- 1Sugarcane Research Institute, Guangxi Academy of Agricultural Sciences/Key Laboratory of Sugarcane Biotechnology and Genetic Improvement (Guangxi), Ministry of Agriculture and Rural Affairs/Guangxi Key Laboratory of Sugarcane Genetic Improvement, Nanning, China
- 2Department of Botany, Mohanlal Sukhadia University, Udaipur, Rajasthan, India
- 3Department of Botany, University of Delhi, Delhi, India
The mutualistic plant rhizobacteria which improve plant development and productivity are known as plant growth-promoting rhizobacteria (PGPR). It is more significant due to their ability to help the plants in different ways. The main physiological responses, such as malondialdehyde, membrane stability index, relative leaf water content, photosynthetic leaf gas exchange, chlorophyll fluorescence efficiency of photosystem-II, and photosynthetic pigments are observed in plants during unfavorable environmental conditions. Plant rhizobacteria are one of the more crucial chemical messengers that mediate plant development in response to stressed conditions. The interaction of plant rhizobacteria with essential plant nutrition can enhance the agricultural sustainability of various plant genotypes or cultivars. Rhizobacterial inoculated plants induce biochemical variations resulting in increased stress resistance efficiency, defined as induced systemic resistance. Omic strategies revealed plant rhizobacteria inoculation caused the upregulation of stress-responsive genes—numerous recent approaches have been developed to protect plants from unfavorable environmental threats. The plant microbes and compounds they secrete constitute valuable biostimulants and play significant roles in regulating plant stress mechanisms. The present review summarized the recent developments in the functional characteristics and action mechanisms of plant rhizobacteria in sustaining the development and production of plants under unfavorable environmental conditions, with special attention on plant rhizobacteria-mediated physiological and molecular responses associated with stress-induced responses.
Introduction
Plant rhizobacteria-mediated abiotic stress reduction occurs directly through hormone induction or indirectly via signaling in the host plant. The direct function in nitrogen fixation, phosphate solubilization, auxin, cytokinin, gibberellin, and abscisic acid production are all documented. It also makes it easier for necessary mineral elements to be absorbed from the rhizospheric soil along with the production of plant growth regulators. However, the indirect roles include the production of metabolites, siderophores, antibiotics, volatile HCN, etc. Some of the compounds that the microbes may produce include deaminase enzyme, microbiocidal enzyme, siderophores, plant hormones, and PO4-solubilizing enzyme (Gujral et al., 2013; Ekinci et al., 2014; Saleem et al., 2015; Kumari and Khanna, 2016; Moustaine et al., 2017). Plants have unique microbiota, and the microbial structure in the rhizosphere is influenced by the bacteria and plants’ production of signal molecules and the chemical composition of root exudates (Zhang et al., 2017; Jalmi and Sinha, 2022). Plant-growth regulators, phytohormones, and various secondary metabolites can be produced by PRs to stimulate plant development (Islam et al., 2014; Kaushal and Wani, 2016) (Figure 1).
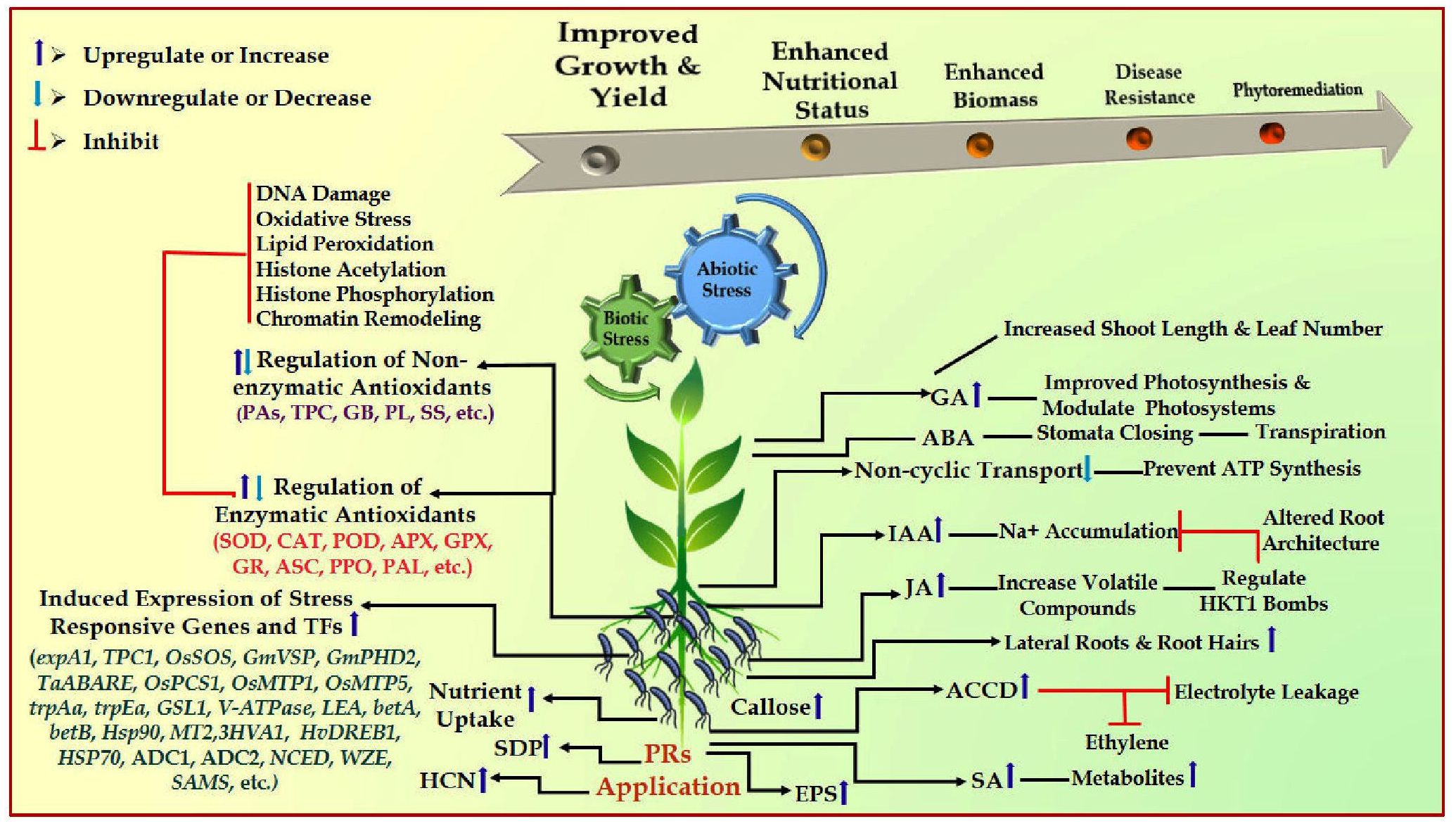
Figure 1 Schematic representation of PRs-mediated abiotic and biotic stress resistance mechanism in plants. ABA, abscisic acid; JA, jasmonic acid, GA, gibberellins, IAA, indole-3-acetic acid, SA, salicylic acid, EPS, exopolysaccharides, HCN, hydrogen cyanide; ACCD, 1-aminocyclopropane-1-carboxylate deaminase; SOD, superoxide dismutase; CAT, catalase; PAL, phenylalanine ammonia-lyase; APX, ascorbate peroxidase; POD, peroxidase; ASC, ascorbate; PPO, polyphenol oxidase; GPX, glutathione peroxidase; GR, glutathione reductase; Pas, polyamines; TPC, total phenolic content; PL, proline; SS, soluble sugar; HSPs, heat shock proteins; HKT—High-affinity K+ transporters; expA1, expansin; TPC1, calcium transporter; ADC1 and ADC2, putrescine synthesis; OsPCS1, phytochelatin synthase; OsMTP1, gene related to metal transport; OsMTP5, gene related to expulsion of excess metal; trpAa, and trpEa, genes related to tryptophan biosynthesis; betA and betB, genes related to betaine biosynthesis; GmVSP and GmPHD2, stress responsive genes; GSL1, gene related to cell wall synthesis; V-ATPase, Vacuolar-H+ -pyrophosphatase; LEA, late embryogenesis abundant; NCED, WZE and SAMS = transcription factors.
The upregulated synthesis of metabolites, such as phytohormone, exopolysaccharides, siderophores, antioxidant enzymes, and volatile compounds, primarily minimizes plant resistance to environmental challenges. The production of phytohormones by rhizobacteria-inoculated plants, including cytokinins (CK), gibberellic acid (GA), indole-3-acetic acid (IAA), and abscisic acid (ABA) is employed during plant stressed conditions. 1-aminocyclopropane-1-carboxylate (ACC) deaminase plays a significant role in conferring stress resistance capacity to plants by downregulating the level of stress-induced ethylene level in plant roots system (Etesami and Maheshwari, 2018; Shahid et al., 2023). Plant-rhizobacteria downregulated the effects of abiotic stresses by modifying the expression of genes associated with the biosynthesis of hormones, i.e., ACO and ACS genes (ethylene biosynthesis), MYC2 (Jasmonate), PR1 (SA), genes encoding antioxidant enzymes, transcription factor NAC1, etc. (Tiwari et al., 2017) (Tables 1, 2). Extensive field trials are required to investigate the interaction between the functional activities of signaling networks and their association. The interaction between PRs and plants based on various factors, such as root composition, strains of bacteria, and exudation patterns from their roots (Kumar et al., 2019). Numerous secondary metabolites and root exudates depend as chemo-attractants in the rhizosphere, attracting beneficial soil bacteria and inhibiting phytopathogens, thereby stimulating a delicate network of signaling between microbes and plants (Ullah et al., 2021; Joshi et al., 2022; Mellidou and Karamanoli, 2022; Joshi et al., 2023). The physiological and molecular responses activated in plants in response to stress resistance are regulated by various key genes with metabolic and regulatory roles. Research demonstrations focusing on plant gene expression following plant-rhizobacteria inoculation may help understand which can be an effective environmentally friendly approach to alleviate the adverse environmental variables (Ferrante et al., 2023; Verma et al., 2023). The formation of the enzyme ACC deaminase by rhizobacteria and reduction in ethylene level had been the main function for enhanced plant growth and resistance ability during different stresses (Bharti et al., 2014; Jalmi and Sinha, 2022).
Eco-physiological and omic responses of plant rhizobacteria required more attention and extensive field research demonstrations to increase stress resistance efficiency. Hence, the present article focused on the interactions between plants and rhizobacteria and their impact on tolerance to adverse agroclimatic variables for agricultural sustainability in an eco-friendly environment.
