- 1Graduate School, Faculty of Agricultural Science, Universidad Austral de Chile, Valdivia, Chile
- 2Institute of Plant Production and Protection, Universidad Austral de Chile, Valdivia, Chile
- 3Institute of Biochemistry and Microbiology, Universidad Austral de Chile, Valdivia, Chile
- 4National Agency for Research and Development of Chile-Millennium Science Initiative Program-Millennium Institute for Integrative Biology (iBio), Santiago, Chile
Enhancing grain yield is a primary goal in the cultivation of major staple crops, including wheat. Recent research has focused on identifying the physiological and molecular factors that influence grain weight, a critical determinant of crop yield. However, a bottleneck has arisen due to the trade-off between grain weight and grain number, whose underlying causes remain elusive. In a novel approach, a wheat expansin gene, TaExpA6, known for its expression in root tissues, was engineered to express in the grains of the spring wheat cultivar Fielder. This modification led to increases in both grain weight and yield without adversely affecting grain number. Conversely, a triple mutant line targeting the gene TaGW2, a known negative regulator of grain weight, resulted in increased grain weight but decreased grain number, potentially offsetting yield gains. This study aimed to evaluate the two aforementioned modified wheat genotypes (TaExpA6 and TaGW2) alongside their respective wild-type counterparts. Conducted in southern Chile, the study employed a Complete Randomized Block Design with four replications, under well-managed field conditions. The primary metrics assessed were grain yield, grain number, and average grain weight per spike, along with detailed measurements of grain weight and dimensions across the spike, ovary weight at pollination (Waddington’s scale 10), and post-anthesis expression levels of TaExpA6 and TaGW2. Results indicated that both the TaExpA6 and the triple mutant lines achieved significantly higher average grain weights compared to their respective wild types. Notably, the TaExpA6 line did not exhibit a reduction in grain number, thereby enhancing grain yield per spike. By contrast, the triple mutant line showed a reduced grain number per spike, with no significant change in overall yield. TaExpA6 expression peaked at 10 days after anthesis (DAA), and its effect on grain weight over the WT became apparent after 15 DAA. In contrast, TaGW2 gene disruption in the triple mutant line increased ovary size at anthesis, leading to improved grain weight above the WT from the onset of grain filling. These findings suggest that the trade-off between grain weight and number could be attributed to the overlapping of the critical periods for the determination of these traits.
1 Introduction
Advancements in the understanding of physiological and molecular control of grain weight and size in wheat and other staple food crops have been significant over the past decade (Brinton and Uauy, 2019; Slafer et al., 2023). This progress has been driven by rapid molecular developments, leading to the identification of key genes, and Quantitative Trait Loci (QTL) linked to grain weight and size in various crops (barley: Yang et al., 2023; rapeseed: Canales et al., 2021; rice: Zuo and Li, 2014; sorghum: Tao et al., 2021; sunflower: Castillo et al., 2018; wheat: Simmonds et al., 2014; Kumar et al., 2016; Simmonds et al., 2016; Adamski et al., 2021; Khan et al., 2022; Tillett et al., 2022; Mira et al., 2023). These studies assume that grain weight, which is a key yield component, could lead to the yield increase required to meet the challenge of food security. However, the relationship between increased grain weight and overall yield improvement is complex due to the reported trade-off between grain weight and grain number in wheat and other crops. In wheat, grain weight improvement has been addressed through various strategies, from classical breeding focusing on increasing grain weight through recurrent selection (Wiersma et al., 2001), to molecular breeding techniques such as mutating the wheat orthologue of rice Grain Weight 2 gene (TaGW2), a known negative regulator of grain size and weight (Song et al., 2007; Yang et al., 2012; Hong et al., 2014; Wang et al., 2018; Zhang et al., 2018). Additional methods include gene introgression, like TaGSNE (Khan et al., 2022), and overexpression of genes such as TaBG1 and TaCYP78A5 (Milner et al., 2021; Guo et al., 2022). Although most of these attempts successfully increased grain weight of wheat, they failed to improve grain yield due to the trade-off between grain weight and number (Wiersma et al., 2001; Okamoto and Takumi, 2013; Brinton et al., 2017; Milner et al., 2021; Mora-Ramirez et al., 2021).
The causes of the trade-off between grain weight and number are not fully understood, despite extensive research (Wiersma et al., 2001; Sadras, 2007; Dwivedi et al., 2021; Fischer, 2022; Slafer et al., 2023). Initial hypotheses and few subsequent studies suggested that growing grains of wheat are limited by the source of assimilates during grain filling (Sinclair and Jamieson, 2006; Golan et al., 2019; Rosati and Benincasa, 2023). However, most of the research has demonstrated that wheat grains are not, or scarcely, limited by assimilate supply during post-anthesis (Slafer and Savin, 1994; Borrás et al., 2004; Fischer, 2008; Serrago et al., 2013; Slafer et al., 2021; Murchie et al., 2023), except under extreme source restriction in high-yielding environments (Beed et al., 2007; Sandaña et al., 2009; Alonso et al., 2018). An alternative explanation involves the increased proportion of smaller distal grains when grain number is increased through breeding or crop management, since distal grains are intrinsically smaller than proximal ones (Acreche and Slafer, 2006; Ferrante et al., 2015, 2017). However, this does not account for situations where grain weight improvements lead to an actual trade-off with grain number (e.g., Wiersma et al., 2001; Brinton et al., 2017; Wang et al., 2018). Notably, interventions that successfully increased grain weight in both proximal and distal grain positions within the spike often resulted in a reduced grain number per spike and area (Wiersma et al., 2001; Okamoto and Takumi, 2013; Brinton et al., 2017; Quintero et al., 2018; Wang et al., 2018; Zhai et al., 2018; Adamski et al., 2021; Milner et al., 2021), confirming a genuine trade-off between yield components. Furthermore, recent genomic studies indicate that many regions associated with grain number and grain weight coincide and have inverse phenotypic effects, suggesting a strong genetic basis for this trade-off (Xie and Sparkes, 2021).
Trade-offs between grain number subcomponents have been documented in wheat. For instance, a higher plant number correlates with a lower number of spikes per plant (Slafer et al., 2021). It is generally accepted these trade-offs are due to the feedback between grain number components, whose settings overlap during the crop cycle (Slafer et al., 2021). For a long time, this explanation left aside the trade-off between grain weight and grain number, as these yield components were thought to have minimal overlap. From this perspective, the critical period for grain number determination accounts for 20 days before and 10 days after anthesis (DAA) (Fischer, 1985; Savin and Slafer, 1991; Abbate et al., 1997), whereas grain weight determination occurs during the grain filling period. However, several evidence now suggest that potential grain weight is established between booting and early grain filling in wheat (Calderini et al., 1999; Calderini and Reynolds, 2000; Ugarte et al., 2007; Hasan et al., 2011; Simmonds et al., 2016; Parent et al., 2017). This process is strongly influenced by maternal tissues, which impose a physical upper limit on grain weight in wheat (Millet and Pinthus, 1980; Calderini and Reynolds, 2000; Xie et al., 2015; Yu et al., 2015; Brinton et al., 2017; Reale et al., 2017; Brinton and Uauy, 2019; Calderini et al., 2021), a phenomenon also observed under increased temperature conditions (Calderini et al., 1999; Ugarte et al., 2007; Kino et al., 2020). The relevance of maternal tissues in determining grain size is also evident in other grain crops, for instance barley (Scott et al., 1983; Ugarte et al., 2007; Radchuk et al., 2011), sorghum (Yang et al., 2009) and sunflower (Lindström et al., 2006, 2007; Rondanini et al., 2009; Castillo et al., 2017). Additionally, the relationship between fruit size and flower ovary size has been reported in berries and fruit trees (Kiwifruit: Lai et al., 1990; Cruz-Castillo, 1992; olive: Rosati et al., 2009; peach: Scorzal et al., 1991; strawberry: Handley and Dill, 2003).
In wheat, various molecular strategies have been explored to increase grain weight. However, only a few have been successful in improving this trait (e.g. Hong et al., 2014; Simmonds et al., 2016; Brinton et al., 2017; Wang et al., 2018; Adamski et al., 2021; Jablonski et al., 2021; Milner et al., 2021; Mora-Ramirez et al., 2021), and even fewer have managed to increase grain weight without a trade-off with grain number (Calderini et al., 2021; Guo et al., 2022). Remarkably, one such improvement was achieved under field conditions at farmer’s plant density rate, where grain weight increased by 12.3% and grain yield by 11.3%, without affecting grain number (Calderini et al., 2021). Against this background, the present study aims to deepen the understanding of the trade-off between grain weight and grain number in wheat by evaluating lines with and without this trade-off. For this assessment, wheat lines previously tested under agronomic conditions were selected. We included the triple mutant line for the TaGW2 gene (Wang et al., 2018), which releases grain growth by breaking the negative control of TaGW2 over grain weight but demonstrates a trade-off with grain number. This gene codes for a RING-type E3 ligase, which mediates proteolysis through the ubiquitin-proteasome pathway (Song et al., 2007). In contrast, the selected genotype without a trade-off is a line with ectopic expression of the α-expansin gene TaExpA6, which is naturally expressed in wheat roots but was cloned with a promoter for expression in growing grains (Calderini et al., 2021). Expansins are small proteins crucial for plant cell growth, facilitating cell wall stress relaxation induced by turgor pressure (McQueen-Mason et al., 1992; Cosgrove, 2021; 2023). The expression of different expansins during wheat grain growth has been documented in wheat (Lin et al., 2005; Lizana et al., 2010; Kino et al., 2020; Xie and Sparkes, 2021; Mira et al., 2023). Both the triple mutant and transgenic lines were evaluated alongside their respective wild types (WT).
The ectopic expression of TaExpA6 and its protein in growing grains of wheat was evident from 10 DAA on (Calderini et al., 2021). This led the authors to propose that the trade-off between grain weight and grain number is potentially associated with the overlapping of the critical window for these two traits determinations. Our study aims to elucidate this trade-off by evaluating two genetically distinct wheat genotypes, known for increased grain weight but differing in the trade-off between both major yield components. These genotypes, along with their respective wild types, were examined under field conditions. By dissecting grain yield components, along with physiological and molecular characteristics at the spike level, the study seeks to minimize confounding variables and clarify the underlying mechanisms of the observed trade-off.
2 Materials and methods
2.1 Field conditions and experimental setup
Two field experiments were conducted on a Typic Hapludand soil at the Universidad Austral de Chile’s Experimental Station (EEAA) in Valdivia (39°47’S, 73°14’W). The first experiment spanned the 2021–2022 growing season (referred to as Exp. 1), while the second was conducted in the 2022–2023 season (Exp. 2). To fulfil the proposed objective, four spring wheat cultivars were selected for both experiments: (i) TaExpA6, a transgenic line expressing the expansin gene TaExpA6 ectopically in grains, as described by Calderini et al. (2021); (ii) its segregant wild type cv. Fielder; (iii) TaGW2, a triple knock-out mutant of TaGW2 gene (referred to as “aabbdd” in Wang et al., 2018); and (iv) its segregant wild type cv. Paragon. These genotypes were chosen due to their contrasting effects on the trade-off between grain weight (GW) and grain number (GN). Specifically, while line TaGW2 exhibits reduced GN, line TaExpA6 improves GW without impacting GN.
The TaExpA6 line features overexpression of TaExpA6 (REFSEQ v.1.1: TraesCS4A02G034200) in the endosperm, aleurone, and pericarp tissues of developing grains. This overexpression is controlled by the wheat puroindoline-b (PinB) gene promoter (REFSEQ v.1.1: TraesCS7B02G431200), as detailed by Gautier et al. (1994) and Digeon et al. (1999). The TaExpA6 line and its segregant WT were developed by Dr. Emma Wallington from the National Institute of Agricultural Botany (NIAB), UK (Calderini et al., 2021). In contrast, the TaGW2 gene in line TaGW2 harbors mutations leading to a truncated, non-functional protein, as reported by Simmonds et al. (2016) and Wang et al. (2018). An in depth description of the mutated alleles can be found in Wang et al. (2018). These GW2 lines were generously provided by Prof. Cristóbal Uauy from John Innes Center, UK.
Exp. 1 was sown on September 21, 2021. Due to phenological differences observed between both groups of lines in Exp. 1, sowing dates in Exp. 2 were modified. Thus, GW2 lines, with longer crop cycles, were sown earlier on August 20, 2022, while the shorter-cycle ExpA6 lines were sown later on September 2, 2022. Both experiments followed a randomized complete block design with four replications. Each plot measured 2 m in length and 1.2 m in width, consisting of 9 rows with 0.15 m spacing, and was sown at a density of 300 plants per square meter.
