- 1ICAR-Central Plantation Crops Research Institute, Kasaragod, Kerala, India
- 2ICAR-Central Plantation Crops Research Institute Regional Station, Vittal, Dakshina Kannada, Karnataka, India
- 3ICAR-Central Plantation Crops Research Institute, Research Centre, Kahikuchi, Assam, India
1 Introduction
Palms encompass over 2,500 species, across 200 genera, ranking second only to grasses (Poaceae) and legumes (Fabaceae) in the realm of agricultural food production and industrial applications. The coconut (Cocos nucifera L.), arecanut (Areca catechu L.), oil palm (Elaeis guineensis Jacq.), and date palm (Phoenix dactylifera L.) are among the economically significant perennial species within the Arecaceae family. Coconut, often referred to as the “tree of life,” is celebrated for its diverse range of applications in food, nutrition, medicine, and various industrial uses (Ramesh et al., 2021). Coconut products encompass edible oil derived from the kernel or testa, tender coconut water, kernel, copra, coconut shell, coconut cake, wood-based products, coir pith, and items resulting from various valorization processes. The unopened spathe is tapped to extract inflorescence sap (neera), which can be further processed into jaggery, sugar, vinegar, and a variety of secondary products (Hebbar et al., 2022).
Arecanut (Areca catechu L.) is a crop in tropical Asia and certain parts of East Africa. In India, it holds a prominent place as a major commercial crop and is also medically important, primarily grown in a few states of the country. Nevertheless, its commercial products are distributed throughout India, and the country undeniably leads in terms of both area under cultivation and production, accounting for 54% of the world’s output. The fruit or nuts of the Areca catechu L. palm, commonly known as betel nut or supari, have a long history of use as a masticatory product by the Indian population, dating back to the Vedic period. As a result, arecanut is deeply intertwined with India’s history and social heritage. On a global scale, the betel quid is used by as many as 600 million people in Asia alone.
Date palm, on the other hand, thrives in arid regions such as Egypt, Iran, Saudi Arabia, and the UAE, among others (Aljohi et al., 2016). In addition to its fruit, date palm seeds also serve as a novel source of edible oil, further expanding its industrial applications (Ali et al., 2015). Oil palm stands out as an economically vital palm species, supplying approximately 35% of the world’s vegetable oil. The genetic improvement of oil palm could play a pivotal role in global nutritional security.
2 Palm problems
Economically important palm species worldwide are experiencing stagnation in yield, a rapid deceleration in land expansion, the effects of climate change, a surge in the cost of production due to agricultural inputs and labor, as well as biotic stressors like the emergence or reemergence of major pests and diseases, among other challenges (Figure 1). The long juvenile phase of palms, which can extend beyond 5-9 years, the time-consuming process of backcross breeding (30 years for date palms and 15-18 years for oil palms), and the reliance on seeds, as the primary propagule, severely hinder palm crop improvement programs. Therefore, expediting the adoption of novel breeding technologies, such as genome editing (GE), is imperative to ensure that missed opportunities in genomics-assisted breeding and genetic engineering, which have already revolutionized other cereal and legume crops, are not lost.
