- 1Yunnan Agricultural University, Kunming, Yunnan, China
- 2Department of Botany & Microbiology College of Science, King Saud University, Riyadh, Saudi Arabia
Background and aims: Nitrogen (N) distribution in plants is intricately linked to key physiological functions, including respiration, photosynthesis, structural development, and nitrogen storage. However, the specific effects of different N morphologies on N accumulation and plant growth are poorly understood. Our research specifically focused on determining how different N morphologies affect N absorption and biomass accumulation.
Methods: This study elucidated the impact of different application rates (CK: 0 g N/plant; T1: 4 g N/plant; T2: 8 g N/plant) of N fertilizer on N and biomass accumulation in tobacco cultivars Hongda and K326 at different growth stages.
Results: Our findings emphasize the critical role of N distribution in various plant parts, including leaves, stems, and roots, in determining the complex mechanisms of N and biomass accumulation in tobacco. We found that in relation to total N, a greater ratio of water-soluble N (Nw) in leaves facilitated N accumulation in leaves. In contrast, an increased ratio of SDS (detergent)-insoluble N (Nin-SDS) in leaves and non-protein N (Nnp) in roots hindered this increase. Additionally, our results indicate that a greater proportion of Nnp in leaves has a negative impact on biomass accumulation in leaves. Furthermore, elevated levels of Nin-SDS, Nw, and Nnp in roots, and Nnp in leaves adversely affected biomass accumulation in tobacco leaves. The Hongda cultivar exhibited greater biomass and N accumulation abilities as compared to K326.
Conclusions: Our findings highlight the significant role of distribution of N morphologies on plant growth, as well as N and biomass accumulation in tobacco plants. Understanding N distribution allows farmers to optimize N application, minimizing environmental losses and maximizing yield for specific cultivars. These insights advance sustainable agriculture by promoting efficient resource use and reducing environmental impact.
1 Introduction
Nitrogen (N) fertilizers contribute significantly to enhancing crop productivity and serve as a cornerstone for addressing global food security by substantially increasing crop yields (Cui et al., 2022). China is the world’s largest fertilizer producer and consumer, and from 1978 to 2006, its fertilizer input contributed 56.18% of the increase in grain yield (Wang et al., 2022b). Previous studies have highlighted the direct correlation between N application, N uptake, and improved plant growth parameters, including tobacco plant height, leaf number, leaf area, and stem diameter by 61.04% (Haghighi et al., 2011; Li et al., 2021). Despite these advances, a comprehensive understanding of the intricate distribution of N across various plant organs remains limited. This knowledge gap calls for a thorough investigation of the effects of diverse N allocation ratios on N and biomass accumulation in tobacco plants, particularly under different N conditions, growth stages, and cultivars.
In plants, water-soluble N (Nw), SDS (detergent)-soluble N (Ns), SDS (detergent)-insoluble N (Nin-SDS), and non-protein N (Nnp) are commonly distributed distinct N morphologies (Liu et al., 2018). Each of these N morphologies plays a specific role within plant leaves; for example, Nw primarily facilitates photosynthesis and storage functions (Qiang et al., 2023), Ns is predominantly linked to photosynthesis including electron transfer and light capture (Viola et al., 2022), Nin-SDS is primarily present in cell wall proteins, which are assumed to contribute to the mechanical toughness of leaves and DNA integrity (Evans and Seemann, 1989; Takashima et al., 2004; Liu et al., 2018, 2023), and Nnp is primarily involved in coordinating leaf expansion and photosynthetic activities (Liu et al., 2018).
Furthermore, the intricate relationship between N allocation and various agronomic measures, particularly N application, has been highlighted as the primary determinant of N distribution in leaves (Tian et al., 2023). High N application has been linked to increased Ns and Nw distributions in soybean and oilseed rape plants, which improves electron transfer, light capture, and overall plant growth (Qiang et al., 2023). It has been reported that increased N application could enhance the light-capturing ability of Panax notoginseng by optimizing the distribution of Ns (Cun et al., 2021). Comprehensive research is crucial for fully understanding the implications of N allocation across various plant organs, particularly at different growth stages and among different cultivars.
The influence of N morphology varied significantly across different growth stages. In earlier growth stages, a greater ratio of Nnp has been observed to promote leaf development, whereas this ratio decreases during later growth stages when leaf expansion ceases (Liu et al., 2018). Qu et al. (2022) highlighted a decline in Ns and Nin-SDS during the flowering to early fruiting stages compared to the seedling stages of cucumbers, indicating a dynamic beneficial impact of these N morphologies during the seedling stages, but adverse effects during the early fruiting stages. Similarly, N allocation also determined the distinct characteristics of cultivars; among different cultivars of pecans, the greater ratio of Ns and Nw in the cultivar YLC35 promoted greater photosynthetic N-use efficiency (Xu et al., 2022), which benefited the growth of plants (Qiang et al., 2023). Conversely, a greater ratio of Nin-SDS in two different N environments adversely affected photosynthesis and respiration abilities in two canola plant cultivars (Liu et al., 2023). Our previous research also indicated that greater proportions of Nw and Ns can enhance N use efficiency, thereby benefiting both biomass and N accumulation (Li et al., 2023).
Understanding N accumulation and growth in tobacco is of utmost importance, considering its significant role in the rural economy of China (Sun et al., 2020). The present study primarily focused on N allocation in leaves, with limited insights into N distribution across different plant organs, morphological variations, and implications for crop growth and development under varying N conditions, growth stages, and cultivars (Hongda and K326). However, long-term unscientific fertilization not only affects yield but also seriously impacts the fragile ecological environment. This study aimed to bridge the existing knowledge gaps by investigating the relationships between N allocation and N and biomass accumulation across different plant organs in flue-cured tobacco cultivars at different growth stages (with a particular emphasis on the Hongda variety, which consistently accumulates more N and biomass under the same N application) under various treatments and at different growth stages. This study aimed to provide valuable insights for optimizing N fertilizer application, producing high-yield flue-cured tobacco leaves and reducing the ecological footprint.
2 Materials and methods
2.1 Experimental site
The study was conducted during two growing seasons from May to September 2021 and 2022 in Yongping County, Dali Prefecture (25.6065°N, 100.2676°E), Yunnan, China. The area receives an average annual precipitation of approximately 919.0 mm and an average temperature of 16.7°C. The soil at the research site was classified as loam and had the following basic physical and chemical properties: 36.58 g kg−1 organic matter, 1.95 g kg−1 total nitrogen, 0.82 g kg−1 total phosphorus, 17.88 g kg−1 total potassium, 233.10 mg kg−1 available nitrogen, 49.34 mg kg−1 available phosphorus, and 236.00 mg kg−1 available potassium.
2.2 Experimental design and conditions
In an open-field pot experiment, we aimed to assess the impact of different N fertilizer application rates on N efficiency in two flue-cured tobacco cultivars (Hongda and K326). Seedlings of the tobacco cultivars Hongda and K326 were transplanted into pots (25 cm × 24 cm), each containing 15 kg of loam soil. To ensure adequate soil moisture, each pot was irrigated with 1,000 mL of water thrice a week. Pots were strategically positioned in the field, maintaining a plant × row spacing of approximately 110 cm × 55 cm, which is consistent with a planting density of 16,500 plants per hectare (Cai et al., 2021). Each treatment consisted of three biological replicates, with 30 plants per cultivar within each replicate. Experiments were performed under three different application rates of N fertilizer (Table 1). Fertilizers were applied as base and top dressings according to standards for tobacco production (Ahmed et al., 2022). At the time of transplantation, the following percentages of fertilizer were added: 80% N, 100% P, and 80% K. Twenty-one days after transplantation, the remaining 20% of the estimated N and K were applied as a top dressing. All management practices were performed according to the guidelines outlined in China’s National Standards for the Tobacco Industry (Li et al., 2022).
2.3 Harvesting and sample analysis
Samples were collected at 25, 50, 75, 100, and 125 days after transplantation of tobacco seedlings. After being harvested, the tobacco plants were divided into five distinct parts: roots, stems, lower leaves, middle leaves, and upper leaves. Three tobacco plants per replicate were uprooted for each treatment at each growth stage. To remove excess soil, the plants were thoroughly rinsed with running water, as described by Hu et al. (2021). The fresh and dry weights of each plant part were recorded before and after curing. To ensure consistency, each plant part was dried at 105°C for 30 min, followed by drying at 75°C for 72 h as part of the curing process. Each dried plant part was sieved through a 2-mm mesh sieve before being crushed and digested with H2SO4-H2O2. According to the method outlined by Liu et al. (2018), the total N content of the digested plant material was determined through continuous flow analysis using an AA3 instrument (Seal Analytical Inc., Southampton, UK).