Impact of plant development, biomass, and productivity
Plant rhizobacteria (PRs) effectively improve plant morphological structures during adverse environmental conditions. Abiotic stresses, such as acidic and alkaline soil, insufficient water supply, low and high temperature, UV-radiation, soil flooding, and contaminated/toxic soil, affect agronomic, anatomical, cellular, and metabolic activities (Glick et al., 2007; Verma et al., 2020a, b). Higher levels of phytohormones, defense-related proteins and enzymes, antioxidants, and epoxypolysaccharides cause PGPR-induced resistance (Kaushal and Wani, 2016). It is accomplished by changing transcriptional and signaling processes, which lead to altered gene expression when PRs are present. Because PRs produce phytohormones that change root shape and improve root development, surface area, uptake, and accumulation of nutrients, plant productivity increases in the presence of PRs (Mellidou and Karamanoli, 2022). They can also increase total plant productivity by helping to induce ACC-deaminase activity in plants. The potential of PRs enhancing plant growth and development varies due to differences in their properties, such as ACC-deaminase activity, IAA generation, root colonization, P-solubilization, etc (Ghosh et al., 2018; Gupta and Pandey, 2019). The defense mechanisms of plants against unfavorable agroclimatic conditions depend on the variation in the development of roots (Khoshru et al., 2023). Different PGPR strains can enhance the overall root system by increasing the total number of root tips, surface area, and structure of the roots under stressful conditions (Brambilla et al., 2022). Lowering the ethylene content increases the plant’s capacity to withstand stress by facilitating improved nutrition and water uptake capacity (Chieb and Gachomo, 2023) (Figure 1).
When under stress, PRs also improve the uptake of water and nutrients. The absorption of nutrients and antioxidant activities are associated with stress management. By diminishing the negative consequences of saline soil, inoculation with Klebsiella oxytoca (Rs-5) containing ACC-deaminase boosted plant establishment and increased the absorption of key mineral nutrients (Yue et al., 2007; Zahir et al., 2012). In a similar way, Pseudomonas spp. inoculation increased the antioxidative enzymatic activities and promoted the growth of plants during unfavorable climatic conditions (Fu et al., 2010; Jalmi and Sinha, 2022) (Table 1).
According to Zahir et al. (2009) and Orozco-Mosqueda et al. (2020), rhizobacterial strains have been explored to have a substantial influence on the improvement of a variety of plants, including cereals, legumes, and vegetables cultivated under challenging conditions. They also enhanced the production of exopolysaccharides and ACC-deaminase activity. PRs enhance plant growth in polluted soil by downregulating the level of ethylene (Dell’Amico et al., 2008). PRs with 1-aminocyclopropane-1-carboxylic acid (ACC) deaminase activity may promote plant development during stress. Compared to uninoculated plants, the inoculated plants with PRs containing ACC-deaminase activity improved plant growth and yield considerably. Pseudomonas sp. and Acinetobacter sp. have increased IAA and ACC-deaminase production in saline soil and enhanced stress tolerance efficiency in barley and oats (Kang et al., 2019).
It can be indicated by the significantly increased levels of chlorophyll, total phenolics, flavonoids, soluble sugars, protein contents, and antioxidative enzymatic activities, as well as the higher expression of stress-related genes, that resulted from inoculating Cd-stressed with Serratia marcescens BM1 in Glycine max L. plants. Phaseolus vulgaris subjected to the rhizobacterial consortia experienced reduced stress caused by salinity and improved overall plant growth and photosynthetic pigments (Gupta and Pandey, 2019). In tomato plants, Streptomyces sp. has been shown to reduce stress and promote growth (Palaniyandi et al., 2014). It has been observed that Burkholderia phytofirmans helps plants under drought stress (Naveed et al., 2014). They generate exopolysaccharides (EPS) during water-deficit conditions, enhancing seed germination and growth. Of all the strains, Pseudomonas fluorescens has the highest capacity to produce EPS and ACC deaminase. The saline rice field was employed by Sultana et al. (2020) to isolate rhizobacterial strains, which they found to enhance stomatal conductance, transpiration, and photosynthetic CO2 assimilation rate, all of which contributed to increased crop yield, fruit and grains quality. According to the latest research, Azospirillum brasilense Sp245 increased Arabidopsis thaliana growth, suggesting that MAMPs produced from plant-rhizobacteria are essential for plant cultivation (Méndez-Gómez et al., 2021) (Tables 1, 2).
Photosynthetic leaf gas exchange and chlorophyll fluorescence efficiency
Plant-rhizobacteria enhance inoculated plants’ photosynthetic response and leaf gas exchange capability during stress (Verma et al., 2020b; Jalmi and Sinha, 2022). By modifying the photosynthetic characteristics, osmolytes production, antioxidant machinery, and expression of stress-related genes, inoculating soybean plants with Serratia marcescens BM1 (PR) provides Cd tolerance to plants (El-Esawi et al., 2020). Under salt stress, Bacillus amyloliquefaciens SQR9 has demonstrated higher efficiency in photosynthesis and overexpression of the RBCS and RBCL genes in Zea mays plants (Chen et al., 2016). During bacterial strain inoculation, Arabidopsis helleri showed elevated photosynthesis and proteins associated with abiotic stress (Khan et al., 2021).
Enhanced photosynthetic pigments and the expression of important genes (RBCS and RBCL) regulating RUBISCO activities during stress condition (Sherin et al., 2022; Amaral et al., 2023). By modulating ion homeostasis, redox potential, photosynthetic CO2 assimilation rate, and the expression of stress-related genes, maize plants inoculated with Serratia liquefaciens KM4 revealed enhanced growth and stress tolerance (El-Esawi et al., 2018). Reduced phenol, flavonoid, and leaf relative water content and photosynthetic responses in maize plants have resulted from salinity stress, which also decreased root damage and water uptake. However, inoculating maize under salt stress with Serratia liquefaciens KM4 enhanced LRWC, photosynthetic characteristics, and the biosynthesis pathways of phenols and flavonoids, enhancing plant stress tolerance efficiency. In comparison to uninoculated plants, rhizobacteria-inoculated maize and white clover have demonstrated enhanced photosynthesis, soluble proteins, sugars, and enzymatic activities following inoculation with HAS31 rhizobacteria (Han et al., 2014) (Figure 1; Table 1).
Uptake and accumulation of mineral nutrients and water balance
By altering the solubility and absorption of nutrients, PRs improve the bioavailability of nutrients in plants under abiotic factors. Through N2-fixation, mobilization, and the promotion of N2-fixers through their secretions, several rhizobacteria can reduce the volume of nitrogen (N2) supplementation required for plant growth (Shah et al., 2022; Khoso et al., 2024). Additionally, they change the shape and surface area of the roots, improving nitrogen bioavailability (Olenska et al., 2020). Elevating ammonium transporters’ expression improves nutritional absorption during stresses (Calvo et al., 2019). According to Gomez-Godínez et al. (2023), phosphorus (P) solubilizing PRs, such as Azotobacter, Bacillus, Burkholderia, Erwinia, Pseudomonas, Serratia, and Rhizobium, generate organic acids that chelate P-bound cations and make it available to plant roots. Furthermore, under Fe-deficient conditions, PRs assist in acquiring iron (Fe) by generating siderophores, which are low molecular weight organic molecules (Mohanty et al., 2021). Reducing metal ion availability and decreasing metal uptake, siderophores that generate PRs enhance plants’ survival under heavy metal stress (Dimkpa et al., 2009; Kumar et al., 2021) (Tables 1, 2).
Ocimum basilicum L. has demonstrated the ability of PRs to enhance nutrient absorption and downregulate abiotic stresses (Rakshapal et al., 2013). Under salinity stress, PRs, such as Pseudomonas sp. and Azospirillum sp., increase nutrient availability, improving plant growth, biomass, and productivity (Noorieh et al., 2013). The application of rhizobial inoculants has been observed to trigger delayed senescence, as evidenced by higher potassium (K) ion levels and lower ethylene and cytokinin production. In plants with a higher K+/Na+ ratio, PRs boost the absorption of K+ ions by synthesizing AtHKT1, a high-affinity ion channel that promotes stress tolerance (Mahmud et al., 2021) (Figure 1).
Biosynthesis of plant hormones and compatible solutes
Along with metabolites and signaling molecules, the majority of rhizobacteria produce phytohormones (Ahmad et al., 2022; Shah et al., 2022). Among these include gibberellic acid, cytokinins, indole acetic acid (IAA), and abscisic acid (ABA) (Tariq et al., 2023). IAA is produced by 80% of soil microorganisms, including Pseudomonas sp., Bacillus sp., Burkholderia sp., and Rhizobium sp (Khan et al., 2021). It has been shown that IAA-producing rhizobacteria stimulate crop production and plant growth when exposed to abiotic stress (Mellidou and Karamanoli, 2022). Numerous IAA-producing rhizobacteria increase root biomass, length, and surface area, which improves nutrient accumulation, uptake, and plant growth (Fasusi et al., 2023). Increased IAA levels also foster lateral roots’ growth, minerals’ absorption, and root exudates’ formation. It is well known that some PRs, including Arthrobacter, Azotobacter, Bacillus, Pseudomonas, and Pantoea, synthesize cytokinins that enhance nutrient availability as well as plant tolerance responses (Shah et al., 2022) (Table 1).
According to dos Santos et al. (2020), gibberellin-releasing PRs such as Azospirillum, Shingomonas, Bacillus amyloliquafaciens, and Bacillus pumilus can also promote plant growth and yield. Regulation of abscisic acid also played a significant role in stress resistance capacity influenced by rhizobacteria (Herrera-Medina et al., 2007). When pepper (Capsicum annum) is inoculated with Serratia nematodiphila (that produces gibberellin), the plant expands more under low-temperature stress, releases more GA4 and ABA, and lower salicylate and jasmonate activities (Kang et al., 2015).