Optimal agronomic management was employed for all plots to prevent biotic and abiotic stress. Fertilization at sowing included 150 kg N ha-1, 150 kg P2O5 ha-1, and 100 kg K2O ha-1. An additional 150 kg N ha-1 was applied at tillering. To address potential aluminum toxicity brought about by low soil pH, the experimental site was treated with 4 Tn ha-1 of CaCO3 one month prior to sowing. Pests and diseases were managed using chemical treatments as per manufacturer recommendations. Drip irrigation supplemented rainfall to avoid water stress throughout the crop cycle.
Meteorological data, including air temperature and incident photosynthetically active radiation (PAR), were recorded daily from sowing until harvest at the Austral Meteorological Station of EEAA (http://agromet.inia.cl/), located approximately 150 m from the experimental plots. Photothermal quotient was calculated as the ratio of mean daily incident radiation to mean temperature above 4.5°C, in line with Fischer (1985).
2.2 Crop sampling and measurements
Crop phenology was recorded twice weekly according to the decimal code scale (Zadoks et al., 1974). At harvest, 45 spikes of main stems were sampled along 1m from the central row of each plot in both experiments. Grain yield per spike, grain number per spike and average grain weight were measured or calculated as previously (Bustos et al., 2013; Quintero et al., 2018). In addition, 10 more main shoot spikes of similar development and size were sampled from each plot to quantify grain weight and dimensions (length and width) at each grain position from every spikelet of the spike. From each spike, half the spikelets (i.e. all the spikelets along one longitudinal side of the spike) were measured, considering the spike symmetry. Grains from positions G1 to G4 (G1 being the closest grain to the rachis and G4 the most distal, if present) within each spikelet were taken out, oven dried (48 h at 65°C) and weighted separately using an electronic balance (Mettler Toledo, XP205DR, Greifensee, Switzerland). The length and width of each grain was recorded using a Marvin Seed Analyzer (Marvitech GmbH, Wittenburg, Germany). Grain number per spike and per spikelet were also recorded.
In Exp. 2, the weight of ovaries from florets at positions G1 to G4 was measured at pollination (stage 10 in Waddington et al., 1983) by sampling 20 ovaries of each floret position from the four central spikelets of five spikes per plot. In this experiment the time-course of grain weight and dimensions were also measured. From anthesis onwards, four main shoot spikes were sampled from each experimental unit twice weekly until physiological maturity to record grain fresh and dry weight and dimensions (i.e. length and width) of six individual grains corresponding to a proximal (G2) and a distal (G3) grain position of two central spikelets. The fresh weight and the length and width of grains were recorded immediately after sampling as described above. Dry weight of grains was measured with the same electronic balance, after drying the samples at 65°C in an oven for 48 h.
In both experiments, grain quality was assessed by measuring grain protein concentration to determine the impact of GW changes on this quality trait. Accordingly, grains from positions G1 to G3 within the four central spikelets of the 10 main shoot spikes samples were bulked at each experimental unit and then milled using a Perten 120 laboratory mill (Huddinge, Sweden). The quantification of total nitrogen was executed using the Kjeldahl method. Protein content was then calculated by multiplying the total nitrogen value by a factor of 5.7, in accordance with the approach of Merrill and Watt (1973) as applied in Lizana and Calderini (2013).
2.3 Time-course expression analyses by reverse transcription quantitative PCR
To elucidate the relationship between grain growth dynamics and gene expression, we conducted time-course expression analyses of PinB::TaExpA6 in the ExpA6 lines and TaGW2 in the GW2 lines. These analyses were performed using reverse transcription quantitative PCR (RT-qPCR) on grains at positions G1 and G2. Specifically, G1 and G2 grains from central spikelets on the main stem spikes were collected from a minimum of eight spikes at 4, 7, 10, 14, and 21 days after anthesis (DAA) for each experimental plot.
Immediately following collection, samples were secured in cryotubes, snap-frozen in liquid nitrogen, and subsequently preserved at -80°C until further processing. Total RNA was isolated using NucleoSpin™ columns (Macherey-Nagel), employing a standardized protocol adapted from Sangha et al. (2010). RNA samples were then subjected to DNaseI (Invitrogen) treatment, and cDNA synthesis was performed using the ImProm-II™ Reverse Transcription System with an input of 500 ng RNA per reaction.
Quantitative PCR (qPCR) was carried out in a 25 μL reaction volume using the Brilliant II SYBR Green PCR Master Mix (Stratagene, Agilent technologies). Primer concentrations were set at 0.2 μM. For TaExpA6, the primers TransgeneTaExpa6_F1 (5’- ATCTCCACCACCACCAAAACA-3’) and TransgeneTaExpa6_R1 (5’-GAAGCAGAACGCGAGAACGG-3’) were used. In the case of TaGW2, genome-specific primers for its homoeologues, as described by Wang et al. (2018), were utilized. Controls without template and transcriptase were included to check for genomic DNA contamination.
The relative mRNA abundance of the target genes in grain tissues was determined using the comparative CT (ΔΔCT) method, as proposed by Pfaffl (2001). The ubiquitin conjugating enzyme (Traes_4AL_8CEA69D2E.1) served as the internal reference gene, amplified with primers Traes_4AL_8CE_esF (5’- CGGGCCCGAAGAGAGTCT-3’) and Traes_4AL_8CE_esR (5’-ATTAACGAAACCAATCGACGGA-3’). Data analysis for gene expression quantification was conducted using LinRegPCR software (Ruijter et al., 2009).
2.4 Statistical analysis
Analysis of variance (ANOVA) was applied to evaluate the effect of genotype on main shoot grain yield and associated traits, by using Statgraphics Centurion 18 software. Fisher’s least significant difference test post hoc and/or Student´s t-test were employed to identify each significant difference within the evaluated group of lines. Additionally, a Two-way ANOVA analysis was performed to assess significant differences in TaExpA6 gene expression between line TaExpA6 and its respective WT, whereas one-way ANOVA analysis was performed to assess significant differences in TaGW2 gene expression in the WT across sampling times using GraphPad Prism 8 software. Linear regression analysis was performed to assess the associations between measured grain traits. A tri-linear model was fitted to estimate the rate and duration of the lag and linear phases of grain filling, and final grain weight in the individual seed weight dynamics.
Extra sum of squares F test was used for the comparison of the slopes and timings between each modified line and its respective WT. To model the dynamics of individual grain water content during the grain filling period, a second order polynomial model was employed. All model fittings were performed using GraphPad Prism 8 software.
3 Results
3.1 Environmental conditions and crop phenology across seasons
Environmental conditions from seedling emergence to physiological maturity were consistent between experiments (Table 1). Temperature variations between Exps. 1 and 2 were minimal, with differences of less than 1°C observed during the emergence-anthesis and grain filling periods. Incident PAR differed between the experiments, being 2.3 MJ m-2 d-1 higher in Exp. 1 than in Exp. 2 during the Emergence-Booting (Em-Bo) and grain filling phases (10.4 vs. 8.1 MJ m-2 d-1 and 12.8 vs. 10.5 MJ m-2 d-1, respectively). However, no significant difference in PAR was noted during the Booting-Anthesis (Bo-An) period. Despite these variations, the photothermal quotient (Q) remained constant at 2.5 MJ m-2 d-1°C-1 across the entire crop cycle in both seasons. A comparative analysis of the ExpA6 and GW2 genotype lines revealed similar weather exposure in both experiments, except for the Bo-An period in Exp. 1, where GW2 lines experienced a mean temperature 2.2°C higher than ExpA6 lines, leading to a 17% lower photothermal quotient for GW2 lines during this phase.
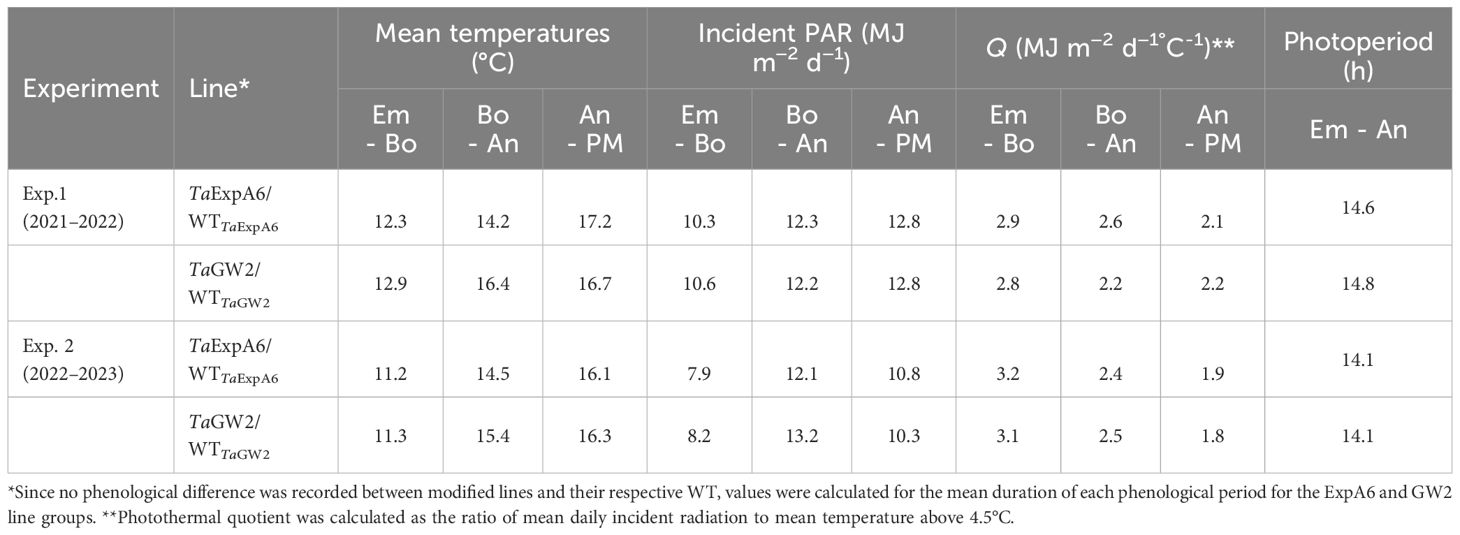
Table 1 Average mean temperature, incident photosynthetically active radiation (PAR), photothermal quotient (Q) and photoperiod during the Emergence-Booting (Em-Bo), Booting-Anthesis (Bo-An) and Anthesis-Physiological Maturity (An-PM) periods in experiments 1 and 2.
The length of the crop cycle averaged 123 days for ExpA6 lines and 142 days for GW2 lines across experiments (Figure 1). In experiments 1 and 2, GW2 lines reached physiological maturity 13 and 26 days later, respectively, than ExpA6 lines. This delay is primarily attributed to the longer Emergence-Booting (Em-Bo) period in GW2 lines, as they exhibited a slower development rate. Post-booting, the differences in phenological stages, specifically Booting-Anthesis (Bo-An) and Anthesis-Physiological Maturity (An-PM), were negligible (i.e. less than 2 days) between the line groups. Despite minor climatic differences, the crop cycle in Exp. 2 extended by 16 and 27 days for ExpA6 and GW2 lines, respectively, compared to Exp. 1. This extension corresponds to an accumulated difference of 84 and 180°Cd for each line group (Supplementary Figure S1). The extended crop cycle in GW2 lines, particularly in Exp. 2, is likely due to their heightened sensitivity to photoperiod compared to ExpA6 lines (see photoperiods in Table 1).