2.4 Nitrogen morphologies in tobacco
The N morphologies were assessed in frozen samples according to Takashima et al. (2004). Nitrogen was divided into four morphologies: water-soluble (Nw), non-protein nitrogen (Nnp), SDS (detergent)-soluble nitrogen (Ns), and SDS (detergent)-insoluble nitrogen (Nin-SDS). One gram of each plant part, including leaves, roots, and stems, was cryogenically frozen in liquid N and subsequently homogenized with 1 mL of 100 mM sodium phosphate buffer [comprising 2 mM MgCl2, 0.4 M d-sorbitol, 5 mM dithiothreitol (DTT), 5 mM iodoacetate, 10 mM NaCl, and 5 mM phenylmethylsulfonyl fluoride]. The supernatant Nw was separated by centrifuging at 12,000 × g at 4°C for 10 min. The residual samples were dissolved in 1 mL of phosphate buffer (containing 3% SDS) for 5 min at 90°C and then centrifuged at 5,500 × g for 8 min to collect the supernatant as Ns. The Nin-SDS residues were purified by washing with anhydrous ethanol (20 mL) and filtered through medium-speed quantitative filter paper. The supernatant was mixed with an equal volume of 20% trichloroacetic acid, filtered through medium-speed quantitative filter paper, and thoroughly rinsed with anhydrous ethanol (20 mL) to denature the N compounds. Three distinct N morphologies were determined from the residue on the quantitative filter paper after natural air-drying and subsequent digestion using H2SO4-H2O2 following Thomas’s method (Thomas et al., 1967). The N content of the digested solution was determined using continuous flow analysis (AA3; Seal Analytical Inc., Southampton, UK). The Nnp content was calculated by subtracting the values of Nw, Ns, and Nin-SDS from the total N content. Percentage of each N morphologies in each organs were calculated using the following equation:
The calculation of N morphology in stems and roots was similar to that for leaves.
2.5 Statistical analysis
The data were statistically analyzed using SPSS (version 23.0; Armonk, NY, USA) for Z score, curve fitting analysis, and analysis of variance (two-way ANOVA). To evaluate significant differences between treatments, the least significant difference (LSD) test was applied, with significance set at p < 0.05. Graphical representations were created using OriginLab Version 2022 (Northampton, MA, USA) and further refined and compiled using Adobe Illustrator Version 2019 (San Jose, CA, USA).
3 Results
3.1 Nitrogen accumulation in the leaves of tobacco cultivars at different growth stages under different rates of N application
Figure 1 shows that higher levels of N application led to increased N accumulation in tobacco leaves during 125 d of growth after transplantation in 2021 (Figure 1A) and 2022 (Figure 1B). During the initial 50 d following transplantation, CK exhibited 18.80% and 32.38% greater N accumulation in tobacco leaves than treatments T1 and T2, respectively, indicating that lower N application resulted in greater N accumulation during the early growth stages. However, at 75 d, higher levels of N application increased N accumulation in tobacco leaves, highlighting the dynamic response of tobacco plants to varying N levels at different growth stages. Additionally, at 125 d, N accumulation in CK was 63.36% and 45.65% lower than N accumulation in T1 and T2, respectively. The Hongda cultivar, known for its superior N efficiency, displayed 15.25% higher N accumulation in leaves than the K326 cultivar.
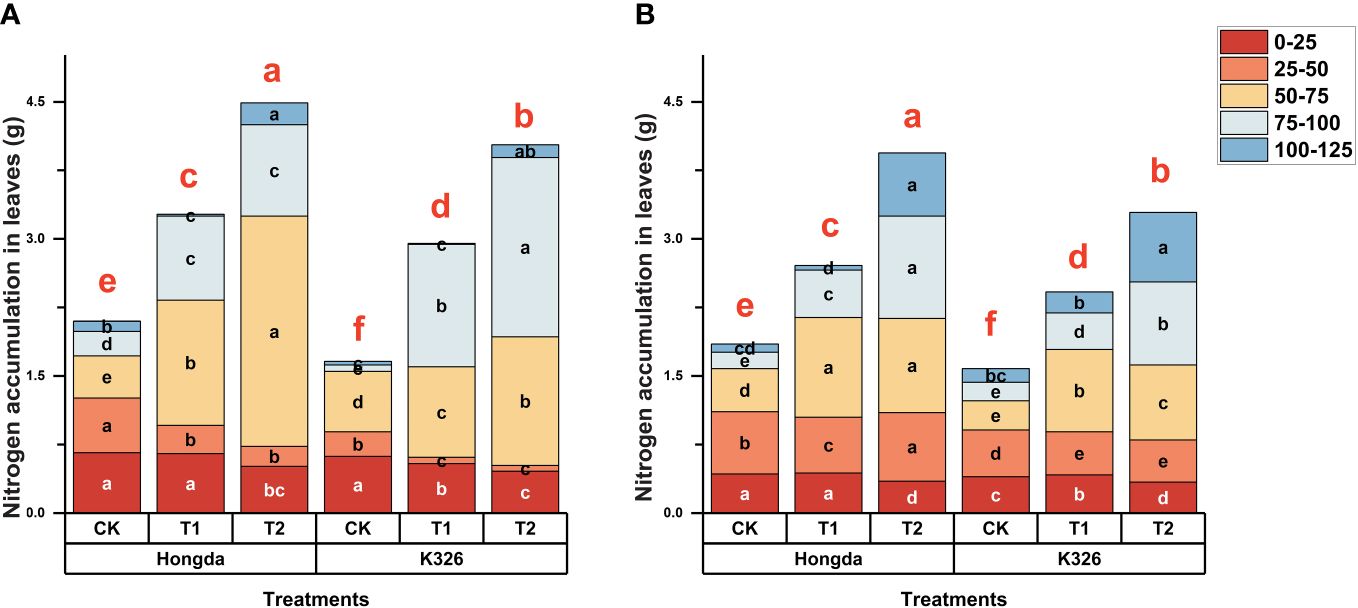
Figure 1 Stacked bar charts illustrating nitrogen accumulation in tobacco plants at different time intervals (0–25 d, 25–50 d, 50–75 d, 75–100 d, and 100–125 d) after transplantation. Here, control (CK): no N fertilizer (0 g/plant), medium (T1): 4 g/plant of pure N, and high (T2): 8 g/plant of pure N. The different colors represent the N accumulation at different growth stages. The total height of the bar demonstrates the difference in N accumulation at 125 d after transplanting. Lowercase letters within the same growth stage indicate significant differences among treatments according to the least significant difference test (LSD, p < 0.05). Specifically, after 125 days of transplantation, the significant differences among treatments are indicated by red lowercase letters above the bar chart (LSD; p < 0.05). (A, B) represent the results for 2021 and 2022, respectively.
3.2 Biomass accumulation in tobacco leaves at different growth stages under different rates of N application
Figure 2 shows a strong correlation between N application and biomass accumulation in tobacco leaves in 2021 (Figure 2A) and 2022 (Figure 2B). This trend is closely correlated with the pattern observed for N accumulation in tobacco leaves. Higher levels of N application corresponded to increased tobacco leaf biomass, particularly at 125 d after transplantation. During the initial stages of tobacco growth (0–50 days after transplanting), CK exhibited 37.89% and 85.93% more biomass accumulation compared to T1 and T2 treatments, respectively. After 75 d of transplantation, higher levels of N application led to increased tobacco leaf biomass production. However after 125 d of transplantation, CK accumulated 56.25% and 44.07% lower biomass than T1 and T2, respectively. Moreover, the Hongda cultivar exhibited 18.23% greater tobacco leaf biomass production than the K326 cultivar under similar treatment conditions in both 2021 and 2022.
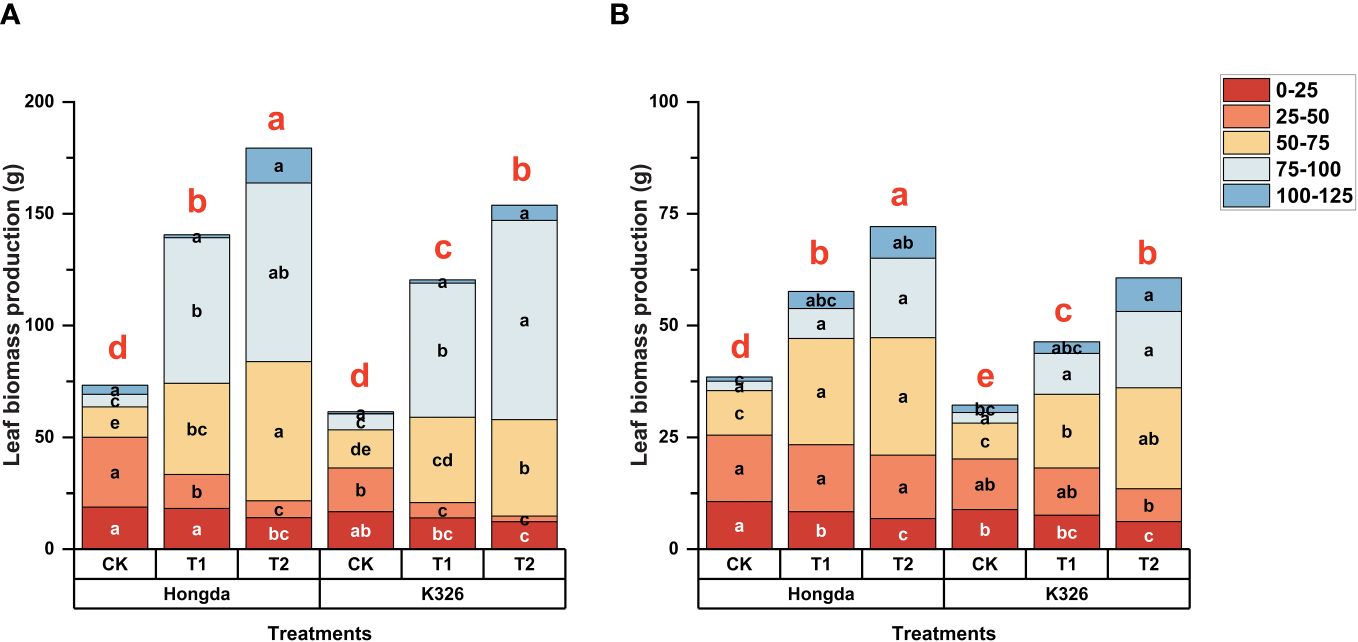
Figure 2 Stacked bar charts illustrating biomass accumulation in tobacco plants at different time intervals (0–25 d, 25–50 d, 50–75 d, 75–100 d, and 100–125 d) after transplantation. Here, control (CK): no N fertilizer (0 g/plant), medium (T1): 4 g/plant of pure N, and high (T2): 8 g/plant of pure N. The different colors represent the N accumulation at different growth stages. The total height of the bar demonstrates the difference in N accumulation at 125 d after transplantation. Lowercase letters within the same growth stage indicate significant differences among treatments according to the least significant difference test (LSD, p < 0.05). Specifically, after 125 days of transplantation, the significant differences among treatments are indicated by red lowercase letters above the bar chart (LSD; p < 0.05). (A, B) represent the results for 2021 and 2022, respectively.