The plant and bacterial species may impact the mechanism of ABA-mediated tolerance to stressful conditions. Under abiotic stress, specific PRs (strains of Rhizobium spp., B. pumilus, B. lycheniformis, Achromobacter xylosoxidans, and Azospirillium brasiliense) serve as ABA-stimulators or ABA-producers (Salomon et al., 2014; Egamberdieva et al., 2017). It can assist plants minimize water loss by activating Ca+2 channels that cause stomatal closure (Goswami and Deka, 2020; Grover et al., 2021). Greater ABA biosynthesis has been observed in Arabidopsis plants inoculated with the spermidine-producing B. megaterium strain (Zhou et al., 2016). By upregulating the gene expression that regulates ABA production, the rhizobacteria inoculation of rice with Pseudomonas fluorescens enhanced the plant’s resistance to stress. The upregulation of TaWRKY and TaMYB expression in ABA-signaling cascades has also been observed. It has also been suggested that specific rhizobacteria can use ABA as a carbon and energy source, limiting ABA uptake throughout the plant organs. These results indicated the changes in ABA-mediated signaling pathways as a means by which inoculated plants can survive abiotic challenges (Olenska et al., 2020; Mellidou and Karamanoli, 2022) (Figure 1).
It has also been demonstrated that using rhizobacteria minimizes the negative effects of ethylene generated under abiotic stress circumstances (Grichko and Glick, 2001; Nadeem et al., 2007; Zahir et al., 2008). Under abiotic stresses, rhizobacteria-inoculated plants have been demonstrated to modify ethylene biosynthesis-related gene expression (Lephatsi et al., 2021; Verma et al., 2021; Fadiji et al., 2022). Plants can be spared the toxicity of ethylene through the presence of rhizobacteria that contain ACC deaminase, which can hydrolyze ACC, the precursor of ET (Mellidou and Karamanoli, 2022).
The impact of Paenibacillus lentimorbus B-30488 inoculation on the reduction of abiotic stress in Arabidopsis thaliana, as well as by modifications in plant hormones and RSA-related gene expression. According to Khoshru et al. (2023), specific PRs also generate polyamines, which enhance root architecture and promote stomatal conductance and photosynthesis. The microbial community in the rhizosphere is mainly influenced by the exudates produced by plant roots, such as organic acids, mucilage, carbohydrates, sugars, and proteins, which also confer tolerance to inoculated rhizobacterial plants (Backer et al., 2018). Under abiotic stress, Azospirillum sp. has been demonstrated to accumulate appropriate solutes such as glutamate, proline, glycine, betaine, and trehalose (Saleena et al., 2002). Phaenibacillus polymyxa has been shown to possess the drought-responsive gene ERD15 (Timmusk and Wagner, 1999). Conjugated phytohormones and flavonoids in root tissue can be extracted or hydrolyzed by Azospirillum, releasing them in their active forms (Spaepen et al., 2007; Dardanelli et al., 2008; Saikia et al., 2010; Fahad et al., 2015).
The mechanisms of photosynthetic activity, hydraulic conductance, osmotic accumulation, and sequestering toxic ions are associated with rhizobacteria-stimulated resilience to stress (Figure 1). Groundnut inoculated with Bradyrhizobium under drought conditions demonstrated stress resistance due to amino acids produced from the nitrogenase to catalyzed the conversion of atmospheric nitrogen (N2) to ammonia (NH3) ions (Delfini et al., 2010; Enebe and Babalola, 2018). Furthermore, nitrogenase assists the supply of nitrogen to inoculated legumes, and these plants have been shown to produce more leaves due to more root nodules (Ferreira et al., 2011). To avoid desiccation, lower toxicity, and promote root growth, PRs also generate polysaccharides (Arora et al., 2010). A vital aspect of stress mitigation under environmental stress at the plant rhizosphere consists of forming biofilm and exopolysaccharide. One fascinating strategy PRs employ to mitigate the impacts of heat stress in plants involves the induction of osmoprotectants and heat shock proteins (HSPs) (Enebe and Babalola, 2018). Under stressful conditions, pepper plants treated with gibberellin-producing rhizobacteria showed a reduction in the level of salicylate and jasmonate. When the bacteria Burkholderia phytofirmans occurs, tomato plants produce more phenolics, proline, and starch under stress (Issa et al., 2018).
In plants under abiotic stress, PRs also improve proline synthesis. Arthrobacter, Bacillus, and Burkholderia are the main rhizobacteria that synthesize proline. Better stress tolerance in rhizobacteria-inoculated plants is mostly due to increased dissolved sugar levels and solute storage. Other potential strategies to reduce oxidative stress include stabilizing membranes, protein–protein complexes, and osmolytes, such as proline, glycine betaine, amino acids, and total sugars (Chieb and Gachomo, 2023).
Influence of enzymatic, non-enzymatic, and lignin biosynthesis
The synthesis of the enzyme ACC deaminase is a well-known mechanism for rhizobacteria-led abiotic stress tolerance (Etesami et al., 2015; Gupta and Pandey, 2019). By lowering ABA levels, plants inoculated with ACC-producing PRs expand more rapidly; the growth hormones regulate the synthesis of secondary metabolites (Kang et al., 2019). By promoting the activity of antioxidant enzymes (SOD, APX, and CAT) and upregulating the genes involved in the ROS pathway, it enhanced stress tolerance (Habib et al., 2016). Because ethylene causes stress-induced H2O2 accumulation and apoptosis induction, ACC deaminase-producing PRs provide plants resistance against abiotic stress by lowering ethylene synthesis. It has been observed that inoculating different crops under stress with strains that include ACC-deaminase enhances plant development (Li et al., 2017; Singh and Jha, 2017; Namwongsa et al., 2019; Danish et al., 2020; Mellidou et al., 2021; Mellidou and Karamanoli, 2022).
Plant-to-microbe communication also occurs by an array of non-hormonal signaling molecules. Microbes produce volatile compounds (VOCs), signaling molecules that control plant growth and modify soil and plant health in response to stress (Ullah et al., 2021). Moreover, plants tolerate heavy metal stress due to rhizobacteria-releasing extracellular polymeric substances (EPS), which primarily help by lowering the metals’ bioavailability in the soil (Mishra et al., 2017). Some species of Bacillus, Azotobacter, Burkholderia, Enterobacter, and Pseudomonas can reprogram plants’ redox states, increasing their tolerance to environmental stresses. During stress, the overproduction of reactive oxygen species (ROS) changes redox states and causes DNA damage, proteins, and membrane fluidity, ultimately resulting in cell death. However, plants inoculated with PRs defended against abiotic stressors by activating their defense mechanisms. Antioxidant enzyme activity enhanced in an array of growth-promoting rhizobacterial species to assist them in combatting oxidative stress (Mitra et al., 2021; Mellidou and Karamanoli, 2022) (Figure 1; Tables 1, 2).
Additionally, rhizobacteria are essential in reducing oxidative damage caused by various stressors, including heavy metals, water deficit, low and high temperatures, salt, and water scarcity. By lowering ROS levels in plant roots, rhizobacteria-induced antioxidant enzymes assist in reducing the stressors that plants experience in the environment. Additionally, they accelerate the growth rate in response to abiotic stressors by promoting the generation of antioxidant enzymes. Better stress tolerance in inoculated plants may be due to increased activities of antioxidant enzymes, such as catalase (CAT), ascorbate peroxidase (APX), or glutathione peroxidase (GPX) (Mellidou et al., 2021; Swain et al., 2021; Fadiji et al., 2022). Ascorbate peroxidase increased when tomato seedlings were inoculated with Enterobacter and subjected to abiotic stress. Gladiolus plants treated with rhizobacteria revealed increased levels of CAT and SOD activities as compared to their control group (Figure 1).
Tomato seedlings inoculated with P. oryzihabitans AXSa06 (having ACC deaminase) experienced mild oxidative stress and enhanced lipid peroxidation to trigger the antioxidant machinery (Mellidou et al., 2021). Under abiotic stress, tomato plants inoculated with a strain of Sphingomonas sp. revealed reduced lipid peroxidation, increased glutathione levels, and antioxidant enzyme activities (Halo et al., 2015; Mellidou and Karamanoli, 2022). In contrast, rhizobacteria inoculation has been demonstrated in additional studies to decrease the production of ROS-scavenging or stress-responsive enzymes that are important for plant protection in stressful environments (Gupta and Pandey, 2019; Goswami and Deka, 2020; Song et al., 2021; Verma et al., 2022a, b, c). The generation of defensive enzymes like chitinase and glucanase to the rhizobacteria stress-tolerance mechanism (García-Fraile et al., 2015). Glycine max plants inoculated with Bacillus firmus SW5 exhibit stress tolerance through alterations in root ultrastructure, antioxidant levels, and stress-related gene expression (El-Esawi et al., 2020). The production of oxalic acid, gluconic acid, and citric acid by stressed rhizobacteria plays a crucial role in the mobilization of heavy metals. Biofilm-forming rhizobacteria were inoculated into Spartina densiflora plants, resulting in increased levels of SOD, CAT, and APOX activities as well as a decrease in the induced oxidative stress index (OSI) (Perez et al., 2019; Khan et al., 2021; Bhat et al., 2022).
In Cicer arietinum plants, Pseudomonas putida MTCC5279 has been shown to reduce stress by enhanced ROS scavenging ability, modulation of membrane integrity, and accumulation of osmolyte (proline, glycine, betaine). These findings have also been validated by differential expression of genes involved in dehydration-responsive element binding, transcription factors expressed under abiotic stress, salicylic acid, jasmonate, transcription activation, SOD, CAT, APX, and GST (Tiwari et al., 2016; Chieb and Gachomo, 2023). In Abelmoschus esculentus plants, the presence of ACC-producing PRs was associated with increased activities of antioxidant enzymes (SOD, APX, and CAT) and up-regulated genes of the ROS pathways (CAT, APX, GR, and DHAR) (Habib et al., 2016). These pathways have also been linked to enhanced POD/CAT activity, decreased cell death, and increased glutathione levels for ROS scavenging. When Dietzia natronolimnaea was inoculated into wheat (Triticum aestivum), it was observed that the ABA-signaling cascade genes, ion transporters, salt overly sensitive (SOS) pathway, and antioxidant enzymes upregulated (Bharti et al., 2016) (Figure 1).