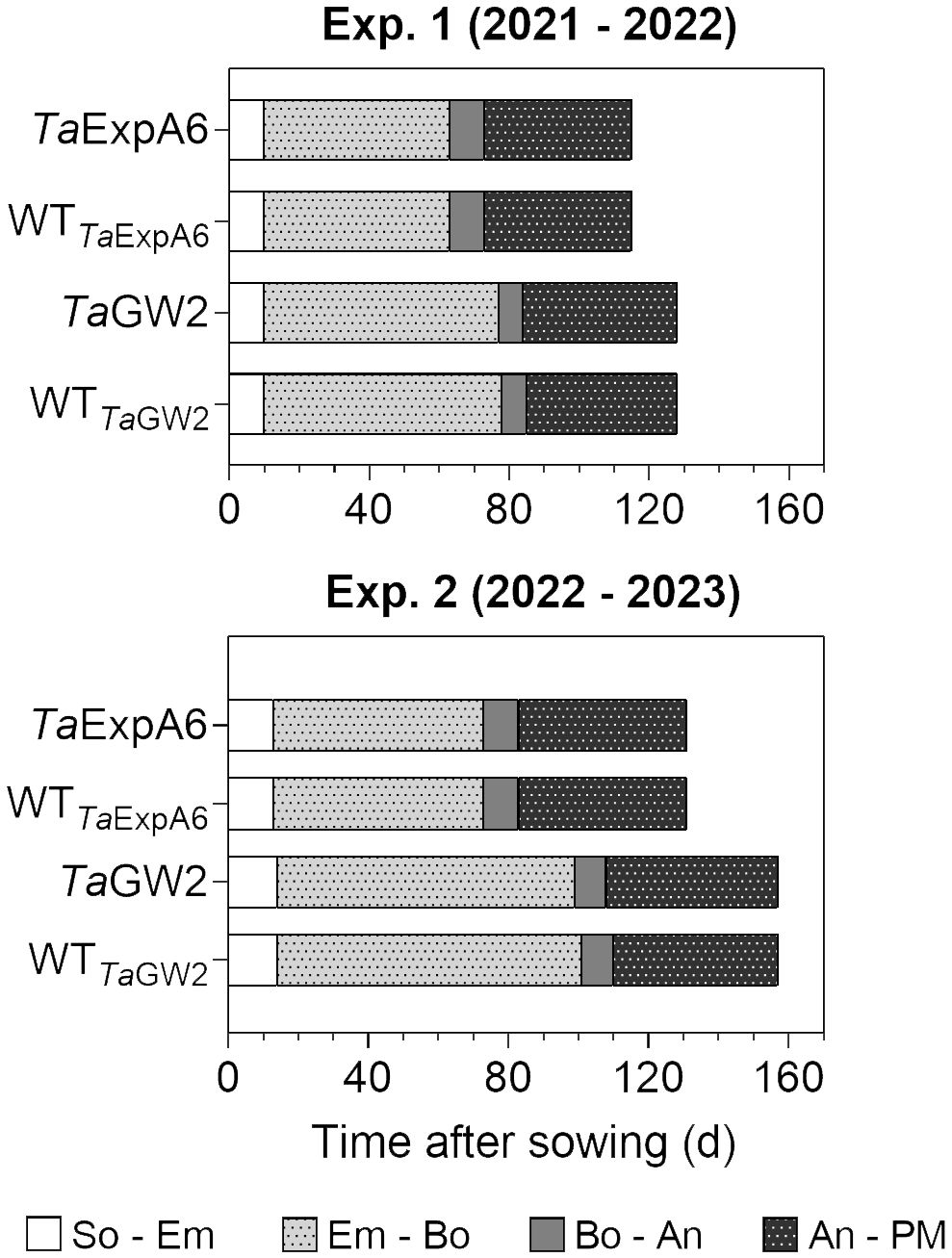
Figure 1 Phenological phases of TaExpA6 and TaGW2 lines, and their respective wild types (WT), from sowing to physiological maturity in experiments 1 and 2. Bars show the duration of each phase in days: from sowing to seedling emergence (So-Em), from seedling emergence to booting (Em-Bo), from booting to anthesis (Bo-An) and from anthesis to physiological maturity (An-PM).
3.2 Grain yield per spike, yield components per spike and quality trait
Differences in grain yield per spike were observed between line groups (P < 0.05), with GW2 lines exhibiting higher yields compared to ExpA6 lines in both experimental seasons, averaging 2.39 g and 1.94 g, respectively (Table 2). Notably, the ectopic expression of TaExpA6 gene resulted in a significant increase in spike yield compared to the wild type (WT), showing increments of 14.0% and 8.2% in Exps. 1 and 2, respectively (P < 0.05). In contrast, the triple mutant of TaGW2 and its WT displayed similar spike yields in both experiments (P < 0.05) (Table 2).
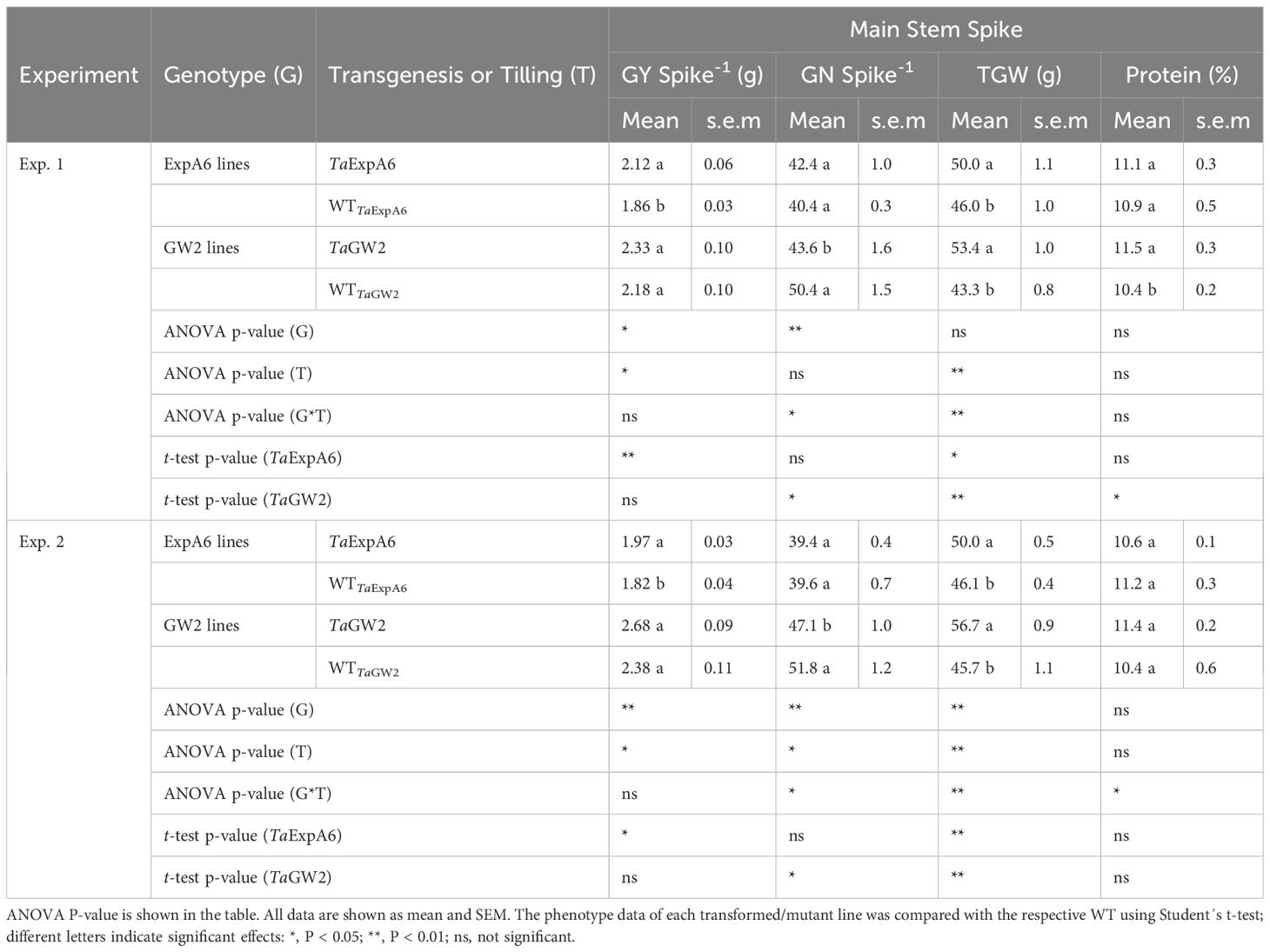
Table 2 Grain yield per spike (GY Spike-1), grain number per spike (GN Spike-1), average grain weight (TGW) and protein concentration (%) of grains recorded in TaExpA6 and TaGW2 lines and their WTs in the field experiments 1 and 2.
Regarding grain yield components, genotype significantly influenced these traits, contingent on the line group (P < 0.05). Average grain weight (TGW) was similar between both groups in Exp. 1 (P > 0.05) but was marginally higher in the GW2 lines (6.6% increase, P < 0.01) in Exp. 2, with weights of 51.2 g and 48.1 g for GW2 and ExpA6 line groups, respectively (Table 2). Both TaExpA6 and TaGW2 lines surpassed the grain weight of their respective WTs in both experiments (P < 0.001). Averaging across Exps. 1 and 2, TaExpA6 and TaGW2 lines exhibited increases in TGW of 8.6% and 23.7% above their WTs, respectively (Table 2). Conversely, contrasting results were found for the grain number per spike; the TaExpA6 line maintained a similar number to its WT (P > 0.05), whereas the TaGW2 line exhibited a reduction in grain number compared to its WT in both experiments, with declines of 13.5% and 9.1% in Exps. 1 and 2, respectively (Table 2; Supplementary Figure S2). These findings align with previous reports indicating no trade-off in the TaExpA6 overexpressed line and a detrimental effect of the TaGW2 loss-of-function triple mutant on grain number.
In addition, we assessed a critical wheat quality trait, such as grain protein concentration. For this analysis, grains from G1 to G3 positions of the four central spikelets were pooled from each experimental unit. The results indicated no significant impact of transgenesis or tilling on grain protein concentration (P > 0.05), although an interaction (P < 0.05) between these factors was observed (Table 2). Grains from plants with the TaExpA6 gene exhibited similar protein concentrations to the WT, highlighting the relevance of the TaExpA6 construct. Conversely, the TaGW2 triple mutant either increased (by 1.1 percentage points) or did not affect grain protein concentration in Experiments 1 and 2, respectively. Furthermore, no correlation (R2 = 0.05; P > 0.05) was observed between grain protein concentration and grain yield per spike across genotypes and experiments, suggesting that the TaGW2 triple mutation improved this quality trait independently from a dilution-concentration effect.
3.3 Individual grain weight and dimensions along the spike
To have a deeper understanding of the impact of TaExpA6 overexpression and the TaGW2 triple mutation, we dissected the spike evaluating grain weight, number and dimensions at each grain position along the spike (usually referred to as spike map) in both experiments. Our analysis revealed that across spikelets and grain positions, TaExpA6 overexpression resulted in increases in grain weight compared to its wild type (WT) in both experiments. Specifically, grain weight enhancement in the TaExpA6 line was observed as follows: in Exp.1, grains at positions G1, G2, G3, and G4 showed increases of 9%, 8%, 11%, and 25%, respectively, and in Exp. 2, increases of 9%, 6%, 10%, and 10% for G1, G2, G3, and G4, respectively (see Figure 2). In comparison, the TaGW2 triple mutant exhibited a more pronounced increase in grain weight above the WT across all grain positions in both experiments: in Exp. 1, increases of 19%, 21%, 30%, and 37% for G1, G2, G3, and G4, respectively, and in Exp. 2, increases of 23%, 23%, 18%, and 19% for the same positions (Figure 2). On the other hand, when averaging across experiments, the ExpA6 lines showed similar grain number per spikelet across the spike (i.e. -1%), while the triple mutation of TaGW2 gene caused a reduction of 11% in this trait in regard to its WT (Supplementary Figure S2).