3.3 Variations in allocation ratios of different nitrogen morphologies in tobacco leaves at different growth stages under different N fertilizer treatments
The different application levels of N fertilizer at various growth stages across the two tobacco cultivars differently induced the distribution of various nitrogen morphologies within the tobacco plant parts (Table 2). A notable trend was observed throughout an extensive 2-year study: Nin-SDS levels in tobacco leaves decreased with increased N application. Conversely, there was an overall increase in the levels of Nw, Ns, and Nnp in the tobacco leaves in response to augmented N application. As the growth of the tobacco plants progressed, a noticeable decrease in the ratio of Nin-SDS was observed, whereas the Ns and Nnp ratios consistently increased. The Hongda cultivar exhibited greater ratios of Nw and Ns in leaves as compared to K326, but showed lower levels of Nnp.
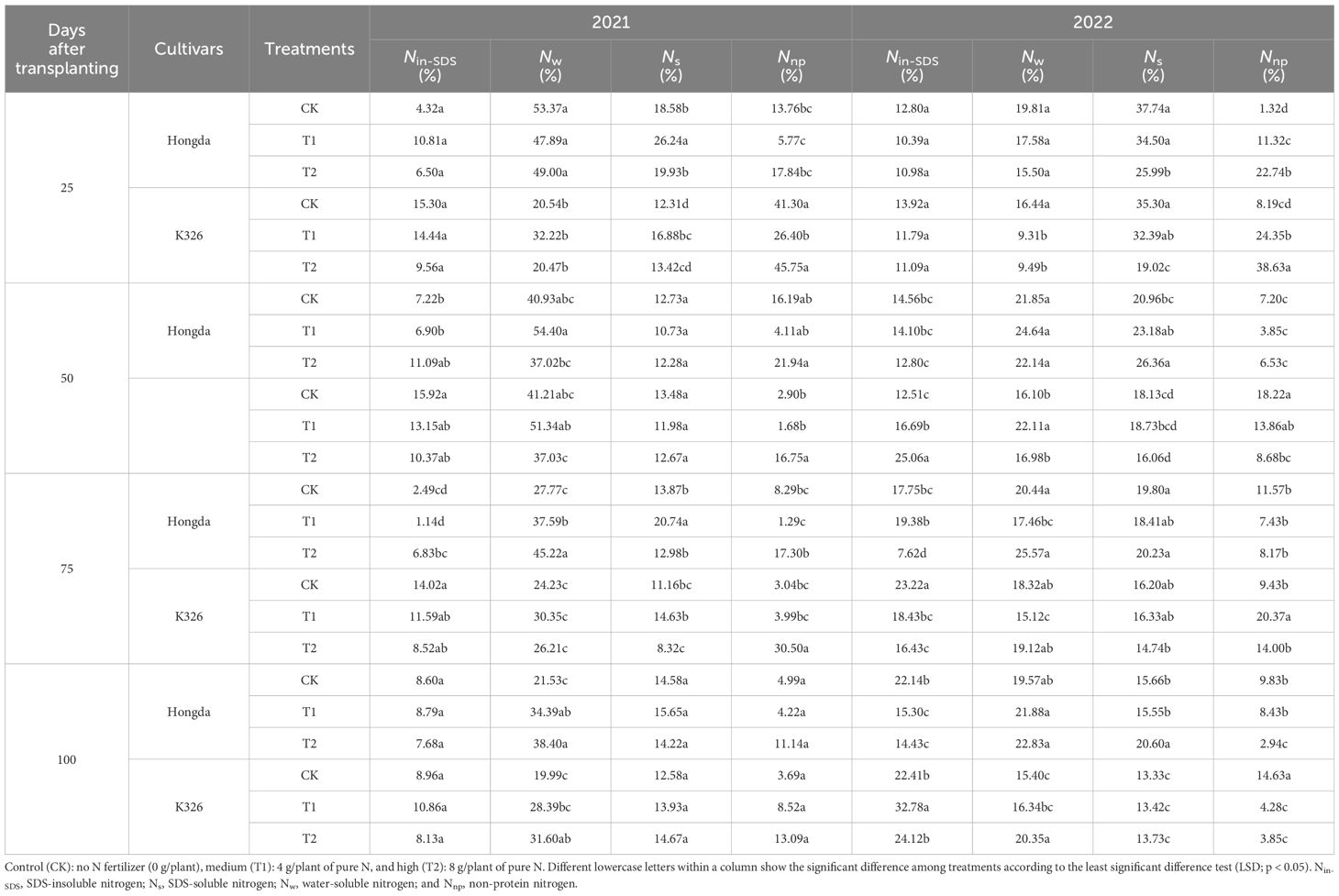
Table 2 Allocation ratios of different nitrogen morphologies in tobacco leaves at different growth stages under various treatments.
3.4 Variations in the ratios of different nitrogen morphologies in tobacco roots at various growth stages under different N fertilizer treatments
Different N application levels, growth stages, and cultivars led to distinct alterations in the distribution of nitrogen morphologies within tobacco roots (Table 3). Over 2 years, a collective decrease in the ratios of Nw, Ns, and Nnp in tobacco roots was observed in response to increasing N application. Simultaneously, the proportion of Nin-SDS decreased as the tobacco plants grew. In comparison to K326, the roots of the Hongda cultivar contained lower proportions of both Nin-SDS and Nnp.
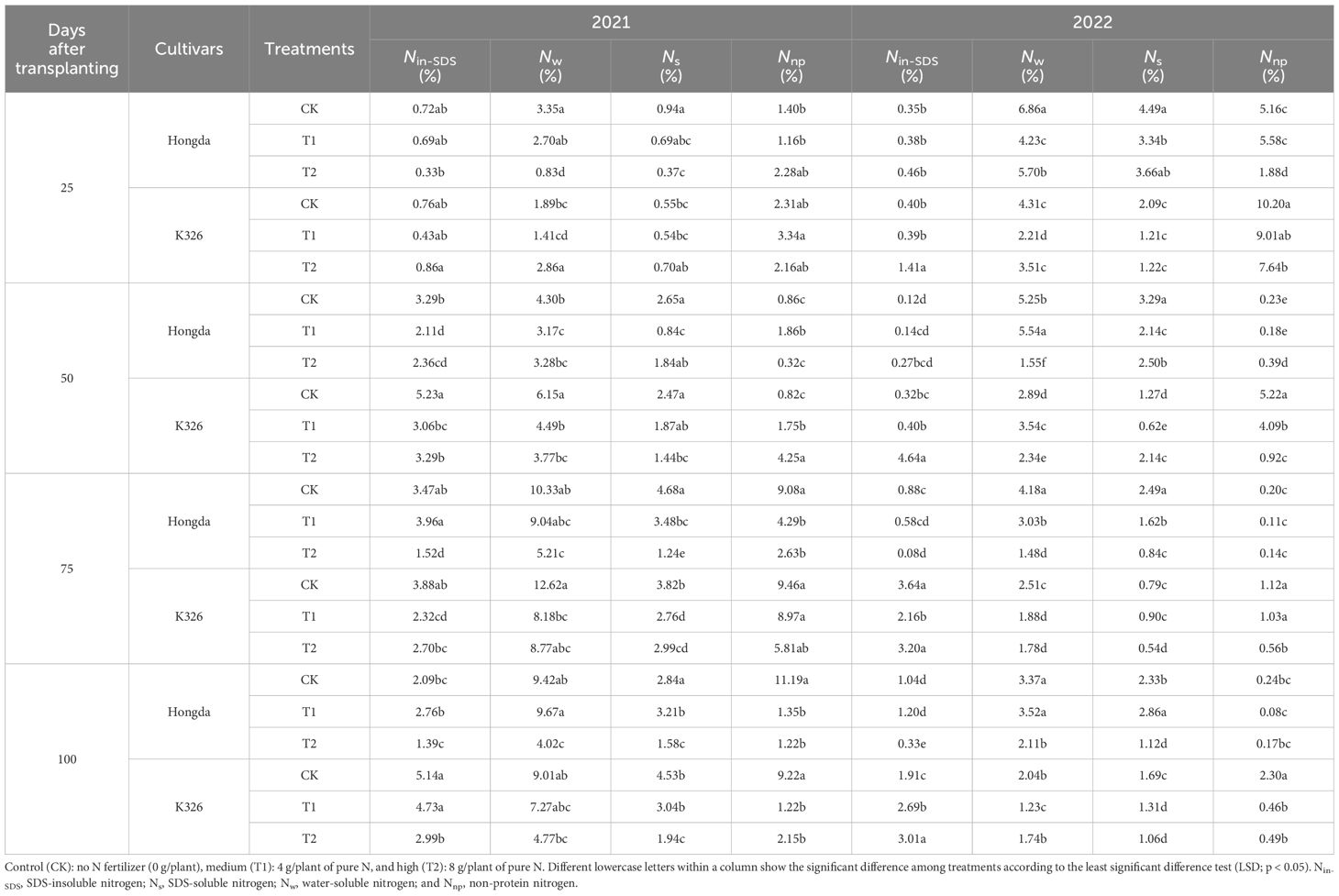
Table 3 Allocation ratios of different nitrogen morphologies in tobacco roots at different growth stages under various treatments.
