s) and the copyright owner(s) are credited and that the original publication in this journal is cited, in accordance with accepted academic practice. No use, distribution or reproduction is permitted which does not comply with these terms.
*Correspondence: Ahmed H. El-Sappah, YWhtZWRfZWxzYXBwYWgyMDA2QHlhaG9vLmNvbQ==; Linghui Wang, emp3d2xoMDhAMTI2LmNvbQ==
†These authors have contributed equally to this work and share first authorship