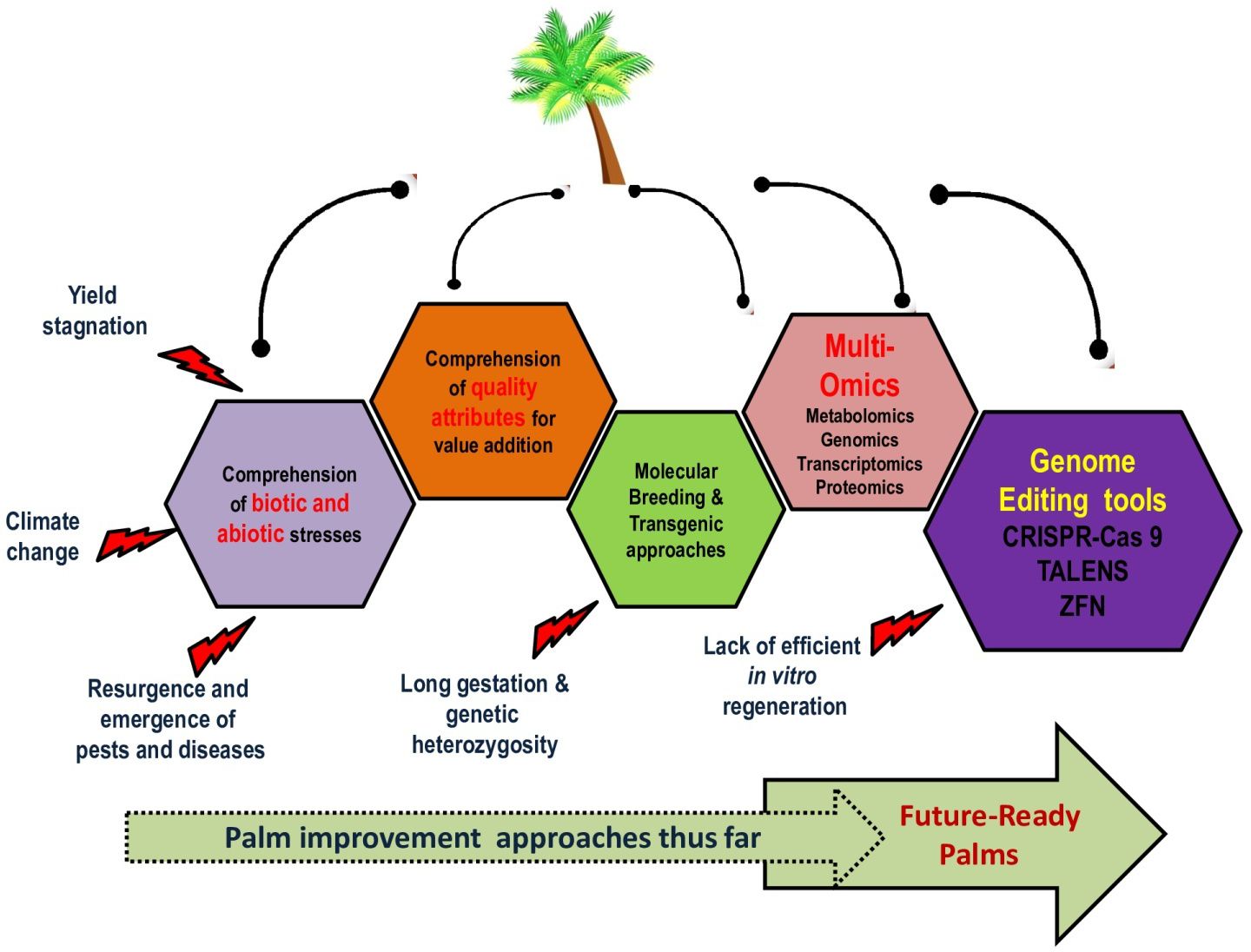
Figure 1 General considerations of research in palms and the defining role of genome editing in developing future-ready palms.
Genome editing (GE) approaches have been transforming the fields of plant breeding and genomics by enabling precise genetic manipulation of crop species. The utilization of CRISPR/Cas9 holds significant promise in revolutionizing agriculture and creating opportunities for innovative developments in plant gene editing systems (Chinnusamy et al., 2023; Saini et al., 2023). While the effectiveness of GE techniques has been demonstrated in other field crops and even in tree crops, it remains a relatively uncharted path for genetic improvement in palm species such as coconut, arecanut, or date palm, with the exception of oil palm. The utilization of genetic engineering technologies for commercial purposes in palms is currently quite limited. In addition to the complexity of palm genomes, significant obstacles related to genetic transformation and the development of efficient regeneration protocols pose major challenges to the widespread adoption of GE technologies.