3.5 Variations in the ratios of different nitrogen morphologies in the stem at various growth stages of tobacco plants under different treatments
The influence of N application, growth stage, and cultivar on the distribution of nitrogen morphologies within tobacco stems was apparent (Table 4). The analysis revealed a pronounced decline in the proportions of Nnp, Nw, and Ns with increasing N application levels. Similarly, the ratio of Nnp in the stems consistently decreased as the tobacco plants developed. Significant disparities between Hongda and K326 were noted, with Hongda demonstrating greater ratios of Nw and Ns in stems but lower proportions of Nnp than K326.
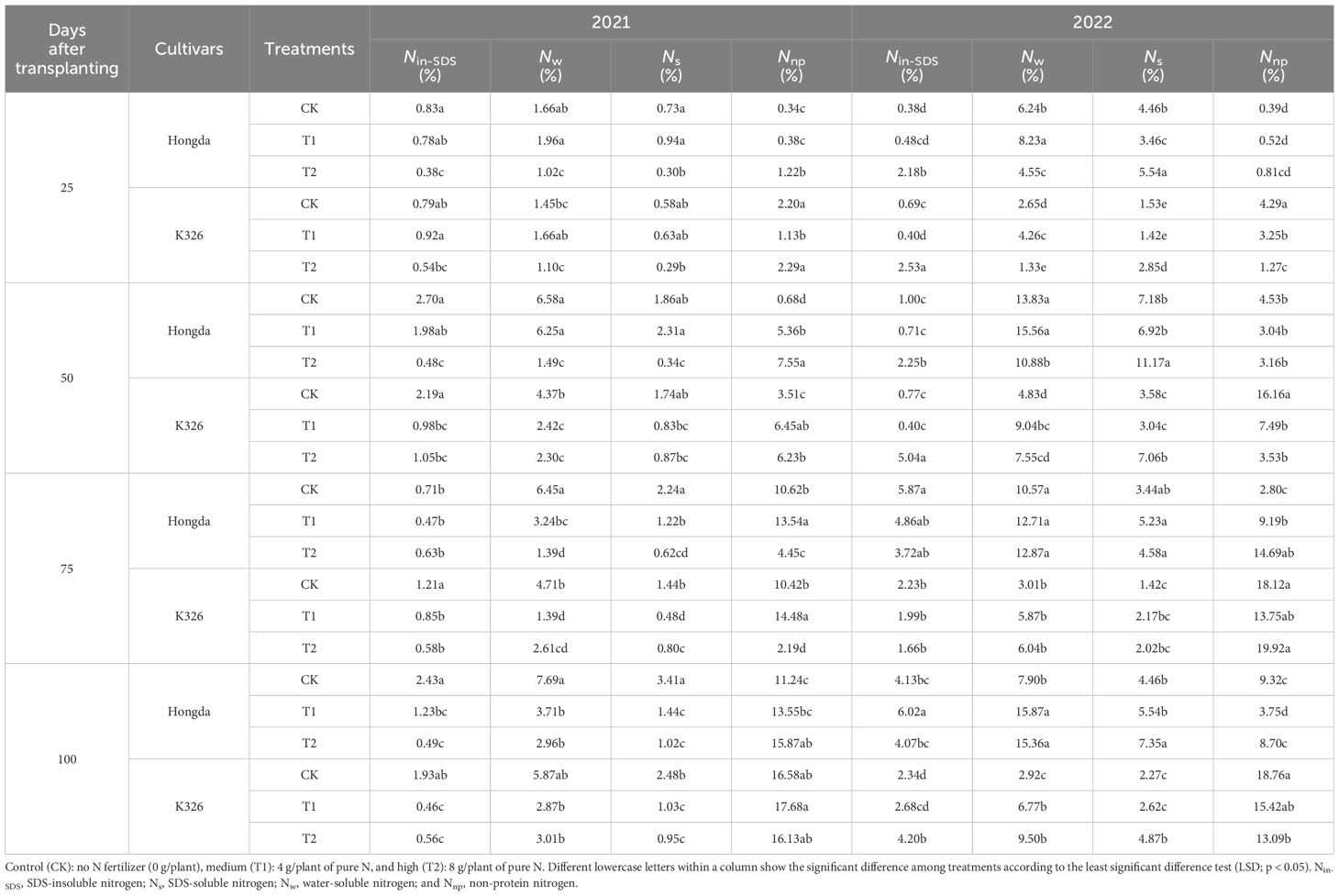
Table 4 Allocation ratios of different nitrogen morphologies in stems at different growth stages and under various treatments of tobacco plants.
3.6 Nitrogen distribution influences nitrogen accumulation in tobacco plants
To explore how various N morphologies affect N and biomass accumulation in flue-cured tobacco, we conducted a standardized data analysis using the Z score method (Supplementary Tables 1–4). A subsequent investigation into the influence of different N morphologies on N accumulation in flue-cured tobacco leaves (Figure 3; Table 5) indicated that increased proportions of Nw in leaves were associated with an increase in overall N accumulation. Moreover, greater proportions of Nin-SDS in leaves and Nnp in roots are linked to a reduction in overall N accumulation. A quadratic function accurately described the relationship between Nw and N accumulation in leaves, shedding light on the intricate dynamics. Notably, cubic curves accurately represented the relationship between Nnp and N accumulation in roots, revealing a complex and nonlinear association between these factors. Similarly, the correlation between Nin-SDS in leaves and N accumulation was effectively characterized by an exponential function, highlighting the intricate and nonlinear interplay between these variables. These findings underscore the multifaceted interactions between different N morphologies and biomass accumulation in flue-cured tobacco leaves, providing valuable insights into the underlying mechanisms that influence crop growth and development.
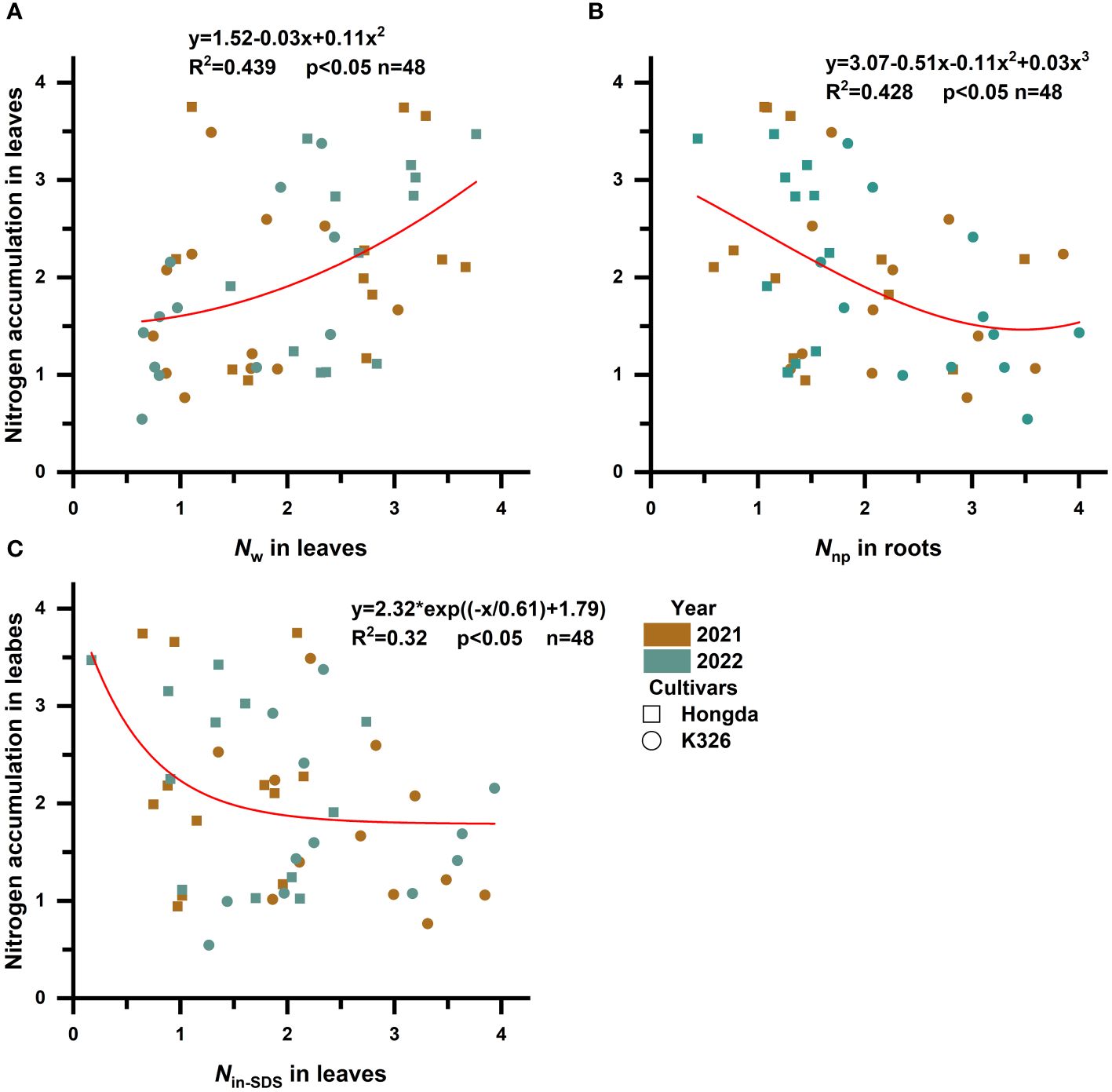
Figure 3 Scatterplot with fit curve illustrates the effects of different distributions of nitrogen morphologies on leaf nitrogen accumulation (Z score). Nin-SDS, SDS-insoluble nitrogen; Ns, SDS-soluble nitrogen; Nw, water-soluble nitrogen; and Nnp, non-protein nitrogen. (A–C) represent the relationship between Nw in leaves, Nnp in roots, and Nin-SDS in leaves in relation to the nitrogen accumulation of the leaves, respectively.