Conclusion and future prospects
Adverse environmental variables severely affect crop growth, development, and output and downregulate the overall socio-economic growth of sustainable agriculture. Different application strategies have been developed to challenge stress, its benefits, and its applications. Nowadays, the requirement for higher food grain productivity and safety, enhanced plant yield, fertility of soil properties, and agricultural sustainability are upregulating. The research demonstrations are shifting toward soil rhizospheric-bio-based engineering to facilitate a better pollution-free environment for combining plants and rhizobacteria. The application of PRs is more beneficial in overcoming stressed conditions besides providing other significant direct and indirect ways to upregulate overall plant responses. PRs are more convenient, economical, and eco-enviro-friendly and can be applied in small cultivating areas to large fields. Variations in the modifications of plant responses under stress have been observed in inoculated plants, and these variations are dependent on the PRs mode of action, which represents the multifactorial processes regulated in stressful environments. The positive symbiotic associanship that plants develop with microbial physiology is fundamental for the plant development, especially in terms of biotic and abiotic stresses. It is necessary to set up deeply extensive field research demonstrations to understand better the interaction between the PRs-mediated signal and the metabolic/molecular reprogramming that improves plant tolerance to unfavorable environmental variables. Multi-strain bacterial strains can be substantial if a single strain of bacteria is not more significant in reducing stress resistance efficiency. The application, duration, and applicability of inoculation are more crucial as unmanaged methods may lead to consistent and correct results. Its successful agro-commercialization will based on the involvement of plant physiologists, plant biologists, plant pathologists, biotechnologists, agro-industrialists, and farmers. A better and deep understanding of the action mechanisms and interactions of plants and associated plant rhizobacteria directly in the matrix of interest can be favored by the adoption of a holistic approach that uses “omic” applications.
Author contributions
KV: Conceptualization, Data curation, Formal analysis, Methodology, Resources, Software, Validation, Writing – original draft. AJ: Conceptualization, Data curation, Formal analysis, Software, Writing – original draft. X-PS: Conceptualization, Data curation, Funding acquisition, Investigation, Project administration, Software, Supervision, Validation, Visualization, Writing – review & editing. QL: Data curation, Formal analysis, Funding acquisition, Resources, Software, Writing – review & editing. LX: Data curation, Formal analysis, Resources, Software, Writing – review & editing. H-rH: Data curation, Formal analysis, Resources, Software, Writing – review & editing. K-CW: Data curation, Funding acquisition, Resources, Software, Supervision, Writing – review & editing. CS: Data curation, Formal analysis, Resources, Software, Writing – review & editing. JA: Data curation, Formal analysis, Resources, Software, Writing – review & editing. Y-RL: Conceptualization, Funding acquisition, Investigation, Methodology, Project administration, Resources, Supervision, Validation, Visualization, Writing – review & editing.
Funding
The author(s) declare financial support was received for the research, authorship, and/or publication of this article. This research was financially supported by the Guangxi Key R & D Program (Guike AB24010140), Guangxi Natural Science Foundation (2021GXNSFAA220022; 2023GXNSFAA026459; 2023GXNSFAA026460), Guangxi Innovation Teams of Modern Agriculture Technology (nycytxgxcxtd-2021–03), Guangxi Characteristic Crop Experimental Station (GTS2022022), National Key Research and Development Project (2022YFD2301102–07), The National Natural Science Foundation of China (31760415; 32060468), Fund of Guangxi Academy of Agricultural Sciences (2021YT011), Science and Technology Major Project of Guangxi (Guike AA22117002–1), Fundamental Research Fund of Guangxi Academy of Agriculture Sciences (2023YM55) and Key Research and Development Program of Nanning (20232060).
Acknowledgments
The authors would like to thank the Guangxi Academy of Agricultural Sciences, Nanning, Guangxi, China, for providing the necessary facilities for this study.
Conflict of interest
The authors declare that the research was conducted in the absence of any commercial or financial relationships that could be construed as a potential conflict of interest.
Publisher’s note
All claims expressed in this article are solely those of the authors and do not necessarily represent those of their affiliated organizations, or those of the publisher, the editors and the reviewers. Any product that may be evaluated in this article, or claim that may be made by its manufacturer, is not guaranteed or endorsed by the publisher.
References
Abdelkefi, N., Louati, I., Mechichi, H. Z., Sayahi, N., El-Sayed, W. S., El Nayal, A., et al. (2024). Enhanced salt stress tolerance in tomato plants following inoculation with newly isolated plant growth-promoting rhizobacteria. Sci. Horticult. 328, 112921. doi: 10.1016/j.scienta.2024.112921
Acuna, J. J., Rilling, J. I., Inostroza, N. G., Zhang, Q., Wick, L. Y., Sessitsch, A., et al. (2024). Variovorax sp. strain P1R9 applied individually or as part of bacterial consortia enhances wheat germination under salt stress conditions. Sci. Rep. 14, 2070. doi: 10.1038/s41598–024-52535–0
Ahmad, H. M., Wang, X., Ijaz, M., Oranab, S., Ali, M. A., Fiaz, S. (2022). Molecular aspects of microRNAs and phytohormonal signaling in response to drought stress: A review. Curr. Issues Mol. Biol. 44, 3695–3710. doi: 10.3390/cimb44080253
Ahmad, M., Imtiaz, M., Nawaz, M. S., Mubeen, F., Sarwar, Y., Hayat, M., et al. (2023). Thermotolerant PGPR consortium B3P modulates physio-biochemical and molecular machinery for enhanced heat tolerance in maize during early vegetative growth. Ann. Microbiol. 73, 34. doi: 10.1186/s13213–023-01736–5
Al-Shwaiman, H. A., Shahid, M., Elgorban, A. M., Siddique, K. H., Syed, A. (2022). Beijerinckia fluminensis BFC-33, a novel multi-stress-tolerant soil bacterium: Deciphering the stress amelioration, phytopathogenic inhibition and growth promotion in Triticum aestivum (L.). Chemosphere 295, 133843. doi: 10.1016/j.chemosphere.2022.133843
Alsiary, W. A., AbdElgawad, H., Madany, M. M. (2023). How could actinobacteria augment the growth and redox homeostasis in barley plants grown in TiO2NPs-contaminated soils? A growth and biochemical study. Plant Physiol. Biochem 202, 107943. doi: 10.1016/j.plaphy.2023.107943
Amaral, J. ,. M., Lobo, A. K., Carmo-Silva, E. (2023). Regulation of Rubisco activity in crops. New Phytol. 241, 35–51. doi: 10.1111/nph.19369
Arora, S., Jha, P. N. (2023). Drought-tolerant Enterobacterbugandensis WRS7 induces systemic tolerance in Triticum aestivum L. (Wheat) under drought conditions. J. Plant Growth Regul. 42, 7715–7730. doi: 10.1007/s00344–023-11044–6
Arora, M., Kaushik, A., Rani, N., Kaushik, C. P. (2010). Effect of cyanobacterial exopolysaccharides on salt stress alleviation and seed germination. J. Environ. Biol. 31, 701–704.
Asif, S., Jan, R., Kim, N., Asaf, S., Lubna, Khan, M. A., et al. (2023). Halotolerantendophytic bacteria alleviate salinity stress in rice (Oryza sativa L.) by modulating ion content, endogenous hormones, the antioxidant system and gene expression. BMC Plant Biol. 23, 494. doi: 10.1186/s12870–023-04517-z
Backer, R., Rokem, J. S., Ilangumaran, G., Lamont, J., Praslickova, D., Ricci, E., et al. (2018). Plant growth-promoting rhizobacteria: Context, mechanisms of action, and roadmap to commercialization of biostimulants for sustainable agriculture. Front. Plant Sci. 9. doi: 10.3389/fpls.2018.01473
Backes, A., Charton, S., Planchon, S., Esmaeel, Q., Sergeant, K., Hausman, J. F., et al. (2021). Gene expression and metabolite analysis in barley inoculated with net blotch fungus and plant growth-promoting rhizobacteria. Plant Physiol. Biochem. 168, 488–500. doi: 10.1016/j.plaphy.2021.10.027
Bai, Y., Zhou, Y., Yue, T., Huang, Y., He, C., Jiang, W., et al. (2023). Plant growth-promoting rhizobacteriaBacillus velezensis JB0319 promotes lettuce growth under salt stress by modulating plant physiology and changing the rhizosphere bacterial community. Environ. Exp. Bot. 213, 105451. doi: 10.1016/j.envexpbot.2023.105451
Basu, S., Kumari, S., Subhadarshini, P., Rishu, A. K., Shekhar, S., Kumar, G. (2023). Plant growth promoting rhizobacterium Bacillus sp. BSE01 alleviates salt toxicity in chickpea (Cicer arietinum L.) by conserving ionic, osmotic, redox and hormonal homeostasis. Physiol. Plantarum. 175, e14076. doi: 10.1111/ppl.14076
Belhassan, M., Farhat, A., Abed, H. E., Chaabeen, Z., Bouzid, F., Elleuch, A., et al. (2024). Isolation and identification of a new Bacillus glycinifermentans strain from date palm rhizosphere and its effect on barley seeds under heavy metal stress. Braz. J. Microbiol. 55, 843–854. doi: 10.1007/s42770–024-01263–8
Bharti, N., Barnawal, D., Awasthi, A., Yadav, A., Kalra, A. (2014). Plant growth promoting rhizobacteria alleviate salinity induced negative effects on growth, oil content and physiological status in Mentha arvensis. Acta Physiol. Plant 36, 45–60. doi: 10.1007/s11738–013-1385–8
Bharti, N., Pandey, S. S., Barnawal, D., Patel, V. K., Kalra, A. (2016). Plant growth promoting rhizobacteria Dietzia natronolimnaea modulates the expression of stress responsive genes providing protection of wheat from salinity stress. Sci. Rep. 6, 34768. doi: 10.1038/srep34768