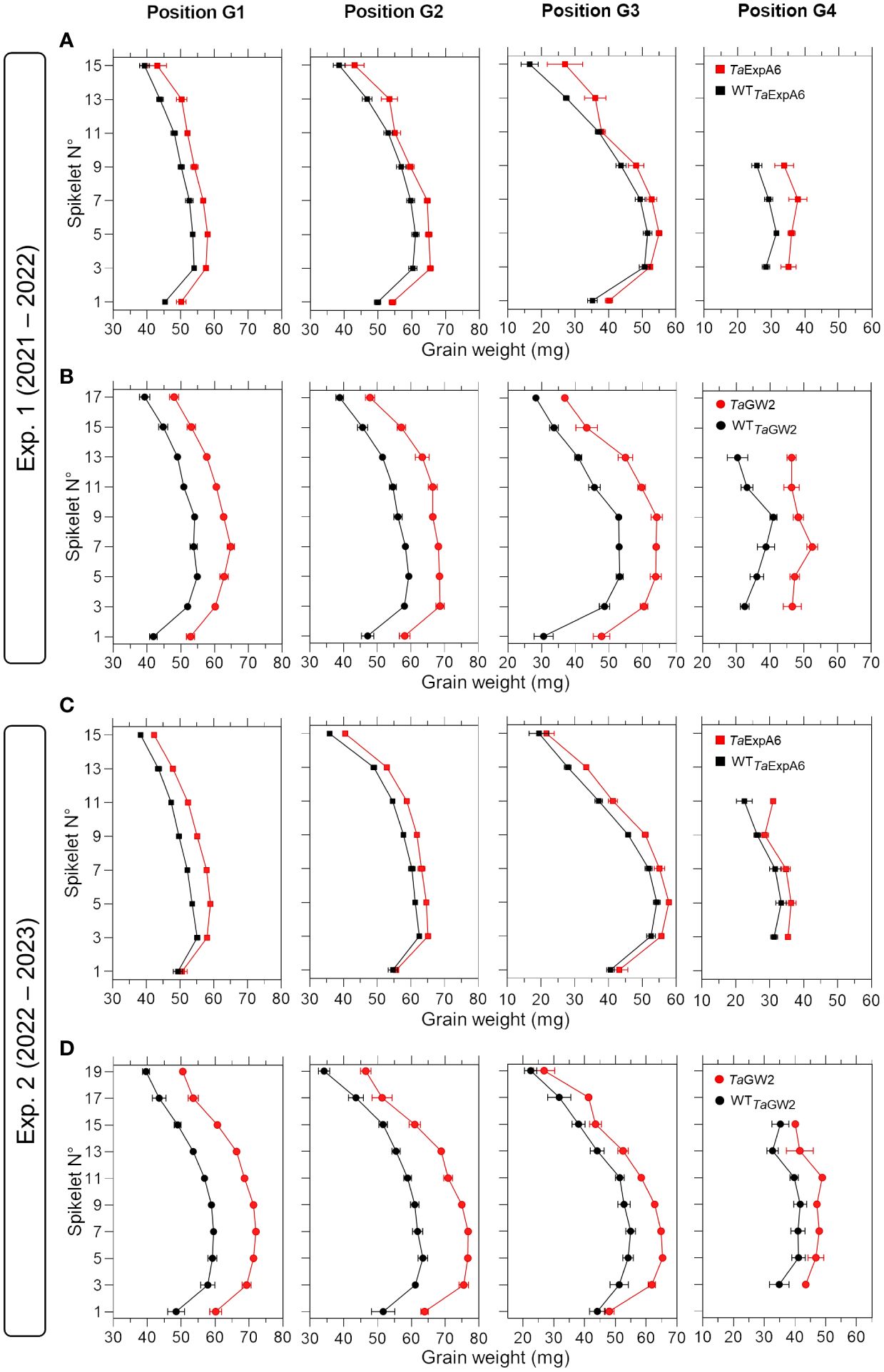
Figure 2 Grain weight in grain positions G1, G2, G3 and G4 from each spikelet along the spike of ExpA6 lines (A, C) and GW2 lines (B, D) in experiments 1 and 2. The transgenic TaExpA6 line and the triple mutant of TaGW2 gene, and their WTs are depicted by red and black symbols, respectively.
Subsequent analysis focused on the relationship between grain dimensions and final grain weight. A strong association (P < 0.001) was observed between grain weight and both grain length and width, with determination coefficients ranging from 0.92 to 0.96 (Figure 3). This relationship was consistent within each genotype group (Supplementary Figure S3).
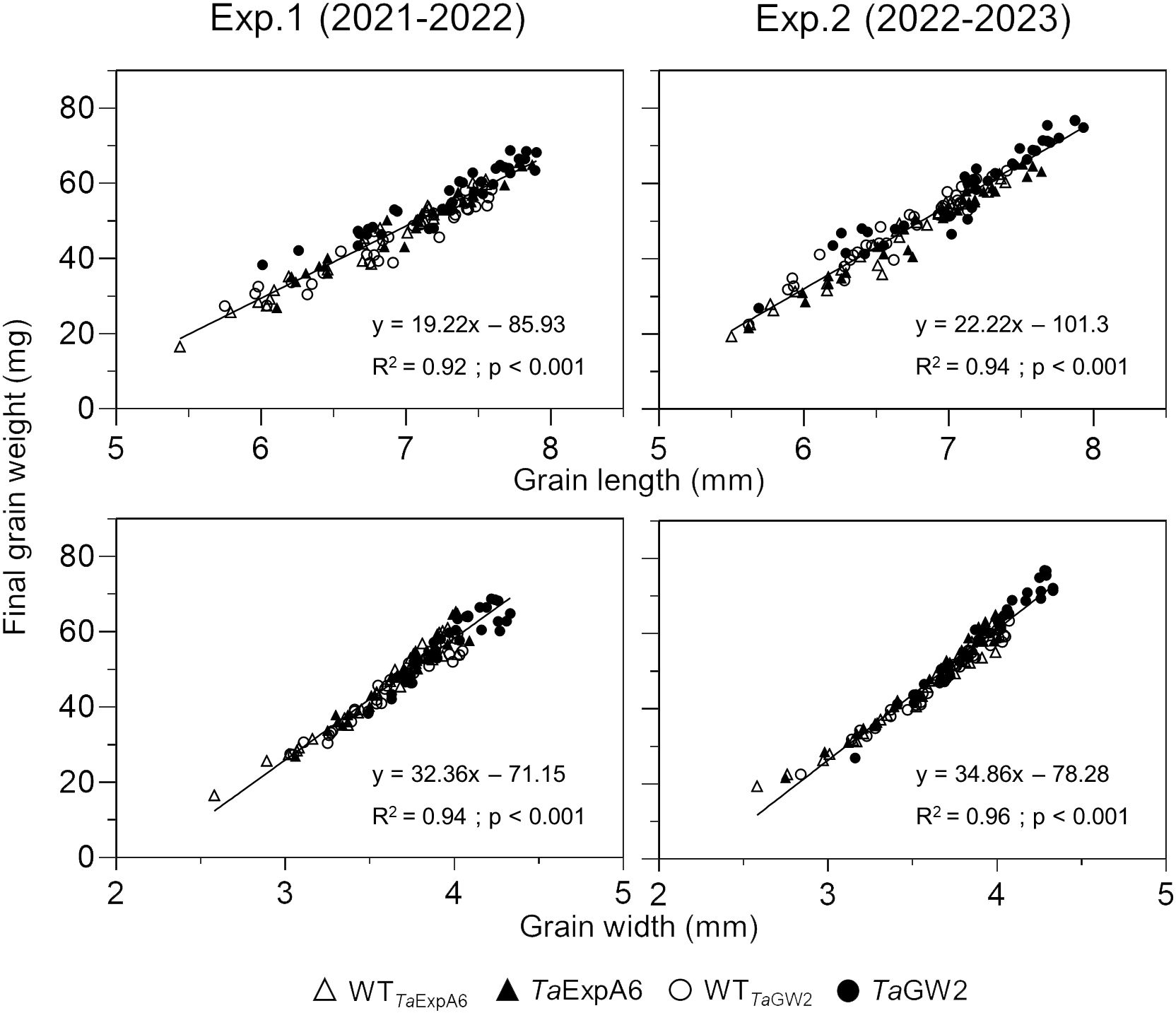
Figure 3 Relationship between grain weight and grain length (upper panel) or grain width (lower panel) of grain positions G1, G2, G3 and G4 from each spikelet along the spike, across genotype groups in experiments 1 (left panel) and 2 (right panel). TaExpA6 line and its WT are denoted by closed and open triangles respectively, while the TaGW2 triple mutant line and its WT are denoted by closed and open circles, respectively.
3.4 Ovary weight, grain weight dynamics and gene expression
In Exp. 2, we assessed ovary weight at pollination (stage 10 according to Waddington et al., 1983) in florets at positions F1, F2, F3 and F4 from the central spikelets of spikes in each evaluated line. A linear association between final grain weight and ovary weight was found across lines and grain positions (Figure 4). In agreement with this association, GW2 lines exhibited increased ovary weight compared to ExpA6 genotypes (Figures 4, 5). However, contrasting results were found between both groups of lines, as the TaGW2 triple mutant showed a significant increase (P < 0.05) in ovary weight compared to its WT in all but the G3 floret position, whereas the TaExpA6 construct showed no significant (P > 0.05) alteration in ovary weight at pollination, mirroring its WT (Figure 5). This suggests that TaGW2 gene mutation impacts ovary size prior to anthesis.
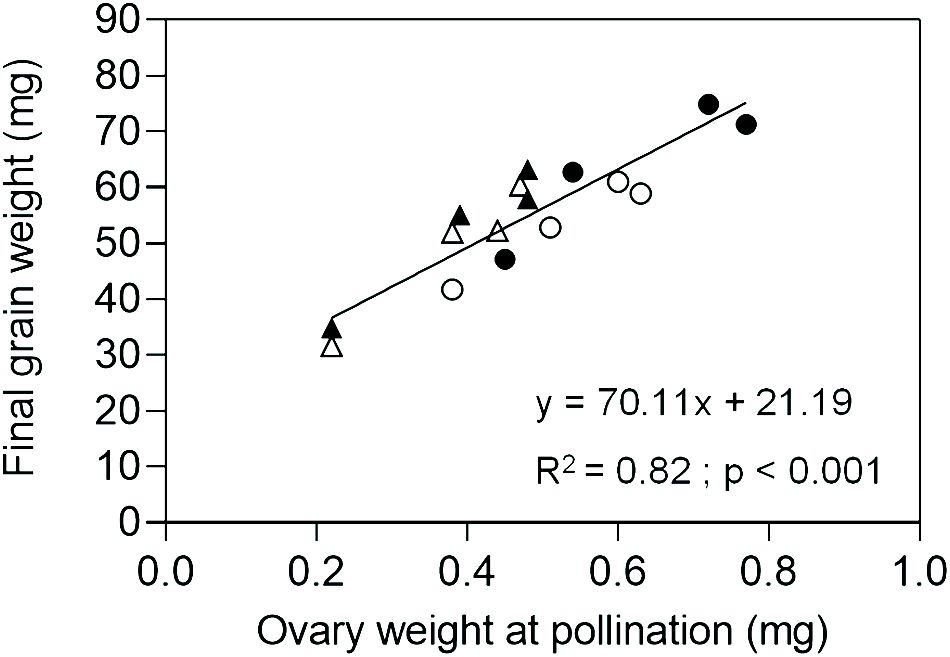
Figure 4 Relationship between final grain weight and ovary weight at pollination (W10, Waddington et al., 1983) of grain positions G1, G2, G3 and G4 from the central spikelets of the spike corresponding to the TaExpA6 line (closed triangles), TaGW2 line (closed circles) and their WTs (open triangles and open circles, respectively).
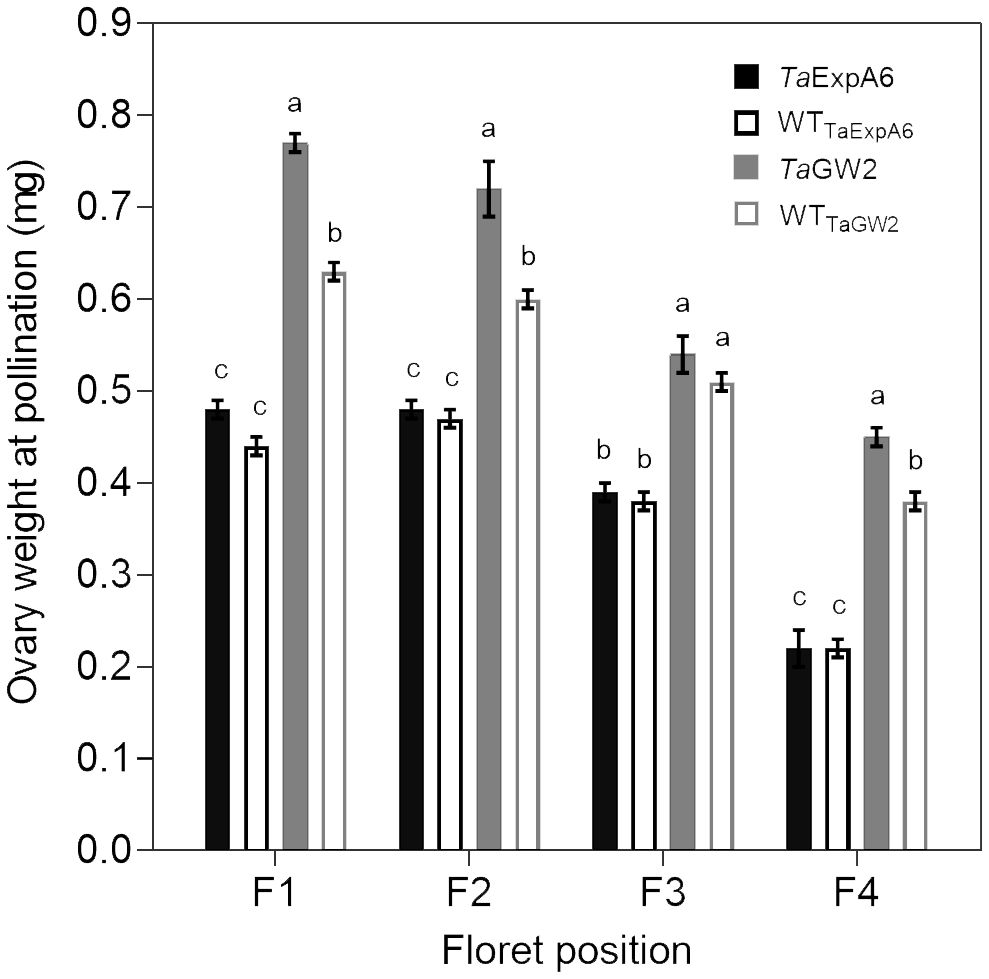
Figure 5 Ovary weight at pollination (W10, Waddington et al., 1983) of florets set at floret positions F1, F2, F3 and F4 from the central spikelets of the spike corresponding to the TaExpA6 line (solid black bars), TaGW2 triple mutant line (solid grey bars) and their WTs (empty black and grey bars, respectively). Different letters indicate significant effects (P < 0.05).