Table 5 Curve fitting analysis illustrates the effects of the distributions of different nitrogen morphologies on nitrogen accumulation in leaves ((Z score+2).).
3.7 Effect of N distribution on biomass accumulation in tobacco plants
It became apparent that the presence of Nin-SDS, Nw, and Nnp in roots and Nnp in leaves hindered the accumulation of biomass in tobacco leaves (Figure 4; Table 6). Notably, the inhibitory effects of Nin-SDS and Nnp on roots and Nnp on leaves did not follow a linear pattern despite their increasing proportions. Specifically, the relationship between Nin-SDS in roots and biomass accumulation in leaves can be described as a cubic function, highlighting the intricate interplay between these variables. Similarly, the relationship between Nw in roots and N accumulation in leaves followed a linear function. Additionally, the association between Nnp in roots and biomass accumulation in leaves can be described as a quadratic function, suggesting a complex interplay between these variables. Finally, the relationship between Nnp and biomass accumulation in leaves can be described as a quadratic function, indicating a complex and nonlinear association between these factors. These functional relationships provide valuable insights into the dynamics of biomass distribution and accumulation in flue-cured tobacco leaves.
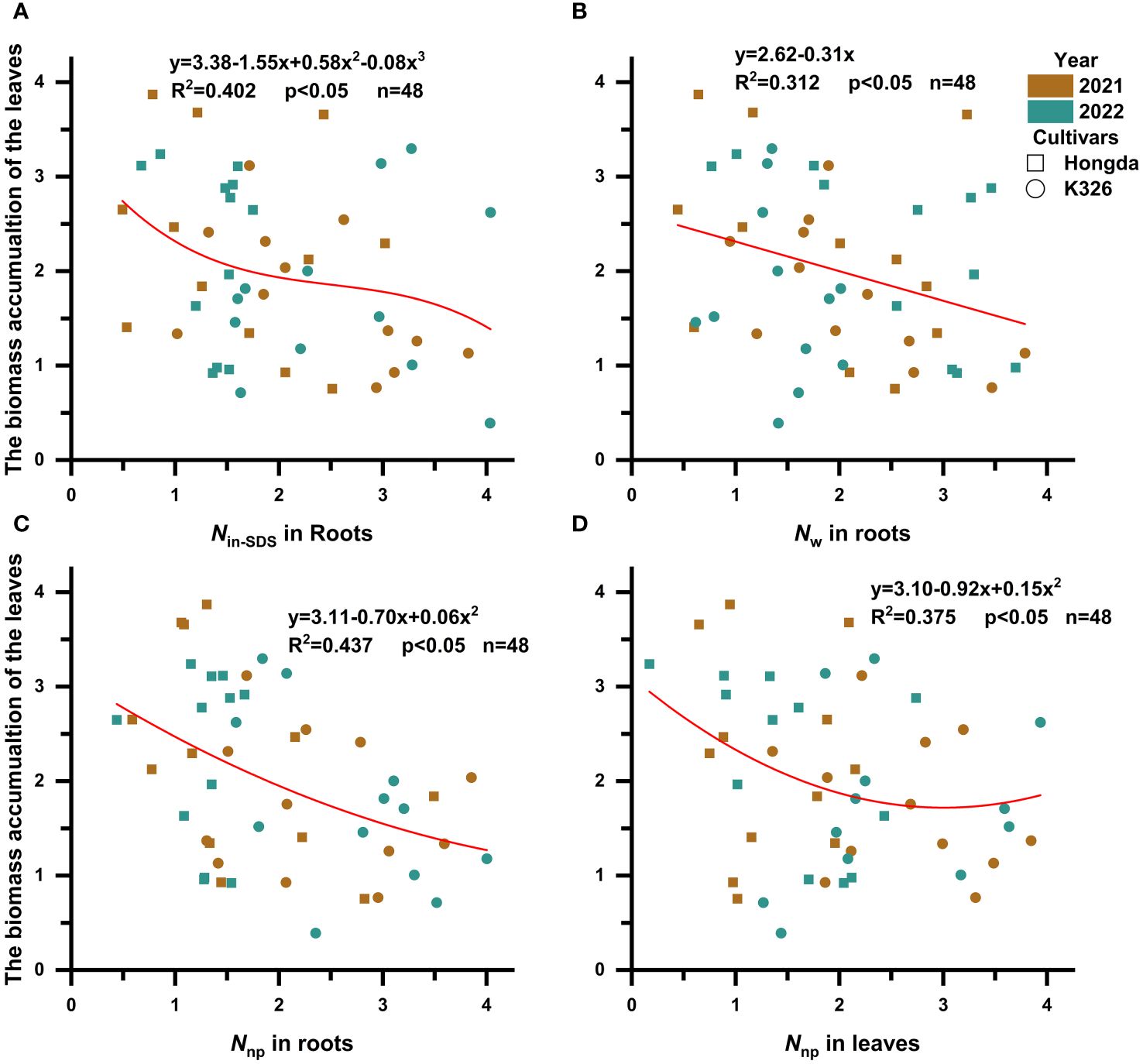
Figure 4 Scatterplot with fit curve illustrates the effects of the distributions of different nitrogen morphologies (Z score) on leaf biomass accumulation (Z score). Nin-SDS, SDS-insoluble nitrogen; Nw, water-soluble nitrogen; Nnp, non-protein nitrogen. (A–D) represent the relationship between Nin-SDS in roots, Nw in roots, Nnp in roots, and Nnp in leaves in relation to the biomass accumulation of the leaves, respectively.
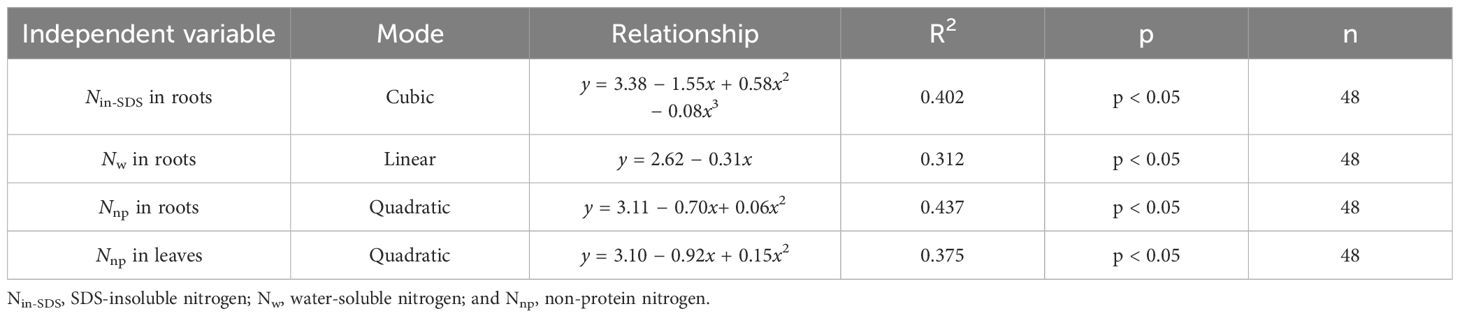
Table 6 Curve fitting analysis illustrates the effects of the distributions of different nitrogen morphologies (Z score+2) on leaf biomass production (Z score+2).
4 Discussion
Nitrogen (N) is a critical factor for the growth and development of plants and influences various metabolic functions and cellular processes that ultimately determine crop yield (Song et al., 2022). Despite the fact that extensive studies demonstrated the role of N in plants and its impact on plant growth (Moose and Below, 2009), the specific distribution of N morphologies across different plant organs and their influence on N and biomass accumulation under varying N application levels, growth stages, and cultivars remain poorly understood. Thus, the present study aimed to investigate the effects of various N fertilizer applications on two distinct cultivars, highlighting their varied N and biomass accumulation capabilities at different growth stages over 2 years (2021 and 2022).