Bhat, B. A., Tariq, L., Nissar, S., Islam, S. T., Islam, S. U., Mangral, Z., et al. (2022). The role of plant-associated rhizobacteria in plant growth, biocontrol and abiotic stress management. J. Appl. Microbiol. 133, 2717–2741. doi: 10.1111/jam.15796
Bhatta, D., Adhikari, A., Kang, S. M., Kwon, E. H., Jan, R., Kim, K. M., et al. (2023). Hormones and the antioxidant transduction pathway and gene expression, mediated by Serratiamarcescens DB1, lessen the lethality of heavy metals (As, Ni, and Cr) in Oryza sativa L. Ecotoxicol. Environ. Saf. 263, 115377. doi: 10.1016/j.ecoenv.2023.115377
Brambilla, S., Stritzler, M., Soto, G., Ayub, N. (2022). A synthesis of functional contributions of rhizobacteria to growth promotion in diverse crops. Rhizosphere 24, 100611. doi: 10.1016/j.rhisph.2022.100611
Calvo, P., Zebelo, S., McNear, D., Kloepper, J., Fadamiro, H. (2019). Plant growth-promoting rhizobacteria induce changes in Arabidopsis thaliana gene expression of nitrate and ammonium uptake genes. J. Plant Interact. 14, 224–231. doi: 10.1080/17429145.2019.1602887
Chandra, P., Khobra, R., Sundha, P., Chandra, A., Sharma, R. K., Singh, G. P. (2024). Bacillus subtilis strain BS87 as a biocontrol agent against spot blotch disease: effect on growth, nutrient status, and antioxidant enzymes in wheat. Acta Physiol. Plant 46, 35. doi: 10.1007/s11738–024-03657–5
Chen, L., Liu, Y., Wu, G., Veronican Njeri, K., Shen, Q., Zhang, N., et al. (2016). Induced maize salt tolerance by rhizosphere inoculation of Bacillus amyloliquefaciens SQR9. Physiol. Plant 158, 34–44. doi: 10.1111/ppl.12441
Chieb, M., Gachomo, E. W. (2023). The role of plant growth promoting rhizobacteria in plant drought stress responses. BMC Plant Biol. 23, 407. doi: 10.1186/s12870–023-04403–8
Danish, S., Zafar-Ul-Hye, M., Mohsin, F., Hussain, M. (2020). ACC-deaminase producing plant growth promoting rhizobacteria and biochar mitigate adverse effects of drought stress on maize growth. PloS One 15, 1–14. doi: 10.1371/journal.pone.0230615
Dardanelli, M. S., Fernandez de Cordoba, F. J., Espuny, M. R., Carvajal, M. A. R., Diaz, M. E. S., Serrano, A. M. G., et al. (2008). Effect of Azospirillum brasilense coinoculated with Rhizobium on Phaseolus vulgaris flavonoids and Nod factor production under salt stress. Soil Biol. Biochem. 40, 2713–2721. doi: 10.1016/j.soilbio.2008.06.016
Delfini, R., Belgoff, C., Fernández, E., Fabra, A., Castro, S. (2010). Symbiotic nitrogen fixation and nitrate reduction in two peanut cultivars with different growth habit and branching pattern structures. Plant Growth Regul. 61, 153–159. doi: 10.1007/s10725–010-9461–1
Dell’Amico, E., Cavalca, L., Andreoni, V. (2008). Improvement of Brassica napus growth under cadmium stress by cadmium resistant rhizobacteria. Soil Biol. Biochem. 40, 74–84. doi: 10.1016/j.soilbio.2007.06.024
Dimkpa, C. O., Merten, D., Svatos, A., Büchel, G., Kothe, E. (2009). Siderophores mediate reduced and increased uptake of cadmium by Streptomyces tendae F4 and sunflower (Helianthus annuus), respectively. J. Appl. Microbiol. 107, 1687–1696. doi: 10.1111/j.1365–2672.2009.04355.x
dos Santos, R. M., Diaz, P. A. E., Lobo, L. L. B., Rigobelo, E. C. (2020). Use of plant growth-promoting rhizobacteria in maize and sugarcane: characteristics and applications. Front. Sustain. Food Syst. 4. doi: 10.3389/fsufs.2020.00136
Egamberdieva, D., Wirth, S. J., Alqarawi, A. A., Abd-Allah, E. F., Hashem, A. (2017). Phytohormones and beneficial microbes: essential components for plants to balance stress and fitness. Front. Microbiol. 8, e02104. doi: 10.3389/fmicb.2017.02104
Ekinci, M., Turan, M., Yildirim, E., Güneş, A., Kotan, R., Dursun, A. (2014). Effect of plant growth promoting rhizobacteria on growth, nutrient, organic acid, amino acid and hormone content of cauliflower (Brassica oleracea L. var. botrytis) transplants. Acta Sci. Pol. Hortorum. Cultus. 13, 71–85.
El-Esawi, M. A., Alaraidh, I. A., Alsahli, A. A., Alzahrani, S. M., Ali, H. M., Alayafi, A. A., et al. (2018). Serratia liquefaciens KM4 Improves salt stress tolerance in maize by regulating redox potential, ion homeostasis, leaf gas exchange and stress-related gene expression. Int. J. Mol. Sci. 19 (11), 3310. doi: 10.3390/ijms19113310
El-Esawi, M. A., Elkelish, A., Soliman, M., Elansary, H. O., Zaid, A., Wani, S. H. (2020). Serratia marcescens BM1 enhances cadmium stress tolerance and phytoremediation potential of soybean through modulation of osmolytes, leaf gas exchange, antioxidant machinery, and stress-responsive genes expression. Antioxidants 9 (1), 43. doi: 10.3390/antiox9010043
El-Sersawy, M. M., Hassan, S. E. D., El-Ghamry, A. A., El-Gwad, A. M. A., Fouda, A. (2021). Implication of plant growth-promoting rhizobacteria of Bacillus spp. as biocontrol agents against wilt disease caused by Fusarium oxysporum Schlecht. In Vicia faba L. Biomol. Concepts. 12, 197–214. doi: 10.1515/bmc-2021–0020
Enebe, M. C., Babalola, O. O. (2018). The influence of plant growth-promoting rhizobacteria in plant tolerance to abiotic stress: A survival strategy. Appl. Microbiol. Biotechnol. 102, 7821–7835. doi: 10.1007/s00253–018-9214-z
Etesami, H., Alikhani, H. A., Hosseini, H. M. (2015). Indole-3-acetic acid (IAA) production trait, a useful screening to select endophytic and rhizosphere competent bacteria for rice growth promoting agents. Methods X. 2, 72–78. doi: 10.1016/j.mex.2015.02.008
Etesami, H., Maheshwari, D. K. (2018). Use of plant growth promoting rhizobacteria (PRs) with multiple plant growth promoting traits in stress agriculture: Action mechanisms and future prospects. Ecotoxicol. Environ. Saf. 156, 225–246. doi: 10.1016/j.ecoenv.2018.03.013
Fadiji, A. E., Orozco-Mosqueda, C., Santoyo, G., Babalola, O. O. (2022). Recent developments in the application of plant growth-promoting drought adaptive rhizobacteria for drought mitigation. Plants 11 (22), 3090. doi: 10.3390/plants11223090
Fahad, S., Hussain, S., Bano, A., Saud, S., Hassan, S., Shan, D., et al. (2015). Potential role of phytohormones and plant growth-promoting rhizobacteria in abiotic stresses: consequences for changing environment. Environ. Sci. Poll. Res. 22, 4907–4921. doi: 10.1007/s11356–014-3754–2
Fasusi, O. A., Babalola, O. O., Adejumo, T. O. (2023). Harnessing of plant growth-promoting rhizobacteria and arbuscular mycorrhizal fungi in agroecosystem sustainability. CABI. Agric. Biosci. 4, 26. doi: 10.1186/s43170–023-00168–0
Ferioun, M., Srhiouar, N., Tirry, N., Belahcen, D., Siang, T. C., Louahlia, S., et al. (2023). Optimized drought tolerance in barley (Hordeumvulgare L.) using plant growth-promoting rhizobacteria (PGPR). Biocatalysis. Agricul. Biotechnol. 50, 102691. doi: 10.1016/j.bcab.2023.102691
Ferrante, R., Campagni, C., Vettori, C., Checcucci, A., Garosi, C., Paffetti, D. (2023). Meta-analysis of plant growth-promoting rhizobacteria interaction with host plants: Implications for drought stress response gene expression. Front. Plant Sci. 14. doi: 10.3389/fpls.2023.1282553
Ferreira, E., Martins, L. M. V., Xavier, G. R., Rumjanek, N. G. (2011). Nodulation and grain yield by cowpea (Vigna unguiculata L. Walp.) inoculated with rhizobia, isolates. Rev. Caatinga. 24, 27–35.
Fu, Q., Liu, C., Ding, N., Lin, Y., Guo, B. (2010). Ameliorative effects of inoculation with the plant growth-promoting rhizobacterium Pseudomonas sp. DW1 on growth of eggplant (Solanum melongena L.) seedlings under salt stress. Agricul. Water Manage. 97, 1994–2000. doi: 10.1016/j.agwat.2010.02.003
Gao, X., Luan, J., Wang, L., Li, H., Wang, Q., Wang, Z., et al. (2023). Effect of the Plant Growth Promoting Rhizobacterium, Cronobacter sp. Y501, for Enhancing Drought Tolerance in Maize (Zea mays L.). J. Soil Sci. Plant Nutr. 23, 2786–2797. doi: 10.1007/s42729–023-01234–2
García-Fraile, P., Menéndez, E., Rivas, R. (2015). Role of bacterial biofertilizers in agriculture and forestry. AIMS. Bioeng. 2, 183–205. doi: 10.3934/bioeng.2015.3.183
Ghosh, P. K., De, T. K., Maiti, T. K. (2018). Role of ACC deaminase as a stress ameliorating enzyme of plant growth-promoting rhizobacteria useful in stress agriculture: A review. In: Meena, V., (eds). Role of rhizospheric microbes in soil. (Springer, Singapore). 57–106. doi: 10.1007/978–981-10–8402-7_3
Glick, B. R., Cheng, Z., Czarny, J., Duan, J. (2007). “Promotion of plant growth by ACC deaminase-producing soil bacteria,” in New Perspectives and Approaches in Plant Growth-Promoting Rhizobacteria Research. Eds. Bakker, P. A. H. M., Raaijmakers, J. M., Bloemberg, G., Höfte, M., Lemanceau, P., Cooke, B. M. (Springer, Dordrecht). doi: 10.1007/978–1-4020–6776-1_8
Gomez-Godínez, L. J., Aguirre-Noyola, J. L., Martínez-Romero, E., Arteaga-Garibay, R. I., Ireta-Moreno, J., Ruvalcaba-Gómez, J. M. (2023). A look at plant-growth-promoting bacteria. Plants 12 (8), 1668. doi: 10.3390/plants12081668
Goswami, M., Deka, S. (2020). Plant growth-promoting rhizobacteria—alleviators of abiotic stresses in soil: a review. Pedosphere 30, 40–61. doi: 10.1016/S1002–0160(19)60839–8
Grichko, V. P., Glick, B. R. (2001). Amelioration of flooding stress by ACC deaminase containing plant growth promoting bacteria. Can. J. Microbiol. 47, 77–80. doi: 10.1016/S0981–9428(00)01212–2
Grover, M., Bodhankar, S., Sharma, A., Sharma, P., Singh, J., Nain, L. (2021). PGPR mediated alterations in root traits: way toward sustainable crop production. Front. Sustain. Food Syst. 4. doi: 10.3389/fsufs.2020.618230
Gujral, M. S., Agrawal, P., Khetmalas, M. B., Pandey, R. (2013). Colonization and plant growth promotion of Sorghum seedlings by endorhizospheric Serratia sp. Acta Biol. Indica. 2, 343–352.