When the time-course of grain weight from positions G2 and G3 was monitored through the grain filling period, both modified lines (TaExpA6 and TaGW2) surpassed their respective WTs in grain weight, though, the onset of these differences varied between groups. The GW2 triple mutant showed higher grain weight than the WT from the starting of measurements at 4 DAA (Figure 6D; Supplementary Figure S4), while the TaExpA6 line exhibited higher grain weights at these grain positions from 20 DAA on (Figure 6A; Supplementary Figure S4). For both line groups, a tri-linear function accurately depicted individual grain weight dynamics (Supplementary Figure S5), with enhanced grain filling rates at the linear phase accounting for the increased final grain weights in both G2 and G3 grains (R2 = 0.99; P < 0.05). No significant difference was observed in the duration of grain filling, which was approximately 40 days (Supplementary Table S1). Higher grain weights in modified lines were also coupled with an increased maximum grain water content (Figures 6A, D; Supplementary Figure S4).
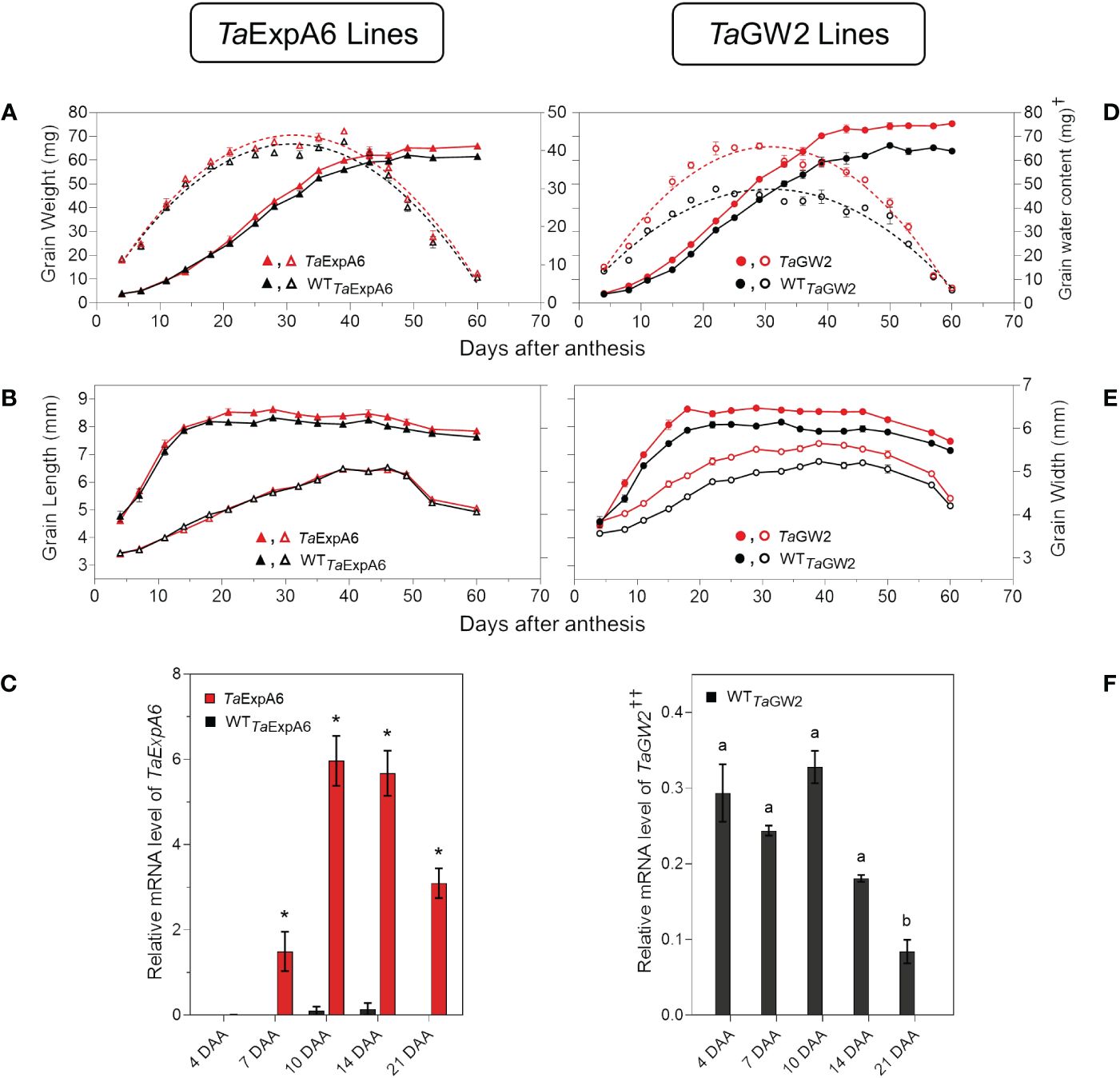
Figure 6 Grain weight dynamics and gene expression. (A) Grain weight and water content dynamics at grain position G2 from the central spikelets of the spike corresponding to the ExpA6 lines and (D) GW2 lines. (B) Grain length and grain width dynamics at the same grain position corresponding to the ExpA6 lines and (E) GW2 lines. (C) Relative TaExpA6 gene expression level in developing grains in the ExpA6 lines; asterisks indicate significant differences (adjusted p-value < 0.05) between line TaExpA6 and its WT according to two-way ANOVA Bonferroni´s test post hoc. (F) Relative TaGW2 gene expression level in developing grains of the GW2 WT line; different letters indicate significant effect (adjusted p-value < 0.05) between developmental times according to Bonferroni´s test post-hoc. In all cases, bars show the standard error of the means. †Note that different scales were used to plot grain water content of ExpA6 and GW2 lines. ††Data shows the average value of TaGW2-A, B and D homoeologue’s expression..
Grain dimension dynamics also varied between the line groups. The TaExpA6 line increased grain length by 3% and 4% in grain positions G2 and G3, respectively, over the WT (P < 0.05) without affecting grain width (Figure 6B; Supplementary Figure S4). Conversely, the GW2 triple mutant improved both grain length and width along grain filling (P < 0.05) by 6.2 and 6.7%, respectively, when both grain positions were averaged (Figure 6E; Supplementary Figure S4). Notably, differences in grain width in the ExpA6 lines became evident only at the ending of dimension dynamics analysis, coinciding with reduced grain water content (< 30%) (Figure 6B; Supplementary Figure S4).
The observed divergence in grain length between the transgenic TaExpA6 line and its WT counterpart became apparent at 20 DAA. This divergence appears to coincide with the expression profile of the TaExpA6 gene. Remarkably, TaExpA6 expression remains undetectable until 5 DAA, subsequently peaking between 10 and 15 DAA (see Figure 6C). Two-way ANOVA revealed highly significant genotype effects for TaExpA6 (p < 0.0001), with Bonferroni’s multiple comparisons test showing consistent and significant changes in mRNA levels at 10, 14, and 21 DAA (adjusted p-value < 0.05, Figure 6E). In contrast, the grain dimensions in the GW2 triple mutant consistently exceeded those of the WT throughout the observation period (Figure 6E), suggesting a constitutive effect of the TaGW2 knockout on grain size. Furthermore, TaGW2 expression was monitored from 4 to 21 DAA, as depicted in Figure 6F. One-way ANOVA revealed a significant effect of developmental stage on TaGW2 expression in the wild-type (p-value: 0.0005). Bonferroni’s test showed significantly lower TaGW2 levels at 21 DAA compared to 4 DAA (adjusted p-value < 0.05, Figure 6F), suggesting a potential role in earlier stages of grain growth.
4 Discussion
This study aimed to elucidate the mechanisms underlying the trade-off between grain weight and grain number in wheat, when grain weight is improved by genetic manipulations. To realize this objective, field evaluations of two genetically distinct wheat line groups, ExpA6 and GW2, were conducted under optimal conditions. The GW2 lines had 20 days longer crop cycle than the ExpA6 lines, but the climatic conditions during critical phenophases between both groups were similar across the two experimental years. As hypothesized, the transgenic and triple mutant lines displayed analogous phenology with their respective wild types, as well as plant height and architecture (data not shown).
Significant increments in grain weight were observed in the manipulated lines over the WTs, although differing in magnitude, i.e. by 8.6 and 23.7% in the TaExpA6 and TaGW2 lines, respectively, without and with trade-off with grain number. These results agree with previous evaluations of the TaExpA6 and TaGW2 lines (Wang et al., 2018; Zhai et al., 2018; Calderini et al., 2021), however, a direct comparison between both groups was feasible in our study as they were assessed in the same experiment sharing the same growing conditions and management. Notably, our study expands on previous work by demonstrating that both manipulations improved individual grain weight and grain dimensions across all grain positions of the spike, a feature not fully addressed in earlier research.
Comparative analyses between genetic resources and elite wheat varieties by Philipp et al. (2018) revealed that breeding process in wheat uniformly increased grain number and yield across the spike without altering individual spikelets relative contribution to overall yield. The observed increase in individual grain weight in our study aligns with these breeding trends, suggesting a similar pattern when grain weight is genetically improved. However, manipulation of TaExpA6 showed a higher impact on distal grains (G3 and G4) than on proximal ones (G1 and G2), while the TaGW2 triple mutant line showed similar increase across these grain positions.
Both genetic manipulations in our study successfully increased individual grain weight through an enhanced grain filling rate, maintaining the same grain filling duration as their respective WTs. Additionally, both modified lines reached higher maximum grain water content than their respective WTs, which has been ascribed as a driver of grain weight potential (Saini and Westgate, 1999; Pepler et al., 2006; Hasan et al., 2011; Alvarez Prado et al., 2013), suggesting increased sink strength which sequentially resulted in a higher grain filling rate and final grain weight. However, the impact of TaExpA6 and TaGW2 manipulations on grain dimensions varied, since the transgenic approach primarily augmented grain length, while the triple mutation affected both length and width. This differential impact suggests distinct underlying mechanisms between manipulations. In the TaExpA6 line, the cloned expansin possibly facilitates cell wall loosening along the grain longitudinal axis, consistent with expansins extensively reported mode of action (McQueen-Mason et al., 1992; Cosgrove, 2000; Wang et al., 2019; Cosgrove, 2023). Additionally, the influence of the TaExpA6 gene would seem more related to cell size than number, considering the cessation of cell proliferation in grains outer layers and starchy endosperm by 6 and 14 DAA, respectively (Olsen et al., 1992; Drea et al., 2005; Radchuk et al., 2011), and the linear relationship found between the length of grains and epidermal cells length during grain filling across wheat ploidies (Muñoz and Calderini, 2015). However, the mechanisms of the overexpression of the gene TaExpA6 remains to be assessed. Conversely, the higher GW obtained in previous studies by manipulation of the TaGW2 gene has been ascribed to increases in both the cell number and size of maternal tissues around anthesis, leading to longer and wider grains (Simmonds et al., 2016; Geng et al., 2017; Zhang et al., 2018). Increases in both grain dimensions in our study are in alignment with these findings. In either case, further histological analysis of grains from ExpA6 and GW2 lines is required to confirm these assumptions. The significance of cell number and size in the outer tissues for potential grain weight previously exposed in bread wheat and across wheat ploidies (Lizana et al., 2010; Muñoz and Calderini, 2015; Brinton et al., 2017; Brinton and Uauy, 2019; Liu et al., 2020; Guo et al., 2022; Tillett et al., 2022), together with the findings from this study, support the hypothesis that potential grain weight is constrained by physical limitations imposed by maternal outer layers.