Liu et al. (2018) and Ghafoor et al. (2021) emphasized the profound impact of N fertilizer application on N and biomass accumulation in tobacco leaves. Our study revealed that treatments T1 and T2 significantly increased N and biomass accumulation in both cultivars compared to those in plants without N fertilizer treatment (CK). Specifically, compared with the CK, treatments T1 and T2 resulted in notable increases in N accumulation of 58.03% and 118.97%, respectively. Similarly, T1 and T2 significantly enhanced biomass accumulation by 77.59% and 126.86%, respectively, indicating the positive influence of N application on tobacco growth. The findings suggested that the biomass and N accumulation followed the “S model” (Yang et al., 2012). The N and biomass accumulation rate initially increased and then decreased. Under CK, compared with those under T1 and T2, an increasing trend were observed at 50 d after transplanting, possibly due to the inhibition resulting from a high-N environment (Xun et al., 2020; Wang et al., 2022a). However, after 75 d, increased N application led to greater N and biomass accumulation rates, ultimately resulting in greater N and biomass accumulation at 125 d after transplanting. Previous studies have emphasized significant differences in NUE among flue-cured tobacco cultivars, which are attributable to variations in their ability to absorb N and accumulate biomass under similar environmental conditions. In earlier studies, Hongda displayed a greater NUE than K326 (Parker, 2008; Liang et al., 2013; Fan et al., 2018). Compared to the K326 cultivar, the Hongda cultivar consistently exhibited enhanced N and biomass accumulation, with increases of 16.18% and 19.83%, respectively, under various treatment conditions. The superior efficiency of Hongda is consistent with earlier research findings. This study monitored the increase in N and biomass in the whole plant and its leaves every 25 d compared to the corresponding periods under CK. Hongda accumulated more biomass and N in the whole plant and leaves 0–50 days after transplanting. Moreover, Hongda plants accumulated more biomass and N under the various N fertilizer treatments at 125 d after transplanting. These results indicate that Hongda possesses greater biomass and N accumulation abilities than K326, which is consistent with previous studies (Parker, 2008; Liang et al., 2013).
In our investigation, Nw (17.5%–32.7% of the total N in the plant) was the predominant morphology in leaves (Supplementary Figure 1A), which is consistent with earlier findings (Niinemets and Tenhunen, 1997; Takashima et al., 2004; Liu et al., 2018). Nw was found to be related to plant respiration and carboxylation. Similarly, Ns is associated with electron transport and light capture systems, accounting for 12.9%–19.6% of the total N in the whole plant, a ratio less than that of Nw, which is also in line with earlier studies (Niinemets and Tenhunen, 1997; Takashima et al., 2004; Liu et al., 2018). The proportions of Nin-SDS and Nnp were lower than those of Ns and Nw, and these morphologies are primarily associated with plant structural and storage functions (Liu et al., 2018). We found that stems exhibited lower N allocation compared to leaves (Supplementary Figure 1B). This distinction can be attributed to the relatively weaker respiration and photosynthetic activity observed in stems than in leaves, as previously suggested (Ávila-Lovera et al., 2017; Tokarz et al., 2021; Salomón et al., 2022). Consequently, noticeable reductions in Nw and Ns levels within the stems were observed. Remarkably, stems contain more Nnp than other N morphologies, and Nnp was also recognized for its significant role in plant development (Liu et al., 2018). Moreover, Fritschi et al. (2013) explained that 73%–80% of the N in the roots of soybeans was mobile, which was closely associated with yield. In our study, the mobile N highlighted by Fritschi et al. (2013) may correspond to the Nnp form of N.
Compared to the N distribution in leaves, a greater proportion of N was allocated to Nw and Nnp in roots (Supplementary Figure 1C). Nw in roots is mostly associated with root respiration, a critical physiological trait that contributes to root resource acquisition strategies. Similarly, root respiration, a fundamental metabolic process responsible for growth, ion mobilization and uptake, and cell maintenance through ATP production, carbon skeleton production, and redox balancing, is closely related to N absorption and biomass accumulation (Han and Zhu, 2021). Similar to Nnp in stems, Nnp in roots also plays a role in remobilizing N, contributing to plant growth (Fritschi et al., 2013; Liu et al., 2018). Among the different treatments, the ratios of Nw, Ns, and Nnp in the tobacco roots decreased with increasing N application, suggesting a decrease in root respiration and storage functions, resulting in a failure to provide sufficient energy for N uptake and biomass accumulation (Han and Zhu, 2021). Nnp in stems also decreased with increasing N application, indicating insufficient N sources available for plant growth (Ávila-Lovera et al., 2017). In leaves, Nin-SDS decreased with increasing N application, whereas Nw and Ns increased with increasing N application, indicating that N application can promote respiration and photosynthesis and facilitate N storage for leaf expansion (Liu et al., 2018).
The distribution of N may account for the observed variation in N uptake and biomass accumulation at different growth stages. During the growth of tobacco plants, the proportion of Nin-SDS in leaves decreased, whereas that of Ns and Nnp increased. Initially, a greater allocation of Nin-SDS to the leaves may facilitate the development of larger leaves, as suggested by Liu et al. (2018). As the plants matured, the increased Ns encouraged robust photosynthesis, whereas elevated Nnp supported leaf expansion, consistent with prior research indicating rapid early-stage leaf growth, followed by deceleration in later stages (Hernández-Montes et al., 2019; He and Qin, 2020). Concurrently, the proportions of Nin-SDS and Nnp in the stems decreased as the tobacco plants continued to develop. Initially, a greater Nin-SDS allocation bolstered the growth of sturdy stems, which is beneficial for overall plant development (Guo et al., 2018). Subsequently, the reduced distribution of Nnp in stems implies a diminished capacity for stem growth, with the utilized N contributing less to storage and promoting plant growth through remobilized N, which is consistent with the findings of Darenova et al. (2020) and Liu et al. (2018). As the tobacco plants developed, the proportion of Nin-SDS in roots decreased. Higher Nin-SDS allocation helped tobacco establish stronger roots in the early stages. Over time, the reduced Nin-SDS distribution in roots indicated a weakened ability of roots to grow and gradually stop, with N being used for respiration, thereby promoting N uptake (Jiang et al., 2023). Plants obtained more vital nutrient absorption abilities in the later stages than in the earlier stages (Khan et al., 2020).
Nitrogen distribution may explain the differences in N uptake and biomass accumulation among different cultivars (Li et al., 2023). The ratio of Nw and Ns in the stems and leaves of the whole plant of Hongda was greater than that in the stems and leaves of the whole plant of K326, suggesting that Hongda had greater respiration and more N sources for stem and leaf growth, contributing to different abilities of N uptake and use (Pan et al., 2016; An et al., 2019). By curve fitting analysis, we determined that Nin-SDS, Nw, and Nnp in roots and Nnp in leaves were the major factors contributing to biomass accumulation in leaves. Nw in leaves, Nnp in roots, and Nin-SDS in leaves were the main factors influencing N accumulation, ultimately determining the differences in N uptake and biomass accumulation abilities among different N applications, stages, and cultivars. Nw in leaves, Nnp in roots, and Nin-SDS in leaves were the primary factors influencing N accumulation. With increasing N fertilizer application, Nw in leaves increases, which enhances carboxylation and respiration and directly provides more energy for N absorption (Khan et al., 2022). The proportion of Nin-SDS in leaves decreased with tobacco growth, promoting N uptake and accelerating N accumulation 75 days after transplanting. Hongda plants had a greater percentage of Nw in their leaves than K326 plants, which improved their photosynthetic ability and promoted N uptake (Balestrini et al., 2020; Su et al., 2020). The distribution of Nw in leaves primarily affected N accumulation at different N fertilizer application levels. In contrast, Nin-SDS in leaves mainly influenced N accumulation in tobacco leaves at different growth stages. Moreover, Nw in leaves primarily affects the N accumulation in different tobacco cultivars. Overall, these N morphologies collectively coordinate N accumulation in tobacco leaves.
Increases in Nin-SDS, Nw, and Nnp in roots and Nnp in leaves were found to adversely affect biomass accumulation. Although increased N fertilizer application has exhibited adverse effects on the ratio of Nw and Nnp in roots, inhibiting root respiration and development (Liu et al., 2018), under an abundant N environment, decreased respiration in roots results in decreased organic carbon compound consumption, which can promote biomass accumulation in tobacco leaves (Weitz et al., 2021). In contrast, Nnp in leaves also increases with increasing N fertilizer application, which decreases the ratio of respiration and photosynthesis abilities and adversely affects leaf development (Niinemets and Tenhunen, 1997; Takashima et al., 2004; Liu et al., 2018). Among the different stages, Nnp in leaves increased with tobacco growth during the various stages, in contrast to the results of Liu et al. (2018), which might be attributed to the focus on plant growth under low-N environments. In contrast, our study examined N allocation under different N conditions. The ratio of Nnp in leaves increased at different stages, adversely affecting leaf growth; however, the ratio of Nin-SDS in roots decreased with tobacco growth, promoting leaf biomass accumulation, indicating that Nnp in leaves is more closely related to leaf biomass accumulation (Liu et al., 2018). Between Hongda and K326, Nin-SDS and Nnp in roots and Nnp in leaves were lower in Hongda than in K326, thereby enhancing leaf growth under similar treatment conditions (An et al., 2019). The allocation of Nw in roots and Nnp in leaves primarily influenced the differences in biomass accumulation among the N application levels. Nnp in leaves primarily affects the disparities in biomass accumulation at different growth stages. Additionally, Nin-SDS and Nnp in roots and Nnp in leaves contributed to determining the differences in biomass accumulation among the varieties.