3 Palm genomic resources
Continuous improvement of genomic resources for palm species is of paramount importance. Over the past decade, there has been a significant increase in the scale of genome assemblies for palms, opening the door to multi-omics studies. Genome assemblies are now available for economically important palm species such as oil palm (Elaeis guineensis Jacq), date palm (Phoenix dactylifera L), coconut (Cocos nucifera L), and arecanut (Areca catechu L), as well as related species like Calamus simplicifolius and Daemonorops jenkinsiana. These assemblies have been complemented by numerous resequencing and transcriptomics approaches (Al-Mssallem et al., 2013; Singh et al., 2013; Wang et al., 2021; Yang et al., 2021; Zhao et al., 2018). In this context, the development of the Arecaceae Multi-omics Database, ArecaceaeMDB (http://arecaceae-gdb.com), is a significant step forward. This database houses genomes of six economically important palms, along with resequencing data from 1631 different accessions, over 800 transcriptome sequences, and 138 metabolome datasets (Yang et al., 2023). However, unlike fruit and nut crops that benefit from well-assembled and high-quality genome sequences (Savadi et al., 2021), palms, such as date palm, present unique challenges due to their dioecious nature, requiring specialized assembly software and additional considerations for the development of effective genomic resources (Hazzouri et al., 2020). Nonetheless, the successful demonstration of the proof-of-concept of CRISPR/Cas9 application in various fruit tree crops, including apple, cacao, coffee, citrus, grape, pomegranate, pear, and walnut (Savadi et al., 2021), as well as in some forest tree crops (Cao et al., 2022) and in palms (Yeap et al., 2021), suggests that this approach may become a mainstay in the long run.
4 Potential applications of CRISPR-Cas9 system in palms
In the context of coconut, the identification of disease susceptibility factors represents a crucial area of research. This is essential to identify genomic regions or genes responsible for disease susceptibility, with the aim of manipulating them through the adoption of gene-editing technologies. Some of the potential gene targets include PR1, PR4, the pathogenesis-related genes transcriptional activator PT15-like gene, thaumatin-like protein, HSP70, and glutathione S-transferase. These genes have been identified as susceptibility factors in the case of root (wilt) disease in coconut (Rajesh et al., 2015; Verma et al., 2017; Arumugam and Hatta, 2022). Considering the diversity of pests affecting palms, adoption of multi-pronged strategies such as CRISPR-Cas9 based sterile insect technique, and targeting female insect reproductive fitness (eg., egg-specific protein encoding gene), or incorporating ovary targeting molecular signals in CRISPR-Cas9 system for heritable genome editing are warranted. These strategies aim to enhance the resilience of palm crops against insect pest damage.
In certain plants characterized by a low transformation efficiency, such as maize, the issue of limited transformation success has been successfully addressed through the over-expression of key regulators of somatic embryogenesis, such as Baby Boom (Bbm) and Wuschel2 (Wus2). While this particular approach has not yet been explored in the context of coconut, there is potential value in enhancing the regeneration process by adopting this method.
Palm products, especially those derived from the minimal processing of tender coconut nuts, require suitable anti-browning agents to prevent enzymatic browning reactions, ensuring visually appealing end products. In addition to the manipulation of agronomic traits, the application of the CRISPR/Cas9 system has been explored to mitigate enzymatic browning. This is achieved by creating mutations in the StPPO2 gene, resulting in a significant reduction in enzymatic browning by up to 75% (González et al., 2020). Similarly, Maioli et al. (2020) discussed the potential application of genome editing technologies in the development of eggplant berries, which exhibited a 52% reduction in PPO activity in edited lines compared to wild types, achieved by knocking out three PPO genes (SmelPPO4, SmelPPO5, and SmelPPO6). These studies demonstrate that, in addition to reducing enzymatic browning, the nutritional potential of post-harvest produce, including their antioxidant potential and phenolic content, is effectively preserved.
Palms in field conditions face a range of abiotic stresses induced by climate change, including monsoon variability, elevated temperature stress, rising atmospheric CO2 levels, and sea-level rise leading to salinity stress. Unlike annual crops, palms endure these abiotic challenges for an extended period during their lifespan, necessitating the adoption of genomics technologies to mitigate these stresses. Genetic mapping of genes associated with abiotic stress tolerance or related traits in mature palms is hindered due to their long juvenile phase and the costs involved in cultivating and maintaining large mapping populations in the field. Additionally, regions where palms are grown are experiencing increased soil salinity and sea-level rise, making it imperative to molecularly characterize abiotic stress response pathways.