The effect of the manipulated lines on grain number per spike was contrasting as expected. The TaExpA6 gene did not affect this yield component, whereas the TaGW2 triple mutation reduced it by 11.3% relative to the WT, across both seasons. This trade-off between grain weight and grain number when grain weight is increased has been widely reported (Wiersma et al., 2001; Brinton et al., 2017; Quintero et al., 2018; Wang et al., 2018; Golan et al., 2019; Milner et al., 2021). In previous studies, modified lines with GW increases comparable to the one observed in line TaExpA6, (i.e. between 5.5% and 8%) have also been associated with concomitant reductions in GN (Brinton et al., 2017; Adamski et al., 2021; Mora-Ramirez et al., 2021). Therefore, the contrasting trade-off observed between both genotype groups in this study would not be attributed to the quantitative difference in the GW increase induced by the molecular manipulation in modified lines. Notably, the magnitude of average grain weight increase did not directly correlate with grain number reduction, refuting the notion of a strict negative compensation between these spike yield components. These results align with the consensus that wheat grain filling is not source-limited (Slafer and Savin, 1994; Borrás et al., 2004; Reynolds et al., 2009; Quintero et al., 2018; Murchie et al., 2023; Slafer et al., 2023). However, an up-regulation of the source as a consequence of improved sink strength displayed by manipulation of TaExpA6 and TaGW2 genes should not be disregarded. In fact, previous studies have demonstrated that a high sink to source ratio can lead to an increased post-anthesis radiation use efficiency (RUE) (Bustos et al., 2013) or extended green canopy duration during grain filling (Lichthardt et al., 2020). Interestingly, the lack of effect on grain number showed by the ectopic expression of the TaExpA6 gene is consistent with two previous experiments at different plant rates (Calderini et al., 2021). The TaExpA6 and TaGW2 genes expression profile along with grain weight dynamics of the transgenic and mutant lines, allowed us to highlight a distinctive pattern in the effect of these manipulations on early grain development. The disruption of TaGW2 gene in the triple mutant line improved grain weight above the WT from the onset of the grain filling period. In contrast, the higher grain weight reached by the TaExpA6 transgenic line became apparent at 21 DAA. Furthermore, the triple mutant exhibited heavier ovaries at pollination than the WT across different positions in the spikelet, indicating that the TaGW2 gene functions in floret tissues before the gynoecium becomes a grain. This finding is in agreement with field experiments carried out by Simmonds et al. (2016), who identified increased carpel size and weight around anthesis as drivers of grain weight increase in TaGW2 knockout mutants. In contrast, ovary weight at pollination did not differ significantly between TaExpA6 line and its WT in the present study, reinforcing the post-anthesis timing of the transgene effect as previously reported (Calderini et al., 2021).
It has been demonstrated that grain number and weight determinations overlap between booting and a week after anthesis (Calderini et al., 1999, 2001; Ugarte et al., 2007; Hasan et al., 2011; Simmonds et al., 2014; Parent et al., 2017; Calderini et al., 2021). This overlap was proposed as the cause of the trade-off between this key yield components in wheat (Calderini et al., 2021). The effectiveness of the TaExpA6 gene in increasing grain weight without a trade-off with grain number supports this hypothesis, given that TaExpA6 expression begins at post-anthesis, particularly from 10 DAA onwards, and its effect on grain length and weight over the WT becomes detectable after 15 DAA. Conversely, when the enhancement commences at pre-anthesis as in TaGW2 triple mutant, showed by both increased ovary size and grain weight, a trade-off occurs. Thus, our results suggest that the trade-off between grain weight and number is attributable to the temporal overlap of determinant periods for these grain components. Previous research found that the likelihood of grain setting in distal florets of wheat associates with ovary size (Calderini and Reynolds, 2000; Guo et al., 2016). However, contrary to our assumption, the higher ovary weight in floret position G4 observed in line TaGW2 was accompanied by a reduction of the number of grains set at this distal position across the spike, which led to the reduced number of grains per spikelet reported in the triple mutant.
In addition to the effects of TaExpA6 and TaGW2 gene manipulations on grain weight and number, recent elucidation of the role of the GNI1 gene offers additional insights into the genetic regulation of these yield components. Research by Sakuma et al. (2019) highlighted that the GNI1 gene, coding for a homeodomain leucine zipper class I transcription factor, plays a pivotal role in floret fertility in wheat. Their findings revealed an evolutionary adaptation in the expression of GNI1, leading to an increase in grain number per spikelet in domesticated wheat, suggesting a genetic basis for the observed trade-off between grain weight and grain number. Complementing this, Golan et al. (2019) proposed the GNI-A1 gene, a variant of GNI1, as a mediator of this trade-off. Remarkably, GNI1 is predominantly expressed in immature spikes before anthesis (Sakuma et al., 2019), further highlighting the relevance of the overlap between grain number and weight determinations as key to unravel the underlying causes for the trade-off. Additionally, Xie and Sparkes (2021) found an overlapping between major regions associated with grain number and grain weight in a mapping population of 226 RILs.
The integration of these insights with the results of our study suggests a multifaceted genetic network influencing wheat yield. While our study focuses on the phenotypic outcomes of TaExpA6 and TaGW2 gene manipulations, the role of the GNI1 gene underscores the importance of genetic control in floret fertility and assimilate allocation. This understanding complements our observations of the trade-off between grain weight and grain number, indicating that both targeted genetic modifications and natural genetic variations, such as those in GNI1, are crucial in determining these key agronomic traits. Therefore, a comprehensive strategy that considers both natural gene variants like GNI1 and targeted modifications such as TaExpA6 and TaGW2 may be essential for the development of wheat varieties that optimally balance grain weight and number.
5 Concluding remarks
In conclusion, the results of this study emphasize the complexity of the genetic control of grain weight and number in wheat. The findings reveal that modification of the TaExpA6 gene enhances grain weight without reducing grain number, indicating a deviation from the traditionally assumed trade-off between these yield components. In contrast, alterations in the TaGW2 gene, while highly increasing grain weight, also result in a reduction in grain number, aligning with the conventional understanding of this trade-off. These outcomes highlight distinct genetic pathways influencing wheat yield traits. The differential impacts of TaExpA6 and TaGW2 on wheat grain development, and the overlapping of both yield components determination between booting and a week after anthesis, offer valuable insights for future overcoming this common bottleneck and to improve grain yield in wheat breeding programs through targeted genetic modifications.
Data availability statement
The original contributions presented in the study are included in the article/Supplementary material, further inquiries can be directed to the corresponding author/s.
Author contributions
LV: Data curation, Formal analysis, Investigation, Supervision, Writing – original draft, Writing – review & editing. JC: Data curation, Methodology, Supervision, Writing – review & editing. DC: Conceptualization, Formal analysis, Funding acquisition, Project administration, Supervision, Writing – original draft.
Funding
The author(s) declare financial support was received for the research, authorship, and/or publication of this article. This research was supported by Project FONDECYT Regular 1211040 (Chilean National Research and Development Agency -ANID-). The authors acknowledge the contribution of PhD scholarship Folio 21220957 (ANID), Chile.
Acknowledgments
The authors wish to warmly thank Dr. Emma Wallington and the National Institute of Agricultural Botany (NIAB, UK) for kindly facilitating the ExpA6 lines, and Prof. Cristobal Uauy and John Innes Center (JIC, UK) for their generous provision of the GW2 lines. Japan Tobacco Inc. is recognized as a holder for the technology included in PureWheat® licenced from Japan Tobacco Inc. We also thank the experimental field staff of the Austral Farming Experimental Station of Universidad Austral de Chile for the technical assistance provided. Technical contributions and management of field trials by Marcelo Castro Moraga and Cristóbal Castro Villalón is truly appreciated. We are also very grateful to María Beatriz Ugalde Jaramillo and Dr. Anita Arenas Miranda (Plant Nutrition and Genomics Lab – UACh) for performing the molecular analysis included in this study. The samples processing by Beatriz Shibar (UACh) and Magda Lobnik is recognized. JC was supported by ANID–Millennium Science Initiative Program–ICN17-022 and FONDECYT Regular 1230833. LV was supported by PhD scholarship 21220957-2022 from ANID, Chile.
Conflict of interest
The authors declare that the research was conducted in the absence of any commercial or financial relationships that could be construed as a potential conflict of interest.
Publisher’s note
All claims expressed in this article are solely those of the authors and do not necessarily represent those of their affiliated organizations, or those of the publisher, the editors and the reviewers. Any product that may be evaluated in this article, or claim that may be made by its manufacturer, is not guaranteed or endorsed by the publisher.
Supplementary material
The Supplementary Material for this article can be found online at: https://www.frontiersin.org/articles/10.3389/fpls.2024.1380429/full#supplementary-material
References
Abbate, P. E., Andrade, F. H., Culot, J. P., Bindraban, P. S. (1997). Grain yield in wheat: Effects of radiation during spike growth period. Field Crops Res. 54, 245–257. doi: 10.1016/S0378-4290(97)00059-2
Acreche, M. M., Slafer, G. A. (2006). Grain weight response to increases in number of grains in wheat in a Mediterranean area. Field Crops Res. 98, 52–59. doi: 10.1016/j.fcr.2005.12.005
Adamski, N. M., Simmonds, J., Brinton, J. F., Backhaus, A. E., Chen, Y., Smedley, M., et al. (2021). Ectopic expression of Triticum polonicum VRT-A2 underlies elongated glumes and grains in hexaploid wheat in a dosage-dependent manner. Plant Cell 33, 2296–2319. doi: 10.1093/plcell/koab119
Alonso, M. P., Abbate, P. E., Mirabella, N. E., Aramburu Merlos, F., Panelo, J. S., Pontaroli, A. C. (2018). Analysis of sink/source relations in bread wheat recombinant inbred lines and commercial cultivars under a high yield potential environment. Eur. J. Agron. 93, 82–87. doi: 10.1016/j.eja.2017.11.007
Alvarez Prado, S., Gallardo, J. M., Serrago, R. A., Kruk, B. C., Miralles, D. J. (2013). Comparative behavior of wheat and barley associated with field release and grain weight determination. Field Crops Res. 144, 28–33. doi: 10.1016/j.fcr.2012.12.018
Beed, F. D., Paveley, N. D., Sylvester-Bradley, R. (2007). Predictability of wheat growth and yield in light-limited conditions. J. Agric. Sci. 145, 63–79. doi: 10.1017/S0021859606006678
Borrás, L., Slafer, G. A., Otegui, M. E. (2004). Seed dry weight response to source–sink manipulations in wheat, maize and soybean: a quantitative reappraisal. Field Crops Res. 86, 131–146. doi: 10.1016/j.fcr.2003.08.002
Brinton, J., Simmonds, J., Minter, F., Leverington-Waite, M., Snape, J., Uauy, C. (2017). Increased pericarp cell length underlies a major quantitative trait locus for grain weight in hexaploid wheat. New Phytol. 215, 1026–1038. doi: 10.1111/nph.14624
Brinton, J., Uauy, C. (2019). A reductionist approach to dissecting grain weight and yield in wheat. J. Integr. Plant Biol. 61, 337–358. doi: 10.1111/jipb.12741
Bustos, D. V., Hasan, A. K., Reynolds, M. P., Calderini, D. F. (2013). Combining high grain number and weight through a DH-population to improve grain yield potential of wheat in high-yielding environments. Field Crops Res. 145, 106–115. doi: 10.1016/j.fcr.2013.01.015
Calderini, D. F., Abeledo, L. G., Savin, R., Slafer, G. A. (1999). Effect of temperature and carpel size during pre-anthesis on potential grain weight in wheat. J. Agric. Sci. 132, 453–459. doi: 10.1017/S0021859699006504
Calderini, D. F., Castillo, F. M., Arenas, M. A., Molero, G., Reynolds, M. P., Craze, M., et al. (2021). Overcoming the trade-off between grain weight and number in wheat by the ectopic expression of expansin in developing seeds leads to increased yield potential. New Phytol. 230, 629–640. doi: 10.1111/nph.17048
Calderini, D. F., Reynolds, M. P. (2000). Changes in grain weight as a consequence of de-graining treatments at pre- and post-anthesis in synthetic hexaploid lines of wheat (Triticum durum x T. tauschii). Funct. Plant Biol. 27, 183–191. doi: 10.1071/PP99066
Calderini, D. F., Savin, R., Abeledo, L. G., Reynolds, M. P., Slafer, G. A. (2001). The importance of the period immediately preceding anthesis for grain weight determination in wheat. Euphytica 119, 199–204. doi: 10.1023/A:1017597923568
Canales, J., Verdejo, J., Carrasco-Puga, G., Castillo, F. M., Arenas-M, A., Calderini, D. F. (2021). Transcriptome analysis of seed weight plasticity in brassica napus. Int. J. Mol. Sci. 22, 4449. doi: 10.3390/ijms22094449
Castillo, F. M., Canales, J., Claude, A., Calderini, D. F. (2018). Expansin genes expression in growing ovaries and grains of sunflower are tissue-specific and associate with final grain weight. BMC Plant Biol. 18, 327. doi: 10.1186/s12870-018-1535-7
Castillo, F. M., Vásquez, S. C., Calderini, D. F. (2017). Does the pre-flowering period determine the potential grain weight of sunflower? Field Crops Res. 212, 23–33. doi: 10.1016/j.fcr.2017.06.029
Cosgrove, D. J. (2000). Loosening of plant cell walls by expansins. Nature 407, 321–326. doi: 10.1038/35030000
Cosgrove, D. J. (2021). Expanding wheat yields with expansin. New Phytol. 230, 403–405. doi: 10.1111/nph.17245
Cosgrove, D. J. (2024). Structure and growth of plant cell walls. Nat. Rev. Mol. Cell Biol. 25, 340–358. doi: 10.1038/s41580-023-00691-y
Cruz-Castillo, J. G., Lawes, G. S., Woolley, D. J. (1992). The influence of the time of anthesis, seed factor(s), and the application of a growth regulator mixture on the growth of kiwifruit. Acta Hortic 297, 475–480. doi: 10.17660/ActaHortic.1992.297.62
Digeon, J.-F., Guiderdoni, E., Alary, R., Michaux-Ferrière, N., Joudrier, P., Gautier, M.-F. (1999). Cloning of a wheat puroindoline gene promoter by IPCR and analysis of promoter regions required for tissue-specific expression in transgenic rice seeds. Plant Mol. Biol. 39, 1101–1112. doi: 10.1023/A:1006194326804
Drea, S. A., Leader, D. J., Arnold, B. C., Shaw, P., Dolan, L., Doonan, J. H. (2005). Systematic spatial analysis of gene expression during wheat caryopsis development. Plant Cell 17, 2172–2185. doi: 10.1105/tpc.105.034058
Dwivedi, S. L., Reynolds, M. P., Ortiz, R. (2021). Mitigating tradeoffs in plant breeding. iScience 24, 102965. doi: 10.1016/j.isci.2021.102965
Ferrante, A., Cartelle, J., Savin, R., Slafer, G. A. (2017). Yield determination, interplay between major components and yield stability in a traditional and a contemporary wheat across a wide range of environments. Field Crops Res. 203, 114–127. doi: 10.1016/j.fcr.2016.12.028
Ferrante, A., Savin, R., Slafer, G. A. (2015). Relationship between fruiting efficiency and grain weight in durum wheat. Field Crops Res. 177, 109–116. doi: 10.1016/j.fcr.2015.03.009
Fischer, R. A. (1985). Number of kernels in wheat crops and the influence of solar radiation and temperature. J. Agric. Sci. 105, 447–461. doi: 10.1017/S0021859600056495
Fischer, R. A. (2008). The importance of grain or kernel number in wheat: A reply to Sinclair and Jamieson. Field Crops Res. 105, 15–21. doi: 10.1016/j.fcr.2007.04.002
Fischer, R. A. (2022). ““History of Wheat Breeding: A Personal View,”,” in Wheat Improvement. Eds. Reynolds, M. P., Braun, H.-J. (Springer, Switzerland), 17–30.
Gautier, M.-F., Aleman, M.-E., Guirao, A., Marion, D., Joudrier, P. (1994). Triticum aestivum puroindolines, two basic cystine-rich seed proteins: cDNA sequence analysis and developmental gene expression. Plant Mol. Biol. 25, 43–57. doi: 10.1007/BF00024197
Geng, J., Li, L., Lv, Q., Zhao, Y., Liu, Y., Zhang, L., et al. (2017). TaGW2–6A allelic variation contributes to grain size possibly by regulating the expression of cytokinins and starch-related genes in wheat. Planta 246, 1153–1163. doi: 10.1007/s00425-017-2759-8
Golan, G., Ayalon, I., Perry, A., Zimran, G., Ade-Ajayi, T., Mosquna, A., et al. (2019). GNI-A1 mediates trade-off between grain number and grain weight in tetraploid wheat. Theor. Appl. Genet. 132, 2353–2365. doi: 10.1007/s00122-019-03358-5
Guo, L., Ma, M., Wu, L., Zhou, M., Li, M., Wu, B., et al. (2022). Modified expression of TaCYP78A5 enhances grain weight with yield potential by accumulating auxin in wheat (Triticum aestivum L.). Plant Biotechnol. J. 20, 168–182. doi: 10.1111/pbi.13704
Guo, Z., Slafer, G. A., Schnurbusch, T. (2016). Genotypic variation in spike fertility traits and ovary size as determinants of floret and grain survival rate in wheat. J. Exp. Bot. 67, 4221–4230. doi: 10.1093/jxb/erw200
Handley, D. T., Dill, J. F. (2003). Vegetative and floral characteristics of six strawberry cultivars associated with fruit size, yield and susceptibility to tarnished plant bug injury (Leuven, Belgium: International Society for Horticultural Science (ISHS), 161–167. doi: 10.17660/ActaHortic.2003.626.21
Hasan, A. K., Herrera, J., Lizana, C., Calderini, D. F. (2011). Carpel weight, grain length and stabilized grain water content are physiological drivers of grain weight determination of wheat. Field Crops Res. 123, 241–247. doi: 10.1016/j.fcr.2011.05.019
Hong, Y., Chen, L., Du, L. P., Su, Z., Wang, J., Ye, X., et al. (2014). Transcript suppression of TaGW2 increased grain width and weight in bread wheat. Funct. Integr. Genomics 14, 341–349. doi: 10.1007/s10142-014-0380-5
Jablonski, B., Szala, K., Przyborowski, M., Bajguz, A., Chmur, M., Gasparis, S., et al. (2021). TaCKX2.2 genes coordinate expression of other taCKX family members, regulate phytohormone content and yield-related traits of wheat. Int. J. Mol. Sci. 22. doi: 10.3390/ijms22084142
Khan, N., Zhang, Y., Wang, J., Li, Y., Chen, X., Yang, L., et al. (2022). TaGSNE, a WRKY transcription factor, overcomes the trade-off between grain size and grain number in common wheat and is associated with root development. J. Exp. Bot. 73, 6678–6696. doi: 10.1093/jxb/erac327
Kino, R. I., Pellny, T. K., Mitchell, R. A. C., Gonzalez-Uriarte, A., Tosi, P. (2020). High post-anthesis temperature effects on bread wheat (Triticum aestivum L.) grain transcriptome during early grain-filling. BMC Plant Biol. 20, 170. doi: 10.1186/s12870-020-02375-7
Kumar, A., Mantovani, E. E., Seetan, R., Soltani, A., Echeverry-Solarte, M., Jain, S., et al. (2016). Dissection of Genetic Factors underlying Wheat Kernel Shape and Size in an Elite × Nonadapted Cross using a High Density SNP Linkage Map. Plant Genome 9, plantgenome2015.2009.0081. doi: 10.3835/plantgenome2015.09.0081
Lai, R., Woolley, D. J., Lawes, G. S. (1990). The effect of inter-fruit competition, type of fruiting lateral and time of anthesis on the fruit growth of kiwifruit (Actinidia deliciosa). J. Hortic. Sci. 65, 87–96. doi: 10.1080/00221589.1990.11516034
Lichthardt, C., Chen, T. W., Stahl, A., Stutzel, H. (2020). Co-evolution of sink and source in the recent breeding history of winter wheat in Germany. Front. Plant Sci. 10, 1771. doi: 10.3389/fpls.2019.01771
Lin, Z., Ni, Z., Zhang, Y., Yao, Y., Wu, H., Sun, Q. (2005). Isolation and characterization of 18 genes encoding α- and β-expansins in wheat (Triticum aestivum L.). Mol. Genet. Genomics 274, 548–556. doi: 10.1007/s00438-005-0029-0
Lindström, L. I., Pellegrini, C. N., Aguirrezábal, L. A. N., Hernández, L. F. (2006). Growth and development of sunflower fruits under shade during pre and early post-anthesis period. Field Crops Res. 96, 151–159. doi: 10.1016/j.fcr.2005.06.006
Lindström, L. I., Pellegrini, C. N., Hernández, L. F. (2007). Histological development of the sunflower fruit pericarp as affected by pre- and early post-anthesis canopy shading. Field Crops Res. 103, 229–238. doi: 10.1016/j.fcr.2007.06.005
Liu, H., Li, H., Hao, C., Wang, K., Wang, Y., Qin, L., et al. (2020). TaDA1, a conserved negative regulator of kernel size, has an additive effect with TaGW2 in common wheat (Triticum aestivum L.). Plant Biotechnol. J. 18, 1330–1342. doi: 10.1111/pbi.13298
Lizana, X. C., Calderini, D. F. (2013). Yield and grain quality of wheat in response to increased temperatures at key periods for grain number and grain weight determination: considerations for the climatic change scenarios of Chile. J. Agric. Sci. 151, 209–221. doi: 10.1017/S0021859612000639
Lizana, X. C., Riegel, R., Gomez, L. D., Herrera, J., Isla, A., Mcqueen-Mason, S. J., et al. (2010). Expansins expression is associated with grain size dynamics in wheat (Triticum aestivum L.). J. Exp. Bot. 61, 1147–1157. doi: 10.1093/jxb/erp380
McQueen-Mason, S., Durachko, D. M., Cosgrove, D. J. (1992). Two endogenous proteins that induce cell wall extension in plants. Plant Cell 4, 1425–1433.
Merrill, A. L., Watt, B. K. (1973). Energy Value of Foods: Basis and Derivation (Washington DC, United States: United States Department of Agriculture (USDA).
Millet, E., Pinthus, M. J. (1980). Genotypic effects of the maternal tissues of wheat on its grain weight. Theor. Appl. Genet. 58, 247–252. doi: 10.1007/BF00265174
Milner, M. J., Bowden, S., Craze, M., Wallington, E. J. (2021). Ectopic expression of TaBG1 increases seed size and alters nutritional characteristics of the grain in wheat but does not lead to increased yields. BMC Plant Biol. 21, 524. doi: 10.1186/s12870-021-03294-x
Mira, J. P., Arenas-M, A., Calderini, D. F., Canales, J. (2023). Integrated transcriptome analysis identified key expansin genes associated with wheat cell wall, grain weight and yield. Plants 12, 2868. doi: 10.3390/plants12152868
Mora-Ramirez, I., Weichert, H., Von Wiren, N., Frohberg, C., De Bodt, S., Schmidt, R. C., et al. (2021). The da1 mutation in wheat increases grain size under ambient and elevated CO(2) but not grain yield due to trade-off between grain size and grain number. Plant Environ. Interact. 2, 61–73. doi: 10.1002/pei3.10041
Muñoz, M., Calderini, D. F. (2015). Volume, water content, epidermal cell area, and XTH5 expression in growing grains of wheat across ploidy levels. Field Crops Res. 173, 30–40. doi: 10.1016/j.fcr.2014.12.010
Murchie, E. H., Reynolds, M., Slafer, G. A., Foulkes, M. J., Acevedo-Siaca, L., Mcausland, L., et al. (2023). A ‘wiring diagram’ for source strength traits impacting wheat yield potential. J. Exp. Bot. 74, 72–90. doi: 10.1093/jxb/erac415
Okamoto, Y., Takumi, S. (2013). Pleiotropic effects of the elongated glume gene P1 on grain and spikelet shape-related traits in tetraploid wheat. Euphytica 194, 207–218. doi: 10.1007/s10681-013-0916-0
Olsen, O. A., Kalla, R., Potter, R. H. (1992). Histo-differentiation and molecular biology of developing cereal endosperm. Seed Sci. Res. 2, 117–131. doi: 10.1017/S0960258500001240
Parent, B., Bonneau, J., Maphosa, L., Kovalchuk, A., Langridge, P., Fleury, D. (2017). Quantifying wheat sensitivities to environmental constraints to dissect genotype x environment interactions in the field. Plant Physiol. 174, 1669–1682. doi: 10.1104/pp.17.00372
Pepler, S., Gooding, M. J., Ellis, R. H. (2006). Modelling simultaneously water content and dry matter dynamics of wheat grains. Field Crops Res. 95, 49–63. doi: 10.1016/j.fcr.2005.02.001
Pfaffl, M. W. (2001). A new mathematical model for relative quantification in real-time RT–PCR. Nucleic Acids Res. 29, e45–e45. doi: 10.1093/nar/29.9.e45
Philipp, N., Weichert, H., Bohra, U., Weschke, W., Schulthess, A. W., Weber, H. (2018). Grain number and grain yield distribution along the spike remain stable despite breeding for high yield in winter wheat. PloS One 13, e0205452. doi: 10.1371/journal.pone.0205452
Quintero, A., Molero, G., Reynolds, M. P., Calderini, D. F. (2018). Trade-off between grain weight and grain number in wheat depends on GxE interaction: A case study of an elite CIMMYT panel (CIMCOG). Eur. J. Agron. 92, 17–29. doi: 10.1016/j.eja.2017.09.007
Radchuk, V., Weier, D., Radchuk, R., Weschke, W., Weber, H. (2011). Development of maternal seed tissue in barley is mediated by regulated cell expansion and cell disintegration and coordinated with endosperm growth. J. Exp. Bot. 62, 1217–1227. doi: 10.1093/jxb/erq348
Reale, L., Rosati, A., Tedeschini, E., Ferri, V., Cerri, M., Ghitarrini, S., et al. (2017). Ovary size in wheat (Triticum aestivum L.) is related to cell number. Crop Sci. 57, 914–925. doi: 10.2135/cropsci2016.06.0511
Reynolds, M. P., Foulkes, M. J., Slafer, G. A., Berry, P., Parry, M. A. J., Snape, J. W., et al. (2009). Raising yield potential in wheat. J. Exp. Bot. 60, 1899–1918. doi: 10.1093/jxb/erp016
Rondanini, D. P., Mantese, A. I., Savin, R., Hall, A. J. (2009). Water content dynamics of achene, pericarp and embryo in sunflower: Associations with achene potential size and dry-down. Eur. J. Agron. 30, 53–62. doi: 10.1016/j.eja.2008.07.002
Rosati, A., Benincasa, P. (2023). Revisiting source versus sink limitations of wheat yield during grain filling. Agron. J. 115, 3197–3205. doi: 10.1002/agj2.21454
Rosati, A., Zipanćič, M., Caporali, S., Padula, G. (2009). Fruit weight is related to ovary weight in olive (Olea europaea L.). Scientia Hortic. 122, 399–403. doi: 10.1016/j.scienta.2009.05.034
Ruijter, J. M., Ramakers, C., Hoogaars, W. M. H., Karlen, Y., Bakker, O., Van Den Hoff, M. J. B., et al. (2009). Amplification efficiency: linking baseline and bias in the analysis of quantitative PCR data. Nucleic Acids Res. 37, e45–e45. doi: 10.1093/nar/gkp045
Sadras, V. O. (2007). Evolutionary aspects of the trade-off between seed size and number in crops. Field Crops Res. 100, 125–138. doi: 10.1016/j.fcr.2006.07.004
Saini, H. S., Westgate, M. E. (1999). “Reproductive Development in Grain Crops during Drought,” in Advances in Agronomy. Ed. Sparks, D. L. (San Diego, USA: Academic Press), 59–96.
Sakuma, S., Golan, G., Guo, Z., Ogawa, T., Tagiri, A., Sugimoto, K., et al. (2019). Unleashing floret fertility in wheat through the mutation of a homeobox gene. Proc. Natl. Acad. Sci. U.S.A. 116, 5182–5187. doi: 10.1073/pnas.1815465116
Sandaña, P. A., Harcha, C. I., Calderini, D. F. (2009). Sensitivity of yield and grain nitrogen concentration of wheat, lupin and pea to source reduction during grain filling. A comparative survey under high yielding conditions. Field Crops Res. 114, 233–243. doi: 10.1016/j.fcr.2009.08.003
Sangha, J. S., Gu, K., Kaur, J., Yin, Z. (2010). An improved method for RNA isolation and cDNA library construction from immature seeds of Jatropha curcas L. BMC Res. Notes 3, 126. doi: 10.1186/1756-0500-3-126
Savin, R., Slafer, G. A. (1991). Shading effects on the yield of an Argentinian wheat cultivar. J. Agric. Sci. 116, 1–7. doi: 10.1017/S0021859600076085
Scorzal, R., May, L. G., Purnell, B., Upchurch, B. (1991). Differences in number and area of mesocarp cells between small- and large-fruited peach cultivars. J. Am. Soc. Hortic. Sci. jashs 116, 861–864. doi: 10.21273/JASHS.116.5.861
Scott, W. R., Appleyard, M., Fellowes, G., Kirby, E. J. M. (1983). Effect of genotype and position in the ear on carpel and grain growth and mature grain weight of spring barley. J. Agric. Sci. 100, 383–391. doi: 10.1017/S0021859600033530
Serrago, R. A., Alzueta, I., Savin, R., Slafer, G. A. (2013). Understanding grain yield responses to source–sink ratios during grain filling in wheat and barley under contrasting environments. Field Crops Res. 150, 42–51. doi: 10.1016/j.fcr.2013.05.016
Simmonds, J., Scott, P., Brinton, J., Mestre, T. C., Bush, M., Del Blanco, A., et al. (2016). A splice acceptor site mutation in TaGW2-A1 increases thousand grain weight in tetraploid and hexaploid wheat through wider and longer grains. Theor. Appl. Genet. 129, 1099–1112. doi: 10.1007/s00122-016-2686-2
Simmonds, J., Scott, P., Leverington-Waite, M., Turner, A. S., Brinton, J., Korzun, V., et al. (2014). Identification and independent validation of a stable yield and thousand grain weight QTL on chromosome 6A of hexaploid wheat (Triticum aestivum L.). BMC Plant Biol. 14, 191. doi: 10.1186/s12870-014-0191-9
Sinclair, T. R., Jamieson, P. D. (2006). Grain number, wheat yield, and bottling beer: An analysis. Field Crops Res. 98, 60–67. doi: 10.1016/j.fcr.2005.12.006
Slafer, G. A., Foulkes, M. J., Reynolds, M. P., Murchie, E. H., Carmo-Silva, E., Flavell, R., et al. (2023). A ‘wiring diagram’ for sink strength traits impacting wheat yield potential. J. Exp. Bot. 74, 40–71. doi: 10.1093/jxb/erac410
Slafer, G. A., Savin, R. (1994). Source—sink relationships and grain mass at different positions within the spike in wheat. Field Crops Res. 37, 39–49. doi: 10.1016/0378-4290(94)90080-9
Slafer, G. A., Savin, R., Pinochet, D., Calderini, D. F. (2021). ““Wheat,”,” in Crop Physiology. Case Histories for Mjor Crops. Eds. Sadras, V., Calderini, D. F. (Academic Press, Elsevier, London), 99–145.
Song, X. J., Huang, W., Shi, M., Zhu, M. Z., Lin, H. X. (2007). A QTL for rice grain width and weight encodes a previously unknown RING-type E3 ubiquitin ligase. Nat. Genet. 39, 623–630. doi: 10.1038/ng2014
Tao, Y., Trusov, Y., Zhao, X., Wang, X., Cruickshank, A. W., Hunt, C., et al. (2021). Manipulating assimilate availability provides insight into the genes controlling grain size in sorghum. Plant J. 108, 231–243. doi: 10.1111/tpj.15437
Tillett, B. J., Hale, C. O., Martin, J. M., Giroux, M. J. (2022). Genes Impacting Grain Weight and Number in Wheat (Triticum aestivum L. ssp. aestivum). Plants (Basel) 11. doi: 10.3390/plants11131772
Ugarte, C., Calderini, D. F., Slafer, G. A. (2007). Grain weight and grain number responsiveness to pre-anthesis temperature in wheat, barley and triticale. Field Crops Res. 100, 240–248. doi: 10.1016/j.fcr.2006.07.010
Waddington, S. R., Cartwright, P. M., Wall, P. C. (1983). A quantitative scale of spike initial and pistil development in barley and wheat. Ann. Bot. 51, 119–130. doi: 10.1093/oxfordjournals.aob.a086434
Wang, W., Li, Q., Tian, F., Deng, Y., Wang, W., Wu, Y., et al. (2019). Wheat NILs contrasting in grain size show different expansin expression, carbohydrate and nitrogen metabolism that are correlated with grain yield. Field Crops Res. 241. doi: 10.1016/j.fcr.2019.107564
Wang, W., Simmonds, J., Pan, Q., Davidson, D., He, F., Battal, A., et al. (2018). Gene editing and mutagenesis reveal inter-cultivar differences and additivity in the contribution of TaGW2 homoeologues to grain size and weight in wheat. Theor. Appl. Genet. 131, 2463–2475. doi: 10.1007/s00122-018-3166-7
Wiersma, J. J., Busch, R. H., Fulcher, G. G., Hareland, G. A. (2001). Recurrent selection for kernel weight in spring wheat. Crop Sci. 41, 999–1005. doi: 10.2135/cropsci2001.414999x
Xie, Q., Mayes, S., Sparkes, D. L. (2015). Carpel size, grain filling, and morphology determine individual grain weight in wheat. J. Exp. Bot. 66, 6715–6730. doi: 10.1093/jxb/erv378
Xie, Q., Sparkes, D. L. (2021). Dissecting the trade-off of grain number and size in wheat. Planta 254, 3. doi: 10.1007/s00425-021-03658-5
Yang, Z., Bai, Z., Li, X., Wang, P., Wu, Q., Yang, L., et al. (2012). SNP identification and allelic-specific PCR markers development for TaGW2, a gene linked to wheat kernel weight. Theor. Appl. Genet. 125, 1057–1068. doi: 10.1007/s00122-012-1895-6
Yang, Z., Van Oosterom, E. J., Jordan, D. R., Hammer, G. L. (2009). Pre-anthesis ovary development determines genotypic differences in potential kernel weight in sorghum. J. Exp. Bot. 60, 1399–1408. doi: 10.1093/jxb/erp019
Yang, X., Wilkinson, L. G., Aubert, M. K., Houston, K., Shirley, N. J., Tucker, M. R. (2023). Ovule cell wall composition is a maternal determinant of grain size in barley. New Phytol. 237, 2136–2147. doi: 10.1111/nph.18714
Yu, X., Li, B., Wang, L., Chen, X., Wang, W., Wang, Z., et al. (2015). Systematic analysis of pericarp starch accumulation and degradation during wheat caryopsis development. PloS One 10, e0138228. doi: 10.1371/journal.pone.0138228
Zadoks, J. C., Chang, T. T., Konzak, C. F. (1974). A decimal code for the growth stages of cereals. Weed Res. 14, 415–421. doi: 10.1111/j.1365-3180.1974.tb01084.x
Zhai, H., Feng, Z., Du, X., Song, Y., Liu, X., Qi, Z., et al. (2018). A novel allele of TaGW2-A1 is located in a finely mapped QTL that increases grain weight but decreases grain number in wheat (Triticum aestivum L.). Theor. Appl. Genet. 131, 539–553. doi: 10.1007/s00122-017-3017-y
Zhang, Y., Li, D., Zhang, D., Zhao, X., Cao, X., Dong, L., et al. (2018). Analysis of the functions of TaGW2 homoeologs in wheat grain weight and protein content traits. Plant J. 94, 857–866. doi: 10.1111/tpj.13903
Keywords: TaExpA6, TaGW2, source-sink, expansins, ovary weight, grain size regulation, grain yield, cereals
Citation: Vicentin L, Canales J and Calderini DF (2024) The trade-off between grain weight and grain number in wheat is explained by the overlapping of the key phases determining these major yield components. Front. Plant Sci. 15:1380429. doi: 10.3389/fpls.2024.1380429
Received: 01 February 2024; Accepted: 20 May 2024;
Published: 11 June 2024.
Edited by:
Iker Aranjuelo, Spanish National Research Council (CSIC), SpainReviewed by:
Santiago Alvarez Prado, National Scientific and Technical Research Council (CONICET), ArgentinaRaffaella Battaglia, Council for Agricultural and Economics Research (CREA), Italy
Luis Morales-Quintana, Autonomous University of Chile, Chile
Jan Hejatko, Central European Institute of Technology (CEITEC), Czechia
Copyright © 2024 Vicentin, Canales and Calderini. This is an open-access article distributed under the terms of the Creative Commons Attribution License (CC BY). The use, distribution or reproduction in other forums is permitted, provided the original author(s) and the copyright owner(s) are credited and that the original publication in this journal is cited, in accordance with accepted academic practice. No use, distribution or reproduction is permitted which does not comply with these terms.
*Correspondence: Daniel F. Calderini, ZGFuaWVsY2FsZGVyaW5pQHVhY2guY2w=