Gupta, S., Pandey, S. (2019). ACC deaminase producing bacteria with multifarious plant growth promoting traits alleviates salinity stress in french bean (Phaseolus vulgaris) plants. Front. Microbiol. 10. doi: 10.3389/fmicb.2019.01506
Habib, S. H., Kausar, H., Saud, H. M. (2016). Plant growth-promoting rhizobacteria enhance salinity stress tolerance in okra through ROS-scavenging enzymes. BioMed. Res. Int. 2016, 6284547. doi: 10.1155/2016/6284547
Halo, B. A., Khan, A. L., Waqas, M., Al-Harrasi, A., Hussain, J., Ali, L., et al. (2015). Endophytic bacteria (Sphingomonas sp. LK11) and gibberellin can improve Solanum lycopersicum growth and oxidative stress under salinity. J. Plant Interact. 10, 117–125. doi: 10.1080/17429145.2015.1033659
Han, Q. Q., Lu, X. P., Bai, J. P., Qiao, Y., Pare, P. W., Wang, S. M., et al. (2014). Beneficial soil bacterium Bacillus subtilis (GB03) augments salt tolerance of white clover. Front. Plant Sci. 5. doi: 10.3389/fpls.2014.00525
Herrera-Medina, M. J., Steinkellner, S., Vierheilig, H., Ocampo Bote, J. A., García Garrido, J. M. (2007). Abscisic acid determines arbuscule development and functionality in the tomato arbuscular mycorrhiza. New Phytol. 175, 554–564. doi: 10.1111/j.1469–8137.2007.02107.x
Islam, F., Yasmeen, T., Ali, Q., Ali, S., Arif, M. S., Hussain, S., et al. (2014). Influence of Pseudomonas aeruginosa as PGPR on oxidative stress tolerance in wheat under Zn stress. Ecotoxicol. Environ. Saf. 104, 285–293. doi: 10.1016/j.ecoenv.2014.03.008
Issa, A., Esmaeel, Q., Sanchez, L., Courteaux, B., Guise, J., Gibon, Y., et al. (2018). Impacts of Paraburkholderia phytofirmans Strain PsJN on Tomato (Lycopersicon esculentum L.) Under High Temperature. Front. Plant Sci. 9. doi: 10.3389/fpls.2018.01397
Jalmi, S. K., Sinha, A. K. (2022). Ambiguities of PGPR-induced plant signaling and stress management. Front. Microbiol. 13. doi: 10.3389/fmicb.2022.899563
Jha, Y., Mohamed, H. I. (2023a). Enhancement of disease resistance, growth potential, and biochemical markers in maize plants by inoculation with plant growth-promoting bacteria under biotic stress. J. Plant Pathol. 105, 729–748. doi: 10.1007/s42161–023-01338–9
Jha, Y., Mohamed, H. I. (2023b). Inoculation with Lysinibacillus fusiformis strain YJ4 and Lysinibacillus sphaericus strain YJ5 alleviates the effects of cold stress in maize plants. Gesunde. Pflanzen. 75, 77–95. doi: 10.1007/s10343–022-00666–7
Joshi, A., Rajput, V. D., Verma, K. K., Minkina, T., Ghazaryan, K., Arora, J. (2023). Potential of Suaeda nudiflora and Suaeda fruticosa to adapt to high salinity conditions. Horticult 9, 74. doi: 10.3390/horticulturae9010074
Joshi, A., Verma, K. K., Rajput, V. D., Minkina, T., Arora, J. (2022). Recent advances in metabolic engineering of microorganisms for advancing lignocellulose-derived biofuels. Bioengineered 13, 8135–8163. doi: 10.1080/21655979.2022.2051856
Kang, S. M., Khan, A. L., Waqas, M., You, Y. H., Hamayun, M., Joo, G. J., et al. (2015). Gibberellin-producing Serratia nematodiphila PEJ1011 ameliorates low temperature stress in Capsicum annum L. Eur. J. Soil Biol. 68, 85–93. doi: 10.1016/j.ejsobi.2015.02.005
Kang, S. M., Shahzad, R., Bilal, S., Khan, A. L., Park, Y. G., Lee, K. E., et al. (2019). Indole-3-acetic-acid and ACC deaminase producing Leclercia adecarboxylata MO1 improves Solanum lycopersicum L. growth and salinity stress tolerance by endogenous secondary metabolites regulation. BMC Microbiol. 19, 80. doi: 10.1186/s12866–019-1450–6
Kaushal, M., Wani, S. P. (2016). Rhizobacterial-plant interactions: Strategies ensuring plant growth promotion under drought and salinity stress. Agricult. Ecosyst. Environ. 231, 68–78. doi: 10.1016/j.agee.2016.06.031
Khan, N., Ali, S., Shahid, M. A., Mustafa, A., Sayyed, R. Z., Curá, J. A. (2021). Insights into the interactions among roots, rhizosphere, and rhizobacteria for improving plant growth and tolerance to abiotic stresses: A review. Cells 10, 1551. doi: 10.3390/cells10061551
Khan, V., Umar, S., Iqbal, N. (2023). Palliating salt stress in mustard through plant-Growth-Promoting rhizobacteria: Regulation of secondary metabolites, osmolytes, antioxidative enzymes and stress ethylene. Plants 12, 705. doi: 10.3390/plants12040705
Khoshru, B., Mitra, D., Joshi, K., Adhikari, P., Islam Rion, M. S., Fadiji, A. E., et al. (2023). Decrypting the multi-functional biological activators and inducers of defense responses against biotic stresses in plants. Heliyon 9 (3), e13825. doi: 10.1016/j.heliyon.2023.e13825
Khoso, M. A., Wagan, S., Alam, I., Hussain, A., Ali, Q., Saha, S., et al. (2024). Impact of plant growth-promoting rhizobacteria (PGPR) on plant nutrition and root characteristics: Current perspective. Plant Stress 11, 100341. doi: 10.1016/j.stress.2023.100341
Kiruthika, A., Vikram, K. V., Nivetha, N., Asha, A. D., Chinnusamy, V., Kumar, A., et al. (2023). Influence of thermotolerant rhizobacteria bacillus spp. on biochemical attributes and antioxidant status of mustard under high temperature stress. Curr. Microbiol. 80, 169. doi: 10.1007/s00284–023-03273–5
Konappa, N., Krishnamurthy, S., Arakere, U. C., Chowdappa, S., Ramachandrappa, N. S. (2020). Efficacy of indigenous plant growth-promoting rhizobacteria and Trichodermastrains in eliciting resistance against bacterial wilt in a tomato. Egyptian. J. Biol. Pest Control. 30, 1–13. doi: 10.1186/s41938–020-00303–3
Kumar, A., Patel, J. S., Meena, V. S., Srivastava, R. (2019). Recent advances of PGPR based approaches for stress tolerance in plants for sustainable agriculture. Biocatalysis. Agricult. Biotechnol. 20, 101271. doi: 10.1016/j.bcab.2019.101271
Kumar, S., Sindhu, S. S., Kumar, R. (2021). Biofertilizers: An ecofriendly technology for nutrient recycling and environmental sustainability. Curr. Res. Microbial. Sci. 3, 100094. doi: 10.1016/j.crmicr.2021.100094
Kumari, P., Khanna, V. (2016). Biodiversity of Pseudomonas and Bacillus possessing both bioantagonistic and plant growth promoting traits in chickpea rhizosphere. Int. J. Sci. Nat. 7, 153–158.