Consequently, the adoption of CRISPR/Cas9 editing in palms involves numerous strategic considerations, including the selection of target genes, the sequence features of sgRNA, the method and tissue used for delivery, and the implementation of appropriate in vitro regeneration protocols. The highly heterozygous nature of palm genomes presents a challenge in designing sgRNAs that effectively match the target gene sequences near a protospacer adjacent motif (PAM) site. Traditional breeding methods, which can take 15-20 years or more, coupled with obstacles in developing genetic engineering-based high oleic acid-producing oil palm lines, have prompted the use of a multiplex CRISPR/Cas9 system to target multiple genomic sites (Bahariah et al., 2023). Given the nutritional significance of dietary oils, the application of CRISPR/Cas9 technology for targeting oil palm genes such as fatty acid desaturase 2 (FAD2) and palmitoyl-acyl carrier protein thioesterase (PAT) to modulate fatty acid metabolism and produce high-oleic acid oil holds significant promise for similar interventions in coconut palms (Bahariah et al., 2023).
Tree crops necessitate rapid genetic modification protocols and the development of genetically modified plants within a few generations as expediently as possible. In this context, the biallelic edits achievable through the CRISPR/Cas9 approach offer a means to swiftly generate genetically homozygous lines, circumventing the need for elaborate breeding methods to introduce homozygosity (Hazzouri et al., 2020). The successful establishment of electroporation-mediated protoplast transformation of sgRNA and genome editing components in oil palm suggests the potential for generating DNA-free genome-edited palms. However, the lack of an effective regeneration protocol for oil palm protoplasts significantly hampers the development of DNA-free genome-edited palms. Moreover, the creation of an efficient in vitro electro-transfection assay in oil palm for rapid assessment and evaluation of gRNA efficiency would substantially reduce the time and cost of transformation and regeneration, particularly for oil palm and other palm species (Yeap et al., 2021). In addition, development of gene editing protocol through de novo induction of meristems in dicots and the efficiency of cut–dip–budding (CDB) delivery system could enable rapid production of genetically modified germplasm (Maher et al., 2020; Cao et al., 2023). By implementing the PEG-mediated delivery system for Cas proteins and sgRNA, coupled with the establishment of a proficient protoplast regeneration system for palms, as successfully demonstrated in Hevea brasiliensis (Fan et al., 2020), we can significantly expedite the genome-editing-mediated breeding process.
Palm genomes are characterized by high allelic heterozygosity due to their outcrossing nature. Consequently, the presence of a high number of single nucleotide polymorphisms (SNPs) in the genome makes GE technologies less efficient in these crops. Thus, resequencing cultivars of interest and incorporating features related to the multi-ploidy nature of palm genomes into web-based algorithms, for sgRNA design in plants, are recommended to enhance the existing genomic resources for homozygous crops and model plants (Sattar et al., 2017). Nevertheless, it is anticipated that the application of CRISPR/Cas9-based genome editing will greatly facilitate the exploration of gene-function relationships and their impact on phenotypic traits, expediting crop improvement programs in palm species (Table 1).