5 Conclusions
Our study demonstrated significant increases in nitrogen and biomass accumulation in treatments T1 and T2 as compared to control (CK). Across various experimental conditions and growth stages, the Hongda cultivar consistently exhibited greater nitrogen and biomass accumulation than the K326 cultivar. The allocation ratios of nitrogen morphologies profoundly influence vital physiological processes such as photosynthesis and respiration, improve nitrogen absorption, and promote optimal plant growth. In response to diverse nitrogen conditions, tobacco plants adapt to these allocation ratios of nitrogen morphologies to efficiently absorb and utilize nitrogen. Hongda showed superior nitrogen use efficiency to K326, which was credited to its scientific honing strategy of allocating nitrogen. Moreover, these allocation ratios play a pivotal role in aiding nitrogen uptake and utilization and facilitating overall plant development across various growth stages. Future studies should focus on investigating the molecular mechanisms underlying nitrogen partitioning to enhance nitrogen use efficiency and plant productivity.
Data availability statement
The original contributions presented in the study are included in the article/Supplementary Material. Further inquiries can be directed to the corresponding authors.
Author contributions
SL: Conceptualization, Data curation, Investigation, Methodology, Software, Validation, Writing – original draft, Writing – review & editing. WA: Conceptualization, Data curation, Methodology, Software, Writing – original draft, Writing – review & editing. TJ: Data curation, Methodology, Software, Writing – original draft. YY: Writing – original draft, Data curation, Formal analysis. LY: Data curation, Formal analysis, Methodology, Writing – original draft. TZ: Data curation, Investigation, Software, Writing – original draft. FM: Formal analysis, Software, Validation, Writing – original draft. SAA: Writing – original, Writing - review & editing. QS: Conceptualization, Data curation, Methodology, Writing – original draft. CG: Conceptualization, Data curation, Software, Writing – original draft. ZZ: Conceptualization, Funding acquisition, Project administration, Resources, Supervision, Writing – original draft, Writing – review & editing.
Funding
The author(s) declare financial support was received for the research, authorship, and/or publication of this article. This study was financially supported by the National Key Research Plan of China (2022YFD1901504).
Acknowledgments
The authors extend their appreciation to the Researchers Supporting Project Number RSP2025R5, King Saud University, Riyadh, Saudi Arabia.
Conflict of interest
The authors declare that the research was conducted in the absence of any commercial or financial relationships that could be construed as a potential conflict of interest.
Publisher’s note
All claims expressed in this article are solely those of the authors and do not necessarily represent those of their affiliated organizations, or those of the publisher, the editors and the reviewers. Any product that may be evaluated in this article, or claim that may be made by its manufacturer, is not guaranteed or endorsed by the publisher.
Supplementary material
The Supplementary Material for this article can be found online at: https://www.frontiersin.org/articles/10.3389/fpls.2024.1377364/full#supplementary-material
Abbreviations
N, Nitrogen; Nw, Water-soluble nitrogen; Ns, SDS (detergent)-soluble nitrogen; Nin-SDS, SDS (detergent)-insoluble nitrogen; Nnp, Non-protein nitrogen.
References
Ahmed, W., Dai, Z., Zhang, J., Li, S., Ahmed, A., Munir, S., et al. (2022). Plant-microbe interaction: mining the impact of native Bacillus amyloliquefaciens WS-10 on tobacco bacterial wilt disease and rhizosphere microbial communities. Microbiol. Spectr. 10, e01471–e01422. doi: 10.1128/spectrum.01471-22
An, F., Chen, T., Li, Q. X., Qiao, J., Zhang, Z., Carvalho, L. J., et al. (2019). Protein cross-interactions for efficient photosynthesis in the cassava cultivar SC205 relative to its wild species. J. Agric. Food Chem. 67, 8746–8755. doi: 10.1021/acs.jafc.9b00046
Ávila-Lovera, E., Zerpa, A. J., Santiago, L. S. (2017). Stem photosynthesis and hydraulics are coordinated in desert plant species. New Phytol. 216, 1119–1129. doi: 10.1111/nph.14737
Balestrini, R., Brunetti, C., Chitarra, W., Nerva, L. (2020). Photosynthetic traits and nitrogen uptake in crops: which is the role of arbuscular mycorrhizal fungi? Plants. 9, 1105. doi: 10.3390/plants9091105
Cai, Q., Zhou, G., Ahmed, W., Cao, Y., Zhao, M., Li, Z., et al. (2021). Study on the relationship between bacterial wilt and rhizospheric microbial diversity of flue-cured tobacco cultivars. Eur. J. Plant Pathol. 160, 265–276. doi: 10.1007/s10658-021-02237-4
Cui, L., Li, D., Wu, Z., Xue, Y., Xiao, F., Gong, P., et al. (2022). Effects of combined nitrification inhibitors on soil nitrification, maize yield and nitrogen use efficiency in three agricultural soils. PloS One 17, e0272935. doi: 10.1371/journal.pone.0272935
Cun, Z., Zhang, J.-Y., Wu, H.-M., Zhang, L., Chen, J.-W. (2021). High nitrogen inhibits photosynthetic performance in a shade-tolerant and N-sensitive species Panax notoginseng. Photosynth. Res. 147, 283–300. doi: 10.1007/s11120-021-00823-5
Darenova, E., Horáček, P., Krejza, J., Pokorný, R., Pavelka, M. (2020). Seasonally varying relationship between stem respiration, increment and carbon allocation of Norway spruce trees. Tree Physiol. 40, 943–955. doi: 10.1093/treephys/tpaa039
Evans, J. R., Seemann, J. R. (1989). The allocation of protein nitrogen in the photosynthetic apparatus: costs, consequences, and control. Photosynthesis. 8, 183–205.
Fan, T. F., He, M. J., Li, C. J., Shi, D. X., Yang, C., Chen, Y. Y., et al. (2018). Physiological dissection revealed that both uptake and assimilation are the major components regulating different growth responses of two tobacco cultivars to nitrogen nutrition. Plant Biol. (Stuttg) 20, 39–49. doi: 10.1111/plb.12642
Fritschi, F. B., Ray, J. D., Purcell, L. C., King, C. A., Smith, J. R., Charlson, D. V. (2013). Diversity and implications of soybean stem nitrogen concentration. J Plant Nutr. 36, 2111–2131. doi: 10.1080/01904167.2012.748800
Ghafoor, I., Habib-Ur-Rahman, M., Ali, M., Afzal, M., Ahmed, W., Gaiser, T., et al. (2021). Slow-release nitrogen fertilizers enhance growth, yield, NUE in wheat crop and reduce nitrogen losses under an arid environment. Environ. Sci. pollut. Res. Int. 28, 43528–43543. doi: 10.1007/s11356-021-13700-4
Guo, Z., Liu, G., Röder, M. S., Reif, J. C., Ganal, M. W., Schnurbusch, T. (2018). Genome-wide association analyses of plant growth traits during the stem elongation phase in wheat. Plant Biotechnol. J. 16, 2042–2052. doi: 10.1111/pbi.12937
Haghighi, H., Daliri, M. S., Mobaser, H. R., Moosavi, A. A. (2011). Effect of different nitrogen and potassium fertilizer levels on quality and quantity yield of flue-cured tobacco (Coker 347). World Applied Sci J. 15, 941–946.
Han, M., Zhu, B. (2021). Linking root respiration to chemistry and morphology across species. Glob Chang Biol. 27, 190–201. doi: 10.1111/gcb.15391
He, J., Qin, L. (2020). Growth and photosynthetic characteristics of sweet potato (Ipomoea batatas) leaves grown under natural sunlight with supplemental LED lighting in a tropical greenhouse. J. Plant Physiol. 252, 153239. doi: 10.1016/j.jplph.2020.153239
Hernández-Montes, E., Tomás, M., Escalona, J. M., Bota, J., Medrano, H. (2019). Leaf growth rate and nitrogen content determine respiratory costs during leaf expansion in grapevines. Physiol. Plant 165, 746–754. doi: 10.1111/ppl.12769
Hu, W., Wei, J., Di, Q., Tao, T., Zhang, J., Liu, J., et al. (2021). Flue-cured tobacco (Nicotiana tabacum L.) leaf quality can be improved by grafting with potassium-efficient rootstock. Field Crops Res. 274, 108305. doi: 10.1016/j.fcr.2021.108305
Jiang, Q., Lin, C., Guo, R., Xiong, D., Yao, X., Wang, X., et al. (2023). Root nitrogen uptake capacity of Chinese fir enhanced by warming and nitrogen addition. Tree Physiol. 43, 31–46. doi: 10.1093/treephys/tpac103
Khan, S., Amaral Júnior, A. T. D., Ferreira, F. R. A., Kamphorst, S. H., Gonçalves, G. M. B., Simone Mendonça Freitas, M., et al. (2020). Limited nitrogen and plant growth stages discriminate well nitrogen use, uptake and utilization efficiency in popcorn. Plants 9 (7), 893. doi: 10.3390/plants9070893
Khan, N. M., Imran, M., Ashraf, M., Arshad, H., Awan, A. R. (2022). Oxytetracycline and ciprofloxacin antibiotics exhibit contrasting effects on soil microflora, nitrogen uptake, growth, and yield of wheat (Triticum aestivum L.). J Soil Sci Plant Nutr. 22, 3788–3797. doi: 10.1007/s42729-022-00927-4
Li, C., Ahmed, W., Li, D., Yu, L., Xu, L., Xu, T., et al. (2022). Biochar suppresses bacterial wilt disease of flue-cured tobacco by improving soil health and functional diversity of rhizosphere microorganisms. Appl. Soil Ecol. 171, 104314. doi: 10.1016/j.apsoil.2021.104314
Li, S., Ahmed, W., Zhang, T., Jiang, T., Mei, F., Shan, Q., et al. (2023). Different morphologies and functional nitrogen accumulation results in the different nitrogen use efficiency of tobacco plants. J Plant Growth Regulation. 42 (9), 5895–5908. doi: 10.1007/s00344-023-10975-4
Li, Y., Chang, D., Zhang, X., Shi, H., Yang, H. (2021). RNA-Seq, physiological, and biochemical analysis of burley tobacco response to nitrogen deficiency. Sci. Rep. 11, 16802. doi: 10.1038/s41598-021-93363-w
Liang, T., Wang, J., Zhang, Y., Xi, J., Zhou, H., Wang, B., et al. (2013). “Spectral characteristics of tobacco cultivars with different nitrogen efficiency and its relationship with nitrogen use,” In Computer and Computing Technologies in Agriculture VII: 7th IFIP WG 5.14 International Conference, CCTA 2013. Revised Selected Papers, Part II 7. (Beijing, China: Springer Berlin Heidelberg). pp. 239–246.