Lephatsi, M. M., Meyer, V., Piater, L. A., Dubery, I. A., Tugizimana, F. (2021). Plant responses to abiotic stresses and rhizobacterial biostimulants: metabolomics and epigenetics perspectives. Metabolites 11 (7), 457. doi: 10.3390/metabo11070457
Li, H., Lei, P., Pang, X., Li, S., Xu, H., Xu, Z., et al. (2017). Enhanced tolerance to salt stress in canola (Brassica napus L.) seedlings inoculated with the halotolerant Enterobacter cloacae HSNJ4. Appl. Soil Ecol. 119, 26–34. doi: 10.1016/j.apsoil.2017.05.033
Liu, X., Chai, J., Zhang, Y., Zhang, C., Lei, Y., Li, Q., et al. (2022). Halotolerant rhizobacteria mitigate the effects of salinity stress on maize growth by secreting exopolysaccharides. Environ. Exp. Bot. 204, 105098. doi: 10.1016/j.envexpbot.2022.105098
Lu, L., Liu, N., Fan, Z., Liu, M., Zhang, X., Tian, J., et al. (2024). A novel PGPR strain, Streptomyces lasalocidi JCM 3373, alleviates salt stress and shapes root architecture in soybean by secreting indole-3-carboxaldehyde. Plant. Cell Environ. 47 (6), 1941–1956. doi: 10.1111/pce.14847
Ma, J., Peng, J., Tian, S., He, Y., Zhang, C., Jia, N., et al. (2023). Streptomyces sp. TOR3209 alleviates cold stress in tomato plants. New Z. J. Crop Horticult. Sci. 51, 662–682. doi: 10.1080/01140671.2022.2066141
Mahmud, K., Missaoui, A., Lee, K., Ghimire, B., Presley, H. W., Makaju, S. (2021). Rhizosphere microbiome manipulation for sustainable crop production. Curr. Plant Biol. 27, 100210. doi: 10.1016/j.cpb.2021.100210
Meena, K. K., Sorty, A. M., Bitla, U., Shinde, A. L., Kumar, S., Wakchaure, G. C., et al. (2023). Stress-responsive gene regulation conferring salinity tolerance in wheat inoculated with ACC deaminase producing facultative methylotrophicactinobacterium. Front. Plant Sci. 14. doi: 10.3389/fpls.2023.1249600
Mellidou, I., Ainalidou, A., Papadopoulou, A., Leontidou, K., Genitsaris, S., Karagiannis, E., et al. (2021). Comparative transcriptomics and metabolomics reveal an intricate priming mechanism involved in PGPR-mediated salt tolerance in tomato. Front. Plant Sci. 12. doi: 10.3389/fpls.2021.713984
Mellidou, I., Karamanoli, K. (2022). Unlocking PGPR-mediated abiotic stress tolerance: what lies beneath. Front. Sustain. Food Syst. 6. doi: 10.3389/fsufs.2022.832896
Méndez-Gómez, M., Castro-Mercado, E., López-Bucio, J., García-Pineda, E. (2021). Azospirillum brasilense Sp245 triggers cytokinin signaling in root tips and improves biomass accumulation in Arabidopsis through canonical cytokinin receptors. Physiol. Mol. Biol. Plants 27, 1639–1649. doi: 10.1007/s12298–021-01036–9
Mishra, J., Singh, R., Arora, N. K. (2017). Alleviation of heavy metal stress in plants and remediation of soil by rhizosphere microorganisms. Front. Microbiol. 8. doi: 10.3389/fmicb.2017.01706
Mitra, D., Mondal, R., Khoshru, B., Shadangi, S., Das Mohapatra, P. K., Panneerselvam, P. (2021). Rhizobacteria mediated seed bio-priming triggers the resistance and plant growth for sustainable crop production. Curr. Res. Microb. Sci. 2, 100071. doi: 10.1016/j.crmicr.2021.100071
Mohanty, P., Singh, P. K., Chakraborty, D., Mishra, S., Pattnaik, R. (2021). Insight into the role of PGPR in sustainable agriculture and environment. Front. Sustain. Food Syst. 5. doi: 10.3389/fsufs.2021.667150
Moustaine, M., Elkahkahi, R., Benbouazza, A., Benkirane, R., Achbani, E. (2017). Effect of plant growth promoting rhizobacterial (PGPR) inoculation on growth in tomato (Solanum lycopersicum L.) and characterization for direct PGP abilities in Morocco. Int. J. Environ. Agricult. Biotechnol. 2, 590–596. doi: 10.22161/ijeab/2.2.5
Mukhtar, T., Ali, F., Rafique, M., Ali, J., Afridi, M. S., Smith, D., et al. (2023). Biochemical Characterization and Potential of Bacillus safensis Strain SCAL1 to Mitigate Heat Stress in Solanum lycopersicum L. J. Plant Growth Regul. 42, 523–538. doi: 10.1007/s00344–021-10571–4
Nadeem, S. M., Zahir, Z. A., Naveed, M., Arshad, M. (2007). Preliminary investigations on inducing salt tolerance in maize through inoculation with rhizobacteria containing ACC deaminase activity. Can. J. Microbiol. 53, 1141–1149. doi: 10.1139/W07–081
Namwongsa, J., Jogloy, S., Vorasoot, N., Boonlue, S., Riddech, N., Mongkolthanaruk, W. (2019). Endophytic bacteria improve root traits, biomass and yield of Helianthus tuberosus L. Under normal and deficit water conditions. J. Microbiol. Biotechnol. 29, 1777–1789. doi: 10.4014/jmb.1903.03062
Naveed, M., Mitter, B., Reichenauer, T. G., Wieczorek, K., Sessitsch, A. (2014). Increased drought stress resilience of maize through endophytic colonization by Burkholderia phytofirmans PsJN and Enterobacter sp. FD17. Environ. Exp. Bot. 97, 30–39. doi: 10.1016/j.envexpbot.2013.09.014
Nikhil, P. T., Faiz, U., Mohapatra, S. (2023). The drought-tolerant rhizobacterium, Pseudomonas putida AKMP7, suppresses polyamine accumulation under well-watered conditions and diverts putrescine into GABA under water-stress, in Oryza sativa. Environ. Exp. Bot. 211, 105377. doi: 10.1016/j.envexpbot.2023.105377
Noorieh, B., Arzanesh, M. H., Mahlegha, G., Maryam, S. (2013). The effect of plant growth promoting rhizobacteria on growth parameters, antioxidant enzymes and microelements of canola under salt stress. J. Appl. Environ. Biol. Sci. 3, 17–27.
Olenska, E., Małek, W., Wójcik, M., Swiecicka, I., Thijs, S., Vangronsveld, J. (2020). Beneficial features of plant growth-promoting rhizobacteria for improving plant growth and health in challenging conditions: a methodical review. Sci. Total. Environ. 743, e14068. doi: 10.1016/j.scitotenv.2020.140682
Orozco-Mosqueda, M. D. C., Glick, B. R., Santoyo, G. (2020). ACC deaminase in plant growth-promoting bacteria (PGPB): An efficient mechanism to counter salt stress in crops. Microbiol. Res. 235, 126439. doi: 10.1016/j.micres.2020.126439
Palaniyandi, S. A., Damodharan, K., Yang, S. H., Suh, J. W. (2014). Streptomyces sp. strain PGPA39 alleviates salt stress and promotes growth of 'Micro Tom' tomato plants. J. Appl. Microbiol. 117, 766–773. doi: 10.1111/jam.12563
Patil, N., Raghu, S., Mohanty, L., Jeevan, B., Basana-Gowda, G., Adak, T., et al. (2023). Rhizosphere bacteria isolated from medicinal plants improve rice growth and induce systemic resistance in host against pathogenic fungus. J. Plant Growth Regul. 43, 770–786. doi: 10.1007/s00344–023-11137–2
Perez, A., Rossano, S., Trcera, N., Verney-Carron, A., Rommevaux, C., Fourdrin, C., et al. (2019). Direct and indirect impact of the bacterial strain Pseudomonas aeruginosa on the dissolution of synthetic Fe (III)-and Fe (II)-bearing basaltic glasses. Chem. Geol. 523, 9–18. doi: 10.1016/j.chemgeo.2019.05.033
Rakshapal, S., Sumit, K. S., Rajendra, P. P., Alok, K. (2013). Technology for improving essential oil yield of Ocimum basilicum L. (sweet basil) by application of bioinoculant colonized seeds under organic field conditions. Ind. Crop Prod. 45, 335–342. doi: 10.1016/j.indcrop.2013.01.003
Raza, A., Hassan, A., Akram, W., Anjum, T., Ali, B. (2024). Seed coating with the synthetic consortium of beneficial Bacillus microbes improves seedling growth and manages Fusarium wilt disease. Sci. Horticult. 325, 112645. doi: 10.1016/j.scienta.2023.112645
Ren, L., Zhang, Y., Zhou, J. L., Wang, G., Mo, Y., Ling, Y., et al. (2024). RL-WG26 mediated salt stress tolerance in rice seedlings: A new insight into molecular mechanisms. Plant Stress 11, 100306. doi: 10.1016/j.stress.2023.100306
Rizvi, A., Ahmed, B., Umar, S., Khan, M. S. (2024). Comprehensive Insights into Sorghum (Sorghum bicolor) Defense Mechanisms Unveiled: Plant Growth-Promoting Rhizobacteria in Combating Burkholderia-Induced Bacterial Leaf Stripe Disease. Plant Stress. 11, 100397. doi: 10.1016/j.stress.2024.100397
Saikia, S. P., Dutta, S. P., Goswami, A., Bahau, B. S., Kanjilal, P. B. (2010). Role of Azospirillum in the improvement of legumes. In: Khan, M.S., Musarrat, J., Zaidi, A. (eds), Microbes for legume improvement. (Vienna: Springer). pp. 389–408. doi: 10.1007/978–3-211–99753-6_16
Saleem, A. R., Bangash, N., Mahmood, T., Khalid, A., Centritto, M., Siddique, M. T. (2015). Rhizobacteria capable of producing ACC deaminase promote growth of velvet bean (Mucuna pruriens) under water stress condition. Int. J. Agric. Biol. 17, 663–667. doi: 10.17957/IJAB/17.3.14.788
Saleena, L., Rangarajan, S., Nair, S. (2002). Diversity of azospirillum strains isolated from rice plants grown in saline and nonsaline sites of coastal agricultural ecosystem. Microb. Ecol. 44, 271–277. doi: 10.1007/s00248–002-2019–7
Salomon, M. V., Bottini, R., de Souza Filho, G. A., Cohen, A. C., Moreno, D., Gil, M., et al. (2014). Bacteria isolated from roots and rhizosphere of Vitis vinifera retard water losses, induce abscisic acid accumulation and synthesis of defense-related terpenes in in vitro cultured grapevine. Physiol. Plant 151, 359–374. doi: 10.1111/ppl.12117
Sarkar, J., Chakraborty, U., Chakraborty, B. (2021). High-temperature resilience in Bacillus safensis primed wheat plants: A study of dynamic response associated with modulation of antioxidant machinery, differential expression of HSPs and osmolyte biosynthesis. Environ. Exp. Bot. 182, 104315. doi: 10.1016/j.envexpbot.2020.104315
Sayahi, N., Djemal, R., Ben Merdes, K., Saidii, M. N., Yengui, M., Gdoura, R., et al. (2022). Characterization of Siccibacter sp. Strain C2 a novel rhizobacterium that enhances tolerance of barley to salt stress. Curr. Microbiol. 79, 239. doi: 10.1007/s00284–022-02930–5
Shaffique, S., Imran, M., Rahim, W., Alomrani, S. O., Yun, B. W., Lee, I. J. (2023). A newly isolated Bacillus pumilus strain SH-9 modulates response to drought stress in soybean via endogenous phytohormones and gene expression (Daegu, South Korea). Plant Stress 10, 100279. doi: 10.1016/j.stress.2023.100279
Shah, G. M., Ali, H., Ahmad, I., Kamran, M., Hammad, M., Shah, G. A., et al. (2022). Nano agrochemical zinc oxide influences microbial activity, carbon, and nitrogen cycling of applied manures in the soil-plant system. Environ. Poll. 293, 118559. doi: 10.1016/j.envpol.2021.118559