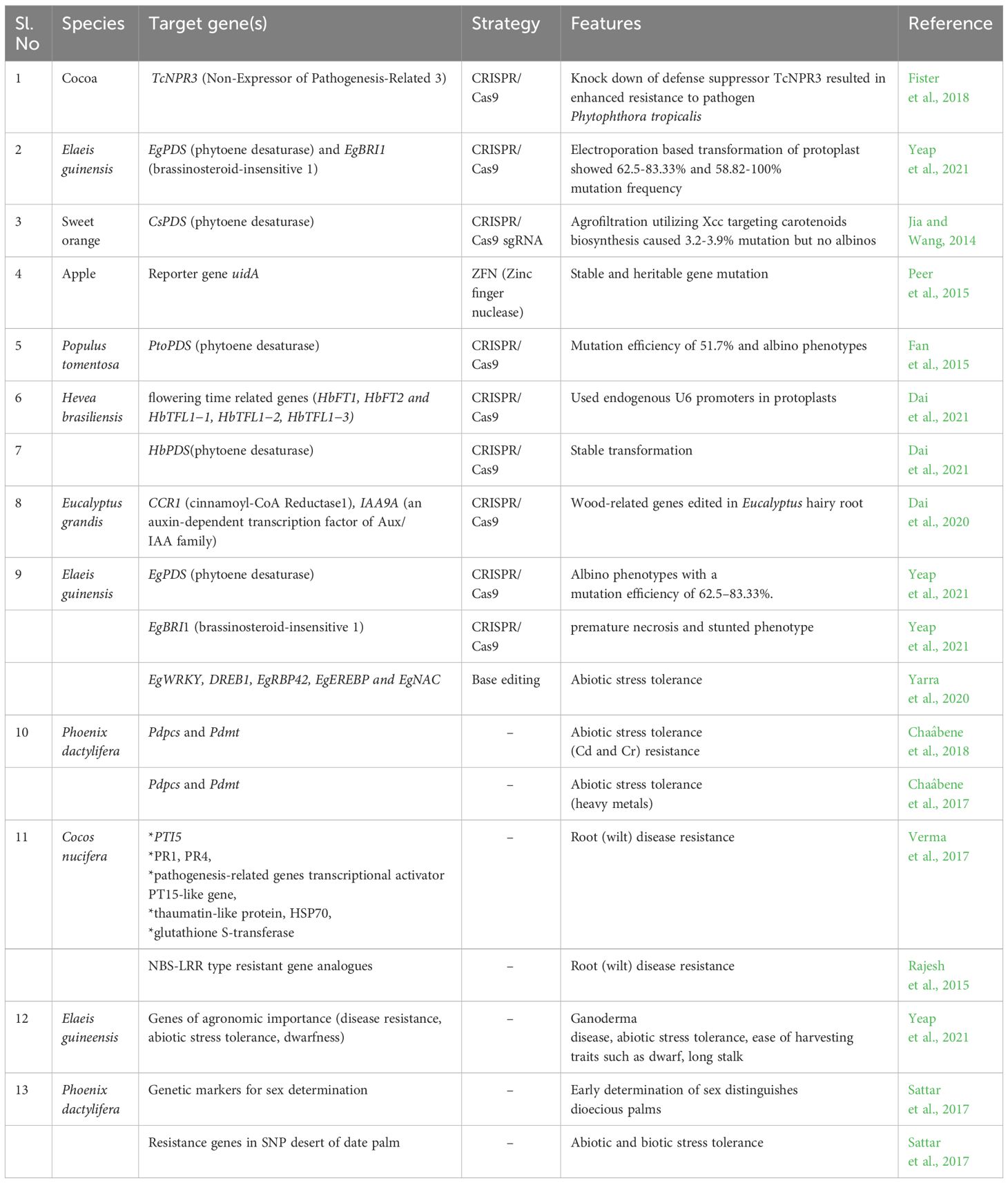
Table 1 Major achievements in genome editing (GE) for tree crops and the prospects for its application in palms.
5 Concluding remarks
Thus far, the CRISPR/Cas9 technology has demonstrated its effectiveness in genome editing of trees. Genome modification has been successfully accomplished in oil palm, and numerous fruit and nut tree crops. Several genes within these tree or perennials have manipulated through genome editing, aimed at enhancing resistance to both biotic and abiotic stressors, manipulating flowering and fruit ripening times, improving plant growth attributes, and enhancing the flavor profiles of their fruits. Innovations have led to the development of modified enzymes, offering increased efficiency in genome editing. Additionally, new and improved systems for gene editing and simultaneous activation of transcription have emerged, which are pertinent to the creation of novel palm varieties with wider applications.
Author contributions
SR: Conceptualization, Formal analysis, Investigation, Resources, Writing – original draft, Writing – review & editing. MR: Formal analysis, Writing – review & editing. KH: Formal analysis, Resources, Writing – review & editing. AD: Formal analysis, Funding acquisition, Writing – review & editing.
Funding
The author(s) declare that financial support was received for the research, authorship, and/or publication of this article. Authors acknowledge the funding for this work from ICAR-Central Plantation Crops Research Institute (ICAR-CPCRI Grant No: 1000766014) and ICAR-NEH fund of ICAR-CPCRI, Kahikuchi.
Conflict of interest
The authors declare that the research was conducted in the absence of any commercial or financial relationships that could be construed as a potential conflict of interest.
Publisher’s note
All claims expressed in this article are solely those of the authors and do not necessarily represent those of their affiliated organizations, or those of the publisher, the editors and the reviewers. Any product that may be evaluated in this article, or claim that may be made by its manufacturer, is not guaranteed or endorsed by the publisher.
References
Ali, M. A., Al-Hattab, T. A., Al-Hydary, I. A. (2015). Extraction of date palm seed oil (Phoenix dactylifera) by soxhlet apparatus. Int. J. Adv. Eng. Technol. 8 (3), 261–271.
Aljohi, H. A., Liu, W., Lin, Q., Zhao, Y., Zeng, J., Alamer, A., et al. (2016). Complete sequence and analysis of coconut palm (Cocos nucifera) mitochondrial genome. PloS One 11 (10), e0163990. doi: 10.1371/journal.pone.0163990
Al-Mssallem, I. S., Hu, S., Zhang, X., Lin, Q., Liu, W., Tan, J., et al. (2013). Genome sequence of the date palm phoenix dactylifera l. Nat. Commun. 4 (1), 2274. doi: 10.1038/ncomms3274
Arumugam, T., Hatta, M. A. D. (2022). Improving coconut using modern breeding technologies: Challenges and Opportunities. Plants 11, 3414. doi: 10.3390/plants11243414
Bahariah, B., Masani, M. Y. A., Fizree, M.P.M.A.A., Rasid, O. A., Parveez, G. K. A. (2023). Multiplex CRISPR/Cas9 gene-editing platform in oil palm targeting mutations in EgFAD2 and EgPAT genes. J. Genet. Eng. Biotechnol. 21, 3. doi: 10.1186/s43141-022-00459-5
Cao, X., Xie, H., Song, M., Lu, J., Ma, P., Huang, B., et al. (2023). Cut–dip–budding delivery system enables genetic modifications in plants without tissue culture. Innovation 4 (1), 100345. doi: 10.1016/j.xinn.2022.100345
Cao, X. H., Vu, G. T. H., Gailing, O. (2022). From genome sequencing to CRISPR-based genome editing for climate-resilient forest. Int. J. Mol. Sci. 23, 966. doi: 10.3390/ijms23020966
Chaâbene, Z., Hakim, I. R., Rorat, A., Elleuch, A., Mejdoub, H., Vandenbulcke, F. (2018). Copper toxicity and date palm (Phoenix dactylifera) seedling tolerance: monitoring of related biomarkers. Environ. Toxicol. Chem. 37 (3), 797–806.
Chaâbene, Z., Imen, R. H., Walid, K., Grubb, C. D., Bassem, K., Franck, V., et al. (2017). The phytochelatin synthase gene in date palm (Phoenix dactylifera l.): Phylogeny, evolution and expression. Ecotoxicol. Environ. Saf. 140, 7–17.
Chinnusamy, V., Schepler-Luu, V., Mangrauthia, S. K., Ramesh, S. V. (2023). Genome editing in plants: a tool for precision breeding and functional genomics. J. Plant Biochem. Biotechnol. 32, 657–660. doi: 10.1007/s13562-023-00867-9
Dai, Y., Hu, G., Dupas, A., Medina, L., Blandels, N., San Clemente, H., et al. (2020). Implementing the CRISPR/cas9 technology in eucalyptus hairy roots using wood-related genes. Int. J. Mol. Sci. 21, 3408. doi: 10.3390/ijms21103408
Dai, X., Yang, X., Wang, C., Fan, Y., Xin, S., Hua, Y., et al. (2021). CRISPR/Cas9-mediated genome editing in hevea brasiliensis. Ind. Crops And Products 164, 113418. doi: 10.1016/j.indcrop.2021.113418
Fan, D., Liu, T., Li, C., Jiao, B., Li, S., Hou, Y., et al. (2015). Efficient CRISPR/Cas9-mediated targeted mutagenesis in Populus in the first generation. Sci. Rep. 5, 12217. doi: 10.1038/srep12217
Fan, Y., Xin, S., Dai, X., Yang, X., Huang, H., Hua, Y. (2020). Efficient genome editing of rubber tree (Hevea brasiliensis) protoplasts using CRISPR/Cas9 ribonucleoproteins. Ind. Crop Prod. 146, 112146. doi: 10.1016/j.indcrop.2020.112146
Fister, A. S., Landherr, L., Maximova, S. N., Guiltinan, M. J. (2018). Transient expression of CRISPR/Cas9 machinery targeting TcNPR3 enhances defense response in Theobroma cacao. Front. Plant Sci. 2;9. doi: 10.3389/fpls.2018.00268
González, M. N., Massa, G. A., Andersson, M., Turesson, H., Olsson, N., Fält, A. S., et al. (2020). Reduced enzymatic browning in potato tubers by specific editing of a polyphenol oxidase gene via ribonucleoprotein complexes delivery of the CRISPR/Cas9 System. Front. Plant Sci. 10. doi: 10.3389/fpls.2019.01649
Hazzouri, K. M., Flowers, J. M., Nelson, D., Lemansour, A., Masmoudi, K., Amiri, K. M. A. (2020). Prospects for the study and improvement of abiotic stress tolerance in date palms in the post-genomics era. Front. Plant Sci. 11. doi: 10.3389/fpls.2020.00293
Hebbar, K. B., Ramesh, S. V., Ghosh, D. K., Beegum, S., Pandiselvam, R., Manikantan, M. R., et al. (2022). Coconut sugar-a potential storehouse of nutritive metabolites, novel bio-products and prospects. Sugar Tech 24 (3), 841–856.
Jia, H., Wang, N. (2014). Xcc-facilitated agroinfiltration of citrus leaves: A tool for rapid functional analysis of transgenes in citrus leaves. Plant Cell Rep. 33, 1993–2001. doi: 10.1007/s00299-014-1673-9
Maher, M. F., Nasti, R. A., Vollbrecht, M., Starker, C. G., Clark, M. D., Voytas, D. F. (2020). Plant gene editing through de novo induction of meristems. Nat. Biotechnol. 38, 84–89. doi: 10.1038/s41587-019-0337-2
Maioli, A., Gianoglio, S., Moglia, A., Acquadro, A., Valentino, D., Milani, A. M., et al. (2020). Simultaneous CRISPR/Cas9 editing of three PPO genes reduces fruit flesh browning in Solanum melongena L. Front. Plant Sci. 11. doi: 10.3389/fpls.2020.607161
Peer, R., Rivlin, G., Golobovitch, G., Lapidot, M., Gal-On, A., Vainstein, A., et al. (2015). Targeted mutagenesis using zinc-finger nucleases in perennial fruit trees. Planta 241, 941–951. doi: 10.1007/s00425-014-2224-x
Rajesh, M. K., Rachana, K. E., Naganeeswaran, S. A., Shafeeq, R., Thomas, R. J., Shareefa, M., et al. (2015). Identification of expressed resistance gene analog sequences in coconut leaf transcriptome and their evolutionary analysis. Turkish J. Agric. Forestry 39 (3), 489–502.
Ramesh, S. V., Pandiselvam, R., Shameena Beegum, P. P., Saravana Kumar, R. M., Manikantan, M. R., Hebbar, K. B. (2021). Review of Cocos nucifera L. testa- derived phytonutrients with special reference to phenolics and its potential for encapsulation. J. Food Sci. Technol. 60, 1–10. doi: 10.1007/s13197-021-05310-2
Saini, H., Thakur, R., Gill, R., Tyagi, K., Goswami, M. (2023). CRISPR/Cas9-gene editing approaches in plant breeding. GM Crops Food 14, pp.1–pp17. doi: 10.1080/21645698.2023.2256930
Sattar, M. N., Iqbal, Z., Tahir, M. N., Shahid, M. S., Khurshid, M., Al-Khateeb, A. A., et al. (2017). CRISPR/Cas9: a practical approach in date palm genome editing. Front. Plant Sci. 8. doi: 10.3389/fpls.2017.01469
Savadi, S., Mangalassery, S., Sandesh, M. S. (2021). Advances in genomics and genome editing for breeding next generation of fruit and nut crops. Genomics 113, 3718–3734. doi: 10.1016/j.ygeno.2021.09.001
Singh, R., Ong-Abdullah, M., Low, E. T. L., Manaf, M. A. A., Rosli, R., Nookiah, R., et al. (2013). Oil palm genome sequence reveals divergence of interfertile species in old and new worlds. Nature 500 (7462), 335–339.
Verma, S. K., Jasrotia, R. S., Iquebal, M., Jaiswal, S., Angadi, U., Rai, A., et al. (2017). Deciphering genes associated with root wilt disease of coconut and development of its transcriptomic database (CnTDB). Physiol. Mol. Plant Pathol. 100, 255–263. doi: 10.1016/j.pmpp.2017.03.011
Wang, S., Xiao, Y., Zhou, Z. W., Yuan, J., Guo, H., Yang, Z., et al. (2021). High-quality reference genome sequences of two coconut cultivars provide insights into evolution of monocot chromosomes and differentiation of fiber content and plant height. Genome Biol. 22, 304. doi: 10.1186/s13059-021-02522-9
Yang, Z., Liu, Z., Xu, H., Li, Y., Huang, S., Cao, G., et al. (2023). ArecaceaeMDB: a comprehensive multi-omics database for Arecaceae breeding and functional genomics studies. Plant Biotechnol. J. 21, 11. doi: 10.1111/pbi.13945
Yang, Y., Huang, L., Xu, C., Qi, L., Wu, Z., Li, J., et al. (2021). Chromosome-scale genome assembly of areca palm (Areca catechu). Mol. Ecol. Resour. 21 (7), 2504–2519.
Yarra, R., Cao, H., Jin, L., Mengdi, Y., Zhou, L. (2020). CRISPR/Cas mediated base editing: A practical approach for genome editing in oil palm. 3 Biotech. 10, 306. doi: 10.1007/s13205-020-02302-5
Yeap, W.-C., Norkhairunnisa, K. C. M., Norfadzilah, J., Muad, M. R., Appleton, D., Harikrishna, K. (2021). An efficient clustered regularly interspaced short palindromic repeat (CRISPR)/CRISPR-associated protein 9 mutagenesis system for oil palm (Elaeis guineensis). Front. Plant Sci. 12. doi: 10.3389/fpls.2021.773656
Keywords: genomics assisted breeding, genome editing, palm improvement, palms, tree crops
Citation: Ramesh SV, Rajesh MK, Das A and Hebbar KB (2024) CRISPR/Cas9 –based genome editing to expedite the genetic improvement of palms: challenges and prospects. Front. Plant Sci. 15:1385037. doi: 10.3389/fpls.2024.1385037
Received: 11 February 2024; Accepted: 28 February 2024;
Published: 13 March 2024.
Edited by:
Cheng Yuan, Yunnan Academy of Tobacco Agricultural Sciences, ChinaReviewed by:
Tong Zhang, South China Agricultural University, ChinaCopyright © 2024 Ramesh, Rajesh, Das and Hebbar. This is an open-access article distributed under the terms of the Creative Commons Attribution License (CC BY). The use, distribution or reproduction in other forums is permitted, provided the original author(s) and the copyright owner(s) are credited and that the original publication in this journal is cited, in accordance with accepted academic practice. No use, distribution or reproduction is permitted which does not comply with these terms.
*Correspondence: SV Ramesh, cmFtZXNoLnN2QGljYXIuZ292Lmlu