Liu, T., Ren, T., White, P. J., Cong, R., Lu, J. (2018). Storage nitrogen co-ordinates leaf expansion and photosynthetic capacity in winter oilseed rape. J. Exp. Bot. 69, 2995–3007. doi: 10.1093/jxb/ery134
Liu, J., Zhang, K., Bi, J., Yu, X., Luo, L., Hu, L. (2023). Mesophyll conductance and N allocation co-explained the variation in photosynthesis in two canola genotypes under contrasting nitrogen supply. Front. Plant Sci. 14, 1171331. doi: 10.3389/fpls.2023.1171331
Moose, S., Below, F. E. (2009). Biotechnology approaches to improving maize nitrogen use efficiency. Mol. Genet. approaches to maize improve. 63, 65–77. doi: 10.1007/978-3-540-68922-5_6
Niinemets, Ü., Tenhunen, J. (1997). A model separating leaf structural and physiological effects on carbon gain along light gradients for the shade-tolerant species Acer saccharum. Plant, Cell Environment. 20, 845–866. doi: 10.1046/j.1365-3040.1997.d01-133.x
Pan, S., Liu, H., Mo, Z., Patterson, B., Duan, M., Tian, H., et al. (2016). Effects of nitrogen and shading on root morphologies, nutrient accumulation, and photosynthetic parameters in different rice genotypes. Sci. Rep. 6, 32148. doi: 10.1038/srep32148
Parker, R. G. (2008). Evaluation of nitrogen sources and rates on yield and quality of modern flue-cured tobacco cultivars (North Carolina State University).
Qiang, B., Zhou, W., Zhong, X., Fu, C., Cao, L., Zhang, Y., et al. (2023). Effect of nitrogen application levels on photosynthetic nitrogen distribution and use efficiency in soybean seedling leaves. J. Plant Physiol. 287, 154051. doi: 10.1016/j.jplph.2023.154051
Qu, F., Peng, T., Jia, Y., Yang, M., Meng, X., Mao, S., et al. (2022). Adjusting leaf nitrogen allocation could promote photosynthetic capacity, and nitrogen accumulation in cucumis sativus L. Environmental Experimental Botany. 198, 104855. doi: 10.1016/j.envexpbot.2022.104855
Salomón, R. L., De Roo, L., Oleksyn, J., Steppe, K. (2022). Mechanistic drivers of stem respiration: A modelling exercise across species and seasons. Plant Cell Environ. 45, 1270–1285. doi: 10.1111/pce.14246
Song, R., Ahmed, W., Tan, Y., Zhao, Z. (2022). Different levels of nitrogen fertilizer in nursery stage positively affect the activity of defense-related enzymes and resistance of tobacco plant to phytophthora nicotianae. Chiang Mai J. Sci. 49, 551–564. doi: 10.12982/CMJS
Su, W., Ahmad, S., Ahmad, I., Han, Q. J. P. (2020). Nitrogen fertilization affects maize grain yield through regulating nitrogen uptake, radiation and water use efficiency, photosynthesis and root distribution. PeerJ. 8. doi: 10.7717/peerj.10291
Sun, H., Sun, X., Wang, H., Ma, X. (2020). Advances in salt tolerance molecular mechanism in tobacco plants. Hereditas 157, 5. doi: 10.1186/s41065-020-00118-0
Takashima, T., Hikosaka, K., Hirose, T. (2004). Photosynthesis or persistence: nitrogen allocation in leaves of evergreen and deciduous Quercus species. Plant Cell Environ. 27, 1047–1054. doi: 10.1111/j.1365-3040.2004.01209.x
Thomas, R., Sheard, R., Moyer, J. (1967). Comparison of conventional and automated procedures for nitrogen, phosphorus, and potassium analysis of plant material using a single digestion 1. Agron. J. 59, 240–243. doi: 10.2134/agronj1967.00021962005900030010x
Tian, G., Qin, H., Liu, C., Xing, Y., Feng, Z., Xu, X., et al. (2023). Magnesium improved fruit quality by regulating photosynthetic nitrogen use efficiency, carbon–nitrogen metabolism, and anthocyanin biosynthesis in ‘Red Fuji’apple. Front. Plant Sci. 14, 1136179. doi: 10.3389/fpls.2023.1136179
Tokarz, K. M., Wesołowski, W., Tokarz, B., Makowski, W., Wysocka, A., Jędrzejczyk, R. J., et al. (2021). Stem photosynthesis-A key element of grass pea (Lathyrus sativus L.) acclimatisation to salinity. Int. J. Mol. Sci. 22 (2), 685. doi: 10.3390/ijms22020685
Viola, S., Roseby, W., Santabarbara, S., Nürnberg, D., Assunção, R., Dau, H., et al. (2022). Impact of energy limitations on function and resilience in long-wavelength Photosystem II. Elife 11, e79890. doi: 10.7554/eLife.79890.sa2
Wang, J., Qin, L., Cheng, J., Shang, C., Li, B., Dang, Y., et al. (2022b). Suitable chemical fertilizer reduction mitigates the water footprint of maize production: evidence from Northeast China. Environ. Sci. pollut. Res. Int. 29, 22589–22601. doi: 10.1007/s11356-021-17336-2
Wang, F., Wang, Q., Yu, Q., Ye, J., Gao, J., Liu, H., et al. (2022a). Is the NH(4) (+)-induced growth inhibition caused by the NH(4) (+) form of the nitrogen source or by soil acidification? Front. Plant Sci. 13, 968707. doi: 10.3389/fpls.2022.968707
Weitz, K. K., Smith, M. L., Hixson, K. K., Hill, E. A., Jansson, J. K., Hofmockel, K. S., et al. (2021). Real-time mass spectrometry measurements of respiration rates in biological systems. J. Am. Soc. Mass Spectrom 32, 648–652. doi: 10.1021/jasms.0c00251
Xu, Q., He, H., He, B., Li, T., Liu, Y., Zhu, S., et al. (2022). Nitrogen Allocation Tradeoffs Within-Leaf between Photosynthesis and High-Temperature Adaptation among Different Varieties of Pecan (Carya illinoinensis [Wangenh.] K. Koch). Plants 11 (21), 2828. doi: 10.3390/plants11212828
Xun, Z., Guo, X., Li, Y., Wen, X., Wang, C., Wang, Y. (2020). Quantitative proteomics analysis of tomato growth inhibition by ammonium nitrogen. Plant Physiol. Biochem. 154, 129–141. doi: 10.1016/j.plaphy.2020.05.036
Keywords: Nicotiana tabacum, nitrogen use efficiency, water-soluble nitrogen, non-protein nitrogen, SDS-insoluble nitrogen
Citation: Li S, Jiang T, Ahmed W, Yang Y, Yang L, Zhang T, Mei F, Alharbi SA, Shan Q, Guo C and Zhao Z (2024) Deciphering the impact of nitrogen morphologies distribution on nitrogen and biomass accumulation in tobacco plants. Front. Plant Sci. 15:1377364. doi: 10.3389/fpls.2024.1377364
Received: 27 January 2024; Accepted: 10 June 2024;
Published: 01 July 2024.
Edited by:
Khurram Shahzad, Lasbela University of Agriculture, Water and Marine Sciences, PakistanReviewed by:
Tajamul Hussain, Oregon State University, United StatesShahid Hussain, Bahauddin Zakariya University, Pakistan
Muhammad Atif Muneer, Fujian Agriculture and Forestry University, China
Copyright © 2024 Li, Jiang, Ahmed, Yang, Yang, Zhang, Mei, Alharbi, Shan, Guo and Zhao. This is an open-access article distributed under the terms of the Creative Commons Attribution License (CC BY). The use, distribution or reproduction in other forums is permitted, provided the original author(s) and the copyright owner(s) are credited and that the original publication in this journal is cited, in accordance with accepted academic practice. No use, distribution or reproduction is permitted which does not comply with these terms.
*Correspondence: Waqar Ahmed, YWhtZWQud2FxYXIxMDgzQHlhaG9vLmNvbQ==; Zhengxiong Zhao, emhhb3p4MDgwMUAxNjMuY29t
†These authors have contributed equally to this work and share first authorship