Shahid, M., Singh, U. B., Khan, M. S., Singh, P., Kumar, R., Singh, R. N., et al. (2023). Bacterial ACC deaminase: Insights into enzymology, biochemistry, genetics, and potential role in amelioration of environmental stress in crop plants. Front. Microbiol. 14. doi: 10.3389/fmicb.2023.1132770
Shahzad, A., Siddique, A., Qin, M., Aslam, U., Naeem, M., Shakoor, A. (2023). Heavy metals mitigation and growth promoting effect of endophytic Agrococcus terreus (MW 979614) in maize plants under zinc and nickel contaminated soil. Front. Microbiol. 14. doi: 10.3389/fmicb.2023.1255921
Sharma, A., Vaishnav, A., Jamali, H., Keswani, C., Srivastava, A. K., Kaushik, R., et al. (2023). Unraveling the plant growth-promoting mechanisms of stenotrophomonas sp. CV83 for drought stress tolerance and growth enhancement in chickpea. J. Plant Growth Regul. 42, 6760–6775. doi: 10.1007/s00344–023-11010–2
Sherin, G., Aswathi, K. R., Puthur, J. T. (2022). Photosynthetic functions in plants subjected to stresses are positively influenced by priming. Plant Stress 4, 100079. doi: 10.1016/j.stress.2022.100079
Singh, R. P., Jha, P. N. (2017). The PGPR stenotrophomonas maltophilia SBP-9 augments resistance against biotic and abiotic stress in wheat plants. Front. Microbiol. 8. doi: 10.3389/fmicb.2017.01945
Song, X. P., Verma, K. K., Tian, D. D., Zhang, X. Q., Liang, Y. J., Huang, X., et al. (2021). Exploration of silicon functions to integrate with biotic stress tolerance and crop improvement. Biol. Res. 54, 19. doi: 10.1186/s40659–021-00344–4
Spaepen, S., Vanderleyden, J., Remans, R. (2007). Indole-3-acetic acid in microbial and microorganism-plant signaling. FEMS Microbiol. Rev. 31, 425–448. doi: 10.1111/j.1574–6976.2007.00072.x
Sultana, S., Paul, S. C., Parveen, S., Alam, S., Rahman, N., Jannat, B., et al. (2020). Isolation and identification of salt-tolerant plant-growth-promoting rhizobacteria and their application for rice cultivation under salt stress. Can. J. Microbiol. 66, 144–160. doi: 10.1139/cjm-2019–0323
Swain, H., Adak, T., Mukherjee, A. K., Sarangi, S., Samal, P., Khandual, A., et al. (2021). Seed biopriming with trichoderma strains isolated from tree bark improves plant growth, antioxidative defense system in rice and enhance straw degradation capacity. Front. Microbiol. 12. doi: 10.3389/fmicb.2021.633881
Tanwir, K., Abbas, S., Hussaan, M., Basit, F., Alomrani, S. O. (2023). Bio-inoculation with Serratia CP-13 enhances Cd detoxification via modulation of phytohormone, gas exchange attributes and nutrient acquisition in maize cultivars cultivated in Cd-stressed soil. Plant Stress 10, 100290. doi: 10.1016/j.stress.2023.100290
Tariq, A., Ullah, I., Sardans, J., Graciano, C., Mussarat, S., Ullah, A., et al. (2023). Strigolactones can be a potential tool to fight environmental stresses in arid lands. Environ. Res. 229, 115966. doi: 10.1016/j.envres.2023.115966
Timmusk, S., Wagner, E. G. (1999). The plant-growth-promoting rhizobacterium Paenibacillus polymyxa induces changes in Arabidopsis thaliana gene expression: a possible connection between biotic and abiotic stress responses. Mol. Plant Microbe Interact. 12 (11), 951–959. doi: 10.1094/MPMI.1999.12.11.951
Tiwari, S., Lata, C., Chauhan, P. S., Nautiyal, C. S. (2016). Pseudomonas putida attunes morphophysiological, biochemical and molecular responses in Cicer arietinum L. during drought stress and recovery. Plant Physiol. Biochem. 99, 108–117. doi: 10.1016/j.plaphy.2015.11.001
Tiwari, S., Prasad, V., Chauhan, P. S., Lata, C. (2017). confers tolerance to various abiotic stresses and modulates plant response to phytohormones through osmoprotection and gene expression regulation in rice. Front. Plant Sci. 8. doi: 10.3389/fpls.2017.01510
Ullah, A., Bano, A., Khan, N. (2021). Climate change and salinity effects on crops and chemical communication between plants and plant growth-promoting microorganisms under stress. Front. Sustain. Food Syst. 5. doi: 10.3389/fsufs.2021.618092
Verma, K. K., Singh, P., Song, X. P., Malviya, M. K., Singh, R. K., Chen, G. L., et al. (2020b). Mitigating climate change for sugarcane improvement: role of silicon in alleviating abiotic stresses. Sugar. Tech. 22, 741–749. doi: 10.1007/s12355–020-00831–0
Verma, K. K., Song, X. P., Budeguer, F., Nikpay, A., Enrique, R., Singh, M., et al. (2022b). Genetic engineering: an efficient approach to mitigating biotic and abiotic stresses in sugarcane cultivation. Plant Signaling Behav. 17, e2108253. doi: 10.1080/15592324.2022.2108253
Verma, K. K., Song, X. P., Li, D. M., Singh, M., Rajput, V. D., Malviya, M. K., et al. (2020a). Interactive role of silicon and plant-rhizobacteria mitigating abiotic stresses: A new approach for sustainable agriculture and climate change. Plants 9, 1055. doi: 10.3390/plants9091055
Verma, K. K., Song, X. P., Li, D. M., Singh, M., Wu, J. M., Singh, R. K., et al. (2022c). Silicon and soil microorganisms improves rhizospheric soil health with bacterial community, plant growth, performance and yield. Plant Signaling Behav. 17, 2104004. doi: 10.1080/15592324.2022.2104004
Verma, K. K., Song, X. P., Singh, M., Tian, D. D., Rajput, V. D., Minkina, T., et al. (2023). “Association of silicon and soil microorganisms induces Stress Mitigation, Increasing Plant Productivity,” in Benefits of Silicon in the Nutrition of Plants. Ed. de Mello Prado, R. (Springer, Cham). doi: 10.1007/978–3-031–26673-7_17
Verma, K. K., Song, X. P., Tian, D. D., Guo, D. J., Chen, Z. L., Zhong, C. S., et al. (2021). Influence of silicon on biocontrol strategies to manage biotic stress for crop protection, performance and improvement. Plants 10, 2163. doi: 10.3390/plants10102163
Verma, K. K., Song, X. P., Yadav, G., Degu, H. D., Parvaiz, A., Singh, M., et al. (2022a). Impact of agroclimatic variables on proteo-genomics in sugarcane (Saccharum spp.) plant productivity. ACS Omega. 7, 22997–23008. doi: 10.1021/acsomega.2c01395
Wei, T., Sun, Y., Yashir, N., Li, X., Guo, J., Liu, X., et al. (2022). Inoculation with rhizobacteria enhanced tolerance of tomato (Solanum lycopersicum L.) plants in response to cadmium stress. J. Plant Growth Regul. 41, 445–460. doi: 10.1007/s00344–021-10315–4
Yildiz, H. N., Altinok, H. H., Dikilitas, M., Günacti, H., Ay, T. (2023). Suppression of tomato bacterial speck disease (Pseudomonas syringaepv. tomato (Okabe) Young, Dye, &Wilkie) via induced systemic resistance by Pseudomonas and Bacillus strains. Bot 101, 391–399. doi: 10.1139/cjb-2022–0066
Yue, H., Mo, W., Li, C., Zheng, Y., Li, H. (2007). The salt stress relief and growth promotion effect of RS-5 on cotton. Plant Soil 297, 139–145. doi: 10.1007/s11104–007-9327–0
Zahir, Z. A., Akhtar, S. S., Ahmad, M., Nadeem, S. M., Nadeem, S. (2012). Comparative effectiveness of Enterobacter aerogenes and Pseudomonas fluorescens for mitigating the depressing effect of brackish water on maize. Int. J. Agric. Biol. 14, 337–344.
Zahir, Z. A., Ghani, U., Naveed, M., Nadeem, S. M., Asghar, H. N. (2009). Comparative effectiveness of Pseudomonas and Serratia sp. containing ACC-deaminase for improving growth and yield of wheat (Triticum aestivum L.) under salt-stressed conditions. Arch. Microbiol. 191, 415–424. doi: 10.1007/s00203–009-0466-y
Zahir, Z. A., Munir, A., Asghar, H. N., Shaharoona, B., Arshad, M. (2008). Effectiveness of rhizobacteria containing ACC deaminase for growth promotion of peas (Pisum sativum) under drought conditions. J. Microbiol. Biotechnol. 18, 958–963.
Zhang, Y., Li, C., Yao, T., Li, M., Lan, X., Wang, Z. (2023). Plant growth–promoting Rhizobacteria enhance salt tolerance in oat by upregulating the antioxidant system and promoting root growth. J. Plant Growth Regul. 42, 3568–3581. doi: 10.1007/s00344–022-10821-z
Zhang, Y., Shen, H., He, X., Thomas, B. W., Lupwayi, N. Z., Hao, X., et al. (2017). Fertilization shapes bacterial community structure by alteration of soil pH. Front. Microbiol. 8. doi: 10.3389/fmicb.2017.01325
Zhou, C., Ma, Z., Zhu, L., Xiao, X., Xie, Y., Zhu, J., et al. (2016). Rhizobacterial strain Bacillus megaterium BOFC15 induces cellular polyamine changes that improve plant growth and drought resistance. Int. J. Mol. Sci. 17, 976. doi: 10.3390/ijms17060976
Zubair, M., Hanif, A., Farzand, A., Sheikh, T. M. M., Khan, A. R., Suleman, M., et al. (2019). Genetic screening and expression analysis of psychrophilic Bacillus spp. reveal their potential to alleviate cold stress and modulate phytohormones in wheat. Microorganisms 7, 337. doi: 10.3390/microorganisms7090337
Keywords: adverse agroclimatic conditions, physiological and omic aspects, plant responses, plant hormones, agricultural sustainability, rhizobacteria
Citation: Verma KK, Joshi A, Song X-P, Liang Q, Xu L, Huang H-r, Wu K-C, Seth CS, Arora J and Li Y-R (2024) Regulatory mechanisms of plant rhizobacteria on plants to the adaptation of adverse agroclimatic variables. Front. Plant Sci. 15:1377793. doi: 10.3389/fpls.2024.1377793
Received: 28 January 2024; Accepted: 07 May 2024;
Published: 23 May 2024.
Edited by:
Dinesh Yadav, Deen Dayal Upadhyay Gorakhpur University, IndiaCopyright © 2024 Verma, Joshi, Song, Liang, Xu, Huang, Wu, Seth, Arora and Li. This is an open-access article distributed under the terms of the Creative Commons Attribution License (CC BY). The use, distribution or reproduction in other forums is permitted, provided the original author(s) and the copyright owner(s) are credited and that the original publication in this journal is cited, in accordance with accepted academic practice. No use, distribution or reproduction is permitted which does not comply with these terms.
*Correspondence: Xiu-Peng Song, eGl1cGVuZ3NvbmdAZ3hhYXMubmV0; Yang-Rui Li, bGl5ckBneGFhcy5uZXQ=
†These authors have contributed equally to this work
‡ORCID: Yang-Rui Li, orcid.org/0000-0002-7